- Mikrobiologisches Institut - Klinische Mikrobiologie, Immunologie und Hygiene, Universitätsklinikum Erlangen and Friedrich-Alexander-Universität Erlangen-Nürnberg, Erlangen, Bavaria, Germany
Infections with parasites of the genus Leishmania lead to a rapid, but transient activation of natural killer (NK) cells. In mice activation of NK cells requires a toll-like-receptor 9-dependent stimulation of dendritic cells (DC) which is followed by the production of IL-12. Although NK cells appear to be non-essential for the ultimate control of cutaneous and visceral leishmaniasis (VL) and can exhibit immunosuppressive functions, they form an important source of interferon (IFN)-γ, which elicits antileishmanial activity in macrophages and helps to pave a protective T helper cell response. In contrast, the cytotoxic activity of NK cells is dispensable, because Leishmania-infected myeloid cells are largely resistant to NK-mediated lysis. In human cutaneous and VL, the functional importance of NK cells is suggested by reports that demonstrate (1) a direct activation or inhibition of NK cells by Leishmania promastigotes, (2) the suppression of NK cell numbers or activity during chronic, non-healing infections, and (3) the recovery of NK cell activity following treatment. This review aims to provide an integrated view on the migration, activation, inhibition, function, and therapeutic modulation of NK cells in experimental and human leishmaniasis.
A Brief Introduction into NK Cells and Immunity to Leishmania
Natural killer (NK) cells are known for their ability to directly lyse host cells that express an altered cell surface phenotype following a viral infection, malignant transformation, or stress reaction. The upregulation of ligands for NK cell-activating receptors (e.g., NKG2D) or the downregulation of ligands for NK cell-inhibitory receptors (e.g., members of the Ly49 family) are relevant stimuli for NK cell activation in these cases (Lanier, 2008; Yokoyama, 2008). In addition to their cytotoxic potential NK cells also function as producers of cytokines such as interferon (IFN)-γ and tumor necrosis factor (TNF), which contribute to the development of type 1 T helper (Th1) cells and activate macrophages for the expression of antimicrobial killing mechanisms (e.g., inducible or type 2 nitric oxide synthase [iNOS, NOS2]) (Bogdan, 2001; Stetson et al., 2003; Laouar et al., 2005; Prajeeth et al., 2011). During systemic infections NK cells were reported to acquire the potential to produce IL-10 and operate as negative regulators of immune responses due to a feedback inhibition of IL-12 release by dendritic cells (DC) (Maroof et al., 2008; Perona-Wright et al., 2009). Finally, in certain settings NK cells might adopt memory-like adaptive activities (Sun et al., 2009).
Leishmaniasis is caused by protozoan parasites of the genus Leishmania that are transmitted by the bites of sand flies. Leishmania parasites exist in two distinct morphological forms: the extracellular, flagellated form that is inoculated into the mammalian host organism by the vector; and the intracellular, aflagellated, or amastigote form, which resides or replicates in various types of target cells (e.g., granulocytes, macrophages, DC, fibroblasts) (Bogdan et al., 2000; Rittig and Bogdan, 2000; Sacks and Noben-Trauth, 2002). Depending on the parasite subgenus [subgenus Leishmania (L.) vs. Viannia (V.)], species and strain, the dose of transmitted parasites, the inoculation site and the quality of the immune response of the infected host, the infection can remain asymptomatic, or turn into a diverse set of clinical disease manifestations. These include local cutaneous leishmaniasis (LCL), diffuse cutaneous leishmaniasis (DCL), disseminated cutaneous leishmaniasis, mucocutaneous or mucosal leishmaniasis (MCL) as well as visceral leishmaniasis (VL) (Murray et al., 2005). The control of all forms of leishmaniasis requires the IL-12-driven differentiation and recruitment of IFN-γ-producing T helper cells (Mougneau et al., 2011), the presence of IFN-γ-activated macrophages capable to kill intracellular amastigotes (Lykens et al., 2010), and mechanisms that prevent hyperinflammation and mediate resolution of the tissue damage (Peters and Sacks, 2006). Clinical cure of leishmaniasis does not lead to sterile immunity. There is lifelong persistence of small amounts of parasites which can be reactivated during phases of immunosuppression (Bogdan et al., 2000; Belkaid et al., 2002; Bogdan, 2008, 2012).
Several mouse models are available for studying human LCL. Both in the subcutaneous (s.c.) footpad and the intradermal (i.d.) ear-infection model inoculation of small or high numbers of parasites leads to non-ulcerative or ulcerative dermal lesions, which in several mouse strains closely reflect the self-healing skin lesions observed in patients with LCL (Mitchell et al., 1982; Belkaid et al., 2000). Mimicking VL in mouse models is somewhat more challenging, as the intradermal injection of Leishmania species known to cause VL in humans (e.g., L. L. donovani, L. L. infantum/L. L. chagasi) does not routinely lead to parasite visceralization (Leclercq et al., 1996; Ahmed et al., 2003); even after i.v. infection of the mice a progressive and sustained increase of the parasite burden is in most mouse strains only observed in the spleen, but not in the liver (Kirkpatrick and Farrell, 1984b; Smelt et al., 1997). Nevertheless, the mouse models for cutaneous and VL have proven useful for studying the innate and adaptive immune response to a diverse set of Leishmania species causing CL (e.g., L. L. major, L. V. braziliensis, L. L. mexicana, L. L. amazonensis) or VL (see above).
In the following I will review the contribution of NK cells to the development of protective immunity in cutaneous and VL of mice and man. For the sake of brevity the names of the parasite species will be given without the subgenus.
Recruitment and Tissue Infiltration of NK Cells
Twenty-four hours after s.c. infection of C57BL/6 mice with L. major NK1.1+ CD3− NK cells were found to accumulate at the inoculation site following the prior infiltration of neutrophils. The immigration of NK cells was associated with an upregulation of the NK cell-activating chemokine IFN-γ-inducible protein (IP-10 or CXCL9) (Muller et al., 2001). Confocal steady-state and intravital two-photon real-time fluorescent imaging revealed that intradermal L. major infection caused the recruitment of NK cells (CD49b+, MECA-79-negative) from the blood to the draining lymph node, thereby leading to a 10-fold increase of the absolute number and a five-fold increase of the percentage of NK cells in lymph nodes within 24 h (Bajenoff et al., 2006). A similar sequential infiltration of the skin and the regional lymph node with neutrophils followed by NK cells (CD49b+) and macrophages was also observed in BALB/c mice after i.d. infection with L. infantum chagasi (Thalhofer et al., 2011).
In mouse VL elicited by i.v. infections with L. donovani, NK cells (CD3−CD49b+NKp46+MHC class II−) were located (1) in the red pulp and marginal zone of the spleen, where they increased three-fold from day 0 to day 28; and (2) within the granuloma (ca. 75%) and the parenchyma (ca. 25%) of the liver, in which the number of NK cells rose by ca. 30% during the first four weeks of infection (Maroof et al., 2008). Likewise, after i.v. infections with L. infantum NK cells were readily detectable in the spleen (Schleicher et al., 2007).
In human leishmaniasis NK cells were detected in lesions of patients with LCL, DCL, ML, or VL (Salaiza Suazo et al., 1999; Seixas Duarte et al., 2008; Tuon et al., 2008; Pereira et al., 2009). However, the findings have to be judged with care as the immunohistological analyses were frequently carried out with antibodies against CD57, a marker that is not selective for NK cells.
Activation of NK Cells
For obvious reasons the kinetics and molecular mechanisms of NK cell activation and deactivation have been best studied in the mouse models of CL and VL. NK cell activation results from the interaction with other cell-types, the stimulation by cytokines, and from the exposure to certain parasite factors. In cutaneous L. major infections of self-healing mouse strains, NK cell cytotoxic activity and IFN-γ production became readily detectable in the draining lymph node at day 1 of infection (Scharton and Scott, 1993; Bajenoff et al., 2006; Liese et al., 2007). The activation required (1) IL-2 released by antigen-primed CD4+ T cells (Scharton and Scott, 1993; Bihl et al., 2010), (2) IL-12 (Laskay et al., 1995; Scharton-Kersten et al., 1995), which was expressed by myeloid DC in a TLR9-dependent manner (Liese et al., 2007), (3) IFN-α/β (expressed by macrophages) and IFN-α/β-induced iNOS (generated by macrophages and NK cells) (Diefenbach et al., 1998, 1999), (4) the receptor tyrosine kinase Tyk2 (Diefenbach et al., 1999; Schleicher et al., 2004) and (5) the transcription factor IRF-2 (Lohoff et al., 2000). In the absence of Tyk2 kinase NK cell activity could be partially restored by treatment with IFN-α/β, but not by exogenous IL-12 (Schleicher et al., 2004).
The activation of NK cells was equally rapid in C57BL/6 mice infected i.v. with L. infantum. IFN-γ-expressing NK cells and NK cell cytotoxic activity were detectable in the spleen as early as 12 h after infection. This early splenic NK cell response was abolished by the depletion of CD11c+ myeloid DC, by a deficiency of the TLR9 receptor, by the deletion of the IL-12p35 subunit or by the treatment of the mice with an anti-IL-2 receptor antibody (Schleicher et al., 2007) (S. Haeberlein, U. Schleicher, and C. Bogdan, unpublished observations). In contrast, in IL-18−/− mice the activation of splenic NK cells was only reduced by 50% (Haeberlein et al., 2010) and in IFN-α/β-receptor−/− mice both the production of IFN-γ and the cytolytic activity of NK cells remained largely intact (Schleicher et al., 2007). A lack of IL-15 did not impair the activation of the residual NK cell population at all (Haeberlein et al., 2010). The NK cell-activating effect of IL-18 in vivo was not due to an improved IL-12-responsiveness, but resulted from an up-regulation of Fas-ligand on the NK cell surface (Haeberlein et al., 2010). Whereas splenic CD11c+ myeloid DC were responsible for the early IL-12 release (Schleicher et al., 2007), the cellular source of IL-18 has not yet been identified (Figure 1). An early (day 1 and day 3) IFN-γ response was also seen in BALB/c mice infected i.v. with L. donovani, but not in C.B-17 scid mice lacking T and B lymphocytes, which supports the role of T cell-derived IL-2 in NK cell activation (see above) and argues against a direct activation of mouse NK cells by this parasite species (Kaye and Bancroft, 1992; Engwerda et al., 1996). IL-12p40+ DC were readily observed in the white pulp of the spleen at day 1 after infection of BALB/c mice with L. donovani (Gorak et al., 1998).
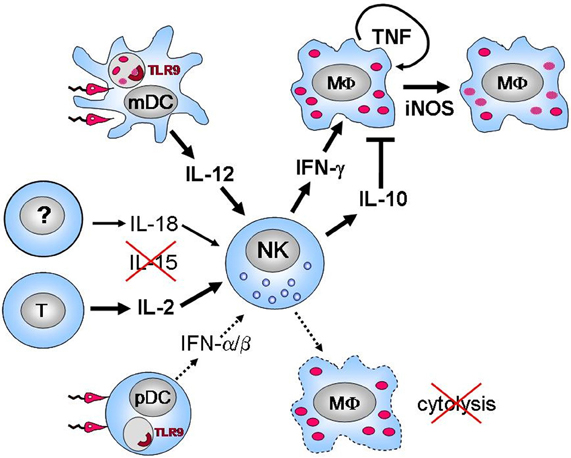
Figure 1. Current of view of the activation and function of NK cells in experimental visceral leishmaniasis (i.v. infection of mice with L. infantum or L. donovani).Leishmania promastigotes are endocytosed by CD11c+ myeloid DC, which subsequently produce the NK cell-activating cytokine IL-12 in a TLR 9-dependent manner (Schleicher et al., 2007). In addition to IL-12 the cytokine IL-2 (in analogy to previous findings in mouse L. major infections (Scharton and Scott, 1993; Bihl et al., 2010) presumably derived from CD4+ T lymphocytes) is also essential for the early activation of NK cells, whereas IL-18 (the source of which is currently unknown) only plays a contributory role and IL-15 is completely dispensable in this process (Haeberlein et al., 2010) (S. Haeberlein, U. Schleicher, and C. Bogdan, unpublished observations). Exposure of plasmacytoid dendritic cells (pDC) to Leishmania promastigotes leads to a massive release of type I interferons (IFN-α/β), which, however, is of minor relevance for the activation of splenic NK cells (Schleicher et al., 2007), unlike to the cutaneous infection model (Diefenbach et al., 1998). Infected myeloid cells such as macrophages (MΦ) are resistant to NK cell lysis in vitro and in vivo. Instead, activated NK cells secrete IFN-γ, which activates MΦ for the killing of intracellular amastigotes, a process that requires TNF and iNOS expressed by the MΦ (Prajeeth et al., 2011). NK cell-derived IL-10 might antagonize this process later during infection (Maroof et al., 2008).
In the mouse models of cutaneous and VL the only parasite factor that has been identified to date to be indirectly involved in the activation of NK cells is Leishmania DNA. Genomic, but not mitochondrial (kinetoplast) DNA of L. major, L. infantum, or L. braziliensis promastigotes activated myeloid and plasmacytoid DC for the production of IL-12 and IFN-α/β, respectively, in a strictly TLR9-dependent manner (Liese et al., 2007; Schleicher et al., 2007) (Figure 1). The genomic DNA of Leishmania completely mimicked the activity of total (live or dead) parasites. Importantly, no evidence was obtained for a direct activation of mouse splenic NK cells by Leishmania DNA or intact promastigotes, which is in line with the absence of TLR9 on highly purified mouse splenic NK cells (Schleicher et al., 2007).
In the human system various products of Leishmania have been described to directly or indirectly activate NK cells. Purified L. major lipophosphoglycan (LPG), the major surface glycophospholipid of promastigotes, was shown to bind to TLR2, to enhance the TLR2 protein expression on the membrane of human NK cells, and to trigger their production of TNF and IFN-γ (Becker et al., 2003). This finding is in accordance with the low levels of TLR2 mRNA previously detected in primary human NK cells (Hornung et al., 2002). In another study, however, live promastigotes of L. aethiopica, L. mexicana, or L. donovani directly activated NK cells from naive human donors in the absence of myeloid cells and IL-12 for the production of IFN-γ even when LPG- or proteophosphoglycan-deficient parasite mutants were used, a finding which questions the stimulatory role of LPG (Nylen et al., 2003). In a later publication by the same group two strains of L. major behaved differently and failed to trigger NK cell IFN-γ release (Lieke et al., 2008). When peripheral blood mononuclear cells (PBMNC) from healthy donors were exposed to the L. braziliensis homolog of the eukaryotic ribosomal elongation and initiation factor 4α (LeIF) (Borges et al., 2001), to the 36 kDa L. major homolog of receptors for activated C-kinase (LACK) (Maasho et al., 2000), or to the cysteine proteinase P-2 of L. aetiopica amastigotes (Nylen et al., 2004), NK cells showed strong expression of IFN-γ. In all these cases, however, the activation of NK cells was dependent on the presence of adherent myeloid cells (producing the NK cell-stimulating cytokines IL-12 and IL-18), which argues against a direct effect of leishmanial products on NK cells. PBMNC from Ethiopian patients with active LCL also reacted to recombinant LACK antigen and to the P-2 and P-8 antigens of L. aethiopica amastigotes, with NK cells showing the most prominent proliferative response (Maasho et al., 2001, 2003).
Together, these data show that the activation of NK cells in mouse and human leishmaniasis primarily occurs via an interaction with myeloid cells and requires the production of NK cell-stimulatory cytokines (Table 1).
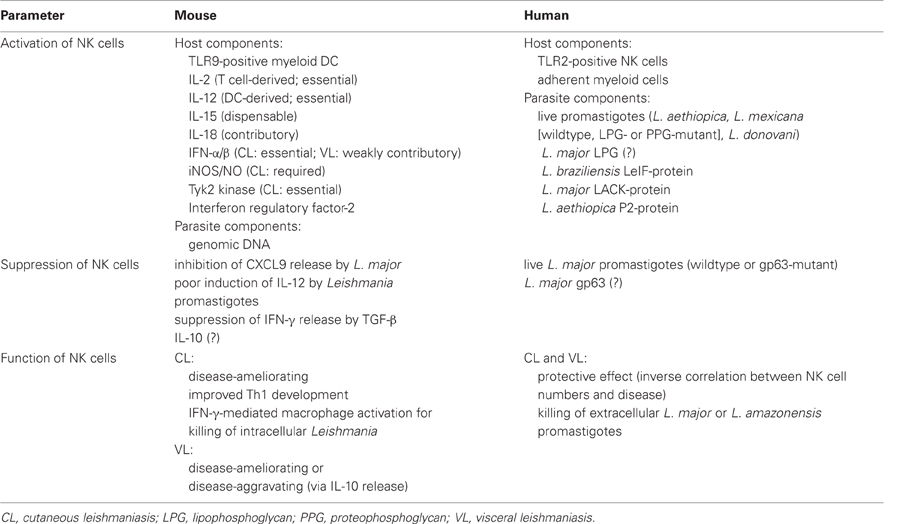
Table 1. Activation, suppression and function of NK cells in mouse and human cutaneous and visceral leishmaniasis (for references see text).
Suppression of NK Cell Activity
In experimental CL (L. major) and VL (L. infantum, L. donovani) the activation of NK cells in the draining lymph node and the spleen is characterized by a strong initial peak (12–48 h post-infection) and a steady decline thereafter (Kirkpatrick and Farrell, 1984a,b; Scharton and Scott, 1993; Schleicher et al., 2007); in experimental VL the NK cell activity might even fall below the levels in uninfected control mice (Kirkpatrick and Farrell, 1984a,b). Several factors might account for this transient NK cell activation and subsequent suppression. First, L. major is able to inhibit the IFN-γ-induced expression of the NK cell-attracting chemokine IP-10 (CXCL9) by neutrophils (van Zandbergen et al., 2002), which might prevent an ongoing influx of NK cells from the blood. Second, the NK cell response might be curtailed by cytokines that are known to inhibit NK cell proliferation and/or activity. In non-healing BALB/c mice, which show a considerably weaker NK cell activity after L. major infection than self-healing mouse strains (Scharton and Scott, 1993; Laskay et al., 1995; Mattner et al., 2004), expression of a dominant negative mutant of the transforming growth factor-β receptor II (dnTGF-βRII) led to a persistently increased number of NK cells and a higher percentage of IFN-γ+ NK cells in the draining lymph nodes as compared to control BALB/c mice (Laouar et al., 2005). This finding is in line with the previous observation that TGF-β is much more abundant in the skin lesions of L. major-infected BALB/c compared to C57BL/6 mice (Stenger et al., 1994) and that the IFN-γ production of BALB/c lymph node cells at day 2 after L. major infection is 20-fold increased in the presence of anti-TGF-β1/β2/β3 antibodies in the culture (Scharton-Kersten et al., 1995). The IFN-γ expression of human NK cells is also susceptible to suppression by TGF-β (Yu et al., 2006), although this has not yet been formally demonstrated in patients with leishmaniasis. Another candidate for the limitation of the NK cell response following Leishmania infections is the cytokine IL-10, which is generated by macrophages, Th1 cells, and regulatory T cells (Assenmacher et al., 1994; Kane and Mosser, 2001; Belkaid et al., 2002; Anderson et al., 2007; Nagase et al., 2007), but also by activated mouse and human NK cells (including those from patients with LCL) (Akuffo et al., 1999; Maasho et al., 2003; Maroof et al., 2008). It should be emphasized that both NK cell-inhibitory and NK cell-stimulatory effects have been described for IL-10 in various settings (e.g., Shibata et al., 1998; Tu et al., 2008). The role of IL-10 for NK cell activation versus suppression in leishmaniasis remains to be defined. Third, certain Leishmania species (e.g., L. tropica, L. mexicana, L. amazonensis, L. donovani), notably in their amastigote stage, are poor inducers of IL-12 production by DC, which might account for the limited NK cell response during prolonged infections in vivo (Bennett et al., 2001; McDowell et al., 2002; Maroof and Kaye, 2008; Sanabria et al., 2008). Fourth, the activation or survival of NK cells might be negatively regulated by the parasite itself. In this respect, intact L. major promastigotes (Lieke et al., 2008), crude antigenic fractions of L. major (Turhan et al., 1997) as well as the 63 kDa glycoprotein of L. major (gp63, leishmanolysin, or Leishmania major surface protease) (Lieke et al., 2008) were shown to inhibit the IFN-γ production, proliferation and/or cytotoxicity of human NK cells. gp63 was shown to bind to purified human NK cells, to inhibit their IL-2-driven proliferation, and to downregulate various NK cell receptors (CD16, CD56, NKp30, NKp44) (Lieke et al., 2008). A dominant NK cell-regulatory function of gp63, however, was questioned by the observation that in a less artificial situation, i.e., in a culture of total PBMNC, the NK cell-suppressive effect of L. major parasites was also observed with gp63-deficient mutants (Lieke et al., 2008). A recently observed final facet of the interaction between human NK cells and Leishmania might be the induction of non-apoptotic NK cell death immediately after their contact with promastigote parasites (Lieke et al., 2011).
Together, these data argue for both direct and indirect modes of NK cell suppression during mouse and human L. major infections (Table 1).
Function of NK Cells in Mouse and Human Leishmaniasis
Phenotypic Analyses
In mouse CL it is thought that NK cells contribute to a protective immune response against Leishmania parasites. This view has been based on the increased tissue parasite load and/or the aggravated course of infection in transiently NK cell-depleted L. major- or L. amazonensis-infected mice and on the disease-amelioriating effect of the in vivo activation or the transfer of NK cells in these models (Laskay et al., 1993; Scharton and Scott, 1993; Laurenti et al., 1999; Sanabria et al., 2008). There are two caveats about the antibody-based NK cell depletion experiments (Laskay et al., 1993; Scharton and Scott, 1993): first, the antibody application was limited to a time period of 2–7 days prior to and 14 days after infection, which offers an explanation that the ultimate outcome of the infection was indistinguishable in the treatment and control group (Laskay et al., 1993). Second, the antibodies used (anti-asialo-GM1; anti-NK1.1) not only target CD3−NK1.1+ NK cells, but also CD3+NK1.1+ natural killer T (NKT) as well as CD8+ T cells that can acquire the expression of NK1.1 or asialo-GM1 (Assarsson et al., 2000; Slifka et al., 2000). NKT cells were later shown to participate in the control of L. major in the skin lesions (Mattner et al., 2006). As an alternative approach, a T- and NK-cell-deficient transgenic C57BL/6 × CBA/J mouse strain expressing high copy numbers of the human CD3ε gene (tgε26) was used, as neonatal transplantation of wild-type bone marrow cells into these mice was found to result in normal amounts of T cells, but a massively reduced number of NK cells. When reconstituted tgε 26 mice (NK−T+) were infected with L. major, the parasite burden in the skin lesion and the clinical course of infection was comparable to the respective NK cell-proficient control mice (Satoskar et al., 1999). This result contrasts with observations made with the above-mentioned dnTGF-βRII BALB/c mice, in which the enhanced number and IFN-γ expression of NK cells led to the control of L. major in otherwise non-healing BALB/c mice (Laouar et al., 2005). With the exception of the activating NK cell receptor Ly49H, which turned out to be dispensable for the course of L. major infection in C57BL/6 mice (Fodil-Cornu et al., 2008), there is no information to date on the possible involvement of other NK cell receptors during the immune response to Leishmania parasites.
In experimental VL the first evidence for a protective role of NK cells was obtained by the analysis of beige (bg/bg) mice, which lack the gene for the lysosomal trafficking regulator (LYST or Beige gene). This genetic defect leads to abnormally enlarged lysosomes and lysosome-related organelles, to degranulation defects and to a defect of NK cell cytotoxicity (Roder and Duwe, 1979), whereas the IFN-γ production of NK cells apparently remains intact (Kawase et al., 1983; Mohan et al., 1997). Although the Beige mutation is not specific for NK cells [reference (Wilson et al., 2008) and references therein], the failure of C57BL6J bg/bg mice to control L. donovani amastigotes in the spleen (Kirkpatrick and Farrell, 1982) could be corrected by the transfer of syngeneic NK cells derived from a IL-2-dependent NK cell line (Kirkpatrick et al., 1985). The finding that the therapeutic effect of recombinant IL-12 in L. donovani-infected mice was prevented in anti-asialo-GM1-treated mice is likewise compatible with a beneficial role of NK cells (Murray and Hariprashad, 1995), but might also result from the activity of NKT cells (Amprey et al., 2004). Independent of a possible protective effect of NK cells in wild-type mice, additional studies in cytokine- or amphotericin B-treated nude mice (B- and T-cell-deficient) and euthymic bg/bg mice (defective NK cells) as well as in the hamster model of VL revealed that NK cells are neither sufficient nor essential to control L. donovani (Murray et al., 1995; Sartori et al., 1999; Murray, 2005). In addition, one study even demonstrated that activated, IL-10-expressing NK cells antagonized the control of L. donovani in the spleen and in the liver as shown by cell depletion and adoptive transfer experiments (Maroof et al., 2008) (Figure 1).
Several findings indicate a protective role of NK cells in human leishmaniasis. These include (1) the enhanced proliferation of NK cells within PBMNC from cured or control individuals residing in an endemic area as compared to patients with active LCL lesions after in vitro stimulation with the respective parasite antigen (L. aethiopica) (Maasho et al., 1998); (2) the influx of NK cells into lesions of DCL patients who showed a response to treatment (Salaiza Suazo et al., 1999; Pereira et al., 2009); (3) the suppression of cytolytic NK cell activity in patients with active VL, which could be restored in vitro by stimulation with IL-2 (Manna et al., 1993); and (4) the normalization of NK cell numbers following successful chemotherapy of VL (Cenini et al., 1993).
Mechanistic Analyses
Despite the caveats discussed above there is no doubt that NK cells are rapidly activated during CL and VL. The phenotypic observations that support a host-protective function of NK cells in leishmaniasis raise the question of possible underlying mechanisms. There is evidence for several scenarios. First, considering the documented in vitro interaction between activated human NK cells and L. major promastigotes (Lieke et al., 2008, 2011) and the susceptibility of L. major to granulysin (Stenger et al., 1998), a major cytotoxic effector molecule of human NK cells and CD8+ T cells, direct lysis of extracellular parasites is feasible. Indeed, two reports showed killing of L. major or L. amazonensis promastigotes by (IL-2 activated) human NK cells (Aranha et al., 2005; Lieke et al., 2011), whereas in two other studies (IL-2 activated) mouse splenic NK cells and/or human peripheral blood NK cells were unable to lyse extracellular L. donovani promastigotes (Manna et al., 2010) or amastigotes (Saha et al., 1999). The relevance of these findings for the control of infection remains unclear as a direct encounter between NK cells and free Leishmania parasites has not yet been demonstrated in vivo (Moreno et al., 2010).
A second mechanism for the protective function of NK cells could be the lysis of Leishmania-infected host cells. This possibility appears principally realistic, because macrophages, DC and NK cells have been detected in close proximity to each other in the medulla and paracortex of L. major-infected lymph nodes (Bajenoff et al., 2006) and in the marginal zone and periarteriolar lymphocytic sheaths of L. donovani-infected spleens (Gorak et al., 1998; Lang et al., 2000; Maroof et al., 2008). Two in vitro studies claimed lysis of BALB/c macrophages infected with L. major or L. amazonensis promastigotes by IL-2-activated splenic killer cells (LAK) (Resnick et al., 1988; Aranha et al., 2005). The meaningfulness of the obtained results, however, is questionable as (1) the LAK preparations were either phenotypically not characterized (Resnick et al., 1988) or contained ca. 15% NKT and/or CD8+ T cells (Aranha et al., 2005), which are known to lyse and activate Leishmania-infected myleoid cells (Stefani et al., 1994; Campos-Martin et al., 2006); (2) extremely high LAK-macrophage ratios (≥10:1) and Leishmania-macrophage ratios (≥40:1) were used (Resnick et al., 1988); and (3) the analysis lacked a quantification and the use of uninfected control target cells (Aranha et al., 2005). Our recent detailed analysis using mouse bone marrow-derived resting, inflammatory peritoneal exudate macrophages or bone marrow-derived DC revealed that neither L. major nor L. infantum promastigotes or amastigotes modulated the expression of activating or inhibitory NK cell ligands. Accordingly, myeloid cells infected with Leishmania parasites did not become targets for the cytolytic activity of activated NK cells in vitro and in vivo (Prajeeth et al., 2011) (Figure 1). Likewise, J774G8-1 tumor macrophage cells infected with L. mexicana were not killed when exposed to normal spleen cells from mouse strains with high or low NK cell activity (Merino and Cruz, 1984). Immature human DC infected with L. infantum showed an upregulation of HLA-E, a ligand for the NK cell inhibitory receptor CD94/NKG2A, which made them also resistant to NK cell-mediated cytotoxicity (Campos-Martin et al., 2006). As expected from these in vitro findings, the clinical course of L. major infection in C57BL/6J bg/bg mice was indistinguishable from the respective control mice (Kirkpatrick and Farrell, 1982). Why the beige mutation caused a lack of parasite control in the spleen of L. donovani-infected mice (Kirkpatrick and Farrell, 1982) despite the resistance of infected myeloid cells to NK cell-mediated lysis, is mechanistically not yet understood. It is possible that the phenotype results from the defect of NK cell cytotoxicity against non-myeloid target cells or from a defect of other cytotoxic cells (e.g., CD8+ T cells). In this context it is worth noting that intracellular parasite antigens were detected within human peripheral blood γ/δ T cell blasts upon exposure to L. donovani amastigotes and that these cells were lysed by human NK cells (Saha et al., 1999).
A third mechanism by which NK cells might convey protection in leishmaniasis is via their production of IFN-γ. Indeed, NK cells that were activated by IL-12 plus IL-18, stimulated macrophages for the killing of intracellular L. infantum amastigotes in an IFN-γ-, TNF-, and iNOS-dependent, but cell contact-independent manner. The source of the TNF was the macrophages rather than the NK cells (Prajeeth et al., 2011). There is also strong evidence that NK cell-derived IFN-γ paves the development of Th1 cells during mouse L. major infections, especially if the TGF-β-mediated suppression of this process is blocked (Laouar et al., 2005). On the other hand, it is important to bear in mind that (1) NK cells as the only source of IFN-γ are insufficient to control a L. major infection (Wakil et al., 1998); (2) Th1 cells will also develop in the absence of NK cell-derived IFN-γ (Wakil et al., 1998; Satoskar et al., 1999) and that (3) vaccine-induced protective immunity against Leishmania parasites can also occur after depletion of NK cells, although the vaccination efficacy might be somewhat reduced (Streit et al., 2001; Remer et al., 2010).
Conclusion and Outlook
The results obtained with experimental mouse models allow the conclusion that NK cells are non-essential for the resolution of CL. The same presumably holds also true for mouse VL, although definitive studies with NK cell-selective reagents or transgenic mice have not yet been performed. Ongoing studies in our group are addressing this issue with genetic models that allow constitutive versus inducible NK cell depletion. Independent of the question whether NK cells are essential or dispensable for the control of Leishmania parasites, there is convincing evidence for a protective function of NK cells in mouse CL, which is mainly based on the IFN-γ-mediated activation of macrophages and development of Th1 cells. In experimental VL it is possible that NK cells also exert immunosuppressive and disease-aggravating effects due to the production of IL-10, whereas the limited studies in patients with VL rather point to a host-protective role of NK cells.
Considering that NK cells are a potent source of IFN-γ, various approaches have been tested in experimental CL to stimulate NK cell activity in order to improve the course of infection or to enhance the protection conveyed by vaccines. Beneficial effects that were associated with the activation of NK cells were previously obtained in L. major- or L. amazonensis-infected mice by prior treatment with the TLR3-agonist polyinosinic:polycytidylic acid (poly I:C) (Laskay et al., 1993), by vaccination with live parasites containing the TLR9 agonist CpG 1826 (Laabs et al., 2009), by injection of an IL-12-expression plasmid (Sakai et al., 2000) or an IL-12-expressing adenovirus (Gabaglia et al., 1999), or by feeding an immunostimulatory fungal β-glucan (Yatawara et al., 2009). Whether the transfer of allogeneic NK cells, which yielded promising results in L. donovani-infected mice (Manna et al., 2010), is a clinically reasonable approach for the treatment of chronic Leishmania infections in humans, awaits further analysis. Attractive future strategies for NK cell-based immunotherapies include the application of reagents that specifically stimulate NK cells (e.g., antibodies crosslinking activating NK cell-receptors).
Conflict of Interest Statement
The author declares that the research was conducted in the absence of any commercial or financial relationships that could be construed as a potential conflict of interest.
Acknowledgments
The preparation of this manuscript and part of the results reported were supported by the Collaborative Research Center CRC 643 (project grant A6) and the IZKF Erlangen (project grant A49).
References
Ahmed, S., Colmenares, M., Soong, L., Goldsmith-Pestana, K., Munstermann, L., Molina, R., and McMahon-Pratt, D. (2003). Intradermal infection model for pathogenesis and vaccine studies of murine visceral leishmaniasis. Infect. Immun. 71, 401–410.
Akuffo, H., Alexis, A., Eidsmo, L., Saed, A., Nylen, S., and Maasho, K. (1999). Natural killer cells in cross-regulation of IL-12 by IL-10 in Leishmania antigen-stimulated blood donor cells. Clin. Exp. Immunol. 117, 529–534.
Amprey, J. L., Im, J. S., Turco, S. J., Murray, H. W., Illarionov, P. A., Besra, G. S., Porcelli, S. A., and Spath, G. F. (2004). A subset of liver NK T cells is activated during Leishmania donovani infection by CD1d-bound lipophosphoglycan. J. Exp. Med. 200, 895–904.
Anderson, C. F., Oukka, M., Kuchroo, V. J., and Sacks, D. (2007). CD4(+)CD25(−)Foxp3(−) Th1 cells are the source of IL-10-mediated immune suppression in chronic cutaneous leishmaniasis. J. Exp. Med. 204, 285–297.
Aranha, F. C. S., Ribeiro, U., Basse, P., Corbett, C. E. P., and Laurenti, M. D. (2005). Interleukin-2 activated natural killer cells may have a direct role in the control of Leishmania amazonensis promastigote and macrophage infection. Scand. J. Immunol. 62, 334–341.
Assarsson, E., Kambayashi, T., Sandberg, J. K., Hong, S., Taniguchi, M., Van Kaer, L., Ljunggren, H. G., and Chambers, B. J. (2000). CD8+ T cells rapidly acquire NK1.1 and NK cell-associated molecules upon stimulation in vitro and in vivo. J. Immunol. 165, 3673–3679.
Assenmacher, M., Schmitz, J., and Radbruch, A. (1994). Flow cytometric determination of cytokines in activated murine T helper lymphocytes: expression of interleukin-10 in interferon-g and in interleukin-4-expressing cells. Eur. J. Immunol. 24, 1097–1101.
Bajenoff, M., Breart, B., Huang, A. Y., Qi, H., Cazareth, J., Braud, V. M., Germain, R. N., and Glaichenhaus, N. (2006). Natural killer cell behavior in lymph nodes revealed by static and real-time imaging. J. Exp. Med. 203, 619–631.
Becker, I., Salaiza, N., Aguirre, M., Delgado, J., Carrillo-Carrasco, N., Gutierrez-Kobeh, L., Ruiz, A., Cervantes, R. Perez Torres, A., Cabrera, N., Gonzalez, A., Maldonado, C., and Isibasi, A. (2003). Leishmania lipophosphoglycan (LPG) activates NK cells through toll-like-receptor-2. Mol. Biochem. Parasitol. 130, 65–74.
Belkaid, Y., Mendez, S., Lira, R., Kadambi, N., Milon, G., and Sacks, D. L. (2000). A natural model of Leishmania major infection reveals a prolonged “silent” phase of parasite amplification in the skin before the onset of lesion formation and immunity. J. Immunol. 165, 969–977.
Belkaid, Y., Piccirillo, C. A., Mendez, S., Shevach, E. M., and Sacks, D. L. (2002). CD4+CD25+ regulatory T cells control Leishmania major persistence and immunity. Nature 420, 502–507.
Bennett, C. L., Misslitz, A., Colledge, L., Aebischer, T., and Blackburn, C. C. (2001). Silent infection of bone marrow-derived dendritic cells by Leishmania mexicana amastigotes. Eur. J. Immunol. 31, 876–883.
Bihl, F., Pecheur, J., Breart, B., Poupon, G., Cazareth, J., Julia, V., Glaichenhaus, N., and Braud, V. M. (2010). Primed antigen-specific CD4+ T cells are required for NK cell activation in vivo upon Leishmania major infection. J. Immunol. 185, 2174–2181.
Bogdan, C. (2008). Mechanisms and consequences of persistence of intracellular pathogens: leishmaniasis as an example. Cell. Microbiol. 10, 1221–1234.
Bogdan, C. (2012). Leishmaniasis in rheumatology, hematology, and oncology: epidemiological, immunological, and clinical aspects and caveats. Ann. Rheum. Dis. 71(Suppl. 2), i60–i66.
Bogdan, C., Donhauser, N., Döring, R., Röllinghoff, M., Diefenbach, A., and Rittig, M. G. (2000). Fibroblasts as host cells in latent leishmaniosis. J. Exp. Med. 191, 2121–2129.
Borges, M. M., Campos-Neto, A., Sleath, P., Grabstein, K. H., Morrissey, P. J., Skeiky, Y. A. W., and Reed, S. G. (2001). Potent stimulation of the innate immune system by a Leishmania brasiliensis recombinant protein. Infect. Immun. 69, 5270–5277.
Campos-Martin, Y., Colmenares, M., Gozalbo-Lopez, B., Lopez-Nunez, M., Savage, P. B., and Martinez-Naves, E. (2006). Immature human dendritic cells infected with Leishmania infantum are resistant to NK-mediated cytolysis but are efficiently recognized by NKT cells. J. Immunol. 176, 6172–6179.
Cenini, P., Berhe, N., Hailu, A., McGinnes, K., and Frommel, D. (1993). Mononuclear cell subpopulations and cytokine levels in human visceral leishmaniasis before and after chemotherapy. J. Infect. Dis. 168, 986–993.
Diefenbach, A., Schindler, H., Donhauser, N., Lorenz, E., Laskay, T., Macmicking, J., Röllinghoff, M., Gresser, I., and Bogdan, C. (1998). Type 1 interferon (IFN-α/β) and type 2 nitric oxide synthase regulate the innate immune response to a protozoan parasite. Immunity 8, 77–87.
Diefenbach, A., Schindler, H., Röllinghoff, M., Yokoyama, W., and Bogdan, C. (1999). Requirement for type 2 NO-synthase for IL-12 singnaling in innate immunity. Science 284, 951–955.
Engwerda, C. R., Smelt, S. C., and Kaye, P. M. (1996). An in vivo analysis of cytokine production during Leishmania donovani infection in scid mice. Exp. Parasitol. 84, 195–202.
Fodil-Cornu, N., Lee, S. H., Belanger, S., Makrigiannis, A. P., Biron, C. A., Buller, R. M., and Vidal, S. M. (2008). Ly49h-deficient C57BL/6 mice: a new mouse cytomegalovirus-susceptible model remains resistant to unrelated pathogens controlled by the NK gene complex. J. Immunol. 181, 6394–6405.
Gabaglia, C. R., Pedersen, B., Hitt, M., Burdin, N., Sercarz, E. E., Graham, F. L., Gauldie, J., and Braciak, T. A. (1999). A single intramuscular injection with an adenovirus-expressing IL-12 protects BALB/c mice against Leishmania major infection, while treatment with an IL-4-expressing vector increases disease susceptibility in B10.D2 mice. J. Immunol. 162, 753–760.
Gorak, P. M. A., Engwerda, C. R., and Kaye, P. M. (1998). Dendritic cells, but not macrophages produce IL-12 immediately following Leishmania donovani infection. Eur. J. Immunol. 28, 687–695.
Haeberlein, S., Sebald, H., Bogdan, C., and Schleicher, U. (2010). IL-18, but not IL-15, contributes to the IL-12-dependent induction of NK-cell effector functions by Leishmania infantum in vivo. Eur. J. Immunol. 40, 1708–1717.
Hornung, V., Rothenfusser, S., Britsch, S., Krug, A., Jahrsdörfer, B., Giese, T., Endres, S., and Hartmann, G. (2002). Quantitative expression of Toll-like receptor 1-10 mRNA in cellular subsets of human peripheral blood mononuclear cells and sensitivity to CpG oligodeoxynucleotides. J. Immunol. 168, 4531–4537.
Kane, M. M., and Mosser, D. M. (2001). The role of IL-10 in promoting disease progression in leishmaniasis. J. Immunol. 166, 1141–1147.
Kawase, I., Brooks, C. G., Kuribayashi, K., Olabuenaga, S., Newman, W., Gillis, S., and Henney, C. S. (1983). Interleukin 2 induces gamma-interferon production: participation of macrophages and NK-like cells. J. Immunol. 131, 288–292.
Kaye, P. M., and Bancroft, G. J. (1992). Leishmania donovani infection in scid mice: lack of tissue response and in vivo macrophage activation correlates with failure to trigger natural killer cell-derived gamma interferon production in vitro. Infect. Immun. 60, 4335–4342.
Kirkpatrick, C. E., and Farrell, J. P. (1982). Leishmaniasis in beige mice. Infect. Immun. 38, 1208–1216.
Kirkpatrick, C. E., and Farrell, J. P. (1984a). Mechanisms of depression of splenic natural killer cell function in C57BL/6 mice infected with Leishmania donovani. Cell. Immunol. 87, 601–612.
Kirkpatrick, C. E., and Farrell, J. P. (1984b). Splenic natural killer-cell activity in mice infected with Leishmania donovani. Cell. Immunol. 85, 201–214.
Kirkpatrick, C. E., Farrell, J. P., Warner, J. F., and Dennert, G. (1985). Participation of natural killer cells in the recovery of mice from visceral leishmaniasis. Cell. Immunol. 92, 163–171.
Laabs, E. M., Wu, W., and Mendez, S. (2009). Vaccination with live Leishmania major and CpG DNA promotes interleukin-2 production by dermal dendritic cells and NK cell activation. Clin. Vaccine Immunol. 16, 1601–1606.
Lang, T., Ave, P., Huerre, M., Milon, G., and Antoine, J. C. (2000). Macrophage subsets harbouring Leishmania donovani in spleens of infected BALB/c mice: localization and characterization. Cell. Microbiol. 2, 415–430.
Lanier, L. L. (2008). Up on the tightrope: natural killer cell activation and inhibition. Nat. Immunol. 9, 495–502.
Laouar, Y., Sutterwala, F. S., Gorelik, L., and Flavell, R. A. (2005). Transforming growth factor-b controls T helper type 1 cell development through regulation of natural killer cell interferon-γ. Nat. Immunol. 6, 600–607.
Laskay, T., Diefenbach, A., Röllinghoff, M., and Solbach, W. (1995). Early parasite containment is decisive for resistance to Leishmania major infection. Eur. J. Immunol. 25, 2220–2227.
Laskay, T., Röllinghoff, M., and Solbach, W. (1993). Natural killer cells participate in the early defense against Leishmania major infection in mice. Eur. J. Immunol. 23, 2237–2241.
Laurenti, M. D., Gidlund, M., Ura, D. M., Sinhorini, I. L., Corbett, C. E., and Goto, H. (1999). The role of natural killer cells in the early period of infection in murine cutaneous leishmaniasis. Braz. J. Med. Biol. Res. 32, 323–325.
Leclercq, V., Lebastard, M., Belkaid, Y., Louis, J., and Milon, G. (1996). The outcome of the parasitic process initiated by Leishmania infantum in laboratory mice. A tissue-dependent pattern controlled by the Lsh and MHC loci. J. Immunol. 157, 4537–4545.
Lieke, T., Nylen, S., Eidsmo, L., McMaster, W. R., Mohammadi, A. M., Khamesipour, A., Berg, L., and Akuffo, H. (2008). Leishmania surface protein gp63 binds directly to human natural killer cells and inhibits proliferation. Clin. Exp. Immunol. 153, 221–230.
Lieke, T., Nylen, S., Eidsmo, L., Schmetz, C., Berg, L., and Akuffo, H. (2011). The interplay between Leishmania promastigotes and human Natural Killer cells in vitro leads to direct lysis of Leishmania by NK cells and modulation of NK cell activity by Leishmania promastigotes. Parasitology 138, 1898–1909.
Liese, J., Schleicher, U., and Bogdan, C. (2007). TLR9 signalling is essential for the innate NK cell response in murine cutaneous leishmaniasis. Eur. J. Immunol. 37, 3424–3434.
Lohoff, M., Duncan, G. S., Ferrick, D., Mittrücker, H.-W., Bischof, S., Prechtl, S., Röllinghoff, M., Schmitt, E., Pahl, A., and Mak, T. W. (2000). Deficiency in the transcription factor interferon regulatory factor (IRF)-2 leads to severely compromised development of natural killer and T helper type 1 cells. J. Exp. Med. 192, 325–335.
Lykens, J. E., Terrell, C. E., Zoller, E. E., Divanovic, S., Trompette, A., Karp, C. L., Aliberti, J., Flick, M. J., and Jordan, M. B. (2010). Mice with a selective impairment of IFN-gamma signaling in macrophage lineage cells demonstrate the critical role of IFN-gamma-activated macrophages for the control of protozoan parasitic infections in vivo. J. Immunol. 184, 877–885.
Maasho, K., McMahon-Pratt, D., Raita, J., Raud, M., Britton, S., Soong, L., and Akuffo, H. (2003). Evaluation of amastigote reactive cells in human cutaneous leishmaniasis caused by Leishmania aethiopica. Clin. Exp. Immunol. 132, 316–322.
Maasho, K., Sanchez, F., Schurr, E., Hailu, A., and Akuffo, H. (1998). Indications of the protectice role of natural killer cells in human cutaneous leishmaniasis in an area of endemicity. Infect. Immun. 66, 2698–2704.
Maasho, K., Satti, I., Nylen, S., Guzman, G., Koning, F., and Akuffo, H. (2000). A Leishmania homologue of receptors for activated C-kinase (LACK) induces both interferon-gamma and interleukin-10 in natural killer cells of healthy blood donors. J. Infect. Dis. 182, 570–578.
Maasho, K., Wolday, D., Edjigu, M., Soderstrom, K., Britton, S., and Akuffo, H. (2001). Induction and abrogation of LACK reactive cells in the evolution of human leishmaniasis. Clin. Exp. Immunol. 124, 255–261.
Manna, P. P., Bharadwaj, D., Bhattacharya, S., Chakrabarti, G., Basu, D., Mallik, K. K., and Bandyopadhyay, S. (1993). Impairment of natural killer cell activity in Indian kala-azar: restoration of activity by interleukin-2, but not by alpha or gamma interferon. Infect. Immun. 61, 3565–3569.
Manna, P. P., Chakrabarti, G., and Bandyopadhyay, S. (2010). Innate immune defense in visceral leishmaniasis: cytokine mediated protective role by allogeneic effector cell. Vaccine 28, 803–810.
Maroof, A., Beattie, L., Zubairi, S., Svensson, M., Stager, S., and Kaye, P. M. (2008). Posttranscriptional regulation of IL-10 gene expression allows natural killer cells to express immunoregulatory function. Immunity 29, 295–305.
Maroof, A., and Kaye, P. M. (2008). Temporal regulation of interleukin-12p70 (IL-12p70) and IL-12-related cytokines in splenic dendritic cell subsets during Leishmania donovani infection. Infect. Immun. 76, 239–249.
Mattner, J., Donhauser, J., Werner-Felmayer, G., and Bogdan, C. (2006). NKT cells mediate organ-specific resistance against Leishmania major infection. Microbes Infect. 8, 354–362.
Mattner, J., Wandersee-Steinhäuser, A., Pahl, A., Röllinghoff, M., Majeau, G. R., Hochman, P. S., and Bogdan, C. (2004). Protection against progressive leishmaniasis by IFN-β. J. Immunol. 172, 7574–7582.
McDowell, M. A., Marovich, M., Lira, R., Braun, M., and Sacks, D. L. (2002). Leishmania priming of human dendritic cells for CD40 ligand-induced interleukin-12p70 secretion is strain and species dependent. Infect. Immun. 70, 3994–4001.
Merino, F., and Cruz, I. (1984). Natural killer activity in experimental cutaneous leishmaniasis. Int. Arch. Allergy Appl. Immunol. 73, 347–351.
Mitchell, G. F., Anders, R. F., Brown, G. V., Handman, E., Roberts-Thomson, I. C., Chapman, C. B., Forsyth, K. P., Kahl, L. P., and Cruise, K. M. (1982). Analysis of infection characteristics and antiparasite immune responses in resistant compared with susceptible hosts. Immunol. Rev. 61, 137–188.
Mohan, K., Moulin, P., and Stevenson, M. M. (1997). Natural killer cell cytokine production, not cytotoxicity, contributes to resistance against blood-stage Plasmodium chabaudi AS infection. J. Immunol. 159, 4990–4998.
Moreno, I., Dominguez, M., Cabanes, D., Aizpurua, C., and Torano, A. (2010). Kinetic analysis of ex vivo human blood infection by Leishmania. PLoS Negl. Trop. Dis. 4:e743. doi: 10.1371/journal.pntd.0000743
Mougneau, E., Bihl, F., and Glaichenhaus, N. (2011). Cell biology and immunology of Leishmania. Immunol. Rev. 240, 286–296.
Muller, K., van Zandbergen, G., Hansen, B., Laufs, H., Jahnke, N., Solbach, W., and Laskay, T. (2001). Chemokines, natural killer cells and granulocytes in the early course of Leishmania major infection in mice. Med. Microbiol. Immunol. 190, 73–76.
Murray, H. W. (2005). Prevention of relapse after chemotherapy in a chronic intracellular infection: mechanisms in experimental visceral leishmaniasis. J. Immunol. 174, 4916–4923.
Murray, H. W., Berman, J. D., Davies, C. R., and Saravia, N. G. (2005). Advances in leishmaniasis. Lancet 366, 1561–1577.
Murray, H. W., and Hariprashad, J. (1995). Interleukin 12 is effective treatment for an established systemic intracellular infection: experimental visceral leishmaniasis. J. Exp. Med. 181, 387–391.
Murray, H. W., Hariprashad, J., and Aguero, B. (1995). Antimicrobial response of a T cell-deficient host to cytokine therapy: effect of interferon-γ in experimental visceral leishmaniasis in nude mice. J. Infect. Dis. 171, 1309–1316.
Nagase, H., Jones, K. M., Anderson, C. F., and Noben-Trauth, N. (2007). Despite increased CD4+Foxp3+ cells within the infection site, BALB/c IL-4 receptor-deficient mice reveal CD4+Foxp3-negative T cells as a source of IL-10 in Leishmania major susceptibility. J. Immunol. 179, 2435–2444.
Nylen, S., Maasho, K., McMahon-Pratt, D., and Akuffo, H. (2004). Leishmanial amastigote antigen P-2 induces major histocompatibility complex class II-dependent natural killer-cell reactivity in cells from healthy donors. Scand. J. Immunol. 59, 294–304.
Nylen, S., Maasho, K., Soderstrom, K., Ilg, T., and Akuffo, H. (2003). Live Leishmania promastigotes can directly activate primary human natural killer cells to produce interferon-gamma. Clin. Exp. Immunol. 131, 457–467.
Pereira, L. I., Dorta, M. L., Pereira, A. J., Bastos, R. P., Oliveira, M. A., Pinto, S. A., Galdino, H. Jr. Mayrink, W., Barcelos, W., Toledo, V. P., Lima, G. M., and Ribeiro-Dias, F. (2009). Increase of NK cells and proinflammatory monocytes are associated with the clinical improvement of diffuse cutaneous leishmaniasis after immunochemotherapy with BCG/Leishmania antigens. Am. J. Trop. Med. Hyg. 81, 378–383.
Perona-Wright, G., Mohrs, K., Szaba, F. M., Kummer, L. W., Madan, R., Karp, C. L., Johnson, L. L., Smiley, S. T., and Mohrs, M. (2009). Systemic but not local infections elicit immunosuppressive IL-10 production by natural killer cells. Cell Host Microbe 6, 503–512.
Peters, N., and Sacks, D. (2006). Immune privilege in sites of chronic infection: Leishmania and regulatory T cells. Immunol. Rev. 213, 159–179.
Prajeeth, C. K., Haeberlein, S., Sebald, H., Schleicher, U., and Bogdan, C. (2011). Leishmania-infected macrophages are targets of NK cell-derived cytokines, but not of NK cell cytotoxicity. Infect. Immun. 79, 2699–2708.
Remer, K. A., Roeger, B., Hambrecht, C., and Moll, H. (2010). Natural killer cells support the induction of protective immunity during dendritic cell-mediated vaccination against Leishmania major. Immunology 131, 570–582.
Resnick, M., Roguel, N., Bercovier, H., Enk, C., Frankenburg, S., and Kedar, E. (1988). Lysis of murine macrophages infected with intracellular pathogens by interleukin 2-activated killer (LAK) cells in vitro. Cell. Immunol. 113, 214–219.
Rittig, M. C., and Bogdan, C. (2000). Leishmania-host cell interaction: complexities and alternative views. Parasitol Today 16, 292–297.
Roder, J., and Duwe, A. (1979). The beige mutation in the mouse selectively impairs natural killer cell function. Nature 278, 451–453.
Sacks, D. L., and Noben-Trauth, N. (2002). The immunology of susceptibility and resistance to Leishmania major in mice. Nat. Rev. Immunol. 2, 845–858.
Saha, A., Chakrabarti, G., Sen, S., and Bandyopadhyay, S. (1999). Leishmania donovani parasites interact with gamma/delta+ human peripheral blood T cells and induce susceptibility to NK cell-mediated lysis. Scand. J. Immunol. 50, 588–595.
Sakai, T., Hisaeda, H., Nakano, Y., Ishikawa, H., Maekawa, Y., Ishii, K., Nitta, Y., Miyazaki, J., and Himeno, K. (2000). Gene gun-mediated delivery of an interleukin-12 expression plasmid protects against infections with the intracellular protozoan parasites Leishmania major and Trypanosoma cruzi in mice. Immunology 99, 615–624.
Salaiza Suazo, N., Volkow, P. Prez Tamayo, R., Moll, H., Gillitzer, R., Prez-Torres, A., Prez-Montfort, R. Delgado Dominguez, J., Velasco-Castrejn, O., Crippa, M., and Becker, I. (1999). Treatment of two patients with diffuse cutaneous leishmaniasis caused by Leishmania mexicana modifies the immunohistological profile, but not the disease outcome. Trop. Med. Int. Health 4, 801–811.
Sanabria, M. X., Vargas-Inchaustegui, D. A., Xin, L., and Soong, L. (2008). Role of natural killer cells in modulating dendritic cell responses to Leishmania amazonensis infection. Infect. Immun. 76, 5100–5109.
Sartori, A., Kaneno, R., Baruzzi, N., and Peracoli, M. T. (1999). Increased natural killer activity does not prevent progression of experimental kala-azar. Rev. Inst. Med. Trop. Sao Paulo 41, 215–219.
Satoskar, A. R., Stamm, L. M., Zhang, X., Satoskar, A. A., Okano, M., Terhorst, C., David, J. R., and Wang, B. (1999). Mice lacking NK cells develop an efficient Th1 response and control cutaneous Leishmania major infection. J. Immunol. 162, 6747–6754.
Scharton-Kersten, T., Afonso, L. C. C., Wysocka, M., Trinchieri, G., and Scott, P. (1995). IL-12 is required for natural killer cell activation and subsequent T helper 1 cell development in experimental leishmaniasis. J. Immunol. 154, 5320–5330.
Scharton, T. M., and Scott, P. (1993). Natural killer cells are a source of IFN-g that drives differentiation of CD4+ T cell subsets and induces early resistance to Leishmania major in mice. J. Exp. Med. 178, 567–578.
Schleicher, U., Liese, J., Knippertz, I., Kurzmann, C., Hesse, A., Heit, A., Fischer, J. A., Weiss, S., Kalinke, U., Kunz, S., and Bogdan, C. (2007). NK cell activation in visceral leishmaniasis requires TLR9, myeloid DCs, and IL-12, but is independent of plasmacytoid DCs. J. Exp. Med. 204, 893–906.
Schleicher, U., Mattner, J., Blos, M., Schindler, H., Röllinghoff, M., Karaghiosoff, M., Müller, M., Werner-Felmayer, G., and Bogdan, C. (2004). Control of Leishmania major in the absence of Tyk2 kinase. Eur. J. Immunol. 34, 519–529.
Seixas Duarte, M. I., Tuon, F. F., Pagliari, C., Kauffman, M. R., and Brasil, R. A. (2008). Human visceral leishmaniasis expresses Th1 pattern in situ liver lesions. J. Infect. 57, 332–337.
Shibata, Y., Foster, L. A., Kurimoto, M., Okamura, H., Nakamura, R. M., Kawajiri, K., Justice, J. P., Van Scott, M. R., Myrvik, Q. N., and Metzger, W. J. (1998). Immunoregulatory roles of IL-10 in innate immunity: IL-10 inhibits macrophage production of IFN-gamma-inducing factors but enhances NK cell production of IFN-gamma. J. Immunol. 161, 4283–4288.
Slifka, M. K., Pagarigan, R. R., and Whitton, J. L. (2000). NK markers are expressed on a high percentage of virus-specific CD8+ and CD4+ T cells. J. Immunol. 164, 2009–2015.
Smelt, S. C., Engweda, C. R., McCrossen, M., and Kaye, P. M. (1997). Destruction of follicular dendritic cells during chronic visceral leishmaniasis. J. Immunol. 158, 3813–3821.
Stefani, M. M. A., Müller, I., and Louis, J. (1994). Leishmania major-specific CD8+ T cells are inducers and targets of nitric oxide produced by parasitized macrophages. Eur. J. Immunol. 24, 746–752.
Stenger, S., Hanson, D. A., Teitelbaum, R., Dewan, P., Niazi, K. R., Froelich, C. J., Ganz, T., Thoma-Uszynski, S., Melian, A., Bogdan, C., Porcelli, S. A., Bloom, B. R., Kresnky, A. M., and Modlin, R. L. (1998). An antimicrobial activity of cytolytic T cells mediated by granulysin. Science 282, 121–125.
Stenger, S., Thüring, H., Röllinghoff, M., and Bogdan, C. (1994). Tissue expression of inducible nitric oxide synthase is closely associated with resistance to Leishmania major. J. Exp. Med. 180, 783–793.
Stetson, D. B., Mohrs, M., Reinhardt, R. L., Baron, J. L., Wang, Z. E., Gapin, L., Kronenberg, M., and Locksley, R. M. (2003). Constitutive cytokine mRNAs mark natural killer (NK) and NK T cells poised for rapid effector function. J. Exp. Med. 198, 1069–1076.
Streit, J. A., Recker, T. J., Filho, F. G., Beverley, S. M., and Wilson, M. E. (2001). Protective immunity against the protozoan Leishmania chagasi is induced by subclinical cutaneous infection with virulent but not avirulent organisms. J. Immunol. 166, 1921–1929.
Sun, J. C., Beilke, J. N., and Lanier, L. L. (2009). Adaptive immune features of natural killer cells. Nature 457, 557–561.
Thalhofer, C. J., Chen, Y., Sudan, B., Love-Homan, L., and Wilson, M. E. (2011). Leukocytes infiltrate the skin and draining lymph nodes in response to the protozoan Leishmania infantum chagasi. Infect. Immun. 79, 108–117.
Tu, Z., Bozorgzadeh, A., Pierce, R. H., Kurtis, J., Crispe, I. N., and Orloff, M. S. (2008). TLR-dependent cross talk between human Kupffer cells and NK cells. J. Exp. Med. 205, 233–244.
Tuon, F. F., Gomes-Silva, A., Da-Cruz, A. M., Duarte, M. I., Neto, V. A., and Amato, V. S. (2008). Local immunological factors associated with recurrence of mucosal leishmaniasis. Clin. Immunol. 128, 442–446.
Turhan, A., Mirshahidi, S., Piskin, A. K., Citak, B., and Imir, T. (1997). Effects of crude antigenic fractions of Leishmania major on natural killer cell cytotoxicity, interferon-gamma and interleukin-4 secretion from peripheric blood lymphocytes of unexposed individuals. Immunol. Lett. 55, 115–118.
van Zandbergen, G., Hermann, N., Laufs, H., Solbach, W., and Laskay, T. (2002). Leishmania promastigotes release a granulocyte chemotactic factor and induce interleukin-8 release but inhibit gamma interferon-inducible protein 10 production by neutrophil granulocytes. Infect. Immun. 70, 4177–4184.
Wakil, A. E., Wang, Z. E., Ryan, J. C., Fowell, D. J., and Locksley, R. M. (1998). Interferon gamma derived from CD4(+) T cells is sufficient to mediate T helper cell type 1 development. J. Exp. Med. 188, 1651–1656.
Wilson, J., Huynh, C., Kennedy, K. A., Ward, D. M., Kaplan, J., Aderem, A., and Andrews, N. W. (2008). Control of parasitophorous vacuole expansion by LYST/Beige restricts the intracellular growth of Leishmania amazonensis. PLoS Pathog. 4:e1000179. doi: 10.1371/journal.ppat.1000179
Yatawara, L., Wickramasinghe, S., Nagataki, M., Takamoto, M., Nomura, H., Ikeue, Y., Watanabe, Y., and Agatsuma, T. (2009). Aureobasidium-derived soluble branched (1,3-1,6) beta-glucan (Sophy beta-glucan) enhances natural killer activity in Leishmania amazonensis-infected mice. Korean J. Parasitol. 47, 345–351.
Yu, J., Wei, M., Becknell, B., Trotta, R., Liu, S., Boyd, Z., Jaung, M. S., Blaser, B. W., Sun, J., Benson, D. M. Jr. Mao, H., Yokohama, A., Bhatt, D., Shen, L., Davuluri, R., Weinstein, M., Marcucci, G., and Caligiuri, M. A. (2006). Pro- and antiinflammatory cytokine signaling: reciprocal antagonism regulates interferon-gamma production by human natural killer cells. Immunity 24, 575–590.
Keywords: cutaneous leishmaniasis, visceral leishmaniasis, natural killer cells, toll-like receptors
Citation: Bogdan C (2012) Natural killer cells in experimental and human leishmaniasis. Front. Cell. Inf. Microbio. 2:69. doi: 10.3389/fcimb.2012.00069
Received: 25 March 2012; Paper pending published: 17 April 2012;
Accepted: 02 May 2012; Published online: 29 May 2012.
Edited by:
Albert Descoteaux, INRS - Institut Armand-Frappier, CanadaCopyright: © 2012 Bogdan. This is an open-access article distributed under the terms of the Creative Commons Attribution Non Commercial License, which permits non-commercial use, distribution, and reproduction in other forums, provided the original authors and source are credited.
*Correspondence: Christian Bogdan, Mikrobiologisches Institut - Klinische Mikrobiologie, Immunologie und Hygiene, Universitätsklinikum, Erlangen, Wasserturmstraß 3/5, D-91054 Erlangen, Germany. e-mail:Y2hyaXN0aWFuLmJvZ2RhbkB1ay1lcmxhbmdlbi5kZQ==