- 1Department of Internal Medicine, Division of Infectious Diseases and International Health, Center for Global Health, University of Virginia, Charlottesville, VA, USA
- 2Department of Biology, University of Virginia, Charlottesville, VA, USA
Enteric infections and diarrheal diseases constitute pervasive health burdens throughout the world, with rates being highest at the two ends of life. During the first 2–3 years of life, much of the disease burden may be attributed to infection with enteric pathogens including Salmonella, rotavirus, and many other bacterial, viral, and protozoan organisms; however, infections due to Clostridium difficile exhibit steady increases with age. Still others, like Campylobacter infections in industrialized settings are high in early life (<2 years old) and increase again in early adulthood (called the “second weaning” by some). The reasons for these differences undoubtedly reside in part in pathogen differences; however, host factors including the commensal intestinal microbial communities, immune responses (innate and acquired), and age-dependant shifts likely play important roles. Interplay of these factors is illustrated by studies examining changes in human gut microbiota with inflammatory bowel disease and irritable bowel syndrome. Recent gut microbial surveys have indicated dramatic shifts in gut microbial population structure from infants to young adults to the elders. An understanding of the evolution of these factors and their interactions (e.g., how does gut microbiota modulate the “inflamm-aging” process or vice versa) through the human life “cycle” will be important in better addressing and controlling these enteric infections and their consequences for both quality and quantity of life (often assessed as disability adjusted life-years or “DALYs”).
Defining Enteric “Disease”
Defining “pathogens” first requires defining “disease.” We propose defining enteric disease as far more than simply passage of unformed stools (i.e., “diarrhea”) from one end of a very long and functionally critical “tube” to sustaining healthy life. Therefore, a broader definition of enteric disease would include: ≥3 or more unformed stools per day and any documented intestinal infection associated with disrupted intestinal absorptive and/or barrier function. This may impair growth in young children or cognitive function. Implicit in this more comprehensive concept of enteric disease is an urgent need for improved biomarkers of impaired intestinal absorptive and barrier function.
Thus, an enteric pathogen may be classified as any microbe that is able to cause enteric “disease” as defined above. Microbial pathogenesis can involve direct invasion, signals triggering host inflammation or other changes, or secreted factors that damage the host directly (e.g., toxins), indirectly (e.g., microbial competition), and/or by exploiting other environmental host-associated factors to thrive.
Host Locale of Enteric Disease
The intestinal epithelium is a heterogeneous mixture of cells poised to respond to the closely positioned microbiota and luminal contents. The tight junctions that exist between intestinal epithelial cells provide a maintained barrier (Marchiando et al., 2010) that when breached by enteric pathogen or toxin can lead to leakage of luminal contents into the underlying lamina propria where immune cells reside and potentially instigate deleterious inflammatory and other physiological responses leading to diarrhea or disrupted absorptive function (Su et al., 2009). Conversely, nutritional status, intestinal microbiota, and stress have been shown to play roles in maintaining barrier function (Brewster et al., 1997; Yang et al., 2006; Zareie et al., 2006). Perturbations of nutritional status and alteration of the intestinal microbiota is evident in persistent malnutrition and enteric infections (Mondal et al., 2012). Changes in the intestinal architecture during a malnourished state include blunted villi, crypt hypertrophy, and altered levels of intraepithelial lymphocytes leading to impaired immune responses, nutrient absorption (Figure 1) and ultimately decline in early childhood growth (Guerrant et al., 2008; Moore et al., 2010).
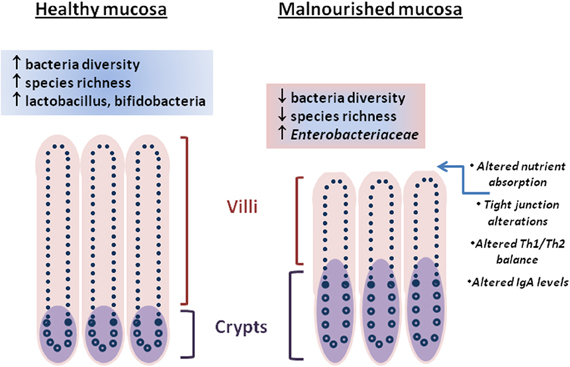
Figure 1. Differences between “healthy” and malnourished intestinal mucosa are represented by architectural changes (villi height/crypt depth, crypt hypertrophy), molecular changes (tight junction alteration, nutrient absorption), immune changes, and the intestinal microbiota. Cross-talk between the intestinal microbiota and intestinal mucosa through metabolites is unclear.
Effects of intestinal flora on the brain and enteric nervous system are now being elucidated. Early childhood enteric disease and malnutrition have been linked with impaired cognitive development (Lorntz et al., 2006; Laus et al., 2011). The profound effect of childhood malnutrition and enteric disease on cognitive function ultimately affects individual productivity in life; moreover, stunting early in life may increase risk for later obesity and possibly other chronic diseases (Guerrant et al., 2008; Victora et al., 2008). Factors such as APOE4 may affect enteric disease and cognitive function. The APOE4 allele in Brazilian children has been correlated with lower diarrhea burdens, and improved cognitive performance (Oria et al., 2005); cellular effects of APOE in the intestine and brain are under study (Vitek et al., 2009; Azevedo et al., 2012). While APOE4 provides protection to children with enteric disease, it is also linked with the neurodegenerative, Alzheimer's disease (Strittmatter et al., 1993). Currently, no apparent links appear to exist between APOE4 and malnutrition in the elderly (Matera et al., 2010); furthermore, limited studies have found associations between cognitive impairment and malnutrition or infections in the elderly, although causality remains unclear (Orsitto et al., 2009; Fagerstrom et al., 2011). The long-term impact of enteric infections in children is a subject of intense research; however, parallel studies in elders are lacking. Increasingly, research addressing the connection(s) between intestine, microbiota, and brain (Grenham et al., 2011) should illuminate host-microbe processes.
Life Stages, Incidence, Pathogens
By expanding the definition of enteric disease, the role of the host:pathogen (H:P) balance throughout life stages can be addressed in several contexts. Globally, the highest rates of diarrhea mortality tend to occur during early and late-life stages (Figure 2A; WHO data); however, rates in countries with the lowest GNI remain high throughout life. The age-associated trend is evident in cases of salmonellosis with high indices in infants and elders ≥65 (Trevejo et al., 2003). E. coli infections impacting early and late life stages are pathogen dependent; furthermore, diarrheagenic E. coli segregate geographically (Qadri et al., 2005; Ochoa et al., 2009; Snedeker et al., 2009; Opintan et al., 2010). Cryptosporidium may have its greatest impact in children <1yo or in already stunted children, (Checkley et al., 1997, 1998, 2008; Putignani and Menichella, 2010) rotaviruses in the first 2 years of life; Campylobacter in early childhood and young adulthood (the “second weaning”) (Ailes et al., 2008; da Silva et al., 2010; Soofi et al., 2011), then C. difficile infections clearly increasing steadily in both frequency and severity with increased age (Zilberberg et al., 2008). Given age-dependent changes in the incidence of enteric diseases, additional factors affecting the H:P balance include: intestinal microbiota, environment/exposure, and immune response.
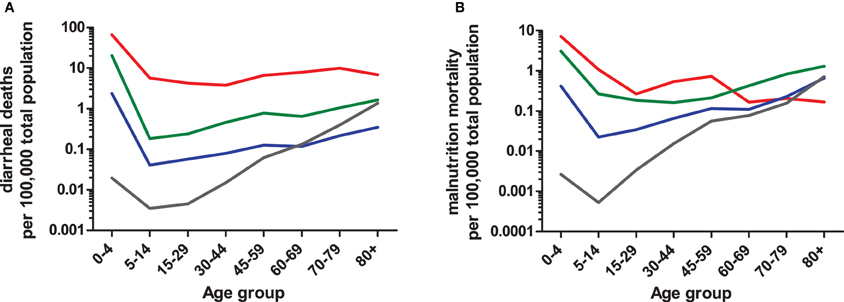
Figure 2. Age-dependent mortality rates due to diarrhea (A) or protein-energy malnutrition (B) based on 2008 WHO data. Numbers of diarrheal or malnutrition from the Global Burden of Disease in 2008 cases were compared to the total population sum of each WHO region (2012). WHO regions were grouped into four categories (i.e., low, low-middle, upper-middle, and upper) based on Gross National Income (GNI) brackets established by the World Bank (2012). The GNI income bracket most represented within a WHO region was used to combine data. For example, the Region of Americas (AMRB) consists of 26 countries, the majority of which (n = 18) are classified as upper-middle income countries and were therefore included in the upper-middle income data. The low-income (red line) category consists of African Region (AFR D and E) and South East Asian Region (SEAR D); low-middle (green line) consists of Region of the Americas (AMR D), Eastern Mediterranean Region (EMR D), Western Pacific Region (WPR B), and SEAR B; upper-middle (blue line) consists of AMR B, EMR B, and European Region (EURB and C); upper (grey line) consists of AMR A, EUR A, and WPR A. The mortality rate data are limited by the use of all-age population data, so actual age-dependent rates may differ.
Age-Dependent Shifts in the Intestinal Microbiota
The gut microbiota play important roles in maintaining the human health, including energy and nutrient extraction (Deguchi et al., 1985; Cummings and Macfarlane, 1991, 1997; Turnbaugh and Gordon, 2009), host immune system modulation and protection against pathogens (van der Waaij et al., 1971; Rolfe, 1997). Understanding of the diversity of human gut microbiota has improved dramatically in recent years largely due to culture-independent 16S rRNA based surveys. Recent studies have shown large interpersonal variations in the gut microbiota as a function of age (Figure 3), health status and diet (Dethlefsen et al., 2006; Ley et al., 2006; Mueller et al., 2006; Woodmansey, 2007; Tannock, 2008; O'Toole and Claesson, 2010; Claesson et al., 2011; Wu et al., 2011). Nevertheless, population based studies show three “enterotypes” with potential diagnostic value (Arumugam et al., 2011). The enterotypes are based on the preponderance of three genera: Bacteroides, Prevotella, and Ruminococcus. It is likely that additional enterotypes will surface when other health-related factors are considered (De et al., 2010; Monira et al., 2011; Wu et al., 2011).
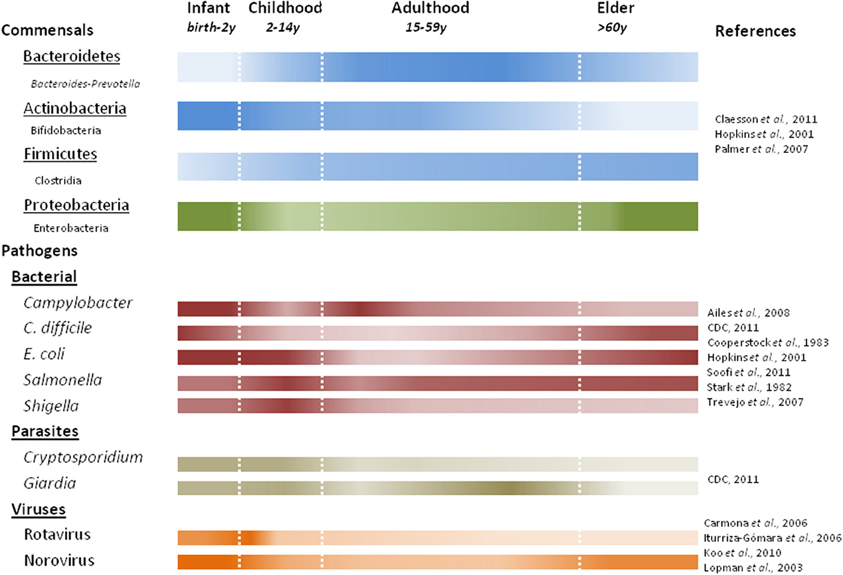
Figure 3. Predominant commensal and pathogenic microbes associated with human life stages. Color intensity of each bar correlates with presence or absence of commensal bacteria at the phylum (underlined) with examples listed below using noted references. Studies characterizing fecal microbiota prior to 2007 quantify the viable counts of bacteria, while later studies use DNA based methods.
The gut microbiota during early infancy is relatively simple but highly dynamic (Favier et al., 2002; Palmer et al., 2007). Incidental exposures (e.g., maternal microbiota, breastfeeding vs. formula) play a major role in seeding the neonatal gut (Bennet et al., 1991; Mandar and Mikelsaar, 1996; Penders et al., 2006; Adlerberth and Wold, 2009). As a result, the progression of early colonization is often chaotic and variable (Palmer et al., 2007). Facultative anaerobes, like E. coli (Hopkins et al., 2001; Salminen and Isolauri, 2006; Mariat et al., 2009) and Gram-negative obligate anaerobes (e.g., Bacteroides-Prevotella) dominate early followed by a predominance of bifidobacteria by three months of age (Mah et al., 2007; Mariat et al., 2009). Infection with Salmonella and pathogenic E. coli pose the greatest risks in early infancy when facultative anaerobes are predominant. Over the first year, through a series of successions and replacements, the infant microbial communities become more similar to one another, converging toward a more stable adult-like profile (Favier et al., 2002; Palmer et al., 2007), characterized by a preponderance of Bacteroidetes and Firmicutes. In addition to the composition difference, quantitative PCR show total bacterial counts in infants to be nearly ten-fold lower than in adults and elders (Mariat et al., 2009).
Gut microbiota in young adults are dominated by Bacteroidetes and Firmicutes (making up approximately 95% of the microbiota) with smaller fractions of Actinobacteria and Proteobacteria (Ley et al., 2006; Andersson et al., 2008; Tap et al., 2009). The peak numbers and diversity in gut microbial composition is achieved near the end of adolescence. Still, each adult's gut appears to harbor a unique microbial community that remains relatively stable through adulthood (Franks et al., 1998; Zoetendal et al., 1998; Vanhoutte et al., 2004; Leser and Molbak, 2009). One recent study suggested that stability may last longer than expected, and that aging starts to affect the gut microbiota after 65 years of age (Biagi et al., 2010). In addition, decreased intestinal motility (Brocklehurst, 1972; Macfarlane et al., 1989), dietary changes (Flint et al., 2007), and “inflamm-aging” (a chronic low-grade inflammation in elders) (Franceschi, 2007; Guigoz et al., 2008) all affect homeostasis of the gut microbial ecosystem. Recent studies indicate dramatic shifts in the composition of gut commensals in elders. When elders were compared to young adults, a decrease in the relative abundance of Bifidobacteria and Firmicutes was observed, accompanied by a commensurate increase in Bacteroides and facultative anaerobes (Hopkins and Macfarlane, 2002; Mariat et al., 2009; Claesson et al., 2011), although large compositional variations were also found among elderly individuals and populations (Mueller et al., 2006; Claesson et al., 2011). The decline in beneficial bifidobacteria is one of the most marked changes in the aging gut, manifested in both microbial abundance and species diversity (Mitsuoka et al., 1974; Benno et al., 1992; Mitsuoka, 1992; He et al., 2001; Hopkins et al., 2001; Hopkins and Macfarlane, 2002). Such a population shift could increase the susceptibility of elders to C. difficile infections (CDI) (Woodmansey, 2007; Guigoz et al., 2008), as a similar but more pronounced composition change has been observed in CDI patients (Hopkins et al., 2001; Hopkins and Macfarlane, 2002). Furthermore, a stable gut microbiota is crucial in preventing C. difficile overgrowth. This is supported by studies showing significant reductions in the microbial diversity in patients with recurrent CDI (Chang et al., 2008), association of CDI onset with altered microbiota composition(s) before antibiotic treatment (De La Cochetiere et al., 2008), and prevention of primary or recurrent CDI through probiotic therapy or fecal transplantation, respectively (Hickson et al., 2007; Khoruts et al., 2010). Bifidobacteria and butyrate-producing bacteria (e.g., Clostridium clusters IV and XIVa) have been suggested to play important roles in providing colonization resistance against C. difficile (Fallani et al., 2006; Rousseau et al., 2011); however, more comprehensive studies are required to substantiate these claims (Gore et al., 2008).
In addition to CDI, changes in the gut microbial composition have been linked to childhood allergies and inflammatory bowel diseases (IBD). It has been proposed that the gut microbiota in infants modulates the mucosal immune response to environmental allergens (Tannock, 2007). Interestingly, differences in the bifidobacterial populations have been associated with atopic diseases and the allergic status of children (Sepp et al., 1997; Ouwehand et al., 2001; Gore et al., 2008; Hong et al., 2010). IBD, such as Crohn's disease and ulcerative colitis are usually diagnosed in adolescence or early adulthood. Several lines of evidence suggest a microbial etiology in the development of IBD (D'Haens et al., 1998; Sellon et al., 1998; Gionchetti et al., 2000; Shen et al., 2001; Sartor, 2004). Previous studies have led to the hypothesis that altered gut microflora, excluding a specific pathogen, contribute to the etiology of IBD. Loss of microbial diversity and richness, especially in members of clostridial cluster IV (e.g., Faecalibacterium prausnitzii), was observed in Crohn's disease patients (Martinez-Medina et al., 2006; Scanlan et al., 2006; Frank et al., 2007; Sokol et al., 2008; Cucchiara et al., 2009). An increased Bacteroidetes:Firmicutes ratio and a predominance of opportunistic Proteobacteria have been reported in pediatric and adult IBD patients (Cucchiara et al., 2009). However, there is an ongoing debate on whether these changes are the cause or an effect of the disease (Sokol et al., 2008; Stecher and Hardt, 2008).
Common Themes of the Microbiota
As already mentioned, a loss of species diversity and richness within the microbiota has been observed in IBD and CDI; in addition, based on their relative abundance, facultative anaerobes including members of Proteobacteria appear to thrive. Increased levels of Proteobacteria are also seen in malnourished children or in patients with celiac's disease (Bonventre, 1990; Monira et al., 2011). On a gross level, these findings also tend to occur with older age when this microbiota “structure” could alter other host functions (e.g., immune response). For example, the relative abundance of Bacteroidetes accounted for <15% of the intestinal community in 71% of malnourished Bangladeshi children compared to >40% relative abundance in 71% of healthy cases.
Exposure and Host Responses
The environment plays a major role in acquisition of the pathogen; in many cases exposure to contaminated food and/or water are the main vehicles for pathogen transmission. Globally, countries in the low and low-middle income brackets face the highest rates of diarrhea-associated mortalities over much of the life cycle (Figure 2). The age-dependent mortality due to diarrhea evident at both ends of the life cycle has dramatic regional differences impacted in part by socio-economic category (e.g., developed vs. developing countries). Additional factors likely include polymicrobial infections, diet, host-microbiota relationship, waning maternal antibody and other regional specific co-morbidities. Indeed, this rationale could be applied to similar rates of diarrhea mortality among elders living in WHO regions falling into upper-middle and upper income categories in contrast to respective regional infant rates. In the case of the USA and Canada (i.e., AMRA), infection with C. difficile in healthcare or long-term care facility environments contribute to age-associated increases in mortality (Zilberberg et al., 2008). Depending on the setting, a patient may be treated with antibiotics that disrupt the “normal” microbiota resulting in susceptibility to opportunistic pathogens. Furthermore, prolonged stay within a hospital environment favors the transfer of nosocomial pathogens, in particular C. difficile (McFarland et al., 1989).
Conversely, exposure to pathogens in early infancy is greatest as children are weaned. In areas with high diarrhea burdens, both introduction of weaning foods and cessation of breastfeeding was associated with increased risk of dehydrating diarrhea (Guerrant et al., 1983; Fuchs et al., 1996). Consequently, increased malnutrition-based mortality is coincident with increased diarrhea mortality (Figure 2B). Adaptations in host immune responses and intestinal microbiota are crucial for the host to survive constant exposure to malnutrition or undernutrition and diarrhea disease throughout much of the life cycle, and these adaptations offer protection in later life when immune status wanes. In neonates, colonization and stabilization of intestinal microbial communities is intertwined with immune system development (Shulzhenko et al., 2011) with similar processes, albeit senescent in nature, occuring in elders (Biagi et al., 2010) suggesting age-dependant alterations of these systems are closely linked to pathogen resistance/susceptibility.
The innate immune system consists of frontline defenses to pathogens (Hooper and Macpherson, 2010) with parallel or subsequent responses by the adaptive arm of the immune system. Maturation and senescence of components in the adaptive immunity have been reviewed elsewhere (Adkins et al., 2004; Cancro et al., 2009). Antibody-mediated protection in utero and postpartum occurs through maternal, passive transfer and is cleared by 5 months of age; the age at which growth shortfalls often start (Victora et al., 2010). The impact of diet affects the host and subsequent adaptive responses; during malnutrition, both B- and T-cell responses are altered. In young mice and children, protein energy malnutrition decreases mucosal IgA responses potentially impacting the course of infection (Green and Heyworth, 1980; McGee and McMurray, 1988; Oberhelman et al., 1991). Conversely, patients with little to no IgA due to common variable immunodeficiency were more likely to be malnourished (Muscaritoli et al., 2001) suggesting important links between the immune system and nutritional status, which have been recently tied to the intestinal microbiota (Shulzhenko et al., 2011). The effect of malnutrition on T-cell responses during enteric infection have been examined in mice and humans with divergent results. In mice, tissue IFNγ increased in response to malnutrition and infection with Cryptosporidium or Heligmosomoides (Ing et al., 2000; Coutinho et al., 2008). Other in vivo studies show that protein-energy malnutrition alone ↑ serum IL-10 and ↓ IFNγ (Hillyer et al., 2007; Monk and Woodward, 2009) suggesting that infection further alters the immune response during malnutrition. Isolation and subsequent in vitro activation of CD4+ cells from malnourished or well-nourished, infected children, resulted in more IL-10 or IFNγ release characteristic of Th2 or Th1 bias, respectively (Rodriguez et al., 2005). The infecting pathogen(s) were not classified in this study, but samples from both gastrointestinal and respiratory infections were analyzed.
Conclusions
The process of aging is associated with continuous changes in environmental exposures that subsequently affect the immune system and host-associated microbiota. Within the host's shifting landscape, infections by pathogens that cause enteric disease likely exploit these shifts with resultant initiation of pathogenesis and/or establishment of a mutualistic relationship with the host (e.g., carrier) leading to potential dissemination of the pathogen. There is still much to be learned about how life stages and pertubations shape the gut microbial population, and how these changes influence health and disease, and predominant enteric pathogens. For whatever population shifts we can associate with disease(s), it is necessary to demonstrate causality and not a mere effect or unrelated association with the diseases to fulfill Koch's postulates. Future insights into the interdependency between environment-host-microbe will be essential for development of novel therapeutic approaches that treat or prevent enteric disease. Understanding the roles of these factors within the host will be complementary to external approaches (e.g., sanitation, water treatment) for controlling or eradicating pathogen dissemination.
Conflict of Interest Statement
The authors declare that the research was conducted in the absence of any commercial or financial relationships that could be construed as a potential conflict of interest.
References
Adkins, B., Leclerc, C., and Marshall-Clarke, S. (2004). Neonatal adaptive immunity comes of age. Nat. Rev. Immunol. 4, 553–564.
Adlerberth, I., and Wold, A. E. (2009). Establishment of the gut microbiota in Western infants. Acta Paediatr. 98, 229–238.
Ailes, E., Demma, L., Hurd, S., Hatch, J., Jones, T. F., Vugia, D., Cronquist, A., Tobin-D'Angelo, M., Larson, K., Laine, E., Edge, K., Zansky, S., and Scallan, E. (2008). Continued decline in the incidence of Campylobacter infections, FoodNet 1996–2006. Foodborne Pathog. Dis. 5, 329–337.
Andersson, A. F., Lindberg, M., Jakobsson, H., Backhed, F., Nyren, P., and Engstrand, L. (2008). Comparative analysis of human gut microbiota by barcoded pyrosequencing. PLoS ONE 3:e2836. doi: 10.1371/journal.pone.0002836
Arumugam, M., Raes, J., Pelletier, E., Le, P. D., Yamada, T., Mende, D. R., Fernandes, G. R., Tap, J., Bruls, T., Batto, J. M., Bertalan, M., Borruel, N., Casellas, F., Fernandez, L., Gautier, L., Hansen, T., Hattori, M., Hayashi, T., Kleerebezem, M., Kurokawa, K., Leclerc, M., Levenez, F., Manichanh, C., Nielsen, H. B., Nielsen, T., Pons, N., Poulain, J., Qin, J., Sicheritz-Ponten, T., Tims, S., Torrents, D., Ugarte, E., Zoetendal, E. G., Wang, J., Guarner, F., Pedersen, O., de Vos, W. M., Brunak, S., Dore, J., Antolin, M., Artiguenave, F., Blottiere, H. M., Almeida, M., Brechot, C., Cara, C., Chervaux, C., Cultrone, A., Delorme, C., Denariaz, G., Dervyn, R., Foerstner, K. U., Friss, C., van de Guchte, M., Guedon, E., Haimet, F., Huber, W., van Hylckama-Vlieg, J., Jamet, A., Juste, C., Kaci, G., Knol, J., Lakhdari, O., Layec, S., Le, R. K., Maguin, E., Merieux, A., Melo, M. R., M'rini, C., Muller, J., Oozeer, R., Parkhill, J., Renault, P., Rescigno, M., Sanchez, N., Sunagawa, S., Torrejon, A., Turner, K., Vandemeulebrouck, G., Varela, E., Winogradsky, Y., Zeller, G., Weissenbach, J., Ehrlich, S. D., and Bork, P. (2011). Enterotypes of the human gut microbiome. Nature 473, 174–180.
Azevedo, O. G., Oliveira, R. A., Oliveira, B. C., Zala-Milatovic, S., Araujo, C. V., Wong, D. V., Costa, T. B., Lucena, H. B., Lima-Junior, R. C., Ribeiro, R. A., Warren, C. A., Lima, A. A., Vitek, M. P., Guerrant, R. L., and Oria, R. B. (2012). Apolipoprotein E COG 133 mimetic peptide improves 5-fluorouracil-induced intestinal mucositis. BMC Gastroenterol. 12, 35.
Bennet, R., Eriksson, M., Tafari, N., and Nord, C. E. (1991). Intestinal bacteria of newborn Ethiopian infants in relation to antibiotic treatment and colonisation by potentially pathogenic gram-negative bacteria. Scand. J. Infect. Dis. 23, 63–69.
Benno, Y., Nakao, H., Uchida, K., and Mitsuoka, T. (1992). Impact of the advances in age on the gastrointestinal microflora of beagle dogs. J. Vet. Med. Sci. 54, 703–706.
Biagi, E., Nylund, L., Candela, M., Ostan, R., Bucci, L., Pini, E., Nikkila, J., Monti, D., Satokari, R., Franceschi, C., Brigidi, P., and De, V. W. (2010). Through ageing, and beyond: gut microbiota and inflammatory status in seniors and centenarians. PLoS ONE 5:e10667. doi: 10.1371/journal.pone.0010667
Bonventre, J. V. (1990). Calcium in renal cells. Modulation of calcium-dependent activation of phospholipase A2. Environ. Health Perspect. 84, 155–162.
Brewster, D. R., Manary, M. J., Menzies, I. S., O'Loughlin, E. V., and Henry, R. L. (1997). Intestinal permeability in kwashiorkor. Arch. Dis. Child 76, 236–241.
Brocklehurst, J. C. (1972). Bowel management in the neurologically disabled. The problems of old age. Proc. R. Soc. Med. 65, 66–69.
Cancro, M. P., Hao, Y., Scholz, J. L., Riley, R. L., Frasca, D., Dunn-Walters, D. K., and Blomberg, B. B. (2009). B cells and aging: molecules and mechanisms. Trends Immunol. 30, 313–318.
Carmona, R. C., Timenetsky, M. C., Morillo, S. G., and Richtzenhain, L. J. (2006). Human rotavirus serotype G9, Sao Paulo, Brazil, 1996–2003. Emerg. Infect. Dis. 12, 963–968.
CDC. (2011). Summary of notifiable diseases: United States, 2009. MMWR Morb. Mortal. Wkly. Rep. 58, 1–100.
Chang, J. Y., Antonopoulos, D. A., Kalra, A., Tonelli, A., Khalife, W. T., Schmidt, T. M., and Young, V. B. (2008). Decreased diversity of the fecal Microbiome in recurrent Clostridium difficile-associated diarrhea. J. Infect. Dis. 197, 435–438.
Checkley, W., Buckley, G., Gilman, R. H., Assis, A. M., Guerrant, R. L., Morris, S. S., Molbak, K., Valentiner-Branth, P., Lanata, C. F., and Black, R. E. (2008). Multi-country analysis of the effects of diarrhoea on childhood stunting. Int. J. Epidemiol. 37, 816–830.
Checkley, W., Epstein, L. D., Gilman, R. H., Black, R. E., Cabrera, L., and Sterling, C. R. (1998). Effects of Cryptosporidium parvum infection in Peruvian children: growth faltering and subsequent catch-up growth. Am. J. Epidemiol. 148, 497–506.
Checkley, W., Gilman, R. H., Epstein, L. D., Suarez, M., Diaz, J. F., Cabrera, L., Black, R. E., and Sterling, C. R. (1997). Asymptomatic and symptomatic cryptosporidiosis: their acute effect on weight gain in Peruvian children. Am. J. Epidemiol. 145, 156–163.
Claesson, M. J., Cusack, S., O'Sullivan, O., Greene-Diniz, R., de, W. H., Flannery, E., Marchesi, J. R., Falush, D., Dinan, T., Fitzgerald, G., Stanton, C., van, S. D., O'Connor, M., Harnedy, N., O'Connor, K., Henry, C., O'Mahony, D., Fitzgerald, A. P., Shanahan, F., Twomey, C., Hill, C., Ross, R. P., and O'Toole, P. W. (2011). Composition, variability, and temporal stability of the intestinal microbiota of the elderly. Proc. Natl. Acad. Sci. U.S.A. 108(Suppl. 1), 4586–4591.
Cooperstock, M., Riegle, L., Woodruff, C. W., and Onderdonk, A. (1983). Influence of age, sex, and diet on asymptomatic colonization of infants with Clostridium difficile. J. Clin. Microbiol. 17, 830–833.
Coutinho, B. P., Oria, R. B., Vieira, C. M., Sevilleja, J. E., Warren, C. A., Maciel, J. G., Thompson, M. R., Pinkerton, R. C., Lima, A. A., and Guerrant, R. L. (2008). Cryptosporidium infection causes undernutrition and, conversely, weanling undernutrition intensifies infection. J. Parasitol. 94, 1225–1232.
Cucchiara, S., Iebba, V., Conte, M. P., and Schippa, S. (2009). The microbiota in inflammatory bowel disease in different age groups. Dig. Dis. 27, 252–258.
Cummings, J. H., and Macfarlane, G. T. (1991). The control and consequences of bacterial fermentation in the human colon. J. Appl. Bacteriol. 70, 443–459.
Cummings, J. H., and Macfarlane, G. T. (1997). Role of intestinal bacteria in nutrient metabolism. JPEN J. Parenter. Enteral Nutr. 21, 357–365.
da Silva, Q. J., Lima, I. F., Havt, A., de Carvalho, E. B., Lima, N. L., Soares, A. M., Mota, R. M., Guerrant, R. L., and Lima, A. A. (2010). Campylobacter jejuni and Campylobacter coli in children from communities in Northeastern Brazil: molecular detection and relation to nutritional status. Diagn. Microbiol. Infect. Dis. 67, 220–227.
D'Haens, G. R., Geboes, K., Peeters, M., Baert, F., Penninckx, F., and Rutgeerts, P. (1998). Early lesions of recurrent Crohn's disease caused by infusion of intestinal contents in excluded ileum. Gastroenterology 114, 262–267.
De, F. C., Cavalieri, D., Di, P. M., Ramazzotti, M., Poullet, J. B., Massart, S., Collini, S., Pieraccini, G., and Lionetti, P. (2010). Impact of diet in shaping gut microbiota revealed by a comparative study in children from Europe and rural Africa. Proc. Natl. Acad. Sci. U.S.A. 107, 14691–14696.
Deguchi, Y., Morishita, T., and Mutai, M. (1985). Comparative studies on synthesis of water-soluble vitamins among human species of bifidobacteria. Agric. Biol. Chem. 49, 13–19.
De La Cochetiere, M. F., Durand, T., Lalande, V., Petit, J. C., Potel, G., and Beaugerie, L. (2008). Effect of antibiotic therapy on human fecal microbiota and the relation to the development of Clostridium difficile. Microb. Ecol. 56, 395–402.
Dethlefsen, L., Eckburg, P. B., Bik, E. M., and Relman, D. A. (2006). Assembly of the human intestinal microbiota. Trends Ecol. Evol. 21, 517–523.
Fagerstrom, C., Palmqvist, R., Carlsson, J., and Hellstrom, Y. (2011). Malnutrition and cognitive impairment among people 60 years of age and above living in regular housing and in special housing in Sweden: a population-based cohort study. Int. J. Nurs. Stud. 48, 863–871.
Fallani, M., Rigottier-Gois, L., Aguilera, M., Bridonneau, C., Collignon, A., Edwards, C. A., Corthier, G., and Dore, J. (2006). Clostridium difficile and Clostridium perfringens species detected in infant faecal microbiota using 16S rRNA targeted probes. J. Microbiol. Methods 67, 150–161.
Favier, C. F., Vaughan, E. E., de Vos, W. M., and Akkermans, A. D. (2002). Molecular monitoring of succession of bacterial communities in human neonates. Appl. Environ. Microbiol. 68, 219–226.
Flint, H. J., Duncan, S. H., Scott, K. P., and Louis, P. (2007). Interactions and competition within the microbial community of the human colon: links between diet and health. Environ. Microbiol. 9, 1101–1111.
Franceschi, C. (2007). Inflammaging as a major characteristic of old people: can it be prevented or cured? Nutr. Rev. 65, S173–S176.
Frank, D. N., St. Amand, A. L., Feldman, R. A., Boedeker, E. C., Harpaz, N., and Pace, N. R. (2007). Molecular-phylogenetic characterization of microbial community imbalances in human inflammatory bowel diseases. Proc. Natl. Acad. Sci. U.S.A. 104, 13780–13785.
Franks, A. H., Harmsen, H. J., Raangs, G. C., Jansen, G. J., Schut, F., and Welling, G. W. (1998). Variations of bacterial populations in human feces measured by fluorescent in situ hybridization with group-specific 16S rRNA-targeted oligonucleotide probes. Appl. Environ. Microbiol. 64, 3336–3345.
Fuchs, S. C., Victora, C. G., and Martines, J. (1996). Case-control study of risk of dehydrating diarrhoea in infants in vulnerable period after full weaning. BMJ 313, 391–394.
Gionchetti, P., Rizzello, F., Venturi, A., Brigidi, P., Matteuzzi, D., Bazzocchi, G., Poggioli, G., Miglioli, M., and Campieri, M. (2000). Oral bacteriotherapy as maintenance treatment in patients with chronic pouchitis: a double-blind, placebo-controlled trial. Gastroenterology 119, 305–309.
Gore, C., Munro, K., Lay, C., Bibiloni, R., Morris, J., Woodcock, A., Custovic, A., and Tannock, G. W. (2008). Bifidobacterium pseudocatenulatum is associated with atopic eczema: a nested case-control study investigating the fecal microbiota of infants. J. Allergy Clin. Immunol. 121, 135–140.
Green, F., and Heyworth, B. (1980). Immunoglobulin-containing cells in jejunal mucosa of children with protein-energy malnutrition and gastroenteritis. Arch. Dis. Child 55, 380–383.
Grenham, S., Clarke, G., Cryan, J. F., and Dinan, T. G. (2011). Brain-gut-microbe communication in health and disease. Front. Physiol. 2:94. doi: 10.3389/fphys.2011.00094
Guerrant, R. L., Kirchhoff, L. V., Shields, D. S., Nations, M. K., Leslie, J., de Sousa, M. A., Araujo, J. G., Correia, L. L., Sauer, K. T., McClelland, K. E., Trowbridge, F. L., and Hughes, J. M. (1983). Prospective study of diarrheal illnesses in northeastern Brazil: patterns of disease, nutritional impact, etiologies, and risk factors. J. Infect. Dis. 148, 986–997.
Guerrant, R. L., Oria, R. B., Moore, S. R., Oria, M. O., and Lima, A. A. (2008). Malnutrition as an enteric infectious disease with long-term effects on child development. Nutr. Rev. 66, 487–505.
Guigoz, Y., Dore, J., and Schiffrin, E. J. (2008). The inflammatory status of old age can be nurtured from the intestinal environment. Curr. Opin. Clin. Nutr. Metab. Care 11, 13–20.
He, F., Ouwehand, A. C., Isolauri, E., Hosoda, M., Benno, Y., and Salminen, S. (2001). Differences in composition and mucosal adhesion of bifidobacteria isolated from healthy adults and healthy seniors. Curr. Microbiol. 43, 351–354.
Hickson, M., D'Souza, A. L., Muthu, N., Rogers, T. R., Want, S., Rajkumar, C., and Bulpitt, C. J. (2007). Use of probiotic Lactobacillus preparation to prevent diarrhoea associated with antibiotics: randomised double blind placebo controlled trial. BMJ 335, 80.
Hillyer, L. M., Maliwichi, H. E., and Woodward, B. (2007). Blood serum interferon-gamma bioactivity is low in weanling mice subjected to acute deficits of energy or both protein and energy. Br. J. Nutr. 97, 528–534.
Hong, P. Y., Lee, B. W., Aw, M., Shek, L. P., Yap, G. C., Chua, K. Y., and Liu, W. T. (2010). Comparative analysis of fecal microbiota in infants with and without eczema. PLoS ONE 5:e9964. doi: 10.1371/journal.pone.0009964
Hooper, L. V., and Macpherson, A. J. (2010). Immune adaptations that maintain homeostasis with the intestinal microbiota. Nat. Rev. Immunol. 10, 159–169.
Hopkins, M. J., and Macfarlane, G. T. (2002). Changes in predominant bacterial populations in human faeces with age and with infection. J. Med. Microbiol. 51, 448–454.
Hopkins, M. J., Sharp, R., and Macfarlane, G. T. (2001). Age and disease related changes in intestinal bacterial populations assessed by cell culture, 16S rRNA abundance, and community cellular fatty acid profiles. Gut 48, 198–205.
Income Brackets defined by Worldbank. (2012). Available online at: http://data.worldbank.org/about/country-classifications/country-and-lending-groups
Ing, R., Su, Z., Scott, M. E., and Koski, K. G. (2000). Suppressed T helper 2 immunity and prolonged survival of a nematode parasite in protein-malnourished mice. Proc. Natl. Acad. Sci. U.S.A. 97, 7078–7083.
Iturriza-Gomara, M., Green, J., Brown, D. W., Ramsay, M., Desselberger, U., and Gray, J. J. (2000). Molecular epidemiology of human group A rotavirus infections in the United Kingdom between 1995 and 1998. J. Clin. Microbiol. 38, 4394–4401.
Khoruts, A., Dicksved, J., Jansson, J. K., and Sadowsky, M. J. (2010). Changes in the composition of the human fecal microbiome after bacteriotherapy for recurrent Clostridium difficile-associated diarrhea. J. Clin. Gastroenterol. 44, 354–360.
Koo, H. L., Ajami, N., Atmar, R. L., and DuPont, H. L. (2010). Noroviruses: the leading cause of gastroenteritis worldwide. Discov. Med. 10, 61–70.
Laus, M. F., Vales, L. D., Costa, T. M., and Almeida, S. S. (2011). Early postnatal protein-calorie malnutrition and cognition: a review of human and animal studies. Int. J. Environ. Res. Public Health 8, 590–612.
Leser, T. D., and Molbak, L. (2009). Better living through microbial action: the benefits of the mammalian gastrointestinal microbiota on the host. Environ. Microbiol. 11, 2194–2206.
Ley, R. E., Turnbaugh, P. J., Klein, S., and Gordon, J. I. (2006). Microbial ecology: human gut microbes associated with obesity. Nature 444, 1022–1023.
Lopman, B. A., Reacher, M., Gallimore, C., Adak, G. K., Gray, J. J., and Brown, D. W. (2003). A summertime peak of “winter vomiting disease”: surveillance of noroviruses in England and Wales, 1995 to 2002. BMC. Public Health 3, 13.
Lorntz, B., Soares, A. M., Moore, S. R., Pinkerton, R., Gansneder, B., Bovbjerg, V. E., Guyatt, H., Lima, A. M., and Guerrant, R. L. (2006). Early childhood diarrhea predicts impaired school performance. Pediatr. Infect. Dis. J. 25, 513–520.
Macfarlane, G. T., Cummings, J. H., Macfarlane, S., and Gibson, G. R. (1989). Influence of retention time on degradation of pancreatic enzymes by human colonic bacteria grown in a 3-stage continuous culture system. J. Appl. Bacteriol. 67, 520–527.
Mah, K. W., Chin, V. I., Wong, W. S., Lay, C., Tannock, G. W., Shek, L. P., Aw, M. M., Chua, K. Y., Wong, H. B., Panchalingham, A., and Lee, B. W. (2007). Effect of a milk formula containing probiotics on the fecal microbiota of asian infants at risk of atopic diseases. Pediatr. Res. 62, 674–679.
Mandar, R., and Mikelsaar, M. (1996). Transmission of mother's microflora to the newborn at birth. Biol. Neonate 69, 30–35.
Marchiando, A. M., Graham, W. V., and Turner, J. R. (2010). Epithelial barriers in homeostasis and disease. Annu. Rev. Pathol. 5, 119–144.
Mariat, D., Firmesse, O., Levenez, F., Guimaraes, V., Sokol, H., Dore, J., Corthier, G., and Furet, J. P. (2009). The Firmicutes/Bacteroidetes ratio of the human microbiota changes with age. BMC Microbiol. 9, 123.
Martinez-Medina, M., Aldeguer, X., Gonzalez-Huix, F., Acero, D., and Garcia-Gil, L. J. (2006). Abnormal microbiota composition in the ileocolonic mucosa of Crohn's disease patients as revealed by polymerase chain reaction-denaturing gradient gel electrophoresis. Inflamm. Bowel Dis. 12, 1136–1145.
Matera, M. G., Sancarlo, D., Panza, F., Gravina, C., D'Onofrio, G., Frisardi, V., Longo, G., D'Ambrosio, L. P., Addante, F., Copetti, M., Solfrizzi, V., Seripa, D., and Pilotto, A. (2010). Apolipoprotein E-related all-cause mortality in hospitalized elderly patients. Age (Dordr.) 32, 411–420.
McFarland, L. V., Mulligan, M. E., Kwok, R. Y., and Stamm, W. E. (1989). Nosocomial acquisition of Clostridium difficile infection. N. Engl. J. Med. 320, 204–210.
McGee, D. W., and McMurray, D. N. (1988). The effect of protein malnutrition on the IgA immune response in mice. Immunology 63, 25–29.
Mitsuoka, T., Hayakawa, K., and Kimura, N. (1974). [The faecal flora of man. II. The composition of bifidobacterium flora of different age groups (author's transl)]. Zentralbl. Bakteriol. Orig. A 226, 469–478.
Mondal, D., Minak, J., Alam, M., Liu, Y., Dai, J., Korpe, P., Liu, L., Haque, R., and Petri, W. A. Jr. (2012). Contribution of enteric infection, altered intestinal barrier function, and maternal malnutrition to infant malnutrition in Bangladesh. Clin. Infect. Dis. 54, 185–192.
Monira, S., Nakamura, S., Gotoh, K., Izutsu, K., Watanabe, H., Alam, N. H., Endtz, H. P., Cravioto, A., Ali, S. I., Nakaya, T., Horii, T., Iida, T., and Alam, M. (2011). Gut microbiota of healthy and malnourished children in bangladesh. Front. Microbiol. 2:228. doi: 10.3389/fmicb.2011.00228
Monk, J. M., and Woodward, B. (2009). Elevated blood interleukin-10 levels and undiminished systemic interleukin-10 production rate prevail throughout acute protein-energy malnutrition in the weanling mouse. Cytokine 47, 126–131.
Moore, S. R., Lima, N. L., Soares, A. M., Oria, R. B., Pinkerton, R. C., Barrett, L. J., Guerrant, R. L., and Lima, A. A. (2010). Prolonged episodes of acute diarrhea reduce growth and increase risk of persistent diarrhea in children. Gastroenterology 139, 1156–1164.
Mueller, S., Saunier, K., Hanisch, C., Norin, E., Alm, L., Midtvedt, T., Cresci, A., Silvi, S., Orpianesi, C., Verdenelli, M. C., Clavel, T., Koebnick, C., Zunft, H. J., Dore, J., and Blaut, M. (2006). Differences in fecal microbiota in different European study populations in relation to age, gender, and country: a cross-sectional study. Appl. Environ. Microbiol. 72, 1027–1033.
Muscaritoli, M., Fanfarillo, F., Luzi, G., Sirianni, M. C., Iebba, F., Laviano, A., Russo, M., Aiuti, F., and Rossi, F. F. (2001). Impaired nutritional status in common variable immunodeficiency patients correlates with reduced levels of serum IgA and of circulating CD4+ T lymphocytes. Eur. J. Clin. Invest. 31, 544–549.
O'Toole, P. W., and Claesson, M. J. (2010). Gut microbiota: changes throughout the lifespan from infancy to elderly. Int. Dairy J. 20, 281–291.
Oberhelman, R. A., Kopecko, D. J., Salazar-Lindo, E., Gotuzzo, E., Buysse, J. M., Venkatesan, M. M., Yi, A., Fernandez-Prada, C., Guzman, M., and Leon-Barua, R. (1991). Prospective study of systemic and mucosal immune responses in dysenteric patients to specific Shigella invasion plasmid antigens and lipopolysaccharides. Infect. Immun. 59, 2341–2350.
Ochoa, T. J., Ecker, L., Barletta, F., Mispireta, M. L., Gil, A. I., Contreras, C., Molina, M., Amemiya, I., Verastegui, H., Hall, E. R., Cleary, T. G., and Lanata, C. F. (2009). Age-related susceptibility to infection with diarrheagenic Escherichia coli among infants from Periurban areas in Lima, Peru. Clin. Infect. Dis. 49, 1694–1702.
Opintan, J. A., Newman, M. J., Ayeh-Kumi, P. F., Affrim, R., Gepi-Attee, R., Sevilleja, J. E., Roche, J. K., Nataro, J. P., Warren, C. A., and Guerrant, R. L. (2010). Pediatric diarrhea in southern Ghana: etiology and association with intestinal inflammation and malnutrition. Am. J. Trop. Med. Hyg. 83, 936–943.
Oria, R. B., Patrick, P. D., Zhang, H., Lorntz, B., de Castro Costa, C. M., Brito, G. A., Barrett, L. J., Lima, A. A., and Guerrant, R. L. (2005). APOE4 protects the cognitive development in children with heavy diarrhea burdens in Northeast Brazil. Pediatr. Res. 57, 310–316.
Orsitto, G., Fulvio, F., Tria, D., Turi, V., Venezia, A., and Manca, C. (2009). Nutritional status in hospitalized elderly patients with mild cognitive impairment. Clin. Nutr. 28, 100–102.
Ouwehand, A. C., Isolauri, E., He, F., Hashimoto, H., Benno, Y., and Salminen, S. (2001). Differences in Bifidobacterium flora composition in allergic and healthy infants. J. Allergy Clin. Immunol. 108, 144–145.
Palmer, C., Bik, E. M., DiGiulio, D. B., Relman, D. A., and Brown, P. O. (2007). Development of the human infant intestinal microbiota. PLoS Biol. 5:e177. doi: 10.1371/journal.pbio.0050177
Penders, J., Thijs, C., Vink, C., Stelma, F. F., Snijders, B., Kummeling, I., van den Brandt, P. A., and Stobberingh, E. E. (2006). Factors influencing the composition of the intestinal microbiota in early infancy. Pediatrics 118, 511–521.
Putignani, L., and Menichella, D. (2010). Global distribution, public health and clinical impact of the protozoan pathogen cryptosporidium. Interdiscip. Perspect. Infect. Dis. 2010, 1–39.
Qadri, F., Svennerholm, A. M., Faruque, A. S., and Sack, R. B. (2005). Enterotoxigenic Escherichia coli in developing countries: epidemiology, microbiology, clinical features, treatment, and prevention. Clin. Microbiol. Rev. 18, 465–483.
Rodriguez, L., Gonzalez, C., Flores, L., Jimenez-Zamudio, L., Graniel, J., and Ortiz, R. (2005). Assessment by flow cytometry of cytokine production in malnourished children. Clin. Diagn. Lab. Immunol. 12, 502–507.
Rolfe, R. D. (1997). “Colonization resistance,” in Gastrointestinal Microbiology, eds R. I. Mackie, B. A. White, and R. E. Isaacson (London: Chapman and Hall), 501–536.
Rousseau, C., Levenez, F., Fouqueray, C., Dore, J., Collignon, A., and Lepage, P. (2011). Clostridium difficile colonization in early infancy is accompanied by changes in intestinal microbiota composition. J. Clin. Microbiol. 49, 858–865.
Salminen, S., and Isolauri, E. (2006). Intestinal colonization, microbiota and probiotics. J. Pediatr. 149, S115–S120.
Sartor, R. B. (2004). Therapeutic manipulation of the enteric microflora in inflammatory bowel diseases: antibiotics, probiotics, and prebiotics. Gastroenterology 126, 1620–1633.
Scanlan, P. D., Shanahan, F., O'Mahony, C., and Marchesi, J. R. (2006). Culture-independent analyses of temporal variation of the dominant fecal microbiota and targeted bacterial subgroups in Crohn's disease. J. Clin. Microbiol. 44, 3980–3988.
Sellon, R. K., Tonkonogy, S., Schultz, M., Dieleman, L. A., Grenther, W., Balish, E., Rennick, D. M., and Sartor, R. B. (1998). Resident enteric bacteria are necessary for development of spontaneous colitis and immune system activation in interleukin-10-deficient mice. Infect. Immun. 66, 5224–5231.
Sepp, E., Julge, K., Vasar, M., Naaber, P., Bjorksten, B., and Mikelsaar, M. (1997). Intestinal microflora of Estonian and Swedish infants. Acta Paediatr. 86, 956–961.
Shen, B., Achkar, J. P., Lashner, B. A., Ormsby, A. H., Remzi, F. H., Brzezinski, A., Bevins, C. L., Bambrick, M. L., Seidner, D. L., and Fazio, V. W. (2001). A randomized clinical trial of ciprofloxacin and metronidazole to treat acute pouchitis. Inflamm. Bowel Dis. 7, 301–305.
Shulzhenko, N., Morgun, A., Hsiao, W., Battle, M., Yao, M., Gavrilova, O., Orandle, M., Mayer, L., Macpherson, A. J., McCoy, K. D., Fraser-Liggett, C., and Matzinger, P. (2011). Crosstalk between B lymphocytes, microbiota and the intestinal epithelium governs immunity versus metabolism in the gut. Nat. Med. 17, 1585–1593.
Snedeker, K. G., Shaw, D. J., Locking, M. E., and Prescott, R. J. (2009). Primary and secondary cases in Escherichia coli O157 outbreaks: a statistical analysis. BMC Infect. Dis. 9, 144.
Sokol, H., Lay, C., Seksik, P., and Tannock, G. W. (2008). Analysis of bacterial bowel communities of IBD patients: what has it revealed? Inflamm. Bowel Dis. 14, 858–867.
Soofi, S. B., Habib, M. A., von, S. L., Khan, M. J., Muhammad, S., Bhutto, N., Khan, M. I., Rasool, S., Zafar, A., Clemens, J. D., Nizami, Q., and Bhutta, Z. A. (2011). A comparison of disease caused by Shigella and Campylobacter species: 24 months community based surveillance in 4 slums of Karachi, Pakistan. J. Infect. Public Health 4, 12–21.
Stark, P. L., Lee, A., and Parsonage, B. D. (1982). Colonization of the large bowel by Clostridium difficile in healthy infants: quantitative study. Infect. Immun. 35, 895–899.
Stecher, B., and Hardt, W. D. (2008). The role of microbiota in infectious disease. Trends Microbiol. 16, 107–114.
Strittmatter, W. J., Saunders, A. M., Schmechel, D., Pericak-Vance, M., Enghild, J., Salvesen, G. S., and Roses, A. D. (1993). Apolipoprotein E: high-avidity binding to beta-amyloid and increased frequency of type 4 allele in late-onset familial Alzheimer disease. Proc. Natl. Acad. Sci. U.S.A. 90, 1977–1981.
Su, L., Shen, L., Clayburgh, D. R., Nalle, S. C., Sullivan, E. A., Meddings, J. B., Abraham, C., and Turner, J. R. (2009). Targeted epithelial tight junction dysfunction causes immune activation and contributes to development of experimental colitis. Gastroenterology 136, 551–563.
Tannock, G. W. (2007). What immunologists should know about bacterial communities of the human bowel. Semin. Immunol. 19, 94–105.
Tannock, G. W. (2008). The search for disease-associated compositional shifts in bowel bacterial communities of humans. Trends Microbiol. 16, 488–495.
Tap, J., Mondot, S., Levenez, F., Pelletier, E., Caron, C., Furet, J. P., Ugarte, E., Munoz-Tamayo, R., Paslier, D. L., Nalin, R., Dore, J., and Leclerc, M. (2009). Towards the human intestinal microbiota phylogenetic core. Environ. Microbiol. 11, 2574–2584.
Trevejo, R. T., Courtney, J. G., Starr, M., and Vugia, D. J. (2003). Epidemiology of salmonellosis in California, 1990-1999, morbidity, mortality, and hospitalization costs. Am. J. Epidemiol. 157, 48–57.
Turnbaugh, P. J., and Gordon, J. I. (2009). The core gut microbiome, energy balance and obesity. J. Physiol. 587, 4153–4158.
van der Waaij, D., Berghuis-de Vries, J. M., and Lekkerkerk, L. (1971). Colonization resistance of the digestive tract in conventional and antibiotic-treated mice. J. Hyg. (Lond.) 69, 405–411.
Vanhoutte, T., Huys, G., Brandt, E., and Swings, J. (2004). Temporal stability analysis of the microbiota in human feces by denaturing gradient gel electrophoresis using universal and group-specific 16S rRNA gene primers. FEMS Microbiol. Ecol. 48, 437–446.
Victora, C. G., Adair, L., Fall, C., Hallal, P. C., Martorell, R., Richter, L., and Sachdev, H. S. (2008). Maternal and child undernutrition: consequences for adult health and human capital. Lancet 371, 340–357.
Victora, C. G., de, O. M., Hallal, P. C., Blossner, M., and Shrimpton, R. (2010). Worldwide timing of growth faltering: revisiting implications for interventions. Pediatrics 125, e473–e480.
Vitek, M. P., Brown, C. M., and Colton, C. A. (2009). APOE genotype-specific differences in the innate immune response. Neurobiol. Aging 30, 1350–1360.
WHO regions. (2012). Available online at: http://www.who.int/choice/demography/regions/en/
Wu, G. D., Chen, J., Hoffmann, C., Bittinger, K., Chen, Y. Y., Keilbaugh, S. A., Bewtra, M., Knights, D., Walters, W. A., Knight, R., Sinha, R., Gilroy, E., Gupta, K., Baldassano, R., Nessel, L., Li, H., Bushman, F. D., and Lewis, J. D. (2011). Linking long-term dietary patterns with gut microbial enterotypes. Science 334, 105–108.
Yang, P. C., Jury, J., Soderholm, J. D., Sherman, P. M., McKay, D. M., and Perdue, M. H. (2006). Chronic psychological stress in rats induces intestinal sensitization to luminal antigens. Am. J. Pathol. 168, 104–114.
Zareie, M., Johnson-Henry, K., Jury, J., Yang, P. C., Ngan, B. Y., McKay, D. M., Soderholm, J. D., Perdue, M. H., and Sherman, P. M. (2006). Probiotics prevent bacterial translocation and improve intestinal barrier function in rats following chronic psychological stress. Gut 55, 1553–1560.
Zilberberg, M. D., Shorr, A. F., and Kollef, M. H. (2008). Increase in adult Clostridium difficile-related hospitalizations and case-fatality rate, United States, 2000–2005. Emerg. Infect. Dis. 14, 929–931.
Keywords: enteric pathogen, intestinal microbiota, malnutrition, diarrhea, age distribution
Citation: Kolling G, Wu M and Guerrant RL (2012) Enteric pathogens through life stages. Front. Cell. Inf. Microbio. 2:114. doi: 10.3389/fcimb.2012.00114
Received: 11 April 2012; Accepted: 08 August 2012;
Published online: 25 August 2012.
Edited by:
Lorenza Putignani, Children's Hospital and Research Institute Bambino Gesù, ItalyReviewed by:
Robert Maier, University of Georgia, USASimon Daefler, Mount Sinai School of Medicine, USA
Copyright © 2012 Kolling, Wu and Guerrant. This is an open-access article distributed under the terms of the Creative Commons Attribution License, which permits use, distribution and reproduction in other forums, provided the original authors and source are credited and subject to any copyright notices concerning any third-party graphics etc.
*Correspondence: Richard L. Guerrant, Department of Internal Medicine, Division of Infectious Diseases and International Health, Center for Global Health, University of Virginia, Charlottesville 22908, VA, USA. e-mail:cmxnOWFAdmlyZ2luaWEuZWR1