- 1Laboratory for Molecular Immunology, Croatian Institute for Brain Research, University of Zagreb School of Medicine, Zagreb, Croatia
- 2Department of Anatomy, University of Zagreb School of Medicine, Zagreb, Croatia
- 3Department of Physiology and Immunology, University of Zagreb School of Medicine, Zagreb, Croatia
- 4Division of Allergy and Immunology, Cincinnati Children’s Hospital, and University of Cincinnati College of Medicine, Cincinnati, OH, United States
Introduction: Rheumatoid arthritis is the most common immune-mediated joint disease, whose pathogenesis includes both innate and acquired immune mechanisms. Fas/Fas ligand system is considered to have a dual role in arthritis, inducing apoptotic cell death of hyperplastic synoviocytes and inflammatory cells, but also exerts proinflammatory effects. In our study, absence of Fas resulted in decreased accumulation of myeloid cells in affected joints.
Methods: Proportions of synovial hematopoietic cells were assessed by flow cytometry in wild-type and Fas −/− mice with antigen-induced arthritis. Effects of myeloid-specific ablation of Fas on the course of antigen-induced arthritis was assessed using Fasfl/LysMCre model. Arthritis was scored visually, histologically and by micro-computerized tomography. Transcriptome of sorted CD11b+Gr-1+ cells was analyzed by microarray, and effects of potential molecular driver Midline-1 (Mid-1) were analyzed in vitro and using Mid1 −/− mice.
Results: Ameliorated antigen-induced arthritis in Fas −/− mice is characterized by the lack of synovial accumulation of myeloid CD11b+Gr-1+ cells. However, myeloid-specific ablation of Fas was not sufficient to ameliorate arthritis, suggesting proinflammatory effects of Fas in multiple cell subsets in arthritis. Myeloid cells from Fas −/− mice downregulated limited number of genes including Mid1. Stimulation of bone marrow cells with low doses of soluble Fas agonist upregulated expression of Mid1. Inactivation of Mid1 had a variable anti-inflammatory effects in vitro and partial anti-arthritic effect in vivo.
Conclusion: Functional Fas is required for the recruitment and accumulation of innate inflammatory cells in arthritic joints. This accumulation is not driven exclusively by mediators expressed in accumulated subset. Mid1 enhances inflammatory polarization of myeloid cells and promotes bone and cartilage degradation in arthritis.
1 Introduction
Rheumatoid arthritis is the most common form of immune-mediated joint destruction, whose manifestation depends on the complex interplay of various cells and mediators (McInnes and Schett, 2007). The disease mechanisms largely depend on the activation of lymphocytes responsible for the recognition of auto-antigens and production of auto-antibodies (Fournier, 2005), but also on the activation of innate inflammatory cells that act as an important contributor to the initiation and progression of many autoimmune diseases (Morell et al., 2017; Takaki-Kuwahara et al., 2019). One of the suggested anti-inflammatory mechanisms is apoptosis of inflammatory cells mediated by Fas (CD95, Apo-1), a typical death receptor and a regulator of immune system homeostasis through the phenomenon of activation induced cell death (French and Tschopp, 2003; Askenasy et al., 2005). However, Fas exerts a dual role in arthritis. It has the ability to hamper the synovial hyperplasia by inducing apoptosis of hyperplastic synoviocytes and inflammatory cells, but also to promote the disease (Calmon-Hamaty et al., 2015). Ligation by soluble Fas ligand, cleaved from the membrane by metalloproteinases (O' Reilly et al., 2009) may activate non-apoptotic signaling pathways, such as nuclear factor κB (NF-κB) or Src kinase c-yes (Xiao et al., 2002; Buchbinder et al., 2018). In hyperplastic synoviocytes, soluble Fas ligand is able to activate ERK1/2, PI3K, caspase 8 and JNK pathways and induce their proliferation, while blockage of JNK pathway reverts the response to soluble Fas ligand to apoptosis (Audo et al., 2014). Soluble Fas ligand is also able to stimulate integrin-dependent adhesion of CD11b+Gr-1+ neutrophils to vascular endothelium via Syk-Btk/PLCγ2/Rap1 signaling pathway in autoimmune conditions (Gao et al., 2016). Several in vivo studies confirmed the proinflammatory role of Fas in arthritis. DBA1/lpr mice, with spontaneous mutation of Fas are resistant to collagen-induced arthritis, which has been ascribed to inefficient stimulation of hyperplastic synoviocytes (Tu-Rapp et al., 2004). A later study by the same authors described inefficient activation of macrophages by the IL-1R or TLR4 (Toll-like receptor 4) in conditions of blocked Fas signaling, due to interaction of FADD with the TLR adaptor protein MyD88, and suggested that Fas ligation promotes activation of the IL-1R-TLR pathways (Ma et al., 2004). Our group has also described ameliorated non-destructive antigen-induced arthritis (AIA) in mice with a gene knockout for Fas (Fas −/−, Adachi et al., 1995) with preserved bone and cartilage architecture, potentially due to decreased apoptosis of osteochondroprogenitor populations (Lazić Mosler et al., 2019). These mice also showed suppressed joint inflammation. To unravel the potential mediators of Fas proinflammatory and proresorptive signaling, in this study we characterized frequencies of main hematopoietic articular populations in Fas-deficient mice with AIA, analyzed the transcriptome of CD11b+Gr-1+ cells as the most altered subset, and assessed the role of downregulated midline 1 (Mid1) gene as a potential proinflammatory and proresorptive mediator in arthritis.
2 Materials and methods
2.1 Mice
Eight to 18-week old female C57BL/6J wild-type (wt) mice, mice deficient in the Fas gene (Fas −/−) and mice deficient for Midline 1 (Mid1 −/−) and 8-12-week old male and female mice with conditional ablation of Fas in myeloid lineage (Fasfl/flx LysMCre+/−) all on the C57BL/6 background were used in experiments. The Fas −/− mice (Adachi et al., 1995) and wt control (C57BL/6) strain were a gift from Dr. M. Simon (Max Planck Institute for Immunobiology, Freiburg, Germany). The Fasfl/fl mice (Stranges et al., 2007) were a gift from Dr. D. Konrad, Division of Pediatric Endocrinology and Diabetology, University Children’s Hospital, Zurich Center for Integrative Human Physiology, University of Zurich, Switzerland. The Mid1 −/− mice and wt control (C57BL/6) strain were a gift from Dr. G. Meroni (Department of Life Sciences, University of Trieste, Trieste, Italy). LysMCre mice (The Jackson Laboratory, stock no. 004781) that express Cre recombinase in myeloid lineage cells, were crossed with Fasfl/fl mice to generate Fasfl/flLysM-Cre+/− mice which were then crossed with Fasfl/fl strain to obtain mice with the conditional ablation of Fas in myeloid lineage (Fasfl/flLysM-Cre+/−) and controls (Fasfl/flLysM-Cre−/−). All colonies were bred and maintained at the animal facility of the Croatian Institute for Brain Research, University of Zagreb, School of Medicine, under standard conditions (10 h light and 14 h dark daily, standard chow (4RR21/25; Mucedola, Italy) and water ad libitum). All animal protocols were approved by the Ethics Committee of the University of Zagreb, School of Medicine (380-59-10106-15-168/235, 380-59-10106-19-111/250) and the National ethics committee (EP 07-2/2015, EP 230/2020) and conducted in accordance with accepted standards of ethical care and use of laboratory animals.
2.2 Antigen-induced arthritis (AIA)
AIA was chosen for its clearly defined onset, localization, and high incidence, ensuring reliable group comparisons and precise sample timing and its suitability for C57BL/6 strain used in this study. Presence of periarticular bone destruction further makes it ideal for investigating its pathogenesis. Because it affects the knee joints rather than just small joints it allows for precise characterization of subchondral bone and cartilage damage. AIA was induced as described previously (Lazić Mosler et al., 2019) (Figure 1A). Briefly, mice were immunized by two subcutaneous (s.c) injections of methylated bovine serum albumin (mBSA, #A1009, Sigma-Aldrich, St Louis, MO, United States) emulsified in complete Freund’s adjuvant (CFA, Sigma-Aldrich). Mice were randomly assigned to one of the experimental groups. Non-immunized control mice (NI) were injected s.c. with 0.1M phosphate buffered saline (PBS). Arthritis was induced 3 weeks after the initial immunization by intra-articular (i.a.) injection of mBSA dissolved in PBS into both knees, under tribromoethanol (Sigma-Aldrich) or ketamine (Richter Pharma AG, Wels, Austria)/xylazine (Alfasan International BV, Woerden, Netherlands) induced anesthesia. Immunized (IMM) and NI controls were injected i.a. with 10 μl of sterile PBS. Mice were sacrificed on day 31 post-immunization (day 10 post-i.a. injection) by cervical dislocation under tribromoethanol or ketamine/xylazine anesthesia, and transverse diameters of each knee were measured 3 times by a vernier scale/caliper. Knees were then harvested for flow cytometry (performed in all NI, IMM, and AIA mice), FACS sorting for microarray analysis (IMM and AIA mice), and validation of microarray results (NI and AIA mice, Figure 1A). The data on the frequencies of cell populations in relation to micro-computerized tomography (µCT) parameters were collected as a part of a wider overarching study characterizing the course of arthritis, inflammation, bone features, and overall synovial cellular composition of Fas deficient mice with arthritis (Lazić Mosler et al., 2019). Arthritis was assessed by 4-point visual score (0 - no arthritis, 1 - discrete localized thickening of the joint capsule, 2 - mild swelling, absence of sharp patellar ligament contour, 3 - clear swelling with diffuse thickening of the joint capsule, 4 - severe swelling and deformity, visible through the skin) and by measuring knee diameters. Knees were then harvested for μCT, RT-PCR, and flow cytometry.
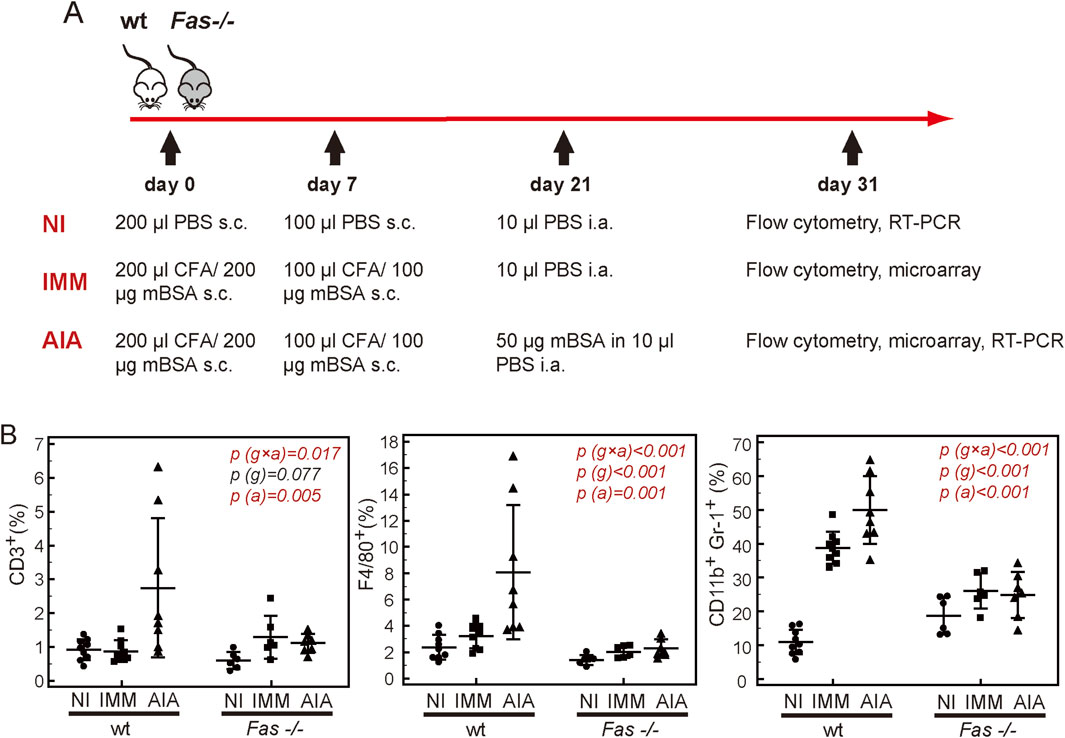
Figure 1. Synovial hematopoietic populations in wild-type (wt) and Fas-deficient mice (Fas −/−). (A) Schematics of experimental design: Initial immunophenotyping was performed in individual non-immunized (NI), immunized control (IMM) and mice with arthritis (AIA) (n > 6). Experiments were repeated 3 times. The data on the frequencies of cell populations in relation to µCT parameters, were collected as a part of a wider overarching study characterizing the course of arthritis in Fas-deficient mice (Lazić Mosler et al., 2019). Microarray analysis included individual samples of sorted CD11b+Gr-1+cells from four IMM and AIA wt and Fas −/− mice, aiming to assess their profile during general immune activation vs. antigen-specific immune-mediated arthritis. RT-PCR validation included RNA samples from bulk-sorted pooled CD11b+Gr-1+cells from minimum 4 NI and 4 AIA wt and Fas −/− mice, or knee tissue extracts from individual NI and AIA wt and Fas −/− mice (n ≥ 4) to confirm alterations of genes of interest during general immune activation. (B) Mice were sacrificed on day 10 following intra-articular (i.a.) injection, and single cell suspensions were labelled with anti-mouse Gr-1-PE, anti-CD11b-PECy7, anti-CD3-APC, and anti-F4/80- APCeF780, and 7-amino-actinomycin D (7-AAD). Proportions of T lymphocytes (CD3+), macrophages (F4/80+), and granulocytes-monocytes (CD11b+, Gr-1+), were determined amongst live cells. Horizontal lines and bars, mean ± SD; p, two-way ANOVA, g, genotype factor, a, arthritis and immunization factor.
2.3 Histology and RNAScope
Knee joints were dissected, released from soft tissues, fixed in 4% formaldehyde at 4°C for 24 h and decalcified for 2 weeks in 14% ethylene-diamine tetra-acetic acid and 4% formalin, then dehydrated, and embedded in paraffin. Frontal 6 μm sections through the knee joint were cut with a Leica SM 2000 R rotational microtome (Leica, Nussloch, Germany). Histological assessment of arthritis severity was performed on sections stained with Goldner-trichrome or toluidine blue, by semi-quantitative scoring described previously (Lazić Mosler et al., 2019). In situ hybridization was performed using RNAscope® kit (Wang et al., 2012) in ACD HybEZTM II Hybridization System (220V) and ACD EZ-Batch Slide System (Advanced Cell Diagnostics, Inc, Minneapolis, MN, SAD), according to the manufacturer’s instructions. Mid1 expression was detected using a specific probe (RNAscope® Probe - Mm-Mid1). RNAscope® Positive Control Probe (Mm-Polr2a) and RNAscope® Negative Control Probe (DapB) were applied to same level sections. Probe binding was detected by horseradish peroxidase substrate diaminobenzidine (DAB). Brown DAB precipitate and hematoxyline counterstained nuclei were visualized using Axio Imager microscope (Carl Zeiss Microscopy GmbH, Jena, Germany).
2.4 Micro-computerized tomography
The distal femora were scanned using a μCT system SkyScan 1076 (Bruker, Kontich, Belgium), at 50kV, 200 μA, and a 0.5 mm aluminum filter, detection pixel size 9 μm, and image capture every 0.4° (for ex vivo scans) or every 1.2° (for in vivo scans) through 180° rotation of the camera. The scanned images were reconstructed using Recon software (Bruker) and analyzed using CTAn software (Bruker). Three-dimensional analysis and reconstruction was performed as described previously (Lazić Mosler et al., 2019), assessing trabecular bone volume (BV/TV, %), trabecular number (Tb.N, mm-2), trabecular thickness (Tb.Th, µm), and trabecular separation (Tb.Sp, mm).
2.5 Flow cytometry and cell sorting
Isolated joints (excision was performed at the level of growth plates) were cleaned from the surrounding muscles and injected with 1 mg/ml collagenase type IV (Sigma-Aldrich) and incubated for 1 h at 37°C. Single cell suspensions were prepared by passing the cells through a 100 μm-cell strainer into FACS tubes. Non-specific antibody binding was blocked by anti-mouse CD16/CD32 (BioLegend, RRID:AB_1574975) for 5 min at RT, and after that cells were labeled by the addition of following antibodies: anti-CD45RA(B220)-FITC (BioLegend, RRID:AB_312991), anti-Ly-6G/Ly-6C(Gr-1)-PE (eBioscience, RRID:AB_466045), anti-CD11b-PECy7 (BioLegend, RRID:AB_312799), anti-CD3-APC (BioLegend, RRID:AB_312677), and anti-F4/80-APCeFluor780 (eBioscience, RRID:AB_2735036), anti-CD95-AF488 (eBioscience, RRID:AB_10671269) and/or anti-CD95-FITC (BD Biosciences, RRID:AB_395329), or corresponding isotype controls (mouse IgG1κ-AF488, eBiosciences, RRID:AB_470230, Armenian hamster IgG2λ-FITC, BD Biosciences, RRID:AB_395165). Dead cells were excluded by binding of 7-amino-actinomycin D (7-AAD, BioLegend) or 4′,6-diamidino-2-phenylindole dihydrochloride (DAPI, Sigma-Aldrich). Proportion of apoptotic cells was determined by binding of Annexin V (BioLegend) and 7-AAD staining, according to the manufacturer’s instructions. Scatter and fluorescent signals were acquired on Attune (Thermo Fisher Scientific) instrument and analyzed by FlowJo software (FlowJo v10, Ashland, OR, United States). Cell sorting was performed by a BD FACSAria I and IIu (BD Biosciences, Franklin Lakes, New Jersey, United States) instrument, as described previously (Ikić Matijašević et al., 2016). CD11b+Gr-1+ cells were sorted in 2 ml collection tubes containing PBS with 2% FCS and used for RNA extraction. Sorting parameters were optimized for high-purity sorting. Sorting purity was determined by a re-analysis of sorted population and was greater than 99.5% for all experiments.
2.6 Cell culture and treatment
Bone marrow cells isolated from wt or Mid1 −/− mice were plated in 48- or 96-well culture plates at a density of 1–2 × 106 cells/0.2–0.5 ml per well of α-MEM supplemented with 10% FCS, (Gibco, Thermo Fisher Scientific). Cell activation was induced by overnight incubation with 10 µg/mL of heat-inactivated Mycobacterium tuberculosis (Difco) at 37°C. In a separate set of experiments cells were, prior to activation, treated with 20–100 µM GSK′364A (Cambridge Research Biochemicals, Billingham, UK), a peptide antagonist of Mid1-PP2A interaction for 1h/37°C. For the assessment of apoptotic and non-apoptotic effects of Fas, cells were treated with 0.01–1 μg/mL anti-mouse CD95 antibody (BD Biosciences, RRID:AB 395326) or the corresponding isotype control (Armenian hamster IgG2λ, BD Biosciences RRID:AB_395162) with or without the addition of 1 μg/mL protein G (Sigma-Aldrich) and incubated for 16–18 h. Untreated cells or cells treated only with 1 μg/mL protein G were used as controls.
2.7 RNA isolation and gene expression analysis
RNA was extracted by Trizol (Thermo Fisher Scientific), as described previously (Kovačić et al., 2007). For microarray analysis samples were pre-cleaned by MagMAX™-96 for Microarrays Kit (Ambion, Thermo Fisher Scientific), and quality assessed by Agilent total RNA Pico chip (Agilent technologies). RNA was then amplified by GeneChip™ WT Pico Kit (Affymetrix, Thermo Fisher Scientific), and cRNA was transcribed, fragmented, labeled and individual samples hybridized to Mouse ST 2.0 arrays (Affymetrix).
2.8 Real-time polymerase chain reaction
Total RNA was converted to cDNA by reverse transcriptase (Thermo Fisher Scientific), and amplified by an ABI 7500 instrument (Applied Biosystems, Thermo Fisher Scientific), using commercially available TaqMan Assays (mouse β-actin, Mm00607939_s1; Erdr1 Mm04214945_uH; Fas, Mm00487425_m1; IL-1β, Mm00434228_m1; IL-6, Mm00446190_m1; Mid1, Mm01166432_m1 Thbs1 Mm00449018_m1; TNF, Mm00443258_m1), as described previously (Kovačić et al., 2007). Samples were amplified individually, while pooled cDNA samples used for construction of standard curve for relative quantification were amplified in duplicates. Negative controls containing RNAse free water instead of sample were used to exclude potential contamination during reverse transcription and PCR amplification.
2.9 Western blot analysis
For protein extraction, knees were homogenized in 1 ml of cell lysing buffer (Cell Signaling Technologies) supplemented with 1 µl Halt Protease Inhibitor Cocktail (Thermo Fisher) and 1 µl Halt Phosphatase Inhibitor Cocktail (Thermo Fisher), lysed by sonication, centrifuged at 14,000 g/10 min/4°C. Supernatants were mixed with NuPAGE® LDS sample buffer (Thermo Fisher) and NuPAGE® reducing agent (Thermo Fisher), denatured at 95°C for 5 min, and proteins separated on 12% stain free gels prepared with TGX Stain-FreeTM FastCast Acrylamide Kit (Bio-Rad) by PAGE-SDS electrophoresis. After gel activation (5 min/UV), proteins were transferred onto a methanol activated PVDF membrane. Total protein amount on membrane was assessed by membrane imaging after brief UV excitation. After blocking with 5% dry milk in 0.1% PBST for 1h/RT, membranes were incubated with rabbit anti-Mid1 antibody (1:500, Thermo Fisher Scientific Cat# PA5-36305, RRID:AB_2553463), overnight at 4°C, and then with HRP conjugated anti-rabbit antibody (1:50,000, Jackson ImmunoResearch) for 1h/RT. Antibody binding was visualized with SuperSignal™ West Femto Maximum Sensitivity Substrate (Thermo Fisher) using ChemiDoc MP™ imaging system (Bio-Rad). Signal intensity was quantified and normalized to total protein signal intensity, using stain free technology (Rivero-Gutiérrez et al., 2014), in Image Lab Software (Bio-Rad).
2.10 Data analysis and interpretation
Data are plotted as individual values, horizontal lines and markers represent means ± SD or medians (IQR). Statistical analysis was performed by MedCalc Statistical Software version 12.5.0 (MedCalc Software bvba, Ostend, Belgium). Depending on the number of factors, distribution, variable type, and the number of comparisons, data were analyzed by t-test and one or two-way ANOVA, or Mann-Whitney and Kruskal-Wallis test. Kolmogorov–Smirnov test was used to assess the normality of the data.
Microarray analysis was done with R, using Bioconductor software (https://bioconductor.org). Values were pre-processed by robust multi-array average (RMA) algorithm. Quality of chips was assessed before and after normalization, showing similar intensities for all chips, and minor variations corrected by normalization. Relative log expression (RLE) was grouped at 0, whereas normalized unscaled standard error (NUSE) was grouped around 1. Correlation coefficients of individual chips were 0.93 and 0.96, before and after processing, respectively. Principal component analysis (PCA) and hierarchical clustering excluded the batch effects. Differences in gene expression were assessed by linear models for microarray analysis (limma) package (Ritchie et al., 2015). Microarray data are available on the GEO repository (Gene expression of sorted synovial myeloid cells from wild type and Fas −/− mice with antigen-induced arthritis and immunized control mice without arthritis, accession number GSE141592) (Kovačić and Lukač, 2019).
3 Results
3.1 Ameliorated AIA in Fas −/− mice is characterized by the lack of accumulation of myeloid CD11b+Gr-1+ cells in synovial compartment
We have previously described reduced epiphyseal bone damage in Fas −/− mice with AIA due to preserved pool of early osteoprogenitor cells and sustained capability to form bone in inflammatory conditions (Lazić Mosler et al., 2019). We proposed that immune disturbances preceding bone loss are also affected by absence of functional Fas. Therefore, in the synovial compartment of wt and Fas −/− non-immunized (NI), immunized control (IMM) and arthritic (AIA) mice, we assessed the proportions of CD3+ T lymphocytes, considered responsible for the immune-mediated pathology, CD11b+Gr-1+ granulocytes-monocytes and F4/80+macrophages involved in the inflammatory component of arthritis (Figure 1A).
The proportions of all populations were higher AIA wt mice, in comparison to all other experimental groups (Figure 1B). The proportion of CD11b+Gr-1+ myeloid cells was significantly higher in the synovial compartment of IMM wt mice in comparison to NI group, with additional increase in wt AIA mice, which was absent in Fas −/− mice (Figure 1B), pointing to the fact that Fas deficiency suppresses accumulation of CD11b+Gr-1+cells in both conditions, general immunization and AIA. A similar trend was observed for F4/80+cells. Proportions of CD11b+Gr-1+ and F4/80+cells were positively associated with joint swelling, but only CD11b+Gr-1+ revealed a strong negative association to the epiphyseal bone volume (Table 1). While T lymphocytes were weakly positively associated with joint swelling (Figure 1B), their increase with immunization and arthritis was not affected by Fas deficiency (Table 1).
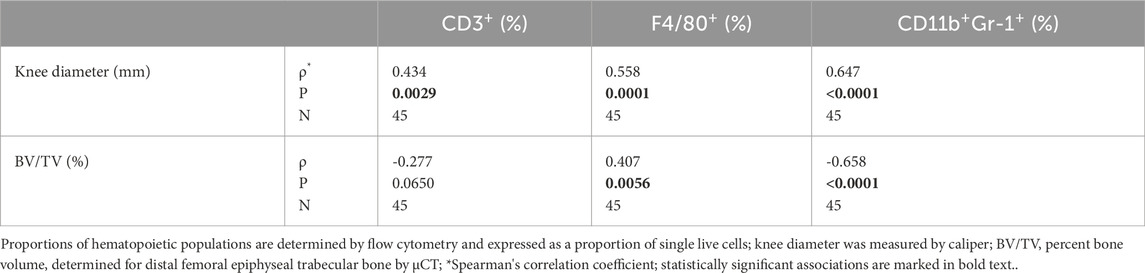
Table 1. Associations of proportions of synovial hematopoietic cell populations with knee diameter and bone volume in antigen-induced arthritis.
3.2 Myeloid cells from Fas −/− mice differentially express several genes
As the differences in proportion of CD11b+Gr-1+ cells between wt and Fas −/− became evident only after immunization, we posited that comparing their transcriptomes in immunized wt and Fas −/− mice would reveal genes responsible for different response to immunization. Since we aimed to distinguish the myeloid cell-specific mediators of transition from immunized condition, characterized by accumulation of inflammatory innate cells, towards the onset of overt arthritis, which are altered by Fas deficiency, we analyzed the gene expression profile of CD11b+Gr-1+ cells from the synovial compartment of IMM and AIA wt and Fas −/− mice purified by FACS. Differential gene expression analysis revealed no differences in expression profiles of myeloid cells from IMM and AIA mice within wt and Fas −/− groups, or when comparing all samples regardless of genotype (Figure 2A), but we found an overexpression of midline 1 (Mid1) and erythroid differentiation regulator 1 (Erdr1), and an underexpression of thrombospondin 1 (Thbs1) when comparing wt mice to Fas −/− mice (Figure 2B). To validate the findings of microarrays, we analyzed the expression of those three genes by RT-PCR in sorted CD11b+Gr-1+ cells and knee joint tissue extracts from NI and AIA mice. In this group of experiments, we used NI mice to address potential changes in selected genes with immune activation. Mid1 and Erdr1 expression profiles were comparable in sorted CD11b+Gr-1+ cells (Figure 2C, upper panels) and knee joint extracts (Figure 2C, lower panels). Expression levels of Erdr1 were similar in NI and AIA wt mice while Mid1 was upregulated in wt AIA mice in comparison to the NI group, which pointed to its potential association with arthritis pathogenesis. Thbs1, similar to microarray data, had a lower expression in wt than in Fas −/− mice. The difference in expression of Thbs1 was more pronounced in sorted CD11b+Gr-1+ cells, while strain-specific and disease-related differences were less pronounced in joint tissue extracts, pointing to other cellular sources of Thbs1. To further distinguish which of differentially expressed genes have a potential role in the pathogenesis of arthritis, we analyzed associations of candidates with knee joint diameters, and expression of proinflammatory cytokines IL-1β, tumor necrosis factor α (TNFα), and IL-6 in the knees of wt mice. Out of three validated genes, Mid1 had the strongest association with joint swelling, and was also the only candidate whose expression was positively associated with expression of all three inflammatory cytokines (Supplementary Table S1). As assessed by in situ RNA hybridization of the sections of knee joints, expression of Mid1 was abundant in subchondral juxta-trabecular bone marrow cells of wt AIA mice and absent in Fas −/− AIA mice (Figure 3A). Similarly to the gene expression level, the Mid-1 protein quantity was significantly reduced in Fas −/− mice in comparison to wt mice (Figure 3B). In addition, we performed a wider assessment of expression in various tissues of AIA mice and observed a generalized downregulation of Mid1 in a multitude of tissues, except in the liver (Figure 3C). However, over the course of AIA, Mid1 expression corresponded to the pattern of expression of proinflammatory IL-1β. During induction, both Mid1 and IL-1β expression levels remain relatively low, with an increase in the early phase of arthritis, and persisting throughout the acute phase (Figure 3D).
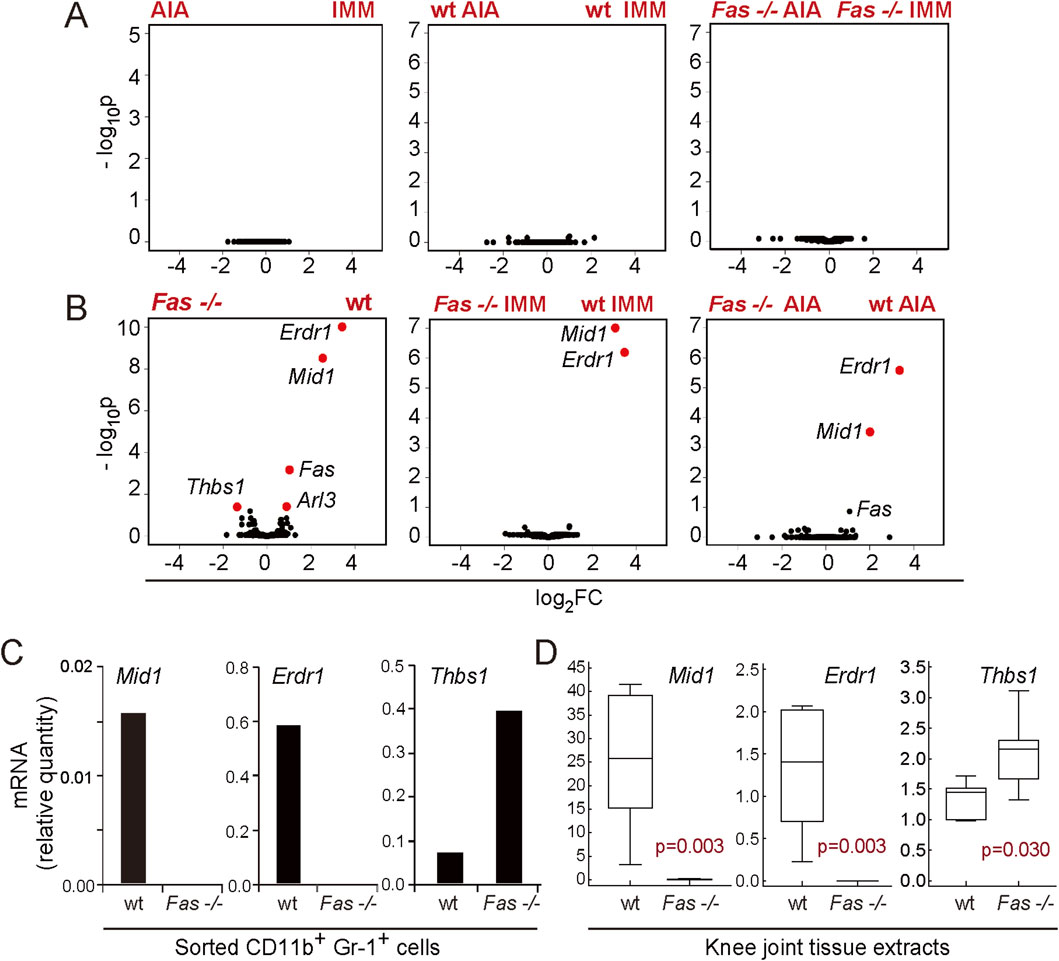
Figure 2. Differential gene expression analysis of synovial myeloid (CD11b+Gr1+) populations from immunized wild-type (wt) and Fas-deficient (Fas −/−) mice on day 10 of antigen-induced arthritis. Volcano plots showing negative logarithm of adjusted p values (-log10p), in relation to the logarithm of gene expression fold-change (log2FC) for differential gene expression analysis between mice with arthritis (AIA) and immunized control mice (IMM) (A) and Fas −/− mice and wt mice (B). Markers of individual genes with significant adjusted p values (Benjamini-Hochberg, BH, p < 0.05) and fold change (absolute FC > 1.5) are marked red. (C) Validation of microarray data by RT-PCR analysis of Erdr1, Mid1, and Thbs1 expression in pooled sorted CD11b+Gr-1+ cells from wt (n = 5), and Fas −/− mice (n = 4) with arthritis (AIA), and knee tissue extracts (D) of wt (n = 6) and Fas −/− AIA mice (n = 7, right panels). Samples for microarray were selected from three separate experiments, based on the RNA quality. Validation of microarray results was performed in two separate experiments, one for analysis of sorted cells and one for the analysis of tissue extracts. Mice were sacrificed on day 10 after arthritis induction. Gene expression is normalized to the β-actin expression. Horizontal line, median; boxes, IQR; whiskers, range; statistical significance is stated on plots (p < 0.05, Mann-Whitney test).
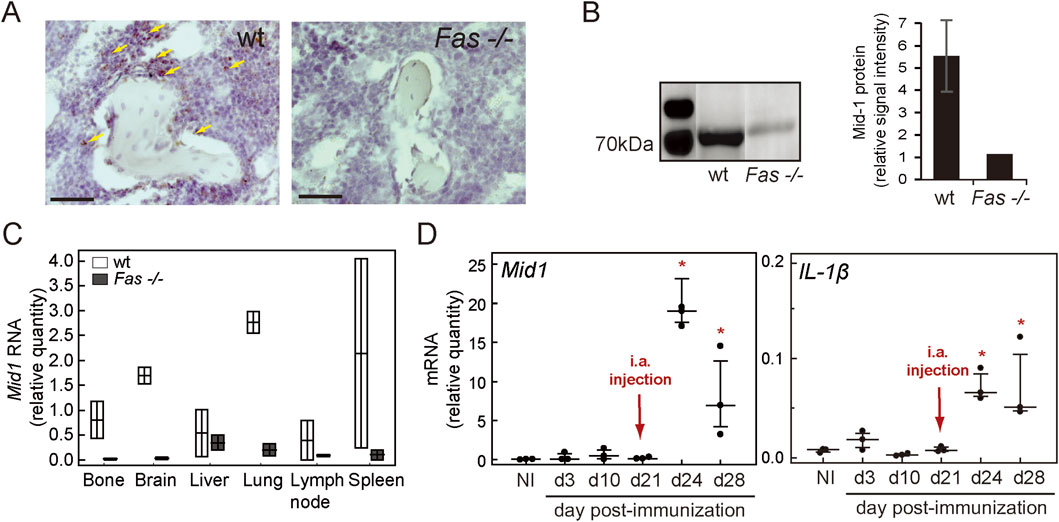
Figure 3. Expression of Mid-1 over the course of AIA. (A) Expression of Mid1 RNA in the knee joints of wt and Fas −/− mice with arthritis, assessed by in situ hybridization (RNAScope). Arrows, brown signal resulting from binding of Mid1 probe, bars, 50 µm. Slides for RNA Scope were selected from a representative experiment used for histology and µCT analysis. Three joints from wt and Fas −/− mice with arthritis were hybridized, with similar results, and representative images are shown. (B) Western blot analysis of Mid-1 expression in knee tissue extracts of wt and Fas −/− AIA mice, and analysis of Mid-1 signal intensity, normalized to total protein signal intensity, acquired from membranes using stain-free technology (Rivero-Gutiérrez et al., 2014). Protein extraction was performed from 6 wt and 2 Fas−/− knees harvested from one experiment. (C) Expression of Mid1 in various tissues of wt (n = 2) and Fas −/− mice (n = 2) with AIA. Tissues were harvested from a single experiment. (D) Expression of Mid1 and IL-1β in total joint tissue extracts of wt mice during immunization (d3-21), early (d24), and 1 week after arthritis induction (d28) in a single experiment. Gene expression was determined by RT-PCR and normalized to the expression of β-actin, n = 3, markers, individual values, horizontal lines, median (IQR), * denotes p < 0.05, in comparison to NI group (Kruskal-Wallis test).
3.3 Expression of Fas in CD11b+Gr-1+ myeloid cells is not required for proinflammatory activity of Fas in arthritis
Since we described a decreased accumulation of myeloid cells as a result of global inactivation of Fas, we posited that this subset requires the expression of functional Fas to home and accumulate in joints during immunization and arthritis induction. Therefore, we hypothesized that myeloid-specific deletion of Fas using LysMCre mice would block their accumulation and abolish their expression of Mid1. We first assessed the efficacy of Fas deletion in various bone marrow subsets, by comparing the expression of Fas on CD11b+Gr-1+ myeloid cells, F4/80+ macrophages, and lymphoid lineage CD3+B220+NK1.1+ cells from mice with conditional ablation of Fas driven by the LysM promoter (Cre+) with cells from the control mice (Cre−) and cells from mice with a global Fas deficiency (Fas −/−). Expression of Fas in CD11b+Gr-1+ myeloid lineage from Cre+ cells was comparable to the levels observed in mice with a global Fas deficiency, but a residual expression was detected on the F4/80+ macrophage subset (Figure 4A). As expected, the lymphoid lineage was not affected by the conditional ablation driven by the LysM promoter (Figure 4A). Both, Cre+ and Cre− mice showed signs of overt arthritis such as increased joint diameters and arthritis visual scores (Figure 4B), as well as a histological presence of a synovial infiltrate and cellular exudate (not shown). This was accompanied by decreased distal epiphyseal BV/TV and trabecular thickness (Figure 4C), revealing the lack of protective effect of Fas inactivation in CD11b+Gr-1+ cells. In addition, expressions of proinflammatory cytokines and Mid1 were comparable in Cre+ and Cre− mice with AIA confirming that expression of Fas in CD11b+Gr-1+ myeloid cells is not necessary for their infiltration and upregulation of Mid1 expression (Figure 4D).
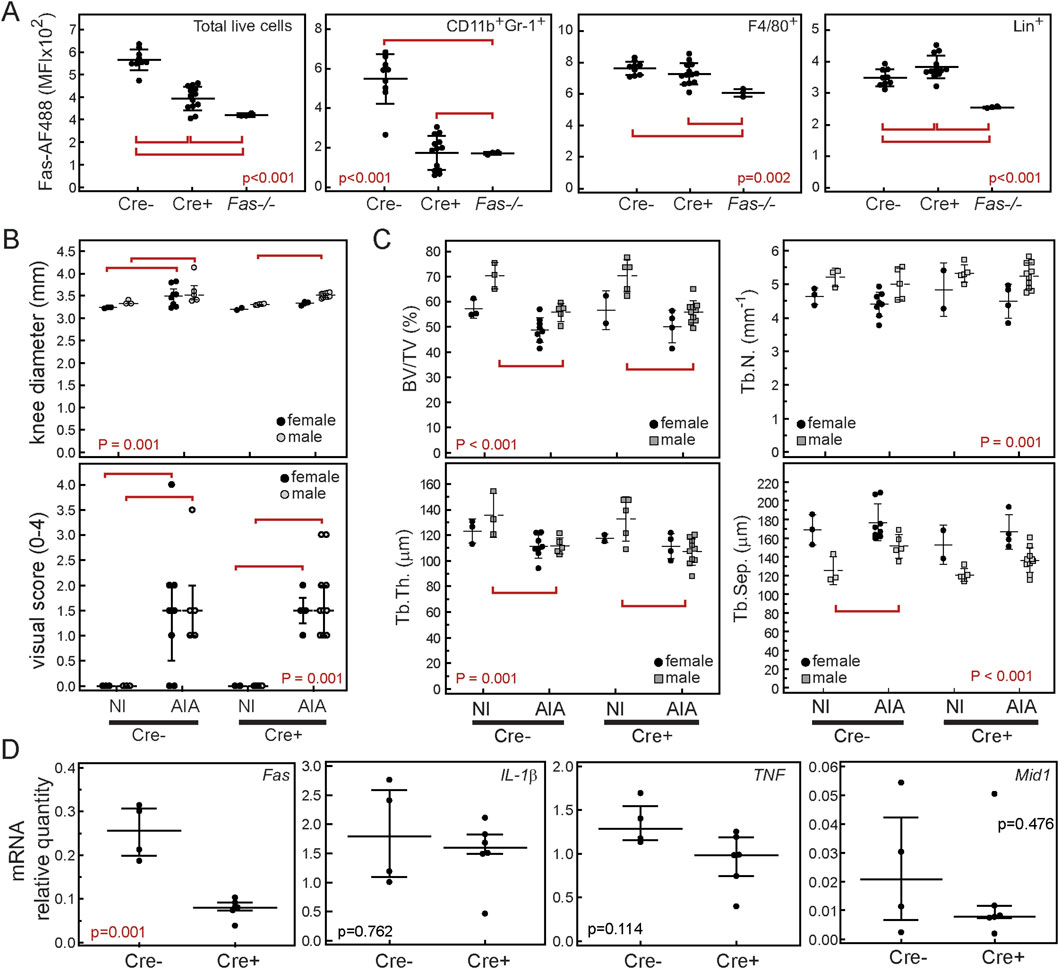
Figure 4. Myeloid-specific ablation of Fas does not ameliorate antigen-induced arthritis (AIA). Fasfl/flLysMCre+/− were crossed with Fasfl/fl mice to produce littermates with conditional ablation of Fas in myeloid cells (Fasfl/flLysMCre+/−, Cre+) and controls (Fasfl/flLysMCre−/−, Cre–). Experiments were repeated 3 times and data are cumulative of minimum 2 representative experiments. (A) Bone marrow cells from Cre−, Cre+ and Fas −/− mice were stained with anti-Fas(CD95)-AF488 or corresponding isotype control, and CD11b-PE, Gr-1-PECy7, F4/80-APCCy7, and CD3/B220/NK1.1-APC antibodies. Mean fluorescence intensities (MFI) for each population are shown as individual values (markers), horizontal lines and bars are mean ± SD. Mice were sacrificed on a day 10 post-i.a. injection and arthritis was assessed by (B) measuring knee diameters and semi quantitative visual soring using appropriate scale (0-no arthritis, 1-discrete localized thickening of the joint capsule, 2-mild swelling, absence of sharp patellar ligament contour, 3-clear swelling with diffuse thickening of the joint capsule, 4-severe swelling and deformity, visible through the skin). (C) Subchondral epiphyseal bone volume was assessed by µCT in Cre-NI− (n = 7 4m/3f), Cre−AIA (n = 13, 5m/8f), Cre+NI (n = 6, 4m/2f), Cre+AIA (n = 14, 10m/4f). The following variables were analyzed in distal femoral epiphyses: trabecular bone volume (BV/TV, %), trabecular number (Tb.N., mm-1), trabecular thickness (Tb.Th., µm), and trabecular separation (Tb.Sep., µm). (D) Expression of Fas, TNF, IL-1β and Mid1 mRNA in total knee joint tissue from Cre− mice with AIA (Cre−, n = 4, 2m/2f), and Cre+ mice with AIA (Cre+, n = 6, 4m/2f). Markers represent individual values, horizontal lines and error bars are mean ± SD (A, B top panel, C) or median (IQR) (B bottom panel, D); statistical significance is marked on plots with red lines connecting experimental groups with p < 0.05 (ANOVA and Student-Newman-Keuls post hoc test, A, B top panel, C; Kruskal-Wallis test, B bottom panel; Mann-Whitney test, D).
3.4 Soluble Fas agonist promotes expression of Mid1 and proinflammatory cytokines
To assess the involvement of Mid-1 in Fas-dependent inflammatory activation, we analyzed in vitro responses of bone marrow cells from wt mice to different modes of Fas activation in resting non-stimulated cells and cells stimulated with heat-inactivated M. tuberculosis as a component of CFA, used to induce inflammatory activation in AIA. The first set of experiments sought to assess how various conditions of cell treatment with agonistic anti-Fas antibody affect their inflammatory response. Cells treated with the antibody and protein G exhibited an apoptotic response, observed by increased annexin V labeling in flow cytometry, which was most pronounced in CD11b+Gr-1+ cells. However, cells treated with agonistic anti-Fas antibody in absence of protein G displayed reduced apoptotic response accompanied by a dose-dependent increase in the expression of IL-1β, TNF and Mid1 genes (Figures 5A,B).
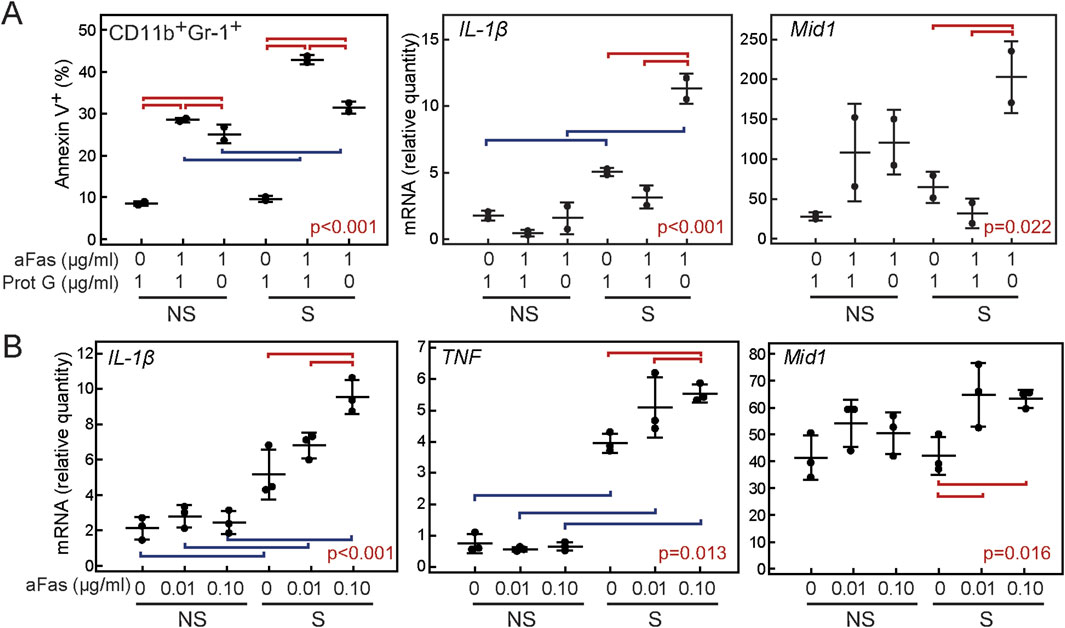
Figure 5. Proinflammatory and apoptotic effect of anti-Fas antibody on cells of myeloid (CD11b+Gr1+) lineage. Inflammation was induced by 2 μg/mL heat-inactivated Mycobacterium tuberculosis, and after incubation for 1 h/37°C, appropriate combinations of anti-Fas antibody, isotype control antibody, and protein G were added. After incubation for 18h/37°C cells were harvested for flow cytometry and/or RNA isolation. (A) Proportion of apoptotic (annexin V+) cells, and expression of proinflammatory IL-1β and Mid1 in groups of non-stimulated (NS) and inflammatory-stimulated cells (S) treated with 1 μg/ml isotype control antibody (NI IgG) and/or 1 μg/ml protein G (Prot G) and/or 1 μg/ml anti-Fas antibody (aFas), (B) Expression of proinflammatory cytokines in groups of non-stimulated (NS) and inflammatory-stimulated cells (S) treated with low doses (0.01–0.1 μg/ml) of anti-Fas antibody. 106 cells were plated per well of a 96-well culture plate. Cells were grown in duplicates (n = 2, A) or triplicates (n = 3, B), and experiments were repeated three times with similar results. Markers, individual values, horizontal lines, mean ± SD. Statistically significant difference within groups of S and NS cells is marked with red lines connecting experimental groups with p < 0.05, statistically significant difference between identically treated S and NS cells is marked with blue lines connecting experimental groups with p < 0.05, p values are marked on plots (ANOVA and Student-Newman-Keuls post-hoc test).
3.5 Inactivation of Mid1 has a variable anti-inflammatory effect in vitro
After we established that low doses of Fas agonist in the absence of protein G may act proinflammatory via stimulation of Mid1 expression, we hypothesized that inactivation of Mid-1 would have an anti-inflammatory effect. We first assessed whether pharmacological inactivation of Mid-1 will reduce proinflammatory cytokine production in bone marrow cells stimulated with heat-inactivated M. tuberculosis. We hypothesized that proinflammatory effect of Mid-1 is mediated by the increased proteasomal degradation of anti-inflammatory PP2A through the interaction with its regulatory subunit α4 (Trockenbacher et al., 2001). This interaction can be blocked by metformin (Kickstein et al., 2010; Demir et al., 2014) or specific antagonistic peptide GSK′364A (Monteiro et al., 2018). Treatment of activated bone marrow cells with both metformin and GSK′364A variably inhibited transcription of proinflammatory IL-1β and TNF, and reduced the level of TNF-α protein, but this effect was not consistent among repeated experiments (Figure 6A). The more pronounced effect of metformin could be attributed to its multiple anti-inflammatory mechanisms (Kristófi and Eriksson, 2021). In the next set of experiments, we assessed whether genetic inactivation of Mid1 would have the ability to block the proinflammatory cytokine expression in anti-Fas-stimulated bone marrow cells. Cells from Mid1 −/− mice revealed a variable decrease in expression of proinflammatory cytokines (Figure 6B), so we concluded that Mid1 signaling is not the exclusive mechanism underlying the proinflammatory effects of Fas.
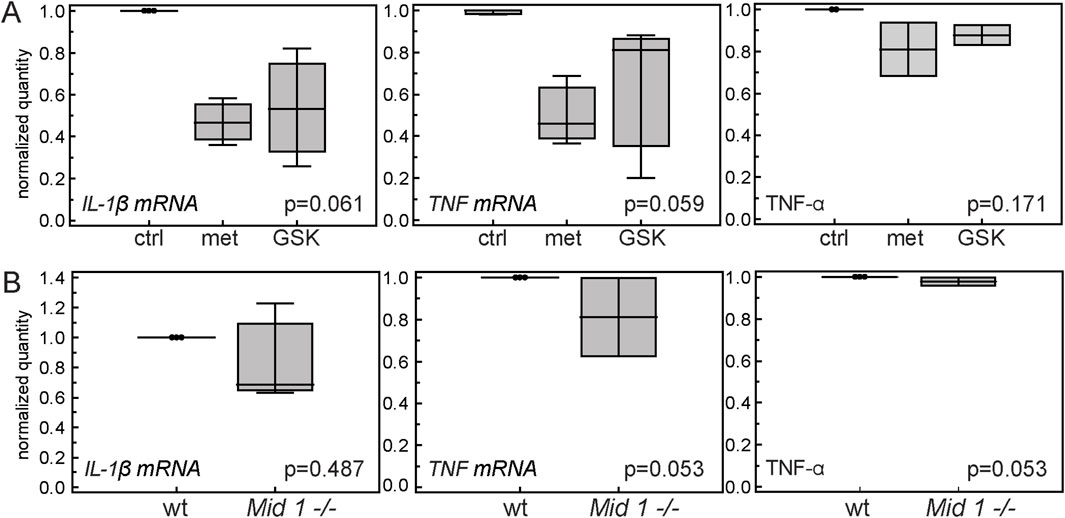
Figure 6. Effects of Mid-1 inactivation on the expression of pro-inflammatory cytokines in inflammatory-stimulated cells. (A) Pharmacological inhibition of Mid-1 in inflammatory-stimulated bone marrow cells. Cells were pre-treated with 20 µM metformin or 20 µM of Mid-1 peptide antagonist (GSK'364A) for 1h/37°C which was followed by the addition of heat inactivated Mycobacterium tuberculosis in final concentration of 10 µg/µL. After overnight incubation at 37°C, expression of proinflammatory cytokines IL-1β, and TNF and concentration of TNF-α were assessed by RT-PCR and ELISA. Experiments were performed in duplicates to quadruplicates, and values are calculated as ratios of means of expression/concentration of cytokine in the stimulated cells treated with metformin or GSK'364A over means of expression/concentration of cytokine in stimulated untreated cells in each experiment (n = 3). (B) Expression of inflammatory cytokines in inflammatory-stimulated bone marrow cells from wt and Mid1 −/− mice treated with agonistic anti-Fas antibody. Cells were treated with 10 µg/µL heat-inactivated Mycobacterium tuberculosis and 0.1 µg/mL anti-Fas antibody. After overnight incubation at 37°C, expression of proinflammatory cytokines IL-1β, and TNF and concentration of TNF-α were assessed by RT-PCR and ELISA. Experiments were performed in duplicates to quadruplicates, and values are calculated as ratios of means of expression/concentration of cytokine in the stimulated cells treated with metformin or GSK'364A over means of expression/concentration of cytokine in stimulated untreated cells in each experiment (n = 3). Horizontal line, median; boxes, IQR; whiskers, range; p values are marked on plots (A, Kruskal-Wallis test; B, Mann- Whitney test).
3.6 Inactivation of Mid1 partially mitigates AIA
We further assessed the effect of Mid1 inactivation on arthritis in Mid1 −/− mice. Mid1 −/− mice developed AIA but joint swelling and arthritis visual score were reduced in comparison to wt AIA mice (Figures 7A,B). The overall histology score, synovial infiltration and bone destruction were clearly increased in Mid1 −/− mice in comparison to control NI Mid1 −/− mice, but the overall score, cartilage degradation and bone resorption were also significantly lower in comparison to wt AIA mice (Figure 7C). Distal epiphyseal trabecular bone volume and trabecular thickness were reduced in both wt and Mid1 −/− mice in comparison to NI controls (Figures 7D,E). However, a significant reduction in trabecular number and increase in trabecular separation were observed in wt mice with AIA, while the number and separation of distal epiphyseal trabeculae in Mid1 −/− mice with AIA were similar to corresponding controls. Taken together these results point to a partial protective effect of Mid1 inactivation on cartilage and bone damage in inflammatory arthritis.
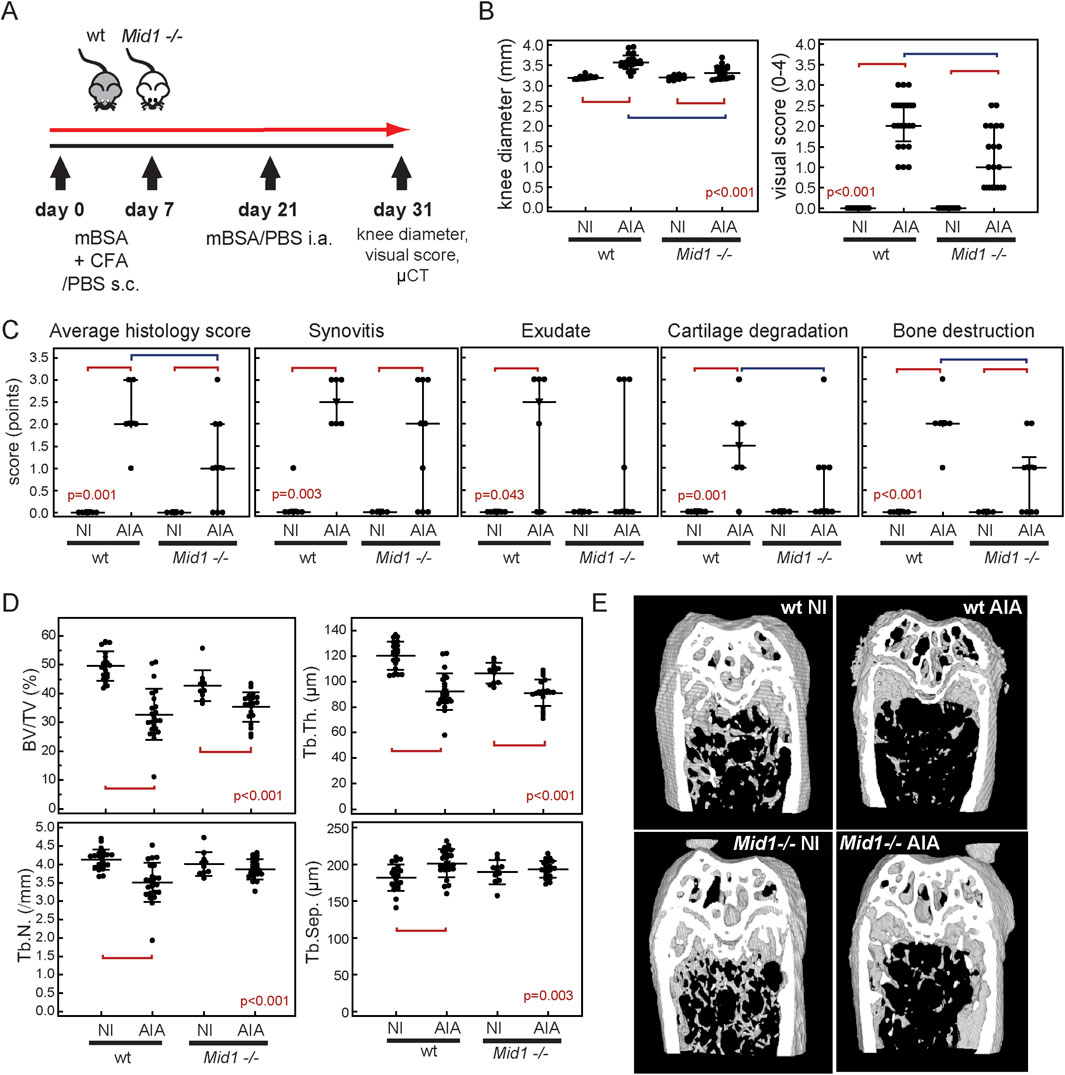
Figure 7. Mid-1 inactivation moderately alleviates antigen-induced arthritis (AIA). (A) AIA was induced in mice with a gene knockout for Mid1 (Mid1 −/−) and wt mice. Visual score, knee diameters and µCT values represent cumulative data from 4 separate experiments, while histology score was assessed in one of the representative experiments. Mice were sacrificed on a day 10 post-i.a. injection and arthritis was assessed by (B) measuring knee diameters and semi quantitative visual soring in wt NI (n = 21), wt AIA (n = 23), Mid1 −/− NI (n = 10), Mid1 −/− AIA (n = 20) using appropriate scale (0-no arthritis, 1-discrete localized thickening of the joint capsule, 2-mild swelling, absence of sharp patellar ligament contour, 3-clear swelling with diffuse thickening of the joint capsule, 4-severe swelling and deformity, visible through the skin). (C) Total histology score in wt NI (n = 6), wt AIA (n = 6), Mid1 −/− NI (n = 4), and Mid1 −/− AIA (n = 9). Scoring was performed according to synovial thickening, presence of exudate in joint space, cartilage degradation, and subchondral bone damage, according to the 3 point scale. (D) Subchondral epiphyseal bone volume was assessed by µCT in wt NI (n = 21), wt AIA (n = 23), Mid1 −/− NI (n = 10), Mid1 −/− AIA (n = 20). The following variables were analyzed in distal femoral epiphyses: trabecular bone volume (BV/TV, %), trabecular number (Tb.N., mm-1), trabecular thickness (Tb.Th., µm), and trabecular separation (Tb.Sep., µm). (E) Representative 3D models of distal femora, from µCT reconstruction images. Markers represent individual values, horizontal lines and error bars are mean ± SD (B left panel, C) or median (IQR) (B right panel, D); statistical significance is marked on plots with red lines connecting experimental groups with p < 0.05 (ANOVA and Student-Newman-Keuls post hoc test, B left panel, C; Kruskal-Wallis test, B right panel, D).
4 Discussion
This study demonstrated that expression of functional Fas is required for activation and recruitment of myeloid cells to the affected joints in the murine AIA. Wild-type mice showed increased accumulation of myeloid cells, particularly of CD11b+Gr-1+ cells even in immunized mice without arthritis, suggesting a role of Fas in their accumulation prior to the arthritis induction by antigen challenge. We also confirmed that the proinflammatory effect of low doses of Fas agonistic antibody is particularly evident on myeloid-lineage cells which points to potential non-apoptotic signaling during AIA (Audo et al., 2014; Buchbinder et al., 2018). Myeloid cell activation during immunization is an important factor for the induction of various experimental disease models based on immunization, such as collagen-induced arthritis and experimental autoimmune encephalomyelitis (Billiau and Matthys, 2011; Rumble et al., 2015; Morell et al., 2017), so functional Fas might be generally required for inflammatory cell activation. Transcriptomes of CD11b+Gr-1+cells in AIA and IMM mice were similar, suggesting their role in creating the inflammatory environment, required for successful induction of immune-mediated joint destruction. However, as the absence of CD11b+Gr-1+ accumulation is one of the main characteristics of attenuated AIA of Fas −/− mice, we proposed that potential molecular drivers of myeloid accumulation are intrinsically differentially expressed. Although the transcriptomes of wt and Fas −/− myeloid cells did not differ in a large number of genes, we identified three putative candidates and validated their expression pattern by RT-PCR. Among them, Mid1 had the strongest association with inflammation markers and its expression was clearly upregulated with the onset of arthritis, suggesting its involvement in the pathogenesis of AIA. Mid1 encodes a microtubule-related E3 ubiquitin-ligase, whose mutation in humans and mice results in developmental anomalies of CNS (Robin et al., 1996; Lancioni et al., 2010). The best described ubiquitination target of Mid1 is PP2A, responsible for dephosphorylation of mitogen-activated protein kinases (MAPK) (Trockenbacher et al., 2001). A deficiency of PP2A leads to increased phosphorylation of MAPK (p38, ERK, JNK) and NF-κB (IKKα/β, NF-κB p65) pathways in bone marrow macrophages, and stimulates the production of TNF-α, IL-6, and IL-10, which suggests its importance in limiting the inflammatory polarization of myeloid cells (Sun et al., 2017). Several studies have documented the involvement of Mid1 in immune regulation. Increased expression of Mid1, dependent on functional TLR4 and TNF-related apoptosis inducing ligand (TRAIL), has been reported in murine bronchial epithelium after exposure to house dust mite or rhinovirus infection. Silencing of Mid1 or pharmacological activation of PP2A decreased the intensity of inflammation, accumulation of inflammatory cells, levels of IL-25, IL-33, CCL20, IL-5, IL-13, NF-κB activity and MAPK phosphorylation (Collison et al., 2013). The same research group reported similar results in the model of experimental allergic esophagitis, with disease amelioration in TRAIL −/− mice (Collison et al., 2015) and proposed a disease-promoting role of Mid-1 downstream of TRAIL in the pathogenesis of pulmonary fibrosis (Collison et al., 2019). TRAIL belongs to the same family as Fas ligand, and signals through TNF family death receptors (DR)5 and DR6, via similar signaling mechanisms as Fas, which suggest a downstream role of Mid-1 in death receptor signaling. Furthermore, Mid1 has been described as an important factor for functioning of cytotoxic T lymphocytes, by affecting their TCR signaling, exocytosis of lytic granules, polarization and migration (Boding et al., 2014a; Boding et al., 2014b). Recent study documented the role of Mid-1 in regulation of T cell entrance to the CNS in a model of experimental autoimmune encephalomyelitis and the ability of Mid-1 to affect mTOR signaling in T lymphocytes (Wei et al., 2024). In addition, apart from immune cells Mid-1 seems to signal through PP2A in endothelial cells, enhancing their expression of ICAM-1 and neutrophil adhesion and extravasation in septic conditions (Du et al., 2023).
We established that expression of Fas in CD11b+Gr-1+ subset is not necessary for their accumulation in affected joints and induction of AIA, pointing to predominance of proinflammatory over proapoptotic effects. Similar results were obtained by Huang and coworkers who studied the K/BxN model of murine arthritis and observed a comparable onset but faster resolution of arthritis in mice with a myeloid-specific deletion of Fas. This study reported a decreased frequency of CD11b+Gr-1+ cells in joints from Cre+ mice without apparent differences in migration or apoptosis of Fas-deficient CD11b+Gr-1+ cells. The faster resolution of arthritis was attributed to altered activation of Fas-deficient macrophages by IL -1β and the endogenous TLR2 ligand gp96 and overexpression of anti-inflammatory IL-10 (Huang et al., 2014). Although we assessed arthritis in subacute phase, we did not observe decreased inflammation or joint destruction. This could be also attributed to preserved expression of Fas in macrophages in our model, or differences between K/BxN and AIA models. In addition, even though myeloid cells represented 40%–50% of the total articular population and the conditional deletion of Fas was reflected in an overall decrease of Fas expression in total joint extracts, we did not observe decreased expression of Mid1. Thus, in the absence of generalized depletion of Fas, Mid1 expression is not completely blocked. Among various tissues from Fas −/− mice, Mid1 expression was reduced in lungs, lymph nodes, spleen, brain, bone and bone marrow with some residual transcriptional activity in liver. Lack of expression of Mid1 and Erdr1 could therefore be a result of constitutively inhibited transcriptional activity due to deficient Fas signaling or their inactivation by potential genomic alterations. Interestingly, Mid1, as well as Erdr1 genes are located on the pseudoautosomal region (PAR) of sex chromosomes which represents the site of pairing and recombination between the X and Y chromosomes during male meiosis. It is genetically unstable, so deletion or duplication events involving various genes including Mid1 and Erdr1 are frequent in this region (Dal Zotto et al., 1998). Taking that into account poses the possibility that reduced Mid1 in Fas −/− mice might be a coincidental result of the PAR genetic instability rather than absence of the Fas signaling. Further studies are required to confirm this possibility.
Several studies described the ability of metformin to block the interaction of Mid1 and α4, a regulatory subunit of PP2A, and thus inhibit the proteasomal degradation of PP2A (Kickstein et al., 2010; Demir et al., 2014). A more specific inhibition of this interaction can be achieved by a peptide antagonist (GSK′364A) resembling the Mid-1-α4 binding site (Monteiro et al., 2018).
In our model, both metformin and GSK′364A had a variable inhibitory effect on TLR2-dependent transcription of IL-1β and TNF in primary murine bone marrow cells. Although proinflammatory activity of Mid-1 interaction with PP2A has been previously documented, inability of GSK′364A and metformin to completely abolish TLR2-dependent transcription of IL-1β and TNF suggests existence of additional, yet undiscovered downstream proinflammatory mediators of Mid-1. Recent studies reported Mid-1-dependent targeting of IRF3 and IFNAR2, acting as a negative feedback mechanism of the innate antiviral immunity (Chen X. et al., 2021; Chen et al., 2022). Moreover, deletion of Mid1 decreased susceptibility of mice to viral myocarditis, pneumonia, and herpes simplex encephalitis by enhancing the production of type I IFN through its interaction with protein phosphatase 1A (PPM1A) responsible for dephosphorylation of TANK binding kinase 1 (TBK1). Phosphorylation of TBK1 is crucial for IRF3 transcription and production of IFN (Fang et al., 2022). In addition, one of the more recently defined Mid-1 ubiquitination targets is non-receptor tyrosine phosphatase PTP1B, whose degradation leads to overactivation of the STAT3 signaling pathway and promotes inflammation and fibrosis in diabetic kidney disease (Chen Q. et al., 2021).
Furthermore, in our model, genetic inactivation of Mid1 did not completely abolish the cytokine production in inflammatory-activated bone marrow cells stimulated with anti-Fas agonistic antibody. This may implicate the existence of additional Mid-1-independent proinflammatory pathways or point to previously documented functional redundancy between Mid-1 and Mid-2 (Granata et al., 2005; Boding et al., 2015). Another potential explanation of inability of Mid-1 inhibition to completely abolish the described cytokine production is that we used total population of bone marrow cells to better model the complexity of interactions of multiple bone marrow-derived lineages, similarly to the interactions occurring during in vivo AIA, instead of purified myeloid populations. In this model, discrete effects within a specific lineage might be avoided or altered by individual variability in bone marrow composition.
In the AIA model, the inactivation of Mid1 partially reduced bone and cartilage damage in AIA, without a significant effect on the synovitis and myeloid cell accumulation in affected joints. Our findings contrast the previous studies that reported proinflammatory activity of Mid-1 in lungs and other tissues (Collison et al., 2013; Collison et al., 2019; Wei et al., 2024; Du et al., 2023), and suggest that proinflammatory activity of Fas is independent of Mid-1. In addition, a recent study reported complete protection of Mid1 −/− mice from development of collagen-induced arthritis (Lin et al., 2024). According to this study Mid-1 promotes synoviocytes proliferation and migration by inducing ubiquitin-mediated proteasomal degradation of dipeptidyl peptidase-4. In our AIA model, overt synovitis was less frequent but not completely absent. The discrepancies between the results could be ascribed to differences in arthritis model, as well as to different approaches to induce Mid1 deletion, so additional mechanistic studies are required to resolve these issues. The reports on direct effects of Mid-1 on bone structure and function are scarce. An increase in Mid1 expression has been documented in autoimmune prone BXSB-Yaa mice, also characterized by alterations in bone structure (Namba et al., 2019). On the other hand, Mid-1 ubiquitinates RIC8 guanine nucleotide exchange factor A, which promotes the progression of osteoarthritis by activating p38 mitogen-activated protein kinase signaling pathway in chondrocytes, thus pointing to a protective effect of Mid-1 signaling in OA (Shen et al., 2021). All of the above points to multifaceted, not yet fully elucidated roles of Mid-1 in bone and cartilage homeostasis.
In conclusion, our results point to the requirement of functional Fas for the proinflammatory component of AIA, responsible for the recruitment and accumulation of innate inflammatory cells in arthritic joints. This accumulation is not driven by intrinsic factors expressed in the accumulated subset. Mid-1 aids in an inflammatory polarization of myeloid cells and promotes bone loss and cartilage degradation in arthritis.
Data availability statement
The original contributions presented in the study are publicly available. This data can be found here: Gene Expression Omnibus (GEO) repository, accession number GSE141592 at https://www.ncbi.nlm.nih.gov/geo/query/acc.cgi?acc=GSE141592.
Ethics statement
The animal study was approved by Ethics Committee of the School of Medicine, University of Zagreb. The study was conducted in accordance with the local legislation and institutional requirements.
Author contributions
NL: Conceptualization, Data curation, Formal Analysis, Investigation, Methodology, Validation, Visualization, Writing – original draft, Writing – review and editing. DF: Formal Analysis, Investigation, Methodology, Writing – original draft, Writing – review and editing, Data curation, Supervision, Visualization. SP: Conceptualization, Formal Analysis, Investigation, Methodology, Validation, Visualization, Writing – original draft, Writing – review and editing. TK: Conceptualization, Funding acquisition, Methodology, Resources, Supervision, Writing – original draft, Writing – review and editing. AS: Conceptualization, Investigation, Methodology, Software, Supervision, Validation, Writing – original draft, Writing – review and editing. MF: Investigation, Methodology, Project administration, Visualization, Writing – original draft, Writing – review and editing. DŠ: Investigation, Methodology, Validation, Visualization, Writing – original draft, Writing – review and editing. MF: Data curation, Investigation, Methodology, Writing – original draft, Writing – review and editing. IR: Data curation, Investigation, Methodology, Validation, Writing – original draft, Writing – review and editing. VK: Conceptualization, Resources, Software, Supervision, Writing – original draft, Writing – review and editing. NZ: Conceptualization, Methodology, Supervision, Validation, Writing – original draft, Writing – review and editing. DG: Conceptualization, Funding acquisition, Investigation, Methodology, Resources, Supervision, Visualization, Writing – original draft, Writing – review and editing. NK: Conceptualization, Formal Analysis, Funding acquisition, Investigation, Methodology, Project administration, Resources, Software, Writing – original draft, Writing – review and editing, Data curation, Supervision, Visualization.
Funding
The author(s) declare that financial support was received for the research and/or publication of this article. This work was supported by the Croatian Science Foundation grants HRZZ-IP-2014-09-7406, HRZZ-IP-2022-10-2285, HRZZ-UIP-2017-05-1965, and HRZZ-DOK-2015-10-9897, and Scientific Center of Excellence for Reproductive and Regenerative Medicine, Republic of Croatia, and by the European Union through the European Regional Development Fund, under grant agreement No. KK.01.1.1.01.0008, project “Reproductive and Regenerative Medicine - Exploring New Platforms and Potentials”.
Acknowledgments
The authors thank the Laboratory for Mineralized Tissues, School of Medicine, University of Zagreb, for access to the 1076 SkyScan µCT instrument; Department for Functional Genomics, Center for Translational and Clinical Research University of Zagreb School of Medicine, for access to Affymetrix equipment and assistance with hybridization of chips; Laboratory for Neurochemistry, Croatian Institute for Brain Research for access to western blot equipment and help with sample preparation and analysis. The authors thank Katerina Zrinski-Petrović for her technical assistance. The authors thank Leah Kottyan for guidance with microarray analysis. A part of the results presented in the article is included in a doctoral thesis (Lukač, 2020).
Conflict of interest
The authors declare that the research was conducted in the absence of any commercial or financial relationships that could be construed as a potential conflict of interest.
The author(s) declared that they were an editorial board member of Frontiers, at the time of submission. This had no impact on the peer review process and the final decision.
Publisher’s note
All claims expressed in this article are solely those of the authors and do not necessarily represent those of their affiliated organizations, or those of the publisher, the editors and the reviewers. Any product that may be evaluated in this article, or claim that may be made by its manufacturer, is not guaranteed or endorsed by the publisher.
Supplementary material
The Supplementary Material for this article can be found online at: https://www.frontiersin.org/articles/10.3389/fcell.2025.1451093/full#supplementary-material
Abbreviations
AIA, antigen-induced arthritis; CFA, complete Freund’s adjuvant; IL, interleukin; IMM, immunized control mice; mBSA, methylated bovine serum albumin; MAPK, mitogen activated protein kinases; NF-κB, nuclear factor κB; NI, non-immunized control mice; PP2A, protein phosphatase 2A; TNFα, tumor necrosis factor α; TRAIL, tumor necrosis factor–related apoptosis inducing ligand; Mid-1, Midline 1 protein; Mid1, midline 1 gene.
References
Adachi, M., Suematsu, S., Kondo, T., Ogasawara, J., Tanaka, T., Yoshida, N., et al. (1995). Targeted mutation in the Fas gene causes hyperplasia in peripheral lymphoid organs and liver. Nat. Genet. 11 (3), 294–300. doi:10.1038/ng1195-294
Askenasy, N., Yolcu, E. S., Yaniv, I., and Shirwan, H. (2005). Induction of tolerance using Fas ligand: a double-edged immunomodulator. Blood 105 (4), 1396–1404. doi:10.1182/blood-2004-06-2364
Audo, R., Calmon-Hamaty, F., Papon, L., Combe, B., Morel, J., and Hahne, M. (2014). Distinct effects of soluble and membrane-bound fas ligand on fibroblast-like synoviocytes from rheumatoid arthritis patients. Arthritis Rheumatol. 66 (12), 3289–3299. doi:10.1002/art.38806
Billiau, A., and Matthys, P. (2011). Collagen-induced arthritis and related animal models: how much of their pathogenesis is auto-immune, how much is auto-inflammatory? Cytokine Growth Factor Rev. 22 (5-6), 339–344. doi:10.1016/j.cytogfr.2011.11.003
Boding, L., Hansen, A. K., Meroni, G., Johansen, B. B., Braunstein, T. H., Bonefeld, C. M., et al. (2014a). Midline 1 directs lytic granule exocytosis and cytotoxicity of mouse killer T cells. Eur. J. Immunol. 44 (10), 3109–3118. doi:10.1002/eji.201344388
Boding, L., Hansen, A. K., Meroni, G., Levring, T. B., Woetmann, A., Ødum, N., et al. (2015). MID2 can substitute for MID1 and control exocytosis of lytic granules in cytotoxic T cells. Apmis 123 (8), 682–687. doi:10.1111/apm.12402
Boding, L., Hansen, A. K., Nielsen, M. M., Meroni, G., Braunstein, T. H., Woetmann, A., et al. (2014b). Midline 1 controls polarization and migration of murine cytotoxic T cells. Immun. Inflamm. Dis. 2 (4), 262–271. doi:10.1002/iid3.44
Buchbinder, J. H., Pischel, D., Sundmacher, K., Flassig, R. J., and Lavrik, I. N. (2018). Quantitative single cell analysis uncovers the life/death decision in CD95 network. PLoS Comput. Biol. 14 (9), e1006368. doi:10.1371/journal.pcbi.1006368
Calmon-Hamaty, F., Audo, R., Combe, B., Morel, J., and Hahne, M. (2015). Targeting the fas/FasL system in rheumatoid arthritis therapy: promising or risky? Cytokine 75 (2), 228–233. doi:10.1016/j.cyto.2014.10.004
Chen, Q., Gao, C., Wang, M., Fei, X., and Zhao, N. (2021a). TRIM18-Regulated STAT3 signaling pathway via PTP1B promotes renal epithelial-mesenchymal transition, inflammation, and fibrosis in diabetic kidney disease. Front. Physiol. 12, 709506. doi:10.3389/fphys.2021.709506
Chen, X., Xu, Y., Tu, W., Huang, F., Zuo, Y., Zhang, H. G., et al. (2021b). Ubiquitin E3 ligase MID1 inhibits the innate immune response by ubiquitinating IRF3. Immunology 163 (3), 278–292. doi:10.1111/imm.13315
Chen, X., Zhao, Q., Xu, Y., Wu, Q., Zhang, R., Du, Q., et al. (2022). E3 ubiquitin ligase MID1 ubiquitinates and degrades type-I interferon receptor 2. Immunology 167 (3), 398–412. doi:10.1111/imm.13544
Collison, A., Hatchwell, L., Verrills, N., Wark, P. A., de Siqueira, A. P., Tooze, M., et al. (2013). The E3 ubiquitin ligase midline 1 promotes allergen and rhinovirus-induced asthma by inhibiting protein phosphatase 2A activity. Nat. Med. 19 (2), 232–237. doi:10.1038/nm.3049
Collison, A. M., Li, J., de Siqueira, A. P., Lv, X., Toop, H. D., Morris, J. C., et al. (2019). TRAIL signals through the ubiquitin ligase MID1 to promote pulmonary fibrosis. BMC Pulm. Med. 19 (1), 31. doi:10.1186/s12890-019-0786-x
Collison, A. M., Sokulsky, L. A., Sherrill, J. D., Nightingale, S., Hatchwell, L., Talley, N. J., et al. (2015). TNF-related apoptosis-inducing ligand (TRAIL) regulates midline-1, thymic stromal lymphopoietin, inflammation, and remodeling in experimental eosinophilic esophagitis. J. Allergy Clin. Immunol. 136 (4), 971–982. doi:10.1016/j.jaci.2015.03.031
Dal Zotto, L., Quaderi, N. A., Elliott, R., Lingerfelter, P. A., Carrel, L., Valsecchi, V., et al. (1998). The mouse Mid1 gene: implications for the pathogenesis of Opitz syndrome and the evolution of the mammalian pseudoautosomal region. Hum. Mol. Genet. 7 (3), 489–499. doi:10.1093/hmg/7.3.489
Demir, U., Koehler, A., Schneider, R., Schweiger, S., and Klocker, H. (2014). Metformin anti-tumor effect via disruption of the MID1 translational regulator complex and AR downregulation in prostate cancer cells. BMC Cancer 14, 52. doi:10.1186/1471-2407-14-52
Du, F., Hawez, A., Ding, Z., Wang, Y., Rönnow, C.-F., Rahman, M., et al. (2023). E3 ubiquitin ligase midline 1 regulates endothelial cell ICAM-1 expression and neutrophil adhesion in abdominal sepsis. Int. J. Mol. Sci. 24 (1), 705. doi:10.3390/ijms24010705
Fang, M., Zhang, A., Du, Y., Lu, W., Wang, J., Minze, L. J., et al. (2022). TRIM18 is a critical regulator of viral myocarditis and organ inflammation J. Biomed Sci. 29 (1), 55. doi:10.1186/s12929-022-00840-z
Fournier, C. (2005). Where do T cells stand in rheumatoid arthritis? Jt. Bone Spine 72 (6), 527–532. doi:10.1016/j.jbspin.2004.12.012
French, L. E., and Tschopp, J. (2003). Protein-based therapeutic approaches targeting death receptors. Cell Death Differ. 10 (1), 117–123. doi:10.1038/sj.cdd.4401185
Gao, L., Gülcüler, G. S., Golbach, L., Block, H., Zarbock, A., and Martin-Villalba, A. (2016). Endothelial cell-derived CD95 ligand serves as a chemokine in induction of neutrophil slow rolling and adhesion. Elife 5, e18542. doi:10.7554/eLife.18542
Granata, A., Savery, D., Hazan, J., Cheung, B. M., Lumsden, A., and Quaderi, N. A. (2005). Evidence of functional redundancy between MID proteins: implications for the presentation of Opitz syndrome. Dev. Biol. 277 (2), 417–424. doi:10.1016/j.ydbio.2004.09.036
Huang, Q. Q., Birkett, R., Koessler, R. E., Cuda, C. M., Haines, G. K., Jin, J. P., et al. (2014). Fas signaling in macrophages promotes chronicity in K/BxN serum-induced arthritis. Arthritis Rheumatol. 66 (1), 68–77. doi:10.1002/art.38198
Ikić Matijašević, M., Flegar, D., Kovačić, N., Katavić, V., Kelava, T., Šućur, A., et al. (2016). Increased chemotaxis and activity of circulatory myeloid progenitor cells may contribute to enhanced osteoclastogenesis and bone loss in the C57BL/6 mouse model of collagen-induced arthritis. Clin. Exp. Immunol. 186 (3), 321–335. doi:10.1111/cei.12862
Kickstein, E., Krauss, S., Thornhill, P., Rutschow, D., Zeller, R., Sharkey, J., et al. (2010). Biguanide metformin acts on tau phosphorylation via mTOR/protein phosphatase 2A (PP2A) signaling. Proc. Natl. Acad. Sci. U. S. A. 107 (50), 21830–21835. doi:10.1073/pnas.0912793107
Kovačić, N., and Lukač, N. (2019). Gene expression of sorted synovial myeloid cells from wild type and Fas-/- mice with antigen-induced arthritis and immunized control mice without arthritis. Gene Expression Omnibus (GEO) repository. GSE141592.
Kovačić, N., Lukić, I. K., Grčević, D., Katavić, V., Croucher, P., and Marušić, A. (2007). The Fas/Fas ligand system inhibits differentiation of murine osteoblasts but has a limited role in osteoblast and osteoclast apoptosis. J. Immunol. 178 (6), 3379–3389. doi:10.4049/jimmunol.178.6.3379
Kristófi, R., and Eriksson, J. W. (2021). Metformin as an anti-inflammatory agent: a short review. J. Endocrinol. 251 (2), R11–r22. doi:10.1530/joe-21-0194
Lancioni, A., Pizzo, M., Fontanella, B., Ferrentino, R., Napolitano, L. M., De Leonibus, E., et al. (2010). Lack of Mid1, the mouse ortholog of the Opitz syndrome gene, causes abnormal development of the anterior cerebellar vermis. J. Neurosci. 30 (8), 2880–2887. doi:10.1523/jneurosci.4196-09.2010
Lazić Mosler, E., Lukač, N., Flegar, D., Fadljević, M., Radanović, I., Cvija, H., et al. (2019). Fas receptor induces apoptosis of synovial bone and cartilage progenitor populations and promotes bone loss in antigen-induced arthritis. Faseb J. 33 (3), 3330–3342. doi:10.1096/fj.201801426R
Lin, L., Huang, Z., Li, W., Liu, X., Li, X., Gao, S., et al. (2024). Mid1 promotes synovitis in rheumatoid arthritis via ubiquitin-dependent post-translational modification. Pharmacol. Res. 205, 107224. doi:10.1016/j.phrs.2024.107224
Lukač, N. (2020). Cellular and molecular mediators of subchondral bone resorption in arthritis (Croatian). PhD. Croatia: University of Zagreb, School of Medicine.
Ma, Y., Liu, H., Tu-Rapp, H., Thiesen, H. J., Ibrahim, S. M., Cole, S. M., et al. (2004). Fas ligation on macrophages enhances IL-1R1-Toll-like receptor 4 signaling and promotes chronic inflammation. Nat. Immunol. 5 (4), 380–387. doi:10.1038/ni1054
McInnes, I. B., and Schett, G. (2007). Cytokines in the pathogenesis of rheumatoid arthritis. Nat. Rev. Immunol. 7 (6), 429–442. doi:10.1038/nri2094
Monteiro, O., Chen, C., Bingham, R., Argyrou, A., Buxton, R., Pancevac Jönsson, C., et al. (2018). Pharmacological disruption of the MID1/α4 interaction reduces mutant Huntingtin levels in primary neuronal cultures. Neurosci. Lett. 673, 44–50. doi:10.1016/j.neulet.2018.02.061
Morell, M., Varela, N., and Marañón, C. (2017). Myeloid populations in systemic autoimmune diseases. Clin. Rev. Allergy Immunol. 53 (2), 198–218. doi:10.1007/s12016-017-8606-7
Namba, T., Ichii, O., Nakamura, T., Masum, M. A., Otani, Y., Otsuka-Kanazawa, S., et al. (2019). Altered morpho-functional features of bones in autoimmune disease-prone BXSB/MpJ- Yaa mice. Exp. Biol. Med. (Maywood) 244 (5), 333–343. doi:10.1177/1535370219832810
O' Reilly, L., Tai, L., Lee, L., Kruse, E. A., Grabow, S., Fairlie, W. D., et al. (2009). Membrane-bound Fas ligand only is essential for Fas-induced apoptosis. Nature 461 (7264), 659–663. doi:10.1038/nature08402
Ritchie, M. E., Phipson, B., Wu, D., Hu, Y., Law, C. W., Shi, W., et al. (2015). Limma powers differential expression analyses for RNA-sequencing and microarray studies. Nucleic Acids Res. 43 (7), e47. doi:10.1093/nar/gkv007
Rivero-Gutiérrez, B., Anzola, A., Martínez-Augustin, O., and de Medina, F. S. (2014). Stain-free detection as loading control alternative to Ponceau and housekeeping protein immunodetection in Western blotting. Anal. Biochem. 467, 1–3. doi:10.1016/j.ab.2014.08.027
Robin, N. H., Opitz, J. M., and Muenke, M. (1996). Opitz G/BBB syndrome: clinical comparisons of families linked to Xp22 and 22q, and a review of the literature. Am. J. Med. Genet. 62 (3), 305–317. doi:10.1002/(sici)1096-8628(19960329)62:3<305::Aid-ajmg20>3.0.Co;2-n
Rumble, J. M., Huber, A. K., Krishnamoorthy, G., Srinivasan, A., Giles, D. A., Zhang, X., et al. (2015). Neutrophil-related factors as biomarkers in EAE and MS. J. Exp. Med. 212 (1), 23–35. doi:10.1084/jem.20141015
Shen, S., Yang, Y., Shen, P., Ma, J., Fang, B., Wang, Q., et al. (2021). circPDE4B prevents articular cartilage degeneration and promotes repair by acting as a scaffold for RIC8A and MID1. Ann. Rheum. Dis. 80 (9), 1209–1219. doi:10.1136/annrheumdis-2021-219969
Stranges, P. B., Watson, J., Cooper, C. J., Choisy-Rossi, C. M., Stonebraker, A. C., Beighton, R. A., et al. (2007). Elimination of antigen-presenting cells and autoreactive T cells by Fas contributes to prevention of autoimmunity. Immunity 26 (5), 629–641. doi:10.1016/j.immuni.2007.03.016
Sun, L., Pham, T. T., Cornell, T. T., McDonough, K. L., McHugh, W. M., Blatt, N. B., et al. (2017). Myeloid-specific gene deletion of protein phosphatase 2A magnifies MyD88-and TRIF-dependent inflammation following endotoxin challenge. J. Immunol. 198 (1), 404–416. doi:10.4049/jimmunol.1600221
Takaki-Kuwahara, A., Arinobu, Y., Miyawaki, K., Yamada, H., Tsuzuki, H., Irino, K., et al. (2019). CCR6+ group 3 innate lymphoid cells accumulate in inflamed joints in rheumatoid arthritis and produce Th17 cytokines. Arthritis Res. Ther. 21 (1), 198. doi:10.1186/s13075-019-1984-x
Trockenbacher, A., Suckow, V., Foerster, J., Winter, J., Krauss, S., Ropers, H. H., et al. (2001). MID1, mutated in Opitz syndrome, encodes an ubiquitin ligase that targets phosphatase 2A for degradation. Nat. Genet. 29 (3), 287–294. doi:10.1038/ng762
Tu-Rapp, H., Hammermüller, A., Mix, E., Kreutzer, H. J., Goerlich, R., Köhler, H., et al. (2004). A proinflammatory role for Fas in joints of mice with collagen-induced arthritis. Arthritis Res. Ther. 6 (5), R404–R414. doi:10.1186/ar1205
Wang, F., Flanagan, J., Su, N., Wang, L. C., Bui, S., Nielson, A., et al. (2012). RNAscope: a novel in situ RNA analysis platform for formalin-fixed, paraffin-embedded tissues. J. Mol. Diagn 14 (1), 22–29. doi:10.1016/j.jmoldx.2011.08.002
Wei, Y., Li, W., Huang, J., Braunstein, Z., Liu, X., Li, X., et al. (2024). Midline-1 regulates effector T cell motility in experimental autoimmune encephalomyelitis via mTOR/microtubule pathway. Theranostics 14 (3), 1168–1180. doi:10.7150/thno.87130
Keywords: rheumatoid arthritis, myeloid lineage, midline 1, Fas, inflammation
Citation: Lukač N, Flegar D, Priselac S, Kelava T, Šućur A, Filipović M, Šisl D, Fadljević M, Radanović I, Katavić V, Zimmermann N, Grčević D and Kovačić N (2025) Midline 1 associated with Fas signaling enhances murine antigen-induced arthritis. Front. Cell Dev. Biol. 13:1451093. doi: 10.3389/fcell.2025.1451093
Received: 18 June 2024; Accepted: 17 April 2025;
Published: 15 May 2025.
Edited by:
Kang Tian, Dalian Medical University, ChinaCopyright © 2025 Lukač, Flegar, Priselac, Kelava, Šućur, Filipović, Šisl, Fadljević, Radanović, Katavić, Zimmermann, Grčević and Kovačić. This is an open-access article distributed under the terms of the Creative Commons Attribution License (CC BY). The use, distribution or reproduction in other forums is permitted, provided the original author(s) and the copyright owner(s) are credited and that the original publication in this journal is cited, in accordance with accepted academic practice. No use, distribution or reproduction is permitted which does not comply with these terms.
*Correspondence: Nataša Kovačić, bmF0YXNhLmtvdmFjaWNAbWVmLmhy