- 1Department of Pharmacy, University of Salerno, Fisciano, Italy
- 2PhD Program in Drug Discovery and Development, University of Salerno, Fisciano, Italy
TFF1, a mucin-associated secreted peptide of gastric mucous cells, is known as a protective agent for stomach epithelium under different stimuli, but its role upon Helicobacter infection is still not clear. In this paper we characterized TFFs expression, with particular attention to TFF1, under Helicobacter infection in gastric cell lines. A mouse model was used to distinguish TFF1 mRNA expression between acute and chronic stages of Helicobacter infection. Our results show that TFF1 expression is induced in infected cells; in addition, the inflammatory response upon Helicobacter infection is inversely associated to pre-existing TFF1 protein levels. In infected mice, TFF1 is initially upregulated in gastric antrum in the acute phase of infection, along with IL-1β and IL-6. Then, expression of TFF1 is gradually silenced when the infection becomes chronic and IFN-γ, CXCL5, and CXCL15 reach higher levels. Our data suggest that TFF1 might help cells to counteract bacteria colonization and the development of a chronic inflammation.
Introduction
Helicobacter pylori is a spiral-shaped, Gram-negative bacterium which colonizes the human gastric mucosa of 50–75% of population all over the world (Calvet et al., 2013). H. pylori infection can cause chronic gastritis in most infected individuals and more severe pathologies in a subset of them, including neoplasia, B-cell lymphoma of mucosal-associated lymphoid tissue (MALT lymphoma) and invasive gastric adenocarcinoma (Cover and Blaser, 2009).
Acute infectious symptoms (nausea, halitosis, dyspepsia, and malaise) tend to resolve within 2 weeks. The poor evidence related to acute infection is from old cases of deliberate ingestion (Marshall et al., 1985; Morris and Nicholson, 1987) or some more recent groups of enrolled volunteers (Graham et al., 2004; Nurgalieva et al., 2005). Acute infection is accompanied by severe gastritis, characterized by infiltration of neutrophils and inflammatory cells. Within the first 14 days of infection a reduction in stomach acid secretion is described (Sobala et al., 1991), together with polymorphonuclear cells infiltration and interleukin 8 (IL-8) induction in gastric biopsies (Graham et al., 2004).
On the other hand, a persistent infection brings to a state termed “chronic superficial gastritis” (Warren, 2000), characterized by different types of tissue-infiltrated leukocytes (lymphocytes, macrophages, neutrophils, mast cells, and dendritic cells). Gastritis severity increases with the level of inflammation, but the pattern of inflammation determines the disease outcome. Host genetic factors, bacterial virulence, environmental factors, and age of infection all together influence the distribution of resulting gastritis (Robinson et al., 2007).
H. pylori infection has been associated with increased risk of developing gastric cancer (GC) together with genetic basis, environmental and nutritional factors. GC is the third most common cause of cancer-related death in the world (Ferro et al., 2015).
Gastric adenocarcinomas are classified according to prognosis into two main groups: early and advanced. Histologically, the most frequently used system is the Lauren classification, which recognizes two main subtypes: intestinal and diffuse (Lauren, 1965). The intestinal subtype is the most frequently diagnosed in older individuals, males more than females, strongly associated with H. pylori infection and it is characterized by malignant epithelial cells that show cohesiveness and glandular differentiation infiltrating the stroma; while the diffuse subtype is more aggressive, generally diagnosed in younger patients (Piazuelo and Correa, 2013).
Even if H. pylori association with intestinal metaplasia is, at this point, assessed, initial molecular gastric perturbation that could take into account subsequent malignant transformation have not been elucidated.
Recently, Trefoil Factor1 (TFF1) role has been studied during H. pylori infection. TFF1 is a mucin-associated secreted peptide that, together with the other two members of the Trefoil Factor Family (TFF2 and TFF3), participates in gastrointestinal mucosa repair (Kjellev, 2009). TFF1 is primarily secreted by the normal stomach mucosa and it is widely considered a gastric specific tumor suppressor (Xiao et al., 2015). Indeed, more than 50% of gastric cancers show a reduced TFF1 expression due to some point mutations (Park et al., 2000), promoter polymorphic variants (Moghanibashi et al., 2012), but mostly to loss of heterozygosity (LOH) and hypermethylation of its promoter (Carvalho et al., 2002).
Trefoil factor family proteins are differently expressed along the gastrointestinal tract; at the stomach level, TFF1 is mainly expressed in the fundus and antrum of gastric mucous cells; TFF2 in the mucous neck cells of fundic glands and basal cells of antral and pyloric glands, as well as duodenum (Brunner's gland) (Aihara et al., 2017); while TFF3 is undetectable in normal gastric mucosa, but strongly expressed in goblet cells of intestinal metaplasia (Im et al., 2013). Looking at the phenotype of genetically modified animals, TFF1 knock-out mice displayed hyperplastic gastric epithelium and 30% of them developed multifocal intraepithelial or intramucosal carcinomas (Lefebvre et al., 1996), while mice lacking TFF2 showed only a minimal phenotype with a slight reduction in proliferation rates in gastric mucosa (Farrell et al., 2002). Nonetheless, H. pylori infected TFF2-deficient mice developed more advanced premalignant lesions of atrophy, metaplasia and dysplasia than wild-type (Peterson et al., 2010). In addition, TFF3 knock-out mice showed impaired intestinal epithelial regeneration after injury (Mashimo et al., 1996). These observations, together with clinical data, led to consider TFF1 as a specific gastric tumor suppressor, although recent studies clarified that its expression is reduced only in the intestinal-type gastric cancers (Im et al., 2013), contrary to patients with diffuse and undifferentiated cancer type characterized by high TFF1 expression (Ren et al., 2006). The other two Trefoil factors are not considered tumor suppressors, even if lack of TFF2 seems to be predisposal to gastric malignancies and TFF3 is strongly up-regulated in intestinal metaplasia and therefore considered a marker of poor prognosis (Im et al., 2013).
The few data on TFF1 expression in biopsy specimens from H. pylori positive compared to negative patients are controversial: Kato and coworkers report no significant change in TFF1 expression (Kato et al., 2004); while other evidence show TFF1 reduction in H. pylori positive patients compared to the uninfected, both at the mRNA (Tomita et al., 2011) and protein levels (Van De Bovenkamp et al., 2005). On the other hand, cellular studies report an increase of TFF1 mRNA expression (Matsuda et al., 2008).
Recently, it has been shown that reconstitution of TFF1 expression in GC cells reduces H. pylori-induced inflammation (Soutto et al., 2015a) and H. pylori-induced activation of oncogenic β–catenin (Soutto et al., 2015b), suggesting a protective role of the trefoil peptide in H. pylori-induced chronic inflammation and carcinogenesis. Nonetheless, data on TFF1 role in prodromal phases of infection prior its chronic conversion are missing. More robust evidence are needed for the other two Trefoil Factors (TFF2 and TFF3) as well, also because a better picture of their expression levels could represent a specific hallmark for gastrointestinal pathologies and likely have prognostic value.
In this paper we characterized TFFs expression, with particular attention to TFF1, under Helicobacter infection in gastric cell models and in mice antrum. Our results demonstrate that TFF1 expression level in infected cells is inversely associated to the degree of inflammation. Interestingly, in infected mice, TFF1 is upregulated in the early phase of infection, while it is gradually silenced right after the acute injury phase.
Materials and Methods
Cell Cultures
The cell lines used in these experiments were: AGS (gastric adenocarcinoma, intestinal type, highly differentiated), KATO III (gastric carcinoma, derived from metastatic site, poorly differentiated), AGS-AC1, a TFF1 inducible hyperexpressing clone described previously (Tosco et al., 2010).
AGS were cultured in Ham's F-12 (Nutrient Mixture F-12 Ham, Euroclone), KATO III in RPMI 1640 (Euroclone), AGS-AC1 clone in DMEM (Euroclone), all media were supplemented with 10% (v/v) fetal bovine serum (FBS, Euroclone), 100 U/ml penicillin and 100 μg/ml streptomycin (Euroclone). Medium for AGS-AC1 was supplemented with 600 μg/ml G-418 (Sigma) and TFF1 expression was induced with 1 μg/ml of doxycycline.
All cell lines were maintained at 37°C in a 5% CO2 atmosphere.
Bacterial Cultures
H. pylori P12 strain, obtained from a German patient with a duodenal ulcer (Schmitt and Haas, 1994) kindly provided by Dr. Marguerite Clyne (University College Dublin) and Helicobacter felis strain ATCC 49179, were cultured on selective Columbia agar (Oxoid) containing 7% (v/v) defibrinated horse blood (Oxoid) and an antibiotic mix (DENT or Skirrow supplement respectively, Oxoid). Bacteria plates were incubated for 3–4 days in a capnophilic atmosphere (10% CO2) as suggested by (Park et al., 2011). For infection experiments, bacteria were scraped from confluent plates using brain heart infusion broth (BHI Difco) supplemented with 7% FBS (v/v). Cultures were assessed for motility and the bacterial optical density at 600 nm (OD600) was measured considering: 1 OD600 = 1 × 108 bacteria/ml.
Helicobacter pylori Colonization Experiments
For each cell line, optimal colonization conditions were determined using different MOIs (multiplicity of infection) and times of infection. Briefly, AGS and KATO III were seeded at 80% of confluence, after 24 h cells were washed three times with PBS to remove medium containing penicillin and streptomycin, and then infected with different concentrations of bacteria and for different times at 10% CO2. AGS-AC1 clone was seeded at 60% of confluence, after 24 h TFF1 expression was induced with doxycycline and, after 48 h of induction, cells were infected in medium without antibiotics and incubated at 10% CO2. For AGS and KATO III MOI 1:30 and 36 h of incubation were selected as optimal infection conditions, while for AGS-AC1 clone MOI 1:150 and 36 h of infection were selected for all further experiments.
Cultures were washed different times with PBS to remove non-adherent bacteria and then harvested with Trypsin 0.05%-EDTA 0.02% and used for RNA, DNA or protein extraction. For each condition, experiments were performed at least in triplicate.
Real Time PCR Analysis
Total RNA was extracted using TRI Reagent (Sigma), 2 μg of total RNA was reverse transcribed into cDNA with M-MLV Reverse Transcriptase (Gene Spin S.r.l) and Real-Time PCR was performed using the Light Cycler 480 II instrument (Roche). Suitable dilutions of cDNA were used for each gene in a 12 μl reaction using Light Cycler 480 Probes Master and Real Time Ready Catalog Assay primers (Roche) for human TFF1 (Conf. number 100027378), human TFF3 (Conf. number 100064753) and human HPRT1 (Conf. number 100027387) following the manufacturer instruction protocol. While for all the other human (IL-8; IL-6; TFF2) and mouse (TFF1; TFF2; TFF3; IL-6; IFN-γ; IL-1β; CXCL-15; CXCL-5; HPRT1) genes, Light Cycler 480 SYBR Green I Master was used, human primers are listed in Supplementary Table 1 and mouse primers in Supplementary Table 2. Results were analyzed using the Delta-Delta CT method and HPRT1 as reference gene.
Western Blot Analysis
The supernatants were clarified by centrifugation at 10,000 g for 10 min at 4°C. To obtain intracellular proteins, cell pellets were incubated in ice-cold lysis buffer (0.1% NP-40 in PBS 1X) for 30 min, sonicated and then centrifuged at 10,000 g for 10 min to remove cellular debris. Five micrograms of intracellular proteins and 20 μl of medium containing extracellular proteins were diluted with Laemmli buffer and subjected to western blot analysis using a polyclonal anti-TFF1 antibody (Tosco et al., 2010) and an anti-tubulin or anti-β actin antibody (Santa Cruz). Chemiluminescent signals of positive bands were detected by ImageQuant LAS 4000 (GE Healthcare, Waukesha, WI, USA) digital imaging system and quantified by ImageQuant TL software.
Ethics Statement
All animal experiments were approved by the Italian Ministero della Salute (Aut. n° 714/2015-PR) and performed according to institutional animal care guidelines (Italian Law 26/2014 based on the European Community Law for Animal Care 2010/63/UE).
Mice Infection
All studies were performed on female C57BL/6 mice (6 weeks old) obtained from Charles River Laboratories International, and maintained in a pathogen-free room. Mice were maintained in groups of five in microisolator cages with ad libitum access to food and water and were fasted overnight prior to infection and samples collection. Animals were infected by oral gavage with H. felis (107 total bacteria per mouse in 100 μl) three times every other day. Control mice received 100 μl of medium. Mice were then maintained on fasting for 5 h after each inoculation. Control group (10 mice) and infected groups were sacrificed by cervical dislocation at 1, 3, 5, 10, 14, and 42 days post-infection (6 mice for day 14 and 8 mice for all the other groups). The stomach was removed and washed with PBS. For molecular analyses antrum was divided in three parts: one was immediately stored in RNA isolation reagent (TRI Reagent, Sigma), frozen in dry ice and stored at −80°C for Real Time experiments; the other two pieces were frozen in dry ice and used for DNA and protein analyses. For histologic analyses, stomachs were cut along the greater curvature, washed with PBS and laid flat on a cutting board. After fixing in a solution of 0.5% PFA, 0.5% Triton and 0.02% NaN3 for 2 h, tissues were incubated in 15% sucrose overnight at 4°C.
Histologic Analysis
After sucrose treatment, tissues were longitudinally dissected into three strips so that each fragment contained gastric cardia, body and antrum. The strips were embedded in OCT (Optimal Cutting Temperature) compound in cryomolds, placed on side to insure representation of all layers from mucosa through serosa in the final stained specimen, sectioned (5-μm slices) and stained with Hematoxylin and Eosin (H&E). The histopathological features of tissues were assessed by light microscopy (Axioplan 2 Imaging Universal Microscope with an AxioCam camera, Zeiss). Histologic scoring criteria of mouse gastritis were assigned according to Rogers (2012).
Immunofluorescence Analysis
Immunofluorescence analyses were performed on stomach tissue sections for the detection of F4/80 (rat anti-F4/80 PE conjugate 1:100; EBioscience cod. 12-4801-82), GR-1 (rat anti-GR-1 1:100, EBioscience cod. 14-5931-85) and TFF1 (rabbit anti-TFF1 1:500, LSBio cod. LS-C155659) proteins. Sections were fixed for 20 min in 4% paraformaldehyde, permeabilized with 0.5% Triton in PBS for 5 min then blocked with 20% goat serum in PBS + 0.5% Triton for 30 min followed by labeling with primary antibody overnight at 4°C. For GR-1 and TFF1 detection, sections were then washed and incubated, respectively, with an Alexa Fluor 488 goat anti-rat and an Alexa Fluor 594 goat anti-rabbit secondary antibody (1:5000) for 2 h at RT protected from the light. Visualization of the nuclei was achieved by Hoechst staining (1:5000, Invitrogen). Sections were mounted with 40% glycerol in PBS. Images were collected using an LSM 510 Laser-scanning Confocal Microscope (Zeiss).
Statistical Analysis
The results are expressed as means ± SEM or ± SD as appropriate. All statistical differences between two groups of data were evaluated by Student's t-test. Statistical analyses and graphing were done using PRISM4 software (GraphPad Software, La Jolla, CA, USA). A p < 0.05 was considered statistically significant.
Results
H. pylori Infection Induces TFF1 Expression in Gastric Cancer Cells
To better understand the role of TFF1 during H. pylori infection, we analyzed two cancer cell lines with significant difference in TFF1 basal levels: AGS, a gastric adenocarcinoma cell line, highly differentiated, intestinal type, which shows very low expression of TFF1, almost undetectable at protein level; KATO III, a signet ring cell carcinoma from a metastatic site, poorly differentiated, with higher expression of the protein (Supplementary Figure 1).
Firstly, we optimized the infection conditions by using different MOIs and different time points for bacterial incubation. Based on IL-8 induction, a cytokine up-regulated upon H. pylori infection (Lee et al., 2013), and a microscopic observation of cellular health, we selected the MOI 1:30 and 36 h of incubation as IL-8 was significantly increased and cellular shape was preserved; conversely, at higher MOIs and prolonged incubation time, both cellular lines showed suffering signals (Supplementary Figure 2).
As shown in Figures 1A–C, IL-8 is more induced in AGS (ca. 40-fold) than in KATO III (ca. 15-fold) cells after H. pylori infection, suggesting that the basal higher level of TFF1 in KATO III might protect cells from the inflammatory process. On the other hand, TFF1 mRNA induction caused by bacterial infection is higher in AGS (ca. 150-fold) (Figure 1D) compared to KATO III cells (ca. 10-fold) (Figure 1B). As a consequence, in infected AGS cells TFF1 protein becomes detectable in either intracellular lysates or in supernatant media as secreted form (Figures 1E,F). These data suggest that cells respond to bacterial insult by producing TFF1 protein, likely to counteract the infection.
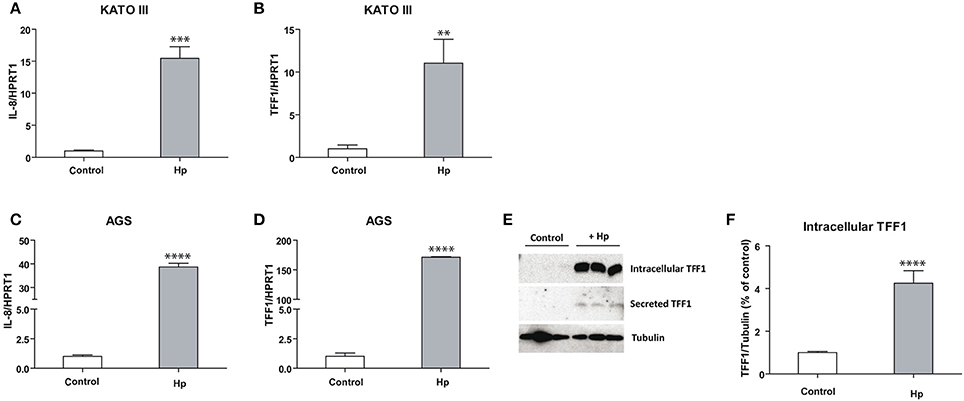
Figure 1. TFF1 and IL-8 transcriptional regulation upon H. pylori infection in KATO III and AGS cells. Real Time PCR analysis of IL-8 (A–C) and TFF1 (B–D) in KATO III and AGS gastric cancer cells after 36 h of H. pylori infection. (E) Western blot analysis of intracellular and secreted TFF1 protein in AGS cell line. (F) Densitometric analysis of intracellular protein signals. Experiments were carried out at least in triplicate and data are expressed as mean ± SD (t-test, **p ≤ 0.01, ***p ≤ 0.001, ****p ≤ 0.0001).
Moreover, the analysis of expression level of the other two members of the Trefoil Factor Family, TFF2 and TFF3, produced different results in the two cellular lines. TFF2 and TFF3 are both induced in infected KATO III cells (Figures 2C,D), while in infected AGS cells TFF2 is slightly induced and TFF3 is strongly reduced (Figures 2A,B).
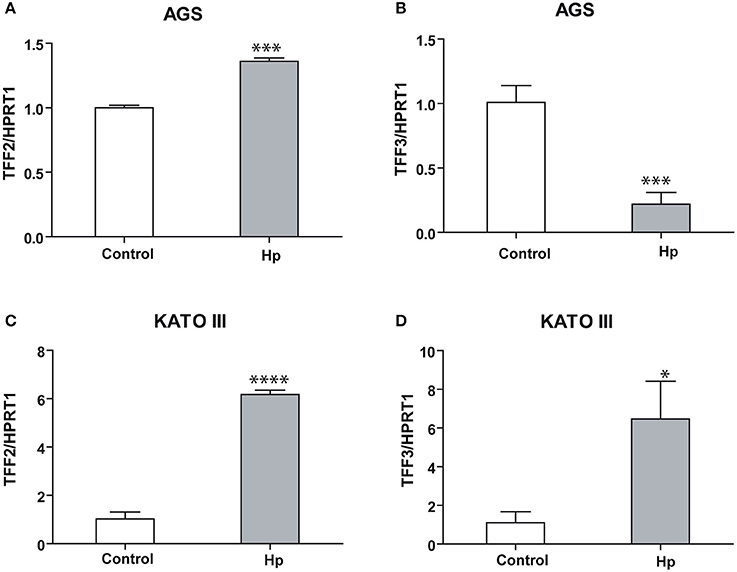
Figure 2. TFF2 and TFF3 transcriptional regulation upon H. pylori infection in KATO III and AGS cells. Real-time PCR analysis of TFF2 and TFF3 mRNA after 36 h of H. pylori infection in AGS (A,B) and KATO III cells (C,D). Experiments were carried out at least in triplicate and data are expressed as mean ± SD. (t-test, *p ≤ 0.05; ***p ≤ 0.001, ****p ≤ 0.0001).
Taken together, these data suggest that TFF1 is induced upon Helicobacter infection in both cellular systems, while TFF2 and TFF3 responses change depending on the host system.
TFF1 Protein Influences TFFs Expression after H. pylori Infection
Based on the previous results, we asked whether the different response to H. pylori infection was due to the different basal level of TFF1 in AGS and KATO III cells. To this aim, we decided to use an inducible hyper-expressing clone of TFF1, AGS-AC1, already characterized in our lab (Tosco et al., 2010), which expresses detectable level of TFF1 only under doxycycline control (Supplementary Figure 3).
As described previously, we optimized the conditions of infection, using different MOIs (1:60, 1:150, 1:300) for 36 h of incubation and selected MOI 1:150 which produced optimal IL-8 up-regulation without cell suffering (Supplementary Figure 4).
As expected, bacterial infection caused IL-8 and IL-6 induction in both AGS-AC1 doxycycline- induced and -uninduced cells (Figures 3A,B). However, we observed a lower induction of both cytokines in hyper-expressing TFF1 AGS-AC1 cells compared to uninduced cells (Figures 3A,B).
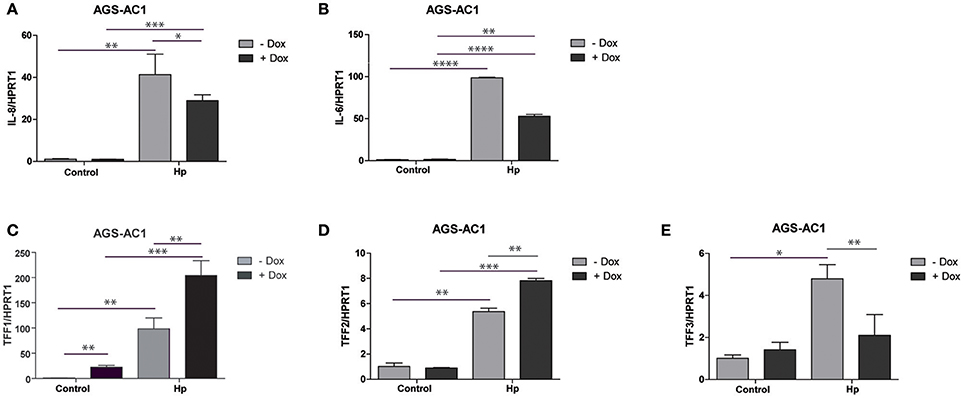
Figure 3. Trefoil factors and cytokines transcriptional regulation upon H. pylori infection in AGS-AC1 clone. Real-time PCR analysis of IL-8 (A), IL-6 (B), TFF1 (C), TFF2 (D) and TFF3 (E) mRNA after 36 h of H. pylori infection in AGS-AC1 cells induced or not with Doxycycline to express TFF1. Experiments were carried out at least in triplicate and data are expressed as mean ± SD (t-test, *p ≤ 0.05; **p ≤ 0.01; ***p ≤ 0.001, ****p ≤ 0.0001).
TFF1 and TFF2 are up-regulated upon infection (Figures 3C,D), in both doxycycline-induced and -uninduced cells, but their levels are significantly higher in TFF1 hyper-expressing cells (dox-induced). On the other hand, TFF3 is up-regulated upon infection only in TFF1 not expressing cells (Figure 3E). The latter data are in contrast with what we observed previously in the aforementioned two different tumor cell lines (Figures 2B,D), likely because other regulatory mechanisms of TFF3 expression might occur in this system.
These results suggest that TFF1 protein is able to influence its own expression as well as TFF2 and TFF3 transcription, in response to outer insults like bacterial infection.
TFF1 Expression is Differently Regulated from Acute to Chronic Helicobacter Infection
In order to verify whether this phenomenon is reproduced in vivo as well, we enrolled a mouse model (C57BL/6 strain) of H. felis infection. This system allows investigating TFF1 expression in either acute or chronic inflammation. Importantly, H. felis represents a better system compared to the adapted H. pylori SS1 strain, because it develops gastric adenocarcinoma through intestinal metaplasia and dysplasia mimicking humans infected with H. pylori (Hayakawa et al., 2013).
Mice were infected as described in methods section and sacrificed at different times, at 24 h after the first injection of the bacterial pathogen up to 6 weeks later (Supplementary Figure 5). The success of infection was assessed by PCR analysis of ureB gene (data not shown). Figure 4A shows that, in gastric antrum of C57BL/6 mice infected with H. felis, TFF1 mRNA is up-regulated at 3, 5, and 8 days post-infection (up to 2-fold compared to naïve), while it is already down-regulated after 14 days (0.3-fold compared to naïve) and remains low up to 6 weeks post-infection (42 days). At this longer time point we were also able to observe reduced TFF1 protein levels (Supplementary Figure 6).
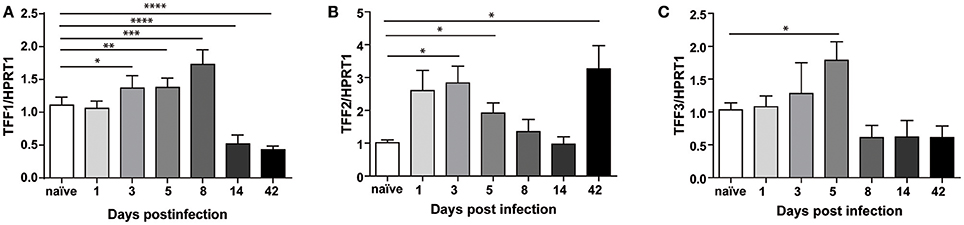
Figure 4. Trefoil Factors transcriptional regulation upon Helicobacter felis infection in C57BL/6 mice. Real Time PCR analysis of TFF1 (A), TFF2 (B), and TFF3 (C) mRNA in gastric antrum of C57BL/6 mice infected with H. felis and sacrificed at the indicated times (d = days; w = weeks) post-infection compared to naïve mice (n: 6–10 for each group). Data are mean ± SEM. (t-test, *p ≤ 0.05, **p ≤ 0.01; ***p ≤ 0.001, ****p ≤ 0.0001).
Regarding the other two members of the family, we measured an up-regulation (up to 2 to 3-fold) at earlier time of infection and different behaviors after 6 weeks when TFF2 keeps increasing (Figure 4B), while TFF3 levels is lower (Figure 4C).
In order to characterize the inflammatory response across the infection, we analyzed the levels of IL-1β, IL-6, IFN-γ, CXCL5, and CXCL15 (Figure 5), reported to be up-regulated upon Helicobacter infection in different studies (Schmitz et al., 2007; Figueiredo et al., 2014).
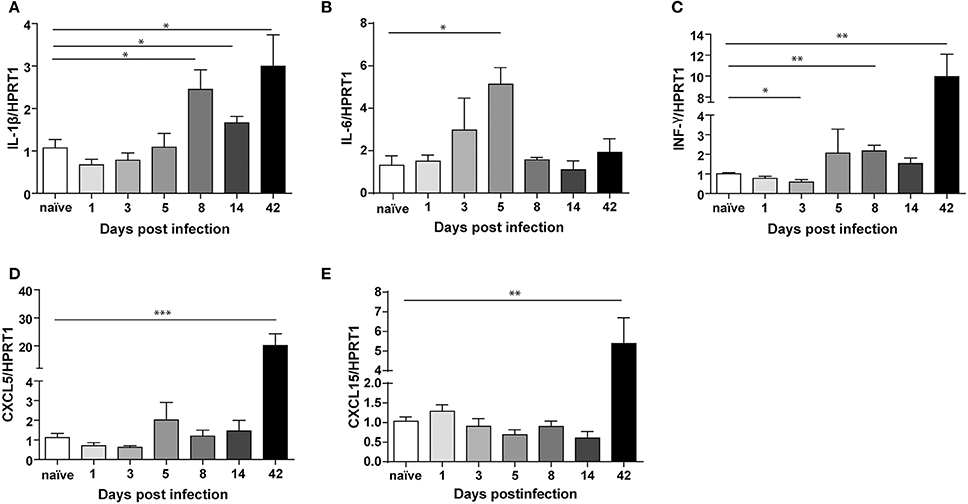
Figure 5. Transcriptional regulation of different cytokines upon Helicobacter felis infection in C57BL/6 mice. Real Time PCR analysis of IL-1β (A), IL-6 (B), INF-γ (C), CXCL5 (D), and CXCL15 (E) mRNA in gastric antrum of C57BL/6 mice infected with H. felis and sacrificed at the indicated times post-infection compared to naïve mice (n: 6–10 for each group). Data are mean ± SEM. (t-test, *p ≤ 0.05; **p ≤ 0.01; ***p ≤ 0.001).
We measured significant levels (2 to 3-fold) of IL-1β (Figure 5A), at 8 days up to 6 weeks post-infection, and increased IL-6 levels (ca. 5-fold) at day 5 post-infection (Figure 5B). These cytokines are among the first ones produced during acute infection in humans (Graham et al., 2004). Moreover, we found a significant up-regulation of IFN-γ already at day 8 post-infection, which reaches higher levels at 6 weeks post-infection (Figure 5B).
On the other hand, CXCL5 (ca. 20-fold) (Figure 5D) and CXCL15 (ca. 5-fold) (Figure 5E) are strongly up-regulated only after 6 weeks, which marks definitely the transition from acute to chronic infection.
Our results demonstrate that TFF1 is up-regulated in the acute phase of infection together with IL-1β and IL-6, while is down-regulated in the chronic phase of infection (after 42 days) when IFN-γ, CXCL5, and CXCL15 increase.
Histologic observation of the entire stomach confirmed what previously measured. Hematoxylin and eosin (H&E) staining revealed essentially no morphologic changes in mice tissues at 5 and 8 days post-infection, yet clear signs of chronic gastritis across the entire tissues after 6 weeks of infection (Figure 6). In particular, mice at 6 weeks post-infection showed extensive infiltration of inflammatory cells at the level of mucosa and submucosa, across the entire tissue from the forestomach to pylorus, whilst samples from mice at 5 and 8 days post-infection showed less cellular infiltration with a specific localization in the corpus submucosa. Figures 6A,D reports representative images of cellular infiltrates in the corpus. Immunofluorescence analyses revealed the presence of F4/80-positive macrophages and Gr-1-positive neutrophils among the cellular infiltrates already at 5 days post-infection.
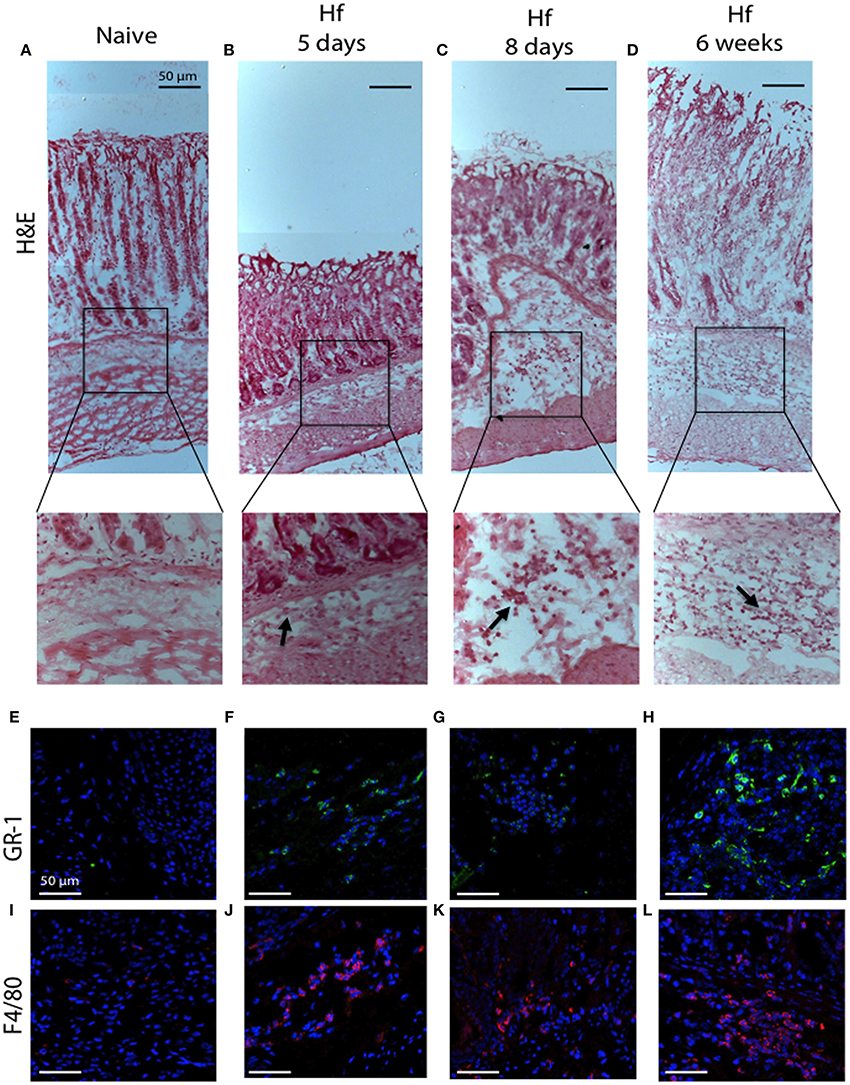
Figure 6. H&E and immunofluorescence analysis of C57BL/6 gastric corpus mucosae representative regions. (A,E,I) naïve mice; (B,F,J) 5 days; (C,G,K) 8 days; (D,H,L) 6 weeks H. felis-infected mice. Staining shows no significant pathological changes in naïve mice. Note the inflammatory cell infiltration in the submucosa (20x magnifications of boxed sections, black arrows) and the positive staining for GR-1 (green) and F4/80 (red) after 5 days and progressively more significant at 6 weeks post-infection. (A–D) 10x; (E–L) 40x. Hoechst for nuclei (blue). Scale bar: 50 μm.
All together, the histological evidence accomplished the “inflammation criterion” and, according to the scoring criteria included in Helicobacter associated mouse gastric histology activity index (HAI) (Rogers, 2012), we assigned a score of 1 to samples at 5 and 8 days post-infection and a score of 2–3 to samples after 6-weeks since mice were infected.
These results corroborate previous observations and confirm that when the infection is acute (up to 8 days) and characterized by few infiltrates, cells respond increasing TFF1 levels. Conversely, during the chronic phase of infection (at 42 days) when cellular infiltrates increase, TFF1 expression is reduced.
Discussion
TFF1 analyses on gastric biopsies from Helicobacter infected patients seem to indicate a reduced expression of the peptide, although data are limited (Van De Bovenkamp et al., 2005; Tomita et al., 2011) and restricted only to chronic infections. Regarding the other two members of the Trefoil Factor Family, gastric TFF2 is described to be silenced by promoter methylation during Helicobacter infection (Peterson et al., 2010), while no evidence is reported for TFF3 on gastric biopsies of Helicobacter infected patients. TFFs expression is always cross-correlated, the level of each of them changes depending on the specific gastrointestinal pathology and they might represent prognostic tools. Therefore, a more complete picture of TFFs expression is needed.
Here, to investigate TFFs expression, with particular attention to TFF1, under Helicobacter infection, we started using different cancer cells with different basal levels of TFF1. These systems were also useful to correlate the inflammatory response to the pre-existing TFF1 level. To better characterize the acute and chronic phases of infection and overcome technical limit due to inadequacy of cancer cell lines (Mustapha et al., 2014), we moved to a mouse model.
We chose three different cellular lines, KATO III, AGS and the inducible clone AGS-AC1: the first one is from a metastatic site, poorly differentiated, with high expression of TFF1 protein; the second one is from a gastric intestinal type, highly differentiated adenocarcinoma, with very low expression of TFF1; the last one is an hyper-expressing inducible clone of AGS. In all these three systems we observed a transcriptional induction of the peptide TFF1 after H. pylori infection, to the extent that TFF1 protein became detectable by Western blot analysis in AGS cells. The choice of the inducible clone was useful to discriminate the cellular response in presence or absence of TFF1 protein in the same cell line. Results from this cellular system showed that when the trefoil peptide is expressed, the pro-inflammatory cytokines (IL-8 and IL-6) induction upon infection is reduced. This result is in agreement with other data (Soutto et al., 2015a) and suggests that basal TFF1 level in the stomach could limit the inflammatory response, while a lower expression could lead to a more severe response.
Moreover, the presence of TFF1 might influence the regulation of TFF2 and TFF3 expression upon infection, since TFF2 turns to be more induced than expected, while TFF3 is not. The regulatory cross-talk among the three Trefoil peptides was already described (Taupin et al., 1999), nevertheless our analysis highlights such crosstalk upon Helicobacter infection. Interestingly, in hyper-expressing clone TFF1 is so strongly induced that its level cannot be explained as the mere sum of dox-induced plus H. pylori-induced mRNA; such result could be justified with an auto-induction mechanism, already described for TFF2 and TFF3 (Bulitta et al., 2002; Sun et al., 2016), and we are currently examining this possibility.
Taken together, our results on cellular systems suggest that during Helicobacter infection the pre-existing TFF1 favors the induction of TFF2 and TFF1 itself, known as gastric protective factors, while limits the up-regulation of TFF3, a negative marker in this district, as well as the cytokine response (IL-8 and IL-6).
However, our cellular data on TFF1 up-regulation upon Helicobacter infection, seem in contrast to in vivo analyses where it is down regulated (Van De Bovenkamp et al., 2005; Tomita et al., 2011). Hence, we based our in vivo experiments on the hypothesis that gastric mucosa, in the acute phase of infection, tries to face bacteria by up-regulating TFF1; when infection persists and becomes chronic, other molecular mechanisms occur and lead to the silencing of TFF1, along with other genes. To our knowledge, only Tomita and coworkers (Tomita et al., 2011) measured TFF1 upon Helicobacter infection in mice, but the analysis was carried out at 18 weeks post-infection, when relevant gastric damages have already happened.
For our in vivo experiments we used C57BL/6 mice infected with Helicobacter felis, since these model showed gastric metaplasia, dysplasia and invasive cancer mimicking H. pylori human infection, thus representing a better system than the H. pylori mouse adapted strain (Hayakawa et al., 2013).
We analyzed TFF1 mRNA in the very early phase of infection at 1, 3, 5, and 8 days post-infection and later at 2 and 6 weeks. We report that, during the acute phase of infection, TFF1 mRNA is significantly up-regulated in the antrum while, starting from 2 weeks post-infection, its level is lowered and at 6 weeks we observe an appreciable reduction of its protein signal. The other two members of the Trefoil Factor Family are both induced up to 5 days post-infection; then, their expression is back to basal levels and only TFF2 is again up-regulated at 6 weeks.
The analysis of mRNA level of different cytokines revealed a different pattern of induction: IL-1β, IL-6, and IFN-γ are up-regulated in the antrum of mice at early time of infection, while CXCL5 and CXCL15 are induced only at 42 days. Infiltrating macrophages and neutrophils start colonization of corpus submucosa at 5 and 8 days post-infection, extending to the entire stomach submucosa at 6 weeks.
Based on these data, we conclude that, in our experimental conditions, when TFF1 is up-regulated (acute phase of infection), there is an innate immune response which ends at 8 days post-infection, while when TFF1 is reduced (at 6 weeks) signs of the chronic phase of infection are visible. Therefore, we postulate that in humans with a compromised TFF1 expression, the inflammatory response might be more severe.
Recently, we have demonstrated that TFF1 promotes H. pylori colonization in cellular models probably through its binding with the rough form of bacterial lipopolysaccharide and that this effect is enhanced by copper (Montefusco et al., 2013). Copper was reported to promote the functional homodimeric form of TFF1 and its binding to form the active cuprocomplex (Tosco et al., 2010; Esposito et al., 2015). In the light of the present work, we postulate that TFF1 presence in the mucous layer, in spite of holding bacteria in the stomach, could block them in a less virulent form, protecting the underlying epithelium from a more severe inflammatory response.
In conclusion, in this study we demonstrated that TFF1 is up-regulated during acute Helicobacter infection and inversely correlated to inflammatory response, suggesting that it could help cells to counteract bacteria and the development of a chronic inflammation.
Author Contributions
SM, AP, and AT conceived and designed the experiments; DE, RE, SM, and MV performed the experiments, RE, SM, AP, and AT analyzed the data; AP and AT wrote the manuscript. All authors reviewed and approved the manuscript.
Funding
This work was supported by University of Salerno intramural funds FARB and P.O.R. Campania FESR 2007–2013-O.O. 2.1 - OCKEY (Oncology and Cardiology Key targets). DE was granted by FUV (Fondazione Umberto Veronesi) fellowship.
Conflict of Interest Statement
The authors declare that the research was conducted in the absence of any commercial or financial relationships that could be construed as a potential conflict of interest.
Supplementary Material
The Supplementary Material for this article can be found online at: https://www.frontiersin.org/articles/10.3389/fcimb.2017.00434/full#supplementary-material
References
Aihara, E., Engevik, K. A., and Montrose, M. H. (2017). Trefoil factor peptides and gastrointestinal function. Annu. Rev. Physiol. 79, 357–380. doi: 10.1146/annurev-physiol-021115-105447
Bulitta, C. J., Fleming, J. V., Raychowdhury, R., Taupin, D., Rosenberg, I., and Wang, T. C. (2002). Autoinduction of the trefoil factor 2 (TFF2) promoter requires an upstream cis-acting element. Biochem. Biophys. Res. Commun. 293, 366–374. doi: 10.1016/S0006-291X(02)00199-7
Calvet, X., Ramírez Lázaro, M. J., Lehours, P., and Mégraud, F. (2013). Diagnosis and epidemiology of Helicobacter pylori infection. Helicobacter 18(Suppl. 1), 5–11. doi: 10.1111/hel.12071
Carvalho, R., Kayademir, T., Soares, P., Canedo, P., Sousa, S., Oliveira, C., et al. (2002). Loss of heterozygosity and promoter methylation, but not mutation, may underlie loss of TFF1 in gastric carcinoma. Lab. Invest. 82, 1319–1326. doi: 10.1097/01.LAB.0000029205.76632.A8
Cover, T. L., and Blaser, M. J. (2009). Helicobacter pylori in health and disease. Gastroenterology 136, 1863–1873. doi: 10.1053/j.gastro.2009.01.073
Esposito, R., Montefusco, S., Ferro, P., Monti, M. C., Baldantoni, D., Tosco, A., et al. (2015). Trefoil factor 1 is involved in gastric cell copper homeostasis. Int. J. Biochem. Cell Biol. 59, 30–40. doi: 10.1016/j.biocel.2014.11.014
Farrell, J. J., Taupin, D., Koh, T. J., Chen, D., Zhao, C. M., Podolsky, D. K., et al. (2002). TFF2/SP-deficient mice show decreased gastric proliferation, increased acid secretion, and increased susceptibility to NSAID injury. J. Clin. Invest. 109, 193–204. doi: 10.1172/JCI0212529
Ferro, A., Peleteiro, B., Malvezzi, M., Bosetti, C., Bertuccio, P., Levi, F., et al. (2015). Worldwide trends in gastric cancer mortality (1980-2011), with predictions to, and incidence by subtype. Eur. J. Cancer 50, 1330–1344. doi: 10.1016/j.ejca.2014.01.029
Figueiredo, C. A., Marques, C. R., Costa Rdos, S., da Silva, H. B., and Alcantara-Neves, N. M. (2014). Cytokines, cytokine gene polymorphisms and Helicobacter pylori infection: friend or foe? World J. Gastroenterol. 20, 5235–5243. doi: 10.3748/wjg.v20.i18.5235
Graham, D. Y., Opekun, A. R., Osato, M. S., El-Zimaity, H. M., Lee, C. K., Yamaoka, Y., et al. (2004). Challenge model for Helicobacter pylori infection in human volunteers. Gut 53, 1235–1243. doi: 10.1136/gut.2003.037499
Hayakawa, Y., Fox, J. G., Gonda, T., Worthley, D. L., Muthupalani, S., and Wang, T. C. (2013). Mouse models of gastric cancer. Cancers 5, 92–130. doi: 10.3390/cancers5010092
Im, S., Yoo, C., Jung, J. H., Choi, H. J., Yoo, J., and Kang, C. S. (2013). Reduced expression of TFF1 and increased expression of TFF3 in gastric cancer: correlation with clinicopathological parameters and prognosis. Int. J. Med. Sci. 10, 133–140. doi: 10.7150/ijms.5500
Kato, S., Matsukura, N., Togashi, A., Masuda, G., Matsuda, N., Yamada, N., et al. (2004). Sex differences in mucosal response to Helicobacter pylori infection in the stomach and variations in interleukin-8, COX-2 and trefoil factor family 1 gene expression. Aliment. Pharmacol. Ther. 20, 17–24. doi: 10.1111/j.1365-2036.2004.01985.x
Kjellev, S. (2009). The trefoil factor family - small peptides with multiple functionalities. Cell. Mol. Life Sci. 66, 1350–1369. doi: 10.1007/s00018-008-8646-5
Lauren, P. (1965). The two histological main types of gastric carcinoma: diffuse and so-called intestinal-type carcinoma. An attempt at a histo-clinical classification. Acta Pathol. Microbiol. Scand. 64, 31–49. doi: 10.1111/apm.1965.64.1.31
Lee, K. E., Khoi, P. N., Xia, Y., Park, J. S., Joo, Y. E., Kim, K. K., et al. (2013). Helicobacter pylori and interleukin-8 in gastric cancer. World J. Gastroenterol. 19, 8192–8202. doi: 10.3748/wjg.v19.i45.8192
Lefebvre, O., Chenard, M. P., Masson, R., Linares, J., Dierich, A., LeMeur, M., et al. (1996). Gastric mucosa abnormalities and tumorigenesis in mice lacking the pS2 trefoil protein. Science 274, 259–262. doi: 10.1126/science.274.5285.259
Marshall, B. J., Armstrong, J. A., McGechie, D. B., and Glancy, R. J. (1985). Attempt to fulfil Koch's postulates for pyloric Campylobacter. Med. J. Aust. 142, 436–439.
Mashimo, H., Wu, D. C., Podolsky, D. K., and Fishman, M. C. (1996). Impaired defense of intestinal mucosa in mice lacking intestinal trefoil factor. Science 274, 262–265. doi: 10.1126/science.274.5285.262
Matsuda, K., Yamauchi, K., Matsumoto, T., Sano, K., Yamaoka, Y., and Ota, H. (2008). Quantitative analysis of the effect of Helicobacter pylori on the expressions of SOX2, CDX2, MUC2, MUC5AC, MUC6, TFF1, TFF2, and TFF3 mRNAs in human gastric carcinoma cells. Scand. J. Gastroenterol. 43, 25–33 doi: 10.1080/00365520701579795
Moghanibashi, M., Mohamadynejad, P., Rasekhi, M., Ghaderi, A., and Mohammadianpanah, M. (2012). Polymorphism of estrogen response element in TFF1 gene promoter is associated with an increased susceptibility to gastric cancer. Gene 492, 100–103. doi: 10.1016/j.gene.2011.10.048
Montefusco, S., Esposito, R., D'Andrea, L., Monti, M. C., Dunne, C., Dolan, B., et al. (2013). Copper promotes TFF1-mediated Helicobacter pylori colonization. PLoS ONE 8:e79455. doi: 10.1371/journal.pone.0079455
Morris, A., and Nicholson, G. (1987). Ingestion of Campylobacter pyloridis causes gastritis and raised fasting gastric pH. Am. J. Gastroenterol. 82, 192–199.
Mustapha, P., Paris, I., Garcia, M., Tran, C. T., Cremniter, J., Garnier, M., et al. (2014). Chemokines and antimicrobial peptides have a cag-dependent early response to Helicobacter pylori infection in primary human gastric epithelial cells. Infect. Immun. 82, 2881–2889. doi: 10.1128/IAI.01517-13
Nurgalieva, Z. Z., Conner, M. E., Opekun, A. R., Zheng, C. Q., Elliott, S. N., Ernst, P. B., et al. (2005). B-cell and T-cell immune responses to experimental Helicobacter pylori infection in humans. Infect. Immun. 73, 2999–3006. doi: 10.1128/IAI.73.5.2999-3006.2005
Park, S. A., Ko, A., and Lee, N. G. (2011). Stimulation of growth of the human gastric pathogen Helicobacter pylori by atmospheric level of oxygen under high carbon dioxide tension. BMC Microbiol. 11:96. doi: 10.1186/1471-2180-11-96
Park, W. S., Oh, R. R., Park, J. Y., Lee, J. H., Shin, M. S., Kim, H. S., et al. (2000). Somatic mutations of the trefoil factor family 1 gene in gastric cancer. Gastroenterology 119, 691–698. doi: 10.1053/gast.2000.16483
Peterson, A. J., Menheniott, T. R., O'Connor, L., Walduck, A. K., Fox, J. G., Kawakami, K., et al. (2010). Helicobacter pylori infection promotes methylation and silencing of trefoil factor 2, leading to gastric tumor development in mice and humans. Gastroenterology 139, 2005–2017. doi: 10.1053/j.gastro.2010.08.043
Ren, J. L., Luo, J. Y., Lu, Y. P., Wang, L., and Shi, H. X. (2006). Molecular forms of trefoil factor 1 in normal gastric mucosa and its expression in normal and abnormal gastric tissues. World J. Gastroenterol. 12, 7361–7364. doi: 10.3748/wjg.v12.i45.7361
Robinson, K., Argent, R. H., and Atherton, J. C. (2007). The inflammatory and immune response to Helicobacter pylori infection. Best Pract. Res. Clin. Gastroenterol. 21, 237–259. doi: 10.1016/j.bpg.2007.01.001
Rogers, A. B. (2012). Histologic scoring of gastritis and gastric cancer in mouse models. Methods Mol. Biol. 921, 189–203. doi: 10.1007/978-1-62703-005-2_22
Schmitt, W., and Haas, R. (1994). Genetic analysis of the Helicobacter pylori vacuolating cytotoxin: structural similarities with the IgA protease type of exported protein. Mol. Microbiol. 12, 307–319. doi: 10.1111/j.1365-2958.1994.tb01019.x
Schmitz, J. M., McCracken, V. J., Dimmitt, R. A., and Lorenz, R. G. (2007). Expression of CXCL15 (Lungkine) in murine gastrointestinal, urogenital, and endocrine organs. J. Histochem. Cytochem. 55, 515–524. doi: 10.1369/jhc.6A7121.2007
Sobala, G. M., Crabtree, J. E., Dixon, M. F., Schorah, C. J., Taylor, J. D., Rathbone, B. J., et al. (1991). Acute Helicobacter pylori infection: clinical features, local and systemic immune response, gastric mucosal histology, and gastric juice ascorbic acid concentrations. Gut 32, 1415–1418. doi: 10.1136/gut.32.11.1415
Soutto, M., Chen, Z., Katsha, A. M., Romero-Gallo, J., Krishna, U. S., Piazuelo, M. B., et al. (2015a). Trefoil factor 1 expression suppresses Helicobacter pylori-induced inflammation in gastric carcinogenesis. Cancer 121, 4348–4358. doi: 10.1002/cncr.29644
Soutto, M., Romero-Gallo, J., Krishna, U., Piazuelo, M. B., Washington, M. K., Belkhiri, A., et al. (2015b). Loss of TFF1 promotes Helicobacter pylori-induced β-catenin activation and gastric tumorigenesis. Oncotarget 6, 17911–17922. doi: 10.18632/oncotarget.3772
Sun, Y., Wang, L., Zhou, Y., Mao, X., and Deng, X. (2016). Human trefoil factor 3 induces the transcription of its own promoter through STAT3. Sci. Rep. 6:30421. doi: 10.1038/srep30421
Taupin, D., Wu, D. C., Jeon, W. K., Devaney, K., Wang, T. C., and Podolsky, D. K. (1999). The trefoil gene family are coordinately expressed immediate-early genes: EGF receptor- and MAP kinase-dependent interregulation. J. Clin. Invest. 103, R31–R38. doi: 10.1172/JCI3304
Tomita, H., Takaishi, S., Menheniott, T. R., Yang, X., Shibata, W., Jin, G., et al. (2011). Inhibition of gastric carcinogenesis by the hormone gastrin is mediated by suppression of TFF1 epigenetic silencing. Gastroenterology 140, 879–891. doi: 10.1053/j.gastro.2010.11.037
Tosco, A., Monti, M. C., Fontanella, B., Montefusco, S., D'Andrea, L., Ziaco, B., et al. (2010). Copper binds the carboxy-terminus of trefoil protein 1 (TFF1), favoring its homodimerization and motogenic activity. Cell. Mol. Life Sci. 67, 1943–1955. doi: 10.1007/s00018-010-0309-7
Van De Bovenkamp, J. H., Korteland-Van Male, A. M., Büller, H. A., Einerhand, A. W., and Dekker, J. (2005). Infection with Helicobacter pylori affects all major secretory cell populations in the human antrum. Dig. Dis. Sci. 50, 1078–1086. doi: 10.1007/s10620-005-2708-4
Warren, J. R. (2000). Gastric pathology associated with Helicobacter pylori. Gastroenterol. Clin. North Am. 29, 705–751. doi: 10.1016/S0889-8553(05)70139-4
Keywords: Helicobacter infection, trefoil factors, TFF1, cytokines, acute infection, chronic infection
Citation: Esposito R, Morello S, Vllahu M, Eletto D, Porta A and Tosco A (2017) Gastric TFF1 Expression from Acute to Chronic Helicobacter Infection. Front. Cell. Infect. Microbiol. 7:434. doi: 10.3389/fcimb.2017.00434
Received: 17 July 2017; Accepted: 21 September 2017;
Published: 09 October 2017.
Edited by:
Jan Potempa, University of Louisville, United StatesReviewed by:
Ponlatham Chaiyarit, Khon Kaen University, ThailandRiti Sharan, Texas Health Science Center, United States
Copyright © 2017 Esposito, Morello, Vllahu, Eletto, Porta and Tosco. This is an open-access article distributed under the terms of the Creative Commons Attribution License (CC BY). The use, distribution or reproduction in other forums is permitted, provided the original author(s) or licensor are credited and that the original publication in this journal is cited, in accordance with accepted academic practice. No use, distribution or reproduction is permitted which does not comply with these terms.
*Correspondence: Amalia Porta, YXBvcnRhQHVuaXNhLml0
Alessandra Tosco, dG9zY29AdW5pc2EuaXQ=
†These authors have contributed equally to this work.