- 1Department of Nephrology, Children’s Hospital of Fudan University, Shanghai Kidney Development and Pediatric Kidney Disease Research Center, Shanghai, China
- 2State Key Laboratory of Genetic Engineering and National Center for International Research of Development and Disease, Institute of Developmental Biology and Molecular Medicine, Fudan University, Shanghai, China
- 3National Key Laboratory of Kidney Diseases, People's Liberation Army General Hospital, Beijing, China
Mutations in ZMYM2 lead to syndromic congenital anomalies of the kidney and urinary tract (CAKUT) in humans. Tbx18 is co-expressed with Zmym2 in mesenchymal compartment of developing mouse ureter, indicating a potential in vivo relevance of the TBX18–ZMYM2 protein interaction in ureter development. The presence of multiple phenotypes beyond the urinary system in CAKUT patients carrying ZMYM2 mutations suggests that ZMYM2 has extensive roles in various developmental processes. This study aims to comprehensively examine the multi-phenotypic consequence of ZMYM2 mutations, with a particular focus on the roles of ZMYM2 in embryonic development, late metanephros formation, and the reproductive, nervous and endocrine systems, in addition to its role in urinary system. Using a new Zmym2 mutant mouse model with an inserted piggyBac transposon (PB), we found that homozygous Zmym2 mutations resulted in severe growth retardation of embryos by embryonic day 9.5 (E9.5D) and lethality from E10.5D. Heterozygous mutations caused morphogenetic issues in the genitourinary system, including duplex kidneys, vesicoureteral reflux (VUR), and cryptorchidism. And these heterozygous mutants exhibited anxiety and aggressive-like behaviors, and glucose metabolism disorders. Additionally, Zmym2 mutations induced duplicated ureteric bud (UB) eruption and abnormal nephrogenic zone extension, contributing to duplex kidney formation. Reduced apoptosis in the nephric duct might have contributed to abnormal ureter-bladder connections, which could explain the observed cases of VUR. Notably, Tbx18 is co-expressed with Zmym2 in mouse kidney, reduced Tbx18 expression in Zmym2 mutants further supports the hypothesis that Zmym2 interacts with Tbx18 during kidney development. Zmym2 PB mouse is the first model to demonstrate roles of Zmym2 in neuroethology and endocrinology, extending its significant beyond genitourinary defects and embryonic development. Further investigation of these phenotypes in CAKUT patients carrying ZMYM2 mutations will enhance our understanding of their phenotypes and improve strategies for early diagnosis, monitoring, and treatment.
1 Introduction
Congenital anomalies of the kidney and urinary tract (CAKUT) are the most frequent birth defect (Loane et al., 2011; van der Ven et al., 2018a). Nearly 50% of chronic kidney disease (CKD) cases in children are caused by CAKUT, often progressing to end-stage kidney disease (ESKD) (Cirillo et al., 2023). Accurate diagnostic evaluation is pivotal to minimize kidney damage and prevent CKD. CAKUT encompasses a broad spectrum of phenotypes arising from defects in the development of urinary system. In murine models, the location and number of ureteric bud (UB) outgrowths and subsequent branching abnormalities lead to various CAKUT phenotypes, ranging from complete renal agenesis (RA) to more subtle defects, such as duplex or bifurcated ureters, vesicoureteral reflux (VUR), or other CAKUT subtypes (Blake and Rosenblum, 2014; Short and Smyth, 2020). Gene mutations or exposure to environmental risk factors disrupt the normal development processes, final, ultimately leading to CAKUT (Kolvenbach et al., 2023).
A substantial proportion of CAKUT cases have genetic basis. To date, approximately 54 monogenic genes have been identified as causative for CAKUT in humans, 135 genes are linked to monogenic multiorgan syndromes with facultative CAKUT (van der Ven et al., 2018b; Seltzsam et al., 2022). ZMYM2 (MIM: 602221) encodes a nuclear zinc finger protein that localizes to the nucleus (Kunapuli et al., 2006), and is part of a transcriptional complex functioning as a corepressor by interacting with various nuclear receptors (Gocke and Yu, 2008). Our previous studies reported that the heterozygous truncating mutations in ZMYM2 cause neurodevelopmental-craniofacial syndrome with variable renal and cardiac abnormalities (Connaughton et al., 2020). Multisystem phenotype caused by ZMYM2 heterozygosity in humans suggests extensive further roles in development. The phenotypes of CAKUT patients carrying ZMYM2 mutation may have been omitted due to insufficient assessment or a follow-up period that did not reach the age of onset of certain phenotypes.
The piggyBac (PB) transposon is a DNA transposon, that often disrupts gene expression upon insertion. The PB transposon mouse model serves as a valuable tool for studying CAKUT facilitating the investigation of pathogenic mechanisms, as well as the prevention, diagnosis and treatment of CAKUT (Ding et al., 2005; Wang et al., 2020). Using a newly generated Zmym2 mutant mouse model with an inserted PB transposon, this study aims to comprehensively examine the multi-phenotypic consequences of Zmym2 mutations, with a particular focus on the roles of Zmym2 in embryonic development, late metanephric formation, and the reproductive, nervous, endocrine systems, and urinary systems.
2 Materials and methods
2.1 Mice
All animal experiments were performed in accordance with protocols approved by the Animal Care and Use Committee of Children’s Hospital of Fudan University (NO. 2021-191). The Zmym2 mutant strain (080214049-HLA) was established on the FVB/N background, and maintained on 12/12-h light/dark cycles. In the Zmym2 PB allele, the PB insertion was mapped in the 15th intron of Zmym2 (Chr:14.57557590, Ensembl ID: ENSMUSG00000021945) (Figure 1). FVB/N strain, Hoxb7-mVnus fluorescent mice were used. Hoxb7-EGFP plasmid was a generous gift from Professor Frank Constatini (Columbia University, New York City, NY) (Wang et al., 2018). When the mice were 6–8 weeks old, they were housed in the same cage with a female to male ratio of 3:1. The female mice were observed for pregnancy at 8:00 a.m. The earliest time when a vaginal plug was observed was counted as E0.5D. After the vaginal plug was observed, the female mice were randomly divided into two groups.
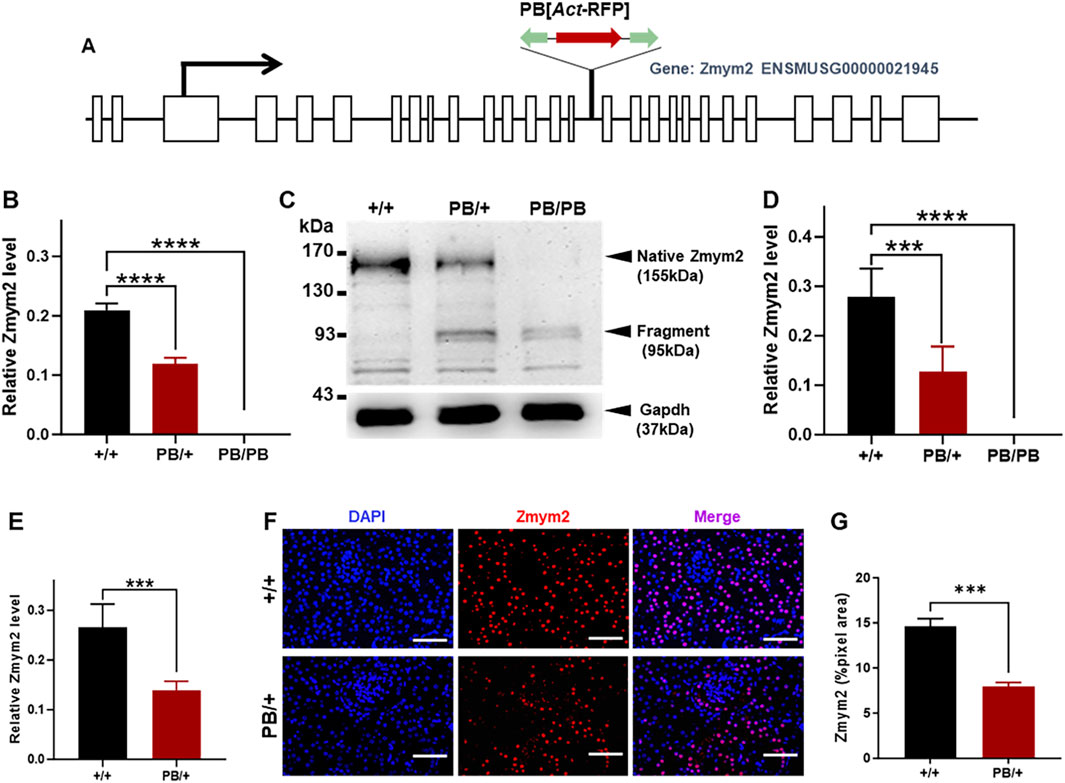
Figure 1. Characterization of the PB insertion in Zmym2 mutant mice. (A) Genomic structure of the Zmym2 PB allele. A PB [Act-RFP] transposon was inserted in the15th intron of Zmym2 on mouse chromosome 14, nucleotide 57,557,590. (B) After PB insertion, Zmym2 mRNA level detected by Realtime-PCR in E9.5D embryos was decreased about 50% in Zmym2 PB/+ group than that in Zmym2 +/+ group, and there was no Zmym2 mRNA expression in Zmym2 PB/PB group. (C, D) Western blots showed that PB transposon insertion resulted in a significant truncated mutation in Zmym2. The protein expression level of Zmym2 in E9.5D embryo was decreased or there was no Zmym2 expression after PB insertion. (E) Zmym2 mRNA expression was approximately 50% reduced in Zmym2 PB/+ kidney than that in Zmym2+/+ kidney. (F, G) Zmym2 was mainly expressed in the nuclear of tubular cells, and weakly expressed in the cells of glomeruli, Scale bars = 50 μm. The quantification results of fluorescence average optical density also confirmed the expression of Zmym2 in Zmym2 PB/+ mice is weaker than in Zmym2 +/+ mice. ***, P < 0.001; ****, P < 0.0001.
2.2 Genotyping polymerase chain reaction
The toes of neonatal mice at postnatal 7 days (P7D) or the yolk sac of E8.5D to E13.5D embryos were dissected from intercrossed Zmym2 PB/+ female mice and used to extract genomic DNA, followed by genotyping polymerase chain reaction (PCR). Offspring with the transposon inserted into the Zmym2 gene were genotyped using the primers P1 (5′-CTGAGATGTCCTAAATGCACAGCG-3′), P2 (5′-TCCAAAAGAGCTGGCATACTAAAGG-3′), P3 (5′-AGCACATTGTACTGTGTTCAGAGAG-3′). Zmym2 PB/PB and Zmym2 PB/+ were amplified by P1 and P3, which produced a 600 bp fragment. Wild type and Zmym2 PB/+ were amplified by P2 and P3, which yielded an 864 bp fragment (Supplementary Figure S1). PCR conditions were as follows: initial denaturation at 93°C for 90 s; 40 cycles of 93°C for 30 s, 57°C for 30 s, and 65°C for 2 min; and a final extension at 65°C for 10 min. Genomic DNA extracted from mouse toes was used as template.
2.3 Gross phenotypic analysis
Phenotypes of Zmym2 PB/+ and Zmym2 +/+ mice were assessed. The animals were anesthetized with CO2.The location, morphology, and number of kidneys, ureters, and bladders of mice in each group were observed under a fluorescence stereoscope. The pregnant mice in difficult groups from E8.5D to E13.5D were anesthetized by CO2, the dissection was performed layer by layer, and the embryos were removed and observed. The kidney primordia were separated under a fluorescence stereomicroscope to observe the number and location of ND and UB. The images were recorded under a microscope (Leica, Germany).
2.4 RNA-sequencing
The RNeasy Mini kit (QIAGEN, Germany) was used to extract the total RNA of E9.5D embryos from the Zmym2 PB/PB and Zmym2 +/+ mice. The sequencing data was filtered with SOAP nuke (v1.5.2), afterward clean reads were obtained and stored in FASTQ format. The clean reads were mapped to the reference genome using HISAT2 (v2.0.4), Bowtie2 (v2.2.5) was applied to align the clean reads to the reference coding gene set, then expression level of gene was calculated by RSEM (v1.2.12). Using Fragments Per Kilobase of exon model per Million mapped fragments (FPKM) algorithm for the expression of standardized, which counts by total exon fragments/[mapped reads (millions) × exon length (kb)], reflects the gene expression level. Essentially, differentially expressed genes (DEGs) analysis was performed using the DESeq2 (v1.4.5) (Abdi, 2007) with fold change ≥2 and false discovery rate (FDR) ≤ 0.001. To take insight to the change of phenotype, GO1 enrichment analysis of annotated different expressed gene was performed by Phyper2 based on Hypergeometric test. The significant levels of terms and pathways were corrected by Q-value (=FDR) with a rigorous threshold (Q-value ≤0.05) by Bonferroni (Tan et al., 2021).
2.5 Immunofluorescence
The E12.5D kidney primordia were isolated and fixed in formaldehyde overnight. After washing with 0.3% Triton X-100/PBS, tissues were blocked by 5% donkey serum (Jackson) and placed on a shaker at 4°C overnight. The tissues were incubated with anti-Caspase 3 antibody (CST, #9662, 1:400), then placed in a shaker at 4°C overnight. The tissues were washed with PBS and incubated with Cy5-donkey anti-rabbit antibody (Jackson, 1:1,000). DAPI (1:1,000) was used to stain the nuclei. Six kidney primordia from each group were used, and the samples were mounted with 50% glycerol/PBS. Immunofluorescence done in the sections of kidney tissues was as previously described in the kidney primordia. Anti-Zmym2 antibody (NOVUS, #NB100-56490, 1:400) and Anti-Tbx18 antibody (4A Biotech, # ABIN522536, 1:400) were used as primary antibodies. Images were taken under a confocal microscope (Leica) and analyze date using ImageJ software.
2.6 Behavioral analyses
To evaluate anxiety, an open field test was conducted. Mice, approximately 8 weeks old, were acclimatized in the experimental room for over 1 h before the test began. The open field consisted of a 40 cm × 40 cm area enclosed by 40 cm high wooden walls painted white. Testing occurred between 13:00 and 17:00, with the arena cleaned between each mouse’s trial. Each mouse was placed in the center of the square, and its behavior was recorded on videotape for 15 min. The duration spent in both the peripheral zone and the center, as well as the distance traveled and instances of rearing in either area, were analyzed using a computerized technique with Etho Vision 3.0 (Noldus, Wageningen, Netherlands) (Walsh et al., 2017).
For the aggressive behavior test, the resident–intruder paradigm Koolhaas et al. (2013) was employed. Each male mouse was isolated for 1 h prior to testing and was evaluated in his home cage (acting as the resident) against a group-housed male intruder (four mice per cage). The behavior was recorded on video for up to 15 min. Key metrics included the latency to the first biting attack and the number of biting attacks during the subsequent 5 min following the first attack. If no biting behavior was observed for 10 min, the test was terminated, and the latency was recorded as 10 min. Testing was conducted between 12:00 and 16:00 and repeated three times for each male at 3-day intervals, ensuring that each male encountered a novel intruder during each trial. To assess locomotor activity, the duration of walking and the number of rearings were recorded for the 5 min following the first attack in the initial trial. If no biting behavior occurred, the latter 5 min of the 10-min period recorded was used to evaluate locomotor activity.
2.7 Glucose homeostasis
Blood glucose concentrations of 0-, 7-, 14-, 21-, 30-, 60-, 90- and180-day-old mice were measured using a glucometer, typically taken in either a random or fasted state. Oral glucose tolerance tests (OGTT) were performed in 90-day-old Zmym2 PB/+ mutant mice and Zmym2 +/+ littermate control mice as previously described (Kennard et al., 2021). Serum insulin concentrations in a random or fasted state and 30, 60, and 120 min after oral glucose application were quantified by ELISA (Abcam, #ab285341).
2.8 Statistical analysis
Numerical data are presented as the mean ± standard deviation. Statistical analysis was performed using GraphPad Prism version 10.0 and SPSS 20.0 statistical software. Two-sided unpaired t tests were used for between-group data, and χ2 tests were used for assessing count data. The significance level was set at p < 0.05, p > 0.05 representing no statistically significant distinction, all n = 6 at least in each group. In all figures, a single asterisk indicates p < 0.05, while double, triple, and quadruple asterisks indicate p < 0.005, p < 0.0005, and p < 0.0001, respectively.
3 Results
3.1 Genetic characterization of Zmym2 PB mutant mice
The PB transposon was inserted into the 15th intron of Zmym2 (Figure 1A). In E9.5D embryos, quantitative PCR analysis showed that Zmym2 mRNA levels were reduced by nearly 100% in homozygous mutants (Zmym2 PB/PB) compared to wild-type littermates (Zmym2 +/+) and by approximately 50% in heterozygous mutants (Zmym2 PB/+) (Figure 1B). Western blot analysis demonstrated that PB transposon insertion resulted in significant truncation of the Zmym2 protein. In the whole embryos tissues at E9.5D, a truncated protein band (95 kDa) was detected in both Zmym2 PB/+ and Zmym2 PB/PB lanes. Native Zmym2 protein (155 kDa) expressed in Zmym2 PB/+ and Zmym2 +/+ groups, and the protein level of native Zmym2 in Zmym2 PB/+ group was reduced to approximately 50% of that in the Zmym2 +/+ group. Notably, the native Zmym2 band is absent in the Zmym2 PB/PB group (Figures 1C, D).
Furthermore, quantitative PCR analysis and immunofluorescence were performed to assess Zmym2 expression levels and distribution in the kidneys of mutant mice. Zmym2 mRNA expression in Zmym2 PB/+ kidneys was approximately 50% of that in Zmym2 +/+ kidneys (Figure 1E). In kidney tissue sections, Zmym2 was predominantly localized in the nuclei of tubular cells, with weak expression detected in glomerular cells. Fluorescence quantification results were consistent with the quantitative PCR results, confirming that Zmym2 expression was lower in Zmym2 PB/+ kidneys than that in Zmym2 +/+ kidneys (Figures 1F, G).
3.2 Zmym2 PB/PB mouse embryos show developmental abnormality and early lethality
Zmym2 PB/+ mice were mated and their neonatal offspring were genotyped by PCR amplification on postnatal 7 days. No Zmym2 PB/PB mice were identified across more than three consecutive generations (Supplementary Figure S1A), indicating that Zmym2 PB/PB embryos are homozygous lethal. This finding is consistent with a previous study and the Mouse Genome Informatics database (MGI:5706016) (Graham-Paquin et al., 2023). To investigate the role of Zmym2 in embryonic development, embryos were collected at different stages. Zmym2 PB/PB embryos were present at mendelian ratios until E9.5D (Figure 2A). However, by E10.5D, embryonic resorption was observed, and no Zmym2 PB/PB embryos were recovered, confirming embryonic lethality (Figure 2B). At E9.5D, most Zmym2 PB/PB embryos exhibited gross phenotypic abnormalities, and a statistically significant reduction in length was noted (Figures 2C, D). Zmym2 PB/PB embryos seemly appeared reduction in length at E8.5D, but there was no statistical difference (Supplementary Figure S1C).
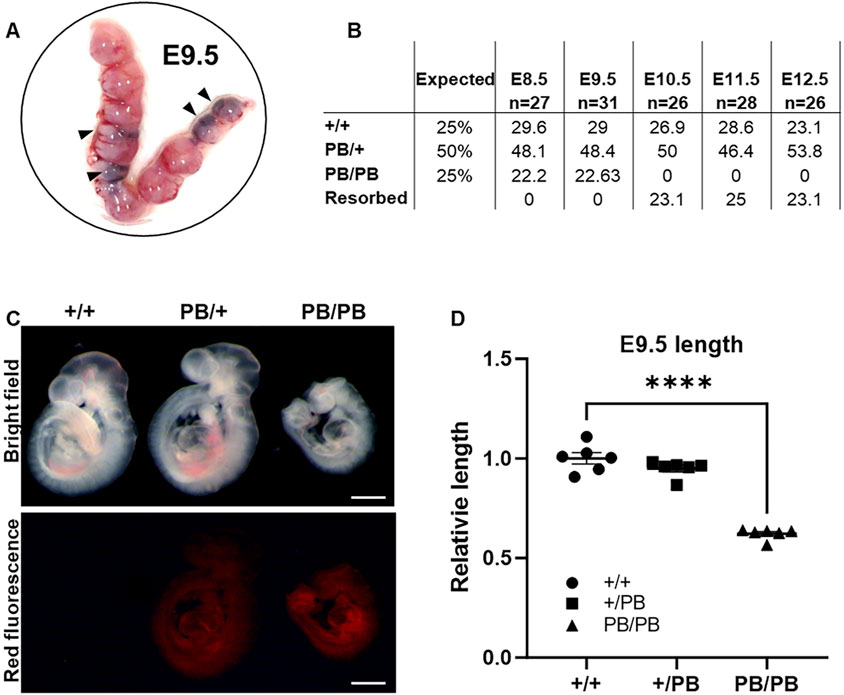
Figure 2. Zmym2 PB mice is homozygous embryo lethal, embryos show developmental delays by E9.5D and lethality from E10.5D to E12.5D. (A) Representative photographs of gravid uteri harvested from Zmym2 PB/+ × Zmym2 PB/+ pregnancies at E9.5D. Arrowhead denote fetal growth retardation. (B) Embryonic viability by genotype from E8.5D to E12.5D compared to the expected Mendelian ratios from Zmym2 PB/+; Zmym2 PB/+ crosses. (C) Images taken of E9.5D Zmym2 PB/PB embryos representing fetal growth retardation. Scale bars = 500 μm. (D) Relative length of embryos (crown to tail) normalized by litter as a ratio to average Zmym2 +/+ length. ****, P < 0.0001.
In contrast, Zmym2 PB/+ mice are viable and fertile. We monitored the general condition (weight, fur, activity and appetite) and survival rates among different experimental groups over a period of 12 weeks (Supplementary Figures S1D, E). The weight of Zmym2 PB/+ mice was normal, and their fur, dietary habits, and activity levels were basically the same as those of Zmym2 +/+ mice. However, a higher proportion of the Zmym2 PB/+ than Zmym2 +/+ mice died throughout the observation period (P60D-P180D).
3.3 Zmym2 PB/+ mice display kidney and urinary malformations, but with normal renal function
A comprehensive analysis of the urinary system was performed in 60 newborn Zmym2 PB/+ mice. Among these, 28 displayed CAKUT phenotypes: 13 (21.7%) had unilateral duplex kidneys (DK), 9 (15.0%) had unilateral hydronephrosis (HN) and 6 (10.0%) had unilateral RA. No significant differences in phenotype distribution were observed between sexes or between left and right kidneys (Figures 3A, C). VUR testing was conducted in all mice (Figure 3B), revealing that unilateral VUR was present in 13 (46.4%) of the 28 CAKUT mice: 4 cases from DK group, 6 cases from HN group, and 3 cases from RA group (Figure 3C). No obvious CAKUT phenotypes were detected in Zmym2 +/+ mice. Histopathological analysis of the newborn Zmym2 PB/+ mice was performed. The structure and morphology of the glomeruli and kidney tubules appeared normal in mice with DK. However, in mice with HN, the numbers of glomeruli and tubules were reduced.
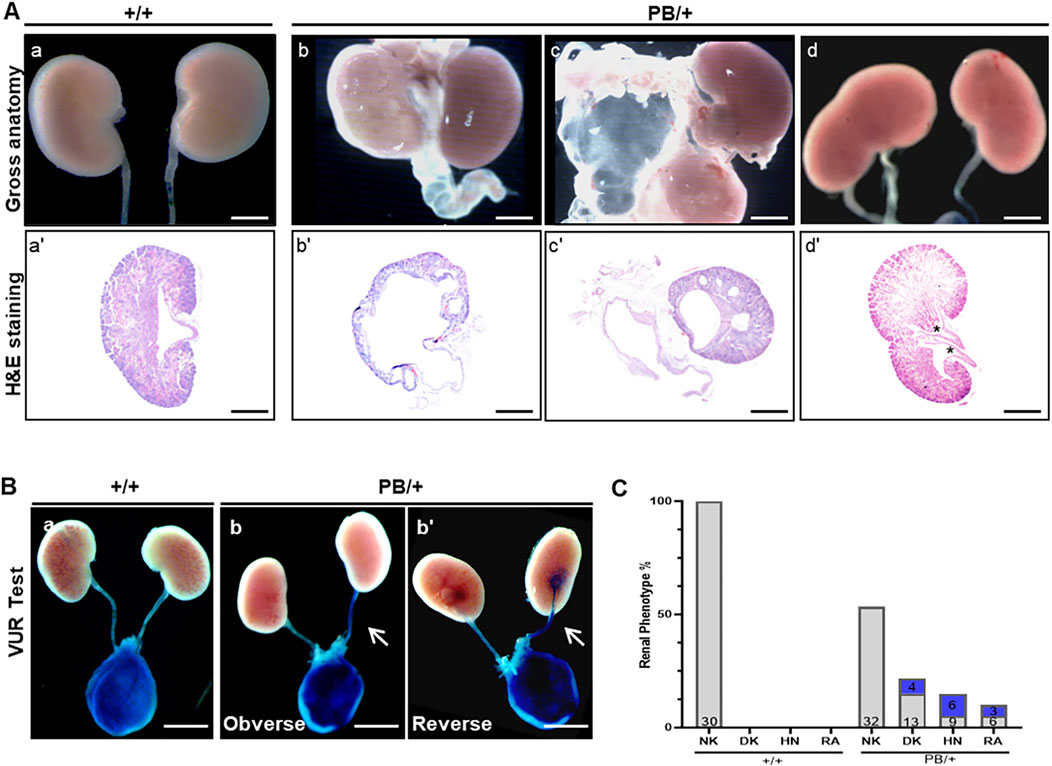
Figure 3. Kidney and urinary tract defects in Zmym2 PB mice. (A) Overview images and H&E staining of the kidneys of newborn mice. Various urinary malformations observed in Zmym2 PB/+ mice. (a, a’) normal kidney; (b, b’) severe hydronephrosis and hydroureter; (c, c’) renal agenesis, and duplex kidney (d, d’). Duplex kidney is defined by double renal pelvises (asterisk). (B) Representative images of the vesicoureteral reflux. (C) Percentages of various urinary malformations. The numbers in the blue filled boxes represent the number of VUR. The numbers at the bottom of each column of the bar chart represents the total numbers of data in each group. Scale bars, 2 mm in (A) (a, b, c, d) and (B); 250 μm in (A) (a’, b’, c’, d’). NK, normal kidneys; DK, duplex kidneys; RA, renal agenesis; HN, hydronephrosis; VUR, vesicoureteral reflux.
To determine whether the Zmym2 mutation affects kidney function, blood urea nitrogen (BUN) and serum creatinine (Scr) levels were measured. Both BUN and Scr levels remained stable over a 180-day period in Zmym2 +/+ and Zmym2 PB/+ mice, with no statistically significant differences between the two groups (Supplementary Figure S2A). Furthermore, H&E staining revealed no structural abnormalities in the glomeruli of either Zmym2 PB/+ or Zmym2 +/+ mice (Supplementary Figure S2B).
3.4 Morphology of testis and mature epididymal sperm from Zmym2 PB/+ mice
The morphology of the testis, seminal vesicles, and caudal epididymis was examined. Most male adult Zmym2 PB/+ mice (77.78%, 7/9) exhibited seminal vesicle atrophy (Figure 4A), with a 32% reduction in seminal vesicle weight compared to Zmym2 +/+ controls (Figure 4B). Additionally, some Zmym2 PB/+ mice displayed unilateral cryptorchidism (22.22%, 2/9), bilateral cryptorchidism with hydrocele (11.11%, 1/9) or intratesticular cysts (11.11%, 1/9). The morphology of mature epididymal sperm in Zmym2 PB/+ mice was normal, with sperm heads exhibiting typical morphology.
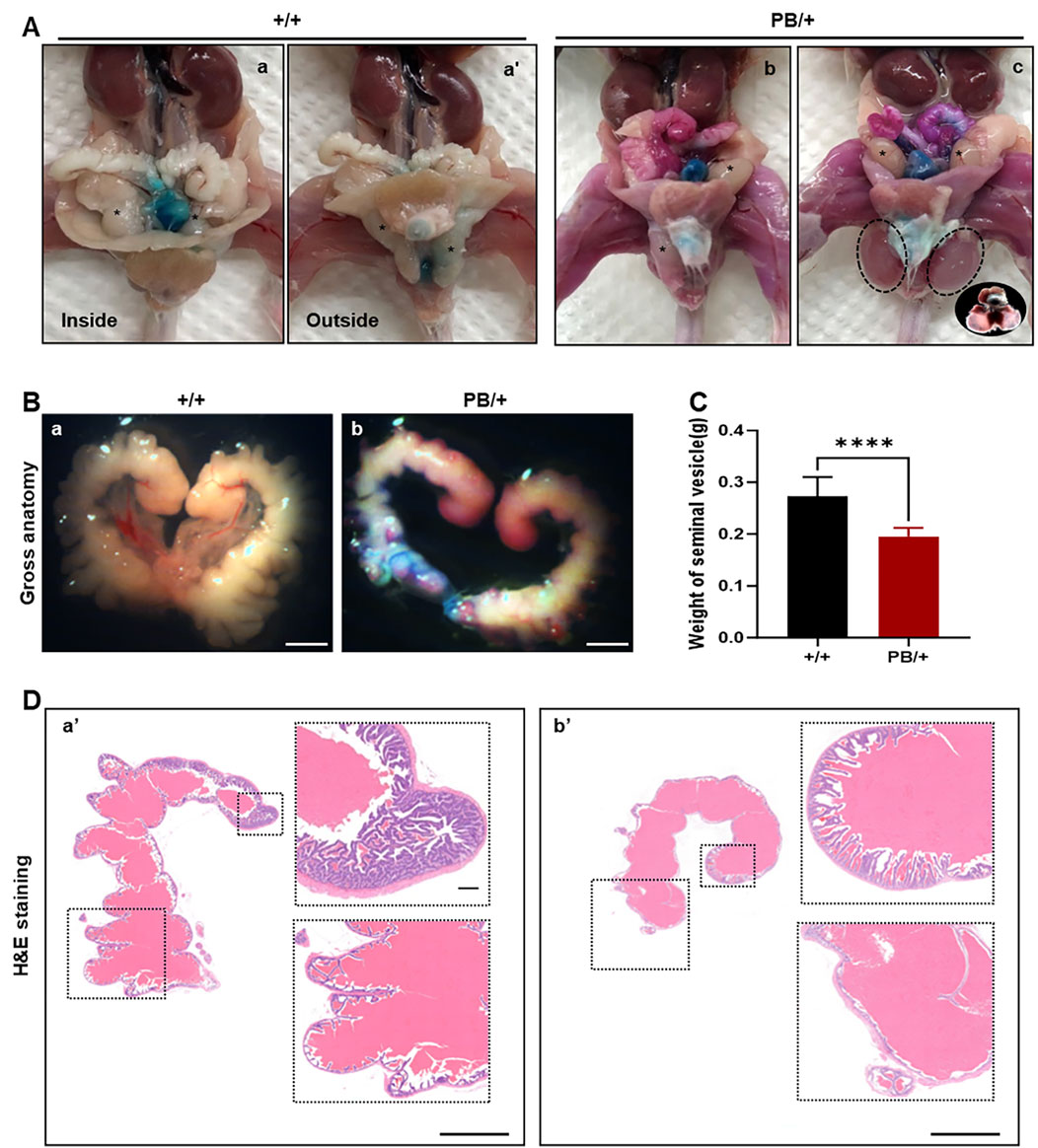
Figure 4. The abnormal manifestations of testis, seminal vesicles and caudal epididymis in Zmym2 PB mice. (A) Gross anatomy of genital system of adult male mice. (a, a’) normal position of testis (asterisk) in Zmym2 +/+ mice, unilateral (b) and bilateral (c) cryptorchidism with hydrocele (circle) in Zmym2 PB/+ mice. (B) Gross phenotype of seminal vesicle atrophy and a 32% decrease in seminal vesicle weight in Zmym2 PB/+ mice (C). (D) H&E staining of seminal vesicle. Mucosal folds of seminal vesicle in Zmym2 PB/+ mice disappeared or reduced compared with Zmym2 +/+ mice. Scale bars, 2 mm in C (a, b); 250 μm in D (a’, b’).
To assess the impact of the Zmym2 mutation on sperm quantity and quality, sperm counts were compared between Zmym2 PB/+ and Zmym2 +/+ mice (Table 1). No significant difference in total sperm number in the epididymis. However, the average path velocity (VAP), straight-line velocity (vSL) and curvilinear velocity (vCL) were significantly reduced in Zmym2 PB/+ mice (p < 0.05). The percentage of progressive mobile sperm (level a and b) was slightly lower in the Zmym2 PB/+ group (p = 0.14), whereas the percentage of sperm with slow velocity (level c) was significantly higher (p < 0.05). These findings indicate that sperm motility is impaired in Zmym2 PB/+ mice due to the Zmym2 mutation.
Despite the observed morphological abnormalities in the testis, seminal vesicles, and epididymis, as well as changes in sperm motility Zmym2 heterozygosity did not appear to affect male fertility. Fertility was assessed by continuously mating Zmym2 +/+ and Zmym2 PB/+ males with 8-week-old wild-type females at a 1:2 male-to-female ratio. To control for genetic background differences, females from different strains (S129 and C57BL/6) were used. No significant differences were observed between the two groups in terms of the ability to induce vaginal plugs or achieve pregnancy in wild-type females. Furthermore, the mean litter size was nearly identical in both groups (Supplementary Table S1), indicating that the Zmym2 mutation does not affect vaginal plug formation or pregnancy rate.
3.5 Zmym2 PB/+ mice demonstrated anxiety and aggressive like behaviors with no apparent abnormalities in the craniofacial structure
The craniofacial morphology, gross brain structure, and histopathology (H&E staining) of brain tissue were examined in Zmym2 PB/+ and Zmym2 +/+ mice. No craniofacial abnormalities were detected in either genotype (Figures 5A–C). Behavioral phenotypes were evaluated in Zmym2 PB/+ mice. Anxiety levels were assessed using the open-field test. Male Zmym2 PB/+ mice were significantly more active than male Zmym2 +/+ controls during the first 15 min of the test and spent less time in the center of the chamber (Figures 5D–G) suggesting increased anxiety-like behavior. However, no significant difference in anxiety levels was observed between female Zmym2 PB/+ and Zmym2 +/+ mice. Aggressive behavior, was assessed using the resident-intruder paradigm. The latency to the first biting attack did not differ between male Zmym2 PB/+ and Zmym2 +/+ mice (Figure 5H). The frequency of biting attacks was similar between genotypes during the first and second trials. However, in the third trial, the frequency of biting attacks was significantly higher in Zmym2 PB/+ mice than in Zmym2 +/+ mice (Figure 5I) indicating that Zmym2 PB/+ mice exhibited increased aggression following repeated encounters with intruders. Locomotor activity was evaluated by recording walking duration and the number of rearings during the first trial. No significant differences were observed between for these measures Zmym2 PB/+ and Zmym2 +/+ mice for either measure (Figures 5J, K).
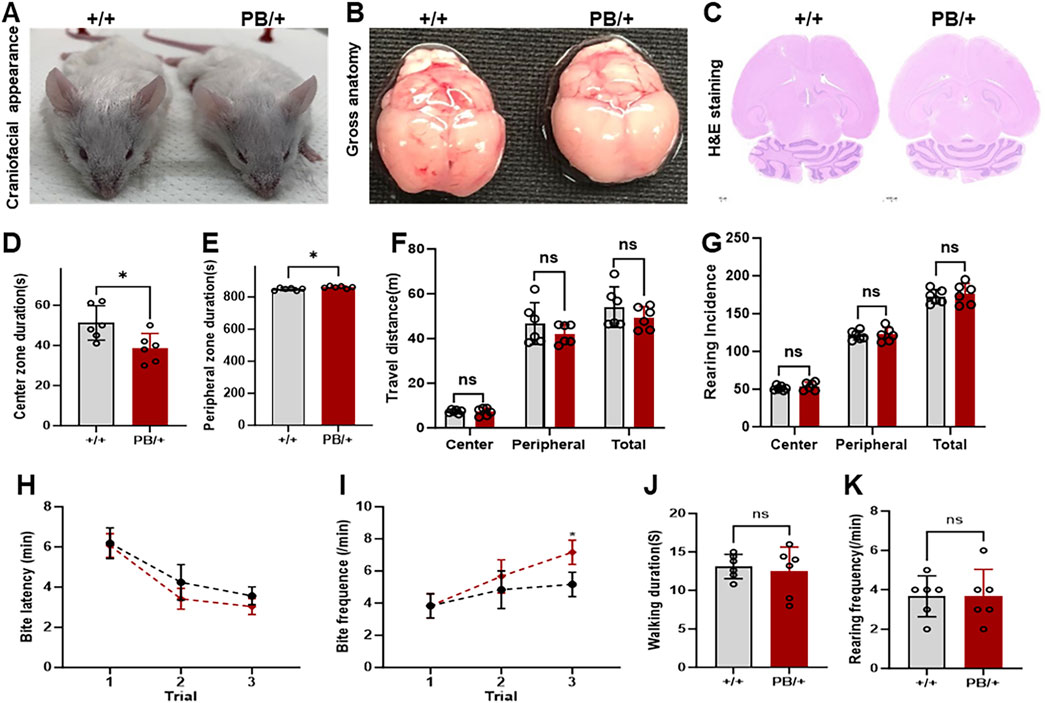
Figure 5. Anxiety and aggressive-like behaviors in Zmym2 PB/+ mice occur without obvious abnormalities in craniofacial structure. (A) General craniofacial appearance observation of adult mice. (B) Overview images of whole brain tissue. (C) HE staining of the largest coronal section of brain tissue. (D–G) Analysis of anxiety. Each mouse was placed in the center of the square (open field) and its behavior was recorded for 15 min. Duration in center zone (D) and peripheral zone (E), (F) distance traveled and (G) incidence of rearings of male adult Zmym2 PB/+ and Zmym2 +/+ mice. (H–K) Analysis of aggressive behavior. The resident–intruder paradigm was used to assess inter-male aggression. Latency (H) and frequency (I) of biting attack during 5 min of the first aggressive behavior test. *, p < 0.05, n = 6 for each experimental group, each column and vertical bar represent the mean and standard deviation (SD) in (D–G, J, K). Each symbol and vertical bar represent the mean and SD in (H, I). ns, no significant difference.
3.6 Disturbance in glucose homeostasis of Zmym2 PB/+ mice
ZMYM2 plays a critical role in embryogenesis, potentially by regulating gene expression and cellular functions essential for development (Graham-Paquin et al., 2023). To further investigate the role of Zmym2 in embryonic development and understand the lethality of Zmym2 PB/PB embryos, mRNA was were extracted from the E9.5D embryos of Zmym2 +/+ and Zmym2 PB/PB mice for RNA-sequencing (RNA-seq). The analysis identified 342 DEGs between wild-type and homozygous mutant mice, including 221 upregulated genes and 121 downregulated genes. These DEGs were categorized and enriched for functions related to DNA-bind transcription factor activity, nervous system development and so on. Notably, the most significantly downregulated DEGs associated with Zmym2 mutation were linked to maturity-onset diabetes of the young (MODY). RNA-seq profiles between Zmym2 +/+ and Zmym2 PB/+ embryos highlight the consistent results that MODY associated genes are the most significantly downregulated DEGs (Figures 6A, B).
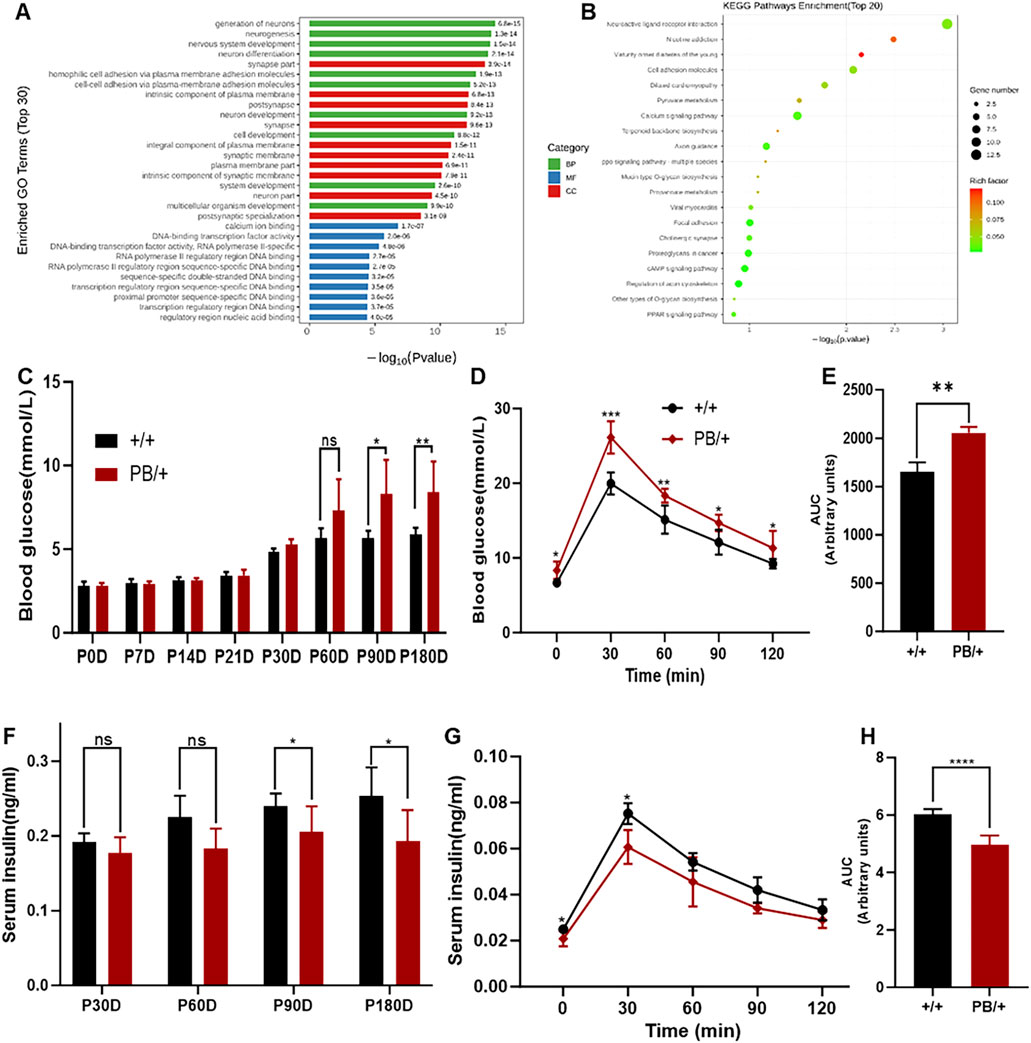
Figure 6. RNA-seq analysis and glucose homeostasis disturbance of Zmym2 PB/+ mice. (A) Gene Ontology (GO) term enrichment analysis. Significantly enriched GO terms were selected based on a Q-value means false discovery rate (FDR) < 0.05. GO terms of the categories of biological processes, cellular components, and molecular functions are depicted in red, green, and blue, respectively. (B) Bubble plots of the GO enrichment of DEGs in the E12.5D embryos FDR or adjusted P-value. Rich ratio is the ratio of the signaling pathway of enrichment on the number of differentially expressed genes and signaling pathways of all the genes. (C) Random blood glucose of Zmym2 PB mice at different ages (0, 7, 14, 21, 30, 60, 90 and 180 days). (D) Oral glucose tolerance test of 90-day-old mice. (E) Area under the glucose curve (AUC) during oral glucose tolerance test. (F) Random serum insulin levels of Zmym2 PB mice at different ages (30, 60, 90 and180 days). Serum insulin concentrations (G) and area under the insulin curve (H) during oral glucose tolerance test in 90-day-old mice. Data represent mean ± SD. *, p < 0.05; **, p < 0.01; ***, p < 0.001; ****, p < 0.0001; n = 6 for each experimental group. BP, Biological processes; CC Cellular components; MM, Molecular functions.
Subsequent glucose metabolism tests in Zmym2 PB/+ mice revealed progressive disturbances in glucose homeostasis until 90 days of age, and then stabilizing (Figure 6C). At 90 days, both random and fasting blood glucose levels were significantly elevated in Zmym2 PB/+ mice and remained stable throughout the study. During the OGTT at 90 days, blood glucose concentrations in Zmym2 PB/+ mice were significantly higher than those in Zmym2 +/+ mice at all measured time points. The area under the glucose curve (AUC) in 90-day-old Zmym2 PB/+ mice was significantly greater than that in Zmym2 +/+ mice (Figures 6D, E). Additionally, random serum insulin levels in Zmym2 PB/+ mice were significantly lower starting from P90D (Figure 6F). During the OGTT performed with 90-day-old mice, serum insulin levels in Zmym2 PB/+ mice were significantly elevated at 30 min post-glucose administration (Figure 6G). However, the AUC for insulin in Zmym2 PB/+ mice was significantly lower than that in Zmym2 +/+ mice. Between 60 and 90 min after glucose administration, AUC insulin levels in Zmym2 PB/+ mice were comparable to those in Zmym2+/+ mice (Figure 6H). The pancreatic morphology and histological appearance of Zmym2 PB/+ mice were unchanged compared to those of Zmym2 +/+ mice (Supplementary Figure S3).
3.7 Zmym2 mutation induces duplicated UB budding and abnormal expression of Tbx18 in kidney
Duplex kidney was the most frequently observed urinary system abnormality in Zmym2 PB/+ mice, occurring in 13 of 28 cases (46.4%). Urinary system malformations originate early in embryonic development, and duplicated UB formation is associated with duplex kidney in mice. To examine the effect of Zmym2 mutation on UB budding, kidney primordia were isolated at E12.5D from Zmym2 PB/+ and Zmym2 +/+ mice. Duplicated UBs were observed in nine of 36 (25.0%) Zmym2 PB/+ samples, whereas no aberrant UB formation occurred in Zmym2 +/+ mice (Figure 7A).
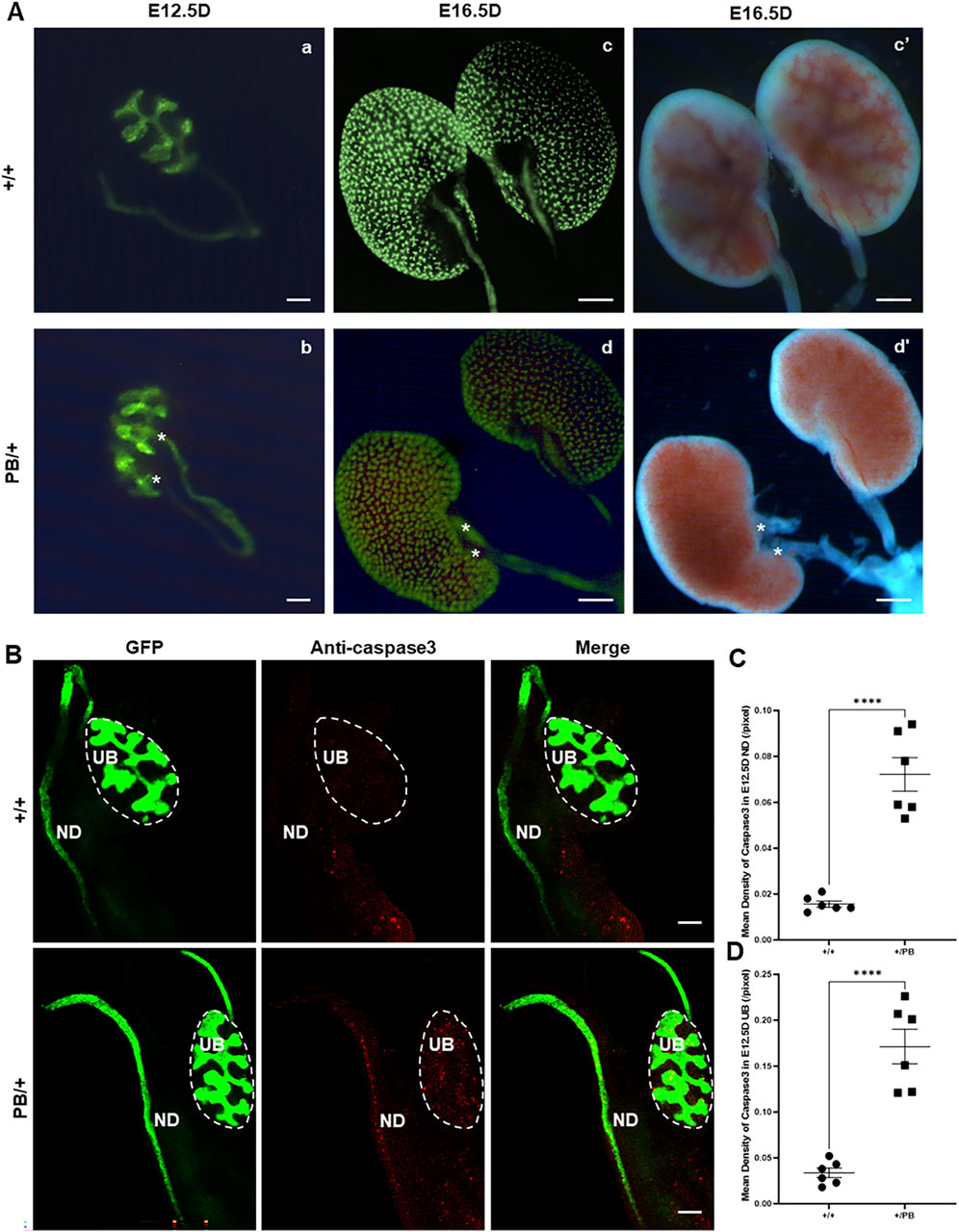
Figure 7. Duplicated UB budding and abnormal apoptosis in Zmym2 PB/+ kidney Primordia. (A) Observation of embryonic kidney development. Two ureteric buds (UBs) formed from one nephric duct (ND) in E12.5D Zmym2 PB/+ kidney primordia, asterisk shows duplicated UBs (b); unilateral duplicated kidneys accompanied with double ureters (red asterisk) in E16.5D Zmym2 PB/+ mice (d and d’). (B) Anti-Caspase3 staining in UB and ND tissues by immunofluorescence. (C, D) Each value of mean density of Caspase3 in E12.5D UB and ND is expressed as the mean ± SD. ****, p < 0.0001. Scale bars, 1 mm in A (c, d, c’, d’); 200 μm in (A) (a, b) and (B). UB, Ureteric Bud, ND, Nephric Duct.
Defects in apoptosis can contribute to various CAKUT phenotypes. Apoptosis in the UB is crucial for normal UB growth and subsequent branching morphogenesis. A wave of apoptosis in the ND that is likely necessary for separating the ureters from the ND (Mendelsohn et al., 2009). The number of apoptotic cells in the ND and UB was compared, revealing a significant increase in caspase3 positive apoptotic cells in these regions in Zmym2 PB/+ mutants (Figures 7B–D), which may contribute to the development of VUR and other CAKUT phenotypes. Previous proteomic analyses identified ZMYM2 as endogenous binding partner of TBX18 in 293 and A549 cells. Tbx18 is co-expressed with Zmym2 in the mesenchymal compartment of the developing ureter in mice, and mutations in TBX18 and in ZMYM2 have recently been linked to CAKUT, supporting the functional relevance of TBX18 and ZMYM2 interaction in ureter development (Ludtke et al., 2022). To investigate the effect of Zmym2 mutation on Tbx18 expression in kidney, immunofluorescence staining and western blot were performed to assess co-localization and expression changes in the kidney tissue. Zmym2 and Tbx18 were primarily co-localized in kidney interstitial cells, with particularly high expression in the nephrogenic zone(Figure 8A). Zmym2 PB/+ kidneys exhibited discontinuous nephrogenic zones, which was consistent with the formation of multiple UBs. In comparing to Zmym2 +/+ group, Tbx18 expression in Zmym2 PB/+ kidney tissue was decreased approximately 50% (Figures 8B, C).
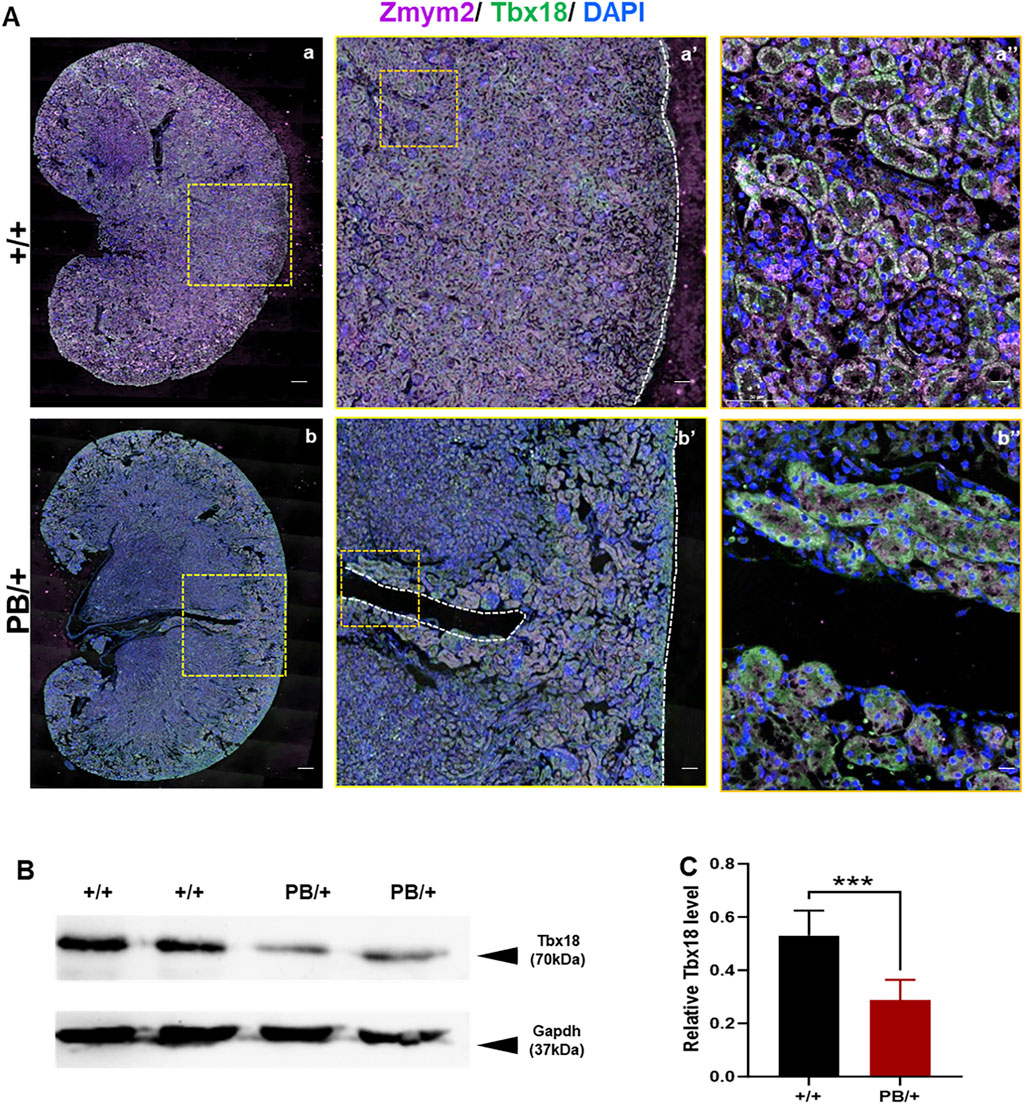
Figure 8. Abnormal expression of Tbx18 in mouse kidney induced by Zmym2 mutant related to mouse kidney development. (A) Immunofluorescence staining of Zmym2 and Tbx18 in the renal tissue of Zmym2 PB mice. Full image of kidney in a, b; focused observation of kidney nephrogenic zone in a’, b’, white dashed line indicates nephrogenic zone; enlarged image to clearly depict subcellular localization in a’’, b’’. Scale bars, 200 μm in a, b; 50 μm in a’, b’; 10 μm in a’’, b’’. (B, C) Western blots showed that the Tbx18 protein expression in kidney tissues was reduced approximately 50% in Zmym2 PB/+ group, compared to Zmym2 +/+ group. ***, p < 0.001.
4 Discussion
In our previous study (Connaughton et al., 2020), a mouse model was generated to replicate the frameshift mutation identified in exon 3 of patient GM1-21 (p. Gly257* [c.766_767dupGT]) using CRISPR-Cas9 gene targeting approach. Zmym2 +/− mice exhibited a spectrum of CAKUT-like defects, including hydroureter, duplex and cystic kidneys, and VUR. Immunofluorescence analysis of E18.5D kidneys without overt malformations showed normal nephrogenesis and branching morphogenesis in Zmym2 +/− animals. No additional phenotypes were observed in Zmym2 +/− mice. In this study, Zmym2 PB mice was successfully generated using PB transposon-based insertional mutagenesis, revealing that Zmym2 was truncated following PB insertion. Zmym2 PB mice exhibited multiple developmental malformations of the urinary system predominantly presenting as duplex kidneys. Duplicated UB formation during early metanephric development and abnormal extension of the nephrogenic zone deep into the kidney contributed to the formation of duplex kidneys. In addition to the urinary system defects, Zmym2 PB/+ mice exhibited developmental abnormalities in the reproductive, nervous, endocrine, and other systems, encompassing a broad spectrum of syndromic CAKUT phenotypes observed in clinical cases. The genetic, clinical, and pathomorphological characteristics of the Zmym2 PB mice were specified, making this a unique and valuable animal model to studying syndromic CAKUT pathogenesis, prognosis and outcomes. To our knowledge, this is the first Zmym2 mutant mouse model in which a single -gene mutation resulted in a wide range of syndromic CAKUT phenotypes similar to those observed in human carrying ZMYM2 mutations. Furthermore, this is first reported Zmym2 truncated mutant mouse model in literature, highlighting both embryonic lethality and abnormalities in kidney primordia.
ZMYM2 is a newly identified factor involved in DNA methylation patterning in early embryonic development (Graham-Paquin et al., 2023). Zmym2 PB homozygous mutants are embryonic lethal, while Zmym2 PB/+ mice are viable and fertile, and capable of normal growth. However, a small proportion of the Zmym2 PB/+ mice died after P60D, with 10.7% of these mice dying by P180D. At the time of death, no significant deterioration in kidney function was observed suggesting that extra-renal factors may be the primary cause. To further investigate the role of Zmym2 in embryonic development and lifespan, RNA-Seq was performed on E9.5D embryos from Zmym2+/+ and Zmym2 PB/PB groups. RNA-Seq data provided unexpected findings, revealing a direct mechanistic link between Zmym2 mutation and MODY. Similar to clinical observations in MODY patients, Zmym2 heterozygous mutant mice exhibited chronic but mild hyperglycemia, with blood glucose levels progressively increasing until 90 days of age. Additionally, these mutant mice displayed significantly reduced fasting serum insulin concentrations and diminished insulin secretory capacity. Due to the stable and mild phenotype, life expectancy was not affected in heterozygous mutant mice before P180D, whereas homozygous mutation was lethal by E10.5D. These findings suggest that some phenotypes may develop progressively with age, emphasizing the importance of considering patient age to improve the diagnostic rates, particularly in younger children. The discovery of HNF1β gene mutations as a cause of developmental kidney disease originally emerged from studies on MODY. HNF1B mutations are associated with CAKUT, a slowly progressive decline in kidney function, pancreatic abnormalities, diabetes, and neurological deficits, among other phenotypes (Clissold et al., 2015). Investigating the genetics and molecular pathways of ZMYM2 is essential for understanding both renal and extra-renal phenotypes, as well as for identifying potential areas for future research on ZMYM2-associated diseases, particularly those involving signaling molecule defects.
Sperm motility is a key parameter in assessing normal semen quality, and men with impaired sperm motility have a reduced likelihood of fertilization, which can lead to infertility. In addition to pathological factors such as radiation and oxidative stress, genetic disorders significantly contribute to sperm dysfunction. ZMYM2 mutations have been linked to abnormal development of the male reproductive system, and the de novo or mosaic occurrence of these mutations strongly suggest that heterozygous truncating mutations in ZMYM2 convey infertility or interfere with germline transmission (Connaughton et al., 2020). In this study, Zmym2 mutations resulted in structural abnormalities in the male reproductive system and reduced sperm motility, however, fertility was not significantly affected. Several factors may account for the discrepancies between human clinical findings and our results (Mao et al., 2016). Notably, genetic background related to reproductive functions differ significantly between humans and mice. Mice generally exhibit higher reproductive capacity than humans. Additionally, men with ZMYM2 mutation may be more susceptible to pathological factors and lifestyle influences, including other genetic disorders, genital tract infections, environmental pollutants, and smoking. Although Zmym2 mutations alone did not induce male infertility in mice, they led to structural abnormalities in the reproductive system and impaired sperm motility, suggesting that ZMYM2 may be one of the genes involved in male infertility. However, the molecular and cellular mechanisms underlying the effects of ZMYM2 mutations remain unclear and warrant further investigation.
Neurological manifestations including microcephaly, developmental delay, intellectual disability, speech delay and infantile hypotonia, have been reported in CAKUT patients carrying heterozygous nonsense or frameshift mutations of ZMYM2. In X. tropicalis, Zmym2 knockdown of leads to craniofacial defects. However, our findings indicate that heterozygous Zmym2 mutations on the FVB/N genetic background do not result in detectable craniofacial abnormalities. This finding is unexpected, as it contrasts with previous studies in X. tropicalis, where craniofacial dysmorphology was reported following morpholino-based Zmym2 knockdown. The observed differences may be attributed to the methods used to deplete Zmym2. Morpholinos targeting Zmym2 could potentially affect homologous family members, leading to an exacerbated phenotype. Additionally, species-specific differences and genetic background variations between X. tropicalis and mice may contribute to the discrepancies (Walsh et al., 2017). Despite extensive analyses, no craniofacial abnormalities were identified in Zmym2 PB mice on the FVB/N background. Notably, microcephaly in patients with ZMYM2 mutations is not fully penetrant, and the severity of intellectual disability varies among individuals, suggesting that other environmental or genetic factors may influence disease. Future studies involving backcrossing Zmym2 mutant mice onto different genetic backgrounds may help elucidate this hypothesis. Even in the absence of obvious craniofacial abnormalities, Zmym2 PB/+ mice exhibited behavioral abnormalities, including anxiety and aggressive-like behaviors, uncovering a previously unrecognized neurologic phenotype.
ZMYM2 loss-of-function mutations can cause CAKUT, however, the underlying mechanisms of embryonic kidney developmental abnormalities remain unclear and require further investigation. Zmym2 was highly expressed in kidney primordia from E9.5D, with peak expression E13.5D. confirming its critical role in kidney development (Supplementary Figure S3A). Duplicated UB formation in Zmym2 mutants was a primary cause of duplex kidney formation, and the reduced apoptosis at the ND in Zmym2 mutants may have contributed to abnormal ureter-bladder connections, leading to VUR. The transcription factor TBX18 regulates patterning and differentiation programs in the primordia of urinary system. Tbx18 is co-expressed with Zmym2 in the mesenchymal compartment of the developing ureter in mice, and both genes are co-expressed in the ureteric mesenchyme at E12.5D, the stage at which Tbx18 is required for tissue specification (Ludtke et al., 2022). Our study confirmed that Zmym2 and Tbx18 were co-localized in kidney tissues, with notably high expression in the nephrogenic zone. Tbx18 expression was reduced in kidney tissues of Zmym2 PB/+ mice, which brought to light that ZMYM2 interacts with TBX18 during the kidney development. Patients carrying ZMYM2 mutations also exhibit extra-renal abnormalities, including cardiac septal defects, skeletal abnormalities, hypoplastic hands, feet and nails, as well as scoliosis (Connaughton et al., 2020). Although these phenotypes were not observed in Zmym2 PB/+ mice, in situ hybridization and IF analyses demonstrated extensive Zmym2 expression in multiple organs at E13.5D and prominently in brain, heart, lung, pancreas, kidney and genital tissues at P0D (Supplementary Figures 3B, C). This suggests that Zmym2 plays essential roles in multiple organs systems. Interestingly, Tbx18-deficient mice exhibit vertebral column malformations, while heterozygous loss of another TBX family member, Tbx20, results in a range of cardiac defects. These findings raise the possibility that ZMYM2 interacts with TBX18 and other TBX family at various developmental sites to regulate transcriptional repression. Further in vivo genetic interaction and molecular studies are necessary to test this hypothesis (Ludtke et al., 2022).
5 Conclusion
This study provides the first comprehensive characterization of phenotypes in Zmym2 mutant mice with the PB insertion, including embryonic lethality, genitourinary defects, anxiety and aggressive-like behavior, and glucose metabolism disorders. Notably, this study offers new insights into the roles of Zmym2 in embryonic development and identifies a potential link between Zmym2 mutation and MODY, as well as a possible relationship between hyperglycemia and lifespan. Additionally, Zmym2 mutations induced duplicated UB formation at the early kidney development stage, and abnormal extension of the nephrogenic zone deep into the kidney are the main causes of duplex kidney formation. Reduced apoptosis in the nephric duct may have contributed to abnormal ureter-bladder connections, leading to VUR. The co-expression of Tbx18 with Zmym2 in the developing kidney and reduction in Tbx18 expression in Zmym2 mutants further support the hypothesis that Zmym2 interacts with Tbx18 during kidney development.
In summary, this study expands the Zmym2 genotype-phenotype spectrum. The Zmym2 PB mouse model is the first to demonstrate roles of Zmym2 in neuroethology and endocrinology, extending its significance beyond genitourinary defects and embryonic development. Further investigation of these phenotypes in CAKUT patients carrying ZMYM2 mutations will enhance our understanding of the disease and improve strategies for early diagnosis, monitoring, and treatment.
Data availability statement
The datasets presented in this study can be found in online repositories. The names of the repository/repositories and accession number(s) can be found in the article/Supplementary Material.
Ethics statement
The animal studies were approved by Animal Care and Use Committee of Children’s Hospital of Fudan University. The studies were conducted in accordance with the local legislation and institutional requirements. Written informed consent was obtained from the owners for the participation of their animals in this study.
Author contributions
RD: Funding acquisition, Project administration, Writing – original draft, Writing – review and editing. YY: Data curation, Methodology, Writing – review and editing, Investigation, Validation. MY: Methodology, Resources, Writing – review and editing. YZ: Investigation, Software, Writing – review and editing. JZ: Investigation, Methodology, Writing – review and editing. TL: Writing – review and editing. XF: Conceptualization, Writing – review and editing. XW: Writing – review and editing, Resources, Supervision. QS: Supervision, Validation, Writing – review and editing, Data curation. HX: Project administration, Supervision, Writing – review and editing, Conceptualization.
Funding
The author(s) declare that financial support was received for the research and/or publication of this article. This study was supported by the National Natural Science Foundation of China (82200743), the Science and Technology Commission of Shanghai (Shanghai Sailing Program, 21YF1403400) and the Shanghai Municipal Health Commission Health Industry Clinical Research Special Youth Project (20234Y0229).
Conflict of interest
The authors declare that the research was conducted in the absence of any commercial or financial relationships that could be construed as a potential conflict of interest.
Generative AI statement
The authors declare that no Generative AI was used in the creation of this manuscript.
Publisher’s note
All claims expressed in this article are solely those of the authors and do not necessarily represent those of their affiliated organizations, or those of the publisher, the editors and the reviewers. Any product that may be evaluated in this article, or claim that may be made by its manufacturer, is not guaranteed or endorsed by the publisher.
Supplementary material
The Supplementary Material for this article can be found online at: https://www.frontiersin.org/articles/10.3389/fcell.2025.1523266/full#supplementary-material
References
Blake, J., and Rosenblum, N. D. (2014). Renal branching morphogenesis: morphogenetic and signaling mechanisms. Semin. Cell Dev. Biol. 36, 2–12. doi:10.1016/j.semcdb.2014.07.011
Cirillo, L., De Chiara, L., Innocenti, S., Errichiello, C., Romagnani, P., and Becherucci, F. (2023). Chronic kidney disease in children: an update. Clin. Kidney J. 16 (10), 1600–1611. doi:10.1093/ckj/sfad097
Clissold, R. L., Hamilton, A. J., Hattersley, A. T., Ellard, S., and Bingham, C. (2015). HNF1B-associated renal and extra-renal disease-an expanding clinical spectrum. Nat. Rev. Nephrol. 11 (2), 102–112. doi:10.1038/nrneph.2014.232
Connaughton, D. M., Dai, R. F., Owen, D. J., Marquez, J., Mann, N., Graham-Paquin, A. L., et al. (2020). Mutations of the transcriptional corepressor ZMYM2 cause syndromic urinary tract malformations cause syndromic urinary tract malformations. Am. J. Hum. Genet. 107 (4), 727–742. doi:10.1016/j.ajhg.2020.08.013
Ding, S., Wu, X., Li, G., Han, M., Zhuang, Y., and Xu, T. (2005). Efficient transposition of the piggyBac (PB) transposon in mammalian cells and mice. Cell 122 (3), 473–483. doi:10.1016/j.cell.2005.07.013
Gocke, C. B., and Yu, H. (2008). ZNF198 stabilizes the LSD1-CoREST-HDAC1 complex on chromatin through its MYM-type zinc fingers. PLoS One 3 (9), e3255. doi:10.1371/journal.pone.0003255
Graham-Paquin, A. L., Saini, D., Sirois, J., Hossain, I., Katz, M. S., Zhuang, Q. K. W., et al. (2023). ZMYM2 is essential for methylation of germline genes and active transposons in embryonic development. Nucleic Acids Res. 51 (14), 7314–7329. doi:10.1093/nar/gkad540
Kennard, M. R., Gatward, L. F. D., Roberts, A. G., White, E. R. P., Nandi, M., and King, A. J. F. (2021). The use of mice in diabetes research: the impact of experimental protocols. Diabet. Med. 38 (12), e14705. doi:10.1111/dme.14705
Kolvenbach, C. M., Shril, S., and Hildebrandt, F. (2023). The genetics and pathogenesis of CAKUT. Nat. Rev. Nephrol. 19 (11), 709–720. doi:10.1038/s41581-023-00742-9
Koolhaas, J. M., Coppens, C. M., de Boer, S. F., Buwalda, B., Meerlo, P., and Timmermans, P. J. (2013). The resident-intruder paradigm: a standardized test for aggression, violence and social stress. J. Vis. Exp. (77), e4367. doi:10.3791/4367
Kunapuli, P., Kasyapaa, C. S., Chin, S. F., Caldas, C., and Cowell, J. K. (2006). ZNF198, a zinc finger protein rearranged in myeloproliferative disease, localizes to the PML nuclear bodies and interacts with SUMO-1 and PML. Exp. Cell Res. 312 (19), 3739–3751. doi:10.1016/j.yexcr.2006.06.037
Loane, M., Dolk, H., Kelly, A., Teljeur, C., Greenlees, R., Densem, J., et al. (2011). Paper 4: EUROCAT statistical monitoring: identification and investigation of ten year trends of congenital anomalies in Europe. Birth Defects Res. A Clin. Mol. Teratol. 91 (Suppl. 1), S31–S43. doi:10.1002/bdra.20778
Ludtke, T. H., Kleppa, M. J., Rivera-Reyes, R., Qasrawi, F., Connaughton, D. M., Shril, S., et al. (2022). Proteomic analysis identifies ZMYM2 as endogenous binding partner of TBX18 protein in 293 and A549 cells. Biochem. J. 479 (1), 91–109. doi:10.1042/BCJ20210642
Mao, S. H., Wu, F., Cao, X. Y., He, M., Liu, N. J., Wu, H. H., et al. (2016). TDRP deficiency contributes to low sperm motility and is a potential risk factor for male infertility. Am. J. Transl. Res. 8 (1), 177–187.
Mendelsohn, C., Chen, F., Dusso, A., Liapis, H., and Swat, W. (2009). Using mouse models to understand normal and abnormal urogenital tract development. Organogenesis 5 (1), 306–314. doi:10.4161/org.8173
Seltzsam, S., Wang, C., Zheng, B., Mann, N., Connaughton, D. M., Wu, C. W., et al. (2022). Reverse phenotyping facilitates disease allele calling in exome sequencing of patients with CAKUT. Genet. Med. 24 (2), 307–318. doi:10.1016/j.gim.2021.09.010
Short, K. M., and Smyth, I. M. (2020). Branching morphogenesis as a driver of renal development. Anat. Rec. 303 (10), 2578–2587. doi:10.1002/ar.24486
Tan, L., Yu, M., Li, Y., Xue, S., Chen, J., Zhai, Y., et al. (2021). Overexpression of Long non-coding RNA 4933425B07Rik causes urinary malformations in mice. Front. Cell Dev. Biol. 9, 594640. doi:10.3389/fcell.2021.594640
van der Ven, A. T., Connaughton, D. M., Ityel, H., Mann, N., Nakayama, M., Chen, J., et al. (2018b). Whole-Exome sequencing identifies causative mutations in families with congenital anomalies of the kidney and urinary tract. J. Am. Soc. Nephrol. 29 (9), 2348–2361. doi:10.1681/ASN.2017121265
van der Ven, A. T., Vivante, A., and Hildebrandt, F. (2018a). Novel insights into the pathogenesis of monogenic congenital anomalies of the kidney and urinary tract. J. Am. Soc. Nephrol. 29 (1), 36–50. doi:10.1681/ASN.2017050561
Walsh, R. M., Shen, E. Y., Bagot, R. C., Anselmo, A., Jiang, Y., Javidfar, B., et al. (2017). Phf8 loss confers resistance to depression-like and anxiety-like behaviors in mice. Nat. Commun. 8, 15142. doi:10.1038/ncomms15142
Wang, H., Zhang, C., Wang, X., Lian, Y., Guo, B., Han, M., et al. (2018). Disruption of Gen1 causes congenital anomalies of the kidney and urinary tract in mice. Int. J. Biol. Sci. 14 (1), 10–20. doi:10.7150/ijbs.22768
Keywords: ZMYM2, piggyBac mice, genitourinary defects, anxiety and aggressive-like behavior, glucose metabolism disorders, Tbx18
Citation: Dai R, Yin Y, Yu M, Zhang Y, Zhang J, Liu T, Fang X, Wu X, Shen Q and Xu H (2025) Genitourinary defects, anxiety and aggressive-like behavior and glucose metabolism disorders in Zmym2 mutant mice with inserted piggyBac transposon. Front. Cell Dev. Biol. 13:1523266. doi: 10.3389/fcell.2025.1523266
Received: 20 November 2024; Accepted: 31 March 2025;
Published: 17 April 2025.
Edited by:
Chunming Cheng, University of Oklahoma, United StatesReviewed by:
Katherine Pfister, University of Pittsburgh, United StatesSonghua Hu, Harvard Medical School, United States
Yulei Tao, University of Oklahoma Health Sciences Center, United States
Copyright © 2025 Dai, Yin, Yu, Zhang, Zhang, Liu, Fang, Wu, Shen and Xu. This is an open-access article distributed under the terms of the Creative Commons Attribution License (CC BY). The use, distribution or reproduction in other forums is permitted, provided the original author(s) and the copyright owner(s) are credited and that the original publication in this journal is cited, in accordance with accepted academic practice. No use, distribution or reproduction is permitted which does not comply with these terms.
*Correspondence: Qian Shen, c2hlbmdxaWFuQHNobXUuZWR1LmNu; Hong Xu, aHh1QHNobXUuZWR1LmNu
†These authors have contributed equally to this work and share first authorship