Circular RNA circ0007360 Attenuates Gastric Cancer Progression by Altering the miR-762/IRF7 Axis
- 1Department of Gastroenterology, The First Affiliated Hospital of Nanchang University, Nanchang, China
- 2Department of Gynecology and Obstetrics, The First Affiliated Hospital of Nanchang University, Nanchang, China
Gastric cancer is a major health burden worldwide. Circular RNAs (circRNAs) are a novel family of noncoding RNAs that are involved in multiple types of cancers, including gastric cancer. As biological functions and the underlying molecular mechanisms of the newly identified circRNA circ0007360 have not been investigated, our present study focused on the role of circ0007360 in the progression of gastric cancer. After characterizing circ0007360 as a cytoplasmic circRNA, we revealed the inhibitory effects of circ0007260 on the survival, migration, invasion, and stemness of gastric cancer cells. Subsequently, miR-762 was identified as a direct target microRNA (miRNA) of circ0007360 and was proved to act as a vital downstream transcript to fulfill the tumor-promoting effects in the absence of circ0007360. Furthermore, we demonstrated that interferon regulatory factor 7 (IRF7), which was validated as a target gene of miR-762, serves as an indirect target of circ0007360 to attenuate the progression of gastric cancer. Moreover, in vivo experiments confirmed the potentiation of gastric cancer cell growth and stemness upon depletion of circ0007360. In summary, our results revealed that activation of the circ0007360/miR-762/IRF7 axis is a novel mechanism for the attenuation of gastric cancer progression. Our study unveils the diagnostic and therapeutic values of circ0007360 in patients with gastric cancer.
Introduction
Gastric cancer, which has adenocarcinoma as a major subtype (accounting for 90% of all cases), is caused by multiple clinical factors. On the one hand, Helicobacter pylori infection is usually considered as an initial stimulus for the tumorigenesis of gastric cancer (Correa, 2013). On the other hand, mutations in inflammatory response genes have been shown to strongly correlate with the initiation of gastric cancer by facilitating the colonization of bacteria (Persson et al., 2011). For example, polymorphisms in genes, such as inflammatory IL1B, are associated with the risk of gastric cancer (Camargo et al., 2006). Because of late diagnosis, the 5-year survival rate of patients with gastric cancer is less than 20% (Anderson et al., 2010). Therefore, developing effective biomarkers for diagnosis and prognosis is of great worth for patients with gastric cancer.
Cancer stem-like cells (CSCs) not only contribute to resistance to chemotherapeutic strategies but also act as a pivotal cell population for the metastasis and recurrence of cancer, including gastric cancer (Takaishi et al., 2009; Garcia-Mayea et al., 2020). Thus, targeting gastric CSCs, which are characterized as a CD44+ population, holds dramatic promise for treating patients with gastric cancer (Bekaii-Saab and El-Rayes, 2017).
Circular RNAs (circRNAs) are a class of noncoding RNAs that form an exonuclease-resistant loop by direct back splicing (Eger et al., 2018). Although some circRNAs are nuclear RNAs, most of them have been demonstrated to localize in the cytoplasm and to serve as sponges for microRNAs (miRNAs) by matching miRNA binding sites (Salzman, 2016; Vo et al., 2019). As a subgroup of small RNAs, miRNAs are well-characterized as posttranscriptional regulators of target messenger RNAs (mRNAs) by binding to their 3′ untranslated regions (3′UTRs) (Matsuyama and Suzuki, 2020). Both circRNAs and miRNAs play vital roles in cancer progression (Goodall and Wickramasinghe, 2021). For instance, circRNA circNRIP1 is capable of negatively regulating the levels of miR-149-5p in gastric cancer cells and thereby potentiating AKT1/mTOR pathway to trigger cancer progression (Zhang et al., 2019).
Since circ0007360 (we also named this circRNA as circular RNA number 4779; circ4779) is an ill-investigated circRNA, hereby we focused on studying the biological effects of circ0007360 on the survival, migration, invasion, and stemness of gastric cancer cells and the underlying molecular mechanism. First, a couple of classical assays were performed to validate the circular characteristic of circ0007360 and its cytoplasmic localization. Subsequently, miR-762 was identified as a downstream miRNA target of circ0007360. Importantly, the absence of miR-762 mitigated the enhancement of cell survival, migration, invasion, and stemness, which was induced by the depletion of circ0007360. Furthermore, we identified interferon regulatory factor 7 (IRF7) as a target gene of miR-762 and performed a series of rescue experiments to validate that IRF7 is an indirect target of circ0007360 to exert its tumor-suppressive role. Importantly, in vivo experiments further consolidated the inhibitory effects of circ0007360 on gastric cancer cell growth and stemness. Our investigation may aid in the development of circ0007360 as a promising prognostic marker and as a therapeutic candidate for the treatment of gastric cancer.
Material and Methods
Cell Culture and Treatment
Human gastric cancer cell lines AGS and MKN-7 were purchased from IMMOCELL (Xiamen, Fujian, China). Both cell lines were maintained in Eagle’s minimum essential medium supplemented with 10% fetal bovine serum, 100 U/ml penicillin, and 100 U/ml streptomycin. All the reagents were purchased from Gibco. Actinomycin D (1 μM; Sigma) was used to check the stability of RNA transcripts by treating cells for the indicated time points.
RNA Fluorescent In Situ Hybridization (FISH)
To check the localization and expression of circ0007360, we used the RNA FISH method. The whole procedure was performed according to the instructions from the Fluorescent in situ Hybridization Kit (Rabobio; C10910). The probes were also purchased from Rabobio as follows: FISH Probe Mix (Red; Rabobio; C10920), h-U6 FISH Probe Mix (Red) (Rabobio; LNC110101), and h-18S FISH probe mix (red; Rabobio; LNC110201).
gDNA Extraction
Total gDNA was isolated from AGS cells using the DNeasy Blood and Tissue Kit (Qiagen; 69504). Divergent and convergent primers were used for polymerase chain reaction (PCR) amplification of circ007360 and GAPDH, respectively. The PCR products from cDNA or gDNA were separated using agarose gel electrophoresis. All primers used for the PCR are listed in Supplementary Table S1.
Cloning
The circ0007360 ectopic expression construct was generated using PCR amplification and ligated to the pCDNA3.1 backbone. Circ0007360 and IRF7 shRNA were generated by inserting the annealed shRNA oligos into the pLKO.1-TRC backbone. The reporter constructs for wild type circ0007360, mutant circ0007360, wild type IRF7 3′UTR, or mutant IRF7 3′UTR were amplified and inserted into the pmirGLO (Promega) backbone. All primers used for cloning are listed in Supplementary Table S1.
Real-Time Quantitative PCR (RT-qPCR)
Total RNA was isolated using the Total RNA Extraction Kit (Vazyme Biotech, Nanjing; China). Subsequently, 1 μg of RNA was reverse transcribed using the HiScript II First Strand cDNA Synthesis Kit (Vazyme Biotech). Next, the cDNA was subjected to quantitative PCR using the ChamQ SYBR Master Mix kit (Vazyme Biotech) and iQ5 qPCR machine (Bio-Rad) to detect the relative expression levels of target genes. The relative levels of various target genes were quantified using the 2−ΔΔCt formula, with 18S RNA as the reference transcript for normalization. All primers used in this study are listed in Supplementary Table S1. RNase R treatment was performed as follows: 1 μg RNA was diluted in 40 μl water supplemented with 4 U RNase R (Thermo Fisher), after which the mixture was incubated for 15 min at 37°C.
Subcellular Fractionation
To detect the localization of circ0007360, the PARIS™ Kit (Thermo Fisher; AM 1921) was used to extract total RNA from the cytoplasm or nucleus. RT-qPCR was performed as described above.
Transfection
Cells were plated in wells of six-well plates and transfected with 4 μg plasmids or 200 pmol miRNA mimics or inhibitors using Lipofectamine 3000 (Invitrogen) according to the manufacturer’s instructions.
Luciferase Reporter Assay
AGS cells were co-transfected with the indicated luciferase reporter plasmids and the miR-762 mimic or mimic NC. At 48 h post-transfection, the cells were lysed and luciferase activity was measured using the Dual-Glo Luciferase Assay System (Promega). Firefly luciferase activity was normalized to Renilla luciferase activity.
MTT Assay
Briefly, 24 h after transfection, the cells were plated at 1 × 103 cells/well in 96-well plates. MTT solution (20 μl, 5 mg/ml; Qiancheng Biotech, China) dissolved in PBS was directly added to each well at the indicated time points. After incubation for 4 h at 37°C, the medium was replaced with 100 μl DMSO, and the absorbance at 490 nm was measured using a SpectraMax Absorbance Reader (Molecular Devices). The results are presented as the mean ± SD (n = 6).
Colony Formation Assay
At 24 h post-transfection, 500 cells were plated in the wells of a six-well plate. After culturing for 2 weeks at 37°C, the cells were fixed with 4% paraformaldehyde for 10 min. Staining was performed using 0.5% crystal violet for 10 min. The stained cell colonies were photographed and counted after three washes with water.
Flow Cytometry Assay
To quantify the frequencies of cells in different cell cycle phases, at 24 h post-transfection, the cells were fixed with 70% ethanol overnight at 4°C. Subsequently, the cells were washed twice with PBS before permeabilization in PBS buffer supplemented with Triton X-100 (0.2%) and RNase (10 μg/ml) for 30 min. Subsequently, the cells were stained with propidium iodide (PI; 20 μg/ml; A211-02; Vazyme Biotech) solution in the dark for 30 min at room temperature (RT), after which 1 × 104 cells were quantified using a NovoCyte flow cytometer (ACEA Biosciences, San Diego, CA, United States).
Similarly, the cell apoptosis assay was initiated by treating the transfected cells with Annexin V-fluorescein isothiocyanate (200 μg/ml) and PI (30 μg/ml) in the dark for 10 min at RT. After several washes with PBS buffer, the NovoCyte flow cytometer was used to determine the frequency of apoptotic cells.
To check the expression of CD44 and EpCAM on the cell surface, cells were stained with isotope-FITC, anti-CD44-FITC, or anti-EpCAM-FITC (all from BioLegend) and the signal was detected using a NovoCyte flow cytometer. In all the assays mentioned above, unstained cells served as negative controls to establish background fluorescence thresholds.
Transwell Assay
For migration assays, at 24 h post-transfection, 5 × 105 serum-starved cells were seeded into the upper chambers of 24-well Transwell plates (Corning, NY, United States). For the evaluation of cell invasion, a diluted Matrigel (BD) pre-coated membrane of the top chamber was used. For both assays, 10% serum medium was added to the bottom wells. After 24 h of incubation, 4% PFA was applied to fix the migrated or invasive cells on the bottom part of the chamber, and the cells were stained with 0.5% toluidine blue before imaging. Five independent fields were selected for statistical analysis.
Tumor Sphere Formation Assay
Upon 24 h transfection, cells were trypsinized and 1 × 103 cells were seeded into wells of 24-well plates containing DMEM/F12 medium (IMMOCELL, China) supplemented with B27 (Gibco), 20 ng/ml EGF (Gibco), 20 ng/ml bFGF (Nowoprotein, China), and 4 μg/ml insulin (Gibco). Fresh medium was added every 3 days and the cells were cultured for 12–18 days before being photographed.
Western Blotting
RIPA buffer (Beyotime) supplemented with 1 × complete protease inhibitor cocktail (Roche) was used to lyse the cells. Protein concentrations were determined using a bicinchoninic acid protein assay kit (Thermo Fisher). Equal amounts of proteins were separated using SDS-PAGE and subsequently transferred onto a 45-μm polyvinylidene difluoride (PVDF) membrane (Bio-Rad). Next, the membranes were blocked with 5% non-fat dry milk in Tris-buffered saline with 0.1% Tween 20 (TBST) for 1 h at RT. The resulting membranes were probed with primary antibodies overnight at 4°C. After three washes with TBST, the membranes were incubated with horseradish peroxidase (HRP)-conjugated secondary antibodies for 2 h at RT. The signal was detected using Clarity™ Western ECL Substrate (Thermo Fisher) and the ChemiDoc Imaging System (Bio-Rad). All antibodies used in this study are listed in Supplementary Table S2.
Animal Experiments
All mouse experiments were conducted in accordance with a protocol approved by the Animal Care and Use Committee of Nanchang University. For tumor growth assays, 5 × 106 AGS cells with or without circ0007360 depletion were subcutaneously injected into the lower back regions of 6-week-old female BALB/c nude mice for 34 days (n = 6). Tumor volumes were monitored every 4 days with calipers from 18 days post-inoculation. Tumor volumes were calculated using the following formula: length × width2/2. The mice were euthanized 34 days after inoculation, after which the tumors were collected and photographed. Moreover, the tumor weight was determined 34 days post-inoculation.
Immunohistochemistry Analysis
First, the tumors from the mice were fixed and embedded. After sectioning, the paraffin was removed by placing the slides in xylene three times, followed by placing them in 100% ethanol twice. Endogenous peroxidase activity was blocked with 0.3% hydrogen peroxide for 20 min, after which the slides were rehydrated in 96, 70, and 50% ethanol, respectively. After antigen retrieval and washing, the slides were slowly cooled to RT, followed by three times washing with PBST. Primary antibody against Ki67 (BD; 550609) diluted (1:100) in 1% BSA solution was used to incubate the slides overnight at 4°C. The slides were washed with PBST three times before incubating with 1:200 diluted biotinylated secondary antibody (DAKO; E0353) for 30 min at RT. After three times washing with PBST, the slides were incubated with Vectastain complex (Vector Laboratories; PK-6100) for 30 min. Subsequently, the slides were washed with PBST three times and developed using the DAB reagent. Next, slides were counterstained with Mayer hematoxylin (Sigma-Aldrich; MHS80) for 45 s and dehydrated. Finally, Entellan mounting medium (Merck; 107961) was used to mount the slides. Images were captured, and the rate of positive cells was analyzed using the AIpathwell software (Servicebio, China).
Statistical Analysis
Statistical analyses were performed using the GraphPad Prism 8 software. All results are shown as the mean ± SD. Unpaired Student’s t-test was used for analysis, and p < 0.05 was considered to be significant (*0.01 < p < 0.05, **0.001 < p < 0.01, ***0.0001 < p < 0.001, ****p < 0.0001). NS, not significant.
Results
Circ0007360 Is a Circular RNA
We started our investigation by checking whether circ0007360 is a real circular RNA. To this end, a convergent primer pair was used to amplify the junction site of circ0007360. Sanger sequencing showed that the junction site could be detected, as predicted (Figure 1A). However, as expected, the PCR products generated by the divergent primer pair could not be detected when genomic DNA (gDNA) was used as a template (Figure 1B). Of note, the convergent primer pair for circ0007360 or GAPDH, which served as a positive control, amplified the expected fragment in both cDNA and gDNA (Figure 1B). Since resistance to exonuclease RNase R is also a gold standard for testing whether an RNA transcript is circular (Jeck and Sharpless, 2014), we treated the cDNA from two gastric cancer cell lines, AGS and MKN-7, with RNase R. RT-qPCR results showed that the levels of ATF6 mRNA, which is the host mRNA of circ0007360, but not circ0007360, were reduced upon RNase R challenge (Figure 1C). Furthermore, a time-course experiment directed by actinomycin D, an inhibitor of transcription, revealed that the stability of circ0007360 was significantly higher than ATF6 mRNA in both gastric cancer cell lines (Figure 1D). Collectively, these results indicate that circ0007360 is a real circular RNA.
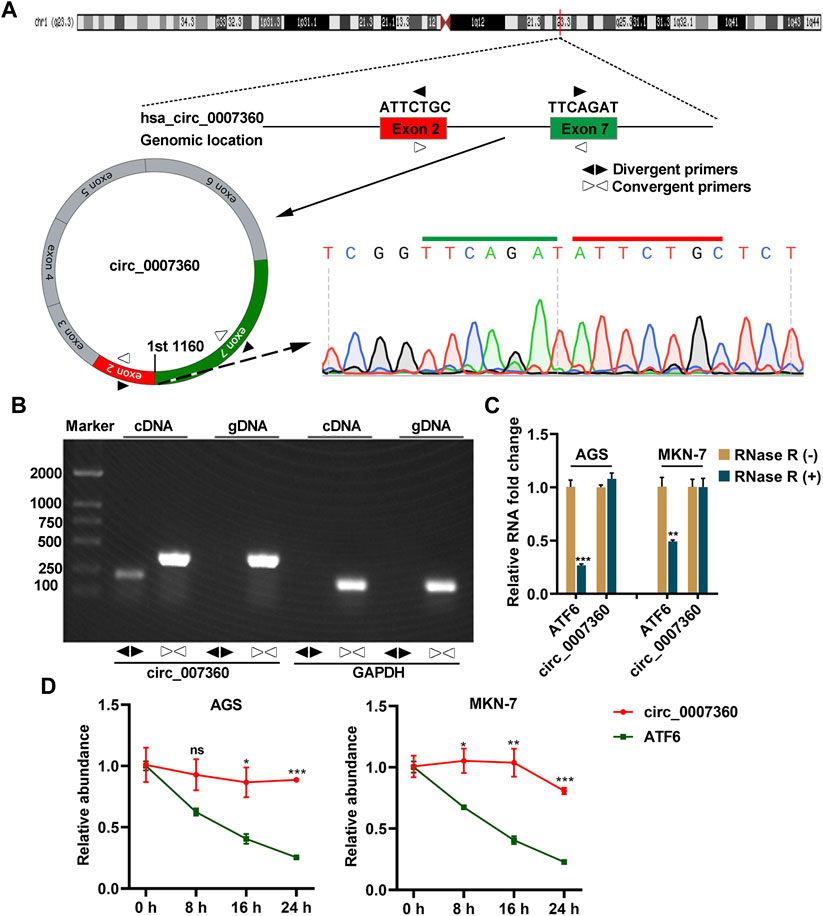
FIGURE 1. Circ0007360 is a circular RNA. (A) A schematic model showing the genomic locus of circ0007360, the location of divergent and convergent primer pairs used for detecting circ0007360 expression, as well as the sanger sequencing result of the junction site. (B) The DNA gel result for detecting the PCR products amplified by the indicated primers for circ0007360 or GAPDH. (C) RT-qPCR quantification of the RNA levels of ATF6 or circ007360 with or without RNase R treatment. (D) RT-qPCR quantification of the RNA levels of ATF6 or circ007360 with or without actinomycin D treatment for the indicated time points.
Circ0007360 Is Mainly Localized in the Cytoplasm
As the localization of circRNAs determines their functions (Hsiao et al., 2017), we next checked the localization of circ0007360 using an RNA FISH assay. Circ0007360 was more abundant in the cytoplasm, where the 18S RNA was localized, than that in the nucleus, where another positive control U6 RNA was localized (Figure 2A). Moreover, subcellular fractionation analysis confirmed the abundant cytoplasmic location of circ0007360 (Figure 2B). Since the most common mechanism of cytosolic circRNAs is acting as sponges for matching miRNAs, we performed in silico prediction for target miRNAs of circ0007360. After analyzing the intersection result from three databases (Targetscan (Agarwal et al., 2015), miRDB (Chen and Wang, 2020) and circMine (Zhang et al., 2021)), six candidate miRNAs were enriched (miR-762, miR-4427, miR-4656, miR-3135b, miR-4680-3p and miR-8077; Figure 2C). Given the fact that miR-762 is a well-annotated miRNA whose promoting role in cancer progression has been reported by many research groups (Li et al., 2015; Ge et al., 2019; Chen et al., 2020), we focused on miR-762 for further experimental validation. Two putative binding sites of miR-762 on circ0007360 was found using the CircMir 1.0 platform (Xu et al., 2021) (Figures 2D,E). To prove the interaction between these two molecules, reporter assays were performed. We found that miR-762 attenuated the luciferase activity of both predicted interacting fragments from circ0007360 (WT), whereas the corresponding mutant circ0007360 (MUT) fragments were not affected by miR-762 overexpression (Figure 2F).
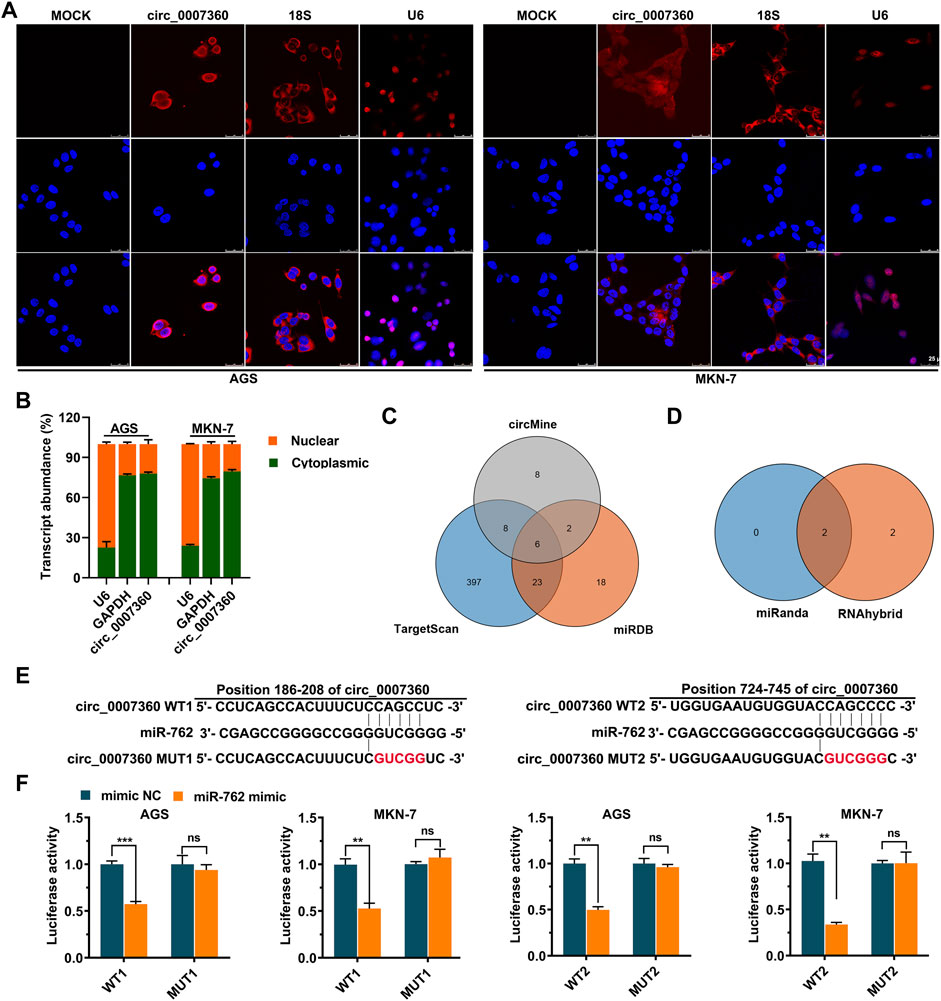
FIGURE 2. Circ0007360 is a cytoplasmic circular RNA that may sponge miR-762. (A) Representative images from RNA fluorescent in situ hybridization (FISH) for detecting circ0007360, 18S, or U6 RNA. DAPI was applied to stain the nucleus. (B) Subcellular fractionation assay to measure the levels of U6, GAPDH, or circ0007360 in the nucleus or cytoplasm. (C) Schematic plot showing the interacting site between wild type (WT) or mutant (MUT) circ0007360 and miR-762. (D) The relative luciferase activity of WT or MUT circ0007360 constructs in the presence or absence of miR-762 mimic.
Circ0007360 Inhibits While miR-762 Promotes the Survival, Migration, and Invasion of Gastric Cancer Cells
To investigate the effects of circ0007360 and miR-762 on the survival of gastric cancer cells, we misexpressed them by transfecting circ0007360 ectopic expressing construct, shRNA against circ0007360, or miRNA inhibitors for miR-762 into cells (Figure 3A). MTT evaluation suggested that overexpression of circ0007360 or depletion of miR-762 mitigated, while inhibition of circ0007360 expression augmented the proliferation of gastric cancer cells (Figure 3B). Moreover, colony formation analysis confirmed the negative or positive effects of circ0007360 and miR-762 on gastric cancer growth, respectively (Figures 3C,D). We then tested whether the cell cycle was altered upon the misexpression of these two RNAs. Results from propidium iodide (PI)-based flow cytometry assay revealed that overexpression of circ0007360 or knockdown of miR-762 alleviated, whereas circ0007360 depletion potentiated the cell cycle progression of gastric cancer cells (Figures 3E,F). In contrast, we found that the apoptosis of cells was enhanced by circ0007360 overexpression or the absence of miR-762 but was inhibited by silencing circ0007360 expression (Figures 3G,H). Furthermore, we examined the migration and invasion of gastric cancer cells in the aforementioned genetic settings. Surprisingly, the migratory and invasive abilities of cells decreased significantly when circ0007360 was ectopically expressed or miR-762 was depleted, while the migration and invasion increased upon knockdown of circ0007360 (Figures 4A,B). Taken together, our results demonstrate the tumor-suppressive effects of circ0007360 and the tumor-promoting effects of miR-762 in two gastric cancer cell lines.
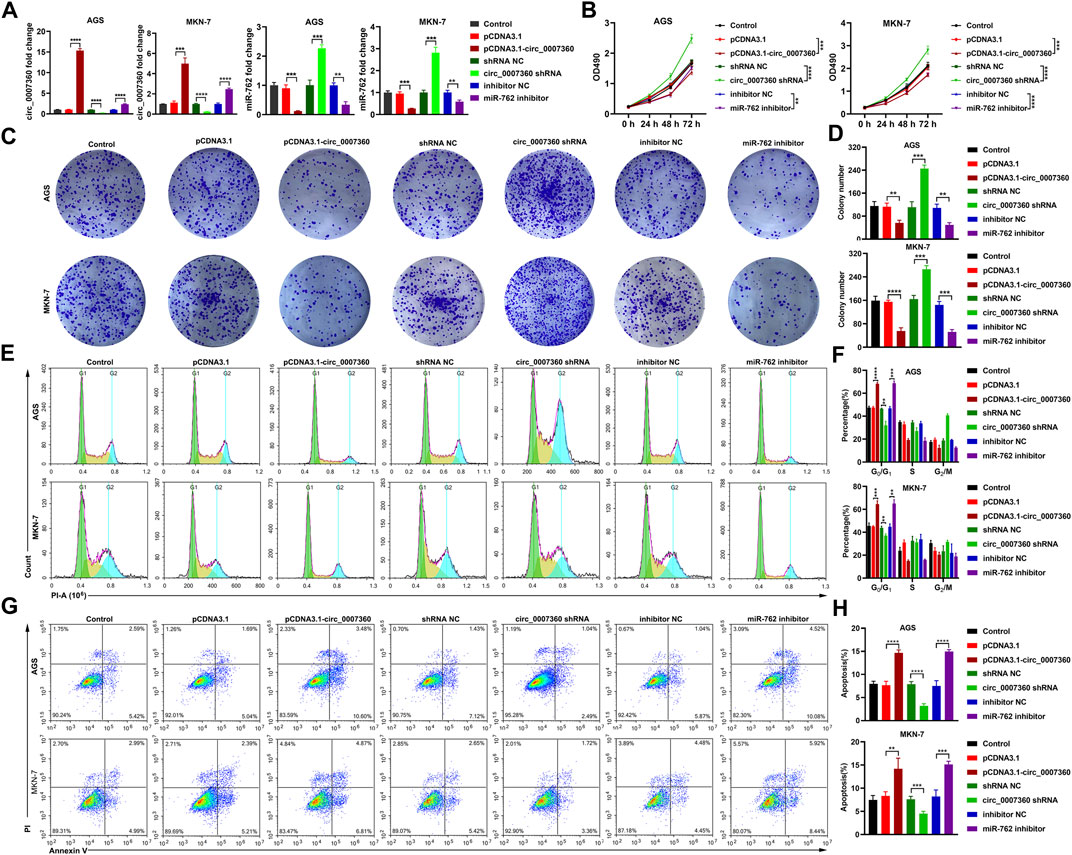
FIGURE 3. Circ0007360 suppresses while miR-762 enhances cell survival. RT-qPCR quantification of circ0007360 or miR-762 expression with ectopic expression of circ0007360, knockdown of circ0007360, or miR-762 in AGS or MKN-7 cells. (B) MTT assay for detecting cell proliferation upon ectopic expression of circ0007360, knockdown of circ0007360, or miR-762. (C,D) Representative images (C) and quantification (D) of colony formation results from cells with ectopic expression of circ0007360, knockdown of circ0007360, or miR-762. (E,F) Representative images (E) and quantification (F) of cell cycle analysis using flow cytometry of cells with ectopic expression of circ0007360, knockdown of circ0007360, or miR-762. (G,H) Representative images (G) and quantification (H) of cell apoptosis analysis using flow cytometry of cells with ectopic expression of circ0007360, knockdown of circ0007360 or miR-762.
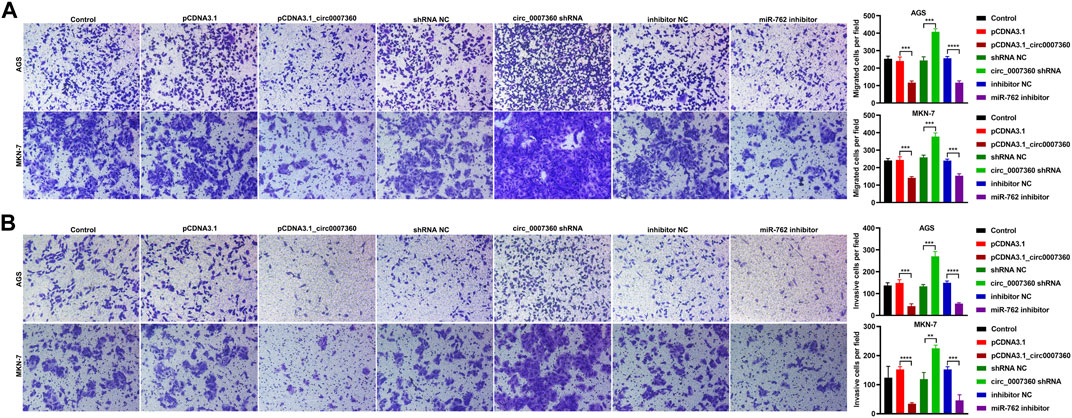
FIGURE 4. Circ0007360 attenuates while miR-762 augments cell migration and invasion. (A,B) Representative images and quantification of transwell results for detecting the migration (A) or invasion (B) in cells with ectopic expression of circ0007360, knockdown of circ0007360, or miR-762.
Circ0007360 Suppresses While miR-762 Enhances the Stemness of Gastric Cancer Cells
Considering the contribution of cancer stem cells to tumor recurrence and chemotherapy resistance (Bekaii-Saab and El-Rayes, 2017), we investigated whether circ0007360 and miR-762 affect the stemness of gastric cancer cells. Data from tumor sphere formation assays showed that circ0007360 overexpression or miR-762 depletion reduced the size of tumor spheres formed by gastric cancer cells (Figure 5A). However, the absence of circ0007360 promoted the size of the tumor spheres (Figure 5A). We then tested the cell surface expression of stem cell markers to further evaluate the stemness of cells at the molecular level. Flow cytometry results revealed that the levels of CD44 and EpCAM were downregulated upon circ0007360 ectopic expression or miR-762 depletion, but the levels of the two markers were upregulated when circ0007360 was silenced (Figure 5B). Furthermore, protein levels of other stemness markers, such as MYC, Nanog, ALDH1, SOX2, and CD133, were also reduced by the overexpression of circ0007360 or miR-762 depletion (Figure 5C). However, knockdown of circ0007360 potentiated the protein levels of these markers (Figure 5C). In conclusion, circ0007360 hinders to, while miR-762 contributes to, the stemness of gastric cancer cells.
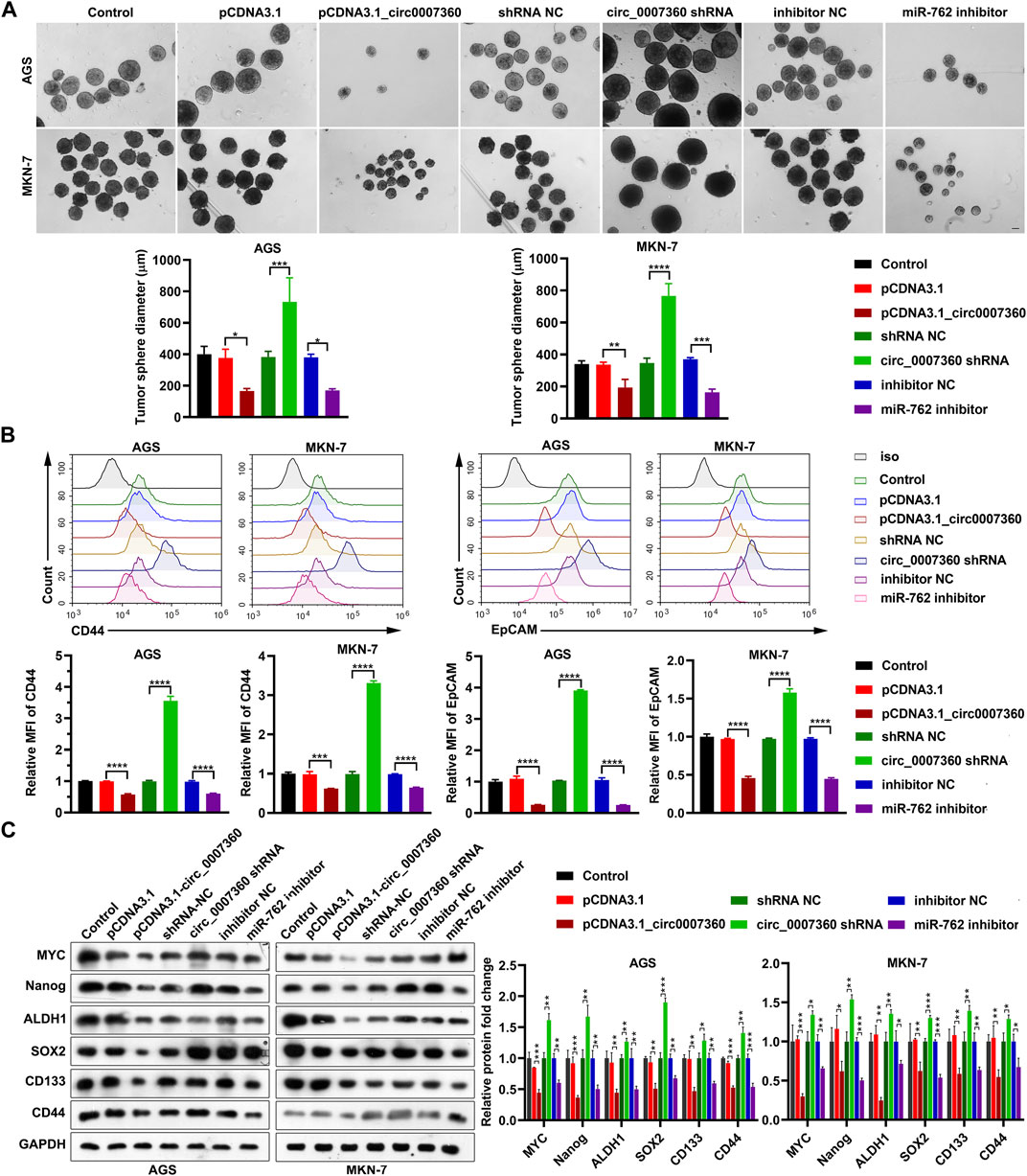
FIGURE 5. Circ0007360 inhibits while miR-762 promotes the stemness of gastric cells. (A) Representative images and quantification of tumor sphere formation results of stemness measured in cells with ectopic expression of circ0007360, knockdown of circ0007360, or miR-762. (B) Representative images and quantification of flow cytometry analysis results of the expression of CD44 and EpCAM on the cell surface in cells with ectopic expression of circ0007360, knockdown of circ0007360, or miR-762. (C) Representative images and quantification of the western blotting detection of stemness markers expressed in cells with ectopic expression of circ0007360, knockdown of circ0007360, or miR-762.
The Mitigation of Gastric Cancer Progression by circ0007360 Is Dependent on Sponging miR-762
To validate the significance of miR-762 in the effects exerted by circ0007360, we proceeded by depleting miR-762 in gastric cancer cells with circ0007360 knockdown (Figure 6A). Surprisingly, we observed that the enhancement of cell proliferation, colony formation abilities, cell cycle progression, cell migration, invasion, and stemness of cells, and the inhibition of cell apoptosis mediated by the absence of circ0007360 were significantly restored when miR-762 expression was silenced by the specific miRNA inhibitors (Figures 6A–I and Supplementary Figure S1). Taken together, the rescue experiments demonstrate that the biological effects triggered by the loss of circ0007360 are highly dependent on the upregulation of miR-762, which serves as a pivotal downstream target miRNA of circ0007360.
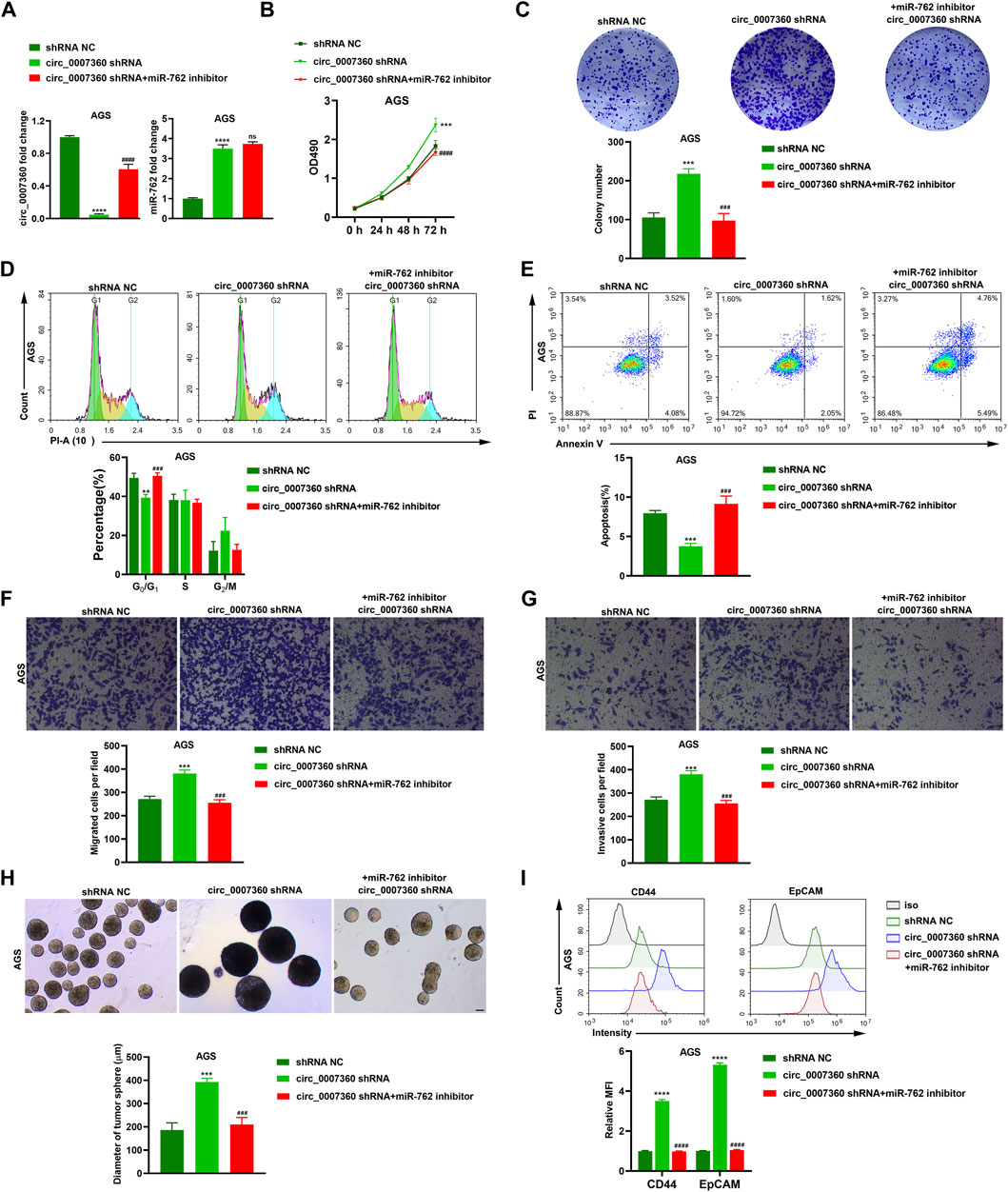
FIGURE 6. Inhibition of miR-762 is responsible for the tumor-suppressive role of circ0007360. (A) RT-qPCR analysis of circ0007360 or miR-762 expression with circ0007360 knockdown and miR-762 re-expression in AGS cells. (B) MTT assay in AGS cells with circ0007360 depletion and miR-762 re-expression. (C) Representative images and quantification of colony formation assays in AGS cells with circ0007360 knockdown and miR-762 re-expression. (D) Representative images and quantification of cell cycle analysis by flow cytometry in AGS cells with circ0007360 knockdown and miR-762 re-expression. (E) Representative images and quantification of cell apoptosis analysis using flow cytometry in AGS cells with circ0007360 knockdown and miR-762 re-expression. (F,G) Representative images and quantification of transwell results for detecting the migration (F) or invasion (G) in AGS cells with circ0007360 knockdown and miR-762 re-expression. (H) Representative images and quantification of tumor sphere formation results of stemness measured in AGS cells with circ0007360 knockdown and miR-762 re-expression. (I) Representative images and quantification of flow cytometry analysis of the expression of CD44 and EpCAM on the cell surface in AGS cells with circ0007360 knockdown and miR-762 re-expression.
IRF7 Is a Target mRNA of miR-762
To screen the target mRNA of miR-762, we mined three well-established databases, miRDIP (Tokar et al., 2018), TargetScan (Agarwal et al., 2015), and miRTarBase (Huang et al., 2020) (Figure 7A). Of note, IRF7 was the only mRNA hit shared by these three databases (Figures 7A,B). Next, we performed a luciferase reporter assay to validate the interaction between miR-762 and the IRF7 3′UTR. As expected, miR-762 dramatically inhibited the activity of the wild type, but not the mutant IRF7 3′UTR (Figure 7C). Consistently, both the mRNA and protein levels of IRF7 increased upon depletion of miR-762 (Figures 7D–F). Moreover, as the upstream modulator of miR-762, circ0007360 positively regulated the levels of IRF7 (Figures 7D–F). Thus, our results demonstrate that circ0007360 may indirectly enhance the expression of IRF7 by decreasing miR-762 levels.
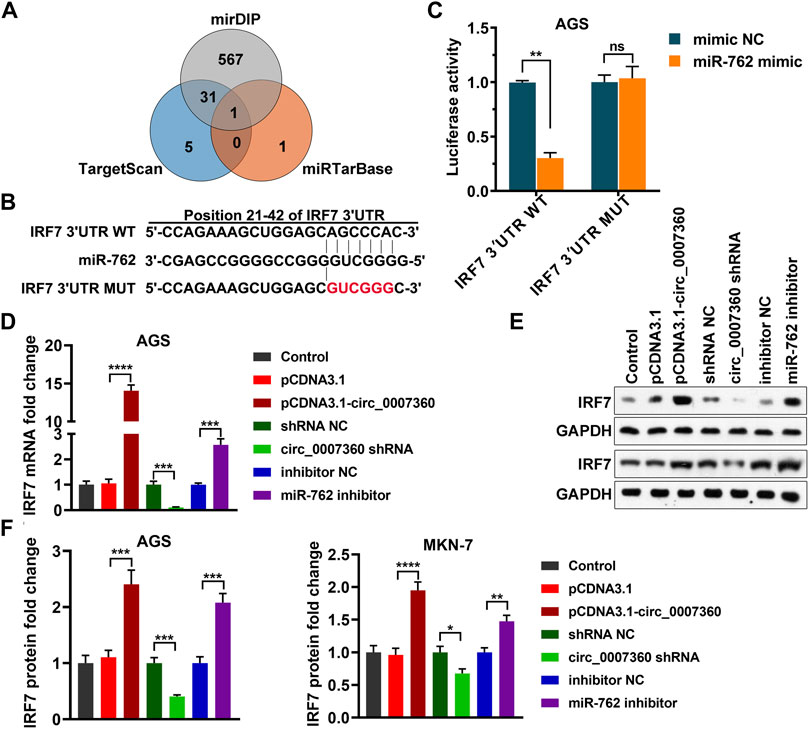
FIGURE 7. IRF7 is a target mRNA of miR-762. (A) Venn diagram indicating the intersection between three databases for predicting the target genes of miR-762. (B) Schematic plot showing the interacting site between the wild type (WT) or mutant (MUT) IRF7 3′UTR and miR-762. (C) The relative luciferase activity of WT or MUT IRF7 3′UTR in the presence or absence of miR-762 mimic. (D,E) RT-qPCR (D) or western blotting (E) quantification of IRF7 expression in AGS cells with ectopic expression of circ0007360, knockdown of circ0007360, or miR-762. (F) Quantification of IRF7 protein levels in AGS or MKN-7 cells with ectopic expression of circ0007360, knockdown of circ0007360, or miR-762.
The Augmentation of Gastric Cancer Progression Triggered by miR-762 Is Dependent on Suppressing IRF7
Since we validated that IRF7 is a downstream target gene of miR-762, the following investigations were performed to confirm the significance of IRF7 in miR-762-induced gastric cancer progression. First, IRF7 was silenced by shRNA in cells depleted of miR-762 (Figures 8A,B). Results from MTT and colony formation assays indicated that inhibition of cell survival resulting from miR-762 knockdown was alleviated when IRF7 was depleted (Figures 8C,D). In line with these data, the effects of miR-762 depletion on cell cycle arrest, apoptosis, migration, invasion, and stemness were rescued upon silencing IRF7 (Figures 8E–J). Thus, our data prove that IRF7 inhibition is responsible for the tumor-promoting function directed by miR-762. Moreover, taking all these in vitro data together, we indicate that the progression of gastric cancer may be attenuated by the circ0007360/miR-762/IRF7 axis.
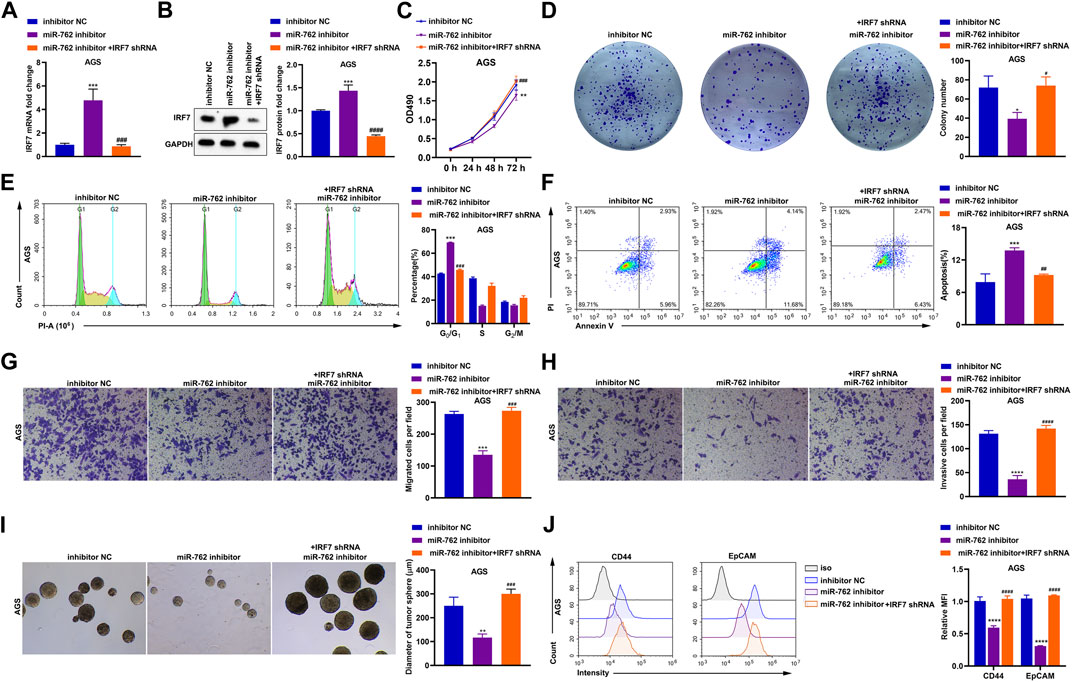
FIGURE 8. The tumor-promoting function of miR-762 is dependent on the downregulation of IRF7. (A) RT-qPCR detection of IRF7 mRNA expression in the presence or absence of miR-762 inhibitor or IRF7 shRNA. (B) Representative images and quantification of western blotting detection of IRF7 protein level in the presence or absence of miR-762 inhibitor or IRF7 shRNA. (C) MTT analysis in AGS cells transfected with or without miR-762 inhibitor or IRF7 shRNA. (D) Representative images and quantification of colony formation result in the presence or absence of miR-762 inhibitor or IRF7 shRNA. (E) Representative images and quantification of cell cycle analysis in the presence or absence of miR-762 inhibitor or IRF7 shRNA. (F) Representative images and quantification of apoptosis analysis in the presence or absence of miR-762 inhibitor or IRF7 shRNA. (G,H) Representative images and quantification of transwell analysis for checking the migration (G) or invasion (H) in the presence or absence of miR-762 inhibitor or IRF7 shRNA. (I) Representative images and quantification of tumor sphere formation in the presence or absence of miR-762 inhibitor or IRF7 shRNA. (J) Flow cytometry quantification of CD44 and EpCAM expression in the presence or absence of miR-762 inhibitor or IRF7 shRNA.
Circ0007360 Suppresses Gastric Cancer Growth and Stemness In Vivo
To further consolidate the aforementioned in vitro results, we performed in vivo experiments to determine the effects of circ0007360 on gastric cancer cells. Therefore, we inoculated AGS cells with or without circ0007360 depletion in mice through subcutaneous injection and found that the tumor volume and tumor weight increased significantly in the circ0007360 knockdown group compared with the control group (Figures 9A–C). Moreover, RT-qPCR results confirmed that miR-762 levels were enhanced, while IRF7 levels were reduced, upon depletion of circ0007360 in the isolated tumors formed by the human gastric cancer cells (Figures 9D–F). Furthermore, we found that absence of circ0007360 resulted in the upregulation of stemness markers expressed, as observed in in vitro assays (Figures 9G,H). Importantly, knockdown of circ0007360 led to an increase in the percentage of Ki67-positive cells in tumors (Figures 9I,J). Collectively, the in vivo mouse experiments confirm the inhibitory role of circ0007360 on tumorigenesis and cell stemness and the existence of the circ0007360/miR-762/IRF7 axis in tumors formed in the mouse xenograft model.
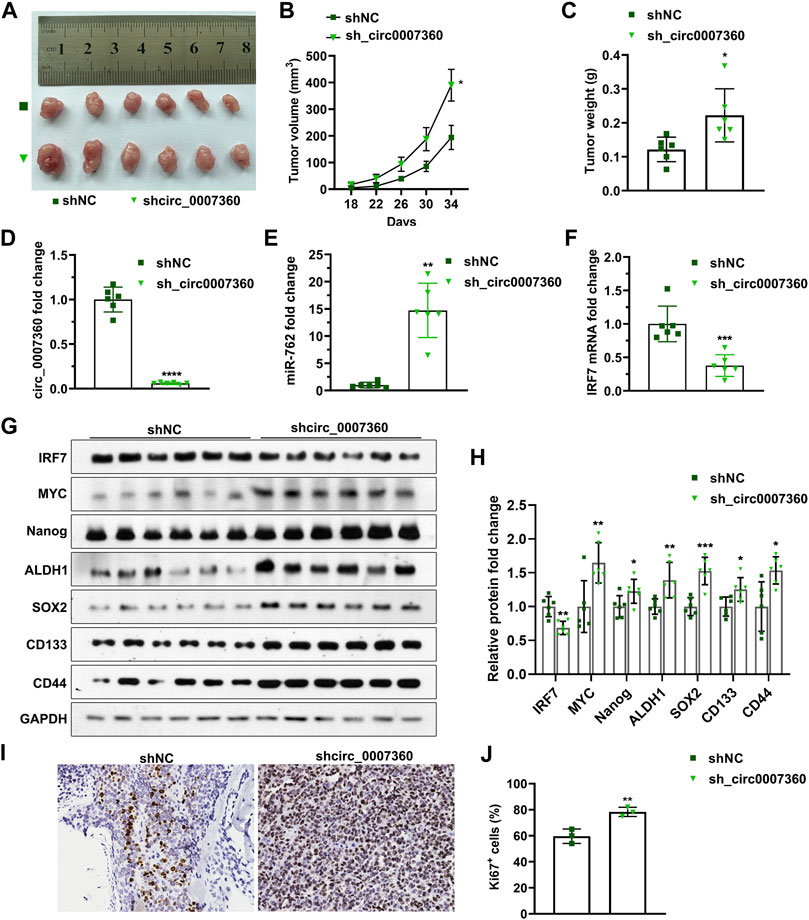
FIGURE 9. Depletion of circ0007360 enhances gastric tumor formation in mice. (A) Representative images of tumors formed by cells with circ0007360 depletion in mice via subcutaneous injection. (B,C) Volume of tumors (B) and quantification of tumor weight (C) formed by AGS cells with circ0007360 depletion via subcutaneous injection in mice. (D–F) RT-qPCR detection of circ0007360 (D), miR-762 (E), or IRF7 (F) RNA levels in tumors formed by AGS cells with circ0007360 depletion via subcutaneous injection in mice. (G,H) Representative images and quantification of western blotting detection of IRF7 and markers involved in cell stemness regulation. (I,J) Representative images (I) and quantification (J) of immunohistochemistry staining for Ki67 in tumors formed by cells with circ0007360 depletion.
Discussion
The present study have unveiled the tumor-suppressive role of circ0007360 in gastric cancer progression and have uncovered the modulation of the miR-762/IRF7 axis as the underlying mechanism. Circ0007360, which is localized on chromosome 1, is a circRNA whose functions are yet to be investigated. Given the fact that circ0007360 is resistant to RNase R and is relatively stable in gastric cancer cells (Figures 1C,D), it is of great value to examine the expression pattern of circ0007360 in gastric cancer patient samples investigate whether circ0007360 can be utilized as a biomarker for gastric cancer diagnosis in the future. To pave the way towards the clinical application of circ0007360, transcriptome data from patients with gastric cancer can be mined to test the correlation between circ0007360 levels and clinicopathological parameters such as cancer stages and subtypes. Moreover, the patient survival data may provide insight into the prognostic significance of circ0007360.
MiR-762 has been reported to be a tumor-promoting miRNA in multiple cancers, such as non-small cell lung cancer and breast cancer (Xu et al., 2021; Tokar et al., 2018; Huang et al., 2020). Although circLPAR1 has been shown to harbor miR-762 by sequence matching in bladder cancer cells, the biological significance of miR-762 in regulating the effects mediated by circLPAR1 in this setting has not been well studied. In our research, circ0007630 is identified, for the first time, as another key upstream sponge for negatively controlling the endogenous levels of miR-762 (Figure 2). Importantly, we consolidates the importance of miR-762 mitigation for accomplishing the tumor-inhibitory role of circ0007360 (Figure 6) and further links IRF7 as a downstream effector of miR-762 (Figures 7, 8). The latter also validates the results from a previous study on breast cancer, which demonstrated that IRF7 is a key target mRNA of miR-762 (Huang et al., 2020).
Recently, several research groups have shown that circRNAs are capable of encoding polypeptides that serve as scaffolds for certain proteins to exert their regulatory effects (Li et al., 2021; Liu et al., 2021; Shen et al., 2021). We examined the protein coding potential of the bone fide polypeptides of circ0007360 and found that the coding probabilities of them are rather low (data not shown). Yet, we cannot rule out the possibility that polypeptides may be generated from circ0007360 and contribute to the effects exerted by circ0007360. In the future investigation, proteome data can be mined to unravel whether polypeptides might be derived from circ0007360.
IRF7 belongs to the interferon regulatory factor family of transcription factors (Ning et al., 2011). In addition to its master role in controlling type I interferon production and immune system regulation, IRF7 acts as an oncogene or tumor suppressor, depending on the cell types used for investigations (Zhang et al., 2004; Andrews et al., 2002). Although the promoter of IRF7 was hypermethylated in gastric cancer samples (Jee et al., 2009), the effect of IRF7 on gastric cancer progression has not been reported. In our study, through loss-of-function experiments, we find that IRF7 acts as a suppressor of the survival, migration, and invasion of gastric cancer cells (Figure 8 and Supplementary Figure S1). This may be further complemented by ectopic expression analysis to better understand the effects of IRF7 on gastric cancer progression. More importantly, we have shown that IRF7 is a negative regulator of stemness in gastric cancer cells. To uncover the mechanism by which IRF7 regulates gastric cancer cell stemness, pathways involved in stemness modulation, such as Wnt/β-catenin signaling (Zhan et al., 2017), can be detected and transcriptomic analysis upon IRF7 misexpression may aid in identifying the transactional target of this protein.
Although we have shown that the circ0007360/miR-762/IRF7 axis is of pivotal significance in gastric cancer progression through in vitro and in vivo models, the correlations between these molecules can be checked in clinical gastric samples by analyzing the patient-derived RNA-seq data or by applying the detection on tissue microarrays from our patient cohort. These in-depth analyses will contribute to unveiling the sophisticated mechanisms of gastric cancer progression and shed light on the therapeutic benefits for patients with gastric cancer.
Data Availability Statement
The original contributions presented in the study are included in the article/Supplementary Material, further inquiries can be directed to the corresponding author.
Ethics Statement
The animal study was reviewed and approved by Animal Care and Use Committee of Nanchang University.
Author Contributions
YX, HC, and ZG designed and performed the experiments, analyzed, interpreted the results and created the Figures. XZ directed the research and supervised the project. YX, HC, and XZ wrote the manuscript. All authors revised the content and approved the final manuscript.
Funding
This research was supported by the Natural Science Foundation of China (No. 81760524 and No. 81560395).
Conflict of Interest
The authors declare that the research was conducted in the absence of any commercial or financial relationships that could be construed as a potential conflict of interest.
Publisher’s Note
All claims expressed in this article are solely those of the authors and do not necessarily represent those of their affiliated organizations, or those of the publisher, the editors and the reviewers. Any product that may be evaluated in this article, or claim that may be made by its manufacturer, is not guaranteed or endorsed by the publisher.
Supplementary Material
The Supplementary Material for this article can be found online at: https://www.frontiersin.org/articles/10.3389/fcell.2022.789073/full#supplementary-material
References
Agarwal, V., Bell, G. W., Nam, J. W., and Bartel, D. P. (2015). Predicting Effective microRNA Target Sites in Mammalian mRNAs. Elife 4. doi:10.7554/eLife.05005
Anderson, W. F., Camargo, M. C., Fraumeni, J. F., Correa, P., Rosenberg, P. S., and Rabkin, C. S. (2010). Age-Specific Trends in Incidence of Noncardia Gastric Cancer in US Adults. Jama 303 (17), 1723–1728. doi:10.1001/jama.2010.496
Andrews, H. N., Mullan, P. B., McWilliams, S., Sebelova, S., Quinn, J. E., Gilmore, P. M., et al. (2002). BRCA1 Regulates the Interferon γ-mediated Apoptotic Response. J. Biol. Chem. 277 (29), 26225–26232. doi:10.1074/jbc.m201316200
Bekaii-Saab, T., and El-Rayes, B. (2017). Identifying and Targeting Cancer Stem Cells in the Treatment of Gastric Cancer. Cancer 123 (8), 1303–1312. doi:10.1002/cncr.30538
Camargo, M. C., Mera, R., Correa, P., Peek, R. M., Fontham, E. T. H., Goodman, K. J., et al. (2006). Interleukin-1β and Interleukin-1 Receptor Antagonist Gene Polymorphisms and Gastric Cancer: A Meta-Analysis. Cancer Epidemiol. Biomarkers Prev. 15 (9), 1674–1687. doi:10.1158/1055-9965.epi-06-0189
Chen, L., Li, Y., and Lu, J. (2020). Identification of Circulating miR-762 as a Novel Diagnostic and Prognostic Biomarker for Non-small Cell Lung Cancer. Technol. Cancer Res. Treat. 19, 1533033820964222. doi:10.1177/1533033820964222
Chen, Y., and Wang, X. (2020). miRDB: an Online Database for Prediction of Functional microRNA Targets. Nucleic Acids Res. 48, D127–D131. doi:10.1093/nar/gkz757
Correa, P. (2013). Gastric Cancer. Gastroenterol. Clin. North America 42 (2), 211–217. doi:10.1016/j.gtc.2013.01.002
Eger, N., Schoppe, L., Schuster, S., Laufs, U., and Boeckel, J.-N. (2018). Circular RNA Splicing. Circular Rnas: Biogenesis and Functions 1087, 41–52. doi:10.1007/978-981-13-1426-1_4
Garcia-Mayea, Y., Mir, C., Masson, F., Paciucci, R., and LLeonart, M. E. (2020). Insights into New Mechanisms and Models of Cancer Stem Cell Multidrug Resistance. Semin. Cancer Biol. 60, 166–180. doi:10.1016/j.semcancer.2019.07.022
Ge, P., Cao, L., Chen, X., Jing, R., and Yue, W. (2019). miR-762 Activation Confers Acquired Resistance to Gefitinib in Non-small Cell Lung Cancer. BMC cancer 19 (1), 1203. doi:10.1186/s12885-019-6416-4
Goodall, G. J., and Wickramasinghe, V. O. (2021). RNA in Cancer. Nat. Rev. Cancer 21 (1), 22–36. doi:10.1038/s41568-020-00306-0
Hsiao, K.-Y., Sun, H. S., and Tsai, S.-J. (2017). Circular RNA - New Member of Noncoding RNA with Novel Functions. Exp. Biol. Med. (Maywood) 242 (11), 1136–1141. doi:10.1177/1535370217708978
Huang, H. Y., Lin, Y. C., Li, J., Huang, K. Y., Shrestha, S., Hong, H. C., et al. (2020). miRTarBase 2020: Updates to the Experimentally Validated microRNA-Target Interaction Database. Nucleic Acids Res. 48 (D1), D148–D154. doi:10.1093/nar/gkz896
Jeck, W. R., and Sharpless, N. E. (2014). Detecting and Characterizing Circular RNAs. Nat. Biotechnol. 32 (5), 453–461. doi:10.1038/nbt.2890
Jee, C. D., Kim, M. A., Jung, E. J., Kim, J., and Kim, W. H. (2009). Identification of Genes Epigenetically Silenced by CpG Methylation in Human Gastric Carcinoma. Eur. J. Cancer 45 (7), 1282–1293. doi:10.1016/j.ejca.2008.12.027
Li, F., Cai, Y., Deng, S., Yang, L., Liu, N., Chang, X., et al. (2021). A Peptide CORO1C-47aa Encoded by the Circular Noncoding RNA Circ-0000437 Functions as a Negative Regulator in Endometrium Tumor Angiogenesis. J. Biol. Chem. 297, 101182. doi:10.1016/j.jbc.2021.101182
Li, Y., Huang, R., Wang, L., Hao, J., Zhang, Q., Ling, R., et al. (2015). microRNA-762 Promotes Breast Cancer Cell Proliferation and Invasion by Targeting IRF7 Expression. Cell Prolif. 48 (6), 643–649. doi:10.1111/cpr.12223
Liu, Y., Li, Z., Zhang, M., Zhou, H., Wu, X., Zhong, J., et al. (2021). Rolling-translated EGFR Variants Sustain EGFR Signaling and Promote Glioblastoma Tumorigenicity. Neuro Oncol. 23, 743–756. doi:10.1093/neuonc/noaa279
Matsuyama, H., and Suzuki, H. I. (2020). Systems and Synthetic microRNA Biology: From Biogenesis to Disease Pathogenesis. Int. J. Mol. Sci. 21 (1). doi:10.3390/ijms21010132
Ning, S., Pagano, J. S., and Barber, G. N. (2011). IRF7: Activation, Regulation, Modification and Function. Genes Immun. 12 (6), 399–414. doi:10.1038/gene.2011.21
Persson, C., Canedo, P., Machado, J. C., El-Omar, E. M., and Forman, D. (2011). Polymorphisms in Inflammatory Response Genes and Their Association with Gastric Cancer: A HuGE Systematic Review and Meta-Analyses. Am. J. Epidemiol. 173 (3), 259–270. doi:10.1093/aje/kwq370
Salzman, J. (2016). Circular RNA Expression: Its Potential Regulation and Function. Trends Genet. 32 (5), 309–316. doi:10.1016/j.tig.2016.03.002
Shen, S., Yang, Y., Shen, P., Ma, J., Fang, B., Wang, Q., et al. (2021). circPDE4B Prevents Articular Cartilage Degeneration and Promotes Repair by Acting as a Scaffold for RIC8A and MID1. Ann. Rheum. Dis. 80, 1209–1219. doi:10.1136/annrheumdis-2021-219969
Takaishi, S., Okumura, T., Tu, S., Wang, S. S. W., Shibata, W., Vigneshwaran, R., et al. (2009). Identification of Gastric Cancer Stem Cells Using the Cell Surface Marker CD44. Stem cells 27 (5), 1006–1020. doi:10.1002/stem.30
Tokar, T., Pastrello, C., Rossos, A. E. M., Abovsky, M., Hauschild, A.-C., Tsay, M., et al. (2018). mirDIP 4.1-integrative Database of Human microRNA Target Predictions. Nucleic Acids Res. 46 (D1), D360–D370. doi:10.1093/nar/gkx1144
Vo, J. N., Cieslik, M., Zhang, Y., Shukla, S., Xiao, L., Zhang, Y., et al. (2019). The Landscape of Circular RNA in Cancer. Cell 176 (4), 869–881. doi:10.1016/j.cell.2018.12.021
Xu, J., Ni, L., Zhao, F., Dai, X., Tao, J., Pan, J., et al. (2021). Overexpression of Hsa_circ_0002874 Promotes Resistance of Non-small Cell Lung Cancer to Paclitaxel by Modulating miR-1273f/MDM2/p53 Pathway. Aging 13 (4), 5986–6009. doi:10.18632/aging.202521
Zhan, T., Rindtorff, N., and Boutros, M. (2017). Wnt Signaling in Cancer. Oncogene 36 (11), 1461–1473. doi:10.1038/onc.2016.304
Zhang, L., Zhang, J., Lambert, Q., Der, C. J., Del Valle, L., Miklossy, J., et al. (2004). Interferon Regulatory Factor 7 Is Associated with Epstein-Barr Virus-Transformed central Nervous System Lymphoma and Has Oncogenic Properties. J. Virol. 78 (23), 12987–12995. doi:10.1128/jvi.78.23.12987-12995.2004
Zhang, W., Liu, Y., Min, Z., Liang, G., Mo, J., Ju, Z., et al. (2021). circMine: a Comprehensive Database to Integrate, Analyze and Visualize Human Disease-Related circRNA Transcriptome. Nucleic Acids Res. 50 (D1), D83–D92. doi:10.1093/nar/gkab809
Keywords: circ0007360, gastric cancer, progression, miR-762, IRF7
Citation: Xing Y, Chen H, Guo Z and Zhou X (2022) Circular RNA circ0007360 Attenuates Gastric Cancer Progression by Altering the miR-762/IRF7 Axis. Front. Cell Dev. Biol. 10:789073. doi: 10.3389/fcell.2022.789073
Received: 04 October 2021; Accepted: 25 January 2022;
Published: 17 February 2022.
Edited by:
Jing Zhang, Shanghai Jiao Tong University, ChinaReviewed by:
Raheleh Roudi, University of Minnesota, United StatesYeqian Zhang, Shanghai JiaoTong University, China
Copyright © 2022 Xing, Chen, Guo and Zhou. This is an open-access article distributed under the terms of the Creative Commons Attribution License (CC BY). The use, distribution or reproduction in other forums is permitted, provided the original author(s) and the copyright owner(s) are credited and that the original publication in this journal is cited, in accordance with accepted academic practice. No use, distribution or reproduction is permitted which does not comply with these terms.
*Correspondence: Xiaodong Zhou, ndyfy02046@ncu.edu.cn
†These authors have contributed equally to this work