- 1Centre for Dengue Research, Faculty of Medical Sciences, University of Sri Jayewardenepura, Nugegoda, Sri Lanka
- 2MRC Human Immunology Unit, MRC Weatherall Institute of Molecular Medicine, University of Oxford, Oxford, United Kingdom
Although infection with the dengue virus (DENV) causes severe dengue, it causes a mild self-limiting illness in the majority of individuals. There is emerging evidence that an aberrant immune response in the initial stages of infection lead to severe disease. Many inflammatory cytokines, chemokines, and lipid mediators are significantly higher in patients with severe dengue compared to those who develop mild infection, during febrile phase of illness. Monocytes, mast cells, and many other cells of the immune system, when infected with the DENV, especially in the presence of poorly neutralizing antibodies, leads to production of pro-inflammatory cytokines and inhibition of interferon signaling pathways. In addition, production of immunosuppressive cytokines such as IL-10 further leads to inhibition of cellular antiviral responses. This dysregulated and aberrant immune response leads to reduced clearance of the virus, and severe dengue by inducing a vascular leak and excessive inflammation due to high levels of inflammatory cytokines. Individuals with comorbid illnesses could be prone to more severe dengue due to low grade endotoxemia, gut microbial dysbiosis and an altered phenotype of innate immune cells. The immunosuppressive and inflammatory lipid mediators and altered phenotype of monocytes are likely to further act on T cells and B cells leading to an impaired adaptive immune response to the virus. Therefore, in order to identify therapeutic targets for treatment of dengue, it would be important to further characterize these mechanisms in order for early intervention. In this review, we discuss the differences in the innate immune responses in those who progress to develop severe dengue, compared to those with milder disease in order to understand the mechanisms that lead to severe dengue.
Introduction
Dengue infections represent one of the most important vector-borne diseases in the world, resulting in significant morbidity and mortality. It is estimated than 105 to 390 million individuals are infected with one of the four dengue viruses (DENV) annually leading to 51 to 96 million apparent dengue infections (Bhatt et al., 2013; Cattarino et al., 2020). Although the infection is asymptomatic or mild in most infected individuals, it can lead to severe forms of disease such as dengue hemorrhagic fever (DHF) and death in some individuals (Cattarino et al., 2020). DHF is shown to occur in ~23.2% of symptomatic individuals, and if untreated, is reported to have case fatality rates (CFRs) of ~20% (Guo et al., 2017). Although CFRs are <1% in most countries due to intense monitoring of patients who develop dengue and meticulous fluid management, CFRs are reported to be around 2.6% in some countries (Guo et al., 2017; Murhekar et al., 2019).
Initial infection with a particular DENV serotype is known as a primary infection, while subsequent infection with any other serotype is known as a secondary dengue infection (WHO, 2011). Secondary dengue infection is thought to be one of the most important risk factors for development of severe disease, in part due to antibody dependent enhancement (ADE) in which poorly neutralizing, highly cross-reactive antibodies enhance infection in FcγR-expressing cells (Chareonsirisuthigul et al., 2007; Chau et al., 2008; Guzman et al., 2013; Syenina et al., 2015; Katzelnick et al., 2017). However, not all secondary dengue infections result in symptomatic infection or DHF and only 15% of secondary dengue infections progress to DHF (Wang et al., 2017). Subclinical dengue infection occurs in an equal proportion of those experiencing a primary or secondary dengue infections (Grange et al., 2014). Therefore, many host factors apart from the presence of poorly neutralizing cross-reactive antibodies could lead to protection or pathogenesis.
In those who develop a symptomatic illness, there can be sudden onset of fever along with headaches, myalgia and arthralgia. This initial febrile phase (early illness) typically lasts for 3 to 6 days and the patient recovers in most instances. However, ~25% of individuals progress to develop complications around day 3 to 6 of illness, which is characterized by fluid leakage leading to pleural effusions, ascites, shock, organ dysfunction and if untreated, can lead to death (WHO, 2011; Malavige and Ogg, 2017). There is emerging evidence that an aberrant immune response in the initial stage of infection, results in endothelial dysfunction and cytokine storms leading to vascular leakage and thus DHF. In this review, we discuss the differences in the innate immune responses in those who progress to develop DHF compared to those with milder disease in order to understand the mechanisms that lead to severe dengue.
Cytokine and Chemokines Signatures in Early Illness Leading to Severe Illness
Many different chemokines and cytokines have been shown to be elevated in severe dengue such as IFN-γ, GM-CSF, IL-10, MIP-1β, IL-1β, IL-8 TNFα, IP-10, MCP-1, and IL-18 (Bozza et al., 2008; Malavige et al., 2013a; Fernando et al., 2016; Kamaladasa et al., 2016; van Wilgenburg et al., 2016; Patro et al., 2019). Certain cytokines such as TNFα and IL-1β have shown to directly cause vascular leak (Hottz et al., 2014; Kamaladasa et al., 2016), while both these cytokines along with IL-18, IP-10, IL-8, and MIP-1β are potent inflammatory cytokines produced by many immune cells (Tang et al., 2020). IL-10 on the other hand is a potent immunosuppressive cytokine, which has been associated with severe dengue (Malavige et al., 2013a,b). Due to the presence of high levels of these cytokines during the critical phase and due to the presence of cross reactive DENV-specific T cells during acute dengue, T cells were implicated as the main source of these cytokines and the drivers of the cytokine storm (Mongkolsapaya et al., 2006; Appanna et al., 2007; Dong et al., 2007). However, more recent data have shown that innate immune cells such as monocytes are one of the main sources of such cytokines and chemokines, while DENV-specific T cells are likely to be protective (Weiskopf et al., 2013, 2015; Singla et al., 2016; Wijeratne et al., 2018). Those who proceeded to develop severe disease had higher levels of many types of inflammatory markers such a C-reactive protein, increased inflammatory lipid mediators and cytokines in very early illness (initial period of the febrile phase), before they proceeded to develop severe disease or vascular leak (Fernando et al., 2016; Jeewandara et al., 2017; Vuong et al., 2020). For instance, CRP levels of >34 mg/L within the first 3 days of illness (before any patients had developed severe disease) was associated with an increased risk of progression to severe illness (Vuong et al., 2020). Similar findings were observed with an inflammatory lipid enzyme mediator sPLA2, where the enzyme activity was highest during early illness in those progressed to develop DHF (Jeewandara et al., 2017).
Although cytokines and chemokines have been extensively studied in in dengue, only a few have studied their changes during different clinical phases of dengue. Due to the dynamic changes in the clinical and laboratory features along with cytokines and chemokines during the febrile phase (before the onset of vascular leak), is likely to be different than those in the critical phase. A study carried out in a relatively small sample of patients showed that IP-10, MCP-1, and MIP-1β were significantly higher in those who progressed to develop plasma leakage, again during the febrile phase compared to the critical phase (Rathakrishnan et al., 2012). A more recent study, done in a large cohort of patients showed that while many cytokines are elevated in patients with dengue during the febrile phase, it was IL-10 that most significantly associated with subsequent development of DHF (Dayarathna et al., 2020, under review). Therefore, collectively these data suggest that the events that trigger the release of these inflammatory mediators occur very early in illness (initial period of the febrile phase), potentially due to the differences in the innate immune responses to the virus.
Several proteins of the DENV have been shown to inhibit IFN signaling pathways, while NS1, which is a secretory protein has shown to directly cause disease pathogenesis (Castillo Ramirez and Urcuqui-Inchima, 2015; Modhiran et al., 2015; Adikari et al., 2016; Puerta-Guardo et al., 2016; Kao et al., 2018). NS1 Ag, which exists in a hexametric form, has been shown to trigger cytokine release from PBMCs through binding to TLR4 and also to contribute directly to vascular leak by disruption of the endothelial glycocalyx (Modhiran et al., 2015; Glasner et al., 2017). NS1 is a major target of antibodies that develop during natural DENV infections (Dejnirattisai et al., 2010) and mice immunized with NS1 or given polyclonal sera of mice immunized with NS1 were shown to have significantly less vascular leak, when challenged with the DENV (Beatty et al., 2015). However some studies have shown that NS1-NS1 antibody complexes contribute to vascular leak by activation of complement and by causing endothelial apoptosis (Avirutnan et al., 2006). Studies in acute dengue have shown that NS1 antibody levels rise in those with severe dengue compared to those with milder forms of illness, during acute illness and that those with milder dengue appeared to recognize different epitopes than those with severe illness (Jayathilaka et al., 2018). The lower than expected efficacy of some dengue vaccines, have been attributed to lack of generation of NS1 antibodies (Halstead, 2018,Nascimento et al., 2018).
The non-structural proteins NS2A and NS3 have been shown to inhibit type I interferon production by degrading the stimulator of interferon genes (STING) and IRF3, which in turn impairs RIG-I and TLR-3 signaling pathways (Castillo Ramirez and Urcuqui-Inchima, 2015). Both NS3, NS2A along with NS4A, NS4B, and NS5 also further inhibit interferon signaling by preventing STAT-1 phosphorylation (Castillo Ramirez and Urcuqui-Inchima, 2015; Kao et al., 2018). DENV strains which produce higher levels of subgenomic RNA and therefore are more capable of inhibiting TRIM-25 activation of RIG-I, have shown to have higher transmission rates and able to cause epidemics (Manokaran et al., 2015). Therefore, inhibition of IFN production appears to be an important mechanism of immune evasion by the DENV, which is associated with increased clinical disease severity and epidemiological fitness. However, inhibition of IFN signaling by the DENV does not explain the occurrence of severe disease in some individuals, and mild/asymptomatic infection in others, who are infected with the same strain of the DENV. It is possible that those who develop more severe illness have higher viral replication within the host cells due to increased viral entry into such cells due to ADE and/or other genetic or immunological factors which influence the type I IFN response, and therefore, more inhibition of IFN signaling pathways.
Monocyte/Macrophage Responses in Severe Dengue
Although the DENV is known to infect many different types of cells, it was shown that monocytes are the cells most commonly infected by the virus (Zanini et al., 2018). Single cell transcriptomics in PBMCs of patients during early illness, before they progressed to severe disease, showed that cells of those who subsequently had severe disease had a unique transcriptomic signature (Zanini et al., 2018). Of the gene signatures associated with progression to severe disease, IFITI and CD163 expression in CD14+CD16+ monocytes and MX2 in B lymphocytes had the highest predictive value, highlighting the important role of monocytes in disease pathogenesis (Zanini et al., 2018). Soluble CD163 which is a marker of macrophage activation syndrome has been previously shown to differentiate those who have severe dengue from DF (Ab-Rahman et al., 2016). Therefore, macrophage activation associated with various cytokines such as TNFα, IL-6, and IL-10 or by oxidative stress appears to be a feature of DHF.
Apart from direct infection by the DENV, monocyte infection is further facilitated in acute dengue, by ADE (Chareonsirisuthigul et al., 2007; Syenina et al., 2015; Katzelnick et al., 2017). Internalization of antigen-antibody complexes is known to further suppress antiviral responses (Ubol et al., 2010; Tsai et al., 2014; Wang et al., 2017). In vitro infection of monocytes with the DENV, in the presence of sub-neutralizing concentrations of DENV-specific antibodies resulted in increased production of IL-10 and suppression of IFNβ and iNOS (Ubol et al., 2010). The high levels of IL-10 resulted in upregulation of negative regulators such as activation of suppressor of cytokine signaling system (SOCS), specifically SOCS-3 and inhibition of JAK-STAT pathways (Ubol et al., 2010) thereby suppressing antiviral defenses (Tsai et al., 2014) (Figure 1).
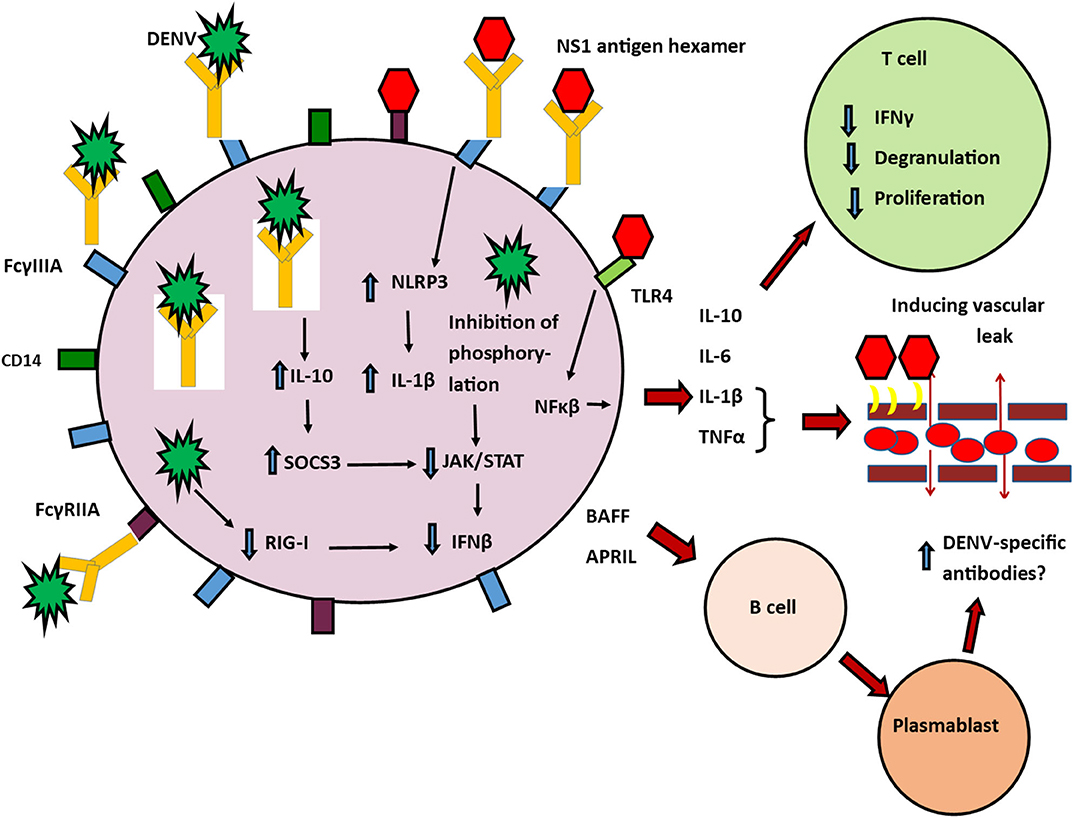
Figure 1. Possible role of monocytes in pathogenesis of severe dengue. Classical and non-classical monocytes have many types of activating FcγRs, that facilitate antibody dependent enhancement (ADE) such as FcγRI, FcγRIIA, and FcγRIIA. FcγRI and FcγRIIA downstream signaling inhibits many antiviral responses by the cell, including inhibition of RIG-I/MDA5 induced type I IFN, TNFα, and IL-12 production. Non-classical (CD14+CD16++ monocytes) are more susceptible to infection through the DENV through ADE by binding of immune complexes to their FcγRIIIA (CD16a) receptors. This leads to induction of IL-10 and reduction of IFNβ and activation of iNOS. High levels of IL-10 induce SOCS3 which further inhibits JAK/STAT signaling pathways and inhibition of IFN production. DENV NS1 induced production of inflammatory cytokines after engagement through TLR-4 and contributes to vascular leak by disruption of the endothelial glycocalyx. Other DENV structural proteins leads to degradation of IFN signaling molecules resulting in impaired RIG-I signaling. DENV non-structural proteins also inhibit STAT-1 phosphorylation and thereby also contributing to reduction of IFN production. NS1 antibody-antigen immune complexes can also bind through FcγRIIIA (CD16a) receptors and induce NLRP3. This altered antiviral defense mechanisms within the cell leads to increased production of inflammatory cytokines and IL-10. IL-1β and TNFα contribute to vascular leak. IL-10 suppresses T cell activation, degranulation and cytokine production. These non-classical monocytes also produce BAFF and APRIL which act on resting B cells and stimulate them to transform into plasma cells, which are possibly responsible for further production of DENV-specific antibodies. NS1 Hexamer,
The DENV,
FcγRIIIA,
CD14,
FcγRIIA,
antibodies,
TLR4.
Certain FcγRs are more efficient at ADE than others. FcγRI, FcγRIIA, and FcγRIIIA are all shown to facilitate ADE while FcγRIIB is less efficient (Boonnak et al., 2008, 2013). FcγRIIA has shown to be more efficient than FcγRI in ADE in the presence of DENV-IgG immune complexes (Rodrigo et al., 2006). Downstream signaling pathways that are activated by engagement of the activating FcγRs (FcγRI and FcγRIIA), has shown to reduce production of TNFα, IL-12, and IFNβ through inhibition of interferon regulatory factor 1 and NFκB gene expression, while increasing the production of IL-10 (Ubol and Halstead, 2010). Engagement of immune complexes with the activating FcγRs in acute DENV infection results in activation of two suppression pathways, which leads to overall impairment of antiviral immune responses (Ubol and Halstead, 2010).
The FcγRIII receptor, which is another activating FcγR, which is important in ADE, has 2 isoforms, FcγRIIIA and FcγRIIIB (CD16a/CD16b). FcγRIIIA is known to bind to immune complexes more efficiently that other FcγRs (Ogonda et al., 2010). Increased expression of FcγRIIIA on non-classical monocytes (CD14+CD16++), has been linked to occurrence of severe anemia and other complications during Plasmodium falciparum infection (Ogonda et al., 2010). Higher levels of afucosylated Fc IgG1 isoforms, which have an enhanced capacity to engage with the activating FcRIIIA, was associated with DHF and shock in those with acute dengue suggesting antibody binding to FcγRIIIA is likely to be associated with severe disease (Wang et al., 2017). We recently showed that differences in antibodies to dengue NS1 antigen could also be associated with protection or severe disease (Jayathilaka et al., 2018). Indeed, in dengue mouse models, anti-NS1-Abs activated NLRP-3 by binding to FcγRIIIs resulting in severe disease (Lien et al., 2015). Therefore, it would be important to further investigate the role of different antibody subclass binding to different FcγRs and association with clinical disease severity.
Monocytes of healthy individuals who had varying severity of dengue in the past also appear to respond significantly different to infection with the DENV. We showed that monocytes of healthy individuals who had severe dengue in the past when infected with different serotypes of the DENV produced higher viral loads, higher levels of IL-10, IL-6, TNFα, and IL-1β and increased expression of RIG-I and NLRP3 when compared to monocytes of those with past non-severe dengue (Kamaladasa et al., 2019). These monocytes produced higher levels of IL-1β in the presence of autologous serum, in the presence of serum from those with past non-severe dengue and in the presence of seronegative serum suggesting that this aberrant responses by monocytes of those with past severe dengue could be an inherent characteristic (Kamaladasa et al., 2019). Although the reasons for this are not clear, it could be epigenetic reprograming in innate immune cells in such individuals due to exposure to different pathogens or other stimuli (Netea et al., 2016).
Mast Cells in Dengue
Mast cells are shown to be permissive to infection by the DENV, which is enhanced in the presence of DENV-specific antibodies (St. John et al., 2013; Syenina et al., 2015). Many proteases and inflammatory mediators produced by mast cells such as chymases, tryptases, platelet activating factor (PAF) and vascular endothelial growth factor (VEGF) have been implicated in vascular leak and disease pathogenesis (Furuta et al., 2012; Jeewandara et al., 2015; Kamaladasa et al., 2016; Tissera et al., 2017; Inokuchi et al., 2018; Malavige et al., 2018). As mast cells reside in tissues and around blood vessels, it has been difficult to determine their infectivity rates by the DENV and phenotypic changes (St. John, 2013). As they also reside in the skin, they can be readily infected and activated during very early infection, when the mosquito injects the DENV into the dermis. Although certain mediators such as PAF and VEGF can also be produced by other cells types such as macrophages, monocytes and endothelial cells (Walterscheid et al., 2002), chymases and tryptases are only produced by mast cells (Krystel-Whittemore et al., 2015). Both chymase and tryptase have been shown to be high in patients with acute dengue during early illness and shown to be an important predictor of progression to severe dengue, suggesting that mast cells indeed do have an important role in disease pathogenesis (Tissera et al., 2017; Rathore et al., 2019). Chymase levels were shown to be higher in those who progressed to develop DHF after day 3 of illness (Rathore et al., 2020). However, it is not yet clear whether the differences between those with primary and secondary dengue associate with increased mast cell activation. Serum tryptase has been shown to be higher in patients with DHF and this rise was especially seen during day early illness (Jeewandara et al., 2017; Rathore et al., 2019). Tryptase has been shown to induce vascular leak by acting on endothelial gap junctions and was shown to induce plasma leakage and shock in animal models, suggesting that it indeed is likely to have an important role in inducing vascular leak in dengue (Rathore et al., 2019). In acute dengue, no differences were observed in tryptase levels in those with primary and secondary dengue (Jeewandara et al., 2017), suggesting that the role of DENV-specific antibodies activating mast cells through their FcγRs should be further investigated.
PAF levels were also shown to be high in those with vascular leak and are known to induce vascular leak (Jeewandara et al., 2015). A PAFR blocker (rupatadine), was shown to inhibit the reduction of trans endothelial electrical resistance and reduce the effect of sera of patients with DHF on endothelial tight junction (Malavige et al., 2018). In addition, it also showed dose dependent effects on reducing the rise in hematocrit in mouse models and reduction in the extent of plasma leakage in patients with acute dengue (Malavige et al., 2018). PAF is also known to activate NFκB and thereby induce production of many inflammatory cytokines and further contributing to disease pathogenesis (Choi et al., 2003). Although the source of PAF is not known in patients with acute dengue, it is possible that many different cell types including mast cells could be contributing to its production. PAF has varying effects on many other immune cells such as reduction in dendritic cell maturation, T cell anergy and reduction in T cell and depending on the levels either increase or a decrease in T cell proliferation (Kelesidis et al., 2015). Therefore, PAF appears to have many potential impacts on antiviral immunity other than inducing vascular leak; and therapeutics that block PAF are may be beneficial by acting through many pathways.
Metabolic Disease and Innate Immunity
Individuals with comorbidities such as metabolic diseases and asthma are more likely to develop DHF and organ dysfunction (Guo et al., 2017; Pang et al., 2017; Wang et al., 2019). The presence of diabetes mellitus along with other metabolic diseases or poorly controlled diabetes mellitus were independent risk factors for development of severe dengue and shock (Lee et al., 2018). However, the mechanisms of severe dengue of those with comorbidities are not known. Patients with metabolic diseases have been shown to have low grade endotoxemia (Neves et al., 2013) due to gut microbial dysbiosis (Shin et al., 2015). Those with DHF have shown to have higher levels of serum lipopolysaccharide (LPS), which can result in activation of many innate immune cells through TLR-4 (van de Weg et al., 2012, 2013). While LPS alone induces many immune cells to produce inflammatory cytokines, LPS was shown to act synergistically with the DENV to further increase cytokine production and PAF, which can contribute to vascular leak and DHF (Kamaladasa et al., 2016). Therefore, such low grade endotoxemia in patients with metabolic disease, could be instrumental in inducing an unfavorable immune response to the DENV resulting in severe dengue. Gut microbial dysbiosis has shown to significantly influence the antiviral responses to vaccines (Lynn and Pulendran, 2018; Lynn et al., 2018). For instance, those who were given antibiotics just before the influenza vaccine, had reduced neutralizing and influenza specific IgG, and dysregulated immune responses, which was attributed to gut microbial dysbiosis (Hagan et al., 2019). Therefore, gut microbial dysbiosis may have a potential to induce severe dengue due to development of a poor quality, suboptimal antibody response.
Metabolic disease and obesity is associated with chronic, low grade inflammation and is associated with differences in monocyte subsets (Friedrich et al., 2019; Figueroa-Vega et al., 2020). While all CD14+CD16+(classical monocytes), CD14dimCD16+ (intermediate) and CD14−CD16++(non-classical) monocytes are susceptible to infection by the DENV, the non-classical monocytes are the main produced of inflammatory cytokines and IL-10, which are implicated in SD (Wong et al., 2012). IFITI and CD163 expression in CD14+CD16+ monocytes, was shown to precede the development of severe dengue (Zanini et al., 2018). As those with metabolic disease and obesity have a higher frequency of the non-classical types of monocytes (Friedrich et al., 2019), increased infection of this subtype by the DENV in such individuals could lead to severe dengue. Increase in mast cell numbers are also seen in adipose tissues of obese individuals, which are thought to contribute to low grade inflammation associated with metabolic syndrome and obesity (Elieh Ali Komi et al., 2020). Therefore, increase infection and activation of mast cells in those with metabolic disease could also lead to more severe disease.
Effect of Innate Immune Responses on Virus Specific T Cells and B Cells
Although the role of T cells in dengue have been debated, emerging evidence suggests that they are likely to have a protective role (Weiskopf et al., 2013, 2015; Wijeratne et al., 2018, 2019). Highly cross reactive T cells are seen in acute dengue, which were thought to contribute to disease pathogenesis by production of high levels of inflammatory cytokines (Mongkolsapaya et al., 2003; Appanna et al., 2007; Dong et al., 2007). Indeed, it has been shown that higher levels of cross-reactive T cells that produce inflammatory cytokines were seen in those with more severe forms of illness rather than those with milder illness (Mongkolsapaya et al., 2006). However, such cross reactive T cells producing high levels of inflammatory cytokines were only seen 14 days since the onset of illness, when the patient was in the recovery phase (Mongkolsapaya et al., 2006). In fact, T cell responses were very low or absent in patients during the critical phase, where vascular leak and the cytokine storm occurs (Mongkolsapaya et al., 2006; Malavige et al., 2013b). This paucity of the DENV-specific T cells in acute dengue, could be due to the massive T cell apoptosis that has been reported in many studies (Mongkolsapaya et al., 2003; Malavige et al., 2012). Indeed, prolonged viremia and persistence of NS1 antigen is seen in those with severe dengue (Wang et al., 2006; Guilarde et al., 2008; Adikari et al., 2016), while early appearance of DENV-specific T cells was associated with milder disease and early clearance of the virus (Wijeratne et al., 2018). Patients with DHF had significantly less DENV-specific T cell responses (Wijeratne et al., 2018), than those with DF and the virus specific T cell responses were restored in vitro, with IL-10 blockade (Malavige et al., 2013b). Many studies have shown that T cell responses are absent or suboptimal in those with DHF during acute illness (Mongkolsapaya et al., 2006; Chau et al., 2008). Since early appearance of IL-10 was the main cytokine that was associated with subsequent progression to severe dengue, IL-10 is likely to also contribute to disease pathogenesis by inhibiting virus specific T cell responses and therefore, a delay in virus clearance.
The expansion of non-classical CD14+CD16+ monocytes has shown to associate with severe dengue (Kwissa et al., 2014; Zanini et al., 2018). DENV-infected CD14+CD16+ monocytes were found to be more efficient at stimulating resting B cells to transformation into plasmablasts by production of BAFF and APRIL (Kwissa et al., 2014). Increase in the frequency of plasmablasts have shown to associate with dengue disease severity (Wrammert et al., 2012; Appanna et al., 2016; Wijesinghe et al., 2020). Although the mechanisms by which the plasmablasts contribute to disease pathogenesis is unclear, it is possible that the increased antibody production by these cells, could contribute to severe disease by ADE.
Summary
There is increasing evidence that a dysfunctional innate immune response leads to severe dengue by impaired production of interferons and increased production of inflammatory cytokines and lipid mediators. This dysregulated and aberrant immune response leads to reduced clearance of the virus, and severe dengue by inducing a vascular leak and by inducing excessive inflammation. Individuals with comorbid illnesses could be prone to more severe dengue due to low grade endotoxemia, gut microbial dysbiosis, and an altered phenotype of innate immune cells. The immunosuppressive and inflammatory lipid mediators and altered phenotype of monocytes are likely to further act on T cells and B cells leading to an impaired adaptive immune response to the virus. Therefore, in order to identify therapeutic targets for treatment of dengue, it would be important to further characterize these mechanisms in order for early intervention.
Author Contributions
GM and GO: conceptualization, critical review, and writing the manuscript. CJ: writing the manuscript. All authors: contributed to the article and approved the submitted version.
Funding
We are grateful to the Accelerating Higher Education Expansion and Development (AHEAD) Operation of the Ministry of Higher Education funded by the World Bank, Centre for Dengue Research, University of Sri Jayewardenepura, and the UK Medical Research Council.
Conflict of Interest
The authors declare that the research was conducted in the absence of any commercial or financial relationships that could be construed as a potential conflict of interest.
References
Ab-Rahman, H. A., Rahim, H., AbuBakar, S., and Wong, P. F. (2016). Macrophage activation syndrome-associated markers in severe Dengue. Int. J. Med. Sci. 13, 179–186. doi: 10.7150/ijms.13680
Adikari, T. N., Gomes, L., Wickramasinghe, N., Salimi, M., Wijesiriwardana, N., Kamaladasa, A., et al. (2016). Dengue NS1 antigen contributes to disease severity by inducing interleukin (IL)-10 by monocytes. Clin. Exp. Immunol. 184, 90–100. doi: 10.1111/cei.12747
Appanna, R., Huat, T. L., See, L. L., Tan, P. L., Vadivelu, J., and Devi, S. (2007). Cross-reactive T-cell responses to the nonstructural regions of dengue viruses among dengue fever and dengue hemorrhagic fever patients in Malaysia. Clin. Vaccine Immunol. 14, 969–977. doi: 10.1128/CVI.00069-07
Appanna, R., Kg, S., Xu, M. H., Toh, Y. X., Velumani, S., Carbajo, D., et al. (2016). Plasmablasts during acute dengue infection represent a small subset of a broader virus-specific memory B cell pool. EBioMedicine 12, 178–188. doi: 10.1016/j.ebiom.2016.09.003
Avirutnan, P., Punyadee, N., Noisakran, S., Komoltri, C., Thiemmeca, S., Auethavornanan, K., et al. (2006). Vascular leakage in severe dengue virus infections: a potential role for the nonstructural viral protein NS1 and complement. J. Infect. Dis. 193, 1078–1088. doi: 10.1086/500949
Beatty, P. R., Puerta-Guardo, H., Killingbeck, S. S., Glasner, D. R., Hopkins, K., and Harris, E. (2015). Dengue virus NS1 triggers endothelial permeability and vascular leak that is prevented by NS1 vaccination. Sci. Transl. Med. 7:304ra141. doi: 10.1126/scitranslmed.aaa3787
Bhatt, S., Gething, P. W., Brady, O. J., Messina, J. P., Farlow, A. W., Moyes, C. L., et al. (2013). The global distribution and burden of dengue. Nature 496, 504–507. doi: 10.1038/nature12060
Boonnak, K., Slike, B. M., Burgess, T. H., Mason, R. M., Wu, S. J., Sun, P., et al. (2008). Role of dendritic cells in antibody-dependent enhancement of dengue virus infection. J. Virol. 82, 3939–3951. doi: 10.1128/JVI.02484-07
Boonnak, K., Slike, B. M., Donofrio, G. C., and Marovich, M. A. (2013). Human FcgammaRII cytoplasmic domains differentially influence antibody-mediated dengue virus infection. J. Immunol. 190, 5659–5665. doi: 10.4049/jimmunol.1203052
Bozza, F. A., Cruz, O. G., Zagne, S. M., Azeredo, E. L., Nogueira, R. M., Assis, E. F., et al. (2008). Multiplex cytokine profile from dengue patients: MIP-1beta and IFN-gamma as predictive factors for severity. BMC Infect. Dis. 8:86. doi: 10.1186/1471-2334-8-86
Castillo Ramirez, J. A., and Urcuqui-Inchima, S. (2015). Dengue virus control of type I IFN responses: a history of manipulation and control. J. Interferon Cytok. Res. 35, 421–430. doi: 10.1089/jir.2014.0129
Cattarino, L., Rodriguez-Barraquer, I., Imai, N., Cummings, D. A. T., and Ferguson, N. M. (2020). Mapping global variation in dengue transmission intensity. Sci. Transl. Med. 12:eaax4144. doi: 10.1126/scitranslmed.aax4144
Chareonsirisuthigul, T., Kalayanarooj, S., and Ubol, S. (2007). Dengue virus (DENV) antibody-dependent enhancement of infection upregulates the production of anti-inflammatory cytokines, but suppresses anti-DENV free radical and pro-inflammatory cytokine production, in THP-1 cells. J. Gen. Virol. 88(Pt. 2), 365–375. doi: 10.1099/vir.0.82537-0
Chau, T. N., Quyen, N. T., Thuy, T. T., Tuan, N. M., Hoang, D. M., Dung, N. T., et al. (2008). Dengue in Vietnamese infants–results of infection-enhancement assays correlate with age-related disease epidemiology, and cellular immune responses correlate with disease severity. J. Infect. Dis. 198, 516–524. doi: 10.1086/590117
Choi, I. W., Kim, Y. S., Kim, D. K., Choi, J. H., Seo, K. H., Im, S. Y., et al. (2003). Platelet-activating factor-mediated NF-kappaB dependency of a late anaphylactic reaction. J. Exp. Med. 198, 145–151. doi: 10.1084/jem.20022129
Dejnirattisai, W., Jumnainsong, A., Onsirisakul, N., Fitton, P., Vasanawathana, S., Limpitikul, W., et al. (2010). Cross-reacting antibodies enhance dengue virus infection in humans. Science 328, 745–748. doi: 10.1126/science.1185181
Dong, T., Moran, E., Vinh Chau, N., Simmons, C., Luhn, K., Peng, Y., et al. (2007). High pro-inflammatory cytokine secretion and loss of high avidity cross-reactive cytotoxic T-cells during the course of secondary dengue virus infection. PLoS ONE 2:e1192. doi: 10.1371/journal.pone.0001192
Elieh Ali Komi, D., Shafaghat, F., and Christian, M. (2020). Crosstalk between mast cells and adipocytes in physiologic and pathologic conditions. Clin. Rev. Allergy Immunol. 58, 388–400. doi: 10.1007/s12016-020-08785-7
Fernando, S., Wijewickrama, A., Gomes, L., Punchihewa, C. T., Madusanka, S. D., Dissanayake, H., et al. (2016). Patterns and causes of liver involvement in acute dengue infection. BMC Infect. Dis. 16:319. doi: 10.1186/s12879-016-1656-2
Figueroa-Vega, N., Marin-Aragon, C. I., Lopez-Aguilar, I., Ibarra-Reynoso, L., Perez-Luque, E., and Malacara, J. M. (2020). Analysis of the percentages of monocyte subsets and ILC2s, their relationships with metabolic variables and response to hypocaloric restriction in obesity. PLoS ONE 15:e0228637. doi: 10.1371/journal.pone.0228637
Friedrich, K., Sommer, M., Strobel, S., Thrum, S., Bluher, M., Wagner, U., et al. (2019). Perturbation of the monocyte compartment in human Obesity. Front. Immunol. 10:1874. doi: 10.3389/fimmu.2019.01874
Furuta, T., Murao, L. A., Lan, N. T., Huy, N. T., Huong, V. T., Thuy, T. T., et al. (2012). Association of mast cell-derived VEGF and proteases in Dengue shock syndrome. PLoS Negl. Trop. Dis. 6:e1505. doi: 10.1371/journal.pntd.0001505
Glasner, D. R., Ratnasiri, K., Puerta-Guardo, H., Espinosa, D. A., Beatty, P. R., and Harris, E. (2017). Dengue virus NS1 cytokine-independent vascular leak is dependent on endothelial glycocalyx components. PLoS Pathog. 13:e1006673. doi: 10.1371/journal.ppat.1006673
Grange, L., Simon-Loriere, E., Sakuntabhai, A., Gresh, L., Paul, R., and Harris, E. (2014). Epidemiological risk factors associated with high global frequency of inapparent dengue virus infections. Front. Immunol. 5:280. doi: 10.3389/fimmu.2014.00280
Guilarde, A. O., Turchi, M. D., Siqueira, J. B. Jr., Feres, V. C., Rocha, B., Levi, J. E., et al. (2008). Dengue and dengue hemorrhagic fever among adults: clinical outcomes related to viremia, serotypes, and antibody response. J. Infect. Dis. 197, 817–824. doi: 10.1086/528805
Guo, C., Zhou, Z., Wen, Z., Liu, Y., Zeng, C., Xiao, D., et al. (2017). Global epidemiology of dengue outbreaks in. 1990-2015: a systematic review and meta-analysis. Front. Cell. Infect. Microbiol. 7:317. doi: 10.3389/fcimb.2017.00317
Guzman, M. G., Alvarez, M., and Halstead, S. B. (2013). Secondary infection as a risk factor for dengue hemorrhagic fever/dengue shock syndrome: an historical perspective and role of antibody-dependent enhancement of infection. Arch. Virol. 158, 1445–1459. doi: 10.1007/s00705-013-1645-3
Hagan, T., Cortese, M., Rouphael, N., Boudreau, C., Linde, C., Maddur, M. S., et al. (2019). Antibiotics-driven gut microbiome perturbation alters immunity to vaccines in Humans. Cell 178, 1313–28.e13. doi: 10.1016/j.cell.2019.08.010
Halstead, S. B. (2018). Which dengue vaccine approach is the most promising, and should we be concerned about enhanced disease after vaccination? There is only one true winner. Cold Spring Harb. Perspect. Biol. 10:a030700. doi: 10.1101/cshperspect.a030700
Hottz, E. D., Medeiros-de-Moraes, I. M., Vieira-de-Abreu, A., de Assis, E. F., Vals-de-Souza, R., Castro-Faria-Neto, H. C., et al. (2014). Platelet activation and apoptosis modulate monocyte inflammatory responses in dengue. J. Immunol. 193, 1864–1872. doi: 10.4049/jimmunol.1400091
Inokuchi, M., Dumre, S. P., Mizukami, S., Tun, M. M. N., Kamel, M. G., Manh, D. H., et al. (2018). Association between dengue severity and plasma levels of dengue-specific IgE and chymase. Arch. Virol. 163, 2337–2347. doi: 10.1007/s00705-018-3849-z
Jayathilaka, D., Gomes, L., Jeewandara, C., Jayarathna, G. S. B., Herath, D., Perera, P. A., et al. (2018). Role of NS1 antibodies in the pathogenesis of acute secondary dengue infection. Nat. Commun. 9:5242. doi: 10.1038/s41467-018-07667-z
Jeewandara, C., Gomes, L., Udari, S., Paranavitane, S. A., Shyamali, N. L., Ogg, G. S., et al. (2017). Secretory phospholipase A2 in the pathogenesis of acute dengue infection. Immun Inflamm Dis. 5, 7–15. doi: 10.1002/iid3.135
Jeewandara, C., Gomes, L., Wickramasinghe, N., Gutowska-Owsiak, D., Waithe, D., Paranavitane, S. A., et al. (2015). Platelet activating factor contributes to vascular leak in acute dengue infection. PLoS Negl. Trop. Dis. 9:e0003459. doi: 10.1371/journal.pntd.0003459
Kamaladasa, A., Gomes, L., Jeewandara, C., Shyamali, N. L., Ogg, G. S., and Malavige, G. N. (2016). Lipopolysaccharide acts synergistically with the dengue virus to induce monocyte production of platelet activating factor and other inflammatory mediators. Antiviral Res. 133, 183–190. doi: 10.1016/j.antiviral.2016.07.016
Kamaladasa, A., Gomes, L., Wijesinghe, A., Jeewandara, C., Toh, Y. X., Jayathilaka, D., et al. (2019). Altered monocyte response to the dengue virus in those with varying severity of past dengue infection. Antiviral Res. 169:104554. doi: 10.1016/j.antiviral.2019.104554
Kao, Y. T., Lai, M. M. C., and Yu, C. Y. (2018). How dengue virus circumvents innate immunity. Front. Immunol. 9:2860. doi: 10.3389/fimmu.2018.02860
Katzelnick, L. C., Gresh, L., Halloran, M. E., Mercado, J. C., Kuan, G., Gordon, A., et al. (2017). Antibody-dependent enhancement of severe dengue disease in humans. Science 358, 929–932. doi: 10.1126/science.aan6836
Kelesidis, T., Papakonstantinou, V., Detopoulou, P., Fragopoulou, E., Chini, M., Lazanas, M. C., et al. (2015). The role of platelet-activating factor in chronic inflammation, immune activation, and comorbidities associated with HIV infection. AIDS Rev. 17, 191–201.
Krystel-Whittemore, M., Dileepan, K. N., and Wood, J. G. (2015). Mast cell: a multi-functional master cell. Front. Immunol. 6:620. doi: 10.3389/fimmu.2015.00620
Kwissa, M., Nakaya, H. I., Onlamoon, N., Wrammert, J., Villinger, F., Perng, G. C., et al. (2014). Dengue virus infection induces expansion of a CD14(+)CD16(+) monocyte population that stimulates plasmablast differentiation. Cell Host Microbe 16, 115–127. doi: 10.1016/j.chom.2014.06.001
Lee, I. K., Hsieh, C. J., Lee, C. T., and Liu, J. W. (2018). Diabetic patients suffering dengue are at risk for development of dengue shock syndrome/severe dengue: emphasizing the impacts of co-existing comorbidity(ies) and glycemic control on dengue severity. J. Microbiol. Immunol. Infect. 53, 69–78. doi: 10.1016/j.jmii.2017.12.005
Lien, T. S., Sun, D. S., Chang, C. M., Wu, C. Y., Dai, M. S., Chan, H., et al. (2015). Dengue virus and antiplatelet autoantibodies synergistically induce haemorrhage through Nlrp3-inflammasome and FcgammaRIII. Thromb. Haemost. 113, 1060–1070. doi: 10.1160/TH14-07-0637
Lynn, D. J., and Pulendran, B. (2018). The potential of the microbiota to influence vaccine responses. J. Leukoc. Biol. 103, 225–231. doi: 10.1189/jlb.5MR0617-216R
Lynn, M. A., Tumes, D. J., Choo, J. M., Sribnaia, A., Blake, S. J., Leong, L. E. X., et al. (2018). Early-life antibiotic-driven dysbiosis leads to dysregulated vaccine immune responses in Mice. Cell Host Microbe 23, 653–660.e5. doi: 10.1016/j.chom.2018.04.009
Malavige, G. N., Gomes, L., Alles, L., Chang, T., Salimi, M., Fernando, S., et al. (2013a). Serum IL-10 as a marker of severe dengue infection. BMC Infect. Dis. 13:341. doi: 10.1186/1471-2334-13-341
Malavige, G. N., Huang, L. C., Salimi, M., Gomes, L., Jayaratne, S. D., and Ogg, G. S. (2012). Cellular and cytokine correlates of severe dengue infection. PLoS ONE 7:e50387. doi: 10.1371/journal.pone.0050387
Malavige, G. N., Jeewandara, C., Alles, K. M., Salimi, M., Gomes, L., Kamaladasa, A., et al. (2013b). Suppression of virus specific immune responses by IL-10 in acute dengue infection. PLoS Negl. Trop. Dis. 7:e2409. doi: 10.1371/journal.pntd.0002409
Malavige, G. N., and Ogg, G. S. (2017). Pathogenesis of vascular leak in dengue virus infection. Immunology 151, 261–269. doi: 10.1111/imm.12748
Malavige, G. N., Wijewickrama, A., Fernando, S., Jeewandara, C., Ginneliya, A., Samarasekara, S., et al. (2018). A preliminary study on efficacy of rupatadine for the treatment of acute dengue infection. Sci. Rep. 8:3857. doi: 10.1038/s41598-018-22285-x
Manokaran, G., Finol, E., Wang, C., Gunaratne, J., Bahl, J., Ong, E. Z., et al. (2015). Dengue subgenomic RNA binds TRIM25 to inhibit interferon expression for epidemiological fitness. Science 350, 217–221. doi: 10.1126/science.aab3369
Modhiran, N., Watterson, D., Muller, D. A., Panetta, A. K., Sester, D. P., Liu, L., et al. (2015). Dengue virus NS1 protein activates cells via Toll-like receptor. 4 and disrupts endothelial cell monolayer integrity. Sci. Transl. Med. 7:304ra142. doi: 10.1126/scitranslmed.aaa3863
Mongkolsapaya, J., Dejnirattisai, W., Xu, X. N., Vasanawathana, S., Tangthawornchaikul, N., Chairunsri, A., et al. (2003). Original antigenic sin and apoptosis in the pathogenesis of dengue hemorrhagic fever. Nat. Med. 9, 921–927. doi: 10.1038/nm887
Mongkolsapaya, J., Duangchinda, T., Dejnirattisai, W., Vasanawathana, S., Avirutnan, P., Jairungsri, A., et al. (2006). T cell responses in dengue hemorrhagic fever: are cross-reactive T cells suboptimal? J. Immunol. 176, 3821–3829. doi: 10.4049/jimmunol.176.6.3821
Murhekar, M. V., Kamaraj, P., Kumar, M. S., Khan, S. A., Allam, R. R., Barde, P., et al. (2019). Burden of dengue infection in India, 2017: a cross-sectional population based serosurvey. Lancet Glob Health 7, e1065–e1073. doi: 10.1016/S2214-109X(19)30250-5
Nascimento, E. J. M., George, J. K., Velasco, M., Bonaparte, M. I., Zheng, L., DiazGranados, C., et al. (2018). Development of an anti-Dengue NS1 IgG ELISA to evaluate exposure to dengue virus. J. Virol. Methods 257, 48–57. doi: 10.1016/j.jviromet.2018.03.007
Netea, M. G., Joosten, L. A., Latz, E., Mills, K. H., Natoli, G., Stunnenberg, H. G., et al. (2016). Trained immunity: a program of innate immune memory in health and disease. Science 352:aaf1098. doi: 10.1126/science.aaf1098
Neves, A. L., Coelho, J., Couto, L., Leite-Moreira, A., and Roncon-Albuquerque, R. (2013). Metabolic endotoxemia: a molecular link between obesity and cardiovascular risk. J. Mol. Endocrinol. 51, R51–R64. doi: 10.1530/JME-13-0079
Ogonda, L. A., Orago, A. S., Otieno, M. F., Adhiambo, C., Otieno, W., and Stoute, J. A. (2010). The levels of CD16/Fc gamma receptor IIIA on CD14+ CD16+ monocytes are higher in children with severe Plasmodium falciparum anemia than in children with cerebral or uncomplicated malaria. Infect. Immun. 78, 2173–2181. doi: 10.1128/IAI.01078-09
Pang, J., Hsu, J. P., Yeo, T. W., Leo, Y. S., and Lye, D. C. (2017). Diabetes, cardiac disorders and asthma as risk factors for severe organ involvement among adult dengue patients: a matched case-control study. Sci. Rep. 7:39872. doi: 10.1038/srep39872
Patro, A. R. K., Mohanty, S., Prusty, B. K., Singh, D. K., Gaikwad, S., Saswat, T., et al. (2019). Cytokine signature associated with disease severity in Dengue. Viruses 11:34. doi: 10.3390/v11010034
Puerta-Guardo, H., Glasner, D. R., and Harris, E. (2016). Dengue virus NS1 disrupts the endothelial glycocalyx, leading to hyperpermeability. PLoS Pathog. 12:e1005738. doi: 10.1371/journal.ppat.1005738
Rathakrishnan, A., Wang, S. M., Hu, Y., Khan, A. M., Ponnampalavanar, S., Lum, L. C., et al. (2012). Cytokine expression profile of dengue patients at different phases of illness. PLoS ONE 7:e52215. doi: 10.1371/journal.pone.0052215
Rathore, A. P., Mantri, C. K., Aman, S. A., Syenina, A., Ooi, J., Jagaraj, C. J., et al. (2019). Dengue virus-elicited tryptase induces endothelial permeability and shock. J. Clin. Invest. 130, 4180–4193. doi: 10.1172/JCI128426
Rathore, A. P. S., Senanayake, M., Athapathu, A. S., Gunasena, S., Karunaratna, I., Leong, W. Y., et al. (2020). Serum chymase levels correlate with severe dengue warning signs and clinical fluid accumulation in hospitalized pediatric patients. Sci. Rep. 10:11856. doi: 10.1038/s41598-020-68844-z
Rodrigo, W. W., Jin, X., Blackley, S. D., Rose, R. C., and Schlesinger, J. J. (2006). Differential enhancement of dengue virus immune complex infectivity mediated by signaling-competent and signaling-incompetent human Fcgamma RIA (CD64) or FcgammaRIIA (CD32). J. Virol. 80, 10128–10138. doi: 10.1128/JVI.00792-06
Shin, N. R., Whon, T. W., and Bae, J. W. (2015). Proteobacteria: microbial signature of dysbiosis in gut microbiota. Trends Biotechnol. 33, 496–503. doi: 10.1016/j.tibtech.2015.06.011
Singla, M., Kar, M., Sethi, T., Kabra, S. K., Lodha, R., Chandele, A., et al. (2016). Immune response to Dengue virus infection in pediatric patients in New Delhi, India–Association of Viremia, inflammatory mediators and monocytes with disease severity. PLoS Negl. Trop. Dis. 10:e0004497. doi: 10.1371/journal.pntd.0004497
St. John, A. L. (2013). Influence of mast cells on dengue protective immunity and immune pathology. PLoS Pathog. 9:e1003783. doi: 10.1371/journal.ppat.1003783
St. John, A. L., Rathore, A. P., Raghavan, B., Ng, M. L., and Abraham, S. N. (2013). Contributions of mast cells and vasoactive products, leukotrienes and chymase, to dengue virus-induced vascular leakage. Elife 2:e00481. doi: 10.7554/eLife.00481
Syenina, A., Jagaraj, C. J., Aman, S. A., Sridharan, A., and St John, A. L. (2015). Dengue vascular leakage is augmented by mast cell degranulation mediated by immunoglobulin Fcgamma receptors. Elife 4:e05291. doi: 10.7554/eLife.05291.008
Tang, Y., Liu, J., Zhang, D., Xu, Z., Ji, J., and Wen, C. (2020). Cytokine storm in COVID-19: the current evidence and treatment strategies. Front. Immunol. 11:1708. doi: 10.3389/fimmu.2020.01708
Tissera, H., Rathore, A. P. S., Leong, W. Y., Pike, B. L., Warkentien, T. E., Farouk, F. S., et al. (2017). Chymase level is a predictive biomarker of dengue hemorrhagic fever in pediatric and adult patients. J. Infect. Dis. 216, 1112–1121. doi: 10.1093/infdis/jix447
Tsai, T. T., Chuang, Y. J., Lin, Y. S., Chang, C. P., Wan, S. W., Lin, S. H., et al. (2014). Antibody-dependent enhancement infection facilitates dengue virus-regulated signaling of IL-10 production in monocytes. PLoS Negl. Trop. Dis. 8:e3320. doi: 10.1371/journal.pntd.0003320
Ubol, S., and Halstead, S. B. (2010). How innate immune mechanisms contribute to antibody-enhanced viral infections. Clin. Vaccine Immunol. 17, 1829–1835. doi: 10.1128/CVI.00316-10
Ubol, S., Phuklia, W., Kalayanarooj, S., and Modhiran, N. (2010). Mechanisms of immune evasion induced by a complex of dengue virus and preexisting enhancing antibodies. J. Infect. Dis. 201, 923–935. doi: 10.1086/651018
van de Weg, C. A., Koraka, P., van Gorp, E. C., Mairuhu, A. T., Supriatna, M., Soemantri, A., et al. (2012). Lipopolysaccharide levels are elevated in dengue virus infected patients and correlate with disease severity. J. Clin. Virol. 53, 38–42. doi: 10.1016/j.jcv.2011.09.028
van de Weg, C. A., Pannuti, C. S., de Araujo, E. S., van den Ham, H. J., Andeweg, A. C., Boas, L. S., et al. (2013). Microbial translocation is associated with extensive immune activation in dengue virus infected patients with severe disease. PLoS Negl. Trop. Dis. 7:e2236. doi: 10.1371/journal.pntd.0002236
van Wilgenburg, B., Scherwitzl, I., Hutchinson, E. C., Leng, T., Kurioka, A., Kulicke, C., et al. (2016). MAIT cells are activated during human viral infections. Nat. Commun. 7:11653. doi: 10.1038/ncomms11653
Vuong, N. L., Le Duyen, H. T., Lam, P. K., Tam, D. T. H., Vinh Chau, N. V., Van Kinh, N., et al. (2020). C-reactive protein as a potential biomarker for disease progression in dengue: a multi-country observational study. BMC Med. 18:35. doi: 10.1186/s12916-020-1496-1
Walterscheid, J. P., Ullrich, S. E., and Nghiem, D. X. (2002). Platelet-activating factor, a molecular sensor for cellular damage, activates systemic immune suppression. J. Exp. Med. 195, 171–179. doi: 10.1084/jem.20011450
Wang, T. T., Sewatanon, J., Memoli, M. J., Wrammert, J., Bournazos, S., Bhaumik, S. K., et al. (2017). IgG antibodies to dengue enhanced for FcgammaRIIIA binding determine disease severity. Science 355, 395–398. doi: 10.1126/science.aai8128
Wang, W. H., Lin, C. Y., Chang, K., Urbina, A. N., Assavalapsakul, W., Thitithanyanont, A., et al. (2019). A clinical and epidemiological survey of the largest dengue outbreak in Southern Taiwan in 2015. Int. J. Infect. Dis. 88, 88–99. doi: 10.1016/j.ijid.2019.09.007
Wang, W. K., Chen, H. L., Yang, C. F., Hsieh, S. C., Juan, C. C., Chang, S. M., et al. (2006). Slower rates of clearance of viral load and virus-containing immune complexes in patients with dengue hemorrhagic fever. Clin. Infect. Dis. 43, 1023–1030. doi: 10.1086/507635
Weiskopf, D., Angelo, M. A., de Azeredo, E. L., Sidney, J., Greenbaum, J. A., Fernando, A. N., et al. (2013). Comprehensive analysis of dengue virus-specific responses supports an HLA-linked protective role for CD8+ T cells. Proc. Natl. Acad. Sci. U.S.A. 110, E2046–E2053. doi: 10.1073/pnas.1305227110
Weiskopf, D., Cerpas, C., Angelo, M. A., Bangs, D. J., Sidney, J., Paul, S., et al. (2015). Human CD8+ T-cell responses against the. 4 dengue virus serotypes are associated with distinct patterns of protein targets. J. Infect. Dis. 212, 1743–1751. doi: 10.1093/infdis/jiv289
WHO (2011). Comprehensive Guidelines for Prevention and control of dengue Fever and Dengue Haemorrhagic Fever. SEARO, New Delhi: World Health Organization.
Wijeratne, D. T., Fernando, S., Gomes, L., Jeewandara, C., Ginneliya, A., Samarasekara, S., et al. (2018). Quantification of dengue virus specific T cell responses and correlation with viral load and clinical disease severity in acute dengue infection. PLoS Negl. Trop. Dis. 12:e0006540. doi: 10.1371/journal.pntd.0006540
Wijeratne, D. T., Fernando, S., Gomes, L., Jeewandara, C., Jayarathna, G., Perera, Y., et al. (2019). Association of dengue virus-specific polyfunctional T-cell responses with clinical disease severity in acute dengue infection. Immun. Inflamm. Dis. 7, 276–285. doi: 10.1002/iid3.271
Wijesinghe, A., Gamage, J., Goonewardena, H., Gomes, L., Jayathilaka, D., Wijeratne, D. T., et al. (2020). Phenotype and functionality of follicular helper T cells in patients with acute dengue infection. J. Biomed. Sci. 27:50. doi: 10.1186/s12929-020-00641-2
Wong, K. L., Chen, W., Balakrishnan, T., Toh, Y. X., Fink, K., and Wong, S. C. (2012). Susceptibility and response of human blood monocyte subsets to primary dengue virus infection. PLoS ONE 7:e36435. doi: 10.1371/journal.pone.0036435
Wrammert, J., Onlamoon, N., Akondy, R. S., Perng, G. C., Polsrila, K., Chandele, A., et al. (2012). Rapid and massive virus-specific plasmablast responses during acute dengue virus infection in humans. J. Virol. 86, 2911–2918. doi: 10.1128/JVI.06075-11
Keywords: dengue, innate immunity, monocytes, mast cells, antibody dependent enhancement, endotoxin, T cells, B cells
Citation: Malavige GN, Jeewandara C and Ogg GS (2020) Dysfunctional Innate Immune Responses and Severe Dengue. Front. Cell. Infect. Microbiol. 10:590004. doi: 10.3389/fcimb.2020.590004
Received: 06 August 2020; Accepted: 15 September 2020;
Published: 23 October 2020.
Edited by:
Vikas Sood, Jamia Hamdard University, IndiaReviewed by:
Henry Puerta-Guardo, Universidad Autónoma de Yucatán, MexicoRebecca Tweedell, St. Jude Children's Research Hospital, United States
Copyright © 2020 Malavige, Jeewandara and Ogg. This is an open-access article distributed under the terms of the Creative Commons Attribution License (CC BY). The use, distribution or reproduction in other forums is permitted, provided the original author(s) and the copyright owner(s) are credited and that the original publication in this journal is cited, in accordance with accepted academic practice. No use, distribution or reproduction is permitted which does not comply with these terms.
*Correspondence: Gathsaurie Neelika Malavige, Z2F0aHNhdXJpZS5tYWxhdmlnZUBuZG0ub3guYWMudWs=