- 1Department of Preventive Environment and Nutrition, Institute of Biomedical Science, Tokushima University, Tokushima, Japan
- 2Graduate School of Technology, Industrial and Social Sciences, Tokushima University, Tokushima, Japan
Campylobacter jejuni invasion is closely related to C. jejuni pathogenicity. The intestinal epithelium contains polarized epithelial cells that form tight junctions (TJs) to provide a physical barrier against bacterial invasion. Previous studies indicated that C. jejuni invasion of non-polarized cells involves several cellular features, including lipid rafts. However, the dynamics of C. jejuni invasion of polarized epithelial cells are not fully understood. Here we investigated the interaction between C. jejuni invasion and TJ formation to characterize the mechanism of C. jejuni invasion in polarized epithelial cells. In contrast to non-polarized epithelial cells, C. jejuni invasion was not affected by depletion of lipid rafts in polarized epithelial cells. However, depletion of lipid rafts significantly decreased C. jejuni invasion in TJ disrupted cells or basolateral infection and repair of cellular TJs suppressed lipid raft-mediated C. jejuni invasion in polarized epithelial cells. In addition, pro-inflammatory cytokine, TNF-α treatment that induce TJ disruption promote C. jejuni invasion and lipid rafts depletion significantly reduced C. jejuni invasion in TNF-α treated cells. These data demonstrated that TJs prevent C. jejuni invasion from the lateral side of epithelial cells, where they play a main part in bacterial invasion and suggest that C. jejuni invasion could be increased in inflammatory condition. Therefore, maintenance of TJs integrity should be considered important in the development of novel therapies for C. jejuni infection.
Introduction
Campylobacter jejuni is a Gram-negative, spiral-shaped, microaerophilic bacterium that is found in birds and domestic animals. C. jejuni causes human bacterial food-borne diseases worldwide, and clinical symptoms are manifested as intestinal inflammation, abdominal pain, and diarrhea (Young et al., 2007). Several studies reported that C. jejuni can adhere to and invade epithelial cells in an infection process that induces secretion of the pro-inflammatory cytokine interleukin (IL)-8 by intestinal epithelial cells (Konkel and Jones, 1989; Hickey et al., 1999). IL-8 production recruit neutrophils to the infection site and subsequently host inflammatory responses to C. jejuni infection. Moreover, the mutant C. jejuni strains lacking invasion activity had attenuated inflammatory responses and several diarrhea symptoms in experimental animal models (Yao et al., 1997). Together these findings indicate that bacterial invasion into host intestinal epithelial cells plays a critical role in C. jejuni pathogenicity.
Previous studies identified several bacterial and host cellular factors involved in C. jejuni adherence and invasion. An extracellular matrix protein, fibronectin, is one of the characterized host cellular factors which interacts with C. jejuni adherence and some reports indicated that C. jejuni binding factor, CadF and FlpA protein, were involved in maximal adherence for the host cell (Monteville et al., 2003; Konkel et al., 2010). Moreover, a surface-exposed bacterial lipoprotein, JlpA, has also been reported as a key adherence factor for C. jejuni and it bound HSP-90α, a heat shock protein in host cells (Jin et al., 2001, 2003). In addition, the bacterial ABC transporter component PEB1 and an autotransporter protein CapA also mediated both adherence and invasion in host epithelial cells (Pei et al., 1998; Ashgar et al., 2007). Bipolar flagella or a major flagellin component FlaA had an important role in both motility of C. jejuni and bacterial invasion into host cells (Wassenaar et al., 1991). In addition to these function, C. jejuni flagella secretion system, similar with a Type III secretion system, was required for maximal cell invasion (Konkel et al., 1999; Christensen et al., 2009; Samuelson et al., 2013). Meanwhile, in the C. jejuni trafficking mechanisms, lipid rafts, which are well-known as cholesterol- and sphingolipid-rich plasma membrane microdomain, were essential for C. jejuni entry via caveolae-mediated endocytosis pathway (Wooldridge et al., 1996). Following to endocytosis, microfilaments and microtubules were required for C. jejuni translocation (Oelschlaeger et al., 1993; Biswas et al., 2003). Importantly, the cytotoxicity in C. jejuni infection was closely related with bacterial invasion ability and is independent of major virulence factor, such as cytoletal distending toxin (CDT) (Kalischuk et al., 2007).
The detail mechanisms of C. jejuni invasion have been investigated in non-polarized epithelial cells. For example, some earlier reports revealed that C. jejuni utilized the host cell scaffolding protein and signaling cascade to invade into host cells, including integrin, epidermal growth factor receptor (EGFR), focal adhesion kinase (FAK), and paxillin (Monteville et al., 2003; Boehm et al., 2011; Eucker and Konkel, 2012). In addition, Rho small GTPase Rac1 and Cdc42 activation also take part in C. jejuni entry (Krause-Gruszczynska et al., 2007). Those findings came from non-polarized epithelial cells using studies. In contrast, there were few report to examine the molecular mechanism of C. jejuni invasion in polarized epithelial cells. Few studies reported that C. jejuni invasion was attenuated by the host barrier function and this attenuation of C. jejuni invasion was mainly mediated by the apical junctional complexes termed tight junctions (TJs) (Beltinger et al., 2008). On the other hand, other studies reported that C. jejuni disrupted TJs and its disruption of TJs promoted C. jejuni invasion into intestinal epithelial cells from the basolateral regions of host cells (Monteville and Konkel, 2002; Chen et al., 2006; van Alphen et al., 2008; Bouwman et al., 2013). Despite some findings of the association between TJs and the invasion in non-polarized epithelial cell, the bacterial invasion mechanisms were poorly understood in polarized epithelial cells. We hypothesized that C. jejuni encountering host cellular factors locates in lateral or basolateral part, but TJs may prevent the C. jejuni encounter with host cellular factors in polarized epithelial cells.
In this study, we examined whether TJ impedes host trafficking mechanisms and contributes to C. jejuni invasion using polarized epithelial cells with both intact or TJ disrupted cell. We found difference in C. jejuni invasion was different between polarized and non-polarized epithelial cells, and C. jejuni invasion process in TJ-disrupted or TJ-unformed polarized cells were similar to that in non-polarized epithelial cells. In addition, calcium switch assay revealed that C. jejuni invasion was tightly regulated by TJ function. Furthermore, pro-inflammatory cytokine-mediated TJ disruption also increased the C. jejuni invasion process in polarized epithelial cells, and the date indicated that TJ disruption induced by both pharmacologic and physiological factor was affective in C. jejuni invasion. Here we firstly indicated the C. jejuni invasion process was similar to intestinal inflammatory state. Our data suggested that TJs interfered with C. jejuni invasion at cellular lateral membranes and physiological TJ disruption such as inflammatory response could facilitate C. jejuni invasion in polarized epithelial cells. Together these findings could help elucidate C. jejuni invasion mechanisms into host intestinal tissues.
Materials and Methods
Bacterial Strains and Culture Conditions
Campylobacter jejuni strains ATCC 700819 (NCTC11168) were obtained from American Type Culture Collection (ATCC). The bacteria were grown in Muller Hinton Broth (DIFCO) under a microaerobic atmosphere (5% O2, 10% CO2, 85%N2) at 37°C for 48 h. The bacteria were then diluted into fresh MH and cultured under a microaerobic atmosphere at 37°C for an additional 36 h. Salmonella enterica serovar enteritidis (S. Enteritidis) was cultured in Luria-Bertani (LB) medium at 37°C with shaking.
Cell Culture
The non-polarized cell lines HeLa, INT407 and polarized cell lines Caco-2 cells were cultured in Dulbecco's modified Eagle's medium (DMEM), high-glucose (Sigma-Aldrich) supplemented with 10% fetal bovine serum (FBS; GIBCO) and 50 μg/ml gentamycin (Sigma-Aldrich). The culture medium was changed every 4 days in Caco-2 cells. The culture medium was changed every 4 days. Polarized cell lines T-84 cells were cultured in Dulbecco's modified Eagle's medium nutrient mixture F-12 HAM (DMEM/F-12, 1:1; Sigma-Aldrich) supplemented with 10% FBS and 50 μg/ml gentamycin. The culture medium was changed every 2 days. All cells were incubated in 37°C in a humidified atmosphere containing 5% CO2.
Regent and Antibody
Ethylene Glycol Tetra-acetic Acid (EGTA) was purchase from Nacalai Tesque. Rhodamine Phalloidin was purchase from Molecular Probes. Methyl-β-cyclodextrin (MβCD), water soluble-cholesterol and FITC-dextran (4 kDa, 10 kDa) were purchase from Sigma-Aldrich. U18666A and Tumor necrosis factor-α (TNF-α) were purchase from Wako. Antibodies to the following were diluted in 3% BSA and used for Immunofluorescence staining ZO-1 (1:200; BD Bioscience), Alexa Fluor 568 (1:200, Molecular Probes).
Infection Protocols
Bacteria were harvested by centrifugation at the 3,000 rpm for 15 min in C. jejuni infection or 12000 rpm for 3 min in S. Enteritidis infection and supernatant was removed. The bacteria were washed by phosphate buffered saline (PBS; 137 mM NaCl, 8.1 mM anhydrous
Na2HPO4, 2.68 mM KCl, 1.47 mM KH2PO4, pH 7.4), centrifuged and resuspended in PBS. The bacteria concentration was adjusted to an optical density of 600 nm (OD600) of 1.0 by PBS. Before infection, the culture medium was removed and replaced with fresh DMEM medium without supplements. Cells were infected at a multiplicity of infection (MOI) of 100–200:1 in C. jejuni infection or 10–20:1 in S. Enteritidis infection. Infected cells were incubated at 37°C in 5% CO2.
Invasion and Adhesion Assay
INT407 and HeLa cells were seeded at a density of 1.0 × 105/well in 24-well plate and cultured for 3 days. Polarized Caco-2 and T84 cells were seeded at a density of 7.5 × 104/well and 2.5 × 105/well in 24-well plate and cultured for 7 days. For basolateral infection, Caco-2 cells were seeded at a density of 1.0 × 105/well in inverted 0.33 cm2 transwell insert containing 3.0 μm pores (Corning). After 6–9 h incubation, the cell seeded inserts were re-inverted into DMEM high glucose and cultured for 1 week. The cell culture medium was replenished every 2 days and cells were used for invasion experiments.
For invasion assay, cells were infected with C. jejuni for 6 h or S. Enteritidis for 1 h. After infection, the cells were incubated with gentamycin containing DMEM (100 or 500 μg/ml for C. jejuni and S. enteritidis, respectively) for 2 h to kill extracellular bacteria. After incubation, the cells were washed with PBS and lysed with 1% Triton-X in PBS for 5 min at 37°C. For adhesion experiments, cells were infected with bacteria at a MOI 20–30:1 for 1 h. After infection, the cells were washed five times with PBS and lysed with 0.1% Triton-X in PBS for 5 min at 37°C. The diluted cell lysates were plated on MH agar plates and incubated for 48 h under a microaerobic atmosphere. The number of intracellular bacteria was determined by counting colony forming units (CFU) and the values were normalized with respect to the protein concentration of the individual cells. Protein concentration was measured by using a BCA Protein Assay Kit (Thermo Fisher) according to the manufacturer's instructions.
Disruption of Tight Junctions (TJs)
To disrupt cellular TJs, differentiated Caco-2 or T-84 cells were incubated with 4 mM EGTA for 20 min or 20 ng/ml TNF-α for 48 h. After treatment, the cells were then washed one time with fresh free DMEM and used for infection.
Inhibitor Study for Lipid Raft-Mediated Bacterial Invasion
To disrupt lipid rafts, cells were treated with various concentrations of MβCD (1, 5, 10 mM), which removes cholesterol from the plasma cell membrane, and U18666A (7.5, 15, 30 μM) to block intracellular cholesterol trafficking and biosynthesis for 1 h prior to infection. The concentration of each inhibitor used in this study was determined by the previous publication of Elmi et al. (2012) and Konkel et al. (2013) for MβCD or Chen et al. (1993) and Field et al. (2008). for U18666A. respectively. About U18666A, we used twice higher concentration in this study compare to the previous reports because of the incubation time difference. We checked host cell viability by protein content in each experiment. In cholesterol complement assays, MβCD treated cells were incubated with water-soluble cholesterol (150 μg/ml) containing DMEM for 1 h. After treatment, the cells were then washed one time with fresh free DMEM and used for infection. To evaluate the effect of these treatment on cells, intracellular cholesterol levels were measured with a cholesterol assay kit (Wako) according to the manufacturer's instructions.
Immunofluorescence Staining
Caco-2 cells were seeded on glass cover slips at a density of 3 × 105/dish and were infected for 6 h with bacteria that had been previously incubated with the CFDA SE cell trace kit reagent (Molecular Probes) for 1 h in accordance with the manufacturer's instructions. After infection, the cells were washed with PBS and fixed with 4% paraformaldehyde in PBS at room temperature for 10 min. After washing three times with PBS, the cells were permeabilized with 0.2% Triton-X-PBS for 10 min and then treated with 3% BSA in PBS for 1 h before washing 3 times with PBS. The cells were incubated with primary antibody overnight at 4°C and washed 3 times with PBS before incubation with a secondary antibody conjugated with Alexa Fluor 568 at room temperature for 60 min and washing 3 times with PBS. For F-actin staining, cells were incubated with Rhodamine Phalloidin diluted in PBS (1: 100) at room temperature for 60 min and washing 3 times with PBS.
Transepithelial Electrical Resistance Measurement and Calcium Switch Assay
Caco-2 cells were seeded at a density of 1.0–2.0 × 105/well on 0.33 cm2 transwells for TER assessment. TER was measured using an EVOM epithelial Volt-Ohm meter (World Precision Instruments) in accordance with the manufacturer's instructions. For calcium switch assays, Caco-2 cells cultured 1 week were treated with EGTA as described above and were then washed with fresh medium and re-introduced into normal medium containing calcium. Cells were incubated at 37°C for indicated time and TER values was measured and invasion assay was performed.
Uptake of FITC-Dextran
Caco-2 cells were seeded at a at a density of 7.5 × 104/well and cultured for 1 week in 24-well plates following treatment with EGTA or MβCD as described above. After treatment, cells were washed with HEPES buffer (10 mM HEPES, 145 mM NaCl, 10 mM glucose, 5 mM KCl, 1 mM MgCl2, 1 mM CaCl2) and incubated with HEPES buffer containing either 0.2 mg/ml 4 kDa or 10 kDa FITC-dextran for 2 h. After incubation, the cells were washed five times with HEPES buffer and lysed with 1% Triton-X. Cell lysates were centrifuged at the 12,000 rpm for 10 min and were collected supernatant. Fluorescence in supernatant was measured by using fluorescence microplate reader at excitation and emission wavelengths of 492 and 520 nm, respectively.
Statistical Analyses
In all case, statistical analysis was performed by using student's t-test for pair date. Date from 3 independent experiments were evaluated. All tests were one-tailed.
Results
Lipid Raft Disruption Does Not Affect C. jejuni Invasion in Polarized Epithelial Cells
Previous studies indicated that C. jejuni can invade host cells via lipid raft-mediated endocytosis in non-polarized epithelial cells (Wooldridge et al., 1996). Here we found that treatment of the non-polarized cell lines INT407 and HeLa (Figures 1A,B, Supplementary Figure 1) with methyl-β-cyclodextrin (MβCD), a potent lipid raft-mediated endocytosis inhibitor that removes cellular cholesterol, significantly and dose-dependently decreased both cellular cholesterol content and C. jejuni invasion. This reduction could be recovered by cholesterol supplementation (Figures 1C,D, Supplementary Figure 1). To further confirm the contribution of lipid rafts to C. jejuni invasion, we analyzed the effect of U18666A, an inhibitor of intracellular cholesterol trafficking, on C. jejuni invasion. U18666A treatment of INT407 cells significantly decreased C. jejuni invasion without affecting the cholesterol content (Figures 1E,F). Together these results indicated that cell surface cholesterol and lipid rafts are essential for C. jejuni invasion of non-polarized epithelial cells. Next, we investigated the dynamics of C. jejuni invasion in polarized epithelial cells using Caco-2 and T-84 cells. In contrast to non-polarized epithelial cells, MβCD treatment of polarized Caco-2 and T-84 cells did not affect C. jejuni invasion, despite the reduction in intracellular cholesterol content (Figures 1G,H, Supplementary Figure 1), suggesting that the dynamics of C. jejuni invasion might differ between non-polarized and polarized epithelial cells.
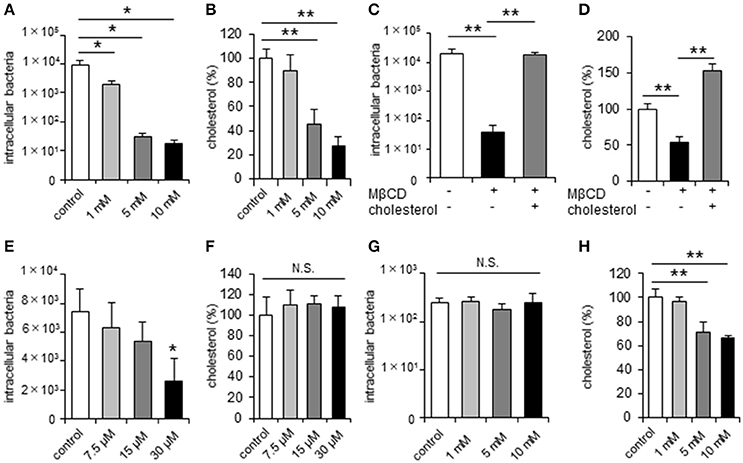
Figure 1. C. jejuni invasion is unchanged by disruption of lipid rafts in polarized epithelial cells. Non-polarized epithelial cells (INT407 cells) and polarized epithelial cells (Caco-2 cells) were pretreated with the indicated inhibitor and infected with C. jejuni for 6 h. The number of intracellular bacteria was measured using a gentamycin protection assay (A,C,E,G). The level of intracellular cholesterol was measured using a cholesterol assay kit (B,D,F,H). (A,B) INT407 cells treated with 1, 5, 10 mM MβCD for 1 h, (C,D) INT407 cells pretreated with 10 mM MβCD for 1 h or MβCD-treated cells subsequently supplemented with 150 μg/μl cholesterol for 1 h prior to infection. (E,F) INT407 cells pretreated with 7.5, 15, 30 μM U18666A for 1 h. (G,H) Caco-2 cells pre-treated with 1, 5, 10 mM MβCD for 1 h. Results are shown as the mean ± SD; n = 4-6. Significant difference from the control or MβCD treated group are shown: N.S.; not significant; *P < 0.05; **P < 0.01.
Lipid Raft Disruption Decreases C. jejuni Invasion in TJ Unformed Cells and Basolateral Surface Infection of Polarized Epithelial Cells
To investigate differences in C. jejuni invasion dynamics between non-polarized and polarized epithelial cells, we focused on tight junctions (TJs) formation in polarized epithelial cells. Cell polarization is established by TJ formation that separates the plasma membrane into apical and basolateral regions. Therefore, we performed invasion assays at different stages of cell culture to evaluate the relationship between TJ formation and C. jejuni invasion process. TJ formation of Caco-2 cells was assessed by measuring Trans Epithelial Resistance (TER) as previously described (Goyer et al., 2016) and this result showed significantly increase of TER values on day 6 post-seeding (Figure 2A), suggesting that mature TJs are present at this time point. As the TER values increased, the amount of C. jejuni invasion decreased. Interestingly, MβCD treatment significantly decreased C. jejuni invasion only in the short term (day 2 or 4 post-seeding) in cultured Caco-2 cells (Figure 2B). Similar results were seen for Caco-2 cells in a fluorescence microscopy assay using a CFDA SE cell tracer kit (Figures 2C–F). Based on these data, we hypothesized that the lateral or basolateral part of cells may contribute to C. jejuni invasion dynamics and C. jejuni susceptibility. Thus, we next performed an invasion assay using inverted-transwell inserts (Figure 2G). C. jejuni invasion was promoted in the basolateral region of cells, and MβCD treatment significantly reduced C. jejuni invasion only in the basolateral region (Figure 2H). These results suggested that basolateral region was critical for bacterial invasion in TJ formed cells, and that TJs provide the interface for the interaction between lipid rafts and invading C. jejuni.
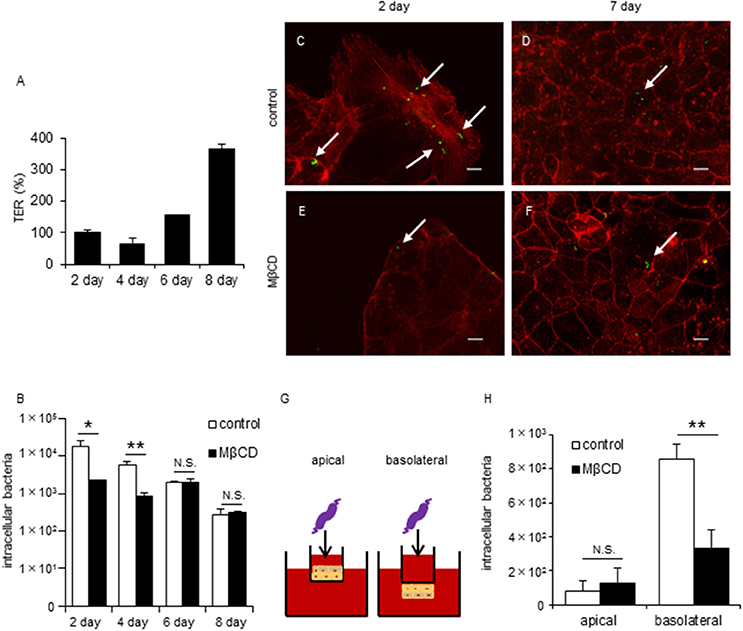
Figure 2. Disruption of lipid rafts reduces C. jejuni invasion of TJ unformed cells or infection of basolateral surfaces in polarized epithelial cells. Caco-2 cells were infected with C. jejuni for 6 h and the number of intracellular bacteria was measured by a gentamycin protection assay (B,H). (A) TER values were measured as a marker of TJ formation at 2, 4, 6, and 8 days post-seeding of Caco-2 cells cultured on transwells. TER values were calculated as the percentage of Day 2 post-seeding TER values. (B) Caco-2 cells cultured for 2, 4, 6, and 8 days following pre-treatment with 1-10 mM MβCD (black bar) or medium only (white bar) for 1 h. (C–F) Caco-2 cells cultured for 2 days (C,E) or 7 days (D,F) after pre-treatment with 1–10 mM MβCD for 1 h and subsequent infection with C. jejuni for 6 h. After infection, the cells were fixed, permeabilized and stained for F-actin (red). C. jejuni (green) was visualized using a CFDA SE cell tracer kit. Arrows indicate intracellular C. jejuni. Untreated control cells (C,D) and cells treated with MβCD alone (E,F) are also shown. Scale bar = 10 μm. (G,H) Caco-2 cells cultured in normal (apical) or inverted fashion (basolateral) on transwell inserts after pre-treatment with 10 mM MβCD for 1 h. Results are shown as the mean ± SD; n = 4. Significant difference from the control group are shown: N.S.; not significant; *P < 0.05; **P < 0.01.
TJ Disruption Is Closely Related to Lipid Raft-Mediated C. jejuni Invasion in Polarized Epithelial Cells
To further assess the role of TJs during C. jejuni invasion of polarized epithelial cells, invasion assays were performed using the Ca2+ chelator EGTA to disrupt TJs. EGTA treatment was associated with lower TER values and altered localization of the TJ marker protein ZO-1 (Supplementary Figure 2). EGTA treatment promoted C. jejuni invasion, which was significantly decreased by MβCD treatment only in cells with disrupted TJs. (Figure 3A). Similar results were observed for T-84 cells and Caco-2 cells following U18666A treatment (Supplementary Figure 2). To determine whether MβCD-mediated suppression of bacterial invasion in the presence of disrupted TJs was specific to C. jejuni, we also evaluated invasion of another human enteric pathogen, S. Enteritidis in polarized cells with disrupted TJs. In contrast to C. jejuni infection, EGTA treatment did not promote S. Enteritidis invasion and MβCD treatment did not decrease S. Enteritidis invasion in polarized cells with disrupted TJs (Figure 3B). Furthermore, we analyzed how EGTA or MβCD treatment affected endocytosis function by measuring uptake of FITC-dextran. EGTA or MβCD treatment had different effects on FITC-dextran uptake than that for C. jejuni invasion (Figure 3C). These results suggested that C. jejuni invasion has greater dependence on intact TJs in polarized cells than endocytosis.
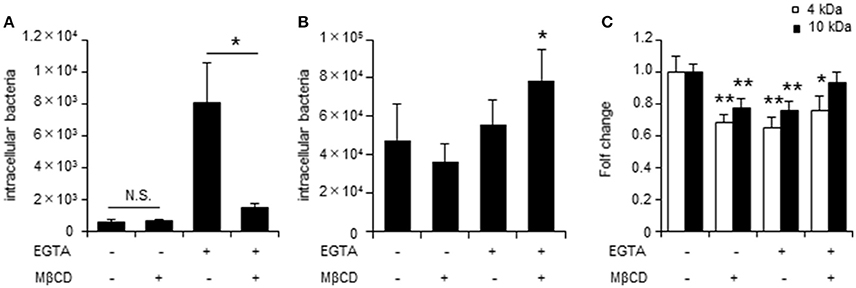
Figure 3. TJ disruption plays a key role in lipid raft-mediated C. jejuni invasion of polarized epithelial cells. The number of intracellular bacteria was measured by gentamycin protection assay. (A) Caco-2 cells were treated with 4 mM EGTA treatment for 20 min followed by 10 mM MβCD for 1 h and were infected with C. jejuni for 6 h. (B) Caco-2 cells pre-treated as in (A) were infected followed with S. Enteritidis for 1 h. (C) Caco-2 cells pre-treated as in (A) were incubated with 0.2 mg/ml 4 kDa (white bar) or 10 kDa FITC-dextran for 2 h. FITC-dextran uptake was evaluated by fluorescence measurements. Results are shown as the mean ± SD; n = 4–6. Significant difference from the control or EGTA treated group are shown: N.S.; not significant; *P < 0.05; **P < 0.01.
TJ Formation Affected C. jejuni Invasion Via Lipid Raft-Mediated Pathways in Polarized Epithelial Cells
Earlier studies reported that Ca2+ reintroduction recovered TER values and increased TJ integrity in EGTA-treated cells (Farshori and Kachar, 1999; Ronaghan et al., 2016). Therefore, we performed a calcium switch assay to examine the effect of TJ integrity on C. jejuni invasion. Consistent with earlier reports, we showed that Ca2+ reintroduction into Caco-2 cells restored the TER value (Figure 4A) and ZO-1 localization (Figures 4B–F) in a time-dependent manner. Furthermore, lipid raft-mediated C. jejuni invasion was significantly decreased following TJ restoration (Figure 4G). These data strongly suggested that cellular lateral or basolateral cell regions that are normally obscured by TJs are crucial for lipid raft-mediated C. jejuni invasion in polarized epithelial cells.
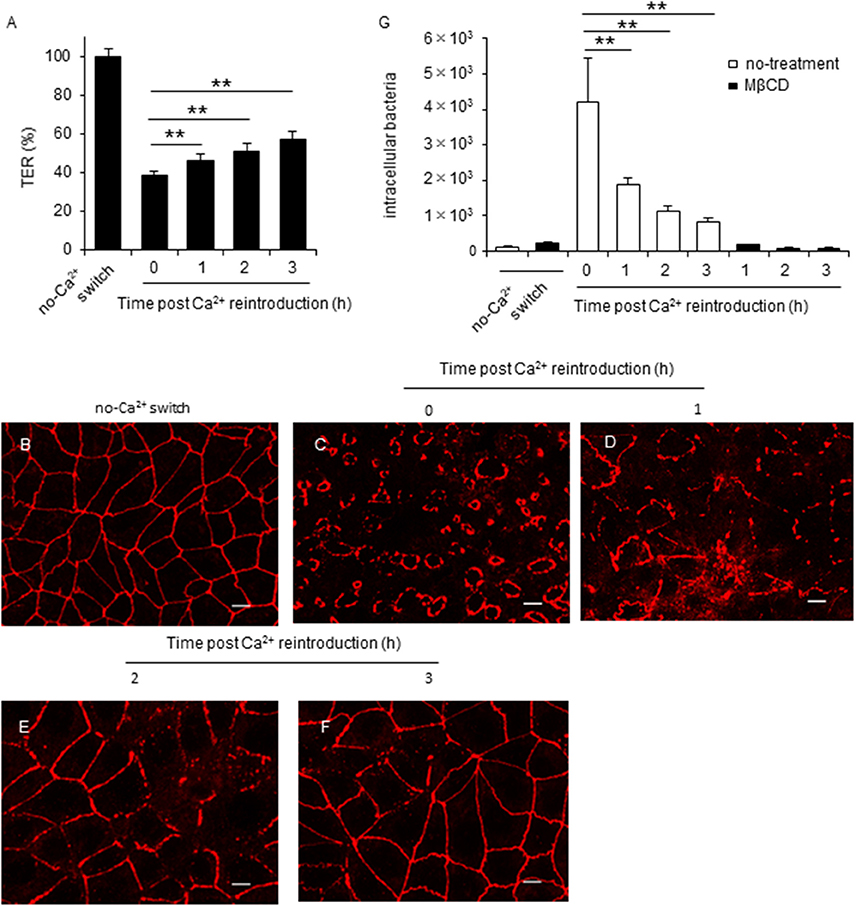
Figure 4. TJ disruption is tightly associated with lipid raft-mediated C. jejuni invasion in polarized epithelial cells. (A–F) Caco-2 cells cultured for 7 days on transwells and treated with 4 mM EGTA for 20 min. Following EGTA treatment, cells were incubated in normal culture medium. During the incubation, the TER value (A) and ZO-1 localization (B–F) were evaluated every hour for 3 h. TER values were calculated as the percentage of the TER value for untreated cells. Scale bar = 10 μm. (G) Caco-2 cells treated with 4 mM EGTA for 20 min were incubated in normal culture medium for the indicated time. After incubation, cells were pretreated with 10 mM MβCD for 1 h and infected with C. jejuni for 6 h. The number of intracellular bacteria was measured using a gentamycin protection assay. Results are shown as the mean ± SD; n = 4. Significant difference from the post Ca2+ reintroduction 0 h group are shown: N.S.; not significant; *P < 0.05; **P < 0.01.
C. jejuni Invasive Factor CapA Is Strongly Associated with Bacterial Invasion in Unpolarized Cells
Campylobacter jejuni invasion proceeds in two steps: adhesion and invasion. However, which step TJs affect is unclear. Here we first checked whether EGTA and MβCD affected C. jejuni adhesion in non-polarized INT407 cells and polarized Caco-2 cells and found no effect on adhesion by the respective compounds (Figures 5A,B). As mentioned above, during the C. jejuni invasion step, MβCD significantly decreased C. jejuni invasion of polarized cells only in EGTA-treated cells (Figure 3A). To further confirm the interaction between C. jejuni invasion and TJs, we used invasion-defective C. jejuni strains. A C. jejuni deletion mutant lacking the autotransporter protein CapA (Ashgar et al., 2007) had significantly lower invasion into host cells, particularly non-polarized cells (Figure 5C). In contrast to WT C. jejuni, invasion of the CapA mutant did not increase after EGTA treatment of Caco-2 cells to disrupt TJs (Figure 5D). These results suggested that TJs do not affect bacterial adhesion, but instead are involved in the invasion step. Moreover, an invasive factor such as CapA might have an important role in C. jejuni invasion dynamics mediated by TJs in polarized cells.
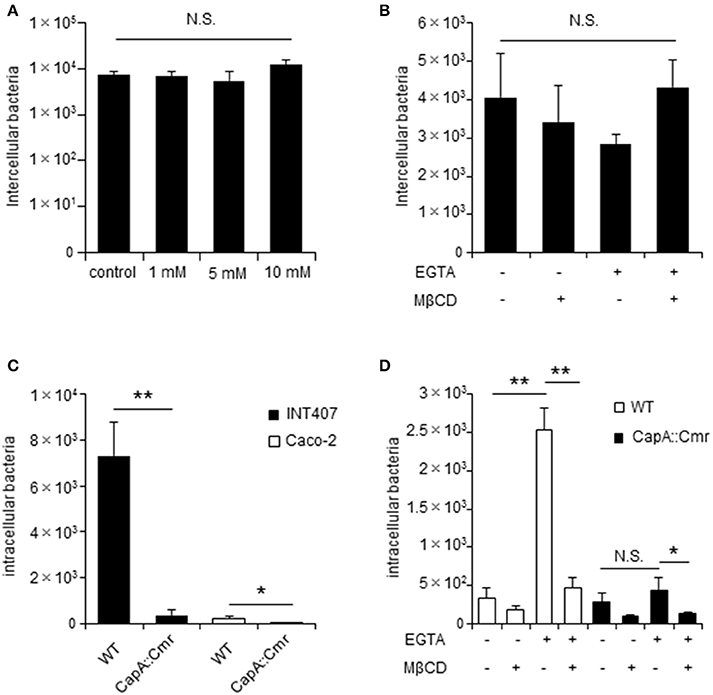
Figure 5. The C. jejuni invasive factor CapA is important for bacterial invasion of in TJs unformed cells. INT407 cells and Caco-2 cells were pretreated with the indicated compound and infected with C. jejuni for 1 or 6 h to evaluate bacterial adherence (A,B) and invasion (D,E). (A) INT407 cells treated with 1–10 mM MβCD for 1 h. (B) Caco-2 cells pretreated with 4 mM EGTA for 20 min followed by treatment with 10 mM MβCD for 1 h. The number of intercellular bacteria was determined by an adhesion assay (A,B). (C) INT407 cells (black bar) and Caco-2 cells (white bar) were infected with wild type (WT) C. jejuni or a CapA mutant strain. (D) Caco-2 cells pretreated with 4 mM EGTA for 20 min followed by 10 mM MβCD for 1 h and infected with WT C. jejuni (white bar) or a CapA mutant strain (black bar). The number of intracellular bacteria was measured by a gentamycin protection assay (C,D). Results are shown as the mean ± SD; n = 4. Significant difference from the control group are shown: N.S.; not significant; *P < 0.05; **P < 0.01.
Intestinal Inflammation Promotes C. jejuni Invasion in Polarized Epithelial Cells
Several previous studies reported that active inflammation disrupts TJ formation and promotes barrier disruption in the intestine (Antoni et al., 2014; Lechuga and Ivanov, 2017) and proinflammatory cytokine, Tumor necrosis factor-α (TNF-α) play an important role in the intestinal barrier disruption (Ma et al., 2004). To investigate the influence of inflammation-mediated TJ disruption in C. jejuni invasion, we performed an invasion assay with polarized Caco-2 cells treated with TNF-α. Treatment of TNF-α significantly decreased TER value (Figure 6A) and induced ZO-1 localization change Figures 6B,C. Additionally, C. jejuni invasion was significantly increased and attenuated followed by MβCD treatment in TNF-α-treated polarized epithelial cells as measured by a gentamycin protection assay (Figure 6D). This result indicated that intestinal inflammation can induce barrier disruption and promote C. jejuni invasion in polarized epithelial cells.
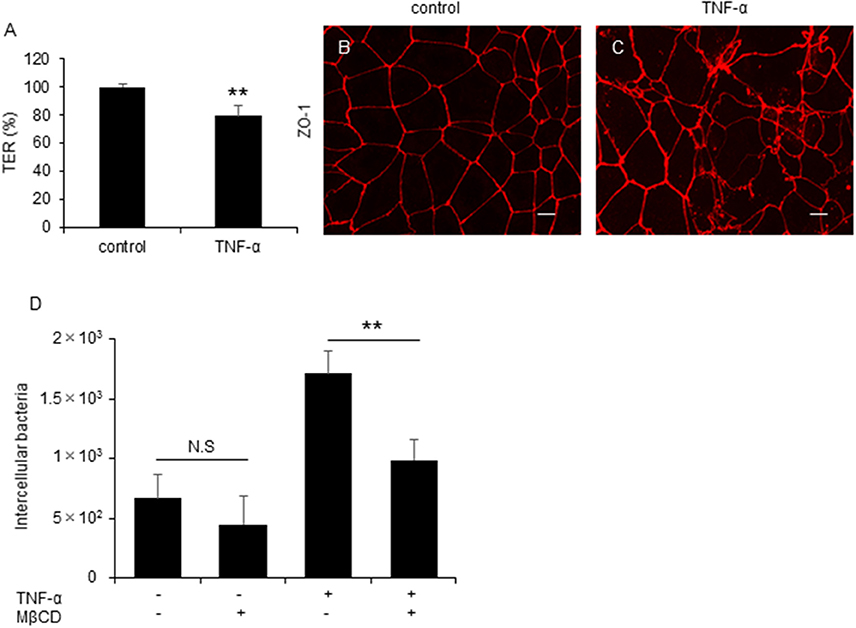
Figure 6. C. jejuni invasion is increased by active inflammation in polarized epithelial cells. (A–C) Caco-2 cells were treated with 20 ng/ml TNF-α for 48 h. Following TNF-α treatment, TER values (A) and ZO-1 localization (B,C) were evaluated. Scale bar = 10 μm. (D) Caco-2 cells were treated with 20 ng/ml TNF-α followed by treatment with 10 mM MβCD for 1 h and were infected with C. jejuni for 6 h. The number of intracellular bacteria was measured by gentamycin protection assay. Results are shown as the mean ± SD n = 4. Significant difference from the control or EGTA treated group are shown: N.S.; not significant; **P < 0.01.
Discussion
Campylobacter jejuni invasion mechanisms have been studied using non-polarized epithelial cells. In this study, we examined whether TJs could prevent C. jejuni invasion by comparing C. jejuni invasion of polarized and non-polarized cells under a variety of treatments to disrupt TJs. Treatment with MβCD, a potent lipid raft-mediated endocytosis inhibitor that removes cellular cholesterol, decreased C. jejuni invasion in non-polarized INT407 cells, but invasion was not affected in polarized Caco-2 cells treated with MβCD (Figures 1A,D). However, in Caco-2 cells, removal of lipid rafts decreased C. jejuni invasion in TJ unformed or disrupted cells (Figures 2B, 3A). These data strongly suggest that TJ formation prevents C. jejuni entry in the lateral part of polarized epithelial cells. On the other hand, a CapA deletion mutant that lacks invasion activity (Ashgar et al., 2007) had decreased invasion into non-polarized epithelial cells and polarized epithelial cells with disrupted TJs (Figures 5C,D). These results suggested that bacterial adhesion or host endocytic uptake function was not related to bacterial invasion of TJ disrupted cells, and that bacterial invasive factors (e.g., CapA) participated in C. jejuni invasion of the lateral regions of TJ disrupted cells. In the final experiment, we investigated the impact of inflammatory cytokine-mediated on TJ suppression in C. jejuni invasion. Similarly, we found that TNF-α treatment increased C. jejuni invasion and the increases of invasion were suppressed by MβCD treatment (Figure 6D). This result indicated that TJ disruption together with severe inflammation promoted C. jejuni invasion in intestinal epithelial cells.
The intestinal epithelium provides a physical barrier against pathogenic bacteria, and the function of this barrier is mainly regulated by TJs (Schneeberger and Lynch, 2004; Suzuki, 2013). On the other hand, C. jejuni infection could induce TJ disruption mediated by proteases contained in bacterial outer membrane vesicles in polarized epithelial cells (Elmi et al., 2016). Thus, barrier dysfunction induced by C. jejuni infection could promote bacterial invasion into host cells, and additionally, bacterial invasion could cause severe inflammation. Some previous report indicated that C. jejuni could induce various pro-inflammatory cytokine production such as IL-6, IL-8 and TNF-α (Hickey et al., 1999; Al-Salloom et al., 2003; Friis et al., 2009) and the pro-inflammatory cytokine on TJs had been investigated. IL-6 could increase TJ permeability by inducing expression of claudin-2, which is pore-forming claudins (Suzuki et al., 2011), and TNF-α is known to cause TJ disruption by decreasing TJ protein occluding and ZO-1 expression (Ma et al., 2004; He et al., 2012). Therefore, severe inflammation associated with C. jejuni infection could lead to barrier disruption and induce further bacterial invasion. Thus, TJ disruption with intestinal inflammation may contribute to a vicious cycle that greatly influences pathogenicity in C. jejuni infection. In addition, various factors could increase the risk of TJ disruption in the several intestinal diseases, including irritable bowel disease, celiac disease, and alcoholic river disease (van Elburg et al., 1993; Piche et al., 2009; Rao, 2009). Therefore, effects from underlying diseases that involve TJ disruption may contribute to C. jejuni infection.
Bacterial invasion is an important step to initiate infection. In general, there are two different routes for pathogen internalization: the transcellular route and the paracellular route. Pathogenic bacteria can enter at the apical surface of the cell before being subsequently endocytosed and trafficked via transcellular mechanisms (Kazmierczak et al., 2001). On the other hand, in the paracellular route, specialized pathogens can cross the epithelial barrier and pass between connected epithelial cells by breaking cell junctional complexes such as TJs (Balkovetz and Katz, 2003). Previous studies revealed that C. jejuni could invade host cells either via the transcellular or paracellular routes (Brás and Ketley, 1999; Boehm et al., 2012). Many studies reported that C. jejuni invasion by the transcellular route was associated with IL-8 production (Hickey et al., 1999; Watson and Galán, 2005). However, relative to the transcellular route, the detailed mechanism and role of the paracellular route in C. jejuni infection is less well understood. Our demonstration indicated that TJ disruption and paracellular mechanisms were subsequently linked to efficient endocytic C. jejuni entry, and that transcellular mechanisms involving the lateral cell membrane, both alone and combined, was important for a better understanding of C. jejuni invasion dynamics in polarized epithelial cells.
In this study, in order to analyze the role of TJs on C. jejuni invasion pathway, we utilized EGTA and TNF-α. However, these compounds also affected Adherens Junctions (AJs), another cell-cell junction complex, in polarized Caco-2 cells (Yi et al., 2009; Goyer et al., 2016). Therefore, further study may need to investigate contribution of AJs on the C. jejuni invasion in polarized epithelial cells. In addition, to examine how C. jejuni could entry into polarized epithelia cells with TJ disruption, we investigated lipid rafts-mediated mechanism by using MβCD and U18666A, that deplete plasma membrane cholesterol. Importantly, previous studies have shown that Caco-2 cells lacking cavolin-1 and caveolin-2, which lipid rafts do not contain caveolae (Bradbury et al., 1999). Thus, Caco-2 cells indicate special type of lipid rafts and C. jejuni can invade into Caco-2 cells via caveolae independent mechanism (Konkel et al., 2013). Interestingly, lipid rafts-mediated endocytosis inhibitor decreased C. jejuni invasion in TJ disrupted Caco-2 cells (Figure 3A, Supplementary Figure 2), and our data suggest that C. jejuni could invade into polarized epithelial cells not caveolae but lipid rafts-mediated pathway. Lipid rafts provide a critical role in the localization of various receptor proteins and control of the subsequent signaling pathway, such as integrin β1 or EGFR (Ringerike et al., 2002; Wang et al., 2010). Additionally, activation of these receptor proteins is strongly related to C. jejuni invasion (Boehm et al., 2011; Eucker and Konkel, 2012; Konkel et al., 2013). Therefore, we hypothesized that C. jejuni could invade into polarized epithelial cells with TJ disruption by utilizing but lipid rafts-related receptor protein and signaling cascade.
Early report indicated that intestinal brush borders, a special type of microdomain in apical parts of host intestinal epithelium, contain lipid rafts (Danielsen and Hansen, 2007). However, our results demonstrated that lipid rafts depletion did not influence C. jejuni invasion in presence of TJ formation in polarized epithelial cells (Figure 1G). Host intestinal epithelium is usually covered with mucus layer, and mucus layer is also considered as defensive line against luminal bacterial infection (McAuley et al., 2007). Therefore, we also hypothesized that host mucus layer might prevent C. jejuni attachment to lipid rafts in apical cell surface and could contribute to C. jejuni invasion process in polarized epithelial cells. Thus, the investigation of interaction between host physiological factors and C. jejuni invasion might be important to reveal invasion mechanism in polarized epithelial cells.
Although our study has apparently demonstrated the role of lipid rafts in C. jejuni invasion in TJ disrupted cells, we were unable to identify the specific receptor for C. jejuni invasion. Hence, the finding in our study are subject to several limitations. First, the concentration of these cholesterol depleting agents, MβCD and U18666A were decided based on previous reports (Chen et al., 1993; Field et al., 2008; Elmi et al., 2012; Konkel et al., 2013), may not specific deplete only lipid rafts cholesterol but also may affect cellular cholesterol contents. Thus, further substantiate evidences regarding C. jejuni invasion, future studies are required to identify the specific receptor of C. jejuni invasion on the lateral cell surface and confirm the relation without inhibitors system such as knockdown or specific targets neutralizing antibody. In addition, while gentamycin protection assay is a mainstay technique in bacterial pathogenesis, it is not without weakness as this method indirectly evaluate the number of intracellular bacteria and have some limitations (et al., 2012). Therefore, another valuable approach, such as fluorescence or electron microscopy (Friis et al., 2005), might be required to investigate more detail the dynamics of C. jejuni invasion.
In conclusion, our study indicates that cellular TJ formation strongly affects C. jejuni invasion dynamics in polarized epithelial cells. In addition, our data supports the view that maintenance of TJ integrity in physiological condition should be considered for the development of improved preventive and therapeutic approaches to treat C. jejuni infections.
Author Contributions
SH and TS designed this research. SH, SA, JK, AN, YS, YK, AYT, and SF performed experiments. SH, MN, TU, and KM analyzed the data. SH, TS, and AKT drafted the manuscript. All authors have read the manuscript and approved its submission.
Funding
This research was supported by a grant-in-aid for scientific research from JSPS Kakenhi (grant number JP 15K00819).
Conflict of Interest Statement
The authors declare that the research was conducted in the absence of any commercial or financial relationships that could be construed as a potential conflict of interest.
Acknowledgments
The authors would like to thank JAM post (www.jamp.com) for the English language review.
Supplementary Material
The Supplementary Material for this article can be found online at: https://www.frontiersin.org/articles/10.3389/fcimb.2018.00015/full#supplementary-material
References
Al-Salloom, F. S., Al Mahmeed, A., Ismaeel, A., Botta, G. A., and Bakhiet, M. (2003). Campylobacter stimulated INT407 cells produce dissociated cytokine profiles. J. Infect. 47, 217–224. doi: 10.1016/S0163-4453(03)00076-8
Antoni, L., Nuding, S., Wehkamp, J., and Stange, E. F. (2014). Intestinal barrier in inflammatory bowel disease. World J. Gastroenterol. 20, 1165–1179. doi: 10.3748/wjg.v20.i5.1165
Ashgar, S. S., Oldfield, N. J., Woolridge, K. G., Jones, M. A., Irving, G. J., Turner, D. P., et al. (2007). CapA, an autotransporter protein of Campylobacter jejuni mediates association with human epithelial cells and colonization of the chicken gut. J. Bacteriol. 189, 1856–1865. doi: 10.1128/JB.01427-06
Balkovetz, D. F., and Katz, J. (2003). Bacterial invasion by a paracellular route: divide and conquer. Microbes Infect. 5, 613–619. doi: 10.1016/S1286-4579(03)00089-3
Beltinger, J., del Buono, J., Skelly, M. M., Thornley, J., Spiller, R. C., Stack, W. A., et al. (2008). Disruption of colonic barrier function and induction of mediator release by strains of Campylobacter jejuni that invade epithelial cells. World J. Gastroenterol. 14, 7345–7352. doi: 10.3748/wjg.14.7345
Biswas, D., Itoh, K., and Sasakawa, C. (2003). Role of microfilaments and microtubules in invasion of INT-407 cells by Campylobacter jejuni. Microbiol. Immunol. 47, 469–473. doi: 10.1111/j.1348-0421.2003.tb03372.x
Boehm, M., Hoy, B., and Rohde, M. (2012). Rapid paracellular transmigration of Campylobacter jejuni across polarized epithelial cells without affecting TER: role of proteolytic-active HtrA cleaving E-cadherin but not fibronectin. Gut Pathog. 4:3.
Boehm, M., Krause-Gruszczynska, M., Rohde, M., Tegtmeyer, N., Takahashi, S., Oyarzabal, O. A., et al. (2011). Major host factors involved in epithelial cell invasion of Campylobacter jejuni: role of fibronectin, integrin beta1, FAK, Tiam-1, and DOCK180 in activating Rho GTPase Rac1. Front. Cell. Infect. Microbiol. 1:17. doi: 10.3389/fcimb.2011.00017
Bouwman, L. I., Niewold, P., and van Putten, J. P. (2013). Basolateral invasion and trafficking of Campylobacter jejuni in polarized epithelial cells. PLoS ONE 8:e54759. doi: 10.1371/journal.pone.0054759
Bradbury, N. A., Clark, J. A., Watkins, S. C., Widnell, C. C., Smith, H. S. IV., and Bridges, R. J. (1999). Characterization of the internalization pathways for cystic fibrosis transmembrane conductance regulator. Am. J. Physiol. 276, L659–L668. doi: 10.1152/ajplung.1999.276.4.L659
Brás, A. M., and Ketley, J. M. (1999). Transcellular translocation of Campylobacter jejuni across human polarized epithelial monolayers. FEMS Microbiol. Lett. 179, 209–215. doi: 10.1111/j.1574-6968.1999.tb08729.x
Chen, H., Born, E., Mathur, S. N., and Field, F. J. (1993). Cholesterol and sphingomyelin syntheses are regulated independently in cultured human intestinal cells, Caco-2: role of membrane cholesterol and sphingomyelin content. J. Lipid Res. 34, 2159–2167.
Chen, M. L., Ge, Z., Fox, J. G., and Schauer, D. B. (2006). Disruption of tight junctions and induction of proinflammatory cytokine responses in colonic epithelial cells by Campylobacter jejuni, Infect. Immun. 74, 6581–6589. doi: 10.1128/IAI.00958-06
Christensen, J. E., Pacheco, S. A., and Konkel, M. E. (2009). Identification of Campylobacter jejuni secreted protein required for maximal invasion of host cells. Mol. Microbiol. 73, 650–662. doi: 10.1111/j.1365-2958.2009.06797.x
Danielsen, E. M., and Hansen, G. H. (2007). Lipid rafts in epithelial brush border: atypical microdomains with specialized functions. Biochim. Biophys. Acta 1617, 1–9.
Elmi, A., Nasher, F., Jagatia, H., Gundogdu, O., Bajaj-Elliott, M., Wren, B., et al. (2016). Campylobacter jejuni outer membrane vesicle-associated proteolytic activity promotes bacterial invasion by mediating cleavage of intestinal epithelial cell E-cadherin and occludin. Cell. Microbiol. 18, 561–572. doi: 10.1111/cmi.12534
Elmi, A., Watson, E., Sandu, P., Gundogdu, O., Mills, D. C., Inglis, N. F., et al. (2012). Campylobacter jejuni outer membrane vesicles play an important role in bacterial interactions with human intestinal epithelial cells. Infect. Immun. 80, 4089–4098. doi: 10.1128/IAI.00161-12
Eucker, T. P., and Konkel, M. E. (2012). The cooperative action of bacterial fibronectin-binding proteins and secreted proteins promote maximal Campylobacter jejuni invasion of host cells by stimulating membrane ruffling. Cell. Microbiol. 14, 226–238. doi: 10.1111/j.1462-5822.2011.01714.x
Farshori, P., and Kachar, B. (1999). Redistribution and phosphorylation of occludin during opening and resealing of tight junctions in cultured epithelial cells. J. Membr. Biol. 170, 147–156. doi: 10.1007/s002329900544
Field, F. J., Watt, K., and Mathur, S. N. (2008). Origins of intestinal ABCA1-mediated HDL-cholesterol. J. Lipid Res. 49, 2605–2619. doi: 10.1194/jlr.M800302-JLR200
Friis, L. M., Keelan, M., and Taylor, D. E. (2009). Campylobacter jejuni drives MyD88 independent interleukin-6 secretion via Toll-like receptor 2. Infect. Immun. 77, 1553–1560. doi: 10.1128/IAI.00707-08
Friis, L. M., Pin, C., Pearson, B. M., and Wells, J. M. (2005). In vitro cell culture methods for investigating Campylobacter invasion mechanisms. J. Microbiol. Methods. 61, 145–160. doi: 10.1016/j.mimet.2004.12.003
Goyer, M., Loiselet, A., Bon, F., and L'Ollivier, C. (2016). Intestinal cell tight junctions limit invasion of Candida albicans through active penetration and endocytosis in the early stages of the interaction of the fungus with the intestinal barrier. PLoS ONE 11:e0149159. doi: 10.1371/journal.pone.0149159
He, F., Peng, J., Deng, X. L., Yang, L. F., Camara, A. D., Omran, A., et al. (2012). Mechanisms of tumor necrosis factor-alpha-induced leaks in intestine epithelial barrier. Cytokine 59, 264–272. doi: 10.1016/j.cyto.2012.04.008
Hickey, T. E., Baqar, S., Bourgeois, A. L., Ewing, C. P., and Guerry, P. (1999). Campylobacter jejuni-stimulated secretion of interleukin-8 by INT407 cells. Infect. Immun. 67, 88–93.
Jin, S., Joe, A., Lytnett, J., Hani, E. K., Sherman, P., and Chan, V. L. (2001). JlpA, a novel surface-exposed lipoprotein specific to Campylobacter jejuni, mediates adherence to host epithelial cells. Mol. Microbiol. 39, 1225–1236. doi: 10.1111/j.1365-2958.2001.02294.x
Jin, S., Song, Y. C., Emili, A., Sherman, P., and Chan, V. L. (2003). JlpA of Campylobacter jejuni interacts with surface-exposed heat shock protein 90 and triggers signaling pathways leading to the activation of NF-κB and p38 MAP kinase in epithelial cells. Cell. Microbiol. 5, 165–174. doi: 10.1046/j.1462-5822.2003.00265.x
Kalischuk, L. D., Inglis, G. D., and Buret, A. G. (2007). Strain-dependent induction of epithelial cell oncosis by Campylobacter jejuni is corelated with invasion ability and is independent of cytolethal distending toxin. Microbiology 153, 2952–2963. doi: 10.1099/mic.0.2006/003962-0
Kazmierczak, B. I., Mostov, K., and Engel, J. N. (2001). Interaction of bacterial pathogens with polarized epithelium. Annu. Rev. Microbiol. 55, 407–435. doi: 10.1146/annurev.micro.55.1.407
Konkel, M. E., and Jones, L. A. (1989). Adhesion to and invasion of HEp-2 cells by Campylobacter spp. Infect. Immun. 57, 2984–2990.
Konkel, M. E., Kim, B. J., Rivera-Amill, V., and Garvis, S. G. (1999). Bacterial secreted proteins are required for the internalization of Campylobacter jejuni into cultured mammalian cells. Mol. Microbiol. 32, 691–701. doi: 10.1046/j.1365-2958.1999.01376.x
Konkel, M. E., Larson, C. L., and Flanagan, R. C. (2010). Campylobacter jejuni FlpA binds fibronectin and is required for maximal host cell adherence. J. Bacteriol. 192, 68–76. doi: 10.1128/JB.00969-09
Konkel, M. E., Samuelson, D. R., Eucker, T. P., Shelden, E. A., and O'Loughlin, J. L. (2013). Invasion of epithelial cells by Campylobacter jejuni is independent of caveolae. Cell Commun. Signal. 11:100. doi: 10.1186/1478-811X-11-100
Krause-Gruszczynska, M., Rohde, M., Hartig, R., Genth, H., Schmidt, G., Keo, T., et al. (2007). Role of the small Rho GTPase Rac1 and Cdc42 in host cell invasion of Campylobacter jejuni. Cell. Microbiol. 9, 2431–2444. doi: 10.1111/j.1462-5822.2007.00971.x
Lechuga, S., and Ivanov, A. I. (2017). Disruption of the epithelial barrier during intestinal inflammation: quest for new molecules and mechanisms. Biochim. Biophys. Acta 1864, 1183–1194. doi: 10.1016/j.bbamcr.2017.03.007
Ma, T. Y., Iwamoto, G. K., Hoa, N. T., Akotia, V., Pedram, A., Boivin, M. A., et al. (2004). TNF-alpha-induced increase in intestinal epithelial tight junction permeability requires NF-kappa B activation. Am. J. Physiol. Gastrointest. Liver Physiol. 286, G367–G376. doi: 10.1152/ajpgi.00173.2003
McAuley, J. L., Linden, S. K., Png, C. W., King, R. M., Pennington, H. L., and Gendler, S. J. (2007). MUC1 cell surface mucin is a critical element of the mucosal barrier to infection. J. Clin. Invest. 117, 2313–2324. doi: 10.1172/JCI26705
Monteville, M. R., and Konkel, M. E. (2002). Fibronectin-facilitated invasion of T84 eukaryotic cells by Campylobacter jejuni occurs preferentially at the basolateral cell surface. Infect. Immun. 70, 6665–6671. doi: 10.1128/IAI.70.12.6665-6671.2002
Monteville, M. R., Yoon, J. E., and Konkel, M. E. (2003). Maximal adherence and invasion of INT 407 cells by Campylobacter jejuni requires the CadF outer-membrane protein and microfilament reorganization. Microbiology 149, 153–165. doi: 10.1128/J.B.01427-06
Ó. Cróinín, T., and Backert, S. (2012). Host epithelial cell invasion by Campylobacter jejuni: trigger or zipper mechanism? Front. Cell. Infect. Microbiol. 2:25.
Oelschlaeger, T. A., Guerry, P., and Kopecko, D. J. (1993). Unusual microtubule-dependent endocytosis mechanisms triggered by Campylobacter jejuni and Citrobacter freundii. Proc. Natl. Acad. Sci. U.S.A. 90, 6884–6888. doi: 10.1073/pnas.90.14.6884
Pei, Z., Burucoa, C., Grignon, B., Baqar, S., Huang, X. Z., Kopecko, D. J., et al. (1998). Mutation in the peb1A locus of Campylobacter jejuni reduces interactions with epithelial cells and intestinal colonization of mice. Infect. Immun. 66, 938–943.
Piche, T., Barbara, G., and Aubert, P. (2009). Impaired intestinal barrier integrity in the colon of patients with irritable bowel syndrome: involvement of soluble mediators. Gut. 58, 196–201. doi: 10.1136/gut.2007.140806
Rao, R. (2009). Endotoxemia and gut barrier dysfunction in alcoholic liver disease. Hepatology 50, 638–644. doi: 10.1002/hep.23009
Ringerike, T., Blystad, F. D., Levy, F. O., Madshus, I. H., and Stang, E. (2002). Cholesterol is important in control of EGF receptor kinase activity but EGF receptors is not concentrated in caveolae. J. Cell Sci. 115, 1331–1440.
Ronaghan, N. J., Shang, J., Iablokov, V., Zaheer, R., Colarusso, P., Dion, S., et al. (2016). The serine protease-mediated increase in intestinal epithelial barrier function is dependent on occludin and requires an intact tight junction. Am. J. Physiol. Gastrointest. Liver Physiol. 311, G466–G479. doi: 10.1152/ajpgi.00441.2015
Samuelson, D. R., Eucker, T. P., Bell, J. A., Dybas, L., Mansfield, L. S., and Konkel, M. E. (2013). The Campylobacter jejuni CiaD effector protein activates MAP kinase signaling pathways and is required for the development of disease. Cell Commun. Signal. 11:79. doi: 10.1186/1478-811X-11-79
Schneeberger, E. E., and Lynch, R. D. (2004). The tight junction: a multifunctional complex. Am. J. Physiol. Cell Physiol. 286, C1213–C1228. doi: 10.1152/ajpcell.00558.2003
Suzuki, T. (2013). Regulation of intestinal epithelial permeability by tight junctions. Cell. Mol. Life Sci. 70, 631–659. doi: 10.1007/s00018-012-1070-x
Suzuki, T., Yoshinaga, N., and Tanabe, S. (2011). Interleukin-6 (IL-6) regulates claudin-2 expression and tight junctions permeability in intestinal epithelium. J. Boil. Chem. 286, 31263–31271. doi: 10.1074/jbc.M111.238147
van Alphen, L. B., Bleumink-Pluym, N. M., Rochat, K. D., van Balkom, B. W., Wösten, M. M., and van Putten, J. P. (2008). Active migration into the subcellular space precedes Campylobacter jejuni invasion of epithelial cells. Cell. Microbiol. 10, 53–66.
van Elburg, R. M., Uil, J. J., Mulder, C. J., and Heymans, H. S. (1993). Intestinal permeability in patients with coeliac disease and relatives of patients with coeliac disease. Gut. 34, 354–357. doi: 10.1136/gut.34.3.354
Wang, C., Yoo, Y., Fan, H., Kim, E., Guan, K. L., and Guan, J. L. (2010). Regulation of integrin β1 recycling to lipid rafts by Rab1a to promote cell migration. J. Biol. Chem. 285, 29398–29405. doi: 10.1074/jbc.M110.141440
Wassenaar, T. M., Bleumink-Pluym, N. M., and van der Zeiist, B. A. (1991). Inactivation of Campylobacter jejuni flagellin genes by homologous recombination demonstrates that flaA but not flaB is required for invasion. EMBO J. 10, 2055–2061.
Watson, R. O., and Galán, J. E. (2005). Signal transduction in Campylobacter jejuni induced cytokine production. Cell. Microbiol. 7, 655–665. doi: 10.1111/j.1462-5822.2004.00498.x
Wooldridge, K. G., Williams, P. H., and Ketley, J. M. (1996). Host signal transduction and endocytosis of Campylobacter jejuni. Microb. Pathog. 21, 299–305. doi: 10.1006/mpat.1996.0063
Yao, R., Burr, D. H., and Guerry, P. (1997). CheY-mediated modulation of Campylobacter jejuni virulence. Mol. Microbiol. 23, 1021–1031. doi: 10.1046/j.1365-2958.1997.2861650.x
Yi, J. Y., Jung, Y. J., Choi, S. S., and Chung, E. (2009) TNF-α downregulates E-cadherin sensitizes response to δ-irradiation in Caco-2 cells. Cancer. Res. Treat. 41, 164–170. doi: 10.4143/crt.2009.41.3.164
Keywords: Campylobacter jejuni, Tight Junctions (TJs), lipid rafts, cell invasion, lateral part of epithelial cells
Citation: Hatayama S, Shimohata T, Amano S, Kido J, Nguyen AQ, Sato Y, Kanda Y, Tentaku A, Fukushima S, Nakahashi M, Uebanso T, Mawatari K and Takahashi A (2018) Cellular Tight Junctions Prevent Effective Campylobacter jejuni Invasion and Inflammatory Barrier Disruption Promoting Bacterial Invasion from Lateral Membrane in Polarized Intestinal Epithelial Cells. Front. Cell. Infect. Microbiol. 8:15. doi: 10.3389/fcimb.2018.00015
Received: 16 October 2017; Accepted: 12 January 2018;
Published: 30 January 2018.
Edited by:
Alfredo G. Torres, University of Texas Medical Branch, United StatesReviewed by:
Thomas Stephens Wilkinson, Swansea University, United KingdomQijing Zhang, Iowa State University, United States
Copyright © 2018 Hatayama, Shimohata, Amano, Kido, Nguyen, Sato, Kanda, Tentaku, Fukushima, Nakahashi, Uebanso, Mawatari and Takahashi. This is an open-access article distributed under the terms of the Creative Commons Attribution License (CC BY). The use, distribution or reproduction in other forums is permitted, provided the original author(s) and the copyright owner are credited and that the original publication in this journal is cited, in accordance with accepted academic practice. No use, distribution or reproduction is permitted which does not comply with these terms.
*Correspondence: Takaaki Shimohata, c2hpbW9oYXRhQHRva3VzaGltYS11LmFjLmpw