- 1Centro Andaluz de Biología del Desarrollo CSIC-Universidad Pablo de Olavide, Sevilla, Spain
- 2Departamento de Biología Celular, Universidad de Sevilla, Sevilla, Spain
- 3GENYO, Granada, Spain
Adhesion to the extracellular matrix (ECM) is required for normal epithelial cell survival. Disruption of this interaction leads to a specific type of apoptosis known as anoikis. Yet, there are physiological and pathological situations in which cells not connected to the ECM are protected from anoikis, such as during cell migration or metastasis. The main receptors transmitting signals from the ECM are members of the integrin family. However, although integrin-mediated cell-ECM anchorage has been long recognized as crucial for epithelial cell survival, the in vivo significance of this interaction remains to be weighed. In this work, we have used the Drosophila wing imaginal disc epithelium to analyze the importance of integrins as survival factors during epithelia morphogenesis. We show that reducing integrin expression in the wing disc induces caspase-dependent cell death and basal extrusion of the dead cells. In this case, anoikis is mediated by the activation of the JNK pathway, which in turn triggers expression of the proapoptotic protein Hid. In addition, our results strongly suggest that, during wing disc morphogenesis, the EGFR pathway protects cells undergoing cell shape changes upon ECM detachment from anoikis. Furthermore, we show that oncogenic activation of the EGFR/Ras pathway in integrin mutant cells rescues them from apoptosis while promoting their extrusion from the epithelium. Altogether, our results support the idea that integrins promote cell survival during normal tissue morphogenesis and prevent the extrusion of transformed cells.
Introduction
To survive or die is a cellular decision controlled by environmental cues and physical stimuli. This life and death decision is deeply influenced by the extracellular matrix (ECM) (Meredith et al., 1993). When cells lose their normal interactions with the ECM, the cell cycle is arrested and a specific form of apoptosis, known as anoikis, is initiated (Frisch and Ruoslahti, 1997). During development, aberrant anoikis alters tissue architecture and function, compromising embryonic viability (Mole et al., 2021). Conversely, programmed anoikis contributes to physiological developmental processes and tissue renewal (Gilmore, 2005; Chiarugi and Giannoni, 2008). Anoikis is also an important surveillance mechanism, ensuring that any cell that loses its appropriate position within a tissue is targeted for death. For this reason, impaired anoikis contributes to the malignancy of many cancer cells, allowing them to survive without ECM anchorage and facilitating their dispersion [reviewed in (Zhong and Rescorla, 2012)]. Unraveling the mechanisms regulating anoikis is therefore crucial to understand both normal morphogenesis and cancer progression.
Although the concept of anchorage-dependent cell survival has been recognized for many years, it was in the early 1990’s when it was demonstrated that cells deprived of ECM attachment undergo anoikis (Meredith et al., 1993; Frisch and Francis, 1994). Anoikis can be considered a multistep process [reviewed in (Zhong and Rescorla, 2012)]. First of all, loss of anchorage results in inactivation of focal complex signalling molecules, including FAK and Src (Giancotti, 2000; Parsons, 2003). As a consequence, several pro-survival pathways such as PI3K/Akt and Raf/MEK/ERK are disrupted [reviewed in (Vachon, 2011)]. The second step in anoikis is the simultaneous disassembly of focal adhesions and the destabilization of the cytoskeleton, which leads to the release of pro-apoptotic Bcl-2 proteins (Martin and Vuori, 2004). The final step is the activation of apoptotic kinases, including JNK, which results in an increase in the expression and phosphorylation of the pro-apoptotic BH3-only proteins BIM an BMF (Girnius and Davis, 2017). However, the role of JNK in anoikis remains controversial, as, depending on the cell type, kinase isoform and stimulus, it can perform pro- or anti-apoptotic functions (Liu and Lin, 2005). Analysing JNK function in different cellular contexts shall help resolving its debated role in anoikis.
The major transmitters of survival signals from the ECM are members of the integrin family (Frisch and Ruoslahti, 1997; Meredith et al., 1993; Vachon, 2011). Integrins are a widely expressed family of heterodimeric transmembrane glycoproteins, composed of an a and a β subunit, that link the ECM to the actin cytoskeleton (Hynes, 1992). The vertebrate integrin family is comprised of 18 a and 8 β transmembrane subunits enabling about 24 different heterodimeric receptors for a diverse array of ECM proteins. However, only some integrins are capable of regulating cell viability and these include the β1 integrin subfamily (Stupack and Cheresh, 2002). The regulation of cell survival mediated by integrins implicates an increasing complexity of players depending on the tissue, cell type, specie and the cell differentiation state, emphasizing the need to study anoikis in a given tissue within its physiological context [reviewed in (Ignotz and Massague, 1987; Frisch and Screaton, 2001; Vachon, 2011)]. Nonetheless, the early embryonic lethality observed in some vertebrate models lacking β1 integrins has long precluded a direct evidence for a role of β1 integrins as survival factors in vivo (Stephens et al., 1995). The use of cell lineage-specific gene deletion approaches and transplantation experiments to circumvent early embryonic lethality has revealed that the role of integrin function in cell survival in vivo is multifaceted and sometimes contradictory. Thus, while depletion of β1 integrins from either the developing mouse lens, the epidermis or the endothelia results in apoptosis (DiPersio et al., 2000; Simirskii et al., 2007; Carlson et al., 2008), conditional deletion in the intestinal epithelium causes anoikis resistance, increased cell proliferation and defective differentiation (Jones et al., 2006). Furthermore, in some situations, cells are protected from anoikis, such as during cell migration or metastasis. In these cases, cells escape death using a variety of strategies, including hyperactivation of receptor tyrosine kinases (Guadamillas et al., 2011; Paoli et al., 2013).
Since the main components of the cell death machinery are evolutionary conserved, Drosophila, due to its unique genetic and cell biological advantages, has become an ideal model system to study apoptosis in the context of a developing organism. As in mammals, the caspase family of cysteine proteases is essential for the regulation of apoptosis also in Drosophila. These proteins are constitutively expressed as procaspases that have low but significant protease activity. To prevent unwanted consequences of basal procaspase activity, living cells express high levels of the Inhibitor of Apoptosis (IAP) proteins, Diap1 and Diap2, which act as E3-ubiquitin ligases and target activator and effector caspases for proteasome degradation [reviewed in (Hay, 2000)]. Upon an apoptotic stimulus, Diap1 antagonists, including hid, reaper (rpr), grim, sickle (skl), jafrac2, and dOmi/HtrA2 are transcriptionally activated and physically interact with Diap1 [reviewed in (Xu et al., 2009)]. This leads to the blockage of its caspase inhibitory function and the degradation of Diap1 by auto-inhibitory ubiquitinization. As a consequence, the caspase cascade is activated and the apoptosome is formed. First of all, initiator pro-caspases, including DRONC, the casp9 ortholog, DREDD and DCP-2, are activated by auto-cleavage. Then, initiator caspases catalyse the activation of the effector pro-caspases drICE and DCP-1, and this in turn cleave their substrates, including cytoskeleton proteins and DNA metabolic proteins (Cooper et al., 2009). However, despite the conservation of the cell death response between flies and vertebrates, little is known about the process of anoikis in Drosophila.
Using the primordium of the Drosophila wing, the wing imaginal disc, as model system, we have studied the implication of integrins in the regulation of epithelial cell survival in the context of a developing tissue. The formation of the wing starts during early embryonic development when about 30 cells are allocated to form the wing imaginal disc primordium. During larval life, disc cells divide and form an epithelial sac comprised of a folded columnar epithelium on one side and a squamous epithelium on the other (Garcia-Bellido and Merrian, 1971). The mature disc will give rise to the adult wing and notum. At the onset of metamorphosis, local cell shape changes promote the folding of the single-layered columnar epithelium into a bi-layered sheet in which epithelial cells face each other along their basal sides (Fristrom et al., 1993). Integrins perform two distinct functions in the wing disc, to hold the two layers together and to regulate the cell shape changes underlying folding (Brabant et al., 1996; Dominguez-Gimenez et al., 2007). However, despite the known role of integrins as survival factors in both benign and malignant epithelia [reviewed in (Gilcrease, 2007)], integrins have not yet been implicated in cell survival in wing disc epithelial cells.
Here, we show that low integrin levels induces caspase-dependent wing disc cell death. This anoikis process is mediated by the activation of the JNK pathway, which in turn induces expression of the proapoptotic gene hid. We also find that integrins cooperate with the EGFR pathway to maintain wing disc epithelial cell survival. Finally, our results demonstrate that an oncogenic version of Ras, RasV12, blocks anoikis in the wing disc and that elimination of integrins from transformed RasV12 cells stimulates their basal extrusion from the wing disc epithelium. Our results unravel new roles for integrins in epithelial cells in vivo, promoting cell survival during tissue morphogenesis and preventing the extrusion of transformed cells.
Materials and Methods
Fly Strains
The following stocks were used: UAS-RasV12 (Lee et al., 1996); nub-Gal4 (Calleja et al., 1996); hid 5′F-GFP (Tanaka-Matakatsu et al., 2009); UAS-DERDN, UAS-Diap1, UAS-puc, ap-Gal4, puc-LacZ, rpr-LacZ, and brk-LacZ (Bloomington Drosophila Stock Center); mysRNAi, UAS-diβ (Martin-Bermudo and Brown, 1999) and hidRNAi (Vienna Drosophila RNA-i Center). To generate mutant clones in the wing disc we used the FRT/FLP technique (Chou and Perrimon, 1992). Mutant clones were marked by the absence of GFP. The following mutant alleles and chromosomes were used: mys11 [also known as mysXG43 (Bunch et al., 1992)] and e22c-Gal4 UAS-flipase (Duffy et al., 1998). The e22c-Gal4 driver is expressed in some posterior wing disc cells and was therefore used in combination with UAS- flp to generate large mys clones. Flies were raised at 25°C.
Immunohistochemistry and Imaging
Wing imaginal discs were stained using standard procedures and mounted in Vectashield (Vector Laboratories, Burlingame, CA, United States). The following primary antibodies were used: goat anti-GFPFICT (Abcam, 1:500), rabbit anti-caspase Dcp1 (Cell Signaling; 1:100), rabbit anti-pJNK (Promega, 1:200), mouse anti-βGal (Promega, 1:1000), mouse anti-βPS (Developmental Studies Hybridoma Bank, DSHB, University of Iowa, United States, 1:50) and mouse anti-myc (Promega, 1:100). The secondary antibodies used were Alexa fluor 488, (Molecular ProbesTM) and Cy3 and Cy5 (Jackson ImmunoReseach Laboratories, Inc.) at 1: 200. DNA was labelled using Hoechst (Molecular Probes, 1:1000). Confocal images were obtained using a Leica SP5-MP-AOBS, equipped with a Plan-Apochromat 40X oil objective (NA 1.4).
Quantification of Fluorescence Intensity
To quantify fluorescent intensity of the different markers, fluorescent signaling was measured from maximum projections of 30 Z-confocal sections taken with 1 μm of interval per wing disc and genotype. Quantifications were made in the wing pouch region, which was manually selected using the FIJI-Image J line selection tool. Microscope settings were maintained between imaging sessions in each experiment. The background value taken from cell-free region was subtracted from all data series. Measurements of whole fluorescence intensity were done dividing the mean of all included pixels intensity by the outlined cell area, using the FIJI-Image J measure tool. Student’s t tests were used for statistical comparisons of fluorescence intensity values.
Results
Integrins are Required for the Survival of Wing Disc Epithelial Cells
To deepen our understanding of the role of integrins as survival factors during epithelia morphogenesis, we tested whether removing integrins in Drosophila wing disc epithelial cells affected their survival. The Drosophila genome contains two integrin β subunits, βPS and βν [reviewed in (Brown, 1993; Yee and Hynes, 1993). βPS, encoded by the gene myospheroid (mys), is the only β chain present in the wing imaginal disc (Brabant et al., 1996); Supplementary Figure S1]. First, we generated mosaic wing disc epithelia containing clones of cells homozygous for the null allele mysXG43 (from now on mys cells, white arrow in Figures 1A–B”). To detect apoptosis, we used an antibody to cleaved effector caspase Dcp-1 (Yu et al., 2002). In control wing imaginal discs, Dcp-1 activity is very low, being detected only occasionally in a few cells scattered throughout the disc (Figure 1A, n = 20, where n represents the number of wing discs analyzed). In contrast, all wing discs containing mys clones showed apoptotic cells within the clone area (Figure 1B, n = 18). Second, we reduced integrin levels in larger areas of the disc using the nubbin (nub) Gal4 line to express a mys RNAi (nub>mys RNAi) in the wing pouch (inset in Figure 1C) region in the center of the disc that gives rise to the adult wing, indicated with a dotted white circle in all figures (Brand and Perrimon, 1993; Calleja et al., 1996). Immunostaining of control (n = 30) and experimental nub>mysRNAi wing imaginal discs (n = 36) with antibodies against the βPS protein and cleaved Dcp-1 showed that the expression of the mys specific RNAi in the wing pouch caused a strong reduction in βPS protein levels (Figures 1C,C’) and a robust increase in apoptosis (Figures 1C,C’’,E). Thus, integrin expression in wing disc epithelial cells prevent them from undergoing cell death. It is known that embryonic epithelial cells undergoing apoptosis are removed from the epithelium by extrusion rather than phagocytosis (Rosenblatt et al., 2001). Similarly, integrin-defective cells were extruded (Figures 1C–C’’) and accumulated between the basal surface of the disc epithelium and the ECM, as seen with an antibody against the ECM component Perlecan (Supplementary Figure S2).
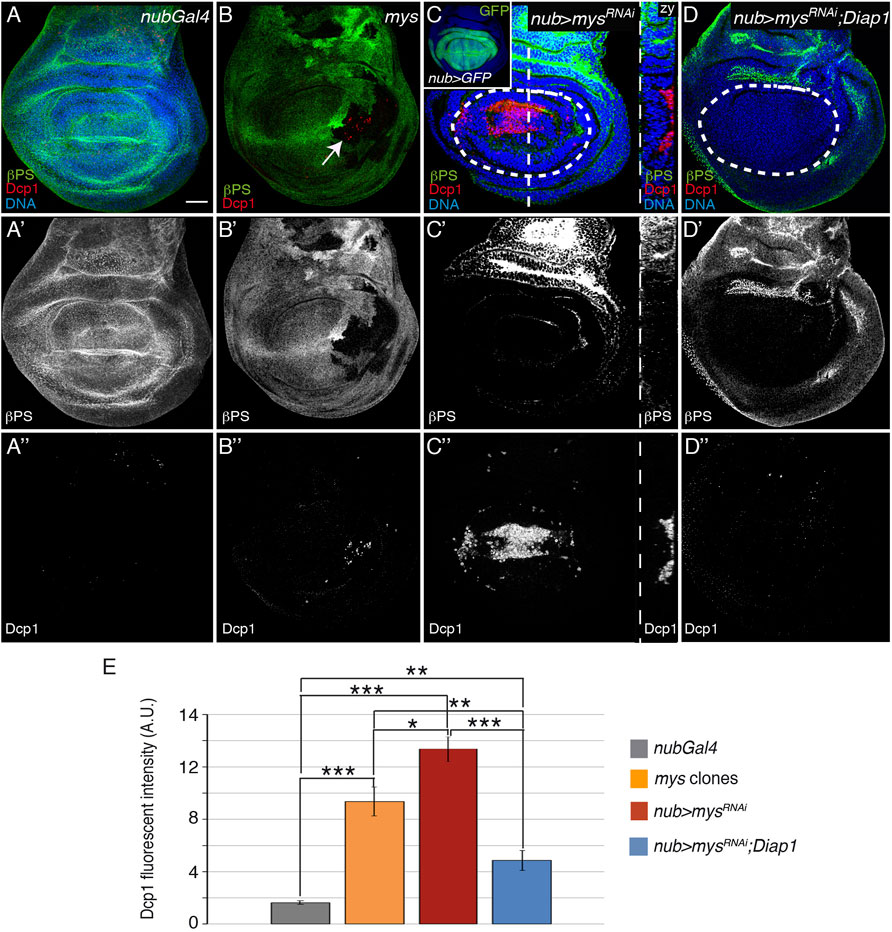
FIGURE 1. Integrins promote cell survival in the wing imaginal disc. (A–D′′) Maximal projection of confocal views of wing imaginal discs from third-instar larvae stained with anti-βPS [green in (A–D), white in (A′–D′)], anti-Dcp1 [red in (A–D), white in (A′′–D′′)] and the nuclear marker Hoechst [DNA, blue in (A–D)]. (A) Control nubGal4 wing disc. (B) Wing disc carrying mys mutant clones (white arrow), marked by the absence of βPS (green). (C) Wing disc expressing GFP or a mys RNAi under the control of nubGal4, nub > GFP (inset) and nub > mysRNAi, respectively. (yz) Confocal yz section along the white dotted line shown in (C). (D) Wing disc co-expressing a mys RNAi and Diap-1 under the control of nubGal4, nub > mysRNAi; Diap1. (E) Quantification of Dcp1 levels in wing discs of the designated genotypes. The dotted white circles indicate the wing pouch area in all figures. The statistical significance of differences was assessed with a t-test, ***, ** and * p values < 0.001, <0.01, and <0.05, respectively. Scale bar in all panels, 30 μm.
Integrins can mediate both signaling and adhesion [reviewed in (Adams and Watt, 1993)]. To address which integrin function is required to facilitate cell survival in the wing imaginal disc, we used a chimeric integrin (diβ) that lacks integrin-adhesive function but maintains its ability to signal (Martin-Bermudo and Brown, 1999; Dominguez-Gimenez et al., 2007). We had previously shown that expression of diβ in the wing disc inhibited the basal localization of endogenous integrin [(Supplementary Figures S3B,B’; (Dominguez-Gimenez et al., 2007)]. Here, we found that it caused an increase in apoptosis (Supplementary Figures S3B,B”), strongly suggesting that integrin signaling is not sufficient to prevent cell death in detached wing disc cells.
Altogether, these results propose that integrin-mediated adhesion to the ECM promotes cell survival in the wing disc epithelium by regulating caspase activity. To further support this hypothesis, we tested whether overexpression of one of the Drosophila IAP (inhibitor of apoptosis) proteins, Diap1, suppressed the cell death caused by expression of mys RNAi and found that it did (Figures 1D-D’’,E, n = 35).
Integrins DownRegulate Caspase Activity by Inhibiting Hid Expression
Previous experiments have shown that overexpression of hid or rpr induces downregulation of Diap1 levels and apoptosis in Drosophila wing imaginal discs (Milan et al., 1997; Bergantinos et al., 2010; Yoo et al., 2002). As Diap1 overexpression rescues cell death due to integrin downregulation, integrins could promote cell survival through the regulation of hid and/or rpr expression. To test this, we analysed hid and rpr expression in control and nub>mysRNAi wing discs, using the hid 5′F-GFP [from now on hidGFP, (Tanaka-Matakatsu et al., 2009)] and rprLacZ (Nordstrom et al., 1996) transcriptional reporters. hidGFP levels in control discs (hidGFP; nubGal4) are hardly detectable, consistent with a low occurrence of cell death in this tissue (Tanaka-Matakatsu et al., 2009); Figures 2A-A’’’,E]. This expression was dramatically upregulated in nub > mysRNAi discs (n = 25, Figures 2B-B’’’). In contrast, the normal expression pattern of rprLacZ, confined to a small region of the notum and two stripes in the D-V and A-P boundaries [(Nordstrom et al., 1996), Supplementary Figures S4A,A’], was not altered in nub > mysRNAi discs (n = 28, Supplementary Figures S4B,B’). These results suggest that integrins block hid, but not rpr expression in normal wing disc epithelial cells to promote cell survival. Furthermore, consistent with the idea that Drosophila hid acts upstream of Diap1 (Wang et al., 1999; Goyal et al., 2000), we found that expression of Diap1 was not able to rescue the increase in hidGFP levels observed in mysRNAi cells (n = 24, Figures 2C–C’’’).
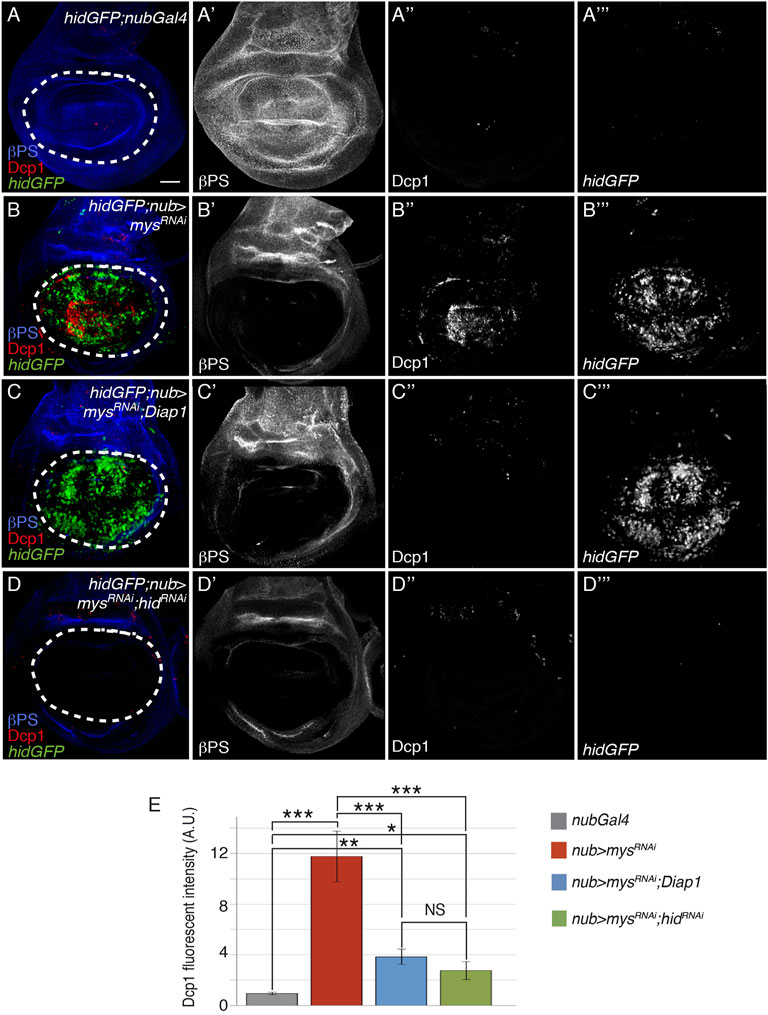
FIGURE 2. Integrins stimulate cell survival through inhibition of hid expression. (A–D‴) Maximal projection of confocal views of third-instar wing imaginal discs stained with anti-βPS [blue in (A–D), white in (A′–D′)], anti-Dcp1 [red in (A–D), white in (A′′–D′′)] and anti-GFP [green in (A–D), white in (A‴–D‴)]. (A–A‴) Control nubGal4 wing disc carrying a reporter of hid expression, hidGFP, hidGFP; nubGal4. (B–B‴) hidGFP wing disc expressing a mys RNAi under the control of nubGal4, hidGFP;nub > mysRNAi. (C–D‴) hidGFP wing disc co-expressing a mys RNAi under the control of nubGal4 with either Diap1, hidGFP; nub > mysRNAi; Diap1 (C–C‴), or a hid RNAi, hidGFP;nub > mysRNAi; hidRNAi (D–D‴). (E) Quantification of Dcp1 levels in wing discs of the indicated genotypes. The statistical significance of differences was assessed with a t-test, ***, ** and * p values < 0.001, <0.01, and <0.05, respectively. NS, non-statistically significant. Scale bar in all panels, 30 μm.
To further confirm hid involvement in integrin loss of function-dependent cell death, we tested whether reducing hid levels could rescue apoptosis in nub > mysRNAi discs. We found that expression of an RNAi against hid (hidGFP; nub > mysRNAi; hidRNAi) resulted in a significant reduction of mys RNAi-induced apoptosis (n = 20, Figures 2A-A’’’, B-B’’’, D-D’’’, E). These findings demonstrate that integrins promote cell survival in wing imaginal discs through the negative regulation of hid expression.
Altering the levels of Decapentaplegic (Dpp), a Drosophila transforming growth factor β homologue), in wing discs results in upregulation of brinker (brk), a transcriptional repressor that prevents apoptosis (Adachi-Yamada et al., 1999; Moreno et al., 2002). However, reducing integrin function does not seem to affect Dpp signalling, as the expression pattern of the brk reporter brkLacZ was not altered in nub > mysRNAi wing discs (n = 26, Supplementary Figure S5B).
Downregulation of Integrin Expression in Wing Discs Induces JNK Activation
As mentioned in the introduction, the role of JNK in anoikis is controversial, as, depending on the cell type and cellular context, it can be either essential (Frisch et al., 1996) or dispensable (Khwaja and Downward, 1997). In Drosophila wing discs, JNK activation triggers apoptosis in response to several stimuli, including distortion of positional information (Adachi-Yamada et al., 1999), TNF signalling (Igaki et al., 2002; Moreno et al., 2002) or irradiation (Luo et al., 2007). To study the role of the JNK pathway in apoptosis induced by integrin loss of function, we analyzed JNK activation using an antibody against phosphorylated JNK (pJNK, Figure 3). Compared to controls (n = 16, Figures 3A,A’,D), pJNK levels were upregulated in nub > mysRNAi discs (n = 18, Figures 3B,B’,D), suggesting that integrins control JNK activity in the wing disc. This was further confirmed using a reporter that monitors the transcription of a target of the JNK pathway, the JNK inhibitor puckered (pucLacZ) (Adachi-Yamada et al., 1999). We found that while in wild type wing discs, pucLacZ expression was restricted to a small proximal region (Adachi-Yamada et al., 1999), Supplementary Figures S6A,A’), in nub > mysRNAi wing discs, pucLacZ levels were strongly upregulated in the pouch (n = 28,Supplementary Figures S6B,B’).
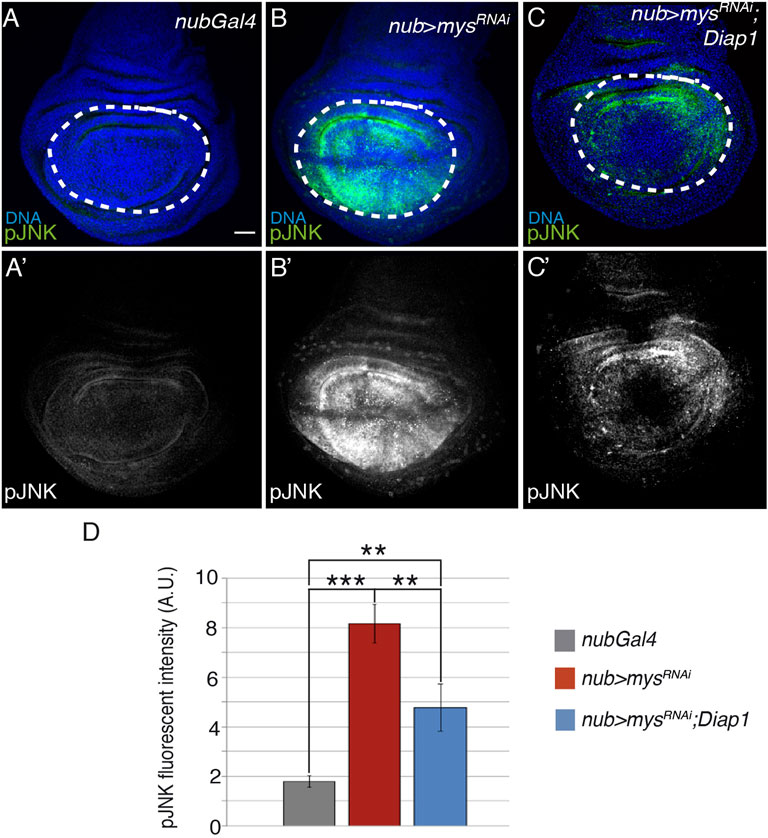
FIGURE 3. Elimination of integrins activates the JNK pathway. (A–C′) Maximal projection of confocal views of wing imaginal discs from third-instar larvae stained with anti-pJNK [green in (A–C), white in (A′–C′)] and the nuclear marker Hoechst [DNA, blue in (A–C)]. (A,A′) Control nubGal4 wing disc. (B,B′) Wing disc expressing a mys RNAi under the control of nubGal4, nub > mysRNAi. (C,C′) Wing disc co-expressing RNAi against mys and Diap1 under the control of nubGal4, nub > mysRNAi; Diap1. (D) Quantification of pJNK levels in wing discs of the indicated genotypes. The statistical significance of differences was assessed with a t-test, *** and ** p values < 0.001 and <0.01, respectively. Scale bar in all panels, 30 μm.
In some contexts, JNK signalling can be activated as a consequence of apoptosis (Kuranaga et al., 2002). To test whether activation of JNK lies upstream or downstream of loss of integrin function, JNK activation was assessed in nub > mysRNAi; Diap1 wing discs (Figure 3). We found that pJNK (n = 20, Figures 3C,D) and pucLacZ (n = 25, Supplementary Figures S6C,C’) levels were still upregulated despite rescue of apoptosis (Figure 1D), suggesting that JNK activation is upstream of cell death due to integrin loss of function in wing disc cells. Stress induced cell death in the wing disc activates JNK upstream of caspase activator proteins, which, in turn, reinforce JNK activation in a positive feedback loop to amplify the apoptotic response (Shlevkov and Morata, 2012). Here, we found that, in fact, the increase in JNK activity of nub > mysRNAi; Diap1 discs was lower than the one observed in nub > mysRNAi discs (Figures 3B–D). This result suggests that the feedback loop between JNK activity and apoptosis observed in stress-induced apoptosis may also operate in integrin loss of function-dependent apoptosis.
JNK Mediates Apoptosis due to Loss of Integrin Function in Wing Disc Cells
To test whether the JNK pathway mediates cell death due to integrin loss of function in wing imaginal discs, we analyzed if overexpression of the JNK inhibitor puc suppressed the cell death caused by mys RNAi (nub > mysRNAi;puc) and found that it did (Figures 4A–D, n = 24).
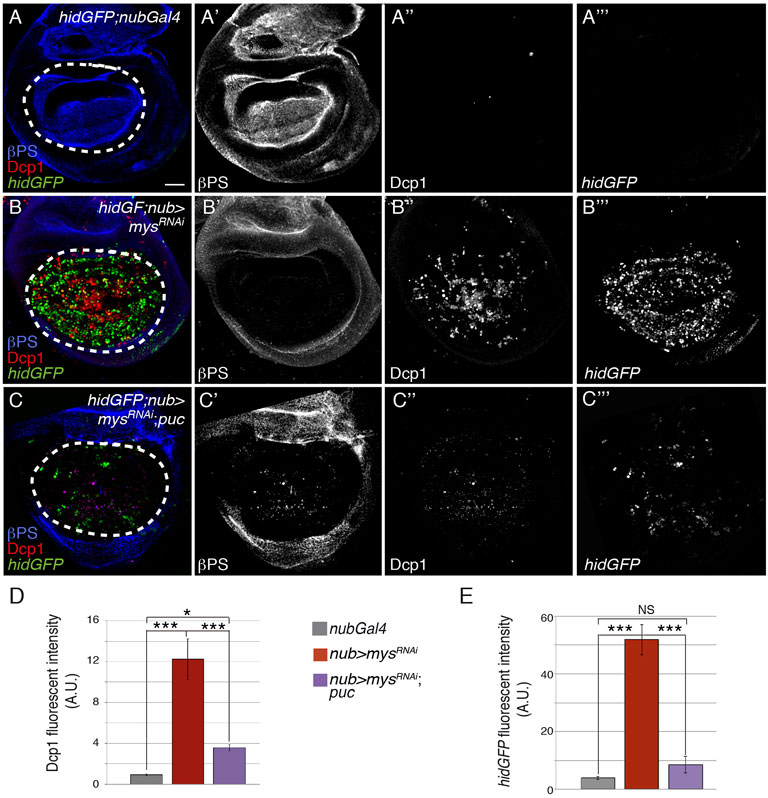
FIGURE 4. Integrins promote cell survival by inhibiting the JNK pathway. (A–C‴) Maximal projection of confocal views of third-instar wing imaginal discs carrying the reporter hidGFP, stained with anti-βPS [blue in (A–C), white in (A′–C′)], anti-Dcp1 [red in (A–C), white in (A′′–C′′)] and anti-GFP [green in (A–C), white in (A‴–C‴)]. (A–A‴) Control hidGFP; nubGal4 wing disc. (B–B‴) hidGFP wing disc expressing a mys RNAi under the control of nubGal4, hidGFP; nub > mysRNAi. (C–C‴) hidGFP wing disc co-expressing a mys RNAi and the negative regulator of the JNK pathway puc, under the control of nubGal4 hidGFP; nub > mysRNAi; puc. (D,E) Quantification of Dcp1 (D) and hidGFP (E) levels in wing discs of the indicated genotypes. The statistical significance of differences was assessed with a t-test, *** and * p values < 0.001, <0.01, and <0.05, respectively. NS, non-statistically significant. Scale bar in all panels, 30 μm.
The relationship between JNK signalling, pro-apoptotic proteins and caspase activation is context-dependent. Thus, the JNK pathway can act upstream or downstream of pro-apoptotic genes such as rpr or hid. For instance, experiments in Drosophila S2 cells suggested that Rpr could stimulate JNK activation through DIAP1 degradation (Kuranaga et al., 2002). In contrast, JNK signalling promotes hid expression (and apoptosis) in eye discs, and it is required for rpr-reporter induction in response to radiation in wing discs (Kuranaga et al., 2002; Moreno et al., 2002). In our system, inhibition of JNK signalling via puc overexpression was able to suppress hid upregulation in nub > mysRNAi wing discs to a large extent (n = 17, Figures 4C-C’’’,E). Altogether, these results allow us to conclude that reduction of integrin function in wing discs leads to apoptosis by stimulating hid transcription through JNK activation.
Integrins Cooperate With the EGFR Pathway to Promote Cell Survival
The EGFR activates a network of signaling pathways promoting survival in many different cellular contexts [reviewed in (Henson and Gibson, 2006)]. In addition, experiments mainly from cell culture have revealed synergistic cooperation between growth factors and integrins in cell survival (Reginato et al., 2003; Miranti and Brugge, 2002). For instance, EGFR promotes survival through regulation of the Ras/MAPK pathway and consequent inhibition of the proapoptotic gene hid in the eye disc (Bergmann et al., 1998; Kurada and White, 1998; Yu et al., 2002). Moreover, wing disc mutant clones for EGFR or for some of their dowmstream effectors, such as Ras, are smaller than their twin spot, suggesting a role for the EGFR pathway in cell survival (Diaz-Benjumea and Garcia-Bellido, 1990; Diaz-Benjumea and Hafen, 1994; Zecca and Struhl, 2002). To directly address a role for EGFR in cell survival in the wing disc, we expressed an EGFR dominant negative construct (UAS-DERDN) under the control of the nub-Gal4 line and found it led to increase cell death in the pouch region (nub > DERDN, n = 21, Figures 5A, A’, C, C’, E). However, in contrast to what happens in nub > mysRNAi wing discs (Figures 5B, B’, E), where cell death was found distributed all over the pouch, expression of DERDN restricted apoptosis to regions of high EGFR activity (Gabay et al., 1997), such as the wing margin and the presumptive vein territories (yellow arrow and asterisks in Figures 5A, A’, C, C, respectively). In addition, and contrary to what happens in the eye, EGFR did not seem to exert its pro-survival function through regulation of hid or rpr transcription, as hidGFP or rpr-lacZ expression were not altered in nub > DERDN wing discs (Figures 5A, A’, C, C”; Supplementary Figure S7). We thus conclude that the EGFR pathway has a limited role in the control of cell survival in the wing disc that is independent of the transcriptional regulation of the pro-apoptotic genes hid and rpr. Finally, we examined a possible cooperation between integrins and the EGFR pathway to mediate cell survival. We found that the simultaneous co-expression of mysRNAi and DERDN in wing discs (nub > mysRNAi; DERDN) enhanced the cell death phenotype caused by the expression of any of them on their own (Figures 5B–E), strongly suggesting that integrins and the EGFR pathway act in paralell to promote cell survival in wing imaginal discs.
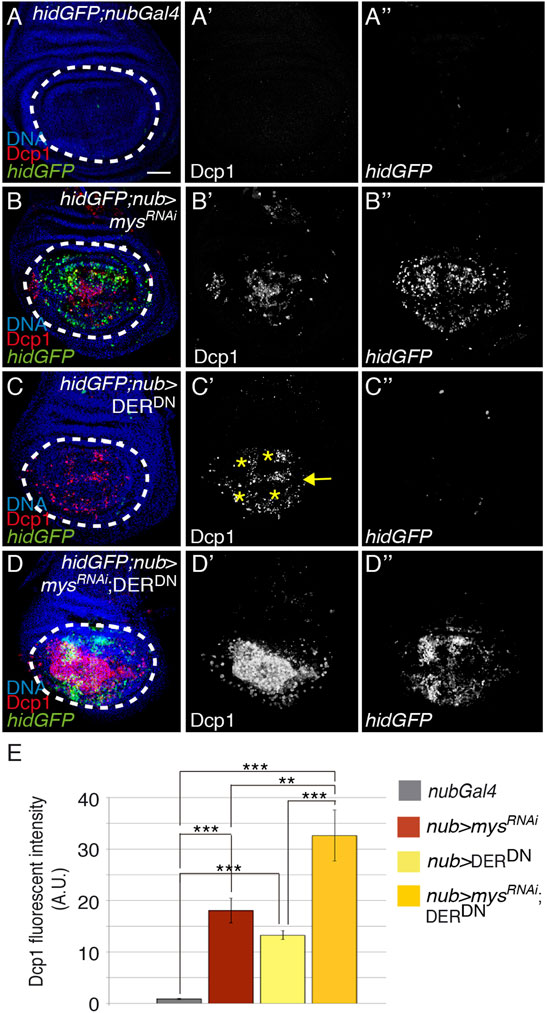
FIGURE 5. Integrins and EGFR cooperate to promote cell survival. (A–D”) Maximal projection of confocal views of wing imaginal discs from third-instar larvae carrying the reporter hidGFP, stained with anti-Dcp1 [red in (A–D), white in (A′–D′)], anti-GFP [green in (A–D), white in (A’’–D’’)] and the nuclear marker Hoechst [DNA, blue in (A–D)]. (A–D’’) Control hidGFP; nubGal4 wing disc. (B–B’’) hidGFP wing disc expressing a mys RNAi under the control of nubGal4, hidGFP;nub > mysRNAi. (C–C’’) hidGFP wing disc expressing a dominant negative form of the EGFR, DERDN, under the control of nubGal4, hidGFP; nub > DERDN. (C–D’’) hidGFP wing disc co-expressing a mys RNAi and DERDN under the control of nubGal4 hidGFP; nub > mysRNAi; DERDN. (E) Quantification of Dcp1 levels in wing discs of the indicated genotypes. The statistical significance of differences was assessed with a t-test, *** and ** p values < 0.001 and <0.01, respectively. NS, non-statistically significant. Scale bar in all panels, 30 μm.
Oncogenic Ras Suppresses Anoikis in Wing Discs
Suppression of anoikis after detachment of cancer cells from the ECM is a key step in tumor metastasis [reviewed in (Simpson et al., 2008)]. In fact, the ability of oncogenic Ras to suppress anoikis has long been considered critical to Ras transformation. However, recent evidence suggests that Ras and other oncogenes can induce both pro- and anti-apoptotic signals depending on the cell type and context [reviewed in (Cox and Der, 2003)]. In Drosophila, Ras overactivation renders cells refractory to stress- and irradiation-induced apoptosis (Bergmann et al., 1998; Kurada and White, 1998; Pinal et al., 2018). To test whether oncogenic Ras could protect cells from anoikis in the wing imaginal disc, we overexpressed an activated form of DRas, RasV12 (Karim and Rubin, 1998) and found it substantially suppressed the cell death phenotype caused by integrin depletion (nub > mysRNAi, rasV12; Figures 6A-A’’, 6B–B’’, C–C’’, D, n = 18). These results demonstrate that oncogenic Ras is able to suppress anoikis in wing imaginal discs. In epithelial cell lines, oncogenic Ras confers resistance to anoikis partly by downregulating the expression of proapoptotic Bcl-2 members (Rak et al., 1995) and by preventing downregulation of antiapoptotic proteins (Rosen et al., 2000). In Drosophila, it appears that the Ras pathway regulates Hid activity at the level of both, protein phosphorylation and gene expression, in hid-induced apoptosis (Bergmann et al., 1998; Kurada and White, 1998). However, we found that, in the Drosophila wing disc epithelium, the ability of oncogenic Ras to confer resistance to anoikis does not involve transcriptional regulation of hid, as hidGFP levels were not altered in nub > mysRNAi; RasV12 (n = 21, Figures 6A’’’, C’’’) discs compared to nub > mysRNAi (n = 20, Figure 6B’’’).
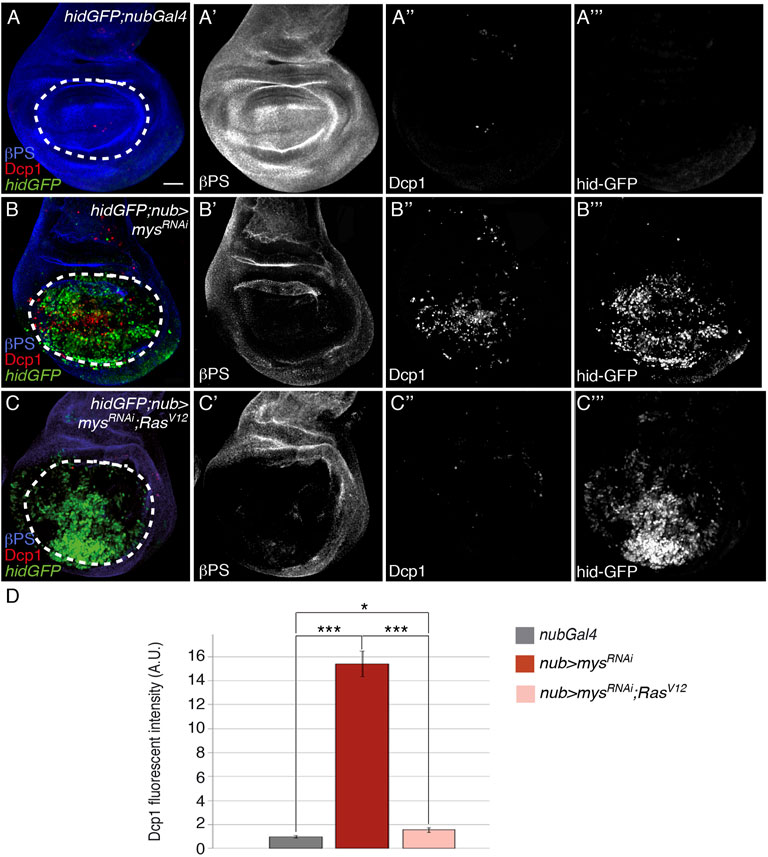
FIGURE 6. Ectopic activation of the Ras pathway rescues anoikis. (A–C’’’) Maximal projection of confocal views of third-instar wing imaginal discs carrying the reporter hidGFP, stained with anti-βPS [blue in (A–C), white in (A′–C′)], anti-Dcp1 [red in (A–C), white in (A’’–C’’)] and anti-GFP [green in (A–C), white in (A‴–C‴)]. (A–A‴) Control hidGFP;nubGal4 wing disc. (B–B‴) hidGFP wing disc expressing a mys RNAi under the control of nubGal4, hidGFP; nub > mysRNAi. (C–C‴) hidGFP wing disc co-expressing a mys RNAi and an activated form of Ras, RasV12, under the control of nubGal4 hidGFP; nub > mysRNAi; RasV12. (D) Quantification of Dcp1 levels in wing discs of the indicated genotypes. The statistical significance of differences was assessed with a t-test, *** and * p values < 0.001 and <0.05, respectively. Scale bar in all panels, 30 μm.
Ectopic expression of RasV12 in the dorsal compartment of wing imaginal discs, by means of the apterous Gal4 (ap) Gal4 line, causes tissue overgrowth and cell shape changes, which results in the formation of ectopic folds [ap > RasV12, n = 20, Figures 7A,A’,C,C’, (Prober and Edgar, 2000; Soler Beatty et al., 2021). Reducing integrin function also alters cell shape causing a mild folding phenotype (Dominguez-Gimenez et al., 2007), Figures 7B, B’ E, E’]. Here, we found that co-expression of mysRNAi and RasV12 enhanced the cell shape and folding phenotype caused by the sole expression of any of them (ap > mysRNAi; RasV12, n = 20, Figures 7D,D’).
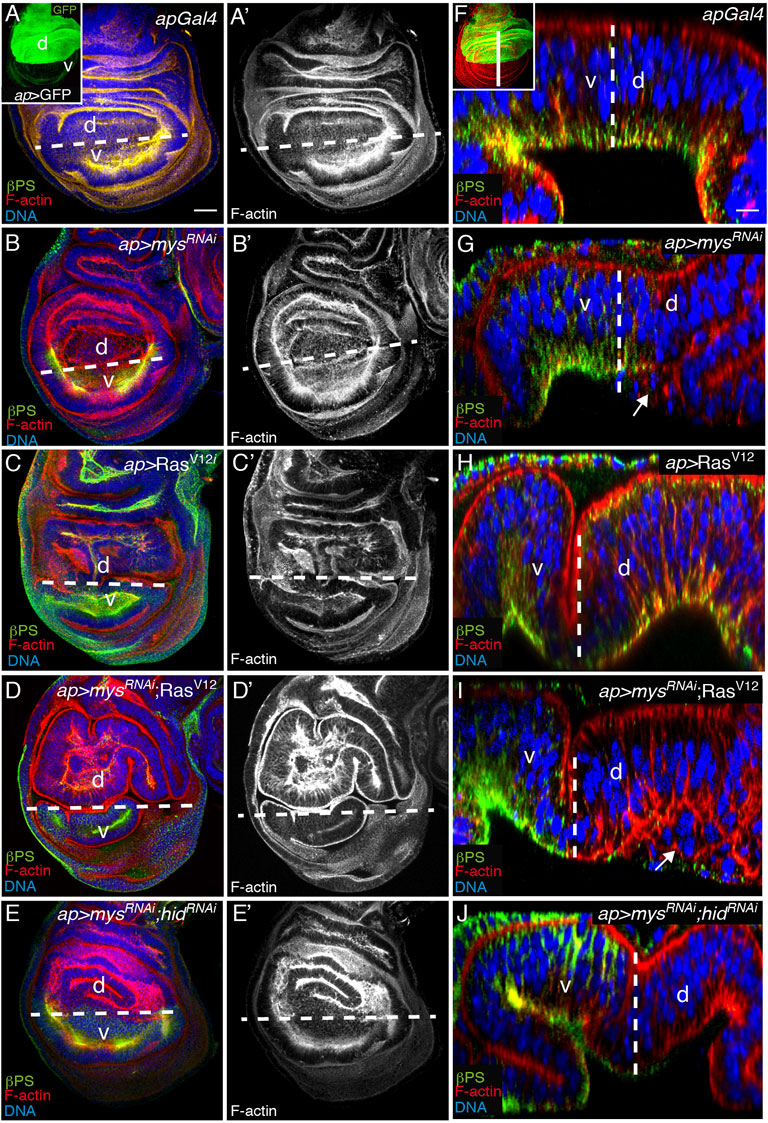
FIGURE 7. Integrin knockdown stimulates basal extrusion of RasV12 tumor cells. (A–J) Wing imaginal discs from third-instar larvae expressing the indicated UAS transgenes under the control of ap-Gal4 (inset in A), stained with anti-βPS [green in (A–E) and (F–J)], the nuclear marker Hoechst [DNA, blue in (A–E,F–J)] and Rhodamine Phalloidin to detect F-actin [red in (A–E) and (F–J) and white in (A′–E′)]. (A–E′) Maximal projection of confocal views of wing imaginal discs. (A,A′) Control apGal4 wing disc. (B,B′) Wing disc expressing a mys RNAi under the control of apGal4, ap > mysRNAi. (C,C′) Wing disc expressing RasV12 under the control of apGal4, ap > RasV12. (D,D′) Wing disc co-expressing mys RNAi and RasV12 under the control of apGal4, ap > mysRNAi; RasV12. (E–E′) Wing disc co-expressing RNAis against mys and hid under the control of apGal4, ap > mysRNAi; hidRNAi. (F–J) Confocal yz sections along the white straight line shown in inset in F of wing discs of the indicated genotypes. White dotted lines in all panels separate dorsal (d) from ventral (v) compartments. White arrows in G and I point to dead (G) and alive (I) cells extruded from the wing disc epithelium. Scale bar 30 μm (A–E′) and 10 μm (F–J).
In this work, we show that reducing integrin expression ensued basal extrusion of the dead cells (Figures 1C–C’’, Figures 7F,G). However, even though oncogenic K-Ras MCDK cells survive and extrude basally, a mechanism proposed to initiate invasion into surrounding tissues (Slattum et al., 2014), we found that expression of RasV12 on its own in wing disc cells (ap > RasV12) did not result in cell extrusion (Figure 7H). In striking contrast, the expression of RasV12 in integrin mutant cells not only allowed their survival (Figure 6) but also induced their basal extrusion beneath the surface of the wing disc epithelia (ap > mysRNAi; RasV12, n = 20, Figure 7I). Furthermore, because depletion of both integrins and hid (ap > mysRNAi; hidRNAi) did not result in cell extrusion (Figure 7J), the basal extrusion of mys; RasV12 wing disc cells was not due only to the ability of RasV12 to rescue apoptosis. From the above results we conclude that integrins prevent extrusion of transformed RasV12 cells.
Discussion
Epithelial cells monitor their environment looking for signals necessary for survival. Among those, attachment to the ECM is critical, as the dominant response of epithelial cells to anchorage loss is anoikis. However, at certain stages of development and during tissue repair epithelial cells survive in an unanchored state. Likewise, cellular transformation is accompanied by an inappropriate anoikis resistance, suggesting that anoikis can be modulated. Molecular mechanisms regulating anoikis have been described in several epithelial cell types reviewed in (Zhan et al., 2004). The relevance of these mechanisms in epithelial cells in a physiological setting is unclear, as most of these studies use immortalized or cancer cell lines. Here, we show that integrins act as survival factors during the development of the epithelial wing disc and that the EGFR/Ras pathway protects epithelial cells from anoikis both in developmental and tumorigenic contexts.
Studies using standard 2D cultures and 3D matrices have revealed a central role for cell-ECM interactions -mostly mediated by integrins- in survival (Stupack and Cheresh, 2002). However, there is still little evidence up to date for a role for integrins as survival factors during tissue morphogenesis. The use of conditional knockouts in mice has revealed that the function of β1 integrins in developmental cell survival is complex and cell type- and differentiation state- specific. Thus, while β1 integrins are required for the survival of cranial cells of the hyoid arch region (Goh et al., 1997), granular cells of the kidney (Mohamed et al., 2020), lens cells (Simirskii et al., 2007), endodermal cells (Carlson et al., 2008) or the epiblast (Mole et al., 2021), it is dispensable for the survival of intestinal epithelial cells (Jones et al., 2006; Benoit et al., 2010). These differences in the contribution of murine β1 integrins to cell survival could depend on the repertoire of integrins expressed in each cell type and on a possible redundancy among them. Hence, the use of simpler model organisms with a markedly smaller number of integrins in their genome should help determine the real role of integrins in cell survival during development. However, there is no clear evidence to date of a function for integrins in cell survival in worms or flies. Our work demonstrates for the first time that integrins are required for epithelial cell survival in wing imaginal discs. We also show that integrins prevent anoikis by blocking the expression of IAP protein antagonists such as Hid. This is in contrast to vertebrate integrins, which seem to control anoikis mainly by controlling the expression and/or activation of anti- and pro-apoptotic Bcl-2 family proteins, known regulators of mitochondrial outer membrane potential and permeability (Stupack and Cheresh, 2002; Scorrano and Korsmeyer, 2003). In fact, mitochondria play crucial roles in the modulation of the anoikis response in mammals [reviewed in (Zhan et al., 2004)]. Drosophila contains two Bcl-2 related proteins, Debcl and Buffy, and one homolog of the mammalian mitochondrial serin protease HtrA2/Omi, which act as pro- or anti-apoptotic factors in developmental programmed cell death and γ irradiation-induced apoptosis (Brachmann et al., 2000; Colussi et al., 2000; Igaki et al., 2000; Quinn et al., 2003). These studies suggest that the mitochondrial regulation of cell death machinery could be conserved in Drosophila (Challa et al., 2007; Igaki et al., 2007). Future experiments are required to test whether mitochondria play any role in the regulation of anoikis in Drosophila, as it is the case in mammals.
Integrins are non-kinase receptors. Consequently, they require kinases to enact signaling pathways blocking apoptosis. The JNK pathway is among the earliest pathways activated upon detachment from the ECM in several systems [reviewed in (Liu and Lin, 2005)]. Yet, the role of JNK in anoikis is controversial, as it can be essential (Frisch et al., 1996) but also dispensable (Khwaja and Downward, 1997). In Drosophila, JNK signalling correlates with amnioserosa cell death during embryonic dorsal closure (Reed et al., 2004). As the amnioserosa undergoes premature disintegration in a proportion of integrin mutant embryos, it has been hypothesized that integrins block JNK-dependent anoikis in amnioserosa cells. However, a definitive proof of integrin depletion-dependent anoikis is still lacking. Our results show that JNK signaling is activated and required for anoikis in wing disc epithelial cells. In mammary epithelial cells, JNK stimulate anoikis by regulating the expression or activity of the mitochondrial regulator proteins Bcl-2 (Lei and Davis, 2003; Hubner et al., 2008; Girnius and Davis, 2017). In contrast, here, we find that JNK induces anoikis by promoting the expression of Hid in the Drosophila wing imaginal disc. The pro-apoptotic role of JNK by modulating the expression of antagonists of IAP proteins is not exclusive to anoikis, as it also occurs in response to radiation in wing discs (Pinal et al., 2018) and to loss of apical determinants in the embryonic epidermis (Kolahgar et al., 2011). A role for the Drosophila JNK pathway on inducing any type of cell death through mitochondrial regulation awaits further investigations.
Extracellular growth factors are required for the survival of most animal cells. Growth factors regulate cell survival by stimulating the antiapoptotic activity of the EGFR/Ras pathway. In the Drosophila embryo, induction of Ras leads to downregulation of hid mRNA levels (Kurada and White, 1998). In the eye disc, ectopic expression of either an inhibitor of the EGFR or a dominant negative form of the receptor results in apoptosis (Freeman, 1996; Sawamoto et al., 1998). Here, we show that the activity of the EGFR is required in the wing disc epithelium for the survival of cells exhibiting high activity of the pathway, such as the wing margin and future vein cells. During morphogenesis, epithelial cells need to detach from the ECM to allow changes in cell and tissue shape. In this context, epithelial cells are required to survive in an unanchored state. This is the case of the wing margin cells, which, at the onset of pupariation, detach from the basement membrane and shorten to drive bending of the wing pouch and formation of the two bi-layer adult wing (Fristrom and Fristrom, 1993). Similarly, vein formation also involves changes in cell shape and adhesion to the underlying basement membrane (De Celis, 2003). We propose that the EGFR pathway protects vein and wing margin cells from anoikis during wing morphogenesis (Figure 8).
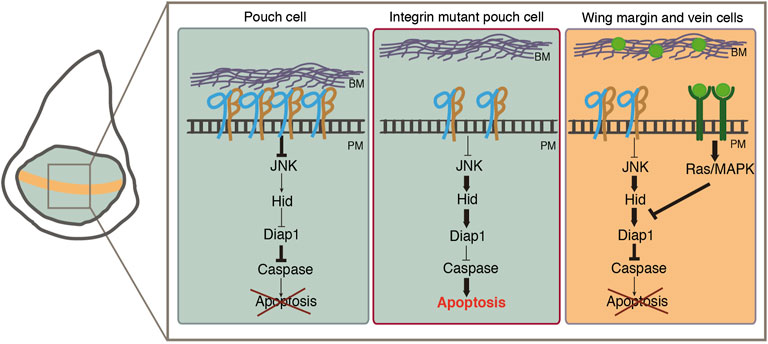
FIGURE 8. Model showing how integrins and the EGFR pathway act together to promote cell survival in wing imaginal disc cells. On the left, schematic representation of a wing disc with the wing pouch colored in green and the wing margin in orange. On the right, proposed molecular mechanism by which integrins regulate cell survival. (Left rectangle) In pouch wing imaginal disc cells, integrin-mediated adhesion to the ECM regulates cell survival by repressing the expression of the pro-apoptotic gene hid via inhibition of JNK activity. (Middle rectangle) In the absence of integrins JNK activity levels increase leading to high levels of Hid expression, caspase activation and apoptosis. (Right rectangle) The EGFR (green) pathway prevents the death of wing margin and vein cells when they detach from the ECM during wing morphogenesis.
Oncogenic Ras has been shown to suppress caspases function in several systems (Cox and Der, 2003). In the Drosophila eye disc, oncogenic Ras blocks hid-induced apoptosis by inhibiting Hid phosphorylation (Bergmann et al., 1998). Here, we show that oncogenic Ras rescues loss of integrin-dependent cell death in the wing disc without decreasing hid transcription levels. These results suggest that the mechanisms by which oncogenic Ras confers resistance to cell death in Drosophila might be cell and stimuli specific. One prominent feature of malignant cells is the presence of altered cell shape and cytoskeleton, which contributes to increase resistance to apoptosis and neoplastic growth (Martin and Leder, 2001). Our results show that, besides blocking cell death, expression of RasV12 enhances the cell shape phenotype due to integrin donwregulation. In the future, it will be interesting to analyse the contribution of aberrant cell shape to anoikis resistance in transformed epithelial wing disc cells.
Finally, cancer cells can spread by extrusion-initiated programs that couple anoikis resistance with cell extrusion. Furthermore, basolateral extrusion is generally thought to promote spread and invasion of tumor cells and, eventually, formation of metastatic lesions (Jiang et al., 2015). However, the mechanisms controlling basal extrusion of tumor cells are still unclear. Here, we show that elimination of integrin function results on basal extrusion of RasV12 cells. We can now use the advantages of the Drosophila model system to increase our understanding of the mechanisms by which downregulation of integrin function induces basal extrusion of cancer cells.
In summary, our results reveal that integrins act as survival factors in the wing disc epithelium. We also demonstrate that integrins cooperate with the EGFR pathway to prevent anoikis during wing disc morphogenesis (Figure 8). Finally, we show that oncogenic activation of the Ras pathway results in anoikis resistance and basal cell extrusion. The identification of the molecular and cellular mechanisms by which integrins act as survival factors will help us to understand not only morphogenesis but also cancer progression.
Data Availability Statement
The raw data supporting the conclusion of this article will be made available by the authors, without undue reservation.
Author Contributions
AV-E, MG-L, TW, and MM-B performed experiments and analyzed data. AV-E designed research and contributed to the writing of the manuscript. MM-B provided financial support, designed research, analyzed data and wrote the manuscript.
Funding
This work was funded by project grants from the Ministerio Español de Ciencia, Innovación y Universidades (MICINN, https://www.ciencia.gob.es/) awarded to MDMB (Nos. BFU2016-80797-R and PID2019-109013GB-100) and from the Junta de Andalucía (Proyecto de Excelencia). AV-E was supported by the PID2019-109013GB-100. We are also grateful to the MdM Unit of Excellence GEM (http://cellcollectives.com/) and to Junta de Andalucía for their continuous funding and support. The funders had no role in study design, data collection and analysis, decision to publish or preparation of the manuscript.
Conflict of Interest
The authors declare that the research was conducted in the absence of any commercial or financial relationships that could be construed as a potential conflict of interest.
Publisher’s Note
All claims expressed in this article are solely those of the authors and do not necessarily represent those of their affiliated organizations, or those of the publisher, the editors and the reviewers. Any product that may be evaluated in this article, or claim that may be made by its manufacturer, is not guaranteed or endorsed by the publisher.
Acknowledgments
We thank Bloomington and VDRC Stock Centers and the Drosophila community for fly stocks and reagents. We also thank Prof. A. González for critical reading of the manuscript.
Supplementary Material
The Supplementary Material for this article can be found online at: https://www.frontiersin.org/articles/10.3389/fcell.2022.892691/full#supplementary-material
References
Adachi-Yamada, T., Fujimura-Kamada, K., Nishida, Y., and Matsumoto, K. (1999). Distortion of Proximodistal Information Causes JNK-dependent Apoptosis in Drosophila Wing. Nature 400, 166–169. doi:10.1038/22112
Adams, J. C., and Watt, F. M. (1993). Regulation of Development and Differentiation by the Extracellular Matrix. Development 117, 1183–1198. doi:10.1242/dev.117.4.1183
Benoit, Y. D., Larrivée, J.-F., Groulx, J.-F., Stankova, J., Vachon, P. H., and Beaulieu, J.-F. (2010). Integrin α8β1 Confers Anoikis Susceptibility to Human Intestinal Epithelial Crypt Cells. Biochem. Biophysical Res. Commun. 399, 434–439. doi:10.1016/j.bbrc.2010.07.107
Bergantiños, C., Corominas, M., and Serras, F. (2010). Cell Death-Induced Regeneration in Wing Imaginal Discs Requires JNK Signalling. Development 137, 1169–1179. doi:10.1242/dev.045559
Bergmann, A., Agapite, J., Mccall, K., and Steller, H. (1998). The Drosophila Gene Hid Is a Direct Molecular Target of Ras-dependent Survival Signaling. Cell 95, 331–341. doi:10.1016/s0092-8674(00)81765-1
Brabant, M. C., Fristrom, D., Bunch, T. A., and Brower, D. L. (1996). Distinct Spatial and Temporal Functions for PS Integrins during Drosophila Wing Morphogenesis. Development 122, 3307–3317. doi:10.1242/dev.122.10.3307
Brachmann, C. B., Jassim, O. W., Wachsmuth, B. D., and Cagan, R. L. (2000). The Drosophila Bcl-2 Family Member dBorg-1 Functions in the Apoptotic Response to UV-Irradiation. Curr. Biol. 10, 547–550. doi:10.1016/s0960-9822(00)00474-7
Brand, A. H., and Perrimon, N. (1993). Targeted Gene Expression as a Means of Altering Cell Fates and Generating Dominant Phenotypes. Development 118, 401–415. doi:10.1242/dev.118.2.401
Brown, N. H. (1993). Integrins holdDrosophila Together. BioEssays 15, 383–390. doi:10.1002/bies.950150604
Bunch, T. A., Salatino, R., Engelsgjerd, M. C., Mukai, L., West, R. F., and Brower, D. L. (1992). Characterization of Mutant Alleles of Myospheroid, the Gene Encoding the Beta Subunit of the Drosophila PS Integrins. Genetics 132, 519–528. doi:10.1093/genetics/132.2.519
Calleja, M., Moreno, E., Pelaz, S., and Morata, G. (1996). Visualization of Gene Expression in Living Adult Drosophila. Science 274, 252–255. doi:10.1126/science.274.5285.252
Carlson, T. R., Hu, H., Braren, R., Kim, Y. H., and Wang, R. A. (2008). Cell-autonomous Requirement for β1 Integrin in Endothelial Cell Adhesion, Migration and Survival during Angiogenesis in Mice. Development 135, 2193–2202. doi:10.1242/dev.016378
Challa, M., Malladi, S., Pellock, B. J., Dresnek, D., Varadarajan, S., Yin, Y. W., et al. (2007). Drosophila Omi, a Mitochondrial-Localized IAP Antagonist and Proapoptotic Serine Protease. EMBO J. 26, 3144–3156. doi:10.1038/sj.emboj.7601745
Chiarugi, P., and Giannoni, E. (2008). Anoikis: a Necessary Death Program for Anchorage-dependent Cells. Biochem. Pharmacol. 76, 1352–1364. doi:10.1016/j.bcp.2008.07.023
Chou, T. B., and Perrimon, N. (1992). Use of a Yeast Site-specific Recombinase to Produce Female Germline Chimeras in Drosophila. Genetics 131, 643–653. doi:10.1093/genetics/131.3.643
Colussi, P. A., Quinn, L. M., Huang, D. C. S., Coombe, M., Read, S. H., Richardson, H., et al. (2000). Debcl, a Proapoptotic Bcl-2 Homologue, Is a Component of the Drosophila melanogaster Cell Death Machinery. J. Cell Biol. 148, 703–714. doi:10.1083/jcb.148.4.703
Cooper, D. M., Granville, D. J., and Lowenberger, C. (2009). The Insect Caspases. Apoptosis 14, 247–256.
Cox, A. D., and Der, C. J. (2003). The Dark Side of Ras: Regulation of Apoptosis. Oncogene 22, 8999–9006. doi:10.1038/sj.onc.1207111
De Celis, J. F. (2003). Pattern Formation in theDrosophila Wing: The Development of the Veins. Bioessays 25, 443–451. doi:10.1002/bies.10258
Diaz-Benjumea, F. J., and Garcia-Bellido, A. (1990). Behaviour of Cells Mutant for an EGF Receptor Homologue of Drosophila in Genetic Mosaics. Proc. Biol. Sci. 242, 36–44.
Dipersio, C. M., Van Der Neut, R., Georges-Labouesse, E., Kreidberg, J. A., Sonnenberg, A., and Hynes, R. O. (2000). alpha3beta1 and Alpha6beta4 Integrin Receptors for Laminin-5 Are Not Essential for Epidermal Morphogenesis and Homeostasis during Skin Development. J. Cell Sci. 113 (Pt 17), 3051–3062. doi:10.1242/jcs.113.17.3051
Domínguez-Giménez, P., Brown, N. H., and Martín-Bermudo, M. D. (2007). Integrin-ECM Interactions Regulate the Changes in Cell Shape Driving the Morphogenesis of the Drosophila Wing Epithelium. J. Cell Sci. 120, 1061–1071. doi:10.1242/jcs.03404
Duffy, J. B., Harrison, D. A., and Perrimon, N. (1998). Identifying Loci Required for Follicular Patterning Using Directed Mosaics. Developmen 125, 2263–2271. doi:10.1242/dev.125.12.2263
Freeman, M. (1996). Reiterative Use of the EGF Receptor Triggers Differentiation of All Cell Types in the Drosophila Eye. Cell 87, 651–660. doi:10.1016/s0092-8674(00)81385-9
Frisch, S., and Francis, H. (1994). Disruption of Epithelial Cell-Matrix Interactions Induces Apoptosis. J. Cell Biol. 124, 619–626. doi:10.1083/jcb.124.4.619
Frisch, S. M., and Ruoslahti, E. (1997). Integrins and Anoikis. Curr. Opin. Cell Biol. 9, 701–706. doi:10.1016/s0955-0674(97)80124-x
Frisch, S. M., and Screaton, R. A. (2001). Anoikis Mechanisms. Curr. Opin. Cell Biol. 13, 555–562. doi:10.1016/s0955-0674(00)00251-9
Frisch, S. M., Vuori, K., Kelaita, D., and Sicks, S. (1996). A Role for Jun-N-Terminal Kinase in Anoikis; Suppression by Bcl-2 and crmA. J. Cell Biol. 135, 1377–1382. doi:10.1083/jcb.135.5.1377
Fristrom, D., and Fristrom, J. (1993). “The Metamorphic Development of the Adult Epidermis,” in The Development of Drosophila. Editors A. Martinez-Arias, and M. Bates (Cold Spring Harbor, New York: Cold Spring Harbor Press), 843–897.
Fristrom, D., Wilcox, M., and Fristrom, J. (1993). The Distribution of PS Integrins, Laminin A and F-Actin during Key Stages in Drosophila Wing Development. Development 117, 509–523. doi:10.1242/dev.117.2.509
Gabay, L., Seger, R., and Shilo, B.-Z. (1997). In Situ activation Pattern of Drosophila EGF Receptor Pathway during Development. Science 277, 1103–1106. doi:10.1126/science.277.5329.1103
Garcia-Bellido, A., and Merriam, J. R. (1971). Parameters of the Wing Imaginal Disc Development ofDrosophila Melanogaster. Dev. Biol. 24, 61–87. doi:10.1016/0012-1606(71)90047-9
Giancotti, F. G. (2000). Complexity and Specificity of Integrin Signalling. Nat. Cell Biol. 2, E13–E14. doi:10.1038/71397
Gilcrease, M. Z. (2007). Integrin Signaling in Epithelial Cells. Cancer Lett. 247, 1–25. doi:10.1016/j.canlet.2006.03.031
Gilmore, A. P. (2005). Anoikis. Cell Death Differ. 12 (Suppl. 2), 1473–1477. doi:10.1038/sj.cdd.4401723
Girnius, N., and Davis, R. J. (2017). JNK Promotes Epithelial Cell Anoikis by Transcriptional and Post-translational Regulation of BH3-Only Proteins. Cell Rep. 21, 1910–1921. doi:10.1016/j.celrep.2017.10.067
Goh, K. L., Yang, J. T., and Hynes, R. O. (1997). Mesodermal Defects and Cranial Neural Crest Apoptosis in Alpha5 Integrin-Null Embryos. Development 124, 4309–4319. doi:10.1242/dev.124.21.4309
Goyal, L., Mccall, K., Agapite, J., Hartwieg, E., and Steller, H. (2000). Induction of Apoptosis by Drosophila Reaper, Hid and Grim through Inhibition of IAP Function. EMBO J. 19, 589–597. doi:10.1093/emboj/19.4.589
Guadamillas, M. C., Cerezo, A., and Del Pozo, M. A. (2011). Overcoming Anoikis - Pathways to Anchorage-independent Growth in Cancer. J. Cell Sci. 124, 3189–3197. doi:10.1242/jcs.072165
Hay, B. A. (2000). Understanding IAP Function and Regulation: a View from Drosophila. Cell Death Differ. 7, 1045–1056. doi:10.1038/sj.cdd.4400765
Henson, E. S., and Gibson, S. B. (2006). Surviving Cell Death through Epidermal Growth Factor (EGF) Signal Transduction Pathways: Implications for Cancer Therapy. Cell. Signal. 18, 2089–2097. doi:10.1016/j.cellsig.2006.05.015
Hübner, A., Barrett, T., Flavell, R. A., and Davis, R. J. (2008). Multisite Phosphorylation Regulates Bim Stability and Apoptotic Activity. Mol. Cell 30, 415–425. doi:10.1016/j.molcel.2008.03.025
Hynes, R. O. (1992). Integrins: Versatility, Modulation, and Signaling in Cell Adhesion. Cell 69, 11–25. doi:10.1016/0092-8674(92)90115-s
Igaki, T., Kanda, H., Yamamoto-Goto, Y., Kanuka, H., Kuranaga, E., Aigaki, T., et al. (2002). Eiger, a TNF Superfamily Ligand that Triggers the Drosophila JNK Pathway. EMBO J. 21, 3009–3018. doi:10.1093/emboj/cdf306
Igaki, T., Kanuka, H., Inohara, N., Sawamoto, K., Núñez, G., Okano, H., et al. (2000). Drob-1, a Drosophila Member of the Bcl-2/CED-9 Family that Promotes Cell Death. Proc. Natl. Acad. Sci. U.S.A. 97, 662–667. doi:10.1073/pnas.97.2.662
Igaki, T., Suzuki, Y., Tokushige, N., Aonuma, H., Takahashi, R., and Miura, M. (2007). Evolution of Mitochondrial Cell Death Pathway: Proapoptotic Role of HtrA2/Omi in Drosophila. Biochem. Biophysical Res. Commun. 356, 993–997. doi:10.1016/j.bbrc.2007.03.079
Ignotz, R., and Massague, J. (1987). Cell Adhesion Protein Receptors as Targets for Transforming Growth Factor-$beta; Action. Cell 51, 189–197. doi:10.1016/0092-8674(87)90146-2
Jiang, W. G., Sanders, A. J., Katoh, M., Ungefroren, H., Gieseler, F., Prince, M., et al. (2015). Tissue Invasion and Metastasis: Molecular, Biological and Clinical Perspectives. Seminars Cancer Biol. 35 (Suppl. l), S244–S275. doi:10.1016/j.semcancer.2015.03.008
Jones, R. G., Li, X., Gray, P. D., Kuang, J., Clayton, F., Samowitz, W. S., et al. (2006). Conditional Deletion of β1 Integrins in the Intestinal Epithelium Causes a Loss of Hedgehog Expression, Intestinal Hyperplasia, and Early Postnatal Lethality. J. Cell Biol. 175, 505–514. doi:10.1083/jcb.200602160
Karim, F. D., and Rubin, G. M. (1998). Ectopic Expression of Activated Ras1 Induces Hyperplastic Growth and Increased Cell Death in Drosophila Imaginal Tissues. Development 125, 1–9. doi:10.1242/dev.125.1.1
Khwaja, A., and Downward, J. (1997). Lack of Correlation between Activation of Jun-NH2-terminal Kinase and Induction of Apoptosis after Detachment of Epithelial Cells. J. Cell Biol. 139, 1017–1023. doi:10.1083/jcb.139.4.1017
Kolahgar, G., Bardet, P.-L., Langton, P. F., Alexandre, C., and Vincent, J.-P. (2011). Apical Deficiency Triggers JNK-dependent Apoptosis in the Embryonic Epidermis of Drosophila. Development 138, 3021–3031. doi:10.1242/dev.059980
Kurada, P., and White, K. (1998). Ras Promotes Cell Survival in Drosophila by Downregulating Hid Expression. Cell 95, 319–329. doi:10.1016/s0092-8674(00)81764-x
Kuranaga, E., Kanuka, H., Igaki, T., Sawamoto, K., Ichijo, H., Okano, H., et al. (2002). Reaper-mediated Inhibition of DIAP1-Induced DTRAF1 Degradation Results in Activation of JNK in Drosophila. Nat. Cell Biol. 4, 705–710. doi:10.1038/ncb842
Lee, T., Feig, L., and Montell, D. J. (1996). Two Distinct Roles for Ras in a Developmentally Regulated Cell Migration. Development 122, 409–418. doi:10.1242/dev.122.2.409
Lei, K., and Davis, R. J. (2003). JNK Phosphorylation of Bim-Related Members of the Bcl2 Family Induces Bax-dependent Apoptosis. Proc. Natl. Acad. Sci. U.S.A. 100, 2432–2437. doi:10.1073/pnas.0438011100
Liu, J., and Lin, A. (2005). Role of JNK Activation in Apoptosis: a Double-Edged Sword. Cell Res. 15, 36–42. doi:10.1038/sj.cr.7290262
Luo, X., Puig, O., Hyun, J., Bohmann, D., and Jasper, H. (2007). Foxo and Fos Regulate the Decision between Cell Death and Survival in Response to UV Irradiation. EMBO J. 26, 380–390. doi:10.1038/sj.emboj.7601484
Martin, S. S., and Leder, P. (2001). Human MCF10A Mammary Epithelial Cells Undergo Apoptosis Following Actin Depolymerization that Is Independent of Attachment and Rescued by Bcl-2. Mol. Cell Biol. 21, 6529–6536. doi:10.1128/mcb.21.19.6529-6536.2001
Martin, S. S., and Vuori, K. (2004). Regulation of Bcl-2 Proteins during Anoikis and Amorphosis. Biochimica Biophysica Acta (BBA) - Mol. Cell Res. 1692, 145–157. doi:10.1016/j.bbamcr.2004.02.008
Martin-Bermudo, M. D., and Brown, N. H. (1999). Uncoupling Integrin Adhesion and Signaling: the Beta PS Cytoplasmic Domain Is Sufficient to Regulate Gene Expression in the Drosophila Embryo. Genes & Dev. 13, 729–739. doi:10.1101/gad.13.6.729
Meredith, J. E., Fazeli, B., and Schwartz, M. A. (1993). The Extracellular Matrix as a Cell Survival Factor. MBoC 4, 953–961. doi:10.1091/mbc.4.9.953
Milán, M., Campuzano, S., and García-Bellido, A. (1997). Developmental Parameters of Cell Death in the Wing Disc of Drosophila. Proc. Natl. Acad. Sci. U.S.A. 94, 5691–5696. doi:10.1073/pnas.94.11.5691
Miranti, C. K., and Brugge, J. S. (2002). Sensing the Environment: a Historical Perspective on Integrin Signal Transduction. Nat. Cell Biol. 4, E83–E90. doi:10.1038/ncb0402-e83
Mohamed, T. H., Watanabe, H., Kaur, R., Belyea, B. C., Walker, P. D., Gomez, R. A., et al. (2020). Renin-Expressing Cells Require β1-Integrin for Survival and for Development and Maintenance of the Renal Vasculature. Hypertension 76, 458–467. doi:10.1161/hypertensionaha.120.14959
Molè, M. A., Weberling, A., Fässler, R., Campbell, A., Fishel, S., and Zernicka-Goetz, M. (2021). Integrin β1 Coordinates Survival and Morphogenesis of the Embryonic Lineage upon Implantation and Pluripotency Transition. Cell Rep. 34, 108834. doi:10.1016/j.celrep.2021.108834
Moreno, E., Yan, M., and Basler, K. (2002). Evolution of TNF Signaling Mechanisms. Curr. Biol. 12, 1263–1268. doi:10.1016/s0960-9822(02)00954-5
Nordstrom, W., Chen, P., Steller, H., and Abrams, J. M. (1996). Activation of thereaperGene during Ectopic Cell Killing inDrosophila. Dev. Biol. 180, 213–226. doi:10.1006/dbio.1996.0296
Paoli, P., Giannoni, E., and Chiarugi, P. (2013). Anoikis Molecular Pathways and its Role in Cancer Progression. Biochimica Biophysica Acta (BBA) - Mol. Cell Res. 1833, 3481–3498. doi:10.1016/j.bbamcr.2013.06.026
Parsons, J. T. (2003). Focal Adhesion Kinase: the First Ten Years. J. Cell Sci. 116, 1409–1416. doi:10.1242/jcs.00373
Pinal, N., Martín, M., Medina, I., and Morata, G. (2018). Short-term Activation of the Jun N-Terminal Kinase Pathway in Apoptosis-Deficient Cells of Drosophila Induces Tumorigenesis. Nat. Commun. 9, 1541. doi:10.1038/s41467-018-04000-6
Prober, D. A., and Edgar, B. A. (2000). Ras1 Promotes Cellular Growth in the Drosophila Wing. Cell 100, 435–446. doi:10.1016/s0092-8674(00)80679-0
Quinn, L., Coombe, M., Mills, K., Daish, T., Colussi, P., Kumar, S., et al. (2003). Buffy, a Drosophila Bcl-2 Protein, Has Anti-apoptotic and Cell Cycle Inhibitory Functions. EMBO J. 22, 3568–3579. doi:10.1093/emboj/cdg355
Rak, J., Mitsuhashi, Y., Erdos, V., Huang, S. N., Filmus, J., and Kerbel, R. S. (1995). Massive Programmed Cell Death in Intestinal Epithelial Cells Induced by Three-Dimensional Growth Conditions: Suppression by Mutant C-H-Ras Oncogene Expression. J. Cell Biol. 131, 1587–1598. doi:10.1083/jcb.131.6.1587
Reed, B. H., Wilk, R., Schöck, F., and Lipshitz, H. D. (2004). Integrin-dependent Apposition of Drosophila Extraembryonic Membranes Promotes Morphogenesis and Prevents Anoikis. Curr. Biol. 14, 372–380. doi:10.1016/j.cub.2004.02.029
Reginato, M. J., Mills, K. R., Paulus, J. K., Lynch, D. K., Sgroi, D. C., Debnath, J., et al. (2003). Integrins and EGFR Coordinately Regulate the Pro-apoptotic Protein Bim to Prevent Anoikis. Nat. Cell Biol. 5, 733–740. doi:10.1038/ncb1026
Rosen, K., Rak, J., Leung, T., Dean, N. M., Kerbel, R. S., and Filmus, J. (2000). Activated Ras Prevents Downregulation of Bcl-XL Triggered by Detachment from the Extracellular Matrix. J. Cell Biol. 149, 447–456. doi:10.1083/jcb.149.2.447
Rosenblatt, J., Raff, M. C., and Cramer, L. P. (2001). An Epithelial Cell Destined for Apoptosis Signals its Neighbors to Extrude it by an Actin- and Myosin-dependent Mechanism. Curr. Biol. 11, 1847–1857. doi:10.1016/s0960-9822(01)00587-5
Sawamoto, K., Taguchi, A., Hirota, Y., Yamada, C., Jin, M.-h., and Okano, H. (1998). Erratum: Argos Induces Programmed Cell Death in the Developing Drosophila Eye by Inhibition of the Ras Pathway. Cell Death Differ. 5, 548. doi:10.1038/sj.cdd.4400398
Scorrano, L., and Korsmeyer, S. J. (2003). Mechanisms of Cytochrome C Release by Proapoptotic BCL-2 Family Members. Biochem. Biophysical Res. Commun. 304, 437–444. doi:10.1016/s0006-291x(03)00615-6
Shlevkov, E., and Morata, G. (2012). A dp53/JNK-Dependant Feedback Amplification Loop Is Essential for the Apoptotic Response to Stress in Drosophila. Cell Death Differ. 19, 451–460. doi:10.1038/cdd.2011.113
Simirskii, V. N., Wang, Y., and Duncan, M. K. (2007). Conditional Deletion of β1-integrin from the Developing Lens Leads to Loss of the Lens Epithelial Phenotype. Dev. Biol. 306, 658–668. doi:10.1016/j.ydbio.2007.04.004
Simpson, C. D., Anyiwe, K., and Schimmer, A. D. (2008). Anoikis Resistance and Tumor Metastasis. Cancer Lett. 272, 177–185. doi:10.1016/j.canlet.2008.05.029
Slattum, G., Gu, Y., Sabbadini, R., and Rosenblatt, J. (2014). Autophagy in Oncogenic K-Ras Promotes Basal Extrusion of Epithelial Cells by Degrading S1P. Curr. Biol. 24, 19–28. doi:10.1016/j.cub.2013.11.029
Soler Beatty, J., Molnar, C., Luque, C. M., De Celis, J. F., and Martín-Bermudo, M. D. (2021). EGFRAP Encodes a New Negative Regulator of the EGFR Acting in Both Normal and Oncogenic EGFR/Ras-driven Tissue Morphogenesis. PLoS Genet. 17, e1009738. doi:10.1371/journal.pgen.1009738
Stephens, L. E., Sutherland, A. E., Klimanskaya, I. V., Andrieux, A., Meneses, J., Pedersen, R. A., et al. (1995). Deletion of Beta 1 Integrins in Mice Results in Inner Cell Mass Failure and Peri-Implantation Lethality. Genes Dev. 9, 1883–1895. doi:10.1101/gad.9.15.1883
Stupack, D. G., and Cheresh, D. A. (2002). Get a Ligand, Get a Life: Integrins, Signaling and Cell Survival. J. Cell Sci. 115, 3729–3738. doi:10.1242/jcs.00071
Tanaka-Matakatsu, M., Xu, J., Cheng, L., and Du, W. (2009). Regulation of Apoptosis of Rbf Mutant Cells during Drosophila Development. Dev. Biol. 326, 347–356. doi:10.1016/j.ydbio.2008.11.035
Vachon, P. H. (20112011). Integrin Signaling, Cell Survival, and Anoikis: Distinctions, Differences, and Differentiation. J. Signal Transduct. 2011, 738137. doi:10.1155/2011/738137
Wang, S. L., Hawkins, C. J., Yoo, S. J., Müller, H.-A. J., and Hay, B. A. (1999). The Drosophila Caspase Inhibitor DIAP1 Is Essential for Cell Survival and Is Negatively Regulated by HID. Cell 98, 453–463. doi:10.1016/s0092-8674(00)81974-1
Xu, D., Woodfield, S. E., Lee, T. V., Fan, Y., Antonio, C., and Bergmann, A. (2009). Genetic Control of Programmed Cell Death (Apoptosis) in Drosophila. Fly 3, 78–90. doi:10.4161/fly.3.1.7800
Yee, G. H., and Hynes, R. O. (1993). A Novel, Tissue-specific Integrin Subunit, Beta Nu, Expressed in the Midgut of Drosophila melanogaster. Development 118, 845–858. doi:10.1242/dev.118.3.845
Yoo, S. J., Huh, J. R., Muro, I., Yu, H., Wang, L., Wang, S. L., et al. (2002). Hid, Rpr and Grim Negatively Regulate DIAP1 Levels through Distinct Mechanisms. Nat. Cell Biol. 4, 416–424. doi:10.1038/ncb793
Yu, S.-Y., Yoo, S. J., Yang, L., Zapata, C., Srinivasan, A., Hay, B. A., et al. (2002). A Pathway of Signals Regulating Effector and Initiator Caspases in the Developing Drosophila Eye. Development 129, 3269–3278. doi:10.1242/dev.129.13.3269
Zecca, M., and Struhl, G. (2002). Subdivision of the Drosophila Wing Imaginal Disc by EGFR-Mediated Signaling. Development 129, 1357–1368.
Zhan, M., Zhao, H., and Han, Z. C. (2004). Signalling Mechanisms of Anoikis. Histol. Histopathol. 19, 973–983. doi:10.14670/HH-19.973
Keywords: integrins, survival, JNK, EGFR, oncogenesis
Citation: Valencia-Expósito A, Gómez-Lamarca MJ, Widmann TJ and Martín-Bermudo MD (2022) Integrins Cooperate With the EGFR/Ras Pathway to Preserve Epithelia Survival and Architecture in Development and Oncogenesis. Front. Cell Dev. Biol. 10:892691. doi: 10.3389/fcell.2022.892691
Received: 09 March 2022; Accepted: 09 May 2022;
Published: 13 June 2022.
Edited by:
Claudia Tanja Mierke, Leipzig University, GermanyReviewed by:
Susan LaFlamme, Albany Medical College, United StatesDan Bergstralh, University of Rochester, United States
Copyright © 2022 Valencia-Expósito, Gómez-Lamarca, Widmann and Martín-Bermudo. This is an open-access article distributed under the terms of the Creative Commons Attribution License (CC BY). The use, distribution or reproduction in other forums is permitted, provided the original author(s) and the copyright owner(s) are credited and that the original publication in this journal is cited, in accordance with accepted academic practice. No use, distribution or reproduction is permitted which does not comply with these terms.
*Correspondence: María D. Martín-Bermudo, bWRtYXJiZXJAdXBvLmVz
†These authors have contributed equally to this work