- 1Department of Bacteriology and Epidemiology, Wageningen Bioveterinary Research, Wageningen University and Research, Lelystad, Netherlands
- 2Faculty of Veterinary Medicine, Dutch Wildlife Health Centre, Utrecht University, Utrecht, Netherlands
- 3National Institute for Public Health and the Environment (RIVM), Bilthoven, Netherlands
- 4GGD Rotterdam Rijnmond, Rotterdam, Netherlands
- 5Netherlands Food and Consumer Product Safety Authority, Utrecht, Netherlands
- 6Department of Medical Microbiology and Infectious Diseases, Canisius Wilhelmina Hospital, Nijmegen, Netherlands
- 7Department of Internal Medicine, Diakonessenhuis, Utrecht, Netherlands
- 8Department of Medical Microbiology en Immunology, St. Antonius Hospital, Nieuwegein, Netherlands
- 9Swedish Defence Research Agency, Umeå, Sweden
Sequence-based typing of Francisella tularensis has led to insights in the evolutionary developments of tularemia. In Europe, two major basal clades of F. tularensis subsp. holarctica exist, with a distinct geographical distribution. Basal clade B.6 is primarily found in Western Europe, while basal clade B.12 occurs predominantly in the central and eastern parts of Europe. There are indications that tularemia is geographically expanding and that strains from the two clades might differ in pathogenicity, with basal clade B.6 strains being potentially more virulent than basal clade B.12. This study provides information on genotypes detected in the Netherlands during 2011–2017. Data are presented for seven autochthonous human cases and for 29 European brown hares (Lepus europaeus) with laboratory confirmed tularemia. Associated disease patterns are described for 25 European brown hares which underwent post-mortem examination. The basal clades B.6 and B.12 are present both in humans and in European brown hares in the Netherlands, with a patchy geographical distribution. For both genotypes the main pathological findings in hares associated with tularemia were severe (sub)acute necrotizing hepatitis and splenitis as well as necrotizing lesions and hemorrhages in several other organs. Pneumonia was significantly more common in the B.6 than in the B.12 cases. In conclusion, the two major basal clades present in different parts in Europe are both present in the Netherlands. In hares found dead, both genotypes were associated with severe acute disease affecting multiple organs. Hepatitis and splenitis were common pathological findings in hares infected with either genotype, but pneumonia occurred significantly more frequently in hares infected with the B.6 genotype compared to hares infected with the B.12 genotype.
Introduction
Tularemia, caused by the bacterium Francisella tularensis, may be regarded as a relatively new disease in Western Europe. Until 1929, the disease was restricted to Russia and parts of Scandinavia. In the late 1930s the disease was first reported in (current) Austria, and additional outbreaks were described in following decades from several other countries like Germany, France, and Belgium (Jusatz, 1952). Italy and Spain are the most recent countries to report cases, in 1964 and 1997, respectively (Dwibedi et al., 2016). In Europe, disease is almost exclusively caused by F. tularensis subsp. holarctica.
Because of the limited genetic variation in F. tularensis, single nucleotide polymorphisms (SNP) and multiple loci variable number of tandem repeats analysis (MLVA) are the preferred genetic markers for molecular typing. A first set of canonical SNPs (canSNPs), representing key positions along the Francisella phylogenetic tree were identified and labeled by serial numbers (Svensson et al., 2009; Vogler et al., 2009) and the resulting phylogenetic structure is still the basis for the current nomenclature for F. tularensis subsp. holarctica genotypes; an acronym using the letter “B” for the subspecies holarctica, and for subclades the characters “Br.” followed by the branch numbers named for the two flanking canSNPs (for instance B.Br.010/011), or the designated identification of a reference strain (for instance B.Br.FTNF002-00).
Since then, there has been a substantial increase in the available molecular data of F. tularensis strains from Europe (Chanturia et al., 2011; Gyuranecz et al., 2012; Karlsson et al., 2013; Afset et al., 2015; Sissonen et al., 2015; Dwibedi et al., 2016; Schulze et al., 2016). Additional serial numbered SNPs have been used to increase the discriminatory power. As a result, the phylogenetic tree for F. tularensis subsp. holarctica has expanded tremendously (Larkeryd et al., 2014). This inherently led to continuous changes in terminology, sometimes making it difficult to compare results from various studies. Nomenclature has been simplified by removing the “Br.” and instead of using the two flanking canSNPs, only the marker that defines the branch is now specified. More important, with new strains and additional subclades being included, major genetic lineages for subspecies holarctica are also distinguished. The current nomenclature defines four basal clades within the subspecies holarctica; B.4, B.6, B.12, and B.16. In Europe, most F. tularensis subsp. holarctica strains belong to basal clades B.6 and B.12.
Basal clade B.6 includes subclades B.7 and B.10. The B.7 subclade (also referred to as genotype B.40 or B.Br.OR96-0246) is predominantly found in Scandinavia (Karlsson et al., 2013; Afset et al., 2015; Sissonen et al., 2015). The B.10 (also designated as B.41) subclade is mainly represented by strains that are members of B.11, previously referred to as the (Franco)Iberian clone (Dempsey et al., 2007). This B.11 subclade is characterized by a 1.59 kb deletion, termed RD23, and matches the B.Br.FTNF002-00 subpopulation as defined by Vogler (Vogler et al., 2009). This B.11 subclade is present in the western part of Europe (Gyuranecz et al., 2012; Dwibedi et al., 2016).
Basal clade B.12 includes subclade B.13 previously described as B.Br.013/014 (Gyuranecz et al., 2012), and can be further divided into subclades B.27 (also known as B.43), B.20 (also known as B.42) and B.23 (Figure 1). A perfect correlation between clade B.12 and Biovar II strains has recently been shown (Karlsson et al., 2016). A unique distinctive feature of Biovar II strains is resistance to erythromycin, oleandomycin and lincomycin (Kudelina and Olsufiev, 1980). Strains belonging to clade B.12 (and Biovar II) are predominantly found in East and Central Europe (Kudelina and Olsufiev, 1980; Gyuranecz et al., 2012; Karlsson et al., 2016).
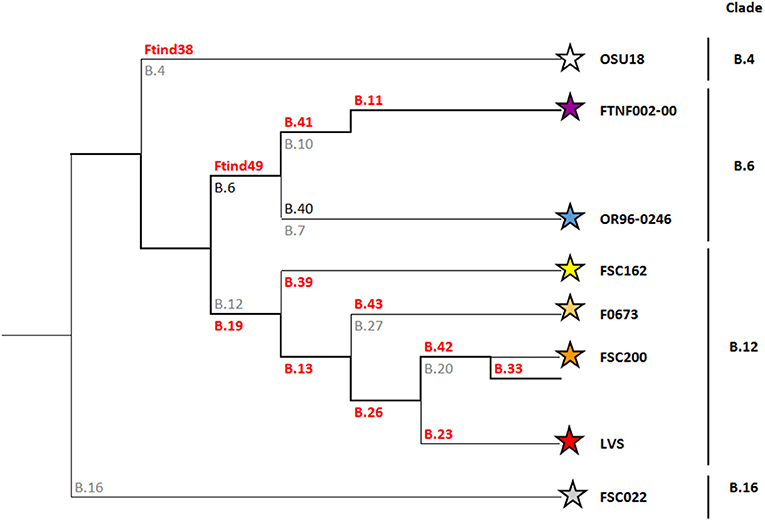
Figure 1. Phylogeny of Francisella tularensis subsp. holarctica. SNP and Indel markers, for which assays have been developed, are depicted in orange. These markers define the four major canSNP clades B.12, B.6, B.16, and B.4, and six subclades (Karlsson et al., 2013). For guidance, previously published corresponding markers are depicted in gray.
Genotyping studies have shown that subspecies holarctica probably originates from Asia or North America and has successfully disseminated worldwide in recent evolutionary time (Johansson et al., 2004; Keim et al., 2007; Vogler et al., 2009; Petersen and Molins, 2010; Karlsson et al., 2013; Lu et al., 2016). However, the spread of the disease within Europe is poorly understood, as are potential differences in pathology and pathogenic potential between genotypes. Pathology was found to be different in European brown hares (Lepus europaeus) infected with the B.6 clade in Switzerland compared with those infected with the B.12 genotype in Switzerland and to the lesions described in Hungary (Gyuranecz et al., 2010; Origgi and Pilo, 2016). These results suggested differences in pathogenesis between the two distinct clades of F. tularensis subsp. holarctica in Europe. In addition, experimental infection of Fischer 344 rats with a B.6 subclade B.11 strain from Italy resulted in a more severe disease presentation when compared to rats infected with a B.12 genotype from Hungary (Kreizinger et al., 2017).
The first reported case of human tularemia in the Netherlands dates back to 1953, when multiple members of a family contracted tularemia by consumption of a European brown hare that was found dead (Hemmes, 1953). No endemic human tularemia cases were reported in the Netherlands until 2011, when a human tularemia case was identified with no history of traveling abroad (Coolen et al., 2013; Maraha et al., 2013). Subsequently, between 2011 and the end of 2017, a total of 17 laboratory confirmed human cases of indigenous tularemia were identified. Surveillance of tularemia in Dutch European brown hares started in 2011, and the first hare case was confirmed in 2013. A total of 29 hare laboratory confirmed cases were diagnosed throughout the country by the end of 2017 (Rijks et al., 2013; Janse et al., 2017). Additionally, tularemia was identified in primates from a shelter house (Bolhuis et al., 2017) and in water specimens (Janse et al., 2017). It is unclear to what extent disease emergence and/or increased awareness and detection explain the recent increase in human and hare cases in the Netherlands. Genetic information from autochthonous strains and associated disease patterns may provide some clues and is expected to improve the understanding of the epidemiology of the disease.
This paper describes the genotyping results based on a hierarchical real-time PCR typing scheme using several canSNPs and canonical insertion deletion mutations (canINDEL) markers. Genotyping results, clinical presentation and likely route of infection are presented for seven autochthonous human cases in which infection with F. tularensis was detected by PCR and/or culture. Additionally, genotyping results from 29 hares are described and pathology findings analyzed per genotype to investigate whether the frequency of occurrence and the severity of lesions differs between clades.
Materials and Methods
Cases in Humans
Of the 17 human cases of indigenous tularemia in the period 2011–2017, laboratory confirmation was based on either serology (n = 6), detection of F. tularensis (n = 6), or both (n = 5). For this study genotyping results from seven patients were available (Table 1). DNA was either obtained from isolates (n = 3) or directly from wound exudate or suppurative lymph node material submitted for diagnostic testing (n = 4). All materials from patients were tested anonymously. The most likely source and location of infection was determined based on patient interviews by local public health services. Ethical approval was not needed according to institutional and national guidelines. It was confirmed by the Utrecht medical ethics committee that the study is exempt from further ethics approval (METC UMC Utrecht, reference number 18-617).
Cases in Hares
DNA used for genotyping of 29 European brown hare cases was obtained directly from specimen material that had been submitted for diagnostic testing. Of those, 27 confirmed hare cases were submitted in context of the Dutch national wildlife disease scanning surveillance program, and two hares were submitted because they were thought to be the source of human infection through skinning of the hares. In the 27 hares from the surveillance program, the presence of F. tularensis genetic material had been confirmed in three tissues (lung, liver and spleen) by a diagnostic PCR-test, as described earlier (Rijks et al., 2013). In the two hares linked to human cases, the specimen materials were muscle and bone marrow, as organs were no longer available at the time of testing. The spatial distributions of the human cases and the hare cases were mapped using ArcGIS© software.
For comparison, significant lesions that were found in the sampled hares were grouped according to the Francisella genotype. Case histories and descriptions of lesions had been recorded in necropsy reports; body condition was scored based on degree of fat storage and muscle development, and gross pathology findings were recorded following a standard protocol. For histopathology, tissue specimens were fixed in 4% phosphate-buffered formalin, embedded in paraffin, cut into 4-μm sections, and stained with hematoxylin and eosin. Tissues examined routinely using histopathology were lung, heart, spleen, liver, stomach, duodenum, jejunum, ileum, caecum, colon, kidney, adrenals, and brain. Other tissues were only sampled and examined if there was a clear indication for lesions.
Genotyping
DNA was obtained from standard specimens that were positive for the presence of F. tularensis by TaqMan real-time PCR using the FTT0376 primers and probe published by Mitchell et al. (2010). Specimen material was processed under BSL3 conditions. All containment facilities comply with national and international laws and regulations concerning biosafety (EU Directive 2000/54/EC) and biosecurity (Code of Conduct of the Royal Netherlands Academy of Sciences, KNAW). DNA isolation for PCR testing was performed using either the DNeasy Blood and Tissue Kit (Qiagen, Hilden, Germany), or NUCLISENS® easyMAG® (Biomerieux, France). For the latter, material from swabs was suspended in PBS from which 500 microliter was added to 2 ml lysis buffer. Whenever isolates were available, about 10 microliter from a fresh culture (BSL3) was suspended in 600 microliter lysis buffer, followed by an inactivation step in a heating block at 80°C during 5 min. After the lysis step, the material was further processed under BSL2 conditions in the easyMAG.
For the identification of subclades of F. tularensis a hierarchical real-time PCR typing scheme based on binary output of PCR results targeting insertion/deletions and SNPs was used (Larsson et al., 2007; Svensson et al., 2009; Larkeryd et al., 2014). This assay can be used on DNA obtained from clinical samples routinely processed without the need for pure cultures of the bacteria.
In four cases, strains were available for whole genome sequencing. The genotyping of the 2011 patient has been described in detail elsewhere (Coolen et al., 2013; Maraha et al., 2013). For the remaining strains, DNA was isolated using a Gentra Puregene kit (QIAGEN GmbH, Germany), and whole-genome sequencing was performed using TruSeq library based paired-end 250 bp sequencing (Illumina MiSeq, San Diego, CA, USA). Demultiplexing of the data was performed with standard Illumina software from the casave pipeline with default settings. The quality of sequence reads was analyzed using FastQC tool (v0.11.5). All artifacts were polished from the Illumina short reads using the BBMap suite (37.66). Draft de novo genome assemblies were created using SPAdes (version 3.10.0; Bankevich et al., 2012) on BBduk adapter-clipped and quality trimmed data (>Q20; BBMap v39.92-Bushnell B. -https://sourceforge.net/projects/bbmap/). Strain typing was performed using CanSNPer on the draft genome assemblies (Larkeryd et al., 2014). Sequence data have been submitted to the European Nucleotide Archive under study number PRJEB27514 and accession numbers ERS2585217, ERS2585218, and ERS2585220 as shown in the Supplementary Table.
Statistical Analyses
To investigate whether the odds of hepatitis, splenitis, pneumonia or adrenalitis were greater in the B.6 genotype than in the B.12 genotype, one-sided Fisher-exact tests were performed in R (R Core Team, 2013). R: A language and environment for statistical computing. R Foundation for Statistical Computing, Vienna, Austria).
Results
Human Cases of Tularemia in the Netherlands
Genotyping results for seven reported cases in humans are summarized in Table 1. All patients included in this study presented with the ulceroglandular or glandular form of tularemia. Based on patient interviews, the most likely locations of infection are shown in Figure 2.
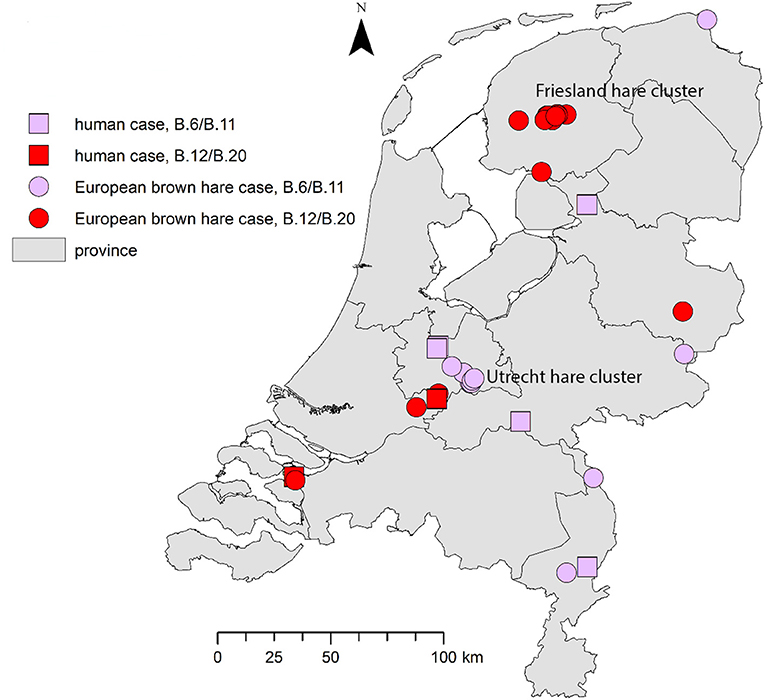
Figure 2. Geographical distribution of tularemia cases in humans and hares. For the human cases the most likely location of infection was determined based on patient interviews by local health authorities. The proven hare-to-human case is shown in the south-west.
F. tularensis subsp. holarctica strains from five patients were assigned to basal clade B.6, the remaining two cases the strains were assigned to B.12 (Table 1). Four of the B.6 strains were further genotyped to subclade B.11 (also referred to as the Iberian clone; B.Br.FTNF002-00), which is typical for Western Europe. No subtyping was performed for the remaining B.6. strain. The two B.12 strains were shown to be members of subclade B.20 (also called B.42), a canSNP group common in Scandinavia, Central and Eastern Europe (Table 1). In one of the B.12 cases an isolate was available for WGS and using the CanSNPer pipeline was found to belong to B.33 which is a subgroup of B.20.
Two of the seven human tularemia cases described in this paper occurred after direct contact with infected hares. In one case, infection was acquired through insect bites in the same area and period, as where and when an infected hare had been found (Table 1; Leenders et al., 2015).
European Brown Hare Cases of Tularemia in the Netherlands
Genotyping results of the 29 European brown hares showed that 12 animals were infected with clade B.6 strains and 17 animals with clade B.12 strains (Table 2). For the B.6 cases, one strain could be further assigned to subclade B.11, while in 13 strains of clade B.12 further subtyping to subclade B.20 was achieved.
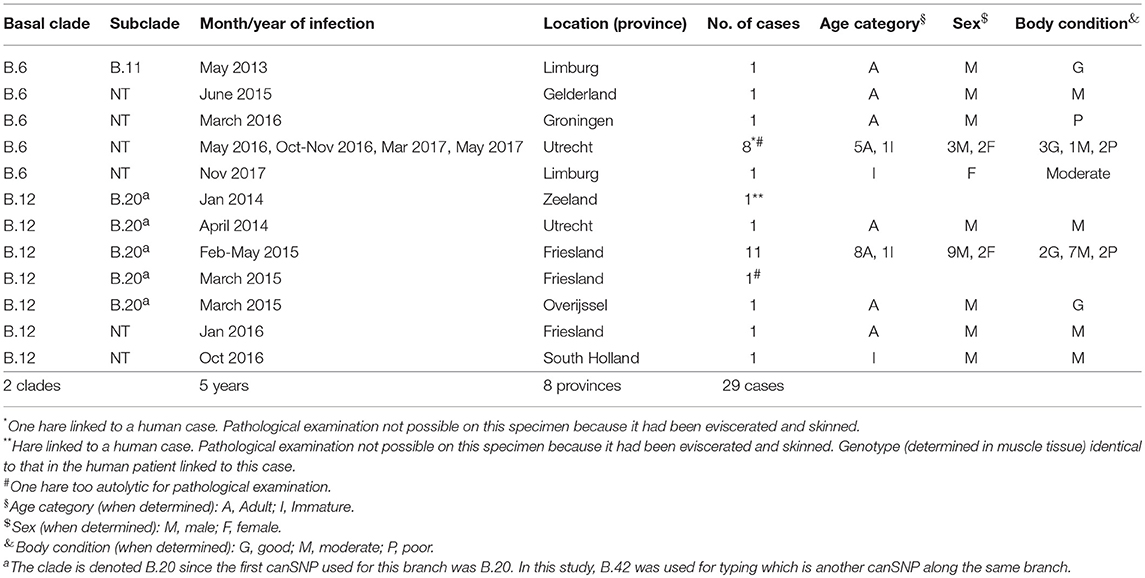
Table 2. Spatiotemporal and host features of European brown hare tularemia cases per Francisella tularensis subspecies holartica genotype.
Both clades are widespread over the country: the B.6 and B.12 cases have been detected in four and five of the 12 Dutch provinces, respectively. Both genotypes were found in the province of Utrecht (Table 2; Figure 2). A number of the European brown hare cases included in this study were temporally and geographically linked; eight of the B.6 cases were found in a region of 2.5 by 12.5 km in direct distance of a meandering riverbed in the period between May 2016 and May 2017. For B.12, 11 hares were part of an outbreak of tularemia that lasted from February to May 2015 in several adjacent municipalities in the province of Friesland (Janse et al., 2017). Other cases were individual submissions belonging to distinct events in space and time (Table 2; Figure 2).
The main reason why hares were submitted to the surveillance program was the finding of multiple dead hares in a restricted area within a short time frame (nine out of 11 B.6 cases; 14 out of 16 B.12 cases). Other reasons were atypical behavior prior to death, such as unusual movement patterns (e.g., a swaying gait), falling over, excessive digging or a freezing state in between bouts of hopping (n = 6); lack of fear (n = 5); or death in an unusual place, interpreted by submitters as lack of hiding behavior (n = 6). In four events, no other dead hares were observed, but there had been atypical behavior (1/11 B.6; 1/16 B.12), a poor general body condition (1/16 B.12), or on the contrary a surprisingly good general body condition (1/11 B.6). Scavengers such as crows were occasionally reported to have been pecking at the carcasses. No information was available on the history of the two hares linked to the human cases.
Pathological investigations were performed on 25 European brown hares, 10 infected with B.6 and 15 with B.12. In both groups, the submitted animals were mainly adult males, with a moderate to good body condition (Table 2). Poor body condition was observed only in 3/10 (30%) of the B.6 cases and 2/15 (13%) of the B.12 cases (Table 2).
Macroscopically, lungs, trachea, spleen, and liver were the organs most affected (Table 3). In the lungs, hemorrhages, hyperemia, edema, emphysema or a combination of these were noticeable in all B.6 cases and in 11/15 (73%) of the B.12 cases. In both groups, the tracheal mucosa was frequently hyperemic, and the lumen sometimes contained blood clots. The spleen was enlarged in all B.6 cases, and in two-thirds of the B.12 cases. Liver lesions like reduced consistency, enlargement, hyperemia, beige coloring, dullness, or a combination of these were observed in 7/10 (70%) of the B.6 cases, and in 11/14 (78%) of the B.12 cases. Single or multiple white or yellowish foci, ranging in size from 1 to ~7 mm in diameter, were observed in seven hares in various organs: the foci were detected in lungs (2 B.6; 1 B.12), liver (1 B.6; 1 B.12), mediastinum (1 B.12), or abdominal serosa (1 B.6). In both groups, the stomach was generally well or moderately filled with food. Enlarged lymph nodes were not recorded, apart from an enlarged mesenteric lymph node in one B.12 case. Lesions suggestive of trauma, such as broken skin or bones, picked eyes and intracranial or neck hemorrhages were observed in 6/10 (60%) B.6 cases, and 8/15 (53%) B.12 cases. Incidental lesions consisted of poor dentition (n = 2), icterus, and a kidney with beige firm areas (amyloidosis).
Histologically, necrotizing lesions and hemorrhages predominated (Table 3). Severe (sub)acute necrotizing hepatitis and splenitis were the most common lesions. Necrotizing hepatitis was observed in 10/10 (100%) of the B.6 cases and 14/15 (93%) of the B.12 cases (Figure 3). Splenitis was observed in 9/10 (90%) of the B.6 cases and 9/13 (69%) of the B.12 cases (Figure 4). Pneumonia, mostly necrotizing, also commonly occurred in the B.6 cases (6/10, 60%), but rarely in the B.12 cases (2/15, 13%). The odds for the occurrence of pneumonia were significantly greater in the B.6 group than in the B.12 group (Fisher exact test; p = 0.02), but this was not the case for hepatitis (Fisher exact test; p = 0.60), splenitis (Fisher exact test; p = 0.25) or adrenalitis (Fisher exact test; p = 0.32). Necrotizing lesions and hemorrhages were observed to a lesser extent in adrenal glands, brain, lymph nodes, and serosa. Necrotizing adrenalitis was observed in 4/9 (44%) of the B.6 cases, and 3/12 (25%) of the B.12 cases. One B.6 case had a severe necrotizing meningoencephalitis. Lymph nodes and serosa were not systematically sampled, but five cases of necrotizing lymphadenitis (three B.6 cases, two B.12 cases) and two cases of B.12 serositis were identified. Multiple organs, also those without necrotizing lesions or hemorrhages, showed hyperemia. Granulomatous lesions were rare (Table 3).
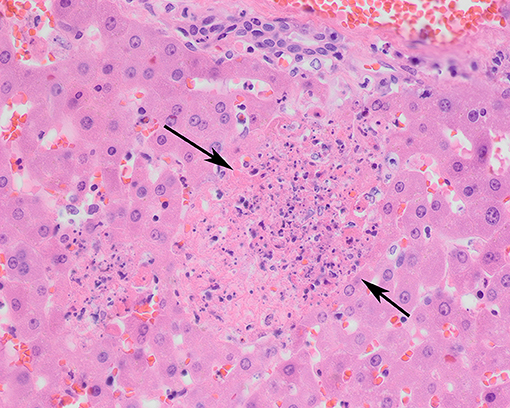
Figure 3. Liver of a tularemia positive hare showing multiple foci of hepatocellular necrosis (arrows). Hematoxylin and eosin stain, magnification x 40.
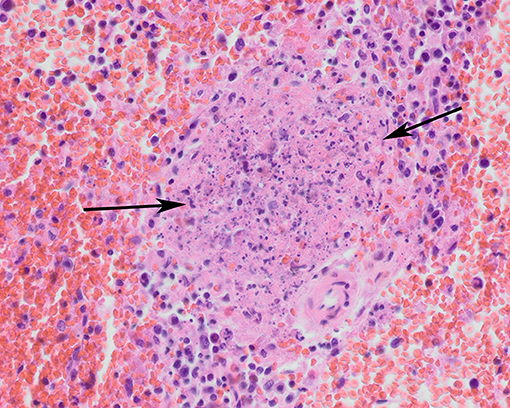
Figure 4. Spleen of a tularemia positive hare showing multiple foci of necrosis (arrows). Hematoxylin and eosin stain, magnification x 40.
Discussion and Conclusions
Genotyping Results and Geographic Distribution
In the Netherlands two different clades of F. tularensis subsp. holarctica, B.6 and B.12, have been found both in humans and in European brown hares. Strains that could be typed in more detail were found to be members of either B.6 subclade B.11 (five human cases and one hare case) or B.12 subclade B.20 (two human cases and 13 hare cases). One isolate of the B.20 subclade could be further typed as B.33 using the CanSNPer platform. As shown in Figure 2, tularemia is widely dispersed over the country, with cases reported from eight of the 12 Dutch provinces.
In Europe, a clear geographic distribution is obvious for the two main basal clades with the B.12 present in the east of Europe and the B.6 in the west (Gyuranecz et al., 2012; Dwibedi et al., 2016), but regional dispersal occurs also within countries. In Germany, several studies showed that both basal clades are present, with considerable genetic diversity (Schulze et al., 2016). Again, B.12 is found in the east of Germany, and only in a few cases in the north-west of the province of Lower Saxony, while clade B.6 is present in the west of Germany (Muller et al., 2013). In Switzerland both clades are circulating as well, with a predominance of the B.6 subclade B.11 (Dwibedi et al., 2016; Origgi and Pilo, 2016; Wittwer et al., 2018). One B.12 strain in our study was assigned to the B.33 subclade, which has also been reported in Germany, Austria, Hungary and Scandinavia (Gyuranecz et al., 2012; Schulze et al., 2016).
In the Netherlands, the spatiotemporal distribution of the human and hare cases in each basal clade group supports the occurrence of local phylogeographic patterns, as were observed in Sweden (Karlsson et al., 2013). Sequence based data combined with anecdotal information showed that clade B.6 may have been introduced in Europe in the 1930s (Jusatz, 1952; Dwibedi et al., 2016). It is likely that this also included the south of the Netherlands, where the first tularemia cases were reported in 1953. We can only speculate whether F. tularensis has been present undetected in the Netherlands in the period in-between or a recent reintroduction has occurred. Indirect support to the former notion comes from recent detection of clade B.12 and clade B.6 in environmental waters in connection with the previously described hare outbreaks in the Netherlands (Janse et al., 2017, 2018). The persistence in water is largely unknown but older literature and more recent research indicate the possibility of prolonged persistence in water courses particularly in endemic regions (Parker et al., 1951; Pomanskaia, 1957; Broman et al., 2011). Thus, it cannot be excluded that the bacterium has been circulating unnoticed in the Netherlands, sustained in the water environment by irregular enzootic amplification cycles in local wildlife or survived during inter-epizootic periods associated with some yet-unknown reservoirs. Alternatively, the current increase in tularemia cases may be the result of a recent reintroduction of the bacterium from neighboring countries.
It is postulated that the persistence and spread of a strain in a certain area, i.e., the occurrence of local phylogeographic patterns, may be associated with strain differences in infectivity and pathogenicity for host species, in combination with ecological differences, i.e., variation in local landscape conditions and the relative abundance of different suitable host vectors (Karlsson et al., 2013; Maurin and Gyuranecz, 2016). This would be an interesting subject for further studies using higher resolution genotyping that also include samples from water and additional wildlife species. Events with grouped hare cases, as observed in Utrecht from May 2016 to May 2017 for the B.6 subclade B.11 and in Friesland from February to May 2015 for the B.12 subclade B.20, will provide good opportunities for this.
Pathology Findings Per Genotype in European Brown Hares
The European brown hare tularemia cases examined in this study generally had severe (sub-)acute necrotizing lesions and hemorrhages in multiple organs. The distribution of the lesions is consistent with disseminated disease or septic shock. In other studies of tularemia in European brown hares, the association of F. tularensis with lesions was confirmed through culture, PCR-tests and immunohistochemistry of multiple organs (Gyuranecz et al., 2010; Origgi and Pilo, 2016; Hestvik et al., 2017). In this study, diagnostic PCR-tests were performed on lung, liver and spleen tissue and in all cases these three tissues were highly positive (data not shown), which is consistent with disseminated F. tularensis infection. The disease course was probably rapid because, unlike in other countries with surveillance programs (Gyuranecz et al., 2010; Origgi and Pilo, 2016; Hestvik et al., 2017), granulomatous and necrotizing granulomatous lesions were rare. The pathology findings support high susceptibility of the affected hares to the disease.
Genotype-associated differences in pathology in the European brown hare have previously been suggested and discussed in the light of pathogenicity and route of infection (Origgi and Pilo, 2016). In the Netherlands, so far there is no evidence for a difference in pathology between the two genotypes present in hares, except for a significantly higher number of pneumonia cases in the B.6 compared to the B.12 clade. There could be a trend toward more necrotizing lesions in the B.6 compared to the B.12 cases, but more data are required to substantiate this.
Hemorrhages were a prominent finding in hare cases. Some of these could be attributed to trauma, which was observed in five B.6 and seven B.12 cases. It is likely that the unusual behavior of the hare promoted the occurrence of scavenger attacks or even self-inflicted trauma, because only a fraction of the traumatic lesions corresponded to lesions consistent with the hare being put down for humane reasons by the submitter. Other hemorrhages, often visible only microscopically, were most likely F. tularensis disease-related lesions. The occurrence of hemorrhages in European brown hare tularemia cases has been previously described (Origgi and Pilo, 2016).
Conclusion
This study shows that F. tularensis subsp. holarctica is endemic and widely distributed in the Netherlands. It is represented by the two basal clades present in Europe, with only slight differences in pathology in European brown hares that were found dead. The main lesions observed in hares for both B.6 cases and B.12 cases were severe (sub-)acute necrotizing hepatitis and splenitis as well as pneumonia in B.6 cases.
The results reported in this study greatly benefitted from the close collaboration between medical, veterinary and wildlife professionals in surveillance, communication and source tracing of F. tularensis cases. Continuation of this One Health approach will help improve insight in how the genetic diversity and phylogeny of F. tularensis evolves in the Netherlands and in Europe.
Author Contributions
JR and MiK equally attributed to the writing of the manuscript. AG, H-JR, JR, MiK, and MM contributed to conception and design of the study. JI and MaK performed the post-mortem examinations. DN, ME, MiK, RD, HeB, HaB, SS, and RP were involved in the diagnostic testing. KM, MF, MG, and RR were involved in the genotyping. JR and MaK summarized the pathological findings. EF, JR, MiK, PT, MM, MS, MV, and RP organized and managed the information in relevant databases. JR constructed Figure 2. All authors contributed to manuscript revision, read and approved the submitted version.
Funding
The work at WBVR was financed by the Dutch Ministry of Agriculture, Nature and Food quality (WOT-01-002-005.02 project). The DWHC is financed by the Dutch Ministry of Agriculture, Nature and Food quality, the Dutch Ministry of Health, Welfare and Sport, and the Faculty of Veterinary Medicine of the University of Utrecht. Work at National Institute for Public Health and the Environment (RIVM) was financed by the Dutch Ministry of Health, Welfare and Sport.
Conflict of Interest Statement
The authors declare that the research was conducted in the absence of any commercial or financial relationships that could be construed as a potential conflict of interest.
Acknowledgments
Natashja Buijs for technical assistance. The following medical microbiological laboratories for submitting diagnostic material or strains and clinical, and epidemiological information: RLM Dordrecht and Gorinchem, Stichting Medische Microbiologie, Roosendaal, Diakonessenhuis Utrecht and Jeroen Bosch Ziekenhuis, Den Bosch. Frans Reubsaet, Amber Hendriks, Maaike van den Beld, Airien Harpal, and Dieneke Hoeve for assistance in confirmatory laboratory diagnosis and Frank Harders, Albert de Boer for sequencing and CanSNPer analyses.
Supplementary Material
The Supplementary Material for this article can be found online at: https://www.frontiersin.org/articles/10.3389/fcimb.2019.00011/full#supplementary-material
References
Afset, J. E., Larssen, K. W., Bergh, K., Larkeryd, A., Sjodin, A., Johansson, A., et al. (2015). Phylogeographical pattern of Francisella tularensis in a nationwide outbreak of tularaemia in Norway, 2011. Euro Surveill. 20, 9–14. doi: 10.2807/1560-7917.ES2015.20.19.21125
Bankevich, A., Nurk, S., Antipov, D., Gurevich, A. A., Dvorkin, M., Kulikov, A. S., et al. (2012). SPAdes: a new genome assembly algorithm and its applications to single-cell sequencing. J. Comput. Biol. 19, 455–477. doi: 10.1089/cmb.2012.0021
Bolhuis, H., Wolters, M., Rijks, J. M., Kik, M. J. L., Koene, M., Oepst, G. et al. (2017). “Management of a tularaemia epizootic in red tailed quenon (cercopithecuss ascanius),” in Proceedings of the 8th Joint conference European Association of Zoo- and Wildlife Veterinarians (EAZWV)/Leibniz Institute for Zoo and Wildlife Research (IZW) (Berlin).
Broman, T., Thelaus, J., Andersson, A. C., Backman, S., Wikstrom, P., Larsson, E., et al. (2011). Molecular detection of persistent francisella tularensis subspecies holarctica in natural waters. Int. J. Microbiol. 2011:851946. doi: 10.1155/2011/851946
Chanturia, G., Birdsell, D. N., Kekelidze, M., Zhgenti, E., Babuadze, G., Tsertsvadze, N., et al. (2011). Phylogeography of Francisella tularensis subspecies holarctica from the country of Georgia. BMC Microbiol. 11:139. doi: 10.1186/1471-2180-11-139
Coolen, J. P., Sjodin, A., Maraha, B., Hajer, G. F., Forsman, M., Verspui, E., et al. (2013). Draft genome sequence of Francisella tularensis subsp. holarctica BD11-00177. Stand. Genomic Sci. 8, 539–547. doi: 10.4056/sigs.4217923
Dempsey, M. P., Dobson, M., Zhang, C., Zhang, M., Lion, C., Gutierrez-Martin, C. B., et al. (2007). Genomic deletion marking an emerging subclone of Francisella tularensis subsp. holarctica in Fra nce and the Iberian Peninsula. Appl. Environ. Microbiol. 73, 7465–7470. doi: 10.1128/AEM.00646-07
Dwibedi, C., Birdsell, D., Larkeryd, A., Myrtennas, K., Ohrman, C., Nilsson, E., et al. (2016). Long-range dispersal moved Francisella tularensis into Western Europe from the East. Microb. Genom. 2:e000100. doi: 10.1099/mgen.0.000100
Gyuranecz, M., Birdsell, D. N., Splettstoesser, W., Seibold, E., Beckstrom-Sternberg, S. M., Makrai, L., et al. (2012). Phylogeography of Francisella tularensis subsp. holarctica, Europe. Emerg. Infect. Dis. 18, 290–293. doi: 10.3201/eid1802.111305
Gyuranecz, M., Szeredi, L., Makrai, L., Fodor, L., Meszaros, A. R., Szepe, B., et al. (2010). Tularemia of European brown hare (Lepus europaeus): a pathological, histopathological, and immunohistochemical study. Vet. Pathol. 47, 958–963. doi: 10.1177/0300985810369902
Hestvik, G., Uhlhorn, H., Sodersten, F., Akerstrom, S., Karlsson, E., Westergren, E., et al. (2017). Tularaemia in European brown hares (Lepus europaeus) and Mountain Hares (Lepus timidus) characterized by histopathology and immunohistochemistry: organ lesions and suggestions of routes of infection and shedding. J. Comp. Pathol. 157, 103–114. doi: 10.1016/j.jcpa.2017.06.003
Janse, I., Maas, M., Rijks, J. M., Koene, M. R., van der Plaats, Q., Engelsma, M. P., et al. (2017). Environmental surveillance during an outbreak of tularaemia in hares, the Netherlands, 2015. Euro Surveill. 22:30607. doi: 10.2807/1560-7917.ES.2017.22.35.30607
Janse, I. R. J., van der Plaats, A. M., de Roda Husman, and van Passel, M. W. J. (2018). Environmental surveillance of zoonotic francisella tularensis in the netherlands. Front. Cell. Infect. Microbiol. 8:140. doi: 10.3389/fcimb.2018.00140
Johansson, A., Farlow, J., Larsson, P., Dukerich, M., Chambers, E., Bystrom, M., et al. (2004). Worldwide genetic relationships among Francisella tularensis isolates determined by multiple-locus variable-number tandem repeat analysis. J. Bacteriol. 186, 5808–5818. doi: 10.1128/JB.186.17.5808-5818.2004
Jusatz, H. J. (1952). [Second report on the propagation of tularemia into middle and western Europe in present time; geomedical investigations on the development in the last decade and epidemiological prognosis]. Z. Hyg. Infektionskr. 134, 350–374.
Karlsson, E., Golovliov, I., Larkeryd, A., Granberg, M., Larsson, E., Ohrman, C., et al. (2016). Clonality of erythromycin resistance in Francisella tularensis. J. Antimicrob. Chemother. 71, 2815–2823. doi: 10.1093/jac/dkw235
Karlsson, E., Svensson, K., Lindgren, P., Bystrom, M., Sjodin, A., Forsman, M., et al. (2013). The phylogeographic pattern of Francisella tularensis in Sweden indicates a scandinavian origin of Eurosiberian tularaemia. Environ. Microbiol. 15, 634–645. doi: 10.1111/1462-2920.12052
Keim, P., Johansson, A., and Wagner, D. M. (2007). Molecular epidemiology, evolution, and ecology of Francisella. Ann. N. Y. Acad. Sci. 1105, 30–66. doi: 10.1196/annals.1409.011
Kreizinger, Z., Erdelyi, K., Felde, O., Fabbi, M., Sulyok, K. M., Magyar, T., et al. (2017). Comparison of virulence of Francisella tularensis ssp. holarctica genotypes B.12 and B.FTNF002-00. BMC Vet. Res. 13:46. doi: 10.1186/s12917-017-0968-9
Kudelina, R. I., and Olsufiev, N. G. (1980). Sensitivity to macrolide antibiotics and lincomycin in Francisella tularensis holarctica. J. Hyg. Epidemiol. Microbiol. Immunol. 24, 84–91.
Larkeryd, A., Myrtennas, K., Karlsson, E., Dwibedi, C. K., Forsman, M., Larsson, P., et al. (2014). CanSNPer: a hierarchical genotype classifier of clonal pathogens. Bioinformatics 30, 1762–1764. doi: 10.1093/bioinformatics/btu113
Larsson, P., Svensson, K., Karlsson, L., Guala, D., Granberg, M., Forsman, M., et al. (2007). Canonical insertion-deletion markers for rapid DNA typing of Francisella tularensis. Emerging Infect. Dis. 13, 1725–1732. doi: 10.3201/eid1311.070603
Leenders, A. C. A. P. E., Notermans, D. W., Koene, M. G. J., Schimmer, B., Swaan, C. M., and Rietveld, A. (2015). Tularaemia back in the Netherlands after 60 years? The Dutch situation in connection with a tularaemia patient and an infected hare. Tijdschr Infect 10, 194–199.
Lu, Y., Yu, Y., Feng, L., Li, Y., He, J., Zhu, H., et al. (2016). Phylogeography of Francisella tularensis from Tibet, China: evidence for an asian origin and radiation of holarctica-type Tularemia. Ticks Tick Borne Dis. 7, 865–868. doi: 10.1016/j.ttbdis.2016.04.001
Maraha, B., Hajer, G., Sjodin, A., Forsman, M., Paauw, A., Roeselers, G., et al. (2013). Indigenous infection with Francisella tularensis holarctica in The Netherlands. Case Rep. Infect. Dis. 2013:916985. doi: 10.1155/2013/916985
Maurin, M., and Gyuranecz, M. (2016). Tularaemia: clinical aspects in Europe. Lancet Infect. Dis. 16, 113–124. doi: 10.1016/S1473-3099(15)00355-2
Mitchell, J. L., Chatwell, N., Christensen, D., Diaper, H., Minogue, T. D., Parsons, T. M., et al. (2010). Development of real-time PCR assays for the specific detection of Francisella tularensis ssp. tularensis, holarctica and mediaasiatica. Mol. Cell Probes 24, 72–76. doi: 10.1016/j.mcp.2009.10.004
Muller, W., Hotzel, H., Otto, P., Karger, A., Bettin, B., Bocklisch, H., et al. (2013). German Francisella tularensis isolates from European brown hares (Lepus europaeus) reveal genetic and phenotypic diversity. BMC Microbiol. 13:61. doi: 10.1186/1471-2180-13-61
Origgi, F. C., and Pilo, P. (2016). Francisella tularensis clades B.FTN002-00 and B.13 are associated with distinct pathology in the european brown hare (Lepus europaeus). Vet. Pathol. 53, 1220–1232. doi: 10.1177/0300985816629718
Parker, R. R., Steinhaus, E. A., Kohls, G. M., and Jellison, W. L. (1951). Contamination of natural waters and mud with Pasteurella tularensis and tularemia in beavers and muskrats in the northwestern United States. Bull. Natl. Inst. Health 193, 1–161.
Petersen, J. M., and Molins, C. R. (2010). Subpopulations of Francisella tularensis ssp. tularensis and holarctica: identification and associated epidemiology. Future Microbiol. 5, 649–661. doi: 10.2217/fmb.10.17
Pomanskaia, L. A. (1957). [Length of preservation of tularemia bacilli on grain and straw]. Zh. Mikrobiol. Epidemiol. Immunobiol. 28, 133–139.
R Core Team (2013). R: A Language and Environment for Statistical Computing, R Foundation for Statistical Computing. R. version 2013 (Vienna). Available online at: https://www.R-project.org.
Rijks, J. M., Kik, M., Koene, M. G., Engelsma, M. Y., van Tulden, P., Montizaan, M. G., et al. (2013). Tularaemia in a brown hare (Lepus europaeus) in 2013: first case in the Netherlands in 60 years. Euro Surveill. 18:20655. doi: 10.2807/1560-7917.ES2013.18.49.20655
Schulze, C., Heuner, K., Myrtennas, K., Karlsson, E., Jacob, D., Kutzer, P., et al. (2016). High and novel genetic diversity of Francisella tularensis in Germany and indication of environmental persistence. Epidemiol. Infect. 144, 3025–3036. doi: 10.1017/S0950268816001175
Sissonen, S., Rossow, H., Karlsson, E., Hemmila, H., Henttonen, H., Isomursu, M., et al. (2015). Phylogeography of Francisella tularensis subspecies holarctica in Finland, 1993-2011. Infect Dis. 47, 701–706. doi: 10.3109/23744235.2015.1049657
Svensson, K., Granberg, M., Karlsson, L., Neubauerova, V., Forsman, M., and Johansson, A. (2009). A real-time PCR array for hierarchical identification of Francisella isolates. PLoS ONE 4:e8360. doi: 10.1371/journal.pone.0008360
Vogler, A. J., Birdsell, D., Price, L. B., Bowers, J. R., Beckstrom-Sternberg, S. M., Auerbach, R. K., et al. (2009). Phylogeography of Francisella tularensis: global expansion of a highly fit clone. J. Bacteriol. 191, 2474–2484. doi: 10.1128/JB.01786-08
Keywords: Francisella tularensis subspecies holarctica, tularemia, Netherlands, genotyping, human, European brown hare (Lepus europaeus), pathology
Citation: Koene M, Rijks J, Maas M, Ruuls R, Engelsma M, van Tulden P, Kik M, IJzer J, Notermans D, de Vries M, Fanoy E, Pijnacker R, Spierenburg M, Bavelaar H, Berkhout H, Sankatsing S, Diepersloot R, Myrtennas K, Granberg M, Forsman M, Roest H-J and Gröne A (2019) Phylogeographic Distribution of Human and Hare Francisella Tularensis Subsp. Holarctica Strains in the Netherlands and Its Pathology in European Brown Hares (Lepus Europaeus). Front. Cell. Infect. Microbiol. 9:11. doi: 10.3389/fcimb.2019.00011
Received: 09 October 2018; Accepted: 15 January 2019;
Published: 11 February 2019.
Edited by:
Caspar Yvan, Centre Hospitalier Universitaire de Grenoble, FranceReviewed by:
Jean Challacombe, Colorado State University, United StatesMarina Santic', University of Rijeka, Croatia
Copyright © 2019 Koene, Rijks, Maas, Ruuls, Engelsma, van Tulden, Kik, IJzer, Notermans, de Vries, Fanoy, Pijnacker, Spierenburg, Bavelaar, Berkhout, Sankatsing, Diepersloot, Myrtennas, Granberg, Forsman, Roest and Gröne. This is an open-access article distributed under the terms of the Creative Commons Attribution License (CC BY). The use, distribution or reproduction in other forums is permitted, provided the original author(s) and the copyright owner(s) are credited and that the original publication in this journal is cited, in accordance with accepted academic practice. No use, distribution or reproduction is permitted which does not comply with these terms.
*Correspondence: Miriam Koene, bWlyaWFtLmtvZW5lQHd1ci5ubA==
†These authors have contributed equally to this work