- Charité – Universitätsmedizin Berlin, Corporate Member of Freie Universität Berlin, Humboldt-Universität zu Berlin, and Berlin Institute of Health, Institute of Virology, Berlin, Germany
Programmed cell death protein (PD-1) and its ligands play a fundamental role in the evasion of tumor cells from antitumor immunity. Less well appreciated is the fact that the PD-1/PD-L1 axis also regulates antiviral immune responses and is therefore modulated by a number of viruses. Upregulation of PD-1 and its ligands PD-L1 and PD-L2 is observed during acute virus infection and after infection with persistent viruses including important human pathogens such as human immunodeficiency virus (HIV), hepatitis C virus (HCV), and hepatitis B virus (HBV). Experimental evidence suggests that insufficient signaling through the PD-1 pathway promotes immunopathology during acute infection by exaggerating primary T cell responses. If chronic infection is established, however, high levels of PD-1 expression can have unfavorable immunological consequences. Exhaustion and suppression of antiviral immune responses can result in viral immune evasion. The role of the PD-1/PD-L1 axis during viral infections is further complicated by evidence that PD-L1 also mediates inflammatory effects in the acute phase of an immune response. In this review, we discuss the intricate interplay between viruses and the PD-1/PD-L1 axis.
Introduction
Programmed cell death 1 (PD-1, also known as CD279) was discovered by Tasuku Honjo et al. at Kyoto University from a screen of genes involved in programmed cell death (Ishida et al., 1992). PD-1 expression is rapidly induced after signaling through the T cell receptor (TCR) and modulated by cytokines (Agata et al., 1996; Yamazaki et al., 2002; Wherry et al., 2007; Chikuma et al., 2009; Terawaki et al., 2011; Ahn et al., 2018). Other types of immune cells such as B cells, natural killer (NK) cells, NKT cells, dendritic cells (DCs), and monocytes also express PD-1 (Sharpe et al., 2007; Keir et al., 2008).
There is ample evidence that PD-1, a member of the immunoglobulin superfamily, regulates the magnitude and quality of T cell responses. It plays a pivotal role in the induction and maintenance of central as well as peripheral tolerance (Nishimura et al., 1999, 2001; Wang et al., 2005; Okazaki and Honjo, 2006; Francisco et al., 2010; Fife and Pauken, 2011). For example, antigen presentation by resting DCs induces peripheral CD8+ T cell tolerance by signaling through PD-1 on CD8+ T cells (Probst et al., 2005). In fact, PD-1 has been called a ‘rheostat’ that calibrates threshold, strength, and duration of T cell responses (Okazaki et al., 2013; Honda et al., 2014). PD-1 belongs to a group of structurally different surface molecules that function as co-inhibitory receptors during immune responses against pathogens and cancer (Attanasio and Wherry, 2016; Hashimoto et al., 2018; Sharpe and Pauken, 2018). These molecules counterbalance co-stimulatory receptors on T cells such as CD28, which bind to CD80 and CD86 on professional APCs and facilitate T cell activation (Esensten et al., 2016).
Clinical studies have shown that blocking the PD-1 pathway is effective against several types of cancer including melanoma, lymphoma, lung, and renal cancer (Sanmamed and Chen, 2018). This type of treatment is referred to as immune checkpoint therapy and the blocking reagents are called immune checkpoint-inhibitors (ICIs). Together with James P. Allison, who worked on another co-inhibitory receptor called cytotoxic T-lymphocyte-associated Protein 4 (CTLA-4), Tasuku Honjo was awarded the Nobel Prize in Physiology or Medicine 2018 for the discovery of cancer therapy by inhibition of negative immune regulation (Wolchok, 2018).
PD-1 interacts with the ligands PD-L1 (CD274; also called B7-H1) and PD-L2 (CD273; also called B7-DC), which show distinct expression patterns. In vitro, PD-1 inhibits T cell activation by recruiting Src homology region 2-containing protein tyrosine phosphatase 2 (SHP2) after interaction with its ligands on APCs (Chen and Flies, 2013; Okazaki et al., 2013; Sharpe and Pauken, 2018). This is associated with dephopshorylation of crucial tyrosine residues within the CD3 complex and CD28. In virus-infected mice lacking SHP2 in T cells, however, PD-1 signaling is not impaired, suggesting the existence of redundant inhibitory pathways downstream of PD-1 (Rota et al., 2018).
PD-L1 is expressed not only by all hematopoietic cells but also by many non-hematopoietic cell types such as endothelial cells and epithelial cells (Sharpe and Pauken, 2018). In contrast, PD-L2 expression is more restricted and can be induced on hematopoietic cells such as DCs, B cells, and monocytes/macrophages. Besides PD-1, there are other known interacting partners for PD-L1 and PD-L2. PD-L1 also binds to CD80 whereas PD-L2 uses RGM domain family member B (RGMB) as an alternative binding partner. Both types of interaction also inhibit immune responses (Butte et al., 2007; Xiao et al., 2014).
Viruses have to overcome strong barriers to replicate in the hostile environment of their hosts (Virgin et al., 2009). An arsenal of weapons helps viruses to subvert antiviral immunity. This includes the exploitation of host inhibitory receptor signaling pathways (Ong et al., 2016). The impact of the PD-1/PD-L1 axis in chronic virus infections is well described whereas its role during the acute phase of viral infections is less clear (Brown et al., 2010; Attanasio and Wherry, 2016). However, whether virus-induced upregulation of PD-1 ligands represents a viral immune evasion strategy or an adaption of the host defense to minimize immunopathology is a moot point. In this review, we highlight the diverse roles of PD-1 and its ligands during virus infections and their implications for host-pathogen interaction.
The Role of the PD-1 Pathway in Acute Virus Infections
In mice acutely infected with lymphocytic choriomeningitis virus (LCMV) strain Armstrong (LCMV Arm) PD-1 is rapidly upregulated on naïve virus-specific CD8+ T cells before they clonally expand (Ahn et al., 2018). In this model of acute LCMV infection, CD4+ T cells are not required for virus clearance, which occurs within 1–2 weeks after infection (Matloubian et al., 1994). Blockade of the PD-1 pathway at this stage further increases effector functions of CD8+ T cells by enhancing granzyme B expression and mechanistic Target of Rapamycin (mTOR) signaling. Consequently, virus elimination is accelerated although the total number of virus-specific CD8+ T cells does not change (Ahn et al., 2018). Similarly, the PD-1/PD-L axis inhibits the differentiation of CD8+ T lymphocytes into polyfunctional cytotoxic T cells during acute infection of mice with murine retrovirus (David et al., 2019). This implies that PD-1 negatively regulates the terminal differentiation of naïve CD8+ T cells into effector CD8+ T lymphocytes during acute virus infection.
After virus clearance, PD-1 expression on virus-specific T cells returns to normal levels (Barber et al., 2006; Blattman et al., 2009). The expanded pool of virus-specific effector T lymphocytes contracts due to increased cell death and memory T cells arise from a subset of fate-permissive effector T cells (Akondy et al., 2017; Omilusik and Goldrath, 2017; Youngblood et al., 2017). There are at least three major memory T cell subsets: central memory T cells (Tcm cells), effector memory T cells (Tem cells), and recently defined tissue-resident memory T cells (Trm cells). Tcm cells lack effector functions but express lymph node homing molecules and circulate through the blood and the secondary lymphoid organs (Sallusto et al., 1999). After stimulation, Tcm cells differentiate into Tem cells that lack lymph node homing molecules and continuously recirculate between blood, lymph and non-lymphoid tissues. Tem cells are bestowed with various effector functions (Sallusto et al., 1999). In contrast, Trm cells do not recirculate (Wakim et al., 2008; Gebhardt et al., 2009; Masopust et al., 2010) and express core phenotypic markers including co-inhibitory receptors such as PD-1 (Hombrink et al., 2016; Kumar et al., 2017; Pallett et al., 2017). Functionally, Trm cells participate in the first line of defense to viruses by establishing an antiviral state and recruiting circulating memory T cells to sites of viral infection (Schenkel et al., 2013, 2014; Ariotti et al., 2014; Carbone and Gebhardt, 2014). Located in multiple anatomical sites including barrier tissue such as lung, skin and gut, Trm cells are indispensable for antiviral immunity and immunosurveillance (Shin, 2018; Wu et al., 2018; Szabo et al., 2019). The functional role of the PD-1/PD-L1 axis for CD8+ Trm cells is unclear at the moment but it may prevent uncontrolled Trm activation and inflammation in virus-infected tissues and other inflammatory conditions. In accordance, blockade of PD-1 on Trm cells increases the severity of eczema in a mouse model of allergic contact dermatitis (Gamradt et al., 2019).
Intriguingly, the number of memory precursor T cells increases if PD-1 is blocked by antibodies during acute LCMV infection, possibly due to faster virus elimination (Ahn et al., 2018). Virus-specific memory CD8+ T cells that develop after the elimination of LCMV persist without antigen and are capable of self-renewal due to homeostatic proliferation in response to IL-7 and IL-15 (Wherry et al., 2004; Surh and Sprent, 2008; Abdelsamed et al., 2017). Although the blockade of the PD-1/PD-L1 axis in mice infected with LCMV Arm increases effector CD8+ T cell function, no excessive tissue damage is observed (Ahn et al., 2018). Similar to the LCMV strain WE (LCMV WE), LCMV Arm does not disseminate but instead is eliminated from infected laboratory mice after acute infection. In contrast, derivatives of LCMV Arm and LCMV WE (“clone 13” and “docile,” respectively) replicate more vigorously and persist (Matloubian et al., 1990; Welsh and Seedhom, 2008). These LCMV strains cause lethal immunopathology in mice deficient of the PD-1/PD-L1 axis (PD-L1 KO mice, PD-1 KO mice) during the acute phase of infection (Barber et al., 2006; Mueller et al., 2010; Frebel et al., 2012; Zinselmeyer et al., 2013; Shaabani et al., 2016). This is due to the killing of LCMV-infected vascular endothelium by CD8+ T cells resulting in vascular leakage with pulmonary edema and severe hypotension (Frebel et al., 2012). In a mouse model of acute viral hepatitis, the absence of PD-1 is associated not only with more rapid virus clearance but also with more severe hepatitis (Iwai et al., 2003). These results imply that the stimulation of the PD-1/PD-L1 axis during the acute phase of virus infection helps to adjust the strength and quality of the cytotoxic CD8+ T cell attack so that the good (virus elimination) and the bad (tissue damage) is balanced, preventing excessive tissue damage.
Virus-Driven PD-L1/2 Expression
Many viruses increase PD-L1/2 expression on hematopoietic cells (Table 1) and non-hematopoietic cells (Table 2). PD-L1/2 expression is regulated by proinflammatory and anti-inflammatory signals (Sun et al., 2018). The promotor regions of PD-L1 and PD-L2, which are paralog genes, are differentially regulated although they show similarly arranged binding sites for transcription factors (Garcia-Diaz et al., 2017).
Type I and type III interferons (IFNs) are important antiviral cytokines. They are induced early in virus-infected barrier tissue such as lung/gut epithelial cells and serve as the first line of antiviral defense (Okabayashi et al., 2011; Wack et al., 2015; Andreakos et al., 2017; Galani et al., 2017; Zanoni et al., 2017; Good et al., 2019; Lazear et al., 2019). Type I IFNs, which in humans include several IFN-α subtypes and IFN-β, increase PD-L1 expression but to a lesser extent than PD-L2 expression (Garcia-Diaz et al., 2017). PD-L2 responds equally well to IFN-γ (type II IFN) and IFN-β (Garcia-Diaz et al., 2017). IL-4 may be an even more potent inducer of PD-L2 (Loke and Allison, 2003) thus accounting for the presence of PD-L2 on monocyte-derived DCs generated in vitro. Blockade or absence of type I IFN signaling during chronic LCMV infection results in reduced PD-L1 expression despite enhanced viral replication (Teijaro et al., 2013; Wilson et al., 2013; Shaabani et al., 2016). Although type I IFNs moderately upregulate PD-L1 (Sun et al., 2018) they increase NK cytotoxicity and allow clonal expansion and memory formation of antiviral cytotoxic CD8+ T cells (Biron et al., 2002; Kolumam et al., 2005; Aichele et al., 2006). Type III IFNs signal through a unique heterodimeric receptor and induce the expression of antiviral IFN-stimulated genes (ISGs) similar to type I IFNs (Davidson et al., 2015). Intriguingly, type III IFNs do not upregulate PD-L1 (Raftery et al., 2018). Accordingly, in this early phase of acute infection the PD-1/PD-L1 axis does not inhibit antiviral immune cells.
Recognition of viruses by pattern recognition receptors (PRRs) also upregulates PD-L1. TLR3 signaling in particular strongly increases PD-L1 levels on DCs (Pulko et al., 2009; Boes and Meyer-Wentrup, 2015; Raftery et al., 2018) whereas RIG-I signaling alone has no significant effect (Raftery et al., 2018). Triggering of TLR3, which transmits downstream signals through the TIR-domain-containing adapter-inducing IFN-β (TRIF), also enhances PD-L1 on other cell types including endothelial cells (Cole et al., 2011) and epithelial cells (Tsuda et al., 2005). In accordance, virus-induced PD-L1 upregulation on neuronal cells is severely impaired in TLR3-deficient mice (Lafon et al., 2008). Recently, viral proteins inducing PD-L1/PD-L2 expression have been identified. For example, HIV Tat protein increases PD-L1 expression on DCs through TNF-α and TLR4 signaling (Planes et al., 2014). The HCV core protein in vitro induces strong PD-L1 upregulation on primary human Kupffer cells and monocytes in a TLR2- and PI3K-dependent manner (Tu et al., 2010; Zhai et al., 2017). In accordance, the PD-L1 levels on monocytes from HCV-infected patients were significantly higher than on monocytes from healthy individuals (Zhai et al., 2017). A recent study has shown that extracellular vesicles (EVs) produced by HBV-infected hepatocytes are endocytosed by circulating monocytes resulting in PD-L1 upregulation (Huang et al., 2017; Kakizaki et al., 2018). Moreover, PD-L1 and PD-L2 are upregulated by hantaviral N protein most likely via hantavirus-induced TLR3 signaling (Raftery et al., 2018). In addition, the latency-associated transcripts (LATs) of herpes simplex virus type 1 (HSV-1) upregulate PD-L1 on mouse neuroblastoma cells by an unknown mechanism (Chentoufi et al., 2011). Remarkably, as of yet no viral immunoevasin has been discovered that directly interacts with the molecules of the PD-1/PD-L1 axis to exploit its immunosuppressive function.
Viral replication can also result in the production of anti-inflammatory cytokines such as IL-10 (Brooks et al., 2006b; Ejrnaes et al., 2006). Cellular IL-10 has been shown to upregulate the expression of PD-1 and PD-L1 in a STAT-3 dependent manner in DCs and monocytes (Curiel et al., 2003; Selenko-Gebauer et al., 2003; Sun et al., 2015; Lamichhane et al., 2017). Accordingly, the absence of cellular IL-10 in LCMV infected mice results in enhanced effector T cell responses, rapid virus elimination, and generation of antiviral memory T cells (Brooks et al., 2006b; Ejrnaes et al., 2006). Intriguingly, during coevolution with their hosts members of the virus family Herpesviridae have acquired numerous genes from their hosts including those that mimic cellular IL-10 (Raftery et al., 2000; Ouyang et al., 2014; Schonrich et al., 2017). These viral IL-10 (vIL-10) molecules act as immunosuppressive cytokines that also paralyze co-stimulatory B7 molecules (Muller et al., 1998; Raftery et al., 2004). It is possible that vIL-10 molecules also increase signaling through the PD-1/PD-L1 axis similar to their cellular counterparts thereby contributing to viral persistence. However, combined blockade of both, IL-10 and PD-L1, during chronic LCMV infection enhances T-cell function more efficiently than a single blockade (Brooks et al., 2008). Thus, IL-10 is pleiotropic and has immunosuppressive functions independent of the PD-1/PD-L1 axis during persisting virus infections (Ouyang et al., 2011).
In the late phase of acute virus infection, type II IFN and several other cytokines including TNF-α and IL-10 are released by immune cells such as CD8+ T cell cells (Zhang and Bevan, 2011). IFN-γ strongly upregulates PD-L1 (Garcia-Diaz et al., 2017; Raftery et al., 2018; Sun et al., 2018). In addition, plasmacytoid DCs (pDCs) migrate into virus-infected tissue and secrete large amounts of type I IFNs (Siegal et al., 1999). These cytokines not only induce antiviral ISGs but also drive inflammatory responses such as secretion of TNF-α, IL-1β, or IL-6 (Davidson et al., 2015), which can further increase PD-L1 on various cell types including endothelial cells and at the same time promote non-lytic virus elimination (Sun et al., 2018). Thus, in the late phase of acute viral infection, PD-L1 is strongly upregulated thereby downregulating terminal differentiation of CD8+ T cells and preventing excessive tissue damage due to uncontrolled cytotoxic attack.
Function of PD-L1 During Acute Virus Infections
PD-L1 expressed on hematopoietic or non-hematopoietic cells has different functions (Keir et al., 2006; Mueller et al., 2010). For example, PD-L1 expression on parenchymal cells of the pancreas rather than hematopoietic cells prevent autoimmune diabetes (Keir et al., 2006). In accordance, during LCMV infection of mice PD-L1 expression on non-hematopoietic cells reduces viral clearance and immunopathology (Keir et al., 2008). Thus, upregulation of PD-L1 expression may protect virus-infected cells from being eliminated by cytotoxic CD8+ T cells. On the other hand, selective absence of PD-L1 on hematopoietic cells results in lethal immunopathology (Mueller et al., 2010). This is best explained by an increase in number and function of cytotoxic CD8+ T lymphocytes, which may overwhelm the PD-L1-conferred protection in non-hematopoietic target cells (Frebel et al., 2012).
Virus-induced PD-L1 on professional APCs may help to focus the antiviral CD8+ T cell response on a few strongly stimulatory, i.e., immunodominant, virus-derived epitopes by increasing the threshold of CD8+ T cell activation. In this way, the majority of weakly immunogenic viral peptides fail to activate CD8+ T cells. The adjustment of the “rheostat” on professional APCs may be necessary to prevent autoimmune disease and maintain peripheral tolerance in the face of a highly inflammatory milieu. Indeed, a recent study has shown that the PD-L1/PD-1 axis regulates T cell responses at the activation stage (Sugiura et al., 2019). CD80, which binds to CD28 and CTLA-4 on T cells, also interacts with PD-L1 (Butte et al., 2007, 2008). Importantly, this interaction occurs only in cis (Chaudhri et al., 2018) and prevents PD-L1 on DCs from co-inhibitory signaling to T cells via PD-1 (Chaudhri et al., 2018; Sugiura et al., 2019). In contrast, the functions of CD28 (co-stimulatory) and CTLA-4 (co-inhibitory) are not impaired by cis-PD-L1/CD80 interactions on DCs (Sugiura et al., 2019). Many viruses upregulate PD-L1 on professional APCs such as DCs (Table 1) either directly or through IFN release. Low PD-L1 levels on uninfected DCs have only a weak impact on T cell activation (Brown et al., 2003) due to cis-PD-L1/CD80 interactions (Figure 1, upper scheme). It is likely, that the high PD-L1 levels on DCs in the context of viral infection will overwhelm the cis-binding capacity of CD80 resulting in increased co-inhibitory signaling via PD-1 (Figure 1, lower scheme). PD-L1 on professional APCs also promotes the induction and maintenance of regulatory T cells (Treg cells; Francisco et al., 2009). Treg cells help to confine the antiviral defense and to prevent immunopathology during virus infections (Veiga-Parga et al., 2013). Taken together, viruses can reprogram DC function in antiviral immune responses by tipping the balance between co-inhibitory and co-stimulatory signals as shown for murine cytomegalovirus (Loewendorf et al., 2004; Benedict et al., 2008) and vaccinia virus (Kleinpeter et al., 2019).
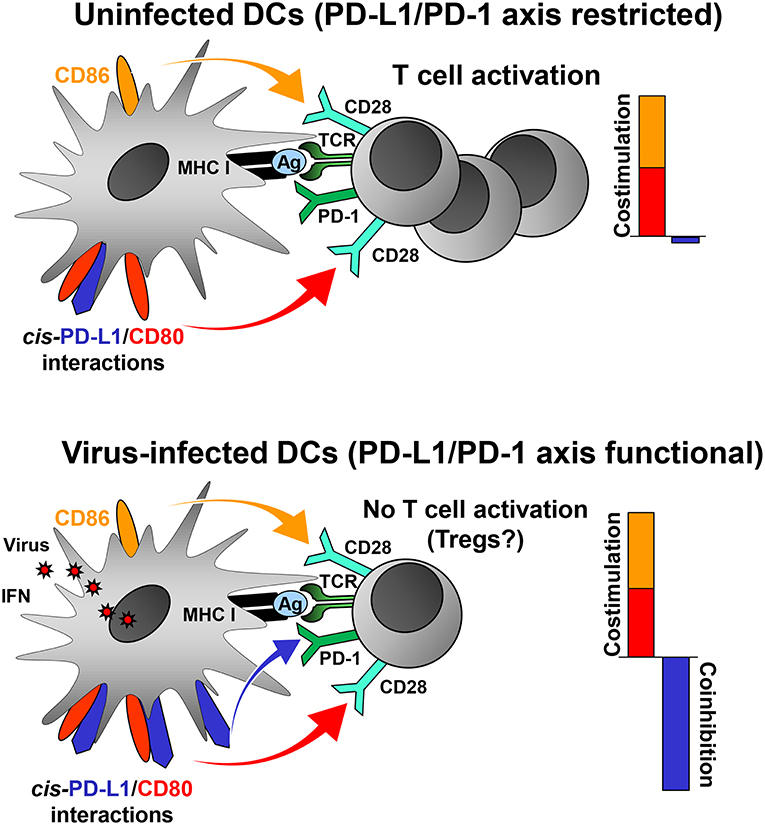
Figure 1. PD-L1 mediated viral regulation of T cell activation. Upper graph: In the absence of viral infection mature dendritic cells (DCs) express relatively low levels of PD-L1. Recognition of cognate antigen (Ag) bound to MHC class I molecules by T cell receptor (TCR) results in upregulation of PD-1 on T cells. DCs express co-stimulatory molecules CD80 and CD86 allowing efficient co-stimulation of T cells via CD28. The PD-1/PD-L1 axis is not co-inhibitory due to restriction by cis-PD-L1/CD80 interactions, and thus T cells are activated. Lower graph: In the context of viral infection DCs upregulate PD-L1 due to exposure to viral PAMPs and high levels of type I IFN. The restricting cis-PD-L1/CD80 interactions are most likely overwhelmed by virus-induced PD-L1 resulting in PD-1 signaling and prevention of T cell activation. The consequences of this for the generation of Tregs is as of yet unknown.
Strong stimulation of the PD-1/PD-L1 does not prevent immunopathology during viral hemorrhagic fever (VHF). VHF is a designation for distinct but pathogenically similar zoonotic diseases that are caused by several enveloped RNA viruses including Ebola virus (EBOV), hantavirus, and dengue virus (DENV) (Paessler and Walker, 2013). VHF viruses target endothelial cells thereby causing vascular leakage (Zampieri et al., 2007; Schonrich et al., 2008; Basler, 2017). In fact, type III IFN and TNF-α, which upregulate PD-L1 on endothelial cells, also mediate dysfunction of the endothelial barrier (Brett et al., 1989; Koh et al., 2004). Virus-specific CD8+ T cells show high levels of PD-1 on the surface during acute infection with EBOV (McElroy et al., 2015). Moreover, fatal EBOV infection is characterized by a high percentage of T cells expressing PD-1 and other co-inhibitory receptors such as CTLA-4 (Ruibal et al., 2016). Monocytes are susceptible to EBOV infection and upregulate production of PD-L1 transcripts in response to EBOV replication (Menicucci et al., 2017), whereas DENV-infected DCs express higher levels of PD-L2 but reduced PD-L1 (Nightingale et al., 2008). In patients with acute hantavirus infection large amounts of soluble PD-L1/PD-L2 are found in the sera indicating that these molecules are strongly upregulated in hantavirus-infected cells in vivo (Raftery et al., 2018). In accordance, strongly increased PD-L1 levels are detected after hantavirus infection of immature DCs in vitro and in hantavirus-infected mice with a humanized immune system (Raftery et al., 2018). In striking contrast, CD8+ T cells do not upregulate PD-1 during acute hantavirus infection (Lindgren et al., 2011).
Taken together, in the acute phase of viral infection virus-specific T cells rapidly upregulate the co-inhibitory receptor PD-1 upon recognition of antigen. Simultaneously, viruses upregulate PD-L1 on hematopoietic and non-hematopoietic cells directly through PRR signaling or indirectly by inducing the release of IFNs and other inflammatory cytokines. Ideally, a tailor-made antiviral CD8+ T cell response eliminates viral pathogens with minimal immunopathology (Figure 2). The antiviral immune response during VHF, however, eliminates viruses at the cost of vascular leakage. The dysregulation of the immune responses could be due to variations in PD-L1 expression (e.g., timing, cell type, or strength), imbalance between co-stimulatory vs. co-inhibitory receptors (failure of “checks and balances”), or altered usage of PD-L1 interaction partners (PD-1, CD80, and possibly additional unknown partners). On the other hand viruses can also manipulate the “checks and balances” of the immune system in such a way that an effective antiviral immune response is prevented helping the pathogen to persist in the organism.
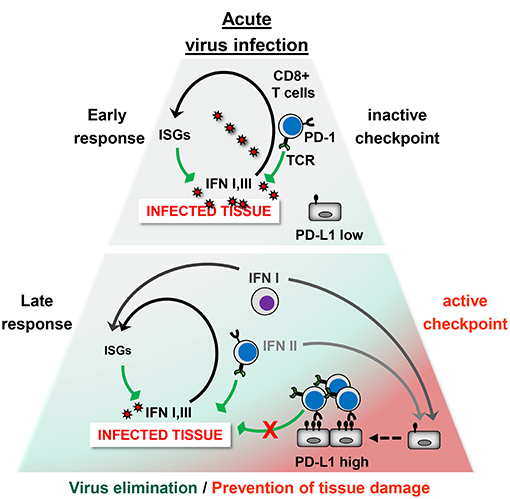
Figure 2. The PD-1/PD-L1 checkpoint in acute virus infection. Early phase: The infected tissue produces type I IFNs and possibly type III IFNs, which strongly induce antiviral IFN-stimulated genes (ISGs) but only moderate PD-L1 levels. Antiviral CD8+ T cells eliminate virus-infected cells. At this stage, the PD-1/PD-L1 checkpoint activity is low and does not restrict the antiviral immune response. Late Phase: Type II IFN and TNF-α is secreted by CD8+ T cells and other immune cells. In addition, hematopoietic cells such as plasmacytoid DCs (pDCs) produce large amounts of type I IFN. This results not only in virus elimination but also increases PD-L1 expression. The high checkpoint activity downregulates terminal differentiation of antiviral CD8+ T cells. Ideally, the strength and quality of the CD8+ T cell response is balanced out in such a way that the viral intruder is eliminated without causing immunopathology.
The PD-1/PD-L1 Axis During Persisting Virus Infections
Chronic Virus Infection
Chronic infections with viruses such as hepatitis B virus, hepatitis C virus (HCV), and human immunodeficiency virus (HIV) represent major causes of chronic disease and death worldwide (Ott et al., 2012; Schweitzer et al., 2015; GBD 2015 HIV Collaborators, 2016; Stanaway et al., 2016). During chronic infection virus particles are continuously released from virus-infected cells and maintain a network of immunosuppressive mechanisms that interfere with virus elimination (Ng et al., 2013). Therefore, T cells enter a state called T cell exhaustion.
T Cell Exhaustion and Partial Restoration of T Cell Function by Blockade of the PD-1/PD-L1 Axis
The first evidence for T cell exhaustion was gathered in paradigmatic experiments using LCMV-infected mice (Zehn and Wherry, 2015; Kahan and Zajac, 2019). Derivatives of LCMV Arm and LCMV WE (LCMV clone13 and LCMV docile, respectively) vigorously replicate and disseminate in mice thereby persisting for more than 100 days (Moskophidis et al., 1993; Gallimore et al., 1998; Zajac et al., 1998). In this model of chronic virus infection, CD4+ T cells are crucial to sustain the virus-specific CD8+ T cell responses (Matloubian et al., 1994). Sustained upregulation of PD-1 and other co-inhibitory receptors such as 2B4, CTLA-4, and lymphocyte-activation gene 3 (Lag3) has become the defining characteristic of exhausted T (Tex) cells (Barber et al., 2006; Wherry et al., 2007; Blackburn et al., 2009; Crawford et al., 2014). These phenotypic changes are accompanied by a multistep loss of T cell effector functions (Speiser et al., 2014; Kahan et al., 2015; Wherry and Kurachi, 2015; McKinney and Smith, 2018). Dependent on the strength of PD-1 signaling CD8+ T lymphocytes gradually lose important effector functions (Wherry et al., 2003; Wei et al., 2013). Some are lost early (such as cytotoxicity, IL-2 production, and proliferation), whereas others (e.g., IFN-γ production) are maintained for a longer time (Wherry et al., 2003; Wei et al., 2013). Finally, Tex cells undergo apoptosis (Kahan et al., 2015). As an underlying mechanism of T cell exhaustion during chronic LCMV infection, PD-1 signaling impairs T cell motility facilitating engagement of inhibitory pathways in T cells (Zinselmeyer et al., 2013). In another experimental setting, PD-L1 blocking antibodies prolong the T cell migration arrest suggesting that PD-1 signaling in fact enhances T cell motility (Honda et al., 2014). The reason for these contrasting results are unclear at the moment. Intriguingly, PD-1-regulated changes in several metabolic pathways occur at the very beginning of Tex cell development underlining the importance of these metabolic processes in the execution of the Tex program (Bengsch et al., 2016; Schurich et al., 2016;McKinney and Smith, 2018).
Several reports have found differences in the transcriptional program and epigenetic profile of Tex cells as compared to memory and effector T lymphocytes (Wherry et al., 2007; Doering et al., 2012; Pauken et al., 2016). In Tex cells derived from LCMV-infected mice, the Pdcd1 regulatory region is completely demethylated and remains so even when virus titers decrease (Youngblood et al., 2011). They do not show antigen-independent persistence driven by IL-7 and IL-15, the hallmark of memory T cells, and instead require the continuous presence of their cognate antigen (Wherry and Ahmed, 2004; Shin et al., 2007). This can be explained by the observation that the TCR-induced transcription factors IRF4, BATF, and NFATc1 not only drive T cell exhaustion but also impair memory T cell development during chronic LCMV infection (Man et al., 2017). Recently, microRNA (miR)-155 has been identified as a key molecule that promotes long-term persistence of Tex cells (Stelekati et al., 2018).
T cells that have been rendered dysfunctional during persisting virus infections can be reinvigorated (Brooks et al., 2006a). Blockade of the PD-1/PD-L1 axis during chronic LCMV infection reinvigorates antiviral T cell functions and reduces viral load (Barber et al., 2006). Of note, CD8+ T cells also become exhausted in the absence of PD-1 (Odorizzi et al., 2015). These experiments show that other coinhibitory receptors contribute to T cell exhaustion. In line with this view, a combined blockade of PD-1 and LAG-3 or PD-1 and Tim-3 synergistically improves antiviral CD8+ T cell responses and viral control in mice with chronic LCMV infection (Blackburn et al., 2009; Jin et al., 2010). Reinvigorated CD8+ T cells in chronically LCMV-infected mice become exhausted again after termination of the PD-L1 blockade (Pauken et al., 2016; Sen et al., 2016; Turner and Russ, 2016). This finding indicates that inflexibility of the epigenetic regulation in Tex cells may limit the success of therapies using ICIs.
The studies of chronic LCMV infection in mice also relate to important human infectious diseases. In a recently established mouse model of HCV infection Tex cells are observed in the liver of mice infected with a newly identified Norway rat hepacivirus (NrHV), which belong to the same virus family as HCV (Billerbeck et al., 2017; Klenerman and Barnes, 2017). In NrHV-infected mice, CD4+ T cells were important to maintain the antiviral CD8+ T cell response similar to the LCMV model of chronic virus infection (Billerbeck et al., 2017). Blockade of the PD-1/PD-L1 axis in early chronic infection reduced the viral load whereas no beneficial effects were observed at later time points (Billerbeck et al., 2017). Moreover, ICIs blocking the PD-1 pathway can reinvigorate to some extent Tex cells in humans chronically infected with HBV or HCV (McKinney and Smith, 2016; Cox et al., 2017; Saeidi et al., 2018; Wykes and Lewin, 2018). Targeting the PD-1/PD-L1 pathway during retroviral infections has beneficial effects for virus control (Velu et al., 2015). PD-1 upregulation is linked to a loss of function in HIV-specific CD8+ T cells, which can be partially reversed in vitro by a blockade of the PD-1/PD-L1 axis (Day et al., 2006; Trautmann et al., 2006). Surprisingly, the context and timing of PD-1 blockade seems to be important for its functional outcome: PD-1 signaling inhibition during stimulation of naive CD8+ T cells results in diminished activation, whereas PD-1 blockade during the T cell effector phase increases activation (Garcia-Bates et al., 2019). PD-1 blockade in rhesus macaques infected with simian immunodeficiency (SIV) rapidly increases the number and functional quality of virus-specific CD8+ T cells (Velu et al., 2009). Intriguingly, the combination of anti-PD-1 antibodies and antiretroviral therapy further improves antiviral CD8+ T cell function in SIV-infected rhesus macaques (Mylvaganam et al., 2018). This observation implies that directly acting antivirals (DAAs) reducing the viral load and ICIs releasing the brake in Tex cells synergistically increase antiviral T cell responses.
Recent data suggest that PD-1 expression does not necessarily reflect T cell failure but rather adaption of T cell function to chronic inflammation (Utzschneider et al., 2013, 2016; Speiser et al., 2014; Staron et al., 2014; Zehn et al., 2016; Barnes, 2018; Petrelli et al., 2018). In fact, at least two CD8+ Tex cell subsets exist that act in concert to mount a partially effective CD8+ T cell response for control of chronic virus infection (Paley et al., 2012). Moreover, Tex cells have the capacity for self-renewal and are not entirely functionally inactive (Paley et al., 2012). The latter finding implies that Tex cells may represent a form of antiviral defense that is evolutionary adapted to the need to control a chronically replicating non-lytic virus with minimal collateral tissue damage and immunopathology. Moreover, experiments in mice with genetic ablation of PD-1 suggest that PD-1 is not required for induction of Tex cells (Odorizzi et al., 2015). In fact, PD-1 may play a pivotal role in maintaining Tex cells by preventing excessive stimulation that leads to proliferation and terminal differentiation (Odorizzi et al., 2015). After the elimination of HCV by DAAs, PD-1 expressing CD8+ T cell populations remain that display characteristics of memory cells including antigen-independent survival and proliferation after re-challenge with antigen (Wieland et al., 2017).
Latent Infection and Reactivation
Viruses that establish latent infection include the members of the family Herpesviridae. In contrast to chronic infection, latent infection is characterized by periodic suspension of virus replication. However, the blueprint of viral particles is preserved in the latently infected host cells enabling the virus to reactivate and resume virus production. It is a matter of debate whether reactivation from latent virus infection creates enough antigenic load to induce exhaustion of antiviral CD8+ T cells. Memory CD8+ T cells recognizing viral antigens in the context of chronic virus infections (e.g., HIV) more frequently express PD-1 than memory CD8+ T cells stimulated by virus periodically reactivating from latency, e.g., human cytomegalovirus (HCMV) (Petrovas et al., 2006). This finding is consistent with the idea that the amount of available antigen regulates PD-1 expression on reactive T cells (Petrovas et al., 2006). In accordance, increased virus replication in immunosuppressed patients with HCMV disease after allogeneic hematopoietic cell transplantation is associated with PD-1 upregulation on T cells (Gallez-Hawkins et al., 2009). In mice with a humanized immune system, HCMV reactivations induced by granulocyte-colony stimulating factor (G-CSF) resulted in a shift toward PD-1 expressing T cells (Theobald et al., 2018). Whether this phenotype corresponds to Tex cells is unclear, however. Upregulation of co-inhibitory receptors such as PD-1 on CD8+ T cells is tightly linked to activation and differentiation and not per se proof of T cell exhaustion (Legat et al., 2013). In addition, studies of HSV-1 infection in mice did not reveal evidence for functional impairment of virus-specific CD8+ T cells during latency and subsequent reactivations (Mackay et al., 2012).
PD-1 expression on brain Trm cells is maintained independently from antigen (Shwetank et al., 2017). Recently, it has been shown that Trm cells provide immunosurveillance in the human brain to eliminate neurotropic viruses (Smolders et al., 2018). In accordance, reactivation of HSV-1 from latently infected neurons of the mouse is controlled by CD8+ Trm lymphocytes (Liu et al., 2000; Khanna et al., 2003; Verjans et al., 2007). These immune cells provide IFN-γ which upregulates PD-L1 on HSV-1-infected neurons (Jeon et al., 2013). CD8+ T cells recognizing subdominant epitopes derived from HSV-1 proteins other than glycoprotein B (gB) but not CD8+ T cells specific for the dominant gB-derived epitope show a partial exhausted phenotype with increased PD-1 expression (Jeon et al., 2013). Blockade of PD-L1 resulted in increased survival of exhausted CD8+ T cells that were non-functional and not protective, however (Jeon et al., 2013). In contrast, it has been reported that HSV-1 LATs promotes functional exhaustion of CD8+ T cells specific for the dominant gB-derived epitope (Allen et al., 2011; Chentoufi et al., 2011).
During coevolution with their host, herpesviruses developed numerous mechanisms to evade the antiviral immune response such as modulation of programmed cell death (Raftery et al., 1999, 2001; Muller et al., 2004; Kather et al., 2010) and downregulation of MHC class I molecules (Schuren et al., 2016). Intriguingly, replication competent varicella-zoster virus (VZV) downregulates MHC class I and PD-L1 molecules in human brain vascular adventitial fibroblasts, perineurial cells, and human lung fibroblasts (Jones et al., 2016). In contrast, VZV upregulates PD-L1 in hematopoietic cells (Jones et al., 2019). The mechanism underlying VZV-associated downregulation of PD-L1 is posttranscriptional in nature but the VZV-encoded protein responsible has not yet been identified (Jones et al., 2016). VZV might target PD-L1 to increase the migration arrest of T cells (Honda et al., 2014). In this way, the virus could more efficiently spread from lung fibroblasts to T cells, which play crucial role in VZV dissemination to the skin (Arvin et al., 2010).
Concluding Remarks
It is a seductive proposition that a virus induces PD-1 ligands in order to inhibit and thus evade the host immune response. On the other hand, recent data on the regulation of PD-L1 expression during viral infection suggest that PD-L1 upregulation is rather a part of the normal innate response induced by IFNs and PRR signaling. The reason for this is still enigmatic. PD-L1 may have a yet not defined immunostimulatory role in the very early phase of viral infection. Later, it may adjust the quantity and quality of the antiviral CD8+ T cell response in such a way that virus is eliminated with minimal collateral tissue damage. The PD-1/PD-L1 axis may also be important to maintain antiviral Trm cells and Tex cells. Virus-induced PD-1 ligand expression as an immune evasion strategy should always be rigorously tested with this in mind.
Author Contributions
All authors listed have made a substantial, direct and intellectual contribution to the work, and approved it for publication.
Conflict of Interest Statement
The authors declare that the research was conducted in the absence of any commercial or financial relationships that could be construed as a potential conflict of interest.
Acknowledgments
The authors acknowledge support from the German Research Foundation (DFG) and the Open Access Publication Fund of Charité – Universitätsmedizin Berlin.
References
Abdelsamed, H. A., Moustaki, A., Fan, Y., Dogra, P., Ghoneim, H. E., and Youngblood, B. (2017). Human memory CD8 T cell effector potential is epigenetically preserved during in vivo homeostasis. J. Exp. Med. 214, 1593–1606. doi: 10.1084/jem.20161760
Agata, Y., Kawasaki, A., Nishimura, H., Ishida, Y., Tsubata, T., Yagita, H., et al. (1996). Expression of the PD-1 antigen on the surface of stimulated mouse T and B lymphocytes. Int. Immunol. 8, 765–772.
Ahn, E., Araki, K., Hashimoto, M., Li, W., Riley, J. L., Cheung, J., et al. (2018). Role of PD-1 during effector CD8 T cell differentiation. Proc. Natl. Acad. Sci. U.S.A. 115, 4749–4754. doi: 10.1073/pnas.1718217115
Aichele, P., Unsoeld, H., Koschella, M., Schweier, O., Kalinke, U., and Vucikuja, S. (2006). CD8 T cells specific for lymphocytic choriomeningitis virus require type I IFN receptor for clonal expansion. J. Immunol. 176, 4525–4529. doi: 10.4049/jimmunol.176.8.4525
Akhmetzyanova, I., Drabczyk, M., Neff, C. P., Gibbert, K., Dietze, K. K., and Zelinskyy, G. (2015). PD-L1 expression on retrovirus-infected cells mediates immune escape from CD8+ T cell killing. PLoS Pathog. 11:e1005224. doi: 10.1371/journal.ppat.1005224
Akondy, R. S., Fitch, M., Edupuganti, S., Yang, S., Kissick, H. T., and Ahmed, R. (2017). Origin and differentiation of human memory CD8 T cells after vaccination. Nature 552, 362–367. doi: 10.1038/nature24633
Allen, S. J., Hamrah, P., Gate, D., Mott, K. R., Mantopoulos, D., and Ghiasi, H. (2011). The role of LAT in increased CD8+ T cell exhaustion in trigeminal ganglia of mice latently infected with herpes simplex virus 1. J. Virol. 85, 4184–4197. doi: 10.1128/JVI.02290-10
Andreakos, E., Salagianni, M., Galani, I. E., and Koltsida, O. (2017). Interferon-lambdas: front-line guardians of immunity and homeostasis in the respiratory tract. Front. Immunol. 8:1232. doi: 10.3389/fimmu.2017.01232
Ariotti, S., Hogenbirk, M. A., Dijkgraaf, F. E., Visser, L. L., Hoekstra, M. E., and Schumacher, T. N. (2014). Skin-resident memory CD8(+) T cells trigger a state of tissue-wide pathogen alert. Science 346, 101–105. doi: 10.1126/science.1254803
Arvin, A. M., Moffat, J. F., Sommer, M., Oliver, S, Che, X., Vleck, S., et al. (2010). Varicella-zoster virus T cell tropism and the pathogenesis of skin infection. Curr. Top. Microbiol. Immunol. 342, 189–209. doi: 10.1007/82_2010_29
Attanasio, J., and Wherry, E. J. (2016). Costimulatory and coinhibitory receptor pathways in infectious disease. Immunity 44, 1052–1068. doi: 10.1016/j.immuni.2016.04.022
Barber, D. L., Wherry, E. J., Masopust, D., Zhu, B., Allison, J. P., and Ahmed, R. (2006). Restoring function in exhausted CD8 T cells during chronic viral infection. Nature 439, 682–687. doi: 10.1038/nature04444
Barnes, E. (2018). Unravelling the fate of functional PD1+ T cells in chronic viral hepatitis. J. Clin. Invest. 128, 573–576. doi: 10.1172/JCI99035
Basler, C. F. (2017). Molecular pathogenesis of viral hemorrhagic fever. Semin. Immunopathol. 39, 551–561. doi: 10.1007/s00281-017-0637-x
Benedict, C. A., Loewendorf, A., Garcia, Z., Blazar, B. R., and Janssen, E. M. (2008). Dendritic cell programming by cytomegalovirus stunts naive T cell responses via the PD-L1/PD-1 pathway. J. Immunol. 180, 4836–4847. doi: 10.4049/jimmunol.180.7.4836
Bengsch, B., Johnson, A. L., Kurachi, M., Odorizzi, P. M., Pauken, K. E., Attanasio, J., et al. (2016). Bioenergetic insufficiencies due to metabolic alterations regulated by the inhibitory receptor PD-1 are an early driver of CD8(+) T cell exhaustion. Immunity 45, 358–373. doi: 10.1016/j.immuni.2016.07.008
Billerbeck, E., Wolfisberg, R., Fahnoe, U., Xiao, J. W., Quirk, C., and Rice, C. M. (2017). Mouse models of acute and chronic hepacivirus infection. Science 357, 204–208. doi: 10.1126/science.aal1962
Biron, C. A., Nguyen, K. B., and Pien, G. C. (2002). Innate immune responses to LCMV infections: natural killer cells and cytokines. Curr. Top. Microbiol. Immunol. 263, 7–27. doi: 10.1007/978-3-642-56055-2_2
Blackburn, S. D., Shin, H., Haining, W. N., Zou, T., Workman, C. J., and Wherry, E. J. (2009). Coregulation of CD8+ T cell exhaustion by multiple inhibitory receptors during chronic viral infection. Nat. Immunol. 10, 29–37. doi: 10.1038/ni.1679
Blattman, J. N., Wherry, E. J., Ha, S. J., van der Most, R. G., and Ahmed, R. (2009). Impact of epitope escape on PD-1 expression and CD8 T-cell exhaustion during chronic infection. J. Virol. 83, 4386–4394. doi: 10.1128/JVI.02524-08
Boasso, A., Hardy, A. W., Landay, A. L., Martinson, J. L., Anderson, S. A., and Shearer, G. M. (2008). PDL-1 upregulation on monocytes and T cells by HIV via type I interferon: restricted expression of type I interferon receptor by CCR5-expressing leukocytes. Clin. Immunol. 129, 132–144. doi: 10.1016/j.clim.2008.05.009
Boes, M., and Meyer-Wentrup, F. (2015). TLR3 triggering regulates PD-L1 (CD274) expression in human neuroblastoma cells. Cancer Lett. 361, 49–56. doi: 10.1016/j.canlet.2015.02.027
Brett, J., Gerlach, H., Nawroth, P., Steinberg, S., Godman, G., and Stern, D. (1989). Tumor necrosis factor/cachectin increases permeability of endothelial cell monolayers by a mechanism involving regulatory G proteins. J. Exp. Med. 169, 1977–1991.
Brooks, D. G., Ha, S. J., Elsaesser, H., Sharpe, A. H., Freeman, G. J., and Oldstone, M. B. (2008). IL-10 and PD-L1 operate through distinct pathways to suppress T-cell activity during persistent viral infection. Proc. Natl. Acad. Sci. U.S.A. 105, 20428–20433. doi: 10.1073/pnas.0811139106
Brooks, D. G., McGavern, D. B., and Oldstone, M. B. (2006a). Reprogramming of antiviral T cells prevents inactivation and restores T cell activity during persistent viral infection. J. Clin. Invest. 116, 1675–1685. doi: 10.1172/JCI26856
Brooks, D. G., Trifilo, M. J., Edelmann, K. H., Teyton, L., McGavern, D. B., and Oldstone, M. B. (2006b). Interleukin-10 determines viral clearance or persistence in vivo. Nat. Med. 12, 1301–1309. doi: 10.1038/nm1492
Brown, J. A., Dorfman, D. M., Ma, F. R., Sullivan, E. L., Munoz, O., and Freeman, G. J. (2003). Blockade of programmed death-1 ligands on dendritic cells enhances T cell activation and cytokine production. J. Immunol. 170, 1257–1266. doi: 10.4049/jimmunol.170.3.1257
Brown, K. E., Freeman, G. J., Wherry, E. J., and Sharpe, A. H. (2010). Role of PD-1 in regulating acute infections. Curr. Opin. Immunol. 22, 397–401. doi: 10.1016/j.coi.2010.03.007
Butte, M. J., Keir, M. E., Phamduy, T. B., Sharpe, A. H., and Freeman, G. J. (2007). Programmed death-1 ligand 1 interacts specifically with the B7-1 costimulatory molecule to inhibit T cell responses. Immunity 27, 111–122. doi: 10.1016/j.immuni.2007.05.016
Butte, M. J., Pena-Cruz, V., Kim, M. J., Freeman, G. J., and Sharpe, A. H. (2008). Interaction of human PD-L1 and B7-1. Mol. Immunol. 45, 3567–3572. doi: 10.1016/j.molimm.2008.05.014
Carbone, F. R., and Gebhardt, T. (2014). A neighborhood watch upholds local immune protection. Science 346, 40–41. doi: 10.1126/science.1259925
Channappanavar, R., Twardy, B. S., and Suvas, S. (2012). Blocking of PDL-1 interaction enhances primary and secondary CD8 T cell response to herpes simplex virus-1 infection. PLoS ONE 7:e39757. doi: 10.1371/journal.pone.0039757
Chaudhri, A., Xiao, Y., Klee, A. N., Wang, X., Zhu, B., and Freeman, G. J. (2018). PD-L1 binds to B7-1 only in Cis on the same cell surface. Cancer Immunol. Res. 6, 921–929. doi: 10.1158/2326-6066.CIR-17-0316
Chen, L., and Flies, D. B. (2013). Molecular mechanisms of T cell co-stimulation and co-inhibition. Nat. Rev. Immunol. 13, 227–242. doi: 10.1038/nri3405
Chentoufi, A. A., Kritzer, E., Tran, M. V., Dasgupta, G., Lim, C. H., and BenMohamed, L. (2011). The herpes simplex virus 1 latency-associated transcript promotes functional exhaustion of virus-specific CD8+ T cells in latently infected trigeminal ganglia: a novel immune evasion mechanism. J. Virol. 85, 9127–9138. doi: 10.1128/JVI.00587-11
Chikuma, S., Terawaki, S., Hayashi, T., Nabeshima, R., Yoshida, T., Shibayama, S., et al. (2009). PD-1-mediated suppression of IL-2 production induces CD8+ T cell anergy in vivo. J. Immunol. 182, 6682–6689. doi: 10.4049/jimmunol.0900080
Cole, J. E., Navin, T. J., Cross, A. J., Goddard, M. E., Alexopoulou, L., and Monaco, C. (2011). Unexpected protective role for Toll-like receptor 3 in the arterial wall. Proc. Natl. Acad. Sci. U.S.A. 108, 2372–2377. doi: 10.1073/pnas.1018515108
Cox, M. A., Nechanitzky, R., and Mak, T. W. (2017). Check point inhibitors as therapies for infectious diseases. Curr. Opin. Immunol. 48, 61–67. doi: 10.1016/j.coi.2017.07.016
Crawford, A., Angelosanto, J. M., Kao, C., Doering, T. A., Odorizzi, P. M., and Wherry, E. J. (2014). Molecular and transcriptional basis of CD4(+) T cell dysfunction during chronic infection. Immunity 40, 289–302. doi: 10.1016/j.immuni.2014.01.005
Curiel, T. J., Wei, S., Dong, H., Alvarez, X., Cheng, P., and Zou, W. (2003). Blockade of B7-H1 improves myeloid dendritic cell-mediated antitumor immunity. Nat. Med. 9, 562–567. doi: 10.1038/nm863
David, P., Megger, D. A., Kaiser, T., Werner, T., Liu, J., and Zelinskyy, G. (2019). The PD-1/PD-L1 pathway affects the expansion and function of cytotoxic CD8(+) T cells during an acute retroviral infection. Front. Immunol. 10:54. doi: 10.3389/fimmu.2019.00054
Davidson, S., Maini, M. K., and Wack, A. (2015). Disease-promoting effects of type I interferons in viral, bacterial, and coinfections. J. Interferon Cytokine Res. 35, 252–264. doi: 10.1089/jir.2014.0227
Day, C. L., Kaufmann, D. E., Kiepiela, P., Brown, J. A., Moodley, E. S., and Walker, B. D. (2006). PD-1 expression on HIV-specific T cells is associated with T-cell exhaustion and disease progression. Nature 443, 350–354. doi: 10.1038/nature05115
Doering, T. A., Crawford, A., Angelosanto, J. M., Paley, M. A., Ziegler, C. G., and Wherry, E. J. (2012). Network analysis reveals centrally connected genes and pathways involved in CD8+ T cell exhaustion versus memory. Immunity 37, 1130–1144. doi: 10.1016/j.immuni.2012.08.021
Ejrnaes, M., Filippi, C. M., Martinic, M. M., Ling, E. M., Togher, L. M., and von Herrath, M. G. (2006). Resolution of a chronic viral infection after interleukin-10 receptor blockade. J. Exp. Med. 203, 2461–2472. doi: 10.1084/jem.20061462
Erickson, J. J., Gilchuk, P., Hastings, A. K., Tollefson, S. J., Johnson, M., and Williams, J. V. (2012). Viral acute lower respiratory infections impair CD8+ T cells through PD-1. J. Clin. Invest. 122, 2967–2982. doi: 10.1172/JCI62860
Esensten, J. H., Helou, Y. A., Chopra, G., Weiss, A., and Bluestone, J. A. (2016). CD28 costimulation: from mechanism to therapy. Immunity 44, 973–988. doi: 10.1016/j.immuni.2016.04.020
Fife, B. T., and Pauken, K. E. (2011). The role of the PD-1 pathway in autoimmunity and peripheral tolerance. Ann. N. Y. Acad. Sci. 1217, 45–59. doi: 10.1111/j.1749-6632.2010.05919.x
Francisco, L. M., Sage, P. T., and Sharpe, A. H. (2010). The PD-1 pathway in tolerance and autoimmunity. Immunol. Rev. 236, 219–242. doi: 10.1111/j.1600-065X.2010.00923.x
Francisco, L. M., Salinas, V. H., Brown, K. E., Vanguri, V. K., Freeman, G. J., and Sharpe, A. H. (2009). PD-L1 regulates the development, maintenance, and function of induced regulatory T cells. J. Exp. Med. 206, 3015–3029. doi: 10.1084/jem.20090847
Frebel, H., Nindl, V., Schuepbach, R. A., Braunschweiler, T., Richter, K., and Oxenius, A. (2012). Programmed death 1 protects from fatal circulatory failure during systemic virus infection of mice. J. Exp. Med. 209, 2485–2499. doi: 10.1084/jem.20121015
Galani, I. E., Triantafyllia, V., Eleminiadou, E. E., Koltsida, O., Stavropoulos, A., and Andreakos, E. (2017). Interferon-lambda mediates non-redundant front-line antiviral protection against influenza virus infection without compromising host fitness. Immunity 46, 875–890 e6. doi: 10.1016/j.immuni.2017.04.025
Gallez-Hawkins, G. M., Thao, L., Palmer, J., Dagis, A., Li, X., and Zaia, J. A. (2009). Increased programmed death-1 molecule expression in cytomegalovirus disease and acute graft-versus-host disease after allogeneic hematopoietic cell transplantation. Biol. Blood Marrow Transplant. 15, 872–880. doi: 10.1016/j.bbmt.2009.03.022
Gallimore, A., Glithero, A., Godkin, A., Tissot, A. C., Pluckthun, A., and Zinkernagel, R. (1998). Induction and exhaustion of lymphocytic choriomeningitis virus-specific cytotoxic T lymphocytes visualized using soluble tetrameric major histocompatibility complex class I-peptide complexes. J. Exp. Med. 187, 1383–1393.
Gamradt, P., Laoubi, L., Nosbaum, A., Mutez, V., Lenief, V., Grande, S., et al. (2019). Inhibitory checkpoint receptors control CD8(+) resident memory T cells to prevent skin allergy. J. Allergy Clin. Immunol. 2019:48. doi: 10.1016/j.jaci.2018.11.048
Garcia-Bates, T. M., Palma, M. L., Shen, C., Gambotto, A., Macatangay, B. J. C., and Mailliard, R. B. (2019). Contrasting roles of the PD-1 signaling pathway in dendritic cell-mediated induction and regulation of HIV-1-specific effector T cell functions. J. Virol. 93:5. doi: 10.1128/JVI.02035-18
Garcia-Diaz, A., Shin, D. S., Moreno, B. H., Saco, J., Escuin-Ordinas, H., and Ribas, A. (2017). Interferon receptor signaling pathways regulating PD-L1 and PD-L2 expression. Cell Rep. 19, 1189–1201. doi: 10.1016/j.celrep.2017.04.031
GBD 2015 HIV Collaborators (2016). Estimates of global, regional, and national incidence, prevalence, and mortality of HIV, 1980-2015: the Global Burden of Disease Study 2015. Lancet HIV 3, e361–e387. doi: 10.1016/S2352-3018(16)30087-X
Gebhardt, T., Wakim, L. M., Eidsmo, L., Reading, P. C., Heath, W. R., and Carbone, F. R. (2009). Memory T cells in nonlymphoid tissue that provide enhanced local immunity during infection with herpes simplex virus. Nat. Immunol. 10, 524–530. doi: 10.1038/ni.1718
Good, C., Wells, A. I., and Coyne, C. B. (2019). Type III interferon signaling restricts enterovirus 71 infection of goblet cells. Sci. Adv. 5:eaau4255. doi: 10.1126/sciadv.aau4255
Grakoui, A., John Wherry, E., Hanson, H. L., Walker, C., and Ahmed, R. (2006). Turning on the off switch: regulation of anti-viral T cell responses in the liver by the PD-1/PD-L1 pathway. J. Hepatol. 45, 468–472. doi: 10.1016/j.jhep.2006.07.009
Gupta, N., Hegde, P., Lecerf, M., Nain, M., Kaur, M., Kalia, M., et al. (2014). Japanese encephalitis virus expands regulatory T cells by increasing the expression of PD-L1 on dendritic cells. Eur. J. Immunol. 44, 1363–1374. doi: 10.1002/eji.201343701
Hashimoto, M., Kamphorst, A. O., Im, S. J., Kissick, H. T., Pillai, R. N., and Ahmed, R. (2018). CD8 T cell exhaustion in chronic infection and cancer: opportunities for interventions. Annu. Rev. Med. 69, 301–318. doi: 10.1146/annurev-med-012017-043208
Hombrink, P., Helbig, C., Backer, R. A., Piet, B., Oja, A. E., and van Lier, R. A. (2016). Programs for the persistence, vigilance and control of human CD8(+) lung-resident memory T cells. Nat. Immunol. 17, 1467–1478. doi: 10.1038/ni.3589
Honda, T., Egen, J. G., Lammermann, T., Kastenmuller, W., Torabi-Parizi, P., and Germain, R. N. (2014). Tuning of antigen sensitivity by T cell receptor-dependent negative feedback controls T cell effector function in inflamed tissues. Immunity 40, 235–247. doi: 10.1016/j.immuni.2013.11.017
Host, K. M., Jacobs, S. R., West, J. A., Zhang, Z., Costantini, L. M., and Damania, B. (2017). Kaposi's sarcoma-associated herpesvirus increases PD-L1 and proinflammatory cytokine expression in human monocytes. MBio 8:5. doi: 10.1128/mBio.00917-17
Huang, Z. Y., Xu, P., Li, J. H., Zeng, C. H., Song, H. F., and Wang, X. F. (2017). Clinical significance of dynamics of programmed death ligand-1 expression on circulating CD14(+) monocytes and CD19(+) B cells with the progression of hepatitis B virus infection. Viral Immunol. 30, 224–231. doi: 10.1089/vim.2016.0122
Ishida, Y., Agata, Y., Shibahara, K., and Honjo, T. (1992). Induced expression of PD-1, a novel member of the immunoglobulin gene superfamily, upon programmed cell death. EMBO J. 11, 3887–3895.
Iwai, Y., Terawaki, S., Ikegawa, M., Okazaki, T., and Honjo, T. (2003). PD-1 inhibits antiviral immunity at the effector phase in the liver. J. Exp. Med. 198, 39–50. doi: 10.1084/jem.20022235
Jeon, S., Rowe, A. M., Carroll, K. L., Harvey, S. A. K., and Hendricks, R. L. (2018). PD-L1/B7-H1 inhibits viral clearance by macrophages in HSV-1-infected corneas. J. Immunol. 200, 3711–3719. doi: 10.4049/jimmunol.1700417
Jeon, S., St. Leger, A. J., Cherpes, T. L., Sheridan, B. S., and Hendricks, R. L. (2013). PD-L1/B7-H1 regulates the survival but not the function of CD8+ T cells in herpes simplex virus type 1 latently infected trigeminal ganglia. J. Immunol. 190, 6277–6286. doi: 10.4049/jimmunol.1300582
Jin, H. T., Anderson, A. C., Tan, W. G., West, E. E., Ha, S. J., and Ahmed, R. (2010). Cooperation of Tim-3 and PD-1 in CD8 T-cell exhaustion during chronic viral infection. Proc. Natl. Acad. Sci. U.S.A. 107, 14733–14738. doi: 10.1073/pnas.1009731107
Jones, D., Blackmon, A., Neff, C. P., Palmer, B. E., Gilden, D., and Nagel, M. A. (2016). Varicella-zoster virus downregulates programmed death ligand 1 and major histocompatibility complex class I in human brain vascular adventitial fibroblasts, perineurial cells, and lung fibroblasts. J. Virol. 90, 10527–10534. doi: 10.1128/JVI.01546-16
Jones, D., Como, C. N., Jing, L., Blackmon, A., Neff, C. P., and Nagel, M. A. (2019). Varicella zoster virus productively infects human peripheral blood mononuclear cells to modulate expression of immunoinhibitory proteins and blocking PD-L1 enhances virus-specific CD8+ T cell effector function. PLoS Pathog. 15:e1007650. doi: 10.1371/journal.ppat.1007650
Kahan, S. M., Wherry, E. J., and Zajac, A. J. (2015). T cell exhaustion during persistent viral infections. Virology 479–480, 180–193. doi: 10.1016/j.virol.2014.12.033
Kahan, S. M., and Zajac, A. J. (2019). Immune exhaustion: past lessons and new insights from lymphocytic choriomeningitis virus. Viruses 11:2. doi: 10.3390/v11020156
Kakizaki, M., Yamamoto, Y., Yabuta, S., Kurosaki, N., Kagawa, T., and Kotani, A. (2018). The immunological function of extracellular vesicles in hepatitis B virus-infected hepatocytes. PLoS ONE 13:e0205886. doi: 10.1371/journal.pone.0205886
Kather, A., Raftery, M. J., Devi-Rao, G., Lippmann, J., Giese, T., and Schonrich, G. (2010). Herpes simplex virus type 1 (HSV-1)-induced apoptosis in human dendritic cells as a result of downregulation of cellular FLICE-inhibitory protein and reduced expression of HSV-1 antiapoptotic latency-associated transcript sequences. J. Virol. 84, 1034–1046. doi: 10.1128/JVI.01409-09
Keir, M. E., Butte, M. J., Freeman, G. J., and Sharpe, A. H. (2008). PD-1 and its ligands in tolerance and immunity. Annu. Rev. Immunol. 26, 677–704. doi: 10.1146/annurev.immunol.26.021607.090331
Keir, M. E., Liang, S. C., Guleria, I., Latchman, Y. E., Qipo, A., and Sharpe, A. H. (2006). Tissue expression of PD-L1 mediates peripheral T cell tolerance. J. Exp. Med. 203, 883–895. doi: 10.1084/jem.20051776
Khanna, K. M., Bonneau, R. H., Kinchington, P. R., and Hendricks, R. L. (2003). Herpes simplex virus-specific memory CD8+ T cells are selectively activated and retained in latently infected sensory ganglia. Immunity 18, 593–603. doi: 10.1016/S1074-7613(03)00112-2
Kleinpeter, P., Remy-Ziller, C., Winter, E., Gantzer, M., Nourtier, V., Kempf, J., et al. (2019). By binding CD80 and CD86, the vaccinia virus' M2 protein blocks their interactions with both CD28 and CTLA4 and potentiates CD80's binding to PD-L1. J. Virol. 2019:19. doi: 10.1128/JVI.00207-19
Klenerman, P., and Barnes, E. J. (2017). Immunology taught by rats. Science 357, 129–130. doi: 10.1126/science.aao0184
Koh, K. P., Wang, Y., Yi, T., Shiao, S. L., Lorber, M. I., and Pober, J. S. (2004). T cell-mediated vascular dysfunction of human allografts results from IFN-gamma dysregulation of NO synthase. J. Clin. Invest. 114, 846–856. doi: 10.1172/JCI21767
Kolumam, G. A., Thomas, S., Thompson, L. J., Sprent, J., and Murali-Krishna, K. (2005). Type I interferons act directly on CD8 T cells to allow clonal expansion and memory formation in response to viral infection. J. Exp. Med. 202, 637–650. doi: 10.1084/jem.20050821
Kumar, B. V., Ma, W., Miron, M., Granot, T., Guyer, R. S., and Farber, D. L. (2017). Human tissue-resident memory T cells are defined by core transcriptional and functional signatures in lymphoid and mucosal sites. Cell Rep. 20, 2921–2934. doi: 10.1016/j.celrep.2017.08.078
Lafon, M., Megret, F., Meuth, S. G., Simon, O., Velandia Romero, M. L., and Wiendl, H. (2008). Detrimental contribution of the immuno-inhibitor B7-H1 to rabies virus encephalitis. J. Immunol. 180, 7506–7515. doi: 10.4049/jimmunol.180.11.7506
Lamichhane, P., Karyampudi, L., Shreeder, B., Krempski, J., Bahr, D., Daum, J., et al. (2017). IL10 Release upon PD-1 blockade sustains immunosuppression in ovarian cancer. Cancer Res. 77, 6667–6678. doi: 10.1158/0008-5472.CAN-17-0740
Lazear, H. M., Schoggins, J. W., and Diamond, M. S. (2019). Shared and distinct functions of type I and type III interferons. Immunity 50, 907–923. doi: 10.1016/j.immuni.2019.03.025
Legat, A., Speiser, D. E., Pircher, H., Zehn, D., and Fuertes Marraco, S. A. (2013). Inhibitory receptor expression depends more dominantly on differentiation and activation than “exhaustion” of human CD8 T cells. Front. Immunol. 4:455. doi: 10.3389/fimmu.2013.00455
Lindgren, T., Ahlm, C., Mohamed, N., Evander, M., Ljunggren, H. G., and Bjorkstrom, N. K. (2011). Longitudinal analysis of the human T cell response during acute hantavirus infection. J. Virol. 85, 10252–10260. doi: 10.1128/JVI.05548-11
Liu, T., Khanna, K. M., Chen, X., Fink, D. J., and Hendricks, R. L. (2000). CD8(+) T cells can block herpes simplex virus type 1 (HSV-1) reactivation from latency in sensory neurons. J. Exp. Med. 191, 1459–1466. doi: 10.1084/jem.191.9.1459
Loewendorf, A., Kruger, C., Borst, E. M., Wagner, M., Just, U., and Messerle, M. (2004). Identification of a mouse cytomegalovirus gene selectively targeting CD86 expression on antigen-presenting cells. J. Virol. 78, 13062–13071. doi: 10.1128/JVI.78.23.13062-13071.2004
Loke, P., and Allison, J. P. (2003). PD-L1 and PD-L2 are differentially regulated by Th1 and Th2 cells. Proc. Natl. Acad. Sci. U.S.A. 100, 5336–5341. doi: 10.1073/pnas.0931259100
Mackay, L. K., Wakim, L., van Vliet, C. J., Jones, C. M., Mueller, S. N., and Carbone, F. R. (2012). Maintenance of T cell function in the face of chronic antigen stimulation and repeated reactivation for a latent virus infection. J. Immunol. 188, 2173–2178. doi: 10.4049/jimmunol.1102719
Maier, H., Isogawa, M., Freeman, G. J., and Chisari, F. V. (2007). PD-1:PD-L1 interactions contribute to the functional suppression of virus-specific CD8+ T lymphocytes in the liver. J. Immunol. 178, 2714–2720. doi: 10.4049/jimmunol.178.5.2714
Man, K., Gabriel, S. S., Liao, Y., Gloury, R., Preston, S., and Kallies, A. (2017). Transcription factor IRF4 promotes CD8(+) T cell exhaustion and limits the development of memory-like T cells during chronic infection. Immunity 47, 1129–1141 e5. doi: 10.1016/j.immuni.2017.11.021
Masopust, D., Choo, D., Vezys, V., Wherry, E. J., Duraiswamy, J., and Ahmed, R. (2010). Dynamic T cell migration program provides resident memory within intestinal epithelium. J. Exp. Med. 207, 553–564. doi: 10.1084/jem.20090858
Matloubian, M., Concepcion, R. J., and Ahmed, R. (1994). CD4+ T cells are required to sustain CD8+ cytotoxic T-cell responses during chronic viral infection. J. Virol. 68, 8056–8063.
Matloubian, M., Somasundaram, T., Kolhekar, S. R., Selvakumar, R., and Ahmed, R. (1990). Genetic basis of viral persistence: single amino acid change in the viral glycoprotein affects ability of lymphocytic choriomeningitis virus to persist in adult mice. J. Exp. Med. 172, 1043–1048.
McElroy, A. K., Akondy, R. S., Davis, C. W., Ellebedy, A. H., Mehta, A. K., and Ahmed, R. (2015). Human Ebola virus infection results in substantial immune activation. Proc. Natl. Acad. Sci. U.S.A. 112, 4719–4724. doi: 10.1073/pnas.1502619112
McKendry, R. T., Spalluto, C. M., Burke, H., Nicholas, B., Cellura, D., and Wilkinson, T. M. (2016). Dysregulation of antiviral function of CD8(+) T cells in the chronic obstructive pulmonary disease lung. Role of the PD-1-PD-L1 Axis. Am. J. Respir. Crit. Care Med. 193, 642–651. doi: 10.1164/rccm.201504-0782OC
McKinney, E. F., and Smith, K. G. (2016). T cell exhaustion and immune-mediated disease-the potential for therapeutic exhaustion. Curr. Opin. Immunol. 43, 74–80. doi: 10.1016/j.coi.2016.09.005
McKinney, E. F., and Smith, K. G. C. (2018). Metabolic exhaustion in infection, cancer and autoimmunity. Nat. Immunol. 19, 213–221. doi: 10.1038/s41590-018-0045-y
McNally, B., Ye, F., Willette, M., and Flano, E. (2013). Local blockade of epithelial PDL-1 in the airways enhances T cell function and viral clearance during influenza virus infection. J. Virol. 87, 12916–12924. doi: 10.1128/JVI.02423-13
Meier, A., Bagchi, A., Sidhu, H. K., Alter, G., Suscovich, T. J., and Altfeld, M. (2008). Upregulation of PD-L1 on monocytes and dendritic cells by HIV-1 derived TLR ligands. AIDS 22, 655–658. doi: 10.1097/QAD.0b013e3282f4de23
Menicucci, A. R., Versteeg, K., Woolsey, C., Mire, C. E., Geisbert, J. B., and Messaoudi, I. (2017). Transcriptome analysis of circulating immune cell subsets highlight the role of monocytes in zaire ebola virus makona pathogenesis. Front. Immunol. 8:1372. doi: 10.3389/fimmu.2017.01372
Moskophidis, D., Lechner, F., Pircher, H., and Zinkernagel, R. M. (1993). Virus persistence in acutely infected immunocompetent mice by exhaustion of antiviral cytotoxic effector T cells. Nature 362, 758–761. doi: 10.1038/362758a0
Mueller, S. N., Vanguri, V. K., Ha, S. J., West, E. E., Keir, M. E., and Ahmed, R. (2010). PD-L1 has distinct functions in hematopoietic and nonhematopoietic cells in regulating T cell responses during chronic infection in mice. J. Clin. Invest. 120, 2508–2515. doi: 10.1172/JCI40040
Muhlbauer, M., Fleck, M., Schutz, C., Weiss, T., Froh, M., Blank, C., et al. (2006). PD-L1 is induced in hepatocytes by viral infection and by interferon-alpha and -gamma and mediates T cell apoptosis. J. Hepatol. 45, 520–528. doi: 10.1016/j.jhep.2006.05.007
Muller, A., Schmitt, L., Raftery, M., and Schonrich, G. (1998). Paralysis of B7 co-stimulation through the effect of viral IL-10 on T cells as a mechanism of local tolerance induction. Eur. J. Immunol. 28, 3488–3498. doi: 10.1002/(SICI)1521-4141(199811)28:11<3488::AID-IMMU3488>3.0.CO;2-Y
Muller, D. B., Raftery, M. J., Kather, A., Giese, T., and Schonrich, G. (2004). Frontline: Induction of apoptosis and modulation of c-FLIPL and p53 in immature dendritic cells infected with herpes simplex virus. Eur. J. Immunol. 34, 941–951. doi: 10.1002/eji.200324509
Mylvaganam, G. H., Chea, L. S., Tharp, G. K., Hicks, S., Velu, V., and Amara, R. R. (2018). Combination anti-PD-1 and antiretroviral therapy provides therapeutic benefit against SIV. JCI Insight 3:18. doi: 10.1172/jci.insight.122940
Ng, C. T., Snell, L. M., Brooks, D. G., and Oldstone, M. B. (2013). Networking at the level of host immunity: immune cell interactions during persistent viral infections. Cell Host Microbe 13, 652–664. doi: 10.1016/j.chom.2013.05.014
Nightingale, Z. D., Patkar, C., and Rothman, A. L. (2008). Viral replication and paracrine effects result in distinct, functional responses of dendritic cells following infection with dengue 2 virus. J. Leukoc. Biol. 84, 1028–1038. doi: 10.1189/jlb.0208105
Nishimura, H., Nose, M., Hiai, H., Minato, N., and Honjo, T. (1999). Development of lupus-like autoimmune diseases by disruption of the PD-1 gene encoding an ITIM motif-carrying immunoreceptor. Immunity 11, 141–151.
Nishimura, H., Okazaki, T., Tanaka, Y., Nakatani, K., Hara, M., Matsumori, A., et al. (2001). Autoimmune dilated cardiomyopathy in PD-1 receptor-deficient mice. Science 291, 319–322. doi: 10.1126/science.291.5502.319
Odorizzi, P. M., Pauken, K. E., Paley, M. A., Sharpe, A., and Wherry, E. J. (2015). Genetic absence of PD-1 promotes accumulation of terminally differentiated exhausted CD8+ T cells. J. Exp. Med. 212, 1125–1137. doi: 10.1084/jem.20142237
Okabayashi, T., Kojima, T., Masaki, T., Yokota, S., Imaizumi, T., Tsutsumi, H., et al. (2011). Type-III interferon, not type-I, is the predominant interferon induced by respiratory viruses in nasal epithelial cells. Virus Res. 160, 360–366. doi: 10.1016/j.virusres.2011.07.011
Okazaki, T., Chikuma, S., Iwai, Y., Fagarasan, S., and Honjo, T. (2013). A rheostat for immune responses: the unique properties of PD-1 and their advantages for clinical application. Nat. Immunol. 14, 1212–1218. doi: 10.1038/ni.2762
Okazaki, T., and Honjo, T. (2006). The PD-1-PD-L pathway in immunological tolerance. Trends Immunol. 27, 195–201. doi: 10.1016/j.it.2006.02.001
Omilusik, K. D., and Goldrath, A. W. (2017). The origins of memory T cells. Nature 552, 337–339. doi: 10.1038/d41586-017-08280-8
Ong, E. Z., Chan, K. R., and Ooi, E. E. (2016). Viral manipulation of host inhibitory receptor signaling for immune evasion. PLoS Pathog. 12:e1005776. doi: 10.1371/journal.ppat.1005776
Ott, J. J., Stevens, G. A., Groeger, J., and Wiersma, S. T. (2012). Global epidemiology of hepatitis B virus infection: new estimates of age-specific HBsAg seroprevalence and endemicity. Vaccine 30, 2212–2219. doi: 10.1016/j.vaccine.2011.12.116
Ouyang, P., Rakus, K., van Beurden, S. J., Westphal, A. H., Davison, A. J., and Vanderplasschen, A. F. (2014). IL-10 encoded by viruses: a remarkable example of independent acquisition of a cellular gene by viruses and its subsequent evolution in the viral genome. J. Gen. Virol. 95(Pt 2), 245–262. doi: 10.1099/vir.0.058966-0
Ouyang, W., Rutz, S., Crellin, N. K., Valdez, P. A., and Hymowitz, S. G. (2011). Regulation and functions of the IL-10 family of cytokines in inflammation and disease. Annu. Rev. Immunol. 29, 71–109. doi: 10.1146/annurev-immunol-031210-101312
Paessler, S., and Walker, D. H. (2013). Pathogenesis of the viral hemorrhagic fevers. Annu. Rev. Pathol. 8, 411–440. doi: 10.1146/annurev-pathol-020712-164041
Paley, M. A., Kroy, D. C., Odorizzi, P. M., Johnnidis, J. B., Dolfi, D. V., and Wherry, E. J. (2012). Progenitor and terminal subsets of CD8+ T cells cooperate to contain chronic viral infection. Science 338, 1220–1225. doi: 10.1126/science.1229620
Pallett, L. J., Davies, J., Colbeck, E. J., Robertson, F., Hansi, N., and Maini, M. K. (2017). IL-2(high) tissue-resident T cells in the human liver: sentinels for hepatotropic infection. J. Exp. Med. 214, 1567–1580. doi: 10.1084/jem.20162115
Pauken, K. E., Sammons, M. A., Odorizzi, P. M., Manne, S., Godec, J., and Wherry, E. J. (2016). Epigenetic stability of exhausted T cells limits durability of reinvigoration by PD-1 blockade. Science 354, 1160–1165. doi: 10.1126/science.aaf2807
Petrelli, A., Mijnheer, G., van Konijnenburg, D. P. H., van der Wal, M. M., Giovannone, B., Mocholi, E., et al. (2018). PD-1+CD8+ T cells are clonally expanding effectors in human chronic inflammation. J. Clin. Invest. 2018:6107. doi: 10.1172/JCI96107
Petrovas, C., Casazza, J. P., Brenchley, J. M., Price, D. A., Gostick, E., and Koup, R. A. (2006). PD-1 is a regulator of virus-specific CD8+ T cell survival in HIV infection. J. Exp. Med. 203, 2281–2292. doi: 10.1084/jem.20061496
Planes, R., BenMohamed, L., Leghmari, K., Delobel, P., Izopet, J., and Bahraoui, E. (2014). HIV-1 Tat protein induces PD-L1 (B7-H1) expression on dendritic cells through tumor necrosis factor alpha- and toll-like receptor 4-mediated mechanisms. J. Virol. 88, 6672–6689. doi: 10.1128/JVI.00825-14
Probst, H. C., McCoy, K., Okazaki, T., Honjo, T., and van den Broek, M. (2005). Resting dendritic cells induce peripheral CD8+ T cell tolerance through PD-1 and CTLA-4. Nat. Immunol. 6, 280–286. doi: 10.1038/ni1165
Pulko, V., Liu, X., Krco, C. J., Harris, K. J., Frigola, X., and Dong, H. (2009). TLR3-stimulated dendritic cells up-regulate B7-H1 expression and influence the magnitude of CD8 T cell responses to tumor vaccination. J. Immunol. 183, 3634–3641. doi: 10.4049/jimmunol.0900974
Raftery, M., Muller, A., and Schonrich, G. (2000). Herpesvirus homologues of cellular genes. Virus Genes 21, 65–75. doi: 10.1023/A:1008184330127
Raftery, M. J., Abdelaziz, M. O., Hofmann, J., and Schonrich, G. (2018). Hantavirus-driven PD-L1/PD-L2 upregulation: an imperfect viral immune evasion mechanism. Front. Immunol. 9:2560. doi: 10.3389/fimmu.2018.02560
Raftery, M. J., Behrens, C. K., Muller, A., Krammer, P. H., Walczak, H., and Schonrich, G. (1999). Herpes simplex virus type 1 infection of activated cytotoxic T cells: induction of fratricide as a mechanism of viral immune evasion. J. Exp. Med. 190, 1103–1114.
Raftery, M. J., Schwab, M., Eibert, S. M., Samstag, Y., Walczak, H., and Schonrich, G. (2001). Targeting the function of mature dendritic cells by human cytomegalovirus: a multilayered viral defense strategy. Immunity 15, 997–1009. doi: 10.1016/S1074-7613(01)00239-4
Raftery, M. J., Wieland, D., Gronewald, S., Kraus, A. A., Giese, T., and Schonrich, G. (2004). Shaping phenotype, function, and survival of dendritic cells by cytomegalovirus-encoded IL-10. J. Immunol. 173, 3383–3391. doi: 10.4049/jimmunol.173.5.3383
Rodriguez-Garcia, M., Porichis, F., de Jong, O. G., Levi, K., Diefenbach, T. J., and Kavanagh, D. G. (2011). Expression of PD-L1 and PD-L2 on human macrophages is up-regulated by HIV-1 and differentially modulated by IL-10. J. Leukoc. Biol. 89, 507–515. doi: 10.1189/jlb.0610327
Rota, G., Niogret, C., Dang, A. T., Barros, C. R., Fonta, N. P., and Guarda, G. (2018). Shp-2 is dispensable for establishing T cell exhaustion and for PD-1 signaling in vivo. Cell Rep. 23, 39–49. doi: 10.1016/j.celrep.2018.03.026
Ruibal, P., Oestereich, L., Ludtke, A., Becker-Ziaja, B., Wozniak, D. M., and Munoz-Fontela, C. (2016). Unique human immune signature of Ebola virus disease in Guinea. Nature 533, 100–104. doi: 10.1038/nature17949
Rutigliano, J. A., Sharma, S., Morris, M. Y., Oguin, T. H. III., McClaren, J. L., and Thomas, P. G. (2014). Highly pathological influenza A virus infection is associated with augmented expression of PD-1 by functionally compromised virus-specific CD8+ T cells. J. Virol. 88, 1636–1651. doi: 10.1128/JVI.02851-13
Saeidi, A., Zandi, K., Cheok, Y. Y., Saeidi, H., Wong, W. F., and Shankar, E. M. (2018). T-cell exhaustion in chronic infections: reversing the state of exhaustion and reinvigorating optimal protective immune responses. Front. Immunol. 9:2569. doi: 10.3389/fimmu.2018.02569
Sallusto, F., Lenig, D., Forster, R., Lipp, M., and Lanzavecchia, A. (1999). Two subsets of memory T lymphocytes with distinct homing potentials and effector functions. Nature 401, 708–712. doi: 10.1038/44385
Sanmamed, M. F., and Chen, L. (2018). A paradigm shift in cancer immunotherapy: from enhancement to normalization. Cell 175, 313–326. doi: 10.1016/j.cell.2018.09.035
Schenkel, J. M., Fraser, K. A., Beura, L. K., Pauken, K. E., Vezys, V., and Masopust, D. (2014). Resident memory CD8 T cells trigger protective innate and adaptive immune responses. Science 346, 98–101. doi: 10.1126/science.1254536
Schenkel, J. M., Fraser, K. A., Vezys, V., and Masopust, D. (2013). Sensing and alarm function of resident memory CD8(+) T cells. Nat. Immunol. 14, 509–513. doi: 10.1038/ni.2568
Schonrich, G., Abdelaziz, M. O., and Raftery, M. J. (2017). Herpesviral capture of immunomodulatory host genes. Virus Genes 53, 762–773. doi: 10.1007/s11262-017-1460-0
Schonrich, G., Rang, A., Lutteke, N., Raftery, M. J., Charbonnel, N., and Ulrich, R. G. (2008). Hantavirus-induced immunity in rodent reservoirs and humans. Immunol. Rev. 225, 163–189. doi: 10.1111/j.1600-065X.2008.00694.x
Schuren, A. B., Costa, A. I., and Wiertz, E. J. (2016). Recent advances in viral evasion of the MHC Class I processing pathway. Curr. Opin. Immunol. 40, 43–50. doi: 10.1016/j.coi.2016.02.007
Schurich, A., Pallett, L. J., Jajbhay, D., Wijngaarden, J., Otano, I., and Maini, M. K. (2016). Distinct metabolic requirements of exhausted and functional virus-specific CD8 T cells in the same host. Cell Rep. 16, 1243–1252. doi: 10.1016/j.celrep.2016.06.078
Schweitzer, A., Horn, J., Mikolajczyk, R. T., Krause, G., and Ott, J. J. (2015). Estimations of worldwide prevalence of chronic hepatitis B virus infection: a systematic review of data published between 1965 and 2013. Lancet 386, 1546–1555. doi: 10.1016/S0140-6736(15)61412-X
Selenko-Gebauer, N., Majdic, O., Szekeres, A., Hofler, G., Guthann, E., Korthauer, U., et al. (2003). B7-H1 (programmed death-1 ligand) on dendritic cells is involved in the induction and maintenance of T cell anergy. J. Immunol. 170, 3637–3644. doi: 10.4049/jimmunol.170.7.3637
Sen, D. R., Kaminski, J., Barnitz, R. A., Kurachi, M., Gerdemann, U., and Haining, W. N. (2016). The epigenetic landscape of T cell exhaustion. Science 354, 1165–1169. doi: 10.1126/science.aae0491
Shaabani, N., Duhan, V., Khairnar, V., Gassa, A., Ferrer-Tur, R., Haussinger, D., et al. (2016). CD169(+) macrophages regulate PD-L1 expression via type I interferon and thereby prevent severe immunopathology after LCMV infection. Cell Death Dis. 7:e2446. doi: 10.1038/cddis.2016.350
Sharpe, A. H., and Pauken, K. E. (2018). The diverse functions of the PD1 inhibitory pathway. Nat. Rev. Immunol. 18, 153–167. doi: 10.1038/nri.2017.108
Sharpe, A. H., Wherry, E. J., Ahmed, R., and Freeman, G. J. (2007). The function of programmed cell death 1 and its ligands in regulating autoimmunity and infection. Nat. Immunol. 8, 239–245. doi: 10.1038/ni1443
Shin, H. (2018). Formation and function of tissue-resident memory T cells during viral infection. Curr. Opin. Virol. 28, 61–67. doi: 10.1016/j.coviro.2017.11.001
Shin, H., Blackburn, S. D., Blattman, J. N., and Wherry, E. J. (2007). Viral antigen and extensive division maintain virus-specific CD8 T cells during chronic infection. J. Exp. Med. 204, 941–949. doi: 10.1084/jem.20061937
Shwetank, Abdelsamed, H. A., Frost, E. L., Schmitz, H. M., Mockus, T. E., Youngblood, B. A., et al. (2017). Maintenance of PD-1 on brain-resident memory CD8 T cells is antigen independent. Immunol. Cell Biol. 95, 953–959. doi: 10.1038/icb.2017.62
Siegal, F. P., Kadowaki, N., Shodell, M., Fitzgerald-Bocarsly, P. A., Shah, K., and Liu, Y. J. (1999). The nature of the principal type 1 interferon-producing cells in human blood. Science 284, 1835–1837.
Smolders, J., Heutinck, K. M., Fransen, N. L., Remmerswaal, E. B. M., Hombrink, P., and Hamann, J. (2018). Tissue-resident memory T cells populate the human brain. Nat. Commun. 9:4593. doi: 10.1038/s41467-018-07053-9
Speiser, D. E., Utzschneider, D. T., Oberle, S. G., Munz, C., Romero, P., and Zehn, D. (2014). T cell differentiation in chronic infection and cancer: functional adaptation or exhaustion? Nat. Rev. Immunol. 14, 768–774. doi: 10.1038/nri3740
Stanaway, J. D., Flaxman, A. D., Naghavi, M., Fitzmaurice, C., Vos, T., Abubakar, I., et al. (2016). The global burden of viral hepatitis from 1990 to 2013: findings from the Global Burden of Disease Study 2013. Lancet 388, 1081–1088. doi: 10.1016/S0140-6736(16)30579-7
Stanciu, L. A., Bellettato, C. M., Laza-Stanca, V., Coyle, A. J., Papi, A., and Johnston, S. L. (2006). Expression of programmed death-1 ligand (PD-L) 1, PD-L2, B7-H3, and inducible costimulator ligand on human respiratory tract epithelial cells and regulation by respiratory syncytial virus and type 1 and 2 cytokines. J. Infect. Dis. 193, 404–412. doi: 10.1086/499275
Staples, K. J., Nicholas, B., McKendry, R. T., Spalluto, C. M., Wallington, J. C., and Wilkinson, T. M. (2015). Viral infection of human lung macrophages increases PDL1 expression via IFNbeta. PLoS ONE 10:e0121527. doi: 10.1371/journal.pone.0121527
Staron, M. M., Gray, S. M., Marshall, H. D., Parish, I. A., Chen, J. H., and Kaech, S. M. (2014). The transcription factor FoxO1 sustains expression of the inhibitory receptor PD-1 and survival of antiviral CD8(+) T cells during chronic infection. Immunity 41, 802–814. doi: 10.1016/j.immuni.2014.10.013
Stelekati, E., Chen, Z., Manne, S., Kurachi, M., Ali, M. A., and Wherry, E. J. (2018). Long-term persistence of exhausted CD8 T cells in chronic infection is regulated by microRNA-155. Cell Rep. 23, 2142–2156. doi: 10.1016/j.celrep.2018.04.038
Sugiura, D., Maruhashi, T., Okazaki, I. M., Shimizu, K., Maeda, T. K., and Okazaki, T. (2019). Restriction of PD-1 function by cis-PD-L1/CD80 interactions is required for optimal T cell responses. Science 2019:7062. doi: 10.1126/science.aav7062
Sun, C., Mezzadra, R., and Schumacher, T. N. (2018). Regulation and function of the PD-L1 checkpoint. Immunity 48, 434–452. doi: 10.1016/j.immuni.2018.03.014
Sun, Z., Fourcade, J., Pagliano, O., Chauvin, J. M., Sander, C., and Zarour, H. M. (2015). IL10 and PD-1 cooperate to limit the activity of tumor-specific CD8+ T cells. Cancer Res. 75, 1635–1644. doi: 10.1158/0008-5472.CAN-14-3016
Surh, C. D., and Sprent, J. (2008). Homeostasis of naive and memory T cells. Immunity 29, 848–862. doi: 10.1016/j.immuni.2008.11.002
Szabo, P. A., Miron, M., and Farber, D. L. (2019). Location, location, location: tissue resident memory T cells in mice and humans. Sci. Immunol. 4:34. doi: 10.1126/sciimmunol.aas9673
Teijaro, J. R., Ng, C., Lee, A. M., Sullivan, B. M., Sheehan, K. C., Welch, M., et al. (2013). Persistent LCMV infection is controlled by blockade of type I interferon signaling. Science 340, 207–211. doi: 10.1126/science.1235214
Telcian, A. G., Laza-Stanca, V., Edwards, M. R., Harker, J. A., Wang, H., and Johnston, S. L. (2011). RSV-induced bronchial epithelial cell PD-L1 expression inhibits CD8+ T cell nonspecific antiviral activity. J. Infect. Dis. 203, 85–94. doi: 10.1093/infdis/jiq020
Terawaki, S., Chikuma, S., Shibayama, S., Hayashi, T., Yoshida, T., Okazaki, T., et al. (2011). IFN-alpha directly promotes programmed cell death-1 transcription and limits the duration of T cell-mediated immunity. J. Immunol. 186, 2772–2779. doi: 10.4049/jimmunol.1003208
Theobald, S. J., Khailaie, S., Meyer-Hermann, M., Volk, V., Olbrich, H., and Stripecke, R. (2018). Signatures of T and B cell development, functional responses and PD-1 upregulation after HCMV latent infections and reactivations in Nod.Rag.gamma mice humanized with cord blood CD34(+) cells. Front. Immunol. 9:2734. doi: 10.3389/fimmu.2018.02734
Trautmann, L., Janbazian, L., Chomont, N., Said, E. A., Gimmig, S., and Sekaly, R. P. (2006). Upregulation of PD-1 expression on HIV-specific CD8+ T cells leads to reversible immune dysfunction. Nat. Med. 12, 1198–1202. doi: 10.1038/nm1482
Tsuda, M., Matsumoto, K., Inoue, H., Matsumura, M., Nakano, T., Mori, A., et al. (2005). Expression of B7-H1 and B7-DC on the airway epithelium is enhanced by double-stranded RNA. Biochem. Biophys. Res. Commun. 330, 263–270. doi: 10.1016/j.bbrc.2005.02.161
Tu, Z., Pierce, R. H., Kurtis, J., Kuroki, Y., Crispe, I. N., and Orloff, M. S. (2010). Hepatitis C virus core protein subverts the antiviral activities of human Kupffer cells. Gastroenterology 138, 305–314. doi: 10.1053/j.gastro.2009.09.009
Turner, S. J., and Russ, B. E. (2016). Can T cells be too exhausted to fight back? Science 354, 1104–1105. doi: 10.1126/science.aal3204
Utzschneider, D. T., Alfei, F., Roelli, P., Barras, D., Chennupati, V., and Zehn, D. (2016). High antigen levels induce an exhausted phenotype in a chronic infectiwithout impairing T cell expansion and survival. J. Exp. Med. 213, 1819–1834. doi: 10.1084/jem.20150598
Utzschneider, D. T., Legat, A., Fuertes Marraco, S. A., Carrie, L., Luescher, I., and Zehn, D. (2013). T cells maintain an exhausted phenotype after antigen withdrawal and population reexpansion. Nat. Immunol. 14, 603–610. doi: 10.1038/ni.2606
Valero-Pacheco, N., Arriaga-Pizano, L., Ferat-Osorio, E., Mora-Velandia, L. M., Pastelin-Palacios, R., and Lopez-Macias, C. (2013). PD-L1 expression induced by the 2009 pandemic influenza A(H1N1) virus impairs the human T cell response. Clin. Dev. Immunol. 2013:989673. doi: 10.1155/2013/989673
Veiga-Parga, T., Sehrawat, S., and Rouse, B. T. (2013). Role of regulatory T cells during virus infection. Immunol. Rev. 255, 182–196. doi: 10.1111/imr.12085
Velu, V., Shetty, R. D., Larsson, M., and Shankar, E. M. (2015). Role of PD-1 co-inhibitory pathway in HIV infection and potential therapeutic options. Retrovirology 12:14. doi: 10.1186/s12977-015-0144-x
Velu, V., Titanji, K., Zhu, B., Husain, S., Pladevega, A., Lai, L., et al. (2009). Enhancing SIV-specific immunity in vivo by PD-1 blockade. Nature 458, 206–210. doi: 10.1038/nature07662
Verjans, G. M., Hintzen, R. Q., van Dun, J. M., Poot, A., Milikan, J. C., and Osterhaus, A. D. (2007). Selective retention of herpes simplex virus-specific T cells in latently infected human trigeminal ganglia. Proc. Natl. Acad. Sci. U.S.A. 104, 3496–3501. doi: 10.1073/pnas.0610847104
Virgin, H. W., Wherry, E. J., and Ahmed, R. (2009). Redefining chronic viral infection. Cell 138, 30–50. doi: 10.1016/j.cell.2009.06.036
Wack, A., Terczynska-Dyla, E., and Hartmann, R. (2015). Guarding the frontiers: the biology of type III interferons. Nat. Immunol. 16, 802–809. doi: 10.1038/ni.3212
Wakim, L. M., Waithman, J., van Rooijen, N., Heath, W. R., and Carbone, F. R. (2008). Dendritic cell-induced memory T cell activation in nonlymphoid tissues. Science 319, 198–202. doi: 10.1126/science.1151869
Wang, J., Yoshida, T., Nakaki, F., Hiai, H., Okazaki, T., and Honjo, T. (2005). Establishment of NOD-Pdcd1-/- mice as an efficient animal model of type I diabetes. Proc. Natl. Acad. Sci. U.S.A. 102, 11823–11828. doi: 10.1073/pnas.0505497102
Wang, X., Zhang, Z., Zhang, S., Fu, J., Yao, J., Jiao, Y., et al. (2008). B7-H1 up-regulation impairs myeloid DC and correlates with disease progression in chronic HIV-1 infection. Eur. J. Immunol. 38, 3226–3236. doi: 10.1002/eji.200838285
Wei, F., Zhong, S., Ma, Z., Kong, H., Medvec, A., Ahmed, R., et al. (2013). Strength of PD-1 signaling differentially affects T-cell effector functions. Proc. Natl. Acad. Sci. U.S.A. 110, E2480–E2489. doi: 10.1073/pnas.1305394110
Welsh, R. M., and Seedhom, M. O. (2008). Lymphocytic choriomeningitis virus (LCMV): propagation, quantitation, and storage. Curr. Protoc. Microbiol. 15A:1. doi: 10.1002/9780471729259.mc15a01s8
Wherry, E. J., and Ahmed, R. (2004). Memory CD8 T-cell differentiation during viral infection. J. Virol. 78, 5535–5545. doi: 10.1128/JVI.78.11.5535-5545.2004
Wherry, E. J., Barber, D. L., Kaech, S. M., Blattman, J. N., and Ahmed, R. (2004). Antigen-independent memory CD8 T cells do not develop during chronic viral infection. Proc. Natl. Acad. Sci. U.S.A. 101, 16004–16009. doi: 10.1073/pnas.0407192101
Wherry, E. J., Blattman, J. N., Murali-Krishna, K., van der Most, R, and Ahmed, R. (2003). Viral persistence alters CD8 T-cell immunodominance and tissue distribution and results in distinct stages of functional impairment. J. Virol. 77, 4911–4927. doi: 10.1128/jvi.77.8.4911-4927.2003
Wherry, E. J., Ha, S. J., Kaech, S. M., Haining, W. N., Sarkar, S., and Ahmed, R. (2007). Molecular signature of CD8+ T cell exhaustion during chronic viral infection. Immunity 27, 670–684. doi: 10.1016/j.immuni.2007.09.006
Wherry, E. J., and Kurachi, M. (2015). Molecular and cellular insights into T cell exhaustion. Nat. Rev. Immunol. 15, 486–499. doi: 10.1038/nri3862
Wieland, D., Kemming, J., Schuch, A., Emmerich, F., Knolle, P., Neumann-Haefelin, C., et al. (2017). TCF1(+) hepatitis C virus-specific CD8(+) T cells are maintained after cessation of chronic antigen stimulation. Nat. Commun. 8:15050. doi: 10.1038/ncomms15050
Wilson, E. B., Yamada, D. H., Elsaesser, H., Herskovitz, J., Deng, J., Cheng, G., et al. (2013). Blockade of chronic type I interferon signaling to control persistent LCMV infection. Science 340, 202–207. doi: 10.1126/science.1235208
Wolchok, J. (2018). Putting the immunologic brakes on cancer. Cell 175, 1452–1454. doi: 10.1016/j.cell.2018.11.006
Wu, X., Wu, P., Shen, Y., Jiang, X., and Xu, F. (2018). CD8(+) resident memory T cells and viral infection. Front. Immunol. 9:2093. doi: 10.3389/fimmu.2018.02093
Wykes, M. N., and Lewin, S. R. (2018). Immune checkpoint blockade in infectious diseases. Nat. Rev. Immunol. 18, 91–104. doi: 10.1038/nri.2017.112
Xiao, Y., Yu, S., Zhu, B., Bedoret, D., Bu, X., Francisco, L., et al. (2014). RGMb is a novel binding partner for PD-L2 and its engagement with PD-L2 promotes respiratory tolerance. J. Exp. Med. 211, 943–959. doi: 10.1084/jem.20130790
Xu, H., Wang, X., Pahar, B., Moroney-Rasmussen, T., Alvarez, X., Lackner, A., et al. (2010). Increased B7-H1 expression on dendritic cells correlates with programmed death 1 expression on T cells in simian immunodeficiency virus-infected macaques and may contribute to T cell dysfunction and disease progression. J. Immunol. 185, 7340–7348. doi: 10.4049/jimmunol.1001642
Yamazaki, T., Akiba, H., Iwai, H., Matsuda, H., Aoki, M., Tanno, Y., et al. (2002). Expression of programmed death 1 ligands by murine T cells and AP. J. Immunol. 169, 5538–5545. doi: 10.4049/jimmunol.169.10.5538
Youngblood, B., Hale, J. S., Kissick, H. T., Ahn, E., Xu, X., and Ahmed, R. (2017). Effector CD8 T cells dedifferentiate into long-lived memory cells. Nature 552, 404–409. doi: 10.1038/nature25144
Youngblood, B., Oestreich, K. J., Ha, S. J., Duraiswamy, J., Akondy, R. S., and Ahmed, R. (2011). Chronic virus infection enforces demethylation of the locus that encodes PD-1 in antigen-specific CD8(+) T cells. Immunity 35, 400–412. doi: 10.1016/j.immuni.2011.06.015
Zajac, A. J., Blattman, J. N., Murali-Krishna, K., Sourdive, D. J., Suresh, M., and Ahmed, R. (1998). Viral immune evasion due to persistence of activated T cells without effector function. J. Exp. Med. 188, 2205–2213.
Zampieri, C. A., Sullivan, N. J., and Nabel, G. J. (2007). Immunopathology of highly virulent pathogens: insights from Ebola virus. Nat. Immunol. 8, 1159–1164. doi: 10.1038/ni1519
Zanoni, I., Granucci, F., and Broggi, A. (2017). Interferon (IFN)-lambda takes the helm: immunomodulatory roles of type III IFNs. Front. Immunol. 8:1661. doi: 10.3389/fimmu.2017.01661
Zehn, D., Utzschneider, D. T., and Thimme, R. (2016). Immune-surveillance through exhausted effector T-cells. Curr. Opin. Virol. 16, 49–54. doi: 10.1016/j.coviro.2016.01.002
Zehn, D., and Wherry, E. J. (2015). Immune memory and exhaustion: clinically relevant lessons from the LCMV model. Adv. Exp. Med. Biol. 850, 137–152. doi: 10.1007/978-3-319-15774-0_10
Zhai, N., Li, H., Song, H., Yang, Y., Cui, A., Li, T., et al. (2017). Hepatitis C virus induces MDSCs-like monocytes through TLR2/PI3K/AKT/STAT3 signaling. PLoS ONE 12:e0170516. doi: 10.1371/journal.pone.0170516
Zhang, N., and Bevan, M. J. (2011). CD8(+) T cells: foot soldiers of the immune system. Immunity 35, 161–168. doi: 10.1016/j.immuni.2011.07.010
Keywords: PD-1, PD-L1, PD-L2, antiviral immune responses, viral immune evasion, virus-induced immunopathogenesis, viruses
Citation: Schönrich G and Raftery MJ (2019) The PD-1/PD-L1 Axis and Virus Infections: A Delicate Balance. Front. Cell. Infect. Microbiol. 9:207. doi: 10.3389/fcimb.2019.00207
Received: 31 January 2019; Accepted: 27 May 2019;
Published: 13 June 2019.
Edited by:
Aleem Siddiqui, University of California, San Diego, United StatesReviewed by:
Namir Shaabani, The Scripps Research Institute, United StatesMasanori Isogawa, Nagoya City University, Japan
Copyright © 2019 Schönrich and Raftery. This is an open-access article distributed under the terms of the Creative Commons Attribution License (CC BY). The use, distribution or reproduction in other forums is permitted, provided the original author(s) and the copyright owner(s) are credited and that the original publication in this journal is cited, in accordance with accepted academic practice. No use, distribution or reproduction is permitted which does not comply with these terms.
*Correspondence: Günther Schönrich, Z3VlbnRoZXIuc2Nob2VucmljaEBjaGFyaXRlLmRl