- 1Graduate Program in Immunology and Infectious Disease, Pennsylvania State University, University Park, PA, United States
- 2Center for Inflammation, Immunity and Infection, Institute for Biomedical Sciences, Georgia State University, Atlanta, GA, United States
- 3Department of Physiology and Pharmacology, University of Toledo College of Medicine and Life Sciences, Toledo, OH, United States
- 4Department of Medical Microbiology and Immunology, University of Toledo College of Medicine and Life Sciences, Toledo, OH, United States
The host immune system is constantly exposed to diverse microbial ligands, including flagellin (FliC; a ligand for TLR5 and NLRC4) and lipopolysaccharide (LPS; a ligand for TLR4), which could induce immune tolerance to subsequent exposure. Herein, we investigated the extent to which FliC induces self-tolerance in vivo and the role of adaptive immunity in mediating such effect. Mice pre-treated with FliC displayed attenuated serum keratinocyte-derived chemokine (KC), interleukin (IL)-6 and IL-18 responses to secondary challenge of FliC. A negative correlation was observed between high anti-FliC titer and reduced KC, IL-6, and IL-18 responses upon FliC re-challenge in WT mice, but not Rag1KO mice, suggesting that adaptive immunity could tolerize TLR5 and NLRC4. However, administration of LPS during FliC pre-treatment impaired the generation of anti-FliC antibodies and resulted in a partial loss of self-tolerance to FliC re-challenge. These findings may be relevant in the context of bacterial infection, as we observed that anti-FliC response are protective against systemic infection by Salmonella typhimurium. Taken together, our study delineates a distinct co-operative and reciprocal interaction between the innate and adaptive arms of immunity in modulating their responses to a bacterial protein.
Introduction
The microbial biomass in the intestine is enriched with diverse microbial-associated molecular patterns (MAMPs) that are recognizable by host pathogen recognition receptors (PRRs). Upon activation, PRRs such as Toll-like receptors (TLR) initiate rapid secretion of cytokines and chemokines to recruit immune cells to the site of microbial transgression. To deter unwarranted inflammatory responses, the host has evolved built-in mechanisms to promote immunotolerance against repeated exposure to MAMPs (Rakoff-Nahoum et al., 2004). These mechanisms include strategic expression of PRRs (e.g., TLR5) on the basolateral side of the epithelia (Gewirtz et al., 2001a), downregulation of surface PRRs (e.g., TLR2, TLR4, MD-2, TLR5) (Medvedev et al., 2000; Abreu et al., 2001; Otte et al., 2004), and secretion of endogenous inhibitors [e.g., soluble CD14, secretory IL-1 receptor antagonist (sIL1Ra), soluble TNF receptor I and II] (Liew et al., 2005). Flagelin [FliC; a ligand for TLR5 (Yoon et al., 2012) and NAIP5-NLRC4 (Tenthorey et al., 2017)] is the monomeric protein constituent of flagella, a whip-like appendage, which provides motility for bacteria. As one of the few protein ligand for TLRs, FliC is exceptional in activating both innate and adaptive immunity. Studies have documented that FliC can function as vaccine adjuvant (Mizel and Bates, 2010) and serve as a major antigen in Crohn's disease (Lodes et al., 2004). However, little is known with respect to the functional properties of anti-FliC antibodies (Ab) and whether they could modulate TLR5 response. Lipopolysaccharide [LPS; a TLR4 ligand] is a bacterial glycolipid that comprises the outer membrane of Gram-negative bacteria. Pre-exposure of macrophages and epithelial cells to FliC or LPS, has been reported to induce hyporesponsiveness to subsequent exposures in vitro (Medvedev et al., 2000; Otte et al., 2004; Sun et al., 2007). LPS pre-treatment could also regulate TLR5 responses to FliC in vitro, though the magnitude of such “cross-tolerance” depends on the doses and cell-type studied (Mizel and Snipes, 2002; Sun et al., 2007; Li et al., 2012).
The purpose of this study was to determine if pre-treating mice with FliC would blunt the innate immune response to FliC re-challenge. We measured serum keratinocyte-derived chemokine (KC) and interleukin (IL)-6 as putative markers for TLR activation. NAIP5-NLRC4 is a cytosolic receptor for FliC, whose activation induces inflammasome; accordingly, we measured serum IL-18 as the marker for its activation. Additionally, we measured the levels of anti-FliC Ab and investigated whether they have a role in dampening the innate immune response upon subsequent FliC re-challenge. We extend this study also to examine the efficacy of anti-FliC Ab against enteric and systemic infection by Salmonella typhimurium.
Materials and Methods
Reagents
FliC from S. typhimurium (SL3201, fljB-) was purified through sequential cation and anion-exchange chromatography as previously described (Gewirtz et al., 2001b). LPS from Escherichia coli 0128:B12 was purchased from Sigma-Aldrich (St. Louis, MO). Reagents and antibodies for ELISA were purchased from R&D Systems (Minneapolis, MN).
Mice
C57BL/6 WT and Rag1KO mice were procured from Jackson Laboratories (Bar Harbor, ME) and bred under specific pathogen-free condition at Pennsylvania State University (PSU). Mice were housed in cages containing corncob bedding and nestlet, fed ad libitum and maintained at 23°C with a 12 h light/dark phase cycle. Animal experiments were approved by the Institutional Animal Care and Use Committee (IACUC) at PSU.
Flagellin and LPS Challenge
Six-weeks-old male WT mice (n = 4) were treated with PBS, LPS (10 μg), FliC (50 μg), LPS→ FliC, and FliC→ LPS (arrow denoting secondary challenge 3 h after the initial treatment). On day 18, mice were bled minimally and assayed for seroreactivity to FliC and LPS. On day 21, mice were re-challenged with FliC (50 μg) and bled after 2 h. In another experiment, six-weeks-old male Rag1KO mice (n = 4) were treated with PBS or FliC at day 0 and re-challenged with FliC at day 21.
Serum Collection
Blood was collected into BD microtainer (BD Biosciences, San Jose, CA) via non-terminal retro-orbital bleeding. Hemolysis-free sera were obtained after centrifugation and stored at −80°C until further analysis.
Cell Culture
Human colon adenocarcinoma cell line HT29 was cultured on 24-well plates in Dulbecco's modified Eagle medium (DMEM) supplemented with 10% fetal bovine serum (FBS) and 1% penicillin and streptomycin. After cells became confluent, the media was replaced with incomplete DMEM without FBS. Cells were co-treated with PBS or FliC (50 ng/mL) in combination with serum (1:50 dilution) from FliC-treated WT, naïve WT, or FliC-treated Rag1KO mice. Cells were incubated for 5 h at 37°C and 5% CO2. Culture supernatant was collected and stored at −80°C until further analysis.
Enzyme-Linked Immunosorbent Assay (ELISA)
Mouse KC and IL-6, and human IL-8 were analyzed using ELISA kits (R&D Systems) according to the manufacturer's instructions. Mouse IL-18 were analyzed via an in-house ELISA as described previously (Zhang et al., 2014). To measure IgG seroreactivity to FliC and LPS, sera were diluted (1:500) and analyzed via ELISA as described previously (Ziegler et al., 2008).
Bacterial Culture
Salmonella enterica subsp. enterica serovar Typhimurium (strain SL3201) were cultured for 15 h in Luria-Bertani (LB) broth at 37°C with shaking (200 rpm). Bacteria CFU were adjusted based on OD at 600 nm.
Murine Models of Salmonella Infection
Six-weeks-old male WT mice were administered PBS or FliC (50 μg; i.p.) at day 0 and 14. On day 21, mice were infected with Salmonella either orally (1 × 108 CFU; n = 5) or via i.p. (1 × 104 CFU; n = 10) as previously described (Vijay-Kumar et al., 2008a). Mice were monitored for body weight loss and mortality.
Statistical Analysis
Values are presented as mean ± SEM. Data were analyzed using one-way analysis of variance (ANOVA) followed by post-hoc Tukey's multiple comparison test. Spearman correlation was used to establish the association of two variables. Kaplan–Meier survival curves were analyzed with log-rank (Mantel-Cox) test. Data were considered significant at p < 0.05. All analyses were performed using GraphPad Prism 6.0 (La Jolla, CA).
Results
LPS Suppresses the Adaptive Immune Response to Flic in vivo
The immune system would be exposed to multiple TLR ligands, particularly during polymicrobial sepsis. While MAMPs have been shown to induce immune tolerance in vitro (Medvedev et al., 2000; Mizel and Snipes, 2002; Otte et al., 2004; Sun et al., 2007; Li et al., 2012), the occurrence of tolerance is relatively under-studied in vivo (De Vos et al., 2009). First, we estimated the cytokine responses in mice challenged with approximately equimolar concentration of 10 μg LPS (~10 kDa) or 50 μg FliC (~50 kDa). LPS-treated mice displayed ~ 2-fold more increase in serum KC and IL-6 than FliC-treated mice (Figures 1A,B). Such observation is consistent with our previous study demonstrating that LPS is more potent in inducing innate immunity, including TNFα response, than FliC (Vijay-Kumar et al., 2008b).
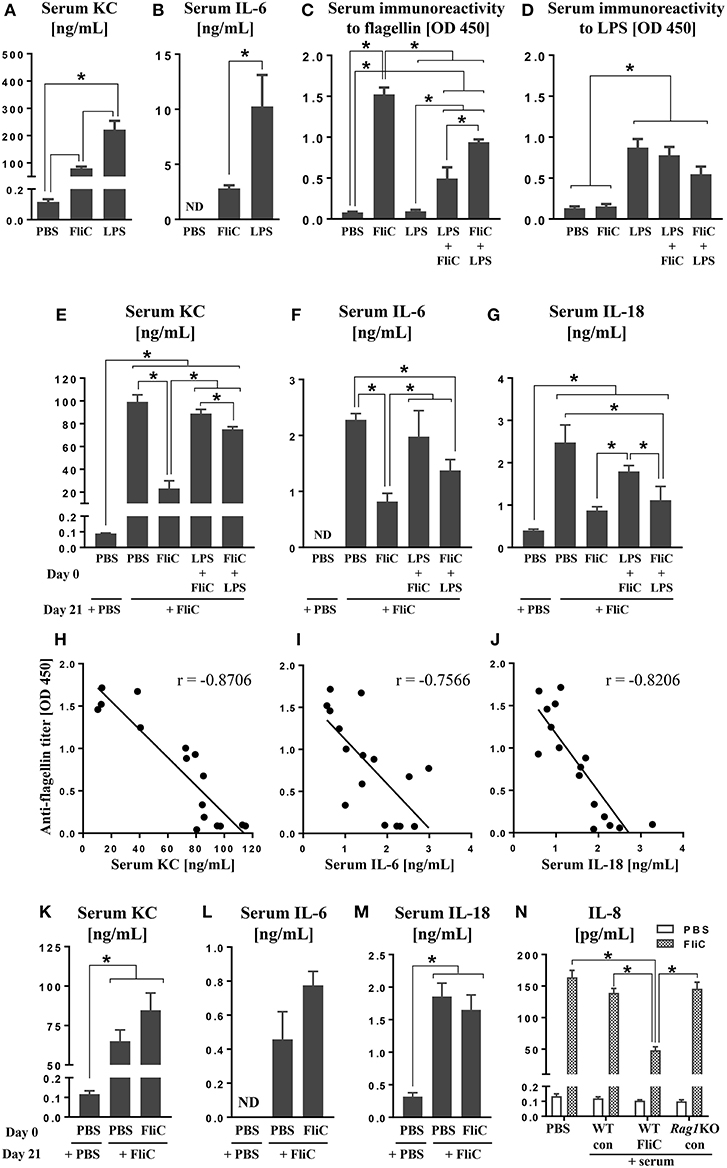
Figure 1. Anti-FliC Ab attenuates TLR5 and NAIP5-NLRC4 cytokine responses to FliC re-challenge. Six week old male WT mice (n = 4) were challenged with either PBS, LPS (10 μg) or FliC (50 μg). Sera were collected after 2 h and analyzed for (A) KC and (B) IL-6. In another experiment, 6 week old WT mice (n = 4) were challenged with PBS, LPS, FliC, LPS→ FliC, FliC→ LPS with the arrow denoting secondary challenge 3 h after the initial treatment. At day 18 post-treatment, sera were collected and measured for (C) seroreactivity to flagellin and (D) seroreactivity to LPS. At day 21 post-treatment, the mice were re-challenged with FliC (50 μg). Sera were collected after 2 h and analyzed for (E) KC, (F) IL-6, and (G) IL-18. Correlations between seroreactivity to FliC against serum (H) KC [Spearman correlation (r = −0.8706; p (two-tailed) < 0.0001)], (I) IL-6 (r = −0.7566; p = 0.0007), (J) IL-18 (r = −0.8206; p = 0.0002). Six week old male Rag1KO mice (n = 4) were pre-treated with either PBS or FliC (50 μg) at day 0. At day 21, mice were challenged with FliC. Sera were collected 2 h post-treatment and analyzed for (K) KC, (L) IL-6, and (M) IL-18. HT29 cells were co-treated with FliC and serum from either FliC-treated WT, naïve WT, or FliC-treated Rag1KO mice. (N) Five-hour culture supernatants were assayed for secretion of IL-8. Results presented as mean ± SEM and are representative of two independent experiments. ND, not detected. Data in (H–J) were analyzed with Spearman correlation test. All other data were analyzed via ANOVA with post-hoc Tukey's test with *p < 0.05.
Studies from our group (Sanders et al., 2006, 2008, 2009; Vijay-Kumar et al., 2010) and others (Letran et al., 2011; Atif et al., 2014; Lopez-Yglesias et al., 2014; Li et al., 2016) demonstrate that TLR5 is required for promoting optimal adaptive responses to FliC. We asked whether such response could be impeded when mice were administered with LPS shortly before or after FliC. Assessment on mice at day 18 post-treatment revealed that their seroreactivity to FliC was in the order of FliC > FliC→ LPS > LPS→ FliC > PBS (Figure 1C). Increased seroreactivity to LPS was also observed in the order of LPS ≥ LPS→ FliC ≥ FliC→ LPS (Figure 1D), albeit not reaching significance, but nonetheless suggest that anti-LPS response could also be disrupted by FliC. The negligible levels of anti-FliC Ab in mice given only LPS, as well as anti-LPS Ab in mice given only FliC, indicated the specificity of the Ab generated (Figures 1C,D).
Anti-FliC Antibodies Dampen the Innate Immune Response to FliC
Next, we investigated whether anti-FliC could modulate TLR5 responses. To address this possibility, we re-challenged the aforementioned FliC/LPS-treated mice on day 21 with FliC. Serum KC, IL-6, and IL-18 induced by FliC were strikingly diminished in FliC pre-treated mice, when compared to naïve mice given FliC for the first time (Figures 1E–G). Mice pre-treated with LPS→ FliC or FliC→ LPS also displayed modest reduction in serum KC, IL-6, and IL-18 following FliC re-challenge (Figures 1E–G). Regression analyses affirmed the strong negative correlations between serum anti-FliC Ab with either KC, IL-6, or IL-18 levels (Figures 1H–J), thus implicating the involvement of adaptive immunity underlying the tolerance to FliC.
Loss of Adaptive Immunity Abrogates Immune Tolerance to FliC Re-challenge
To address whether adaptive immunity is required for the tolerance to FliC, we employed RAG1-deficient (Rag1KO) mice, which lack mature T and B cells and, therefore, are unable to generate any Ab. Unlike WT mice, Rag1KO mice were not refractory to FliC re-challenge (Figures 1K–M) regardless of whether they have or have not been previously exposed to FliC. Such outcomes imply that the tolerance observed in WT mice is likely antibody-dependent. To affirm such notion, we co-treated HT29 cell line (a model intestinal epithelia) with FliC and serum from FliC-treated or untreated WT mice; FliC-treated Rag1KO mice sera was used as negative control. As anticipated, only serum with anti-FliC suppressed FliC-induced secretion of IL-8 (human homolog of KC) from HT29 cells (Figure 1N).
Adaptive Response to FliC Protects Mice Against Salmonella Infection
Having demonstrated the role of anti-FliC in mitigating TLR5 response, we next investigated their physiological significance in a natural infection model of S. typhimurium. Compared to controls, mice pre-treated with FliC displayed a slight trend toward a delayed body weight loss and mortality upon oral infection (Figures 2A,B). Such protection was more pronounced when Salmonella was administered intraperitoneally to mimic systemic infection (Figures 2C,D). These findings suggest that adaptive immunity to FliC are not bystander responses, but may have a role in conferring protection against enteric and systemic infection by Salmonella.
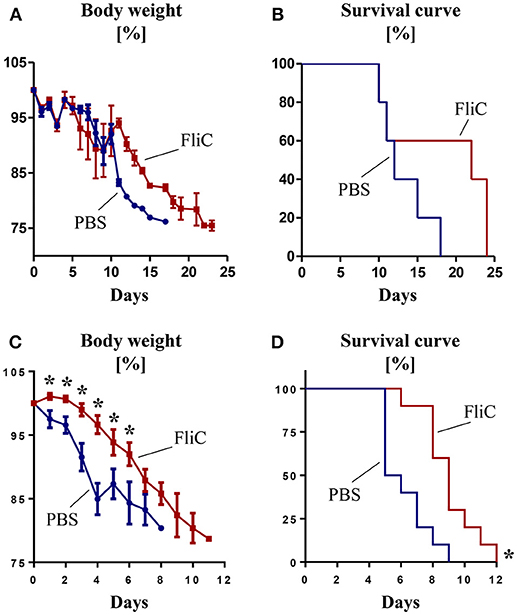
Figure 2. Adaptive immunity to FliC protects mice from Salmonella infection. Six week old male WT mice were treated with FliC (50 μg) on day 0 and 14, and infected with Salmonella typhimurium on day 21. (A) Percent body weight and (B) Kaplan–Meier survival curve for mice receiving oral infection (108 CFU bacteria; n = 10). (C) Percent body weight and (D) Kaplan-Meier survival curve for mice receiving intraperitoneal infection (104 CFU bacteria; n = 10). Results presented as mean ± SEM. Data (A–D) are pooled from two independent experiments. *p < 0.05 (Student's t test) for (A,C); *p < 0.005 [Log-rank (Mantel-Cox) test] for (B,D).
Discussions
Here we delineate a reciprocal interaction between the innate and adaptive immunity in modulating host responses to FliC in vivo. Our results demonstrate that anti-FliC Ab are capable of exerting tolerance to subsequent FliC re-challenge. The attenuation in cytokine responses downstream of TLR5 and NLRC4 could be, in part, due to formation of FliC-Ab complex that may undergo rapid degradation via Fc receptor-mediated phagocytosis. While the binding sites between TLR5 and FliC have been elucidated (Smith et al., 2003), the antibody binding sites to FliC is not clear. Further studies are required to interrogate the antibody isotype variants of anti-FliC Ab, as well as the epitope and paratope interaction between FliC and anti-FliC, respectively. Deciphering such molecular interactions may help in designing anti-FliC Ab with high affinity and avidity for passive immunization to treat flagellated bacterial infections.
Intriguingly, administration of LPS during the initial FliC challenge interfered with the anti-FliC response. As TLR4 and TLR5 share a common signaling pathway (i.e., MyD88) (Mizel and Snipes, 2002; Sato et al., 2002; Otte et al., 2004), it is possible that activation of the former by LPS could have dampened the latter. Such cross-tolerance may explain the suboptimal anti-FliC Ab response that requires proper TLR5 function. LPS could also disrupt proper antigen processing or presentation of FliC, potentially via inducing proteases that degrade FliC or serving as a competing antigen. Despite LPS being regarded as a potent vaccine adjuvant (Coffman et al., 2010), it is interesting to note that it has poor adjuvanicity in potentiating anti-FliC Ab generation.
Though we observed modest improvement in overall survival in FliC-treated mice over naïve mice against Salmonella infection, the extent of protection appears to depend on the route and course of infection. Since oral infection requires the pathogen to first colonize and breach the mucosal barrier, this would provide opportunity for Salmonella to alter FliC expression and thus evade the host innate immune system. Interaction with the host cell membrane lysophospholipid cues Salmonella to secrete FliC monomers (Subramanian and Qadri, 2006), thus activating innate immunity (Gewirtz et al., 2001b) and recruiting macrophages to serve as carriers for dissemination. Upon transitioning from extra- to intra-cellular phase of infection, Salmonella could downregulate their FliC expression (Cummings et al., 2005, 2006; Alaniz et al., 2006). On the flip side, administration of Salmonella directly into systemic circulation renders them more susceptible to anti-FliC Ab since the pathogen may not sufficiently and timely downregulates FliC expression.
Although anti-FliC Ab are integral for immunity against Salmonella, their functional capacities are poorly characterized. It was not until much recently that anti-FliC Ab are shown not to be mere bystanders, but could mediate anti-bacterial activity in humans (Maclennan et al., 2008; Ramachandran et al., 2016). Our present study extends this body of knowledge by elucidating that anti-FliC Ab also counter-regulates innate immunity following repeated exposure to FliC and that this regulation could be hindered by LPS.
Author Contributions
BY performed mouse experiments, in vitro assays and analyzed the results. AG and MV-K assisted with data analysis and provided critical discussions. BY and MV-K wrote the manuscript.
Funding
This work was supported by a National Institutes of Health (NIH) R01 grants to MV-K (DK097865-01A1) and AG (DK061417 and DK083890).
Conflict of Interest Statement
The authors declare that the research was conducted in the absence of any commercial or financial relationships that could be construed as a potential conflict of interest.
Acknowledgments
We thank Dr. Piu Saha, University of Toledo, for technical assistance with cell culture and ELISA.
Abbreviations
Ab, antibodies; FliC, flagellin; IL, interleukin; KC, keratinocyte-derived chemokine; LPS, lipopolysaccharide; MAMPs, microbial-associated molecular patterns; PRRs, pathogen recognition receptors; TLR, Toll-like receptors.
References
Abreu, M. T., Vora, P., Faure, E., Thomas, L. S., Arnold, E. T., and Arditi, M. (2001). Decreased expression of Toll-like receptor-4 and MD-2 correlates with intestinal epithelial cell protection against dysregulated proinflammatory gene expression in response to bacterial lipopolysaccharide. J. Immunol. 167, 1609–1616. doi: 10.4049/jimmunol.167.3.1609
Alaniz, R. C., Cummings, L. A., Bergman, M. A., Rassoulian-Barrett, S. L., and Cookson, B. T. (2006). Salmonella typhimurium coordinately regulates FliC location and reduces dendritic cell activation and antigen presentation to CD4+ T cells. J. Immunol. 177, 3983–3993. doi: 10.4049/jimmunol.177.6.3983
Atif, S. M., Uematsu, S., Akira, S., and McSorley, S. J. (2014). CD103-CD11b+ dendritic cells regulate the sensitivity of CD4 T-cell responses to bacterial flagellin. Mucosal Immunol. 7, 68–77. doi: 10.1038/mi.2013.25
Coffman, R. L., Sher, A., and Seder, R. A. (2010). Vaccine adjuvants: putting innate immunity to work. Immunity 33, 492–503. doi: 10.1016/j.immuni.2010.10.002
Cummings, L. A., Barrett, S. L., Wilkerson, W. D., Fellnerova, I., and Cookson, B. T. (2005). FliC-specific CD4+ T cell responses are restricted by bacterial regulation of antigen expression. J. Immunol. 174, 7929–7938. doi: 10.4049/jimmunol.174.12.7929
Cummings, L. A., Wilkerson, W. D., Bergsbaken, T., and Cookson, B. T. (2006). In vivo, fliC expression by Salmonella enterica serovar Typhimurium is heterogeneous, regulated by ClpX, and anatomically restricted. Mol. Microbiol. 61, 795–809. doi: 10.1111/j.1365-2958.2006.05271.x
De Vos, A. F., Pater, J. M., Van Den Pangaart, P. S., De Kruif, M. D., Van 'T Veer, C., and Van Der Poll, T. (2009). In vivo lipopolysaccharide exposure of human blood leukocytes induces cross-tolerance to multiple TLR ligands. J. Immunol. 183, 533–542. doi: 10.4049/jimmunol.0802189
Gewirtz, A. T., Navas, T. A., Lyons, S., Godowski, P. J., and Madara, J. L. (2001a). Cutting edge: bacterial flagellin activates basolaterally expressed TLR5 to induce epithelial proinflammatory gene expression. J. Immunol. 167, 1882–1885. doi: 10.4049/jimmunol.167.4.1882
Gewirtz, A. T., Simon, P. O, Jr., Schmitt, C. K., Taylor, L. J., Hagedorn, C. H., O'brien, A. D., et al. (2001b). Salmonella typhimurium translocates flagellin across intestinal epithelia, inducing a proinflammatory response. J. Clin. Invest. 107, 99–109. doi: 10.1172/JCI10501
Letran, S. E., Lee, S. J., Atif, S. M., Uematsu, S., Akira, S., and Mcsorley, S. J. (2011). TLR5 functions as an endocytic receptor to enhance flagellin-specific adaptive immunity. Eur. J. Immunol. 41, 29–38. doi: 10.1002/eji.201040717
Li, N., Quidgley, M. C., Kobeissy, F. H., Joseph, J., and Neu, J. (2012). Microbial cell components induced tolerance to flagellin-stimulated inflammation through Toll-like receptor pathways in intestinal epithelial cells. Cytokine 60, 806–811. doi: 10.1016/j.cyto.2012.08.003
Li, W., Yang, J., Zhang, E., Zhong, M., Xiao, Y., Yu, J., et al. (2016). Activation of NLRC4 downregulates TLR5-mediated antibody immune responses against flagellin. Cell. Mol. Immunol. 13, 514–523. doi: 10.1038/cmi.2015.33
Liew, F. Y., Xu, D., Brint, E. K., and O'neill, L. A. (2005). Negative regulation of toll-like receptor-mediated immune responses. Nat. Rev. Immunol. 5, 446–458. doi: 10.1038/nri1630
Lodes, M. J., Cong, Y., Elson, C. O., Mohamath, R., Landers, C. J., Targan, S. R., et al. (2004). Bacterial flagellin is a dominant antigen in Crohn disease. J. Clin. Invest. 113, 1296–1306. doi: 10.1172/JCI200420295
Lopez-Yglesias, A. H., Zhao, X., Quarles, E. K., Lai, M. A., Vandenbos, T., Strong, R. K., et al. (2014). Flagellin induces antibody responses through a TLR5- and inflammasome-independent pathway. J. Immunol. 192, 1587–1596. doi: 10.4049/jimmunol.1301893
Maclennan, C. A., Gondwe, E. N., Msefula, C. L., Kingsley, R. A., Thomson, N. R., White, S. A., et al. (2008). The neglected role of antibody in protection against bacteremia caused by nontyphoidal strains of Salmonella in African children. J. Clin. Invest. 118, 1553–1562. doi: 10.1172/JCI33998
Medvedev, A. E., Kopydlowski, K. M., and Vogel, S. N. (2000). Inhibition of lipopolysaccharide-induced signal transduction in endotoxin-tolerized mouse macrophages: dysregulation of cytokine, chemokine, and toll-like receptor 2 and 4 gene expression. J. Immunol. 164, 5564–5574. doi: 10.4049/jimmunol.164.11.5564
Mizel, S. B., and Bates, J. T. (2010). Flagellin as an adjuvant: cellular mechanisms and potential. J. Immunol. 185, 5677–5682. doi: 10.4049/jimmunol.1002156
Mizel, S. B., and Snipes, J. A. (2002). Gram-negative flagellin-induced self-tolerance is associated with a block in interleukin-1 receptor-associated kinase release from toll-like receptor 5. J. Biol. Chem. 277, 22414–22420. doi: 10.1074/jbc.M201762200
Otte, J. M., Cario, E., and Podolsky, D. K. (2004). Mechanisms of cross hyporesponsiveness to Toll-like receptor bacterial ligands in intestinal epithelial cells. Gastroenterology 126, 1054–1070. doi: 10.1053/j.gastro.2004.01.007
Rakoff-Nahoum, S., Paglino, J., Eslami-Varzaneh, F., Edberg, S., and Medzhitov, R. (2004). Recognition of commensal microflora by toll-like receptors is required for intestinal homeostasis. Cell 118, 229–241. doi: 10.1016/j.cell.2004.07.002
Ramachandran, G., Tennant, S. M., Boyd, M. A., Wang, J. Y., Tulapurkar, M. E., Pasetti, M. F., et al. (2016). Functional activity of antibodies directed towards flagellin Proteins of non-typhoidal Salmonella. PLoS ONE 11:e0151875. doi: 10.1371/journal.pone.0151875
Sanders, C. J., Franchi, L., Yarovinsky, F., Uematsu, S., Akira, S., Nunez, G., et al. (2009). Induction of adaptive immunity by flagellin does not require robust activation of innate immunity. Eur. J. Immunol. 39, 359–371. doi: 10.1002/eji.200838804
Sanders, C. J., Moore, D. A. III., Williams, I. R., and Gewirtz, A. T. (2008). Both radioresistant and hemopoietic cells promote innate and adaptive immune responses to flagellin. J. Immunol. 180, 7184–7192. doi: 10.4049/jimmunol.180.11.7184
Sanders, C. J., Yu, Y., Moore, D. A. III, Williams, I. R., and Gewirtz, A. T. (2006). Humoral immune response to flagellin requires T cells and activation of innate immunity. J. Immunol. 177, 2810–2818. doi: 10.4049/jimmunol.177.5.2810
Sato, S., Takeuchi, O., Fujita, T., Tomizawa, H., Takeda, K., and Akira, S. (2002). A variety of microbial components induce tolerance to lipopolysaccharide by differentially affecting MyD88-dependent and -independent pathways. Int. Immunol. 14, 783–791. doi: 10.1093/intimm/dxf046
Smith, K. D., Andersen-Nissen, E., Hayashi, F., Strobe, K., Bergman, M. A., Barrett, S. L., et al. (2003). Toll-like receptor 5 recognizes a conserved site on flagellin required for protofilament formation and bacterial motility. Nat. Immunol. 4, 1247–1253. doi: 10.1038/ni1011
Subramanian, N., and Qadri, A. (2006). Lysophospholipid sensing triggers secretion of flagellin from pathogenic salmonella. Nat. Immunol. 7, 583–589. doi: 10.1038/ni1336
Sun, J., Fegan, P. E., Desai, A. S., Madara, J. L., and Hobert, M. E. (2007). Flagellin-induced tolerance of the Toll-like receptor 5 signaling pathway in polarized intestinal epithelial cells. Am. J. Physiol. Gastrointest. Liver Physiol. 292, G767–G778. doi: 10.1152/ajpgi.00447.2006
Tenthorey, J. L., Haloupek, N., Lopez-Blanco, J. R., Grob, P., Adamson, E., Hartenian, E., et al. (2017). The structural basis of flagellin detection by NAIP5: a strategy to limit pathogen immune evasion. Science 358, 888–893. doi: 10.1126/science.aao1140
Vijay-Kumar, M., Aitken, J. D., Kumar, A., Neish, A. S., Uematsu, S., Akira, S., et al. (2008a). Toll-like receptor 5-deficient mice have dysregulated intestinal gene expression and nonspecific resistance to Salmonella-induced typhoid-like disease. Infect. Immun. 76, 1276–1281. doi: 10.1128/IAI.01491-07
Vijay-Kumar, M., Aitken, J. D., Sanders, C. J., Frias, A., Sloane, V. M., Xu, J., et al. (2008b). Flagellin treatment protects against chemicals, bacteria, viruses, and radiation. J. Immunol. 180, 8280–8285. doi: 10.4049/jimmunol.180.12.8280
Vijay-Kumar, M., Carvalho, F. A., Aitken, J. D., Fifadara, N. H., and Gewirtz, A. T. (2010). TLR5 or NLRC4 is necessary and sufficient for promotion of humoral immunity by flagellin. Eur. J. Immunol. 40, 3528–3534. doi: 10.1002/eji.201040421
Yoon, S. I., Kurnasov, O., Natarajan, V., Hong, M., Gudkov, A. V., Osterman, A. L., et al. (2012). Structural basis of TLR5-flagellin recognition and signaling. Science 335, 859–864. doi: 10.1126/science.1215584
Zhang, B., Chassaing, B., Shi, Z., Uchiyama, R., Zhang, Z., Denning, T. L., et al. (2014). Viral infection. Prevention and cure of rotavirus infection via TLR5/NLRC4-mediated production of IL-22 and IL-18. Science 346, 861–865. doi: 10.1126/science.1256999
Ziegler, T. R., Luo, M., Estivariz, C. F., Moore, D. A. III, Sitaraman, S. V., Hao, L., Bazargan, N., et al. (2008). Detectable serum flagellin and lipopolysaccharide and upregulated anti-flagellin and lipopolysaccharide immunoglobulins in human short bowel syndrome. Am. J. Physiol. Regul. Integr. Comp. Physiol. 294, R402–R410. doi: 10.1152/ajpregu.00650.2007
Keywords: LPS, innate immunity, immune tolerance, antibodies, salmonella, infection
Citation: Yeoh BS, Gewirtz AT and Vijay-Kumar M (2019) Adaptive Immunity Induces Tolerance to Flagellin by Attenuating TLR5 and NLRC4-Mediated Innate Immune Responses. Front. Cell. Infect. Microbiol. 9:29. doi: 10.3389/fcimb.2019.00029
Received: 12 December 2018; Accepted: 31 January 2019;
Published: 19 February 2019.
Edited by:
Prajwal Gurung, The University of Iowa, United StatesReviewed by:
Kai Yang, Indiana University School of Medicine, United StatesYuan He, Wayne State University School of Medicine, United States
Copyright © 2019 Yeoh, Gewirtz and Vijay-Kumar. This is an open-access article distributed under the terms of the Creative Commons Attribution License (CC BY). The use, distribution or reproduction in other forums is permitted, provided the original author(s) and the copyright owner(s) are credited and that the original publication in this journal is cited, in accordance with accepted academic practice. No use, distribution or reproduction is permitted which does not comply with these terms.
*Correspondence: Matam Vijay-Kumar, bWF0YW12aWpheS5rdW1hckB1dG9sZWRvLmVkdQ==