- 1Department of Reproductive Medicine, Guangzhou Women and Children’s Medical Center Liuzhou Hospital, Liuzhou, Guangxi, China
- 2Department of Reproductive Medicine, Liuzhou maternity and Child Healthcare Hospital, Liuzhou, Guangxi, China
- 3Guangxi Clinical Research Center for Obstetrics and Gynecology, Liuzhou, Guangxi, China
- 4Liuzhou Key Laboratory of Gynecologic Tumor, Liuzhou, Guangxi, China
Background: Thalassemia is a hereditary blood disorder that can impact fertility due to various factors such as iron overload and endocrine disruption. While the effects of iron overload on fertility outcomes in transfusion-dependent thalassemia (TDT) have been well-documented, there is limited data on how NTDT affects assisted reproductive technology (ART) outcomes. This study aims to assess the fertility and pregnancy outcomes of NTDT patients compared to thalassemia carriers (TC) patients in IVF and frozen embryo transfer (FET) cycles.
Methods: This retrospective cohort study analyzed 6,911 female patients who underwent autologous IVF treatment at a private reproductive center between January 2013 and December 2022. The study included women who were carriers of thalassemia or diagnosed with NTDT. ART outcomes, including oocyte retrieval rate, embryo development (maturation rate, number of fertilized oocytes and blastocyst formation rate), clinical pregnancy rate, live birth rate, and miscarriage rate, were compared between NTDT and TC patients. Propensity score matching (PSM) and multivariable adjustments for potential confounders were applied in the statistical analyses.
Results: NTDT patients had a significantly lower oocyte retrieval rate (0.88 vs. 0.93, p < 0.05) and a longer interval from medication initiation to oocyte retrieval (13.35 days vs. 12.38 days, p < 0.05) compared to TC patients. However, NTDT patients exhibited higher oocyte maturation rates and a greater number of fertilized oocytes. Despite these differences in embryo development metrics, there were no statistically significant differences in clinical pregnancy rates and live birth rates between NTDT and TC patients in both fresh embryo transfer (IVF-ET) and FET cycles (p > 0.05). These findings suggest that while NTDT may affect certain aspects of embryo development, it does not significantly impact overall pregnancy outcomes in ART.
Conclusion: This study provides valuable insights into ART outcomes for NTDT patients, showing that, despite challenges in oocyte retrieval, their fertility and pregnancy outcomes are comparable to those of thalassemia carriers. Clinicians should consider individualized treatment plans and provide comprehensive counseling for NTDT patients, focusing on their specific fertility characteristics, to optimize ART outcomes. Further research is needed to explore the underlying mechanisms affecting embryo development in NTDT patients and to confirm these findings in broader populations.
Introduction
Recent advances in techniques like embryo culture, vitrification, genetic screening, and cycle splitting have led to significant breakthroughs in assisted reproductive technology (ART), notably improving treatment success rates, especially cumulative live birth rates (CLBR per ITT) (Esteves et al., 2019). As a result, patient consultations have evolved. With the maturation of genetic screening, patients now focus not only on pregnancy success but also on selecting the healthiest embryos to maximize outcomes and minimize treatment risks (Madero et al., 2023).
Thalassemia is a common hereditary disorder affecting around 5% of the global population, with higher carrier rates in regions like China, India, Southeast Asia, and the Mediterranean, presenting a significant public health challenge (Rund, 2016). It is classified into α-thalassemia and β-thalassemia, with α-thalassemia including Hb Bart syndrome and HbH disease, and β-thalassemia encompassing major, intermedia, and minor forms. Carriers typically exhibit mild microcytic anemia, which does not lead to significant health issues and usually requires no treatment (Langer et al., 1993; Tamary et al., 1993). Thalassemia is further categorized into transfusion-dependent thalassemia (TDT) and non-transfusion-dependent thalassemia (NTDT). TDT patients, mainly those with severe β-thalassemia, need regular blood transfusions to manage anemia and prevent complications, often experiencing endocrine disorders due to iron overload, including menstrual irregularities and infertility (Musallam et al., 2021; Castaldi and Cobellis, 2016). NTDT patients, including those with β-thalassemia intermedia and HbH disease, do not require transfusions but still experience ineffective red blood cell production and iron metabolism issues (Musallam et al., 2021). Research on how these conditions affect fertility in NTDT patients is limited, making it crucial to understand their impact for clinical counseling and treatment decisions.
Third-generation IVF technology (preimplantation genetic diagnosis, PGD) is crucial in assisted reproduction for high-risk thalassemia patients, allowing for the selection of embryos free from genetic diseases, thus reducing the risk of affected offspring and improving treatment success (Ren et al., 2024). According to expert consensus (Yan et al., 2023), PGD is recommended when one or both partners are carriers of thalassemia gene mutations and at risk of having affected children. Due to the high costs of PGD and the relatively low genetic risk in NTDT patients, who often have mild symptoms, most of these patients undergo embryo culture and transfer using first- or second-generation IVF technologies. However, the impact of NTDT on embryo development and pregnancy outcomes in assisted reproduction is not well understood.
In this retrospective cohort analysis, we included all patients who underwent autologous IVF at the Reproductive Medicine Center of Liuzhou Hospital, Guangzhou Women and Children’s Medical Center, from January 2013 to December 2022, with thalassemia gene carriers serving as a control group. The aim is to assess whether NTDT affects fertility and provide data to optimize treatment strategies. A better understanding of thalassemia’s impact on assisted reproduction will help healthcare providers set more accurate expectations, improve patient compliance, and enhance treatment outcomes.
Materials and methods
This is a retrospective cohort data analysis conducted at an infertility center in southeastern China. The study population consisted of patients who underwent autologous in vitro fertilization (IVF) treatment at the Reproductive Medicine Center of Liuzhou Hospital, Guangzhou Women and Children’s Medical Center, between January 2013 and December 2022. The treatment cycles included fresh IVF-embryo transfer (IVF-ET) and frozen embryo transfer (FET). Inclusion criteria for the cycles were as follows: female patients who were either carriers of thalassemia or non-transfusion-dependent thalassemia (confirmed by hematological testing). Cycles involving male patients who were thalassemia carriers or non-transfusion-dependent thalassemia, as well as those using oocyte donation, sperm donation, or frozen-thawed oocytes, were excluded from the study. The study was approved by the Ethics Committee of Liuzhou Hospital, Guangzhou Women and Children’s Medical Center (No. 2024-238).
Patients’ ages were obtained from their identity card information, while the duration of infertility was self-reported by the patients. Details about the infertility diagnosis, ovarian stimulation protocol, and fertilization methods were recorded by the attending physicians. Height and weight were measured during the clinic visit, and body mass index (BMI) was calculated as weight (kg) divided by height (m) squared. Detailed information related to embryos, including the grade and number of embryos transferred, was recorded by the reproductive laboratory. Serum hormone levels were obtained from the clinical laboratory and genetic laboratory databases.
Stimulation protocol
Patients underwent ovarian stimulation, transvaginal oocyte retrieval, and subsequent blastocyst cryopreservation based on the treatment physician’s judgment. During the treatment, either an antagonist protocol or an agonist protocol was used. The treatment plan included subcutaneous injections of gonadotropins (Gn), with follicular development monitored via transvaginal ultrasound and serum estradiol, luteinizing hormone (LH), and progesterone levels to assess ovarian response. For oocyte maturation, human chorionic gonadotropin (hCG) and/or gonadotropin-releasing hormone agonist (GnRH-a) were administered. Specific treatment protocols and medication use followed established standard procedures from prior literature (Fan et al., 2022), with adjustments made based on individual patient characteristics.
Fertilization and embryo culture
In this study, fertilization was performed using either conventional insemination or intracytoplasmic sperm injection (ICSI) at the discretion of the treating physician. In the conventional fertilization process, semen samples were prepared under a laminar flow hood, with 0.8–1 mL per well, and the insemination time was adjusted according to follicular maturity and other factors, typically occurring 4–6 h after oocyte retrieval. The sperm concentration was calculated at 50,000 sperm per oocyte, ensuring that no more than six oocytes were inseminated per well. Granulosa cells were removed 4–5 h after fertilization, and viable oocytes were transferred into culture medium. For ICSI, morphologically normal sperm were selected, immobilized, and injected into MII-stage oocytes using micromanipulation techniques. After injection, the cytoplasm was aspirated to ensure the sperm entered the oocyte. All fertilized oocytes were transferred into culture medium and briefly cultured in an incubator. Embryo development was assessed 16–18 h after fertilization. Embryo transfer typically took place 24–48 h post-fertilization, following routine IVF procedures.
Fresh and thawing embryo transfer
In this study, patients underwent either fresh cycle embryo transfer or FET, based on the judgment of the treating physician. In the fresh cycle, patients received ovarian stimulation, and ovulation was triggered with hCG when follicles reached appropriate size. After oocyte retrieval, fertilization was performed, and the embryos were cultured to the blastocyst stage. The appropriate embryos for transfer were selected based on their development, with transfer typically occurring on day 3 after oocyte retrieval. However, in some cases, blastocysts on day 5 or 6 were transferred depending on the circumstances. For FET, patients underwent embryo transfer at least 2 months after the fresh cycle. Endometrial preparation for FET could involve a natural cycle, clomiphene citrate-induced cycle, or hormone replacement therapy. Once the endometrium reached the appropriate thickness, embryos were thawed and evaluated. Only embryos with a survival rate of over 50% were considered for transfer. A maximum of two embryos or blastocysts were transferred per FET cycle.
Outcome variables
Various cycle outcomes were examined, including the number of oocyte retrievals, the number of oocytes retrieved, oocyte retrieval rate (oocytes retrieved per punctured follicle), the number of mature oocytes, maturation rate (the percentage of mature oocytes per retrieval cycle), the number of fertilized oocytes, fertilization rate (fertilization rate per mature oocyte), the number of blastocysts cultured, blastocyst formation rate, the time from stimulation to oocyte retrieval (in days), and the time from stimulation to pregnancy (in months). The primary outcomes included pregnancy rate, live birth rate, miscarriage rate in NTDT patients compared to TC, as well as pregnancy rate, live birth rate, and miscarriage rate following frozen embryo transfer. Clinical pregnancy was defined as the observation of an intrauterine gestational sac via ultrasound in early pregnancy, or the recording of live birth, ectopic pregnancy, or miscarriage. Live birth was defined as the birth of one or more live infants. Miscarriage was defined as the loss of an intrauterine pregnancy before 20 weeks of gestation.
Covariates
The covariates in this study included several baseline factors in women that could influence IVF or intracytoplasmic sperm injection (ICSI) cycles, cycle outcomes, and FET-related parameters. Female covariates included age, BMI, anti-Müllerian hormone (AMH) levels, serum estradiol (E2), follicle-stimulating hormone (FSH), luteinizing hormone (LH), the number of embryos transferred, duration of infertility, fertilization method (IVF or ICSI), infertility type (primary or secondary), infertility diagnosis (e.g., tubal factor, male factor, diminished ovarian reserve, endometriosis, etc.), the timing of embryo transfer (day 3, day 5/6), and ovarian stimulation protocol (agonist, antagonist, mild stimulation, natural cycle, etc.). For FET, covariates included female age, BMI, the number of embryos transferred, duration of infertility, fertilization method (IVF or ICSI), infertility type (primary or secondary), timing of embryo transfer (day 3, day 5/6), and endometrial preparation method (natural cycle or programmed cycle). These covariates were assessed to evaluate the impact of various factors on pregnancy outcomes and were analyzed as potential confounders.
Propensity score matching (PSM)
To reduce confounding and balance baseline characteristics between the two study groups, PSM was used. PSM is a statistical technique that matches individuals from different groups based on their estimated probability (propensity score) of receiving a treatment, given their baseline characteristics. In this study, a 1:2 matching ratio was used to match NTDT with TC patients based on several baseline covariates. The propensity scores were estimated using logistic regression, and patients who could not be matched were excluded from the analysis. This method helped balance the groups, making the comparison of ART outcomes between NTDT and TC patients more robust and minimizing confounding bias.
Statistical analysis
All data analyses were performed using SPSS 26.0 and the R statistical language in RStudio, with a two-sided significance level set at 0.05. There were no missing data for outcome variables, and the missing rate for other variables was below 5%. For missing data, the median imputation method was applied, which is suitable for skewed data, to minimize the impact of extreme values and ensure the representativeness of the data.
For statistical comparisons, Student’s t-test and Mann-Whitney U test were used to compare continuous variables, while the chi-square test was used for categorical variables. For the analysis of pregnancy outcomes, we first fitted an unadjusted model and then further fitted a multivariable-adjusted model, incorporating all baseline factors into the adjusted model to control for potential confounders. To further explore the relationship between pregnancy outcomes and various covariates, we performed interaction effect analysis to assess the interactions between different covariates. All relevant covariates were included in the interaction effect model to analyze their potential impact on pregnancy outcomes.
Results
From January 2013 to December 2022, a total of 6,911 patients from the Reproductive Medicine Center met the inclusion criteria. Among them, 2,908 patients (42.1%) underwent fresh embryo transfer cycles, while 4,002 patients (57.9%) underwent FET cycles. In the fresh embryo transfer cycles, 2,346 patients were in the TC group, and 562 patients were in the NTDT group. In the FET cycles, 2,995 patients were in the TC group, and 1,007 patients were in the NTDT group.
Propensity score matching
Table 1 shows the baseline characteristics before and after propensity score matching (PSM) for 13 baseline factors. The data are reported on a per-cycle basis. In both the fresh embryo transfer and FET cycles, 1:2 propensity score matching was performed between the TC and the NTDT group. In the fresh embryo transfer cycles, 13 variables were matched, including: female age, BMI, AMH, E2, FSH, LH, number of embryos transferred, infertility duration, fertilization method, type of infertility, infertility diagnosis, day of transfer, and ovarian stimulation protocol. In the FET cycles, 8 variables were matched, including: female age, BMI, number of embryos transferred, infertility duration, fertilization method, type of infertility, day of transfer, and endometrial preparation (Supplementary Table S2).
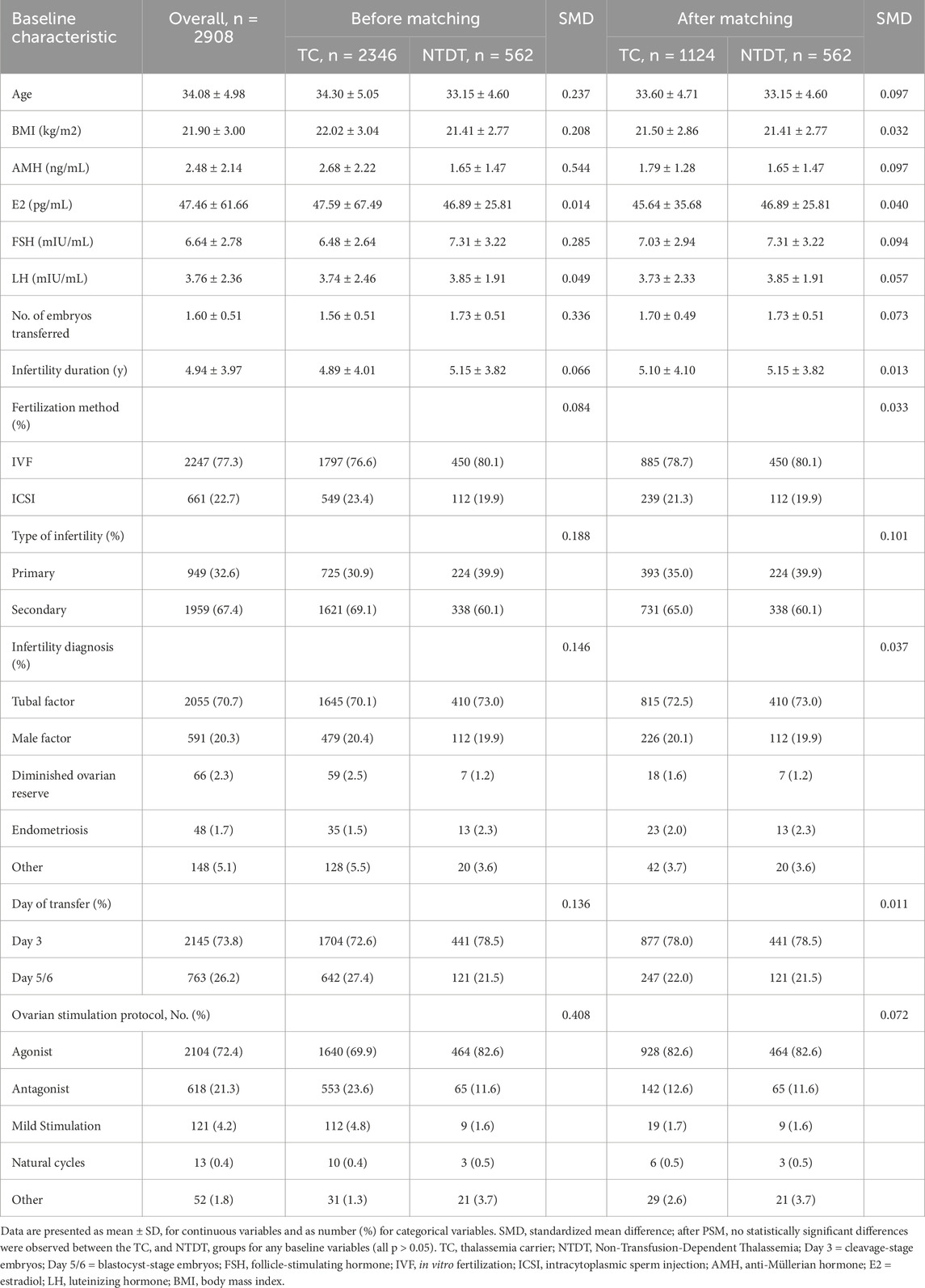
Table 1. Baseline characteristics of women undergoing fresh cycles for TC and NTDT groups before and after 1:2 propensity score matching.
In the fresh cycle, before matching, there were significant differences between the two groups in variables such as female age (SMD = 0.237), BMI (SMD = 0.208), AMH (SMD = 0.544), FSH (SMD = 0.285), and ovarian stimulation protocol (SMD = 0.408). After matching, the SMD values for these variables significantly decreased to less than 0.1, indicating improved balance in baseline characteristics between the two groups (Table 1). In the frozen-thawed cycle, before matching, there were differences between the two groups in variables such as female age (SMD = 0.120), number of embryos transferred (SMD = 0.404), and fertilization method (SMD = 0.201). After matching, the SMD values for these variables also significantly decreased (e.g., number of embryos transferred SMD = 0.078, fertilization method SMD = 0.043), further improving the balance between the two groups (Supplementary Table S2).
Cycle characteristics and embryo outcomes
Table 2 reports the cycle characteristics and embryo outcome data after applying the propensity score model, comparing TC group with NTDT group. The NTDT group had a significantly longer interval between the start of medication and oocyte retrieval compared to the TC group (13.35 days vs. 12.38 days, p < 0.001), but their oocyte retrieval rate was significantly lower than that of the TC group (0.88 vs. 0.93, p = 0.018). Moreover, the NTDT group had a significantly higher maturation rate than the TC group (1 vs. 0.94, p = 0.015), and they also had a significantly greater number of fertilized oocytes (6 vs. 5, p = 0.005). Although both groups showed similar outcomes for most embryo parameters (such as blastocyst formation rate and number of blastocysts), the time interval from ovarian stimulation to the first positive hCG result was significantly longer in the NTDT group compared to the TC group (0.97 months vs. 0.93 months, p < 0.001).
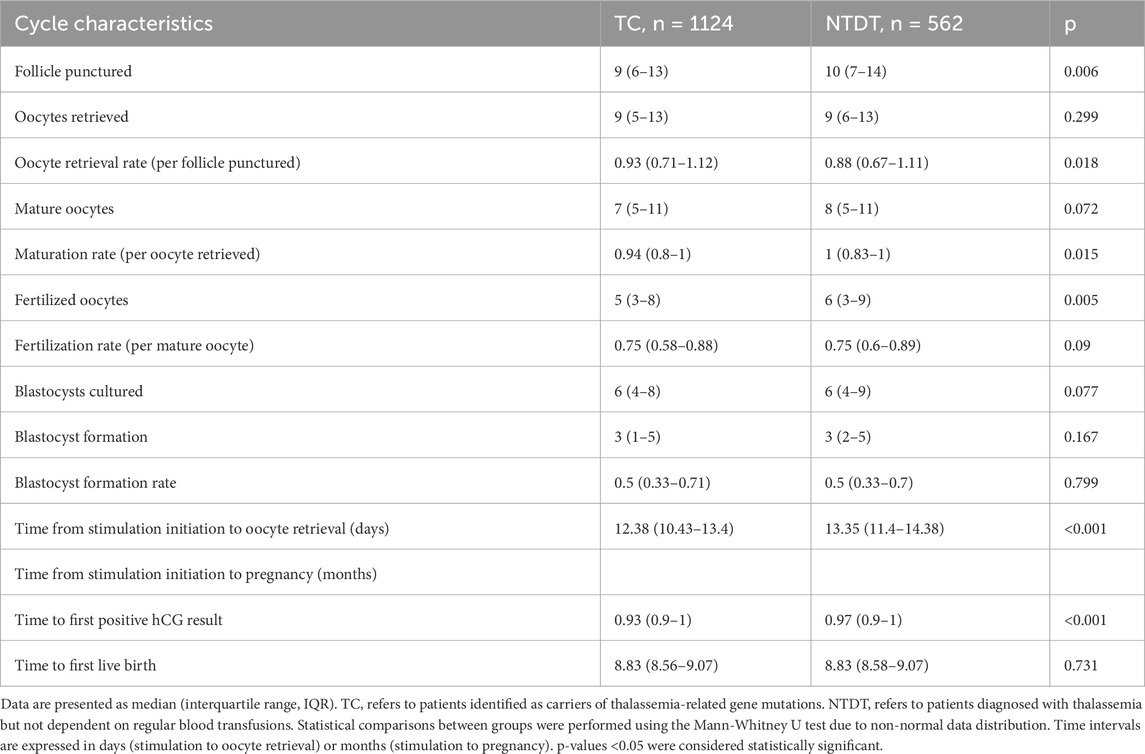
Table 2. Cycle characteristics and embryonic outcomes in thalassemia carriers and non-transfusion-dependent thalassemia patients after propensity score matching.
Additionally, we conducted an interaction analysis between baseline variables and embryo outcomes for the TC and NTDT groups (Supplementary Table S1). The results indicated that lower AMH levels (interaction estimate −0.4566, p = 0.0089) and lower E2 levels (interaction estimate −0.0179, p = 0.0404) were significantly associated with fewer punctured follicles in the NTDT group. Furthermore, lower E2 levels were significantly associated with a longer oocyte retrieval duration in the NTDT group (interaction estimate −0.0095, p = 0.0163). Moreover, the effect of FSH levels on maturation rate showed a significant interaction between the two groups (interaction estimate −0.0068, p = 0.0036). Other baseline variables, such as BMI and LH, did not show significant interactions (p > 0.05).
Pregnancy outcomes
Table 3 summarizes the pregnancy outcomes for TC group and NTDT group in both fresh embryo transfer cycles and FET cycles. In the fresh embryo transfer cycles, the clinical pregnancy rate and live birth rate were slightly higher in the TC group compared to the NTDT group (52.3% vs. 47.9%, 44.8% vs. 41.1%), but the differences were not significant (aRR = 0.90, 95% CI: 0.78–1.05; aRR = 0.90, 95% CI: 0.77–1.06). The miscarriage rate was similar between the two groups (7.6% vs. 6.8%, aRR = 0.93, 95% CI: 0.63–1.36). In the FET cycles, the pregnancy outcomes in the TC group and NTDT group were also similar. The clinical pregnancy rate and live birth rate in the TC group were 49.9% and 39.6%, respectively, while in the NTDT group, they were 47.9% and 39.0%. After adjustment, the differences remained statistically insignificant (aRR = 0.94, 95% CI: 0.84–1.05; aRR = 0.97, 95% CI: 0.86–1.09). The miscarriage rate was slightly lower in the TC group compared to the NTDT group (9.3% vs. 7.7%, aRR = 0.83, 95% CI: 0.64–1.09). Interaction analysis results (Supplementary Table S3) also indicated that there was no statistically significant interaction between thalassemia subgroups (TC group vs. NTDT group) and baseline variables on clinical pregnancy outcomes (p > 0.05). This further supports the consistency of the impact of thalassemia carrier status on pregnancy outcomes across different baseline characteristics.
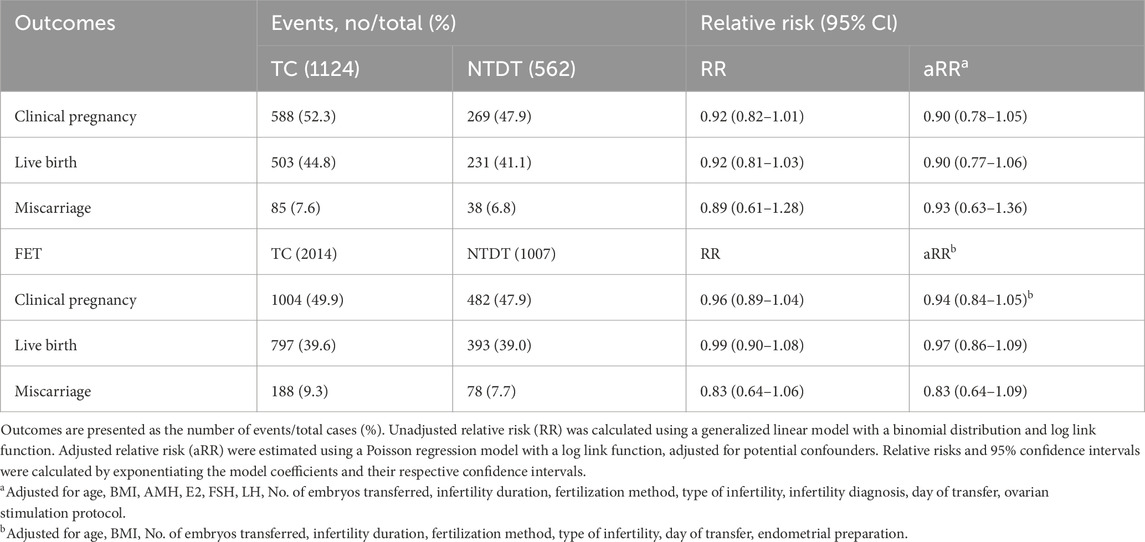
Table 3. Pregnancy outcomes in fresh and FET cycles for TC and NTDT patients after propensity score matching.
Discussion
Thalassemia patients often face unique counseling issues in assisted reproductive treatment, particularly regarding embryo health, pregnancy success rates, and genetic risks. These concerns tend to exacerbate patients’ anxiety, especially after multiple failed transfers or miscarriages, which can lead to increased psychological burden. Therefore, providing scientifically grounded and objective fertility counseling has become a pressing challenge. The aim of this study is to provide valuable information regarding NTDT patients in the assisted reproductive process, addressing the gap in existing literature. We analyzed data from 6,911 patients and found that, although the oocyte retrieval rate was lower in the NTDT group, several embryonic development indicators were superior to those in the TC group. Further analysis revealed that the NTDT group had lower pregnancy outcomes (clinical pregnancy rate and live birth rate) compared to the TC group, although the differences did not reach statistical significance. Clearly, the advantage of the NTDT group in embryonic development did not fully translate into higher clinical pregnancy and live birth rates, which may be related to specific physiological mechanisms, differences during embryo transfer, or uterine environment factors.
The reasons for female infertility in thalassemia patients are multifactorial. One of the most common causes is the interference of iron overload with the endocrine system. Iron accumulation due to long-term blood transfusion therapy can lead to hypogonadotropic hypogonadism (HH) (Castaldi and Cobellis, 2016), resulting in menstrual absence and infertility. One mechanistic hypothesis suggests that oxidative stress (OS) caused by iron overload may affect the hypothalamic-pituitary-ovarian axis, thus inhibiting ovarian function (Roussou et al., 2013). Studies have shown that free radicals produced by iron overload can lead to oxidative damage to cell membranes and DNA, which not only affects the endocrine system but may also cause abnormal follicular development (Devine et al., 2012). In our study, the oocyte retrieval rate in the NTDT group was significantly lower than that in the TC group (0.88 vs. 0.93, P = 0.018), which may be attributed to follicular development abnormalities caused by iron overload. Additionally, iron accumulation can cause dysfunction in endocrine glands, particularly the hypothalamus and pituitary, leading to insufficient secretion of gonadotropins (LH, FSH) (Roussou et al., 2013), which in turn affects ovarian hormone synthesis and ovarian reserve function. Our interaction analysis results also support this mechanism, as low AMH levels (interaction estimate −0.4566, p = 0.0089) and low E2 levels (interaction estimate −0.0179, p = 0.0404) were closely associated with a decrease in follicle count in the NTDT group, suggesting that endocrine dysfunction may be an important factor contributing to the lower oocyte retrieval rate in the NTDT group.
Although iron overload-induced oxidative stress exacerbates the negative effects on oocyte maturation, embryo cleavage, and blastocyst formation (Gao et al., 2023; Deng et al., 2020), as observed in previous studies, the findings in the context of thalassemia major patients with iron overload are not entirely consistent. Studies have shown that, despite ovarian dysfunction in women with thalassemia major, the fertilization rate and cleavage rate during in IVF are actually higher than in the control group, with no significant difference in oocyte quality, such as the formation rate of high-quality embryos (Mensi et al., 2019). Interestingly, embryos carrying thalassemia genotypes even demonstrated better development than normal embryos (Piyamongkol et al., 2012). Our study also confirms these findings: the oocyte maturation rate and fertilized oocyte count in the NTDT group were significantly higher than those in the TC group, with no significant difference in blastocyst formation rate between the two groups. This suggests that, despite the potential oxidative stress caused by iron overload in the NTDT group, its impact on oocyte maturation and fertilization is more complex and may be modulated by other factors.
The situation is more complicated when it comes to implantation and subsequent pregnancy, as these processes involve the timely interaction between the embryo and the endometrium, both of which are regulated by multiple complex signaling pathways and interactions between cells and between cells and the extracellular matrix (Hannan et al., 2011; Tepekoy et al., 2015; Kurian and Modi, 2019). In our study, both in fresh and frozen-thawed embryo transfer cycles, the clinical pregnancy rate and live birth rate in the NTDT group were lower than those in the TC group, although the differences did not reach statistical significance. Existing literature offers an interesting perspective on this trend: excess iron accumulation may alter the uterine microenvironment by inducing oxidative stress and local inflammatory responses, thereby reducing endometrial receptivity (Li et al., 2021; Chen et al., 2021). Under normal conditions, the endometrium needs to maintain proper energy metabolism and cell viability to support successful embryo implantation. However, iron overload may cause mitochondrial dysfunction, reducing ATP production and impairing the metabolism and survival of endometrial cells (Gao et al., 2019). Additionally, iron accumulation is closely associated with apoptosis and iron-dependent cell death (ferroptosis), which not only reduces the number of suitable endometrial cells but may also inhibit cell proliferation and differentiation, further impairing endometrial receptivity (Ng et al., 2020). However, current evidence is primarily based on mouse models and is largely focused on iron overload research. Therefore, future studies need to further validate and expand these findings in a broader clinical population to better understand the specific role of iron overload in pregnancy outcomes in different contexts.
The clinical implications of the study findings are as follows: 1) The NTDT group exhibited a higher oocyte maturation rate, but the oocyte retrieval rate was lower, and the retrieval time was longer. Clinically, it is important to tailor treatment plans according to the specific condition of NTDT patients, optimizing oocyte retrieval and embryo quality. 2) Although there may be differences in oocyte retrieval rates and embryo quality in NTDT patients, pregnancy outcomes in both fresh embryo transfer cycles and frozen embryo transfer cycles were not significantly affected. Therefore, patients should not overly worry when choosing an embryo transfer plan, but rather focus on factors such as medical history, ovarian reserve, and hormone levels. Nevertheless, this study has limitations. First, as a retrospective analysis, we are unable to establish causality and can only reveal associations between different subgroups. Future prospective studies are needed to more clearly define the causal relationships between these factors and pregnancy outcomes. Second, despite the use of propensity score matching (PSM) to control for 13 baseline factors, there may still be unaccounted confounding factors, such as genetic background or environmental influences, which could limit the generalizability of the results.
Conclusion
This study provides valuable insights for the treatment of thalassemia patients in assisted reproduction. Although there were no significant differences in pregnancy outcomes between the fresh and frozen embryo transfer cycles in NTDT patients, differences in oocyte retrieval rates and maturation rates suggest the need for individualized treatment plans. For thalassemia patients, assisted reproduction specialists should focus on ovarian response characteristics, provide scientifically based treatment plans, and offer psychological counseling to improve treatment success rates and pregnancy outcomes.
Data availability statement
The raw data supporting the conclusions of this article will be made available by the authors, without undue reservation.
Ethics statement
The studies involving humans were approved by Ethics Committee of Liuzhou Hospital, Guangzhou Women and Children’s Medical Center. The studies were conducted in accordance with the local legislation and institutional requirements. Written informed consent for participation was not required from the participants or the participants’ legal guardians/next of kin in accordance with the national legislation and institutional requirements.
Author contributions
LF: Writing–original draft, Writing–review and editing. LW: Writing–review and editing. NT: Writing–review and editing. ZL: Writing–review and editing. WL: Writing–review and editing. LN: Writing–review and editing. JL: Writing–review and editing. WH: Writing–original draft, Writing–review and editing.
Funding
The author(s) declare that financial support was received for the research, authorship, and/or publication of this article. This work was supported by the Guangxi Science and Technology Plan Project (GuiKe AD22035223), the Guangxi Medical Reserve Talent Program, the Project of Liuzhou Science and Technology Bureau [Liu Ketong (2023) No. 40], the Self-funded Project by the Health commission of Guangxi Zhuang Autonomous Region (Z-B20221568, Z-B20231540 and Z-B20232032), the Optimization and Application of Preimplantation Genetic Diagnosis Platform for Thalassemia in Guangxi Region (2023YRZ0103), Medical Youth Reserve Talent Training Program of the Health Commission of Guangxi Zhuang Autonomous Region [Gui Wei Ren Fa (2025) No. 5].
Conflict of interest
The authors declare that the research was conducted in the absence of any commercial or financial relationships that could be construed as a potential conflict of interest.
Generative AI statement
The author(s) declare that no Generative AI was used in the creation of this manuscript.
Publisher’s note
All claims expressed in this article are solely those of the authors and do not necessarily represent those of their affiliated organizations, or those of the publisher, the editors and the reviewers. Any product that may be evaluated in this article, or claim that may be made by its manufacturer, is not guaranteed or endorsed by the publisher.
Supplementary material
The Supplementary Material for this article can be found online at: https://www.frontiersin.org/articles/10.3389/fcell.2025.1573572/full#supplementary-material
References
Castaldi, M. A., and Cobellis, L. (2016). Thalassemia and infertility. Hum. Fertil. (Camb) 19, 90–96. doi:10.1080/14647273.2016.1190869
Chen, X., Zhou, Y., Wu, D., Shu, C., Wu, R., Li, S., et al. (2021). Iron overload compromises preimplantation mouse embryo development. Reprod. Toxicol. 105, 156–165. doi:10.1016/j.reprotox.2021.08.010
Deng, T., Xie, J., Ge, H., Liu, Q., Song, X., Hu, L., et al. (2020). Tauroursodeoxycholic acid (TUDCA) enhanced intracytoplasmic sperm injection (ICSI) embryo developmental competence by ameliorating endoplasmic reticulum (ER) stress and inhibiting apoptosis. J. Assist. Reprod. Genet. 37, 119–126. doi:10.1007/s10815-019-01627-2
Devine, P. J., Perreault, S. D., and Luderer, U. (2012). Roles of reactive oxygen species and antioxidants in ovarian toxicity. Biol. Reprod. 86, 27. doi:10.1095/biolreprod.111.095224
Esteves, S. C., Humaidan, P., Roque, M., and Agarwal, A. (2019). Female infertility and assisted reproductive technology. Panminerva Med. 61, 1–2. doi:10.23736/S0031-0808.18.03553-X
Fan, L., Tang, N., Yao, C., Wei, X., Tang, Y., Li, J., et al. (2022). Association between fresh embryo transfers and frozen-thawed embryo transfers regarding live birth rates among women undergoing long gonadotropin-releasing hormone antagonist protocols. Front. Cell Dev. Biol. 10, 884677. doi:10.3389/fcell.2022.884677
Gao, L., Zhang, C., Zheng, Y., Wu, D., Chen, X., Lan, H., et al. (2023). Glycine regulates lipid peroxidation promoting porcine oocyte maturation and early embryonic development. J. Anim. Sci. 101, skac425. doi:10.1093/jas/skac425
Gao, M., Yi, J., Zhu, J., Minikes, A. M., Monian, P., Thompson, C. B., et al. (2019). Role of mitochondria in ferroptosis. Mol. Cell 73, 354–363.e3. doi:10.1016/j.molcel.2018.10.042
Hannan, N. J., Evans, J., and Salamonsen, L. A. (2011). Alternate roles for immune regulators: establishing endometrial receptivity for implantation. Expert Rev. Clin. Immunol. 7, 789–802. doi:10.1586/eci.11.65
Kurian, N. K., and Modi, D. (2019). Extracellular vesicle mediated embryo-endometrial cross talk during implantation and in pregnancy. J. Assist. Reprod. Genet. 36, 189–198. doi:10.1007/s10815-018-1343-x
Langer, A. L. (1993). Beta-thalassemia. In: M. P. Adam, J. Feldman, G. M. Mirzaa, R. A. Pagon, S. E. Wallace, and A. Amemiya, eds. GeneReviews(®). Seattle (WA): university of Washington, seattle copyright © 1993-2025, university of Washington, seattle. Seattle, WA: University of Washington, Seattle. All rights reserved.
Li, S., Zhou, Y., Huang, Q., Fu, X., Zhang, L., Gao, F., et al. (2021). Iron overload in endometriosis peritoneal fluid induces early embryo ferroptosis mediated by HMOX1. Cell Death Discov. 7, 355. doi:10.1038/s41420-021-00751-2
Madero, J. I., Manotas, M. C., García-Acero, M., López Cáceres, A., and López Jaimes, C. (2023). Preimplantation genetic testing in assisted reproduction. Minerva Obstet. Gynecol. 75, 260–272. doi:10.23736/S2724-606X.21.04805-3
Mensi, L., Borroni, R., Reschini, M., Cassinerio, E., Vegetti, W., Baldini, M., et al. (2019). Oocyte quality in women with thalassaemia major: insights from IVF cycles. Eur. J. Obstet. Gynecol. Reprod. Biol. X 3, 100048. doi:10.1016/j.eurox.2019.100048
Musallam, K. M., Cappellini, M. D., Viprakasit, V., Kattamis, A., Rivella, S., and Taher, A. T. (2021). Revisiting the non-transfusion-dependent (NTDT) vs. transfusion-dependent (TDT) thalassemia classification 10 years later. Am. J. Hematol. 96, E54–E56. doi:10.1002/ajh.26056
Ng, S. W., Norwitz, S. G., Taylor, H. S., and Norwitz, E. R. (2020). Endometriosis: the role of iron overload and ferroptosis. Reprod. Sci. 27, 1383–1390. doi:10.1007/s43032-020-00164-z
Piyamongkol, W., Vutyavanich, T., and Sanguansermsri, T. (2012). Preimplantation genetic diagnosis of alpha-thalassemia-SEA using novel multiplex fluorescent PCR. J. Assist. Reprod. Genet. 29, 95–102. doi:10.1007/s10815-011-9641-6
Ren, Z., Huang, P., Wang, Y., Yao, Y., Ren, J., Xu, L., et al. (2024). Technically feasible solutions to challenges in preimplantation genetic testing for thalassemia: experiences of multiple centers between 2019 and 2022. J. Assist. Reprod. Genet. 41, 3225–3235. doi:10.1007/s10815-024-03240-4
Roussou, P., Tsagarakis, N. J., Kountouras, D., Livadas, S., and Diamanti-Kandarakis, E. (2013). Beta-thalassemia major and female fertility: the role of iron and iron-induced oxidative stress. Anemia 2013, 617204. doi:10.1155/2013/617204
Rund, D. (2016). Thalassemia 2016: modern medicine battles an ancient disease. Am. J. Hematol. 91, 15–21. doi:10.1002/ajh.24231
Tamary, H., and Dgany, O. (1993). Alpha-thalassemia. In: M. P. Adam, J. Feldman, G. M. Mirzaa, R. A. Pagon, S. E. Wallace, and A. Amemiya, eds. GeneReviews(®). Seattle (WA): university of Washington, seattle copyright © 1993-2025, university of Washington, seattle. Seattle, WA: University of Washington, Seattle. All rights reserved.
Tepekoy, F., Akkoyunlu, G., and Demir, R. (2015). The role of Wnt signaling members in the uterus and embryo during pre-implantation and implantation. J. Assist. Reprod. Genet. 32, 337–346. doi:10.1007/s10815-014-0409-7
Keywords: non-transfusion-dependent thalassemia, thalassemia carriers, live birth rate, assisted reproductive technology, clinical pregnancy
Citation: Fan L, Wei L, Tang N, Li Z, Li W, Nong L, Li J and Huang W (2025) Thalassemia and assisted reproduction: non-transfusion-dependent thalassemia shows no significant effect on live birth rates after embryo transfer. Front. Cell Dev. Biol. 13:1573572. doi: 10.3389/fcell.2025.1573572
Received: 09 February 2025; Accepted: 10 March 2025;
Published: 21 March 2025.
Edited by:
Satoshi Kishigami, University of Yamanashi, JapanReviewed by:
Filiz Tepekoy, University of Derby, United KingdomTatsuyuki Ogawa, Kameda Medical Center, Japan
Copyright © 2025 Fan, Wei, Tang, Li, Li, Nong, Li and Huang. This is an open-access article distributed under the terms of the Creative Commons Attribution License (CC BY). The use, distribution or reproduction in other forums is permitted, provided the original author(s) and the copyright owner(s) are credited and that the original publication in this journal is cited, in accordance with accepted academic practice. No use, distribution or reproduction is permitted which does not comply with these terms.
*Correspondence: Wenjie Huang, YTE3Mzc3NTUyMjQyQDE2My5jb20=