- 1Doctorado en Farmacología, Universidad de Guadalajara, Guadalajara, Mexico
- 2Biotecnología Médica y Farmacéutica, Centro de Investigación y Asistencia en Tecnología y Diseño del Estado de Jalisco, Guadalajara, Mexico
Tuberculosis (TB) remains as the first cause of death among infectious diseases worldwide. Global incidence of tuberculosis is in part coincident with incidence of type 2 diabetes (T2D). Incidence of T2D is recognized as a high-risk factor that may contribute to tuberculosis dissemination. However, mechanisms which favor infection under T2D are just starting to emerge. Here, we first discuss the evidences that are available to support a metabolic connection between TB and T2D. Then, we analyze the evidences of metabolic changes which occur during T2D gathered thus far for its influence on susceptibility to M. tuberculosis infection and TB progression, such as hyperglycemia, increase of 1AC levels, increase of triglycerides levels, reduction of HDL-cholesterol levels, increased concentration of lipoproteins, and modification of the activity of some hormones related to the control of metabolic homeostasis. Finally, we recognize possible advantages of metabolic management of immunity to develop new strategies for treatment, diagnosis, and prevention of tuberculosis.
Introduction
Infection with Mycobacterium tuberculosis, which in susceptible people leads to either active or latent tuberculosis (TB), remains as a high-burden health problem globally. It is calculated that in 2017, TB caused 1.3 millions deaths, and 10.0 million new cases were reported (WHO, 2018). Some disorders have been recognized as risk factors to develop pulmonary TB, such as HIV coinfection, malnutrition, tobacco smoking, and type 2 diabetes (T2D) (WHO, 2018).
T2D is a chronic metabolic disorder that essentially affect the function of pancreatic β-cells, resulting in progressive development of insuline resistance and chronic inflammation (Defronzo et al., 2015). Several meta-analysis show that T2D is associated with a two- to four-fold increased risk of active TB, even multidrug-resistant TB (Amare et al., 2013; Al-Rifai et al., 2017; Liu et al., 2017; Hayashi and Chandramohan, 2018). As a risk factor for developing TB, T2D has attracted attention by its projected increase and its prevalente worldwide. In 2018, it was estimated that T2D affected one of each 10 individuals globally (425 million people around the world) and it is projected that the number of cases of T2D may increase by 40% in 2045 (IDF, 2017). Hence, an increase in the global burden of T2D poses a higher risk of TB spread worldwide in the upcoming years.
Epidemiological data about comorbidity between TB and T2D show that there is a close relationship between both diseases. A recent meta-analysis showed that 16% of newly-diagnosed TB patients have T2D and up to 4.1% of T2D patients develop TB (Wilkinson et al., 2017). In 2017, close to 800,000 newly-diagnosed TB cases were attributed to T2D, and T2D was the risk factor that contributed to most TB cases in countries like China and India, even above HIV infection (WHO, 2018). However, the incidence of TB-T2D can be higher than reported, because the American Association of Diabetes (ADA) estimates that around 50% of T2D patients remain undiagnosed and the WHO has found higher rates of undiagnosed TB mainly in low-income countries (American Diabetes Association, 2018a; WHO, 2018). Both WHO and the International Union against Tuberculosis and Lung Disease recommend to diagnose T2D in newly-diagnosed TB patients, with the aim of generating pharmacological strategies that effectively contribute to the clinical management of T2D and TB (Liu et al., 2019).
In addition to its importance on TB prevalence, patients with T2D have more severe manifestations of TB than non-T2D ones (Carreira et al., 2012; Figure 1A) and have a lower response to anti-TB treatment (OR 2.93) (Viswanathan and Gawde, 2014) compared to patients without T2D. Moreover, the recurrence and reactivation of LTBI, which potentially contributes to TB dissemination, is higher in T2D patients than in non-T2D ones (OR = 1.83) (Jimenez-Corona et al., 2013).
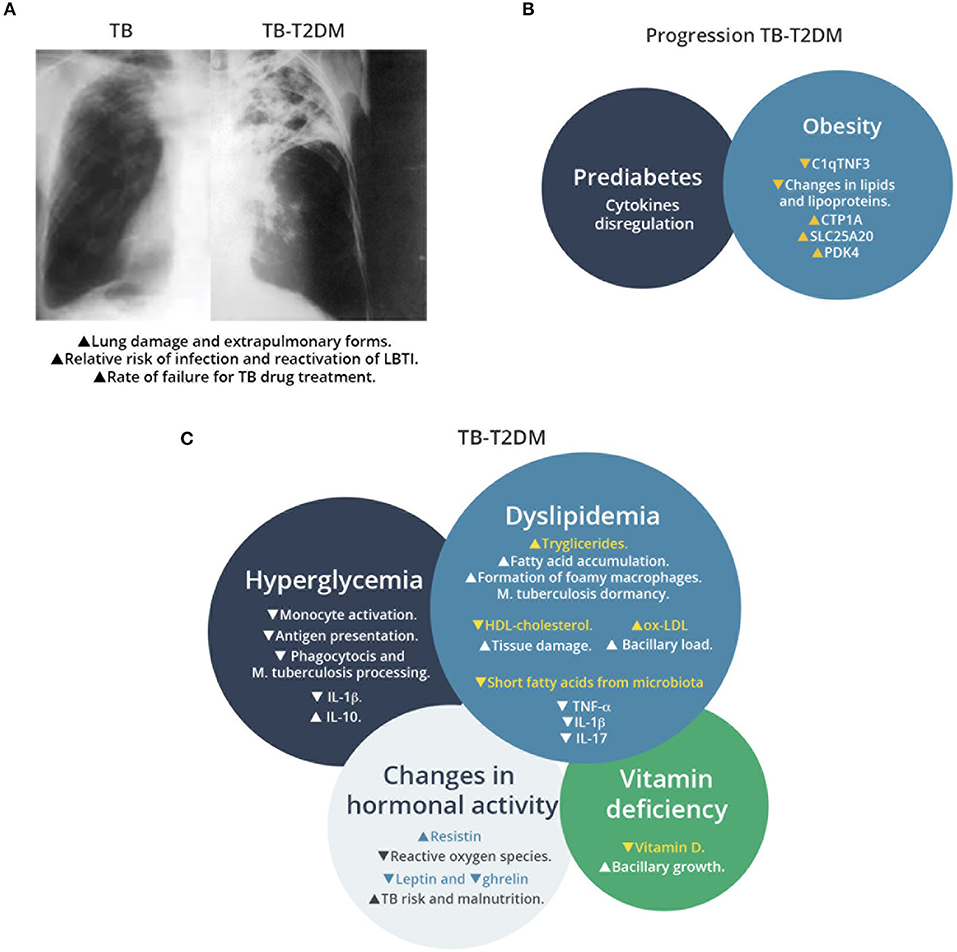
Figure 1. Overview of TB-T2D comorbidity, factors associated to T2D that increase susceptibility to M. tuberculosis infection, and metabolic changes that affect the host immune response. (A) Schematic representation of chest radiography of M. tuberculosis infected lung in patients with or without T2D. Comorbidity increases the lung damage associated to M. tuberculosis infection. (B) Factors associated to progression of TB in T2D patients. Prediabetes and obesity contribute to TB susceptibility with progressive changes in T2D patients, as recent signatures for TB progression have revealed recently. (C) Effects of metabolic changes in TB-T2D on the host response to M. tuberculosis infection. TB-T2D patients suffer hyperglycemia, dyslipidemia, changes in hormonal activity and vitamin deficiency. These conditions result in higher manifestations of M. tuberculosis infection.
Some relevant innate and adaptive immunity mechanisms that are necessaries for M. tuberculosis clearance have been shown to be compromised in patients with TD2M, as recently reviewed (Restrepo, 2016; Kumar Nathella and Babu, 2017; Rao et al., 2019). However, a causal relationship between T2D and TB has not been completely addressed. Even though the role of hyperglycemia on the immunity against M. tuberculosis has been widely described (Magee et al., 2018), new evidences about other metabolic changes that occur during T2D progression are emerging, such as the effects of dyslipidemia, vitamin deficiency, and changes in hormonal activity, which will be discussed in specific sections below. Here, we discuss diverse findings ranging from in vitro studies to clinical research, which support the connection between T2D progression and TB susceptibility in a metabolic-dependent way and present a global connection of feasible events that may occur during this comorbidity, which might create an appropriate environment to M. tuberculosis infection, and we also present evidences of the role of the treatment of T2D on this response.
Prediabetes and Progression to TB
Prediabetes is defined as a stage where glucose levels do not meet with the criteria for T2D but are too high to be considered as normal. Prediabetes is diagnosed by a fasting blood glucose (FBG) values between 100 and 125 mg/dL or 2 h oral glucose tolerance test (OGTT) between 140 and 199 mg/dL or Hemoglobin 1AC between 5.7 and 6.4% (American Diabetes Association, 2019a). Even though a time-regulated interaction between T2D and TB remains unresolved, some evidences suggest that progression from prediabetes to T2D may influence the susceptibility to M. tuberculosis infection (Figure 1B). An study of household contacts of active TB patients (who had a higher risk to develop TB) showed that prediabetes is present in at least 27% of them (Shivakumar et al., 2018), while another study in western India that included 1,073 participants, revealed that more than one-half of newly diagnosed TB patients had T2D or pre-T2D (Mave et al., 2017).
Prediabetes induces changes in cytokines production that are related to control of M. tuberculosis infection. A study in prediabetic-TB patients showed that they had higher circulating concentrations of IFN-γ, TNF-α, IL-12, IL-17, IL-1β, GM-CSF (cytokines that favors Th1 response) and also had higher concentrations of IL-5, IL-10, and TGF-β (cytokines related to regulation of cytokine response) than TB patients without prediabetes (Kumar et al., 2014). This dysregulation of cytokines levels found in plasma may compromise the immune response against M. tuberculosis, and suggest that progressive changes in immune response related to T2D progression may influence the susceptibility to TB.
Progression of T2D in obese patients produces changes that can be related to an increased TB susceptibility through modulation of adypocytokines such as the C1q tumor necrosis factor related protein-3 (C1qTNF3 or CTRP-3). C1qTNF3 is a cytokine produced by macrophages and adipocytes that reduces inflammation generated by adipocytes (Kopp et al., 2010; Schmid et al., 2014). A study in obese patients showed that T2D induces a reduction in the plasmatic concentrations of this cytokine as compared with non-T2D patients (Elsaid et al., 2019). A study conducted in South Africa and Gambia, showed that patients who progress from a Latent TB Infection (LTBI) to active TB (LTBI defined as TST+, Quantiferon TB assay+) have lower concentrations of C1qTNF3 in plasma than LTBI patients who did not progress to TB during a more than 1 year follow-up (Penn-Nicholson et al., 2019). These findings suggest that the reduction in C1qTNF3 might be a potential contributor to the increased risk for TB in people with T2D, and might be a factor worth evaluating in the clinic.
In formally established T2D (as opposed to pre-T2D), some additional disorders in metabolism may occur, such as hyperglycemia, dyslipidemia, changes in lipoprotein concentrations, and changes in hormonal profiles (Olokoba et al., 2012; Carrera Boada and Martinez-Moreno, 2013). These alterations seem to be an adequate environment for M. tuberculosis infection to thrive in T2D subjects, likely improving persistence of mycobacteria and allowing them to consolidate the pulmonar infection and its effects (Figure 1C).
Clinical Features of T2D and Their Effects on Response to M. tuberculosis Infection
Effect of Hyperglycemia on Susceptibility to M. tuberculosis During T2D
T2D is characterized by an increase in blood concentration of glucose (measured as FBG >126 mg/dL or measured as 2 h postprandial glucose >200 mg/dL), known as hyperglycemia, and higher proportions of 1AC in blood (>6.5%) (American Diabetes Association, 2018a).
High glucose concentration in blood has been related to defects in host response against M. tuberculosis infection. An study in Japan, where FBG levels in 522 TB-confirmed patients were tested, showed a positive association (+1.31) between glucose intolerance and the development of TB (OR 3.15, C.I. 1.12–8.88; Hayashi et al., 2014). This positive association was also found in an a cross-sectional study in African individuals, where subjects with random blood glucose (RBG) concentration ≥11.1 mmol/L had 2.15 times the odds of prevalent TB than individuals with a RBG concentration <11.1 mmol/L (Bailey et al., 2016).
Rationale dissection of the effect of hyperglycemia indicates that it can affect the activity of macrophages. Macrophages are one of the first cells to encounter mycobacteria and are the main cells where mycobacteria resides during infection (Srivastava et al., 2014).
Montoya-Rosales and collaborators (Montoya-Rosales et al., 2016) cultured monocyte-derived macrophages from human U-937 cell line in the presence of different concentrations of glucose in the medium, and observed a negative correlation between glucose concentration and phagocytosis of M. tuberculosis. In the same system, the authors described a positive correlation between glucose concentration and induction of LL-37 antimicrobial peptide, and with the induction of anti-inflammatory cytokines such as TGF-β and IL-10 (Montoya-Rosales et al., 2016).
Another defect in macrophage function caused by hyperglycemia is the expression of receptors related to antigen presentation and related to T cell activation. A study where alveolar macrophages from T2D patients were infected with M. tuberculosis H37Rv, reported reduced levels of expression of CD86, CD80, and HLA-DR, molecules that participate in antigen presentation and Th response; and also a reduced induction of IL-6, IL-1β, IL-10, and IL-12 before and after the infection (Lopez-Lopez et al., 2018). Taken together, the works by Montoya-Rosales et al. (2016) and Lopez-Lopez et al. (2018) suggest a potential mechanistic explanation that contribute to partially explain the influence of hyperglycemia on the susceptibility to M. tuberculosis infection in T2D patients, but studies in vivo are needed to clearly establish the role of hyperglycemia on TB during TB-T2D comorbidity.
Glycemic control is commonly measured by hemoglobin 1AC because it represents the concentration of glucose found in blood 2 or 3 months before the actual measurement has been done (American Diabetes Association, 2018a). A glycemic level over the recommended by ADA (1AC <7%, preprandial capillary glucose between 83 and 130 mg/dL and peak postprandial capillary plasma glucose <180 mg/dL; American Diabetes Association, 2019b) has been correlated with higher prevalence of TB among T2D patients (Almeida-Junior et al., 2016) and with extended lung damage caused by TB, detected by computed tomography (Xia et al., 2018), or by radiological examinations (Huang et al., 2017) in patients with TB-T2D. However, a mechanistic explanation about the role of 1AC in promoting lung pathology in TB-T2D remains undescribed.
Regarding the role of an increase in 1AC levels and its effects on innate immune response, a study in India determined the levels of monocyte activator markers (CD164, sCD163, and CRP) in plasma of TB, T2D, T2D, and healthy controls (Kumar et al., 2019). In this study, 1AC of either TB or TB-T2D patients was positively correlated with levels of these markers. In addition, authors demonstrated that in patients who were diabetic before developing TB, the levels of these markers were increased compared with those of healthy controls, suggesting that changes in monocyte activation marker profiles coincident with changes in 1AC may be progressive. In another study conducted in Indonesia, cytokines in blood were determined in two defined groups of patients depending on their 1AC levels (<7 and >7%). Patients with 1AC >7% had the same levels of IFN-γ and IL-12 than the patients with 1AC <7%, but had lower levels of vitamin A (Ginandjar et al., 2016). Deficiency in vitamin A levels has been recently associated to a higher risk of TB in Moroccan patients (Qrafli et al., 2017), and this vitamin was found in lower levels in TB patients than in healthy controls (Oh et al., 2017). Whether or not there is a correlation between low 1AC levels and decreased vitamin A (or vice versa) remains to be determined.
Even though 1AC levels have not been found to be related to anti-TB treatment success in both T2D and non-T2D patients (Tabarsi et al., 2014), recent evidence suggest that higher levels of 1AC in TB patients may produce a delayed time to sputum conversion in TB patients receiving anti-TB treatment (Nurwidya et al., 2018). More efforts are needed to better understand the relationship between 1AC and the susceptibility to M. tuberculosis infection.
Effect of Diabetic Dyslipidemia on Susceptibility to M. tuberculosis During T2D
T2D is associated with some alterations in lipid of lipids and lipoproteins, a condition described as diabetic dyslipidemia (Carmena, 2008). This disorder comprises high levels of triglycerides, reduction of high-density lipoprotein (HDL) cholesterol concentrations and high levels of low-density lipoproteins (LDL) in blood (Wu and Parhofer, 2014; Cuevas et al., 2016).
A recent analysis by 1H-Nuclear Magnetic Resonance of lipid metabolic markers (lipoproteins, fatty acids, glycerides, amino acids, and glycolytic molecules) in plasma samples from TB, T2D, and TB-T2D patients showed that TB-T2D comorbidity displays characteristics of both diseases, such as reduced concentration of amino acids (indicator of waste) and lower dyslipidemia than expected only in T2D (Vrieling et al., 2018). In the same study, authors recognized that there were differences in the profiles among individuals, perhaps attributable to the chronicity of T2D and the time lapse of comorbidity TB-T2D. This suggested that there is a dynamism between both diseases that generates differences in lipid environments, which, in turn, could lead to different responses against infection.
As for the effects of triglycerides on TB, an in vitro study using PBMC-derived macrophages and THP-1 derived macrophages, showed that M. tuberculosis incorporates triglycerides from the host to infection sites in conditions of hypoxia (Daniel et al., 2011; Guerrini et al., 2018). This condition creates a fatty acid-rich environment for M. tuberculosis that favor the use of lipids as the major source of energy during M. tuberculosis infection, as documented in granulomas in rabbits, marmosets, and humans (Guerrini et al., 2018). A recent systematic review and meta-analysis has reinforced this concept by finding a positive correlation between latent infection by M. tuberculosis (defined as TST+ or IGRA+) with T2D, OR = 1.18 (1.06–1.30; Lee et al., 2017).
Accumulation of lipids in macrophages leads to formation of cytokine secreting foam cells in T2D patients. A study where J774 murine macrophages were exposed to serum of T2D patients, showed that serum triglycerides levels correlated with induction of lipid accumulation in these cells and consequently, in the formation of foamy macrophages (Cui et al., 2010). It is known that formation of foamy macrophages contributes to persistence of bacteria and to the tissue pathology during TB (Russell et al., 2009). Nevertheless, the direct effect of high levels of triglycerides in T2D patients on the formation of foamy macrophages during TB infection has not been addressed in clinical studies.
The observed reduction in HDL-cholesterol in T2D patients, has also been observed in patients with pulmonary tuberculosis (Deniz et al., 2007; Rao, 2009). This reduction in HDL-cholesterol was recently identified as a risk factor for severe lung lesions in patients with TB-T2D (Dong et al., 2018). However, the role of the reduction in HDL-cholesterol on the host response against M. tuberculosis is still unaddressed, and need to be explored in future studies.
Further to the above mentioned increase in concentration of triglycerides in T2D patients, they had higher concentrations of oxidized-LDL (Ox-LDL) in serum than healthy controls (Vrieling et al., 2019). In guinea pigs, it has been demonstrated that ox-LDL accumulates in lungs during active infection by M. tuberculosis (Parish et al., 2012), which is in line with findings in vitro that indicates that ox-LDL is taken up by macrophages in environments rich in glucose (Hayek et al., 2005). The effect of ox-LDL on bacilli activity was addressed by Parish and collaborators, who performed a study using alveolar macrophages obtained from guinea pigs loaded with Ox-LDL and then infected with M. tuberculosis H37Rv. They observed that Ox-LDL-treated macrophages had higher bacillary loads than untreated macrophages after 3 days of culture (Parish et al., 2012). Coincidentally, this effect has also been observed in primary human macrophages exposed to Ox-LDL before infection with M. tuberculosis H37Rv, where there was an increase of the bacterial burden in a dose-dependent manner within macrophages in vitro (Vrieling et al., 2019). These evidences reinforce the fact that the higher concentrations of Ox-LDL found in T2D patients may contribute to a higher susceptibility to TB progression and suggest that diabetic dyslipidemia is closely related to TB susceptibility.
Physiopathological Mechanisms Altered in T2D and Their relationship With TB Susceptibility
Hormones Related to Blood Glucose Control and Their Possible Roles During TB-T2D
Leptin and ghrelin are two hormones related to control of blood glucose concentration that are related to malnutrition during TB (Chang et al., 2013; Mexitalia et al., 2017). In a study where the concentration of leptin and ghrelin in blood was measured in TB, TB-T2D, and healthy Chinese people, there were found lower levels of leptin in TB-T2D patients as compared with TB patients, and higher levels of ghrelin were observed in TB-T2D patients than in TB patients (Zheng et al., 2013). These data suggest that T2D may increase susceptibility to TB through affecting appetite through these changes in ghrelin and leptin, which are related to a higher susceptibility to M. tuberculosis infection (Buyukoglan et al., 2007).
Resistin is a protein suggested to be part of the development of insulin resistance in humans and mice (Benomar et al., 2013), and it has been recognized as a key molecule that links obesity and T2D (Steppan et al., 2001). Resistin participates in increasing expression of proinflammatory cytokines such as TNF-α, IL-6, IL-12, and monocyte chemoattractant protein (MCP)-1 in PBMCs, macrophages, and hepatic stellate cells via the nuclear factor-κB (NF-κB) pathway (Bokarewa et al., 2005). It was shown that patients with T2D had higher levels of resistin in serum and this increase correlated with a diminished capability of THP-1 human macrophages to induce the production of reactive species of oxygen (ROS) in vitro against a challenge with M. tuberculosis (Chao et al., 2015). Another study in patients without T2D, found higher concentrations of serum resistin in TB patients than in healthy controls, and this concentration diminishes during 6 months of treatment with a standard anti-tuberculosis treatment (Ehtesham et al., 2011). These evidences suggest TB induces a metabolic change in the production of resistin, which has effects in both metabolic and immunologic response and derives in macrophage defective functions.
Production of intestinal incretins, proteins released after food intake and which stimulate insulin secretion (Nauck and Meier, 2018), such as GLP (glucagon-like peptide) is reduced in patients with T2D (Mannucci et al., 2000). GLP is degraded by the enzyme dipeptidyl peptidase IV (DPP-4) and as a part of the antidiabetic treatment, blockers of DPP4 are commonly used for glucose control (Deacon, 2019). A recent study found a negative correlation in blood levels of DPP4 and the chemoattraction of cells to lung in TB infected humans (Blauenfeldt et al., 2018). According to this study, TB patients had a lower amount of DPP4 than healthy controls and this was correlated with TB pathology through the recruitment of Th1 T cells to the site of infection. Even though the effect on blood glucose levels through the use of DPP4 blockers during TB-T2D on the host response to M. tuberculosis infection has not been addressed, the hormonal control of appetite and blood glucose may cause modulation of the of immune response.
Modifications in Microbiota in T2D Patients and Its Role on Immunity Against Mycobacteria
Patients with T2D have modifications in the composition of their intestinal microbiota that may lead to tuberculosis susceptibility (Zhang et al., 2013). In T2D patients, there is a significant reduction in the numbers of bacteria that produces short-chain fatty acids (SCFA) (Larsen et al., 2010; Morrison and Preston, 2016). The role of SCFA in response to M. tuberculosis was explored in vitro by Lachmandas and collaborators by exposing isolated Peripheral Blood Mononuclear Cells (PBMCs) to SCFA (acetate, propionate or butyrate) and measuring cytokines that participate in the proinflammatory and anti-inflammatory response against a stimulus with M. tuberculosis H37Rv lysates in vitro. Treatment with SCFA decreased induction of TNF-α, IL-1β, and IL-17, while it did not modify the induction of IL-6, IFN-γ, or IL-22 (Lachmandas et al., 2016). A reduced production of TNF-α or IL-1β is related with higher bacillary burden, while a reduced production in IL-17 has been related to lower migration of T cells to the site of infection, conducting to severe scenarios of infection (Domingo-Gonzalez et al., 2016). Even though Lachmandas and collaborators did not demonstrate that SCFA concentrations affected bacillary load in this in vitro model, it was clear that they affected host response against M. tuberculosis infection and left open the opportunity to experimentally address their influence in vivo using suitable models.
Vitamin D Deficiency in T2D and Its Effect on Immune Response Against Mycobacteria
Vitamin D is known to play a role in the control of TB infection (Kearns and Tangpricha, 2014). In addition, vitamin D is related with the control of blood glucose in T2D though modulation of insulin resistance and insulin secretion (Norman et al., 1980). Vitamin D deficiency is common in T2D patients, and some studies have related this deficiency with an increased risk of TB. A recent study has correlated the presence of pre-T2D and T2D with lower levels of vitamin D in TB patients in China (Zhao et al., 2017). Another study in recently diagnosed TB patients in China, either with TB or TB-T2D, revealed that there is an association between diminished vitamin D levels in serum and TB (OR = 3.26, C.I. 1.56–6.82) or TB-T2D (OR = 2.27, C.I. 1.05–4.92) (Wang et al., 2017).
Regarding the evidences linking vitamin D deficiency to a higher risk of TB, an study in Tanzania, revealed that vitamin D deficiency is related to an increased risk of TB only in patients with persistent hyperglycemia (OR = 4.0, C.I. 0.86–18.54) (Boillat-Blanco et al., 2016), and another study in China showed that patients with more than 10 years of T2D diagnosis have lower levels of vitamin D (Zhao et al., 2018). In addition to these data, an study in Chinese patients showed that both T2D and pre-T2D people have lower levels of vitamin D in serum (Zhao et al., 2017). These results suggest that there is a time-dependent relationship between vitamin D, hyperglycemia and TB risk.
A study in Mexico, where monocytes obtained from T2D patients (with confirmed vitamin D deficiency) were infected with M. tuberculosis H37Ra, showed that bacterial replication was higher in monocytes from T2D patients than in monocytes from healthy controls (Herrera et al., 2017), suggesting that vitamin D (among other possible defects as already described above) contribute to reduced control of M. tuberculosis in cells derived from T2D patients.
Treatment of T2D and its Impact on TB Susceptibility
Effect of Anti-hyperglycemic Drugs on the Host Response to M. tuberculosis Infection
According to ADA, metformin is the preferred initial treatment for hyperglycemia in T2D patients (American Diabetes Association, 2018b) and, even though a recent systematic review found evidence that suggest the glycemic control may have a favorable effect on anti-TB treatments outcomes (Shewade et al., 2017), its influence on the host response against M. tuberculosis is controversial.
Two recent retrospective studies in Taiwan showed that the treatment of hyperglycemia with metformin is a factor that prevents TB in T2D patients [HR 0.552, 95% C.I. 0.493–0.617 (Tseng, 2018) and HR 0.84, 95% C.I. 0.74–0.96 (Lee M. C. et al., 2018)]. In spite of its protective role, there is no conclusive information about the effectiveness of adjunctive therapy with metformin and anti-TB treatment. A recent report suggest that metformin acts by reducing concentration of circulating metalloproteinases (MPP-1, -2, and -8), and it is correlated with reduced bacterial burden in TB-T2D patients treated with metformin and antibiotics (Kumar et al., 2018). However, a study conducted in Seoul showed that treatment of TB-T2D patients with metformin plus the anti-TB treatment, did not have an effect on the sputum culture conversion nor TB recurrence within 1 year after treatment completion (Lee Y. J. et al., 2018), which is in line of findings of not increased efficacy of TB treatment by administration of metformin in mice (Dutta et al., 2017). These evidences suggest that control of hyperglycemia may contribute to the maintenance of a metabolic environment that may be able to reduce the susceptibility to TB throughout mechanisms that act independently of antibiotic activity.
A study where PBMCs from healthy controls were cultured with glibenclamide, a sulphonylurea also used to reduce hyperglycemia, produced a reduction in M1 markers (CD14+ CD16–), and increased M2 markers (CD14+ CD16+) in these cells independently of infection with BCG. Moreover, when PBMC obtained from subjects who were treated with oral glibenclamide were infected with M. tuberculosis, they exhibited an impaired capability to kill bacilli (Kewcharoenwong et al., 2018). While to date, there are no studies reported that correlate the use of glibenclamide with TB susceptibility or anti-TB treatment efficacy, some studies in healthy subjects have demonstrated that there are pharmacological interactions between glibenclamide and glimepiride (another sulphonylurea used to control of hyperglycemia) (Surekha et al., 1997; Niemi et al., 2000). Future studies in this regard are needed to clarify the role of glibenclamide on TB treatment efficacy.
Effect of Pharmacological Treatment of, or Vaccination With BCG, on Diabetic Dyslipidemia and Host Response to M. tuberculosis Infection
As we described above, some metabolic changes associated with diabetic dyslipidemia can be actively implicated in the susceptibility of the host to M. tuberculosis infection. Treatment of dyslipidemia has been shown to influence the capability to respond to M. tuberculosis infection. Lee and collaborators identified in a retrospective study that Taiwanese individuals with T2D and treated with statins had a lower risk of developing active TB (RR 0.76, 95% C.I. 0.60–0.97), than individuals with T2D and no treatment with statins (Lee et al., 2015). However, treatment of dyslipidemia had no influence on the efficacy of a combined therapy formed by antibiotic-treatment and statins or fibrates against TB, as a cohort study showed recently (Chen et al., 2019). These evidences suggest that control of diabetic dyslipidemia should have different effects on the host response to M. tuberculosis depending on the stage of TB, before activation of infection, or when infection is established.
Vaccination with BCG produces changes on parameters related to diabetic dyslipidemia. An study where guinea pigs were vaccinated or not with BCG, showed that vaccination produces a lower accumulation of Ox-LDL at the site of infection after challenge with M. tuberculosis H37Rv than accumulation observed in unvaccinated guinea pigs (Parish et al., 2012). This effect regarding the accumulation of ox-LDL is in agreement with results obtained with peritoneal macrophages isolated from hyperlipidemic APOE*Leiden. CETP mice vaccinated with BCG, which after vaccination, had lower concentrations of non-HDL cholesterol than unvaccinated mice, and this event correlated with lower formation of foamy cells (Van Dam et al., 2016). These data suggest that immunization with BCG has also an effect on lipid metabolism and may contribute to the protection afforded against TB by pathways other than those directly involved in immune responses.
Concluding Remarks
Recently, a systematic review showed that more than 70% of new drug efficacy trials have not considered the T2D population in their protocols (Lutfiana et al., 2019). We strongly believe research in TB should encompass efforts to understand how the presence of risk factors like T2D may compromise the efficacy and/or immunogenicity of vaccines, or affect the sensibility of diagnostic methods, or even the therapeutic effect of new drugs.
As a mathematical modeling of TB-T2D shows, control of T2D may lead to a significant reduction in TB incidence, and the uncontrolled raise of T2D should have the opposite effect (Pan et al., 2015). We think that a better understanding of the mechanisms that couple metabolic changes with TB susceptibility will lead to the design of better therapies, which would focus on the management of the TB-T2D comorbidity. We do not rule out the use of drugs that are traditionally used in the T2D treatment as a host-directed therapy against TB, as metformin and statins, even though further studies are needed to fully ascertain their efficacy as a complement for the treatment of TB. Furthermore, we suggest that the design and evaluation of current and future vaccine candidates against TB, or diagnostic methods that aim to early diagnose TB before the onset of overt disease in T2D patients, should consider including their evaluation in T2D preclinical models, as well as in human volunteers whenever a clinical trial is designed and performed.
T2D patients undergo some metabolic modifications that may facilitate the establishment, maintenance, and progression of M. tuberculosis infection. Since the onset of a prediabetic stage, the metabolic environment compromises the host response and produces changes that allows infection and favors disease progression and its worsening. In this sense, identification of biomarkers of progression to TB in prediabetic patients is needed to better understand the transition point between progression or not to TB. Recent efforts to elucidate a metabolic signature of progression to TB revealed that some metabolic networks may be related to progression to TB (Duffy et al., 2019). In particular, genes that encode for molecules that participate in fatty-acid metabolism and protein catabolism resulted as significantly associated to TB progression: CTP1A, which codes for carnitine palmitoyltransferase 1A was downregulated in obesity (Orellana-Gavalda et al., 2011); SLC25A20, which codes for carnitine acyl-carnitine translocase and has been observed to contribute to insulin secretion in mouse models of obesity (Soni et al., 2014); and PDK4, which codes for pyruvate dehydrogenase kinase, a key enzyme that participates in control of blood glucose in an insuline-dependent manner (Lee, 2014), and is increased in diabetic rats (Wu et al., 1998).
During T2D and TB comorbidity, the basal levels of glucose, 1AC, triglycerides, HDL-cholesterol, lipoproteins, and hormones related to the control of metabolic parameters creates an environment that supports bacterial survival and their spread (Figure 1). Finally, we contend that a better understanding of how immunity is modulated by metabolic signatures, will allow us to identify targets for treatment of TB-T2D patients, which lead to an improved control of T2D and resolution of TB disease by an enhanced clearance of M. tuberculosis from infected cells.
Author Contributions
CS-C and MF-V extensively discussed the manuscript. All authors wrote the manuscript, reviewed drafts, and approved submission of this work.
Funding
CS-C and WL-R received financial support from CONACYT, Ph.D. fellowship 432019 and 337689, respectively.
Conflict of Interest
The authors declare that the research was conducted in the absence of any commercial or financial relationships that could be construed as a potential conflict of interest.
Acknowledgments
The effect of T2D on humoral response to TB is being evaluated thanks to project FONCICYT-GACD265796 (MF-V).
References
Almeida-Junior, J. L., Gil-Santana, L., Oliveira, C. A., Castro, S., Cafezeiro, A. S., Daltro, C., et al. (2016). Glucose metabolism disorder is associated with pulmonary tuberculosis in individuals with respiratory symptoms from Brazil. PLoS ONE 11:e0153590. doi: 10.1371/journal.pone.0153590
Al-Rifai, R. H., Pearson, F., Critchley, J. A., and Abu-Raddad, L. J. (2017). Association between diabetes mellitus and active tuberculosis: a systematic review and meta-analysis. PLoS ONE 12:e0187967. doi: 10.1371/journal.pone.0187967
Amare, H., Gelaw, A., Anagaw, B., and Gelaw, B. (2013). Smear positive pulmonary tuberculosis among diabetic patients at the Dessie referral hospital, Northeast Ethiopia. Infect. Dis. Poverty 2:6. doi: 10.1186/2049-9957-2-6
American Diabetes Association (2018a). 2. Classification and diagnosis of diabetes: standards of medical care in diabetes-2018. Diabetes Care 41, S13–S27. doi: 10.2337/dc18-S002
American Diabetes Association (2018b). 8. Pharmacologic approaches to glycemic treatment: standards of medical care in diabetes-2018. Diabetes Care 41, S73–S85. doi: 10.2337/dc18-S008
American Diabetes Association (2019a). 2. Classification and diagnosis of diabetes: standards of medical care in diabetes-2019. Diabetes Care 42, S13–S28. doi: 10.2337/dc19-S002
American Diabetes Association (2019b). 6. Glycemic targets: standards of medical care in diabetes-2019. Diabetes Care 42, S61–S70. doi: 10.2337/dc19-S006
Bailey, S. L., Ayles, H., Beyers, N., Godfrey-Faussett, P., Muyoyeta, M., Du Toit, E., et al. (2016). The association of hyperglycaemia with prevalent tuberculosis: a population-based cross-sectional study. BMC Infect. Dis. 16:733. doi: 10.1186/s12879-016-2066-1
Benomar, Y., Gertler, A., De Lacy, P., Crepin, D., Ould Hamouda, H., Riffault, L., et al. (2013). Central resistin overexposure induces insulin resistance through Toll-like receptor 4. Diabetes 62, 102–114. doi: 10.2337/db12-0237
Blauenfeldt, T., Petrone, L., Del Nonno, F., Baiocchini, A., Falasca, L., Chiacchio, T., et al. (2018). Interplay of DDP4 and IP-10 as a potential mechanism for cell recruitment to tuberculosis lesions. Front. Immunol. 9:1456. doi: 10.3389/fimmu.2018.01456
Boillat-Blanco, N., Bovet, P., Ramaiya, K. L., Mganga, M., Minja, L. T., Saleh, L., et al. (2016). Association between tuberculosis, diabetes and 25 hydroxyvitamin D in Tanzania: a longitudinal case control study. BMC Infect. Dis. 16:626. doi: 10.1186/s12879-016-1960-x
Bokarewa, M., Nagaev, I., Dahlberg, L., Smith, U., and Tarkowski, A. (2005). Resistin, an adipokine with potent proinflammatory properties. J. Immunol. 174, 5789–5795. doi: 10.4049/jimmunol.174.9.5789
Buyukoglan, H., Gulmez, I., Kelestimur, F., Kart, L., Oymak, F. S., Demir, R., et al. (2007). Leptin levels in various manifestations of pulmonary tuberculosis. Mediators Inflamm. 2007:64859. doi: 10.1155/2007/64859
Carmena, R. (2008). Riesgo elevado de disfunción lipoproteica en la diabetes mellitus tipo 2. Rev Esp Cardiol Supl. 8, 19C−26C. doi: 10.1016/S1131-3587(08)73551-9
Carreira, S., Costeira, J., Gomes, C., Andre, J. M., and Diogo, N. (2012). Impact of diabetes on the presenting features of tuberculosis in hospitalized patients. Rev. Port. Pneumol. 18, 239–243. doi: 10.1016/j.rppneu.2012.04.001
Carrera Boada, C. A., and Martinez-Moreno, J. M. (2013). Pathophysiology of diabetes mellitus type 2: beyond the duo “insulin resistance-secretion deficit”. Nutr. Hosp. 28(Suppl. 2), 78–87. doi: 10.3305/nh.2013.28.sup2.6717
Chang, S. W., Pan, W. S., Lozano Beltran, D., Oleyda Baldelomar, L., Solano, M. A., Tuero, I., et al. (2013). Gut hormones, appetite suppression and cachexia in patients with pulmonary TB. PLoS ONE 8:e54564. doi: 10.1371/journal.pone.0054564
Chao, W. C., Yen, C. L., Wu, Y. H., Chen, S. Y., Hsieh, C. Y., Chang, T. C., et al. (2015). Increased resistin may suppress reactive oxygen species production and inflammasome activation in type 2 diabetic patients with pulmonary tuberculosis infection. Microbes Infect. 17, 195–204. doi: 10.1016/j.micinf.2014.11.009
Chen, Y. T., Kuo, S. C., Chao, P. W., and Chang, Y. Y. (2019). Use of lipid-lowering agents is not associated with improved outcomes for tuberculosis patients on standard-course therapy: a population-based cohort study. PLoS ONE 14:e0210479. doi: 10.1371/journal.pone.0210479
Cuevas, M. A., and Alonso, K. R. (2016). Dislipidemia diabética. Rev. Méd. Clín. Las Condes 27, 152–159. doi: 10.1016/j.rmclc.2016.04.004
Cui, X., Kushiyama, A., Yoneda, M., Nakatsu, Y., Guo, Y., Zhang, J., et al. (2010). Macrophage foam cell formation is augmented in serum from patients with diabetic angiopathy. Diabetes Res. Clin. Pract. 87, 57–63. doi: 10.1016/j.diabres.2009.10.011
Daniel, J., Maamar, H., Deb, C., Sirakova, T. D., and Kolattukudy, P. E. (2011). Mycobacterium tuberculosis uses host triacylglycerol to accumulate lipid droplets and acquires a dormancy-like phenotype in lipid-loaded macrophages. PLoS Pathog. 7:e1002093. doi: 10.1371/journal.ppat.1002093
Deacon, C. F. (2019). Physiology and pharmacology of DPP-4 in glucose homeostasis and the treatment of type 2 diabetes. Front. Endocrinol. 10:80. doi: 10.3389/fendo.2019.00080
Defronzo, R. A., Ferrannini, E., Groop, L., Henry, R. R., Herman, W. H., Holst, J. J., et al. (2015). Type 2 diabetes mellitus. Nat. Rev. Dis. Primers 1:15019. doi: 10.1038/nrdp.2015.19
Deniz, O., Gumus, S., Yaman, H., Ciftci, F., Ors, F., Cakir, E., et al. (2007). Serum total cholesterol, HDL-C and LDL-C concentrations significantly correlate with the radiological extent of disease and the degree of smear positivity in patients with pulmonary tuberculosis. Clin. Biochem. 40, 162–166. doi: 10.1016/j.clinbiochem.2006.10.015
Domingo-Gonzalez, R., Prince, O., Cooper, A., and Khader, S. A. (2016). Cytokines and chemokines in Mycobacterium tuberculosis infection. Microbiol. Spectr. 4:TBTB2-0018-2016. doi: 10.1128/microbiolspec.TBTB2-0018-2016
Dong, Z., Shi, J., Dorhoi, A., Zhang, J., Soodeen-Lalloo, A. K., Tan, W., et al. (2018). Hemostasis and lipoprotein indices signify exacerbated lung injury in TB with diabetes comorbidity. Chest 153, 1187–1200. doi: 10.1016/j.chest.2017.11.029
Duffy, F. J., Weiner, J. III., Hansen, S., Tabb, D. L., Suliman, S., Thompson, E., Maertzdorf, J., et al. (2019). Immunometabolic signatures predict risk of progression to active tuberculosis and disease outcome. Front. Immunol. 10:527. doi: 10.3389/fimmu.2019.00527
Dutta, N. K., Pinn, M. L., and Karakousis, P. C. (2017). Metformin adjunctive therapy does not improve the sterilizing activity of the first-line antitubercular regimen in mice. Antimicrob. Agents Chemother. 61:e00652-17. doi: 10.1128/AAC.00652-17
Ehtesham, N. Z., Nasiruddin, M., Alvi, A., Kumar, B. K., Ahmed, N., Peri, S., et al. (2011). Treatment end point determinants for pulmonary tuberculosis: human resistin as a surrogate biomarker. Tuberculosis 91, 293–299. doi: 10.1016/j.tube.2011.04.007
Elsaid, H. H., Elgohary, M. N., and Elshabrawy, A. M. (2019). Complement c1q tumor necrosis factor-related protein 3 a novel adipokine, protect against diabetes mellitus in young adult Egyptians. Diabetes Metab. Syndr. 13, 434–438. doi: 10.1016/j.dsx.2018.10.004
Ginandjar, P., Saraswati, L. D., and Widjanarko, B. (2016). Profile of glycated-hemoglobin, antioxidant vitamin and cytokine levels in pulmonary tuberculosis patients: a cross sectional study at Pulmonary Diseases Center Semarang City, Indonesia. Biomed. J. 39, 354–360. doi: 10.1016/j.bj.2016.01.011
Guerrini, V., Prideaux, B., Blanc, L., Bruiners, N., Arrigucci, R., Singh, S., et al. (2018). Storage lipid studies in tuberculosis reveal that foam cell biogenesis is disease-specific. PLoS Pathog. 14:e1007223. doi: 10.1371/journal.ppat.1007223
Hayashi, S., and Chandramohan, D. (2018). Risk of active tuberculosis among people with diabetes mellitus: systematic review and meta-analysis. Trop. Med. Int. Health 23, 1058–1070. doi: 10.1111/tmi.13133
Hayashi, S., Takeuchi, M., Hatsuda, K., Ogata, K., Kurata, M., Nakayama, T., et al. (2014). The impact of nutrition and glucose intolerance on the development of tuberculosis in Japan. Int. J. Tuberc. Lung Dis. 18, 84–88. doi: 10.5588/ijtld.13.0495
Hayek, T., Hussein, K., Aviram, M., Coleman, R., Keidar, S., Pavoltzky, E., et al. (2005). Macrophage foam-cell formation in streptozotocin-induced diabetic mice: stimulatory effect of glucose. Atherosclerosis 183, 25–33. doi: 10.1016/j.atherosclerosis.2005.02.018
Herrera, M. T., Gonzalez, Y., Hernandez-Sanchez, F., Fabian-San Miguel, G., and Torres, M. (2017). Low serum vitamin D levels in type 2 diabetes patients are associated with decreased mycobacterial activity. BMC Infect. Dis. 17:610. doi: 10.1186/s12879-017-2705-1
Huang, L. K., Wang, H. H., Lai, Y. C., and Chang, S. C. (2017). The impact of glycemic status on radiological manifestations of pulmonary tuberculosis in diabetic patients. PLoS ONE 12:e0179750. doi: 10.1371/journal.pone.0179750
IDF (2017). IDFDiabetes Atlas. Available online at: https://www.diabetesatlas.org
Jimenez-Corona, M. E., Cruz-Hervert, L. P., Garcia-Garcia, L., Ferreyra-Reyes, L., Delgado-Sanchez, G., Bobadilla-Del-Valle, M., et al. (2013). Association of diabetes and tuberculosis: impact on treatment and post-treatment outcomes. Thorax 68, 214–220. doi: 10.1136/thoraxjnl-2012-201756
Kearns, M. D., and Tangpricha, V. (2014). The role of vitamin D in tuberculosis. J. Clin. Transl. Endocrinol. 1, 167–169. doi: 10.1016/j.jcte.2014.08.002
Kewcharoenwong, C., Prabowo, S. A., Bancroft, G. J., Fletcher, H. A., and Lertmemongkolchai, G. (2018). Glibenclamide reduces primary human monocyte functions against tuberculosis infection by enhancing M2 polarization. Front. Immunol. 9:2109. doi: 10.3389/fimmu.2018.02109
Kopp, A., Bala, M., Buechler, C., Falk, W., Gross, P., Neumeier, M., et al. (2010). C1q/TNF-related protein-3 represents a novel and endogenous lipopolysaccharide antagonist of the adipose tissue. Endocrinology 151, 5267–5278. doi: 10.1210/en.2010-0571
Kumar Nathella, P., and Babu, S. (2017). Influence of diabetes mellitus on immunity to human tuberculosis. Immunology 152, 13–24. doi: 10.1111/imm.12762
Kumar, N. P., Banurekha, V. V., Nair, D., Sridhar, R., Kornfeld, H., Nutman, T. B., et al. (2014). Coincident pre-diabetes is associated with dysregulated cytokine responses in pulmonary tuberculosis. PLoS ONE 9:e112108. doi: 10.1371/journal.pone.0112108
Kumar, N. P., Moideen, K., Bhootra, Y., Nancy, A., Viswanathan, V., Shruthi, B. S., et al. (2019). Elevated circulating levels of monocyte activation markers among tuberculosis patients with diabetes co-morbidity. Immunology 156, 249–258. doi: 10.1111/imm.13023
Kumar, N. P., Moideen, K., Viswanathan, V., Shruthi, B. S., Sivakumar, S., Menon, P. A., et al. (2018). Elevated levels of matrix metalloproteinases reflect severity and extent of disease in tuberculosis-diabetes co-morbidity and are predominantly reversed following standard anti-tuberculosis or metformin treatment. BMC Infect. Dis. 18:345. doi: 10.1186/s12879-018-3246-y
Lachmandas, E., Van Den Heuvel, C. N., Damen, M. S., Cleophas, M. C., Netea, M. G., and Van Crevel, R. (2016). Diabetes mellitus and increased tuberculosis susceptibility: the role of short-chain fatty acids. J. Diabetes Res. 2016:6014631. doi: 10.1155/2016/6014631
Larsen, N., Vogensen, F. K., Van Den Berg, F. W., Nielsen, D. S., Andreasen, A. S., Pedersen, B. K., et al. (2010). Gut microbiota in human adults with type 2 diabetes differs from non-diabetic adults. PLoS ONE 5:e9085. doi: 10.1371/journal.pone.0009085
Lee, I. K. (2014). The role of pyruvate dehydrogenase kinase in diabetes and obesity. Diabetes Metab. J. 38, 181–186. doi: 10.4093/dmj.2014.38.3.181
Lee, M. C., Chiang, C. Y., Lee, C. H., Ho, C. M., Chang, C. H., Wang, J. Y., et al. (2018). Metformin use is associated with a low risk of tuberculosis among newly diagnosed diabetes mellitus patients with normal renal function: a nationwide cohort study with validated diagnostic criteria. PLoS ONE 13:e0205807. doi: 10.1371/journal.pone.0205807
Lee, M. R., Huang, Y. P., Kuo, Y. T., Luo, C. H., Shih, Y. J., Shu, C. C., et al. (2017). Diabetes mellitus and latent tuberculosis infection: a systematic review and metaanalysis. Clin. Infect. Dis. 64, 719–727. doi: 10.1093/cid/ciw836
Lee, M. Y., Lin, K. D., Hsu, W. H., Chang, H. L., Yang, Y. H., Hsiao, P. J., et al. (2015). Statin, calcium channel blocker and Beta blocker therapy may decrease the incidence of tuberculosis infection in elderly Taiwanese patients with type 2 diabetes. Int. J. Mol. Sci. 16, 11369–11384. doi: 10.3390/ijms160511369
Lee, Y. J., Han, S. K., Park, J. H., Lee, J. K., Kim, D. K., Chung, H. S., et al. (2018). The effect of metformin on culture conversion in tuberculosis patients with diabetes mellitus. Korean J. Intern. Med. 33, 933–940. doi: 10.3904/kjim.2017.249
Liu, M., Liu, S. W., Wang, L. J., Bai, Y. M., Zeng, X. Y., Guo, H. B., et al. (2019). Burden of diabetes, hyperglycaemia in China from to 2016: findings from the 1990 to 2016, global burden of disease study. Diabetes Metab. 45, 286–293. doi: 10.1016/j.diabet.2018.08.008
Liu, Q., Li, W., Xue, M., Chen, Y., Du, X., Wang, C., et al. (2017). Diabetes mellitus and the risk of multidrug resistant tuberculosis: a meta-analysis. Sci. Rep. 7:1090. doi: 10.1038/s41598-017-01213-5
Lopez-Lopez, N., Martinez, A. G. R., Garcia-Hernandez, M. H., Hernandez-Pando, R., Castaneda-Delgado, J. E., Lugo-Villarino, G., et al. (2018). Type-2 diabetes alters the basal phenotype of human macrophages and diminishes their capacity to respond, internalise, and control Mycobacterium tuberculosis. Mem. Inst. Oswaldo Cruz 113:e170326. doi: 10.1590/0074-02760170326
Lutfiana, N. C., Van Boven, J. F. M., Masoom Zubair, M. A., Pena, M. J., and Alffenaar, J. C. (2019). Diabetes mellitus comorbidity in patients enrolled in tuberculosis drug efficacy trials around the world: a systematic review. Br. J. Clin. Pharmacol. 85, 1407–1417. doi: 10.1111/bcp.13935
Magee, M. J., Salindri, A. D., Kyaw, N. T. T., Auld, S. C., Haw, J. S., and Umpierrez, G. E. (2018). Stress hyperglycemia in patients with tuberculosis disease: epidemiology and clinical implications. Curr. Diab. Rep. 18:71. doi: 10.1007/s11892-018-1036-y
Mannucci, E., Ognibene, A., Cremasco, F., Bardini, G., Mencucci, A., Pierazzuoli, E., et al. (2000). Glucagon-like peptide (GLP)-1 and leptin concentrations in obese patients with Type 2 diabetes mellitus. Diabetic Med. 17, 713–719. doi: 10.1046/j.1464-5491.2000.00367.x
Mave, V., Meshram, S., Lokhande, R., Kadam, D., Dharmshale, S., Bharadwaj, R., et al. (2017). Prevalence of dysglycemia and clinical presentation of pulmonary tuberculosis in Western India. Int. J. Tuberc. Lung Dis. 21, 1280–1287. doi: 10.5588/ijtld.17.0474
Mexitalia, M., Dewi, Y. O., Pramono, A., and Anam, M. S. (2017). Effect of tuberculosis treatment on leptin levels, weight gain, and percentage body fat in Indonesian children. Korean J. Pediatr. 60, 118–123. doi: 10.3345/kjp.2017.60.4.118
Montoya-Rosales, A., Castro-Garcia, P., Torres-Juarez, F., Enciso-Moreno, J. A., and Rivas-Santiago, B. (2016). Glucose levels affect LL-37 expression in monocyte-derived macrophages altering the Mycobacterium tuberculosis intracellular growth control. Microb. Pathog. 97, 148–153. doi: 10.1016/j.micpath.2016.06.002
Morrison, D. J., and Preston, T. (2016). Formation of short chain fatty acids by the gut microbiota and their impact on human metabolism. Gut Microbes 7, 189–200. doi: 10.1080/19490976.2015.1134082
Nauck, M. A., and Meier, J. J. (2018). Incretin hormones: their role in health and disease. Diabetes Obes. Metab. 20(Suppl. 1), 5–21. doi: 10.1111/dom.13129
Niemi, M., Kivisto, K. T., Backman, J. T., and Neuvonen, P. J. (2000). Effect of rifampicin on the pharmacokinetics and pharmacodynamics of glimepiride. Br. J. Clin. Pharmacol. 50, 591–595. doi: 10.1046/j.1365-2125.2000.00295.x
Norman, A. W., Frankel, J. B., Heldt, A. M., and Grodsky, G. M. (1980). Vitamin D deficiency inhibits pancreatic secretion of insulin. Science 209, 823–825. doi: 10.1126/science.6250216
Nurwidya, F., Ratnawati, Wijaya, D., Nazaruddin, A., and Burhan, E. (2018). The relationship between hemoglobin A1C levels and sputum conversion time in indonesian patients with new cases of pulmonary tuberculosis. J. Nat. Sci. Biol. Med. 9, 217–221. doi: 10.4103/jnsbm.JNSBM_24_18
Oh, J., Choi, R., Park, H. D., Lee, H., Jeong, B. H., Park, H. Y., et al. (2017). Evaluation of vitamin status in patients with pulmonary tuberculosis. J. Infect. 74, 272–280. doi: 10.1016/j.jinf.2016.10.009
Olokoba, A. B., Obateru, O. A., and Olokoba, L. B. (2012). Type 2 diabetes mellitus: a review of current trends. Oman Med. J. 27, 269–273. doi: 10.5001/omj.2012.68
Orellana-Gavalda, J. M., Herrero, L., Malandrino, M. I., Paneda, A., Sol Rodriguez-Pena, M., Petry, H., et al. (2011). Molecular therapy for obesity and diabetes based on a long-term increase in hepatic fatty-acid oxidation. Hepatology 53, 821–832. doi: 10.1002/hep.24140
Pan, S.-C., Ku, C.-C., Kao, D., Ezzati, M., Fang, C.-T., and Lin, H.-H. (2015). Effect of diabetes on tuberculosis control in 13 countries with high tuberculosis: a modelling study. Lancet Diabetes Endocrinol. 3, 323–330. doi: 10.1016/S2213-8587(15)00042-X
Parish, T., Palanisamy, G. S., Kirk, N. M., Ackart, D. F., Obregón-Henao, A., Shanley, C. A., et al. (2012). Uptake and accumulation of oxidized low-density lipoprotein during Mycobacterium tuberculosis infection in guinea pigs. PLoS ONE 7:34148. doi: 10.1371/journal.pone.0034148
Penn-Nicholson, A., Hraha, T., Thompson, E. G., Sterling, D., Mbandi, S. K., Wall, K. M., et al. (2019). Discovery and validation of a prognostic proteomic signature for tuberculosis progression: a prospective cohort study. PLoS Med. 16:e1002781. doi: 10.1371/journal.pmed.1002781
Qrafli, M., El Kari, K., Aguenaou, H., Bourkadi, J. E., Sadki, K., and El Mzibri, M. (2017). Low plasma vitamin A concentration is associated with tuberculosis in Moroccan population: a preliminary case control study. BMC Res. Notes 10:421. doi: 10.1186/s13104-017-2737-z
Rao, S. (2009). Serum cholesterol, HDL, LDL levels in pulmonary tuberculosis. Infect. Dis. Clin. Pract. 17, 99–101. doi: 10.1097/IPC.0b013e3181934367
Rao, S. R. M, Iqbal, K., Haroon, F., and Hasan, Z. (2019). Impact of diabetes on mechanisms of immunity against Mycobacterium tuberculosis. J Pak Med Assoc. 69, 94–98. Available online at: https://jpma.org.pk/article-details/9007?article_id=9007
Restrepo, B. I. (2016). Diabetes and tuberculosis. Microbiol. Spectr. 4, 595-606. doi: 10.1128/microbiolspec.TNMI7-0023-2016
Russell, D. G., Cardona, P. J., Kim, M. J., Allain, S., and Altare, F. (2009). Foamy macrophages and the progression of the human tuberculosis granuloma. Nat. Immunol. 10, 943–948. doi: 10.1038/ni.1781
Schmid, A., Kopp, A., Hanses, F., Karrasch, T., and Schaffler, A. (2014). C1q/TNF-related protein-3 (CTRP-3) attenuates lipopolysaccharide (LPS)-induced systemic inflammation and adipose tissue Erk-1/-2 phosphorylation in mice in vivo. Biochem. Biophys. Res. Commun. 452, 8–13. doi: 10.1016/j.bbrc.2014.06.054
Shewade, H. D., Jeyashree, K., Mahajan, P., Shah, A. N., Kirubakaran, R., Rao, R., et al. (2017). Effect of glycemic control and type of diabetes treatment on unsuccessful TB treatment outcomes among people with TB-Diabetes: a systematic review. PLoS ONE 12:e0186697. doi: 10.1371/journal.pone.0186697
Shivakumar, S., Chandrasekaran, P., Kumar, A. M. V., Paradkar, M., Dhanasekaran, K., Suryavarshini, N., et al. (2018). Diabetes and pre-diabetes among household contacts of tuberculosis patients in India: is it time to screen them all? Int. J. Tuberc. Lung Dis. 22, 686–694. doi: 10.5588/ijtld.17.0598
Soni, M. S., Rabaglia, M. E., Bhatnagar, S., Shang, J., Ilkayeva, O., Mynatt, R., et al. (2014). Downregulation of carnitine acyl-carnitine translocase by miRNAs 132 and 212 amplifies glucose-stimulated insulin secretion. Diabetes 63, 3805–3814. doi: 10.2337/db13-1677
Srivastava, S., Ernst, J. D., and Desvignes, L. (2014). Beyond macrophages: the diversity of mononuclear cells in tuberculosis. Immunol. Rev. 262, 179–192. doi: 10.1111/imr.12217
Steppan, C. M., Bailey, S. T., Bhat, S., Brown, E. J., Banerjee, R. R., Wright, C. M., et al. (2001). The hormone resistin links obesity to diabetes. Nature 409, 307–312. doi: 10.1038/35053000
Surekha, V., Peter, J. V., Jeyaseelan, L., and Cherian, A. M. (1997). Drug interaction: rifampicin and glibenclamide. Natl. Med. J. India 10, 2.
Tabarsi, P., Baghaei, P., Marjani, M., Vollmer, W. M., Masjedi, M. R., and Harries, A. D. (2014). Changes in glycosylated haemoglobin and treatment outcomes in patients with tuberculosis in Iran: a cohort study. J. Diabetes Metab. Disord. 13:123. doi: 10.1186/s40200-014-0123-0
Tseng, C. H. (2018). Metformin decreases risk of tuberculosis infection in type 2 diabetes patients. J. Clin. Med. 7:264. doi: 10.3390/jcm7090264
Van Dam, A. D., Bekkering, S., Crasborn, M., Van Beek, L., Van Den Berg, S. M., Vrieling, F., et al. (2016). BCG lowers plasma cholesterol levels and delays atherosclerotic lesion progression in mice. Atherosclerosis 251, 6–14. doi: 10.1016/j.atherosclerosis.2016.05.031
Viswanathan, A. A., and Gawde, N. C. (2014). Effect of type II diabetes mellitus on treatment outcomes of tuberculosis. Lung India 31, 244–248. doi: 10.4103/0970-2113.135764
Vrieling, F., Ronacher, K., Kleynhans, L., Van Den Akker, E., Walzl, G., Ottenhoff, T. H. M., et al. (2018). Patients with concurrent tuberculosis and diabetes have a pro-atherogenic plasma lipid profile. EBioMedicine 32, 192–200. doi: 10.1016/j.ebiom.2018.05.011
Vrieling, F., Wilson, L., Rensen, P. C. N., Walzl, G., Ottenhoff, T. H. M., and Joosten, S. A. (2019). Oxidized low-density lipoprotein (oxLDL) supports Mycobacterium tuberculosis survival in macrophages by inducing lysosomal dysfunction. PLoS Pathog. 15:e1007724. doi: 10.1371/journal.ppat.1007724
Wang, Q., Ma, A., Han, X., Zhang, H., Zhao, S., Liang, H., et al. (2017). Is low serum 25-hydroxyvitamin D a possible link between pulmonary tuberculosis and type 2 diabetes? Asia Pac. J. Clin. Nutr. 26, 241–246. doi: 10.6133/apjcn.032016.02
Wilkinson, K. A., Workneh, M. H., Bjune, G. A., and Yimer, S. A. (2017). Prevalence and associated factors of tuberculosis and diabetes mellitus comorbidity: a systematic review. PLoS ONE 12:e0175925. doi: 10.1371/journal.pone.0175925
Wu, L., and Parhofer, K. G. (2014). Diabetic dyslipidemia. Metab. Clin. Exp. 63, 1469–1479. doi: 10.1016/j.metabol.2014.08.010
Wu, P., Sato, J., Zhao, Y., Jaskiewicz, J., Popov, K. M., and Harris, R. A. (1998). Starvation and diabetes increase the amount of pyruvate dehydrogenase kinase isoenzyme 4 in rat heart. Biochem. J. 329, 197–201. doi: 10.1042/bj3290197
Xia, L. L., Li, S. F., Shao, K., Zhang, X., and Huang, S. (2018). The correlation between CT features and glycosylated hemoglobin level in patients with T2DM complicated with primary pulmonary tuberculosis. Infect. Drug Resist. 11, 187–193. doi: 10.2147/IDR.S146741
Zhang, X., Shen, D., Fang, Z., Jie, Z., Qiu, X., Zhang, C., et al. (2013). Human gut microbiota changes reveal the progression of glucose intolerance. PLoS ONE 8:e71108. doi: 10.1371/journal.pone.0071108
Zhao, X., Yuan, Y., Lin, Y., Zhang, T., Bai, Y., Kang, D., et al. (2018). Vitamin D status of tuberculosis patients with diabetes mellitus in different economic areas and associated factors in China. PLoS ONE 13:e0206372. doi: 10.1371/journal.pone.0206372
Zhao, X., Yuan, Y., Lin, Y., Zhang, T., Ma, J., Kang, W., et al. (2017). Vitamin D status in tuberculosis patients with diabetes, prediabetes and normal blood glucose in China: a cross-sectional study. BMJ Open 7:e017557. doi: 10.1136/bmjopen-2017-017557
Keywords: Mycobacterium tuberculosis infection, type 2 diabetes, hyperglycemia, diabetic dyslipidemia, hormones
Citation: Segura-Cerda CA, López-Romero W and Flores-Valdez MA (2019) Changes in Host Response to Mycobacterium tuberculosis Infection Associated With Type 2 Diabetes: Beyond Hyperglycemia. Front. Cell. Infect. Microbiol. 9:342. doi: 10.3389/fcimb.2019.00342
Received: 03 July 2019; Accepted: 23 September 2019;
Published: 04 October 2019.
Edited by:
Igor Kramnik, Boston University, United StatesReviewed by:
Kai Huang, University of Texas Medical Branch at Galveston, United StatesRobert Cody Sharp, University of Florida Health, United States
Copyright © 2019 Segura-Cerda, López-Romero and Flores-Valdez. This is an open-access article distributed under the terms of the Creative Commons Attribution License (CC BY). The use, distribution or reproduction in other forums is permitted, provided the original author(s) and the copyright owner(s) are credited and that the original publication in this journal is cited, in accordance with accepted academic practice. No use, distribution or reproduction is permitted which does not comply with these terms.
*Correspondence: Mario Alberto Flores-Valdez, ZmxvcmVzdkBjaWF0ZWoubXg=; ZmxvcmVzdno5MUBnbWFpbC5jb20=