- 1Department of Immunobiology, University of Arizona, Tucson, AZ, United States
- 2BIO5 Institute, University of Arizona, Tucson, AZ, United States
- 3Valley Fever Center for Excellence, University of Arizona, Tucson, AZ, United States
While some bacteria can thrive for generations in minerals and salts, many require lavish nutrition and specific chemicals to survive to the point where they can be observed and researched. Although researchers once boiled and rendered animal flesh and bones to obtain a media that facilitated bacterial growth, we now have a plethora of formulations and manufacturers to provide dehydrated flavors of historical, modified, and modern media. The purpose of media has evolved from simple isolation to more measured study. However, in some instances, media formulated to aid the metabolic, nutritional, or physical properties of microbes may not be best suited for studying pathogen behavior or resilience as a function of host interactions. While there have been comparative studies on handfuls of these media in Streptococcus pneumoniae, this review focuses on describing both the historical and modern composition of common complex (Todd Hewitt and M17), semi-defined (Adams and Roe), and defined pneumococcal media (RPMI and Van de Rijn and Kessler), key components discovered/needed for cultivation/growth enhancement, and effects these different media have on bacterial phenotypes and experimental outcomes. While many researchers find the best conditions to grow and experiment on their bacteria of choice, the reasons for some researchers to use a specific medium is at best, not discussed, and at worst, arbitrary. As such, the goal of this review is to highlight the differences in pneumococcal media to encourage investigators to challenge their decisions on why they use a given medium, discuss the recipe, and explain their reasoning.
Isolation Media for Pneumococcus in the 19th Century
Streptococcus pneumoniae, or the pneumococcus, was fortuitously discovered on two continents simultaneously. In France, Louis Pasteur was studying the etiology of rabies, and in North America, George M. Sternberg was studying malaria. In North America, the future Surgeon General Sternberg inoculated two rabbits with his saliva to test whether an innocuous substance would produce an immune response. To his amazement, both rabbits died from a cause of death distinct from malaria (Sternberg, 1881). Sternberg inoculated additional rabbits with his saliva as well as dogs, chickens, guinea pigs, and rats. This led to septicemia in the rabbits and guinea pigs, but not in the dogs or chickens. However, 1 ml of serum from a recently diseased rabbit put into a dog proved fatal (Sternberg, 1881). Three months later, Pasteur investigated a hospitalized child in the later stages of rabies to procure a saliva sample. When the sample was later inoculated into rabbits two days later, the rabbits died, but not from rabies. Upon microscopic examination, Pasteur observed an “8” shaped microorganism with a halo surrounding it and was only successful in obtaining cultures in veal broth (Pasteur, 1881).
Sternberg encouraged other investigators to test their saliva and later learned that saliva from some, but not all, led to death of the rabbits. This result created speculation about the nature of sepsis and whether it was attributed to putrefaction (decaying of organic matter) (Sternberg, 1881; Talamon et al., 1883). Other types of putrid inoculums, such as sewage, could produce sepsis. However, this, at the time, was mostly attributed to bacilli (Sternberg, 1881). Sternberg found that incubating saliva to the point of putrefaction (usually 24 h at 37˚ C) curtailed its lethality. Further, attempts to isolate the organism (which he would later call Micrococcus pasteuri) straight from saliva into artificial media proved impossible. It is unknown whether this was due to the nature of these media, or what we know now to be the bacterium’s inherent autolytic ability. He tried cultivating the organism in urine, gelatin, and dog bouillon (a broth or extract made by stewing the subject in water) unsuccessfully; however, similarly to Pasteur, if the inoculum was amplified in rabbit’s blood, then it would grow on rabbit flesh bouillon (Sternberg, 1881). A bacteriology manual from 1896 written by Sternberg explains bouillon preparation as ~500 g of chopped meat per liter of water, cooked for 30 minutes in a glass flask or enameled iron kettle, then filtered, neutralized, cooked again to coagulate and remove albuminoids, filtered, and finally sterilized (Sternberg, 1896). Others from the era utilize a different procedure; half a kg of chopped meat is soaked in 1 L of cold water for 24 hours, strained to the same volume, combined with 1% peptone w/w (partial enzymatic digestion or acid hydrolysis of yeast, animal, or plant tissues), and 0.5% NaCl w/w (Stedman, 1900). The mixture was then alkalinized by addition of sodium carbonate, heated to coagulate and precipitate albumins, filtered, and finally sterilized by autoclave.
In November 1883, French physician Charles Talamon was the first to publish the isolation of a pure culture of the pneumococcus (Coccus lanceolatus) (Talamon et al., 1883). He described taking his inoculum from the blood of rabbits and culturing it in “bouillon de Liebig,” from Liebig’s Extract of Meat Company which was famous in the late 1800s for making a variety of products from meat sources (Cansler, 2013). The preparation of bouillon de Liebig included peptone plus sodium chloride, in combination with meat (by record, most likely beef) infusion (the product of allowing the meat to stay in a solvent for a prolonged period of time), and then alkalizing by either adding NaHCO3 directly or passing the medium through a carbonate column. The addition of the infusion or extract supplemented the peptone by adding minerals, phosphates, and vitamins. This medium differed from those prepared in labs by skipping the coagulation and removal of albumin from the meat infusion. However, it is now known that albumin can actually promote pneumococcal growth at low concentrations (Ruiz et al., 2011).
In 1892, William H. Welch published a research article describing the history of the pneumococcus (Micrococcus lanceolatus), as well as many characteristics of the organism (Welch, 1892). He described the pneumococcus’ preference for alkaline media and its ability to grow on solid media such as nutrient agar, which is 5 g Liebig bouillon, 30 g peptone, 5 g cane sugar (sucrose), and 15 g agar, and “glycerine agar,” which is nutrient agar + 5% glycerine/glycerol. In his communication, Welch mentioned his preference for a modification of Guarnieri’s formulation which contains per liter 950 g of meat infusion, 5 g of NaCl, 10 g of peptone, 6-8 g agar, and 30-40 g of gelatin (a product of cooking collagen) with slightly alkaline pH (Welch, 1892).
Sources of Variability in Media Components
Variability in media was a major problem during earlier investigations on S. pneumoniae. Media were composed of a few undefined components and prepared by different methods. Different meat sources, qualities, and preparation (soaked vs. cooked) greatly affected the nutritional components of the media. As such, Welch and others had challenges in obtaining quality peptone capable of sustaining pneumococcal growth, even within the same brand (Fennel and Fisher, 1919). Peptones supply a source of amino acids and nitrogen for bacterial growth. However, because the raw input can be sourced from plants (soy), animals (milk, fetal sera) and microbes (yeast), their amino acid profiles are diverse. There are several methods used to process raw protein sources. For example, peptones derived from albumins are subjected to gastric and pancreatic digestion, acid hydrolysis, heating, and precipitation (Zimbro and Power, 2009). Thermal degradation of bulk protein can convert amino acids such as tryptophan to indoles or phenols (Kato et al., 1971). During acid hydrolysis, tryptophan can be completely lost, cystine can be partially broken down, and labile vitamins are mostly destroyed (Zimbro and Power, 2009). Enzymatic digest is gentler, but each proteolytic enzyme has a distinct cleavage pattern, which leads to the diverse array of molecular weight products. Finally, all of these changes take place before the media are autoclaved.
Another nuisance involving peptones is their ability to retain carbohydrate contaminants. In 1950, The American Journal of Public Health conducted a survey that evaluated 450 samples of peptones and 60 samples of bacteriological culture media for the presence of fermentable carbohydrates (Vera, 1950). The samples were tested against several bacteria including Streptococcal spp. The study concluded that all peptone sample sources, and most meat sources were not suitable for bacterial fermentation tests as they still contained fermentable substances detected via acid or acid gas production. Recent documentation provided by different manufacturers also indicates the presence of carbohydrates in peptones from diverse origins (Klotz et al., 2017). This observation was significant, given that large segments of pneumococcal DNA are devoted to carbohydrate metabolism and transport (Bidossi et al., 2012). Additional work by Troxler et al. demonstrated that choice of carbon source had an impact on capsule expression and growth as sugars like fructose are not converted into capsule precursors thus limiting capsule production (Troxler et al., 2019).
Other points of media variability include vitamin and trace metal content. Not surprisingly, the accumulation of vitamins and trace metals vastly differs between animal and plant sources of peptones. For example, while biotin (vitamin B7), nicotinic acid (vitamin B3, nicotinic acid, or niacin is a precursor for nicotinamide adenine dinucleotide), and pantothenic acid (vitamin B5 and necessary for Coenzyme A production) were deemed important vitamins (Rane and Subbarow, 1940; Badger, 1944), Stokes et al. reported that the concentration of these three vitamins varied significantly between different nitrogenous sources (Stokes et al., 1944). This report describes a 60-fold difference in pantothenic acid concentration, 34-fold in nicotinic acid, and 17-fold on biotin among different nitrogen sources.
Trace metals are often important catalytic components for enzymatic reactions, and in doing so, affect gene expression, bacterial growth, and development. They can also be toxic in excess. As such, a bacterium’s ability to maintain metal homeostasis is essential for survival. However, historically, there have been discrepancies in trace metal concentrations (Grant and Pramer, 1962; Nolan and Nolan, 1972). A modern analysis reports 2-fold differences in zinc and iron concentrations, and a 40-fold difference for copper between yeast extracts and casein or meat sources (Klotz et al., 2017). In high concentrations, copper has been shown to be toxic to pneumococcal growth by inhibiting nucleotide synthesis (Johnson et al., 2015). High zinc concentrations have also shown to be toxic to the pneumococcus by competing with manganese uptake (Eijkelkamp et al., 2014). Elevated exogenous iron has been implicated in enhancing pneumococcal biofilm formation and competence (Trappetti et al., 2011).
Perhaps not part of a specific medium perse, but the culturing environment is also important for growing bacteria. In the 1930’s Gladstone et al. illustrated the importance that CO2 can have in the cultivation of other bacteria (Gladstone et al., 1935). CO2 greatly improves S. pneumoniae growth as specifically demonstrated in 1942 by Kempner and Schlayer (Kempner and Schlayer, 1942). Under ambient CO2 concentrations (~300 ppm), S. pneumoniae grew in peptone, beef infusion, and glucose broth at higher pH (7.8 vs. 7.4). They postulated that adding CO2 should lower the pH and thus, reduce pneumococcal growth, but that the opposite occurred. It is known that the pneumococcus possesses a highly conserved, β-class carbonic anhydrase (turning CO2 and water into carbonic acid) that is necessary for growth in ambient air (Smith and Ferry, 2000; Burghout et al., 2010). As such, modern aerobic cultivation of pneumococcal species often requires, or is improved by providing an enriched 5% CO2-atmosphere as the benefits of CO2 outweighed the resultant media acidity.
Growth media development and use have come full circle. From local raw feedstocks, to gradually refined requirements for optimal cultivation, and back to undefined media for convenience’s sake. However, while most manufacturing processes have been standardized to minimize variance across batches in modern media, they still rely on disparate ingredients for the cultivation of microorganisms. The road to discovering essential nutrients required for pneumococcal growth has been challenging given the fact that so many serotypes exist (100 different capsules define these serotypes) with some being more fastidious than others. While in some cases, the determination of specific ingredients tested a hypothesis, in other cases, it was trial and error to achieve a predetermined goal such as obtaining a pure culture. In the following paragraphs, we introduce some of these media (Table 1) and define them to the extent that historical records allow.
Pneumococcal Media
Todd Hewitt
Todd Hewitt broth with yeast extract is a popular liquid media used for the cultivation of S. pneumoniae. Its inception stems from studies by Todd and Hewitt on the metabolic functions of the pneumococcus (Todd and Hewitt, 1932). The earlier version of this broth constituted of a meat broth with added 2% proteose peptone (enzymatic digest of protein), diluted tenfold in a buffered salt medium, bubbled with CO2 to reach a pH of 6.8, and lastly supplemented with glucose (Table 2). They later added to this medium by supplementing with metals and phosphate (Hewitt, 1932). Hewitt’s experiments (discussed below) not only demonstrated the importance of phosphate addition to media as a buffering agent, but it was also significant for the determining metabolism of Streptococci. These studies culminated with the current version of Todd Hewitt broth, later streamlined by Updyke with Difco in the commercially available dehydrated version (Updyke et al., 1953) (Table 3).
The original Todd Hewitt formulation was used to describe lactic acid formation from glucose breakdown in cultures of hemolytic Streptococci sp. (Todd and Hewitt, 1932). Moreover, Todd and Hewitt found that when inorganic phosphate was added to their formulation, there was a significant increase in the percentage of glucose breakdown and this effect was concentration dependent. Experiments by Mason et al., 1981 expanded on the glucose-phosphate idea. They discovered a correlation between high phosphate concentrations inhibiting pyruvate kinase in starved Streptococcus lactis cells (Mason et al., 1981). Starved cells returned to homeostasis after glucose addition (Mason et al., 1981).
Currently, different manufacturers of Todd Hewitt broth further adjust their formulations, for example: BD Bacto and Hardy Diagnostics add 3.1 g/L of beef heart infusion, while HiMedia adds 10.1 g/L, and Millipore includes 10 g/L (Table 3). Further, Hardy Diagnostics adds yeast in their powder while other formulations, it can be added separately. Additionally, the other peptone sources also differ by including enzymatic digest of milk protein or enzymatic digestion of animal tissues. Due to the known carbohydrates in peptone, and their effect on many cellular processes not limited to metabolism and capsule production, it is conceivable that these differences could affect the outcome of an experiment.
Rane and Subarrow
Rane and Subarrow (Table 4) devised a defined medium to determine the nutritional requirements of at least five different serotypes (Rane and Subbarow, 1940). Rane and Subbarow substituted the complex component gelatin with a defined mixture of amino acids and other additives in their basal medium I to make basal medium II. From this new formulation, they identified choline, pantothenic acid, nicotinic acid, and amino acids arginine, histidine, and glutamic acid as essential for growth (Rane and Subbarow, 1940).
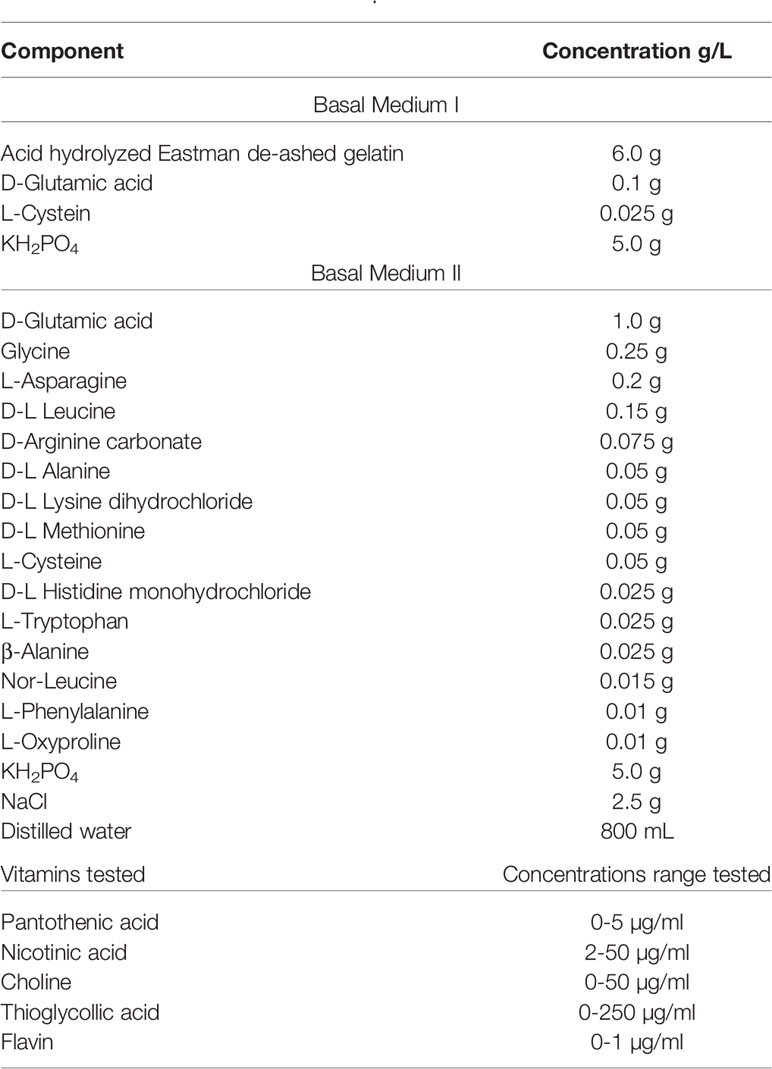
Table 4 Rane and Subbarow, 1940.
Choline is an important structural component of Streptococcal teichoic acid in the cell wall based on cell fractionation experiments (Mosser and Tomasz, 1970). It was also determined that choline incorporation in the cell wall was necessary for the autolytic process, as cells treated with ethanolamine (EA), which can incorporate itself into the teichoic acid, were resistant to autolysis. Briles et al. in 1970 furthered these studies and demonstrated that cells initially grown with 3H-labeled choline, which were later moved to EA media, resulted in cells that couldn’t separate post division, and thus formed long chains instead of the usual diplococci phenotype (Tomasz, 1968; Briles and Tomasz, 1970). Additionally, it was demonstrated that cells grown without choline were less likely to uptake DNA during transformation citing inability to respond to competence factor (Tomasz, 1968; Tomasz et al., 1971).
Adams and Roe
Adams and Roe (Table 5) devised a medium to grow more fastidious pneumococcal serotypes as they were unable to subculture their isolates with the Rane and Subarrow medium and examine sulfonamide resistant bacteria (Adams and Roe, 1945). This medium is based on a 1942 recipe for growing hemolytic Streptococci (Bernheimer et al., 1942). It is a semi-defined medium that contains hydrolyzed casein as an undefined ingredient as meat infusion and peptones had sulfonamide inhibitors. While arginine and histidine were determined to be essential for pneumococcal growth, they were not added explicitly here. However, undefined components (e.g., casein digest) can contain as much as 4.8% arginine (Klotz et al., 2017) and 4.8% histidine (Becton-Dickinson, 2006). After this enzymatic hydrolysis, significant amounts of these amino acids are available in small and more bio-available oligopeptides. With their new medium, Adams and Roe were able to both subculture their strains and identify asparagine as crucial to sulfonamide-resistant Streptococcus (Adams and Roe, 1945).
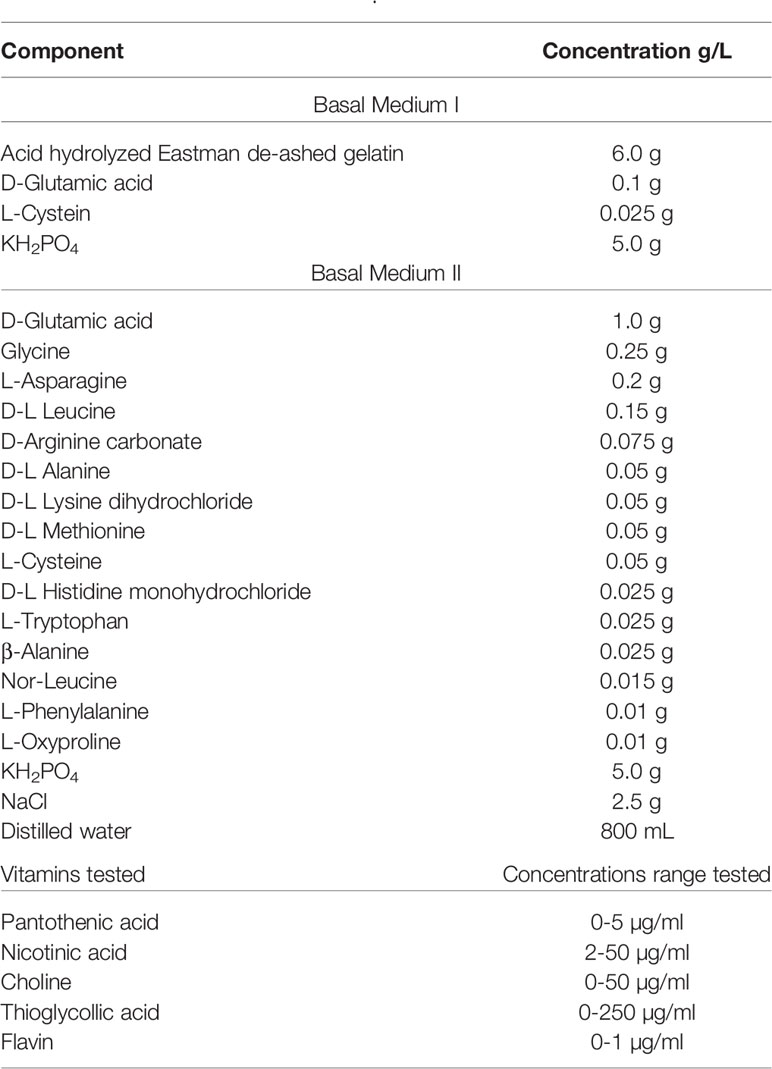
Table 5 Adams and Roe, 1945.
Hoeprich would extensively modify this medium five years later: adding L-tyrosine increasing concentrations of iron, potassium, phosphate, glucose, glutamine, asparagine, uracil, adenine, choline, Vitamins B1-3, 5-7 (thiamine, riboflavin, nicotinic acid, pantothenate, pyridoxine, and biotin respectively) omitting copper and calcium; and decreasing the concentrations of zinc and manganese (Hoeprich, 1955). The addition of uracil to growth media was not pioneered by Hoeprich, as this ingredient appeared in earlier versions of Rane and Subarrow’s medium and in other groups studying Streptococci. Nevertheless, many of the preceding media include uracil or uridine (glycosylated uracil) as an important component.
The physiological relevance of uracil was explored by Carvalho et al. in 2013 where they demonstrated that the lack of uracil increased the lag phase of D39 but had no effect on the R6 strain (and unencapsulated derivative of D39) (Carvalho et al., 2013). Ultimately, uracil was found to be involved in pneumococcal capsule production by 1) measuring less capsule production in uracil deficient media; 2) a spontaneous D39 capsule production mutant was largely nonresponsive in growth in the presence of uracil; and 3) the native D39 cps promoter was 25% lower without uracil present demonstrating effects at a transcriptional level (Carvalho et al., 2018).
Lacks and Hotchkiss
Another widely used, semi-defined media is Lacks and Hotchkiss (Table 6), also referred to as a modified Adams and Roe (1945). It has been the base for many modern media. This medium incorporated complex components such as yeast extract, casein hydrolysate, and BSA (Lacks and Hotchkiss, 1960). It also eliminated pure components that were deemed important to growth in previous work such as choline, arginine, histidine, and glutamic acid/glutamine. In 1966, Lacks media was revised to include: asparagine, glutamine, adenine, choline chloride, sodium bicarbonate and catalase (Lacks, 1966). S. pneumoniae is known to produce high levels of H2O2 but lacks catalase (Tettelin et al., 2001). In fact, the level of H2O2 that S. pneumoniae can make approaches low mM levels and can be detected in the breath of pneumococcal infected individuals (Majewska et al., 2004; Erttmann and Gekara, 2019).
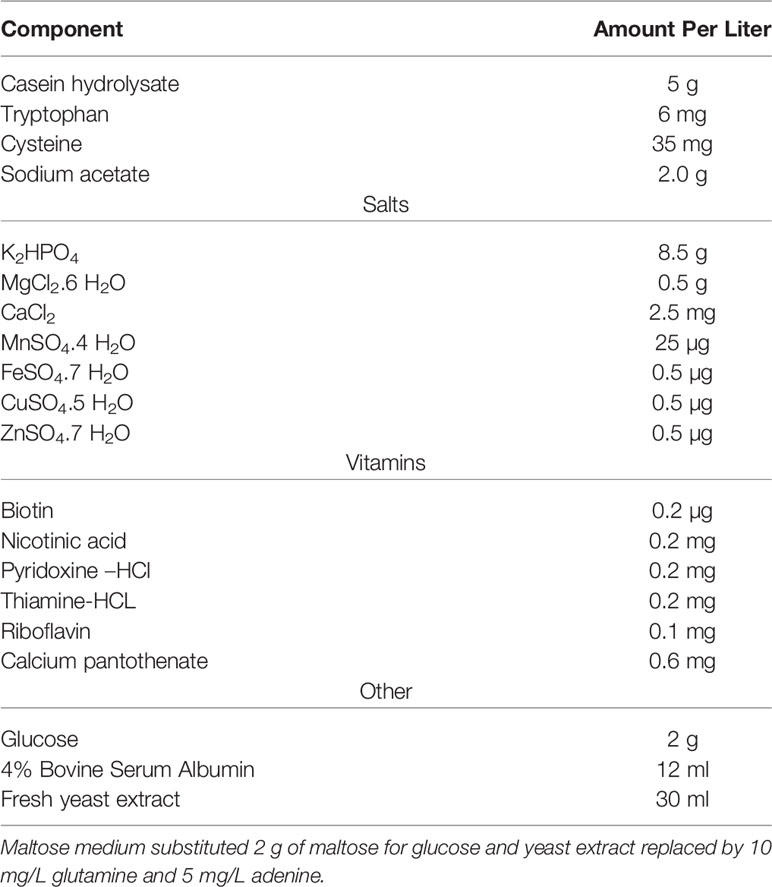
Table 6 Lacks and Hotchkiss, 1960.
Sicard
In 1964, Sicard developed a fully synthetic (defined) medium as the others “have proven inadequate for the culture of strains used in this laboratory” (Table 7) (Sicard, 1964). He was working with a type 2 rough strain or Avery’s (Avery et al., 1944). While known at the time, Sicard’s work solidified that different strains of the pneumococcus varied in the media that they could grow. Sicard used either linoleic acid, spermidine, or bovine serum albumin in this new medium (see Table 7). Unsaturated fatty acids (such as oleic, linoleic, and linolenic acids) have been reported to improve the growth of S. anginosus as well as increase the production of capsule polysaccharide under low CO2 conditions (Matsumoto et al., 2019). Furthermore, Gullett et al. (2019) demonstrated that host polyunsaturated fatty acids are bound by a protein called FakB3 to enable the utilization of polyunsaturated fatty acids for incorporation into its membrane and therefore, minimize the metabolic expenditure of de novo membrane precursor synthesis (Gullett et al., 2019).
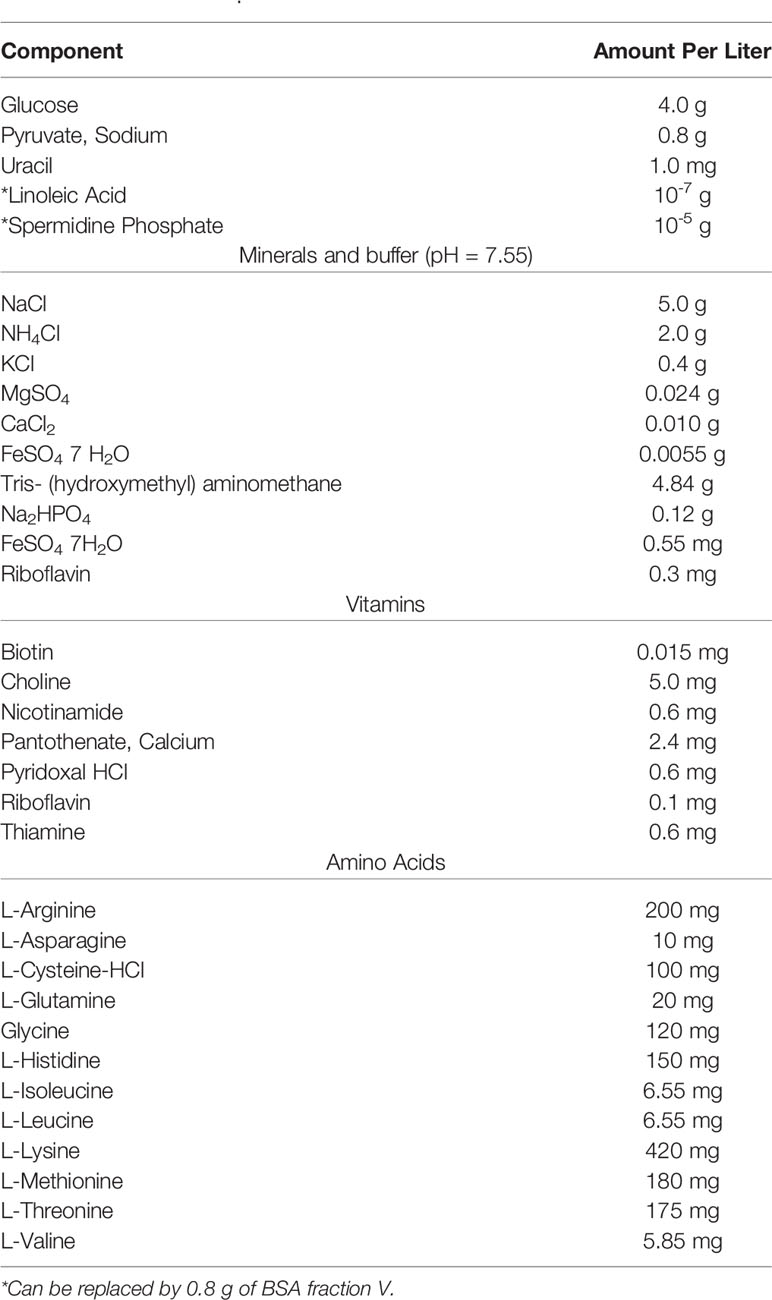
Table 7 Sicard, 1964.
M17
M17 was developed in 1975 as a complex media for the improved cultivation of lactic acid bacteria particularly, lactic acid producing Streptococci sp. (Terzaghi and Sandine, 1975) (Table 8). M17 improved on its predecessor M16 with the addition of disodium glycerophosphate (GP). Phosphate was omitted in M16, relying on peptone and acetate for buffering capacity, as it would precipitate with calcium during phage assays. While having buffering capacity, GP can form a soluble complex with calcium. With this increased soluble interaction with metal, GP helped maintain the pH of the growth medium leading to improvement in cultivation as previously with some Streptococci sp., they had observed a pH below 6 after 24-hour growth at 30°C. Presently, different manufacturers offer a near-identical product through their websites. However, the Millipore Sigma formulation contains a combination of digested protein source (Table 8). Usually, but not in all cases, the product arrives without a carbon source for the investigator to provide post sterilization. The suggestion is 14.6 mM (5 g/L) of lactose; however, other sugars have also been used in the literature most commonly, glucose.
Van de Rijn and Kessler
Van de Rijn and Kessler formulated a chemically defined medium (CDM) that would yield high growth, short lag phase, and most notably, accurate virulence factor production for Group A streptococci (GAS), after previous CDMs did not support their needs in these areas (Table 9) (Van De Rijn and Kessler, 1980). The main purpose of obtaining this faster lag phase was to eliminate the selection and adaptation of bacterial during transfer from complex media, mostly, in reference to making M protein, for which, they were successful. This medium was also successful in that it allowed for growth small inoculum to be used with a short lag time and the doubling time was comparable to other complex media (Todd Hewitt) with a higher buffering capacity. They empirically determined this medium’s components which contained vitamins, minerals, trace metals (except zinc and copper), purines, sodium bicarbonate, and phosphates (Table 9).
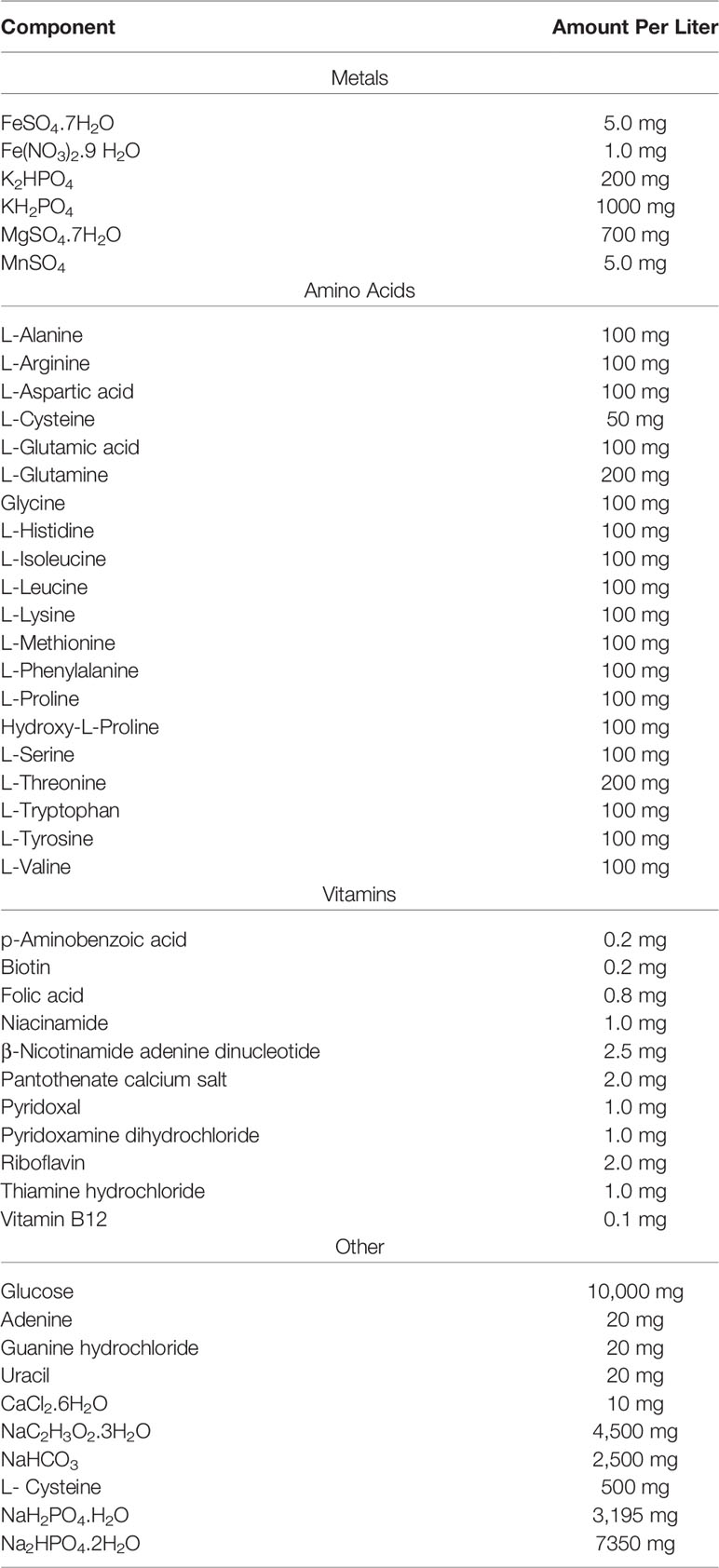
Table 9 Van De Rijn and Kessler, 1980.
RPMI 1640
RPMI 1640 is a completely defined, well-known cell culture formulated at Roswell Park Memorial Institute (Table 10). Growth in this medium was likely adopted due to it being defined and its popularity in culturing mammalian cells, in which both (presumably) host cells and bacteria would have the same nutritional availability. RPMI was used for the cultivation of the pneumococcus, with a few modifications such as the addition of 0.4% BSA, 1% vitamin solution from Sicard, and 2 mM glutamine (Sicard, 1964; Brown et al., 2001). In 2014, Schulz devised a number of supplements suitable for the cultivation of the pneumococcus in RPMI 1640 which included 30.5 mM glucose, 0.65 mM uracil, 0.27 mM adenine, 1.1 mM glycine, 0.24 mM choline chloride, 1.7 mM NaH2PO4·H2O, 3.8 mM Na2HPO4, and 27 mM NaHCO3 (Schulz et al., 2014).
Host-Like or Niche-Specific Media
For early clinical microbiology, a rate-limiting step was consistent isolation and propagation. Understandably, early culture media were nutrient-rich to ensure replication of the inoculum for differential diagnosis or experimentation. We will later highlight that pneumococcal growth rates, gene transcription, protein expression, and virulence (including the capsule) are known to vary by media type and O2 concentration. However, these rich media were not designed to reflect the abundance (or chemical form) of nutrients in vivo. A prime example is the choice of carbon source, or fermentable sugar, where glucose is often used. S. pneumoniae grows fast and vigorous in glucose, but rarely encounters this sugar except in the blood and cerebrospinal fluid (CSF) niches. The host-pathogen interface is a critical area of pneumococcal research and several studies have developed or used media intended to replicate conditions within the hosts, especially given the range of niches (e.g., nasopharynx and pulmonary epithelia, blood, CSF) in which S. pneumoniae persists.
It has been shown that S. pneumoniae grows equally fast in minimal media supplemented with porcine gastric mucin versus culture in BHI (Yesilkaya et al., 2008). Mucins are large proteins, extremely abundant in the inner walls of respiratory epithelia, and heavily glycosylated leading to molecular weights in mega-Dalton range. Despite a relatively small genome, S. pneumoniae encodes dozens of deglycosylases, proteases, and importers which enable the pathogen to turn an innate immune barrier (mucous) into a buffet (Bidossi et al., 2012; Buckwalter and King, 2012).
Most recently, van Beek et al. explored metal availability in the host nasopharynx by measuring the levels of free and protein bound metals in human nasal fluid of healthy individuals (van Beek et al., 2020). When comparing their CDM as defined by the Kloosterman et al. paper for “extending the molecular toolbox” of the pneumococcus against nasal fluid, it was found that nasal fluid contained higher levels of calcium (~10x), magnesium (~1.5x), copper (~10x), and iron (~12x) while having lower concentrations of manganese (>250x), cobalt (>15x), and zinc (~1.5x) (Kloosterman et al., 2006; van Beek et al., 2020). Of note, most of the iron, copper, and zinc in the nasal fluid were protein-bound and not free. To better mimic the host environment, van Beek et al. made an in vivo mimicking CDM, termed IVM-CDM, that more closely resembled the nasal fluid with no metal concentration differing by more than 20% (van Beek et al., 2020). This host-pathogen interface is of particular interest in pneumococcal colonization and by creating a chemical defined media containing similar concentrations of metals available in the nasopharynx, van Beek et al. was able to bring the field closer to a recipe that truly resembles the host niche.
Using the IVM-CDM, van Beek et al. also highlighted nine proteins that were surface exposed, conserved, most part of the core genome (8/9), and expressed in increased abundance during colonization as potential vaccine antigens. Five of these proteins induced antibody responses in mice—PsaA (Pneumococcal surface adhesin A), MetQ (D-methionine-binding lipoprotein), AdcAII (zinc-binding lipoprotein), PrtA (serine protease, and AliA (Oligopeptide-binding protein)—with AliA having the most protective effect as measured by reduced bacterial load (van Beek et al., 2020). As such, growing bacteria in media mimicking their native environment here and detailed in other protocols (Suárez and Texeira, 2019) is an effective method in the quest to make new treatments and vaccines.
Blood Agar Plates
One of the benefits of growing S. pneumoniae in blood agar plates is their distinctive colony morphology and alpha-hemolytic characteristic which quickly aids in its identification. However, even this simple method isn’t standardized among investigators. Two commonly used bases for blood agar plates are tryptic soy agar or Columbia agar (Tables 11 and 12). These base media also differ in their composition, with Columbia agar having a wider source of amino acids as well as the addition of corn starch (Ellner and Stoessel, 1966), which enhances hemolytic reactions as well as marking differences in colony morphology between Streptococcal spp. The most used blood sources are defibrinated 3-10% (5% being most common) sheep or horse blood, but rabbit blood has also been used as an agar base. Mammalian blood is a poorly defined media supplement as it contains tens of thousands of protein isotypes in various states (e.g., phosphorylated, glycosylated, lipidated). This is especially true with lysed blood.
Early comparisons between the species’ blood (with or without cell lysis) in culture media demonstrated that the sensitivity of bacteria to sulfonamide and diaminopyridine. Ferone et al. reasoned that this effect of lower effectiveness was due to an excess of a supplemental nutrient (Ferone et al., 1975). It was important to remove the source of inhibition—i.e., the active factor(s) in the blood—using solid Mueller-Hinton Broth (MHB) plates. To test their hypothesis, they supplemented this media with a downstream product, thymidine, which requires folate metabolism. By supplying this downstream metabolite, any drug targeting folate or nucleotide synthesis would have little effect. Ferone et al. found that horse and human blood contain thymidine phosphorylase (absent in sheep’s blood), also known as Harper-Cawston factor (Harper and Cawston, 1945). This protein phosphorylates, then cleaves thymidine into thymine and 2-deoxyribose-1-phosphate. Naturally, some bacteria are less efficient using thymine over thymidine, which led to results that varied by the species of blood used. It is likely that abundant and resilient proteins (e.g. catalase) retain substantial activity—despite the heat shock of mixing with liquid agar—and interact with media nutrients and bacterial metabolites as small molecules diffuse through the agar matrix demonstrated in antimicrobial disk diffusion assays. However, little is known regarding the persistence and dynamics of blood protein biochemistry in agar or co-culture with pathogens.
Anaerobic Growth
Pneumococcus is habitually cultured as an aerobe with 5% CO2 atmosphere, where it seems to thrive despite lacking catalase or cytochromes. However, it is a facultative anaerobe. Many different methods have been used to produce hypoxic environments such as Gas-Pak® systems, anaerobic chambers, and oxygen scavengers. Anoxic growth has been previously reported to facilitate the isolation of clinical samples. Howden used an atmosphere of 90% hydrogen and 10% CO2 to facilitate the isolation of clinical isolates (Howden, 1976). Four years later Wu et al. compared three methods for the recovery of Streptococcus from lower respiratory secretions and found that using a Gas-Pak® allowed them to recover 93% of their clinical specimens (Wu et al., 1980). The increased isolation was hypothesized to be due to a shortened lag phase during anaerobic growth and a reduction in autolysin production under anaerobic conditions (Howden, 1976; Nagaoka et al., 2019). However, conversely, Baesman and Strand (1984) compared isolation using aerobic and anaerobic methods (a Bio-Bag Environmental Chamber A anaerobic system), observing no significant difference between either (Baesman and Strand, 1984).
Despite the hundreds of isolates gathered across these three studies, none of them mentioned the serotype for any isolate. While the CDC published a widely used isolate culture method using broth enrichment (Todd Hewitt broth supplemented with 1 mL of rabbit serum), followed by real-time PCR of lytA (pneumococcal autolysin) for identification in 2010 (da Gloria Carvalho et al., 2010), Tothpal et al. performed a major survey of 256 pneumococcal strains under different experimental conditions including temperature and oxygen availability while including typing (Tothpal et al., 2019). For an anaerobic environment, they utilized the oxygen scavenger Oxyrase®. This study showed that there was significant variation between the serotype growth in anaerobic and aerobic environments, and variations thereof—such as serotype 4— which grew to higher optical density when cultured aerobically with catalase than anaerobically (Tothpal et al., 2019). In spite of ambient O2, catalase use in the aerobic growth media supported higher maximum growth. Therefore, culture density for some serotypes is linked to the flux and concentration of H2O2 (in the presence of glucose), and was determined by capsule switching experiments, not linked to the capsular type alone.
While this is not an exhaustive list of all pneumococcus solid and liquid media, it covers most reported growth media. Considering S. pneumoniae is genetically diverse with an estimate of 50% core genome at the species level, there is a strong possibility that variations in the nutritional value of these media can have an impact on bacterial physiology (Donati et al., 2010).
Media Matters: Effects of Media on Gene Expression and Metabolism
There are many nuances to microbial culture media, especially those reported for growing S. pneumoniae. It is evident that these media can vary in amino acid composition, abundance of vitamins, metals, carbon source availability, and more. In the previous sections, we described how different media was developed to grow fastidious pneumococcal serotypes (to higher OD and with shorter lag time) and mimic the host environment. Below we present specific examples of how media can change capsule dynamics, gene expression, metabolism, protein profiles, and antibiotic susceptibility. Ultimately, studies rarely focus on comparing microbial behavior at the genetic, proteomic, and metabolomic level in different media, thus this is a limitation in finding examples.
Antibiotic Susceptibility
While the host makes itself non-hospitable to pathogenic bacteria, sometimes it needs assistance in the form of an antibiotic. Bacteria are quite adaptable in forming resistance to these drugs by mutating the target of the antimicrobial compound or by making efflux pumps to export them. It is important to have a standardized measure of bacterial susceptibly to a given antibiotic. Antimicrobial susceptibility testing (AST) is done in vitro using media like blood agar plates usually with the base of Mueller-Hinton Broth (MHB), a rich medium, to find minimum inhibitory concentration (MIC) (Clinical and Laboratory Standards Institute, 2012). For the pneumococcus, the standard AST procedure as defined by the Clinical and Laboratory Standards Institute (CLSI) uses cation adjusted MHB (calcium at 20 to 25 mg/L and magnesium at 10 to 12.5 mg/L) with 2.5-5% lysed horse blood (Clinical and Laboratory Standards Institute, 2012). AST is critical in tracking geographic and phenotypic changes over time in global antibiotic resistance. When developing candidate antibiotics, this same assay is used to establish the feasibility and capability of a hit compound. Given what we have briefly reviewed on media design and use, such a rich media may not be suitable and may be a limitation of these assays.
While several laboratories have investigated AST in other organisms (Rajput et al., 2019; Salazar et al., 2020), Ersoy et al. in 2017 looked at the pneumococcus as a bacterium of study (Ersoy et al., 2017). In comparing these effects of host-like media and other complex media on antibiotic resistance, the MICs of some drugs varied by at least a factor of 4 (Ersoy et al., 2017). Overall, Ersoy et al. found that the standard AST of MHB frequently failed to adequately identify antibiotic susceptibility in vivo. They also provide evidence that host-like media could give be more predictive of MIC in vivo (Ersoy et al., 2017). In one example, the folate-targeting drug trimethoprim was shown to be effective against most tested serotypes of S. pneumoniae using standard AST as defined by the CLSI, but ineffective in the host-like conditions Lacks and modified Lacks medium (Clinical and Laboratory Standards Institute, 2012). Following up, mice were infected with S. pneumoniae and treated with trimethoprim, which failed to protect any of the ten mice. In this case, and in many others that they tested, the standard MIC test did not accurately predict the drug’s efficacy, but the host-like conditions did. These measurements extended themselves to different serotypes demonstrating that two serotypes (6 and 23) were more resistant to ampicillin and ceftriaxone with the D39 strain exhibiting less resistance to azithromycin and erythromycin. Ersoy et al. also examined the influence of NaHCO3 concentration on resistance finding that as a trend adding physiological levels of sodium bicarbonate to MHB improved the in vivo prediction (Ersoy et al., 2017).
While conclusions regarding resistance and susceptibility differ from those obtained by CLSI methods, and thus must be looked at with scrutiny, it is nevertheless striking, but not surprising that host-like media give more reliable results than complex media. Especially in the search for new antibacterial mechanisms, standard AST media may obfuscate the effects of an otherwise promising drug. The MIC of any drug against a target organism not just a function of genome but can be strongly impacted by changes in the environment. It is further complicated by the dynamics of the other bacteria in the environment which could convey unexpected resistance (Sorg et al., 2016). The nutritional environment is a dynamic and strong factor in the laboratory, but especially at the host-pathogen interface.
Capsule
S. pneumoniae dedicates 30% of its membrane transport systems to carbohydrates as it is their only source of energy (Buckwalter and King, 2012). Therefore, it is not far-fetched to imagine that quantity and type of carbon source is linked to gene expression. An example of how carbon sources can impact gene expression is illustrated by Troxler et al. (2019). This work investigated capsule thickness using FITC dextran exclusion assay for serotype 6B and 7F under different carbon sources (glucose, fructose, and sucrose) on CDM, which here was modified Lacks medium with single carbon sources added. They found a significant decrease in capsule thickness when cultured on fructose as the only carbon source and an increase in capsule thickness when grown on glucose or sucrose as confirmed by transmission electron microscopy (TEM). The capsule was mostly generated by the glucose carbons while the fructose carbons were metabolized mostly for glycolysis. Additionally, they tested intracellular metabolites from all three carbon sources using 31P labeled NMR and confirmed the accumulation of capsule precursors made from each carbon source in a capsule knockout model. Finally, gene expression was studied using RNA-seq. This revealed changes in the peptidoglycan synthesis genes glmS and nagA as well as pyrimidine synthesis genes changing in a carbon source dependent manner, indicating an important role for carbohydrate sources in nutrient restricted media. This point is especially poignant in the host where these sources are limited and therefore affect capsule size. Capsule size has a negative correlation with binding to luminal mucus and positive correlation with spreading to other host surfaces (Nelson et al., 2007).
Hathaway et al. tested pneumococcal mutants that could switch their capsules between two different types to determine if serotype was an important factor for growth and carriage of pneumococcus (Hathaway et al., 2012). This paper found that making different capsules is energetically costly and as such, available nutrients affect colonization in terms of long-term vs invasiveness, and growth (Hathaway et al., 2012). Inadvertently, this study found that capsule thickness increased when the pneumococcus was cultivated in rich media such as brain heart infusion with fetal calf serum as compared to cultivation in minimal media (Hathaway et al., 2012). Taken together, these studies imply that the choice and components of media directly impact capsule production and therefore can influence measures of infection.
Co-infections can also affect how the pneumococcus obtains and processes carbohydrates and morphology. This is most notably manifested in its co-infections with influenza and Haemophlius influenzae. Viral influenza infections increase pneumococcal access to sialic acid in the nasopharynx (Siegel et al., 2014). Sialic acid, which in addition to its ability to be a sole carbon source can also be a major component in pneumococcal capsule (Charland et al., 1995; Marion et al., 2011). During long term co-culture (more than 10 days) with H. influenzae, as without H. influenzae there was no pneumococcal growth, glucose and lactose produced varying outcomes in culturability with the latter causing a morphology change (small colony variant) and reduce capsule size (Tikhomirova et al., 2018). Co-infection between these two pathogens can also lead to increased pneumococcal biofilm production, however, resulting in decreased infection (Weimer et al., 2011).
Transcriptomics and Proteomics
The availability of resources causes many binary changes leading to a cascade of effects at the genetic level. In an ambitious study, Aprianto et al. categorized the transcriptomes of the pneumococcus by mimicking various host environments and made a graphical user interface (https://veeninglab.com/pneumoexpress) that allows the end user to see the different expression profiles (Aprianto et al., 2018). To achieve the 22 conditions that mimicked various stages and types of infection, they varied sugar type and concentration, protein, CO2, temperature, acidity, and presence of epithelial cells selecting Sicard’s defined medium as the backbone of many of the conditions (Sicard, 1964; Aprianto et al., 2018). This study was notable due to the few comprehensive environment transcriptome databases that exist for model bacteria. The authors state that one limitation is that they did not vary metal ions which also play a large role in infection, however, above, we detailed the effects of metals in finding vaccine candidates (van Beek et al., 2020). Aprianto et al. found that there were 498 conditionally expressed genes based on the media conditions with ~10% of these genes involved in regulating carbohydrate import (many of which are under the control of CcpA (catabolite control protein A). Further, they found that growth in C+Y (not to be confused with CY media ATCC MD-2906) had a vastly different transcription profile than those related to infection or competence but was similar to other conditions like transmission indicating that the response was not completely tied to the media (Aprianto et al., 2018). Mining this data set will occur for years to come, likely finding more differences in how the pneumococcus behaves in various environments.
Another example of additives impacting phenotypes is documented by Ferrándiz et al. (2019). In this work, the authors were characterizing stress proteins in nutrient restricted conditions in semisynthetic AGCH (Adenine Glutamine Casein Hydrolysate) medium also known as modified Lacks media (Ottolenghi and Hotchkiss, 1962), in comparison to effects on nutrient rich conditions (Todd-Hewitt broth + yeast extract) (Ferrándiz et al., 2019). They found that when the general stress gene gls24B was knocked out, the mutant was able to grow only in the presence of BSA and growth was concentration dependent. While unable to determine what about the BSA was responsible for the phenotype, the effect was also observed using protease-treated BSA, but not with acid-digested BSA (Ferrándiz et al., 2019). Interestingly, BSA binds fatty acids which still need to be removed and fatty acid metabolism was downregulated in the mutant (Davis and Dubos, 1947; Chen, 1967).
In 2009, Pandya et al. used a combination of microarray and RT-PCR to study the transcriptional profile of a type 4 strain serially cultured on two broths (Pandya et al., 2009). As a control, mRNA from S. pneumoniae grown in Todd-Hewitt yeast extract broth was compared to the same strain cultivated 50-100 passages on blood agar plates (Pandya et al., 2009). They found that selection on blood agar increased the number of differentially expressed genes from 113 genes after 50 passages to 706 genes (or approximately 1/3 of the pneumococcus genome) after 100 passages. Of the genes most differentially expressed, roughly a third of the genes (160) were annotated as hypothetical, 60 genes of unknown function, and 93 virulence genes—including the capsule locus. Consistent with previous reports, genes associated with adhesion and colonization (e.g., neuraminidase and choline binding proteins) were downregulated during blood agar cultivation (Gosink et al., 2000; Brittan et al., 2012). While neuraminidase and choline binding proteins support bacterial colonization and dissemination respectively, neuraminidase cleaves sialic acids in the blood which recruits host complement (Attali et al., 2008; Syed et al., 2019).
These transcriptional changes highlight what occurs when the pneumococcus transitions to other environments in the host such as the blood. Bae et al. cultured D39 on Todd Hewitt agar ± 5% sheep blood and documented a more general shift in protein expression by using 2-D gel electrophoresis and matrix assisted laser desorption ionization-time of flight mass spectrometry (MALDI-TOF) spectra (Bae et al., 2006). One major change they saw with added blood was with metabolism. Lactate oxidase (lctO), which catalyzes the oxidation of lactate and O2 to pyruvate and H2O2, had lower expression (3.5 fold), L-lactate dehydrogenase (ldh), which reversibly converts pyruvate and NADH (nicotinamide adenine dinucleotide with hydrogen) to lactate and NAD+, had higher expression (4.2 fold), and pyruvate oxidase (spxB), which converts pyruvate, phosphate and O2 to acetyl phosphate and H2O2 was observed as a spot, but not increased (Bae et al., 2006). These changes mimic bacterial transition to the anaerobic conditions of the blood, an environment where ldh has been found to be essential (Gaspar et al., 2014). Both spxB (the major contributor of and making H2O2) and lcto are upregulated limited aeration conditions compared to anaerobic growth in BHI (Echlin et al., 2016; Lisher et al., 2017). Interestingly, it is H2O2 made by SpxB and LctO that causes α-hemolysis in blood (McDevitt et al., 2020).
Zinc import mutants grown on zinc restrictive media demonstrate morphological defects as revealed by bright field microscopy and cryo-electron microscopy (Bayle et al., 2011). These mutants were also unable to colonize a mouse model 48 hours after In or IP inoculation, indicating reduced virulence (Bayle et al., 2011). Carbon source can also play a role in how the pneumococcus maintains metal homeostasis. In fact, the addition of hemoglobin has been shown to stimulate pneumococcal usage of host carbohydrates the essentially helping it to adapt to the host environment (Akhter et al., 2020). Lack of iron also affects the pneumococcus, which produces no known siderophores and thus, must scavenge it via hemoglobin, heme, hemin, or take in free iron (uncommon in the host), by hindering virulence and causing dysbiosis in metabolism (Tai et al., 1993; Akhter et al., 2020). Lastly, Afzal et al. demonstrated in D39 that ascorbic acid as the carbon source in M17 led to decrease in intracellular zinc, manganese and iron (Afzal et al., 2015).
Finally, one of the most striking examples of the effects of media on bacterial physiology was illustrated by Hoyer et al. (2018). In this work, an unencapsulated variant of pneumococcus was grown on chemically defined media (RPMI 1640 & supplements) and complex media (Todd-Hewitt) under iron limiting conditions and control. Proteomic analysis was performed comparing gene expression between growth mediums. They found that 43.7% of pneumococcal proteins were uniquely expressed in one of the two mediums. This study highlights how varying a single nutrient impact nearly half of the organisms’ proteins. They also noted that many proteins involved in pathogenesis were downregulated in nutrient-restricted conditions. Among the genes differentially expressed was the iron acquisition gene piuA (SPD_1652) which was highly expressed under CDM vs. rich medium. Morphological differences were also revealed using scanning electron microscopy. Denser DNA was noted by TEM under nutrient restricted conditions, suggesting a decrease in active transcription. Finally, a decrease in ribosomal proteins detected via LC–MS/MS under CDM conditions indicating a slower growth rate.
Suggestions and Conclusions
Throughout this process, the authors encountered numerous reports of papers using “CDM” as their bacterial media. However, as we have seen throughout this process, not all CDMs are the same or created equal. Further, in many cases, primary sources of the media were not cited, but instead, had 3-4 degrees of separation. While we understand that stating “as described in x et al.” can save on space, due to the variability in media, we strongly suggest listing the recipe for the CDM used in the paper in the supplemental text, especially if modifications were made (along with listing why modifications were made) or at the very least, citing the primary source paper containing the recipe in addition to the original method. Further, choice of media is often taken as given — based on training, tradition, or studies read and, in many cases, the reasons behind said choice of media are not given. As such, we encourage for the reasoning behind using a given medium be explained in the text.
As discussed, the media choice for S. pneumoniae dramatically impacts experimental outcomes. The host-pathogen interface is a critical area of pneumococcal research and several studies have developed or used media intended to replicate conditions within the host. Survival in the disparate niches (e.g., nasopharynx and pulmonary epithelia, blood, cerebrospinal fluid) in which S. pneumoniae persist—this is no simple task and a testament to this organism’s adaptation. Based on varying media components, it seems possible that many experimental outcomes may have been and could still be accidentally influenced by decisions regarding media. As we illustrated here, the choice of media matters for far more than organism growth and propagation. We sincerely hope that in choosing pneumococcal and all other bacterial media, that the examples provided above will be considered to enhance future research studies.
Author Contributions
YS-R and MJ conceptualized, wrote, and edited this manuscript. All authors contributed to the article and approved the submitted version.
Funding
NIGMS 1R35128653.
Conflict of Interest
The authors declare that the research was conducted in the absence of any commercial or financial relationships that could be construed as a potential conflict of interest.
Acknowledgments
We thank Joe W. Alvin, Magdalene Y. So and Jorge E. Vidal for critically reading the manuscript.
References
Adams M. H., Roe A. S. (1945). A Partially Defined Medium for Cultivation of Pneumococcus. J. Bacteriol. 49, 401–409. doi: 10.1128/JB.49.4.401-409.1945
Afzal M., Shafeeq S., Kuipers O. P. (2015). Ascorbic acid-dependent gene expression in Streptococcus pneumoniae and the activator function of the transcriptional regulator UlaR2. Front. Microbiol. 6, 72–72. doi: 10.3389/fmicb.2015.00072
Akhter F., Womack E., Vidal J. E., Le Brenton Y., McIver K. S., Pawar S., et al. (2020). Hemoglobin stimulates vigorous growth of Streptococcus pneumoniae and shapes the pathogen’s global transcriptome. Sci. Rep. 10, 15202. doi: 10.1038/s41598-020-71910-1
Aprianto R., Slager J., Holsappel S., Veening J. W. (2018). High-resolution analysis of the pneumococcal transcriptome under a wide range of infection-relevant conditions. Nucleic Acids Res. 46, 9990–10006. doi: 10.1093/nar/gky750
Attali C., Frolet C., Durmort C., Offant J., Vernet T., Di Guilmi A. M. (2008). Streptococcus pneumoniae choline-binding protein E interaction with plasminogen/plasmin stimulates migration across the extracellular matrix. Infect. Immun. 76 (2), 466–476. doi: 10.1128/IAI.01261-07
Avery O. T., Macleod C. M., Mccarty M. (1944). Studies on the Chemical Nature of the Substance Inducing Transformation of Pneumococcal Types : Induction of Transformation by a Desoxyribonucleic Acid Fraction Isolated from Pneumococcus Type Iii. J. Exp. Med. 79, 137–158. doi: 10.1084/jem.79.2.137
Bae S. M., Yeon S. M., Kim T. S., Lee K. J. (2006). The effect of protein expression of Streptococcus pneumoniae by blood. J. Biochem. Mol. Biol. 39, 703–708. doi: 10.5483/BMBRep.2006.39.6.703
Baesman R. K., Strand C. L. (1984). Detection of Streptococcus pneumoniae in lower respiratory tract specimens by anaerobic culture technique. J. Clin. Microbiol. 19, 550–551. doi: 10.1128/JCM.19.4.550-551.1984
Bayle L., Chimalapati S., Schoehn G., Brown J., Vernet T., Durmort C. (2011). Zinc uptake by Streptococcus pneumoniae depends on both AdcA and AdcAII and is essential for normal bacterial morphology and virulence. Mol. Microbiol. 82 (4), 904–916. doi: 10.1111/j.1365-2958.2011.07862.x
Bernheimer A. W., Gillman W., Hottle G. A., Pappenheimer A. M. (1942). An Improved Medium for the Cultivation of Hemolytic Streptococcus. J. Bacteriol. 43, 495–498. doi: 10.1128/JB.43.4.495-498.1942
Bidossi A., Mulas L., Decorosi F., Colomba L., Ricci S., Pozzi G., et al. (2012). A functional genomics approach to establish the complement of carbohydrate transporters in Streptococcus pneumoniae. PLoS One 7, e33320. doi: 10.1371/journal.pone.0033320
Briles E. B., Tomasz A. (1970). Radioautographic evidence for equatorial wall growth in a gram-positive bacterium. Segregation of choline-3H-labeled teichoic acid. J. Cell Biol. 47 (3), 786–790. doi: 10.1083/jcb.47.3.786
Brittan J. L., Buckeridge T. J., Finn A., Kadioglu A., Jenkinson H. F. (2012). Pneumococcal neuraminidase A: an essential upper airway colonization factor for Streptococcus pneumoniae. Mol. Oral. Microbiol. 27, 270–283. doi: 10.1111/j.2041-1014.2012.00658.x
Brown J. S., Gilliland S. M., Holden D. W. (2001). A Streptococcus pneumoniae pathogenicity island encoding an ABC transporter involved in iron uptake and virulence. Mol. Microbiol. 40, 572–585. doi: 10.1046/j.1365-2958.2001.02414.x
Buckwalter C. M., King S. J. (2012). Pneumococcal carbohydrate transport: Food for thought. Trends Microbiol. 20 (11), 517–522. doi: 10.1016/j.tim.2012.08.008
Burghout P., Cron L. E., Gradstedt H., Quintero B., Simonetti E., Bijlsma J. J. E., et al. (2010). Carbonic anhydrase is essential for Streptococcus pneumoniae growth in environmental ambient air. J. Bacteriol. 192, 4054–4062. doi: 10.1128/JB.00151-10
Cansler C. (2013). Where"s the Beef? (Science History Institute). Available at: https://www.sciencehistory.org/distillations/article/where%E2%80%99s-beef (Accessed 2020).
Carvalho S. M., Kuipers O. P., Neves A. R. (2013). Environmental and nutritional factors that affect growth and metabolism of the pneumococcal serotype 2 strain D39 and its nonencapsulated derivative strain R6. PLoS One 8 (3), e58492. doi: 10.1371/journal.pone.0058492
Carvalho S. M., Kloosterman T. G., Manzoor I., Caldas J., Vinga S., Martinussen J., et al. (2018). Interplay Between Capsule Expression and Uracil Metabolism in Streptococcus pneumoniae D39. Front. Microbiol. 9, 321. doi: 10.3389/fmicb.2018.00321
Charland N., Kellens J. T. C., Caya F., Gottschalk M. (1995). Agglutination of Streptococcus suis by sialic acid-binding lectins. J. Clin. Microbiol. 33 (8), 22202221. doi: 10.1128/JCM.33.8.2220-2221.1995
Chen R. F. (1967). Removal of fatty acids from serum albumin by charcoal treatment. J. Biol. Chem. 242, 173–181. doi: 10.1016/S0021-9258(19)81445-X
Clinical and Laboratory Standards Institute (2012). Clinical and Laboratory Standards Methods for Dilution Antimicrobial Susceptibility Test for Bacteria That Grow Aerobically. Approved standard-ninth edition (Clinical and Laboratory Standards Institute).
da Gloria Carvalho M., Pimenta F. C., Jackson D., Roundtree A., Ahmad Y., Millar E. V., et al. (2010). Revisiting pneumococcal carriage by use of broth enrichment and PCR techniques for enhanced detection of carriage and serotypes. J. Clin. Microbiol. 48, 1611–1618. doi: 10.1128/JCM.02243-09
Davis B. D., Dubos R. J. (1947). The Binding of Fatty Acids by Serum Albumin, a Protective Growth Factor in Bacteriological Media. J. Exp. Med. 86, 215–228. doi: 10.1084/jem.86.3.215
Donati C., Hiller N. L., Tettelin H., Muzzi A., Croucher N. J., Angiuoli S. V., et al. (2010). Structure and dynamics of the pan-genome of Streptococcus pneumoniae and closely related species. Genome Biol. 11, R107–R107. doi: 10.1186/gb-2010-11-10-r107
Echlin H., Frank M. W., Iverson A., Chang T. C., Johnson M. D., Rock C. O., et al. (2016). Pyruvate oxidase as a critical link between metabolism and capsule biosynthesis in Streptococcus pneumoniae. PLoS Pathog. 12, e1005951. doi: 10.1371/journal.ppat.1005951
Eijkelkamp B. A., Morey J. R., Ween M. P., Ong C.-L. Y., Mcewan A. G., Paton J. C., et al. (2014). Extracellular Zinc Competitively Inhibits Manganese Uptake and Compromises Oxidative Stress Management in Streptococcus pneumoniae. PLoS One 9, e89427–e89427. doi: 10.1371/journal.pone.0089427
Ellner P. D., Stoessel C. J. (1966). The role of temperature and anticoagulant on the in vitro survival of bacterial in blood. J. Infect. Dis. 116, 238–242. doi: 10.1093/infdis/116.2.238
Ersoy S. C., Heithoff D. M., Barnes L. T., Tripp G. K., House J. K., Marth J. D., et al. (2017). Correcting a Fundamental Flaw in the Paradigm for Antimicrobial Susceptibility Testing. EBioMedicine 20, 173–181. doi: 10.1016/j.ebiom.2017.05.026
Erttmann S. F., Gekara N. O. (2019). Hydrogen peroxide release by bacteria suppresses inflammasome-dependent innate immunity. Nat. Commun. 10, 3493. doi: 10.1038/s41467-019-11169-x
Fennel E. A., Fisher M. B. (1919). Adjustment of reaction of culture mediums. J. Infect. Dis. 25, 444–451. doi: 10.1093/infdis/25.6.444
Ferone R., Bushby S. R., Burchall J. J., Moore W. D., Smith D. (1975). Identification of Harper-Cawston factor as thymidine phosphorylase and removal from media of substances interfering with susceptibility testing to sulfonamides and diaminopyrimidines. Antimicrob. Agents Chemother. 7, 91–98. doi: 10.1128/AAC.7.1.91
Ferrándiz M. J., Cercenado M. I., Domenech M., Tirado-Vélez J. M., Escolano-Martínez M. S., Yuste J., et al. (2019). An Uncharacterized Member of the Gls24 Protein Superfamily Is a Putative Sensor of Essential Amino Acid Availability in Streptococcus pneumoniae. Microb. Ecol. 77, 471–487. doi: 10.1007/s00248-018-1218-9
Gaspar P., Al-Bayati F. A., Andrew P. W., Neves A. R., Yesilkaya H. (2014). Lactate dehydrogenase is the key enzyme for pneumococcal pyruvate metabolism and pneumococcal survival in blood. Infect. Immun. 82, 5099–5109. doi: 10.1128/IAI.02005-14
Gladstone G. P., Fildes P., Richardson G. M. (1935). Carbon Dioxide as an Essential Factor in the Growth of Bacteria. Br. J. Exp. Pathol. 16, 335–348.
Gosink K. K., Mann E. R., Guglielmo C., Tuomanen E. I., Masure H. R. (2000). Role of novel choline binding proteins in virulence of Streptococcus pneumoniae. Infect. Immun. 68, 5690–5695. doi: 10.1128/IAI.68.10.5690-5695.2000
Grant C. L., Pramer D. (1962). Minor element composition of yeast extract. J. Bacteriol. 84, 869–870. doi: 10.1128/JB.84.4.869-870.1962
Gullett J. M., Cuypers M. G., Frank M. W., White S. W., Rock C. O. (2019). A fatty acid-binding protein of Streptococcus pneumoniae facilitates the acquisition of host polyunsaturated fatty acids. J. Biol. Chem. 294 (44), 16416–16428. doi: 10.1074/jbc.RA119.010659
Harper G. J., Cawston W. C. (1945). The in-vitro determination of the sulphonamide sensitivity of bacteria. J. Pathol. Bacteriol. 57, 59–66. doi: 10.1002/path.1700570109
Hathaway L. J., Brugger S. D., Morand B., Bangert M., Rotzetter J. U., Hauser C., et al. (2012). Capsule type of Streptococcus pneumoniae determines growth phenotype. PLoS Pathog. 8, e1002574. doi: 10.1371/journal.ppat.1002574
Hewitt L. F. (1932). Bacterial metabolism: Glucose breakdown by pneumococcus variants and the effect of phosphate thereon. Biochem. J. 26, 464–471. doi: 10.1042/bj0260464
Hoeprich P. D. (1955). C14 Labelling of Diplococcus pneumoniae. J. Bacteriol. 69, 682–688. doi: 10.1128/JB.69.6.682-688.1955
Howden R. (1976). Use of anaerobic culture for the improved isolation of Streptococcus pneumoniae. J. Clin. Pathol. 29, 50–53. doi: 10.1136/jcp.29.1.50
Hoyer J., Bartel J., Gomez-Mejia A., Rohde M., Hirschfeld C., Hess N., et al. (2018). Proteomic response of Streptococcus pneumoniae to iron limitation. Int. J. Med. Microbiol. 308, 713–721. doi: 10.1016/j.ijmm.2018.02.001
Johnson M. D. L., Kehl-Fie T. E., Rosch J. W. (2015). Copper intoxication inhibits aerobic nucleotide synthesis in Streptococcus pneumoniae. Metallomics 7, 786–794. doi: 10.1039/C5MT00011D
Kato S., Kurata T., Fujimaki M. (1971). Thermal Degradation of Aromatic Amino Acids. Agric. Biol. Chem. 35, 2106–2112. doi: 10.1080/00021369.1971.10860191
Kempner W., Schlayer C. (1942). Effect of CO(2) on the Growth Rate of the Pneumococcus. J. Bacteriol. 43, 387–396. doi: 10.1128/JB.43.3.387-396.1942
Kloosterman T. G., Bijlsma J., Kok J., Kuipers O. P. (2006). To have neighbour’s fare: extending the molecular toolbox for Streptococcus pneumoniae. Microbiol. (Reading England) 152 (Pt 2), 351–359. doi: 10.1099/mic.0.28521-0
Klotz S., Kuenz A., Prüße U. (2017). Nutritional requirements and the impact of yeast extract on the d-lactic acid production by Sporolactobacillus inulinus. Green Chem. 19, 4633–4641. doi: 10.1039/C7GC01796K
Lacks S., Hotchkiss R. D. (1960). A study of the genetic material determining an enzyme activity in pneumococcus. Biochim. Et Biophys. Acta 39, 508–518. doi: 10.1016/0006-3002(60)90205-5
Lacks S. (1966). Integration efficiency and genetic recombination in pneumococcal transformation. Genetics 53, 207–235. doi: 10.1093/genetics/53.1.207
Lisher J. P., Tsui H. T., Ramos-Montanez S., Hentchel K. L., Martin J. E., Trinidad J. C., et al. (2017). Biological and chemical adaptation to endogenous hydrogen peroxide production in Streptococcus pneumoniae D39. mSphere 2, e291–e216. doi: 10.1128/mSphere.00291-16
Majewska E., Kasielski M., Luczynski R., Bartosz G., Bialasiewicz P., Nowak D. (2004). Elevated exhalation of hydrogen peroxide and thiobarbituric acid reactive substances in patients with community acquired pneumonia. Respir. Med. 98 (7), 669–676. doi: 10.1016/j.rmed.2003.08.015
Marion C., Burnaugh A. M., Woodiga S. A., King S. J. (2011). Sialic acid transport contributes to pneumococcal colonization. Infect. Immun. 79, 1262–1269. doi: 10.1128/IAI.00832-10
Mason P. W., Carbone D. P., Cushman R. A., Waggoner A. S. (1981). The importance of inorganic phosphate in regulation of energy metabolism of Streptococcus lactis. J. Biol. Chem. 256 (4), 1861–1866. doi: 10.1016/S0021-9258(19)69886-8
Matsumoto Y., Miyake K., Ozawa K., Baba Y., Kusube T. (2019). Bicarbonate and unsaturated fatty acids enhance capsular polysaccharide synthesis gene expression in oral streptococci, Streptococcus anginosus. J. Biosci. Bioeng. 128 (5), 511–517. doi: 10.1016/j.jbiosc.2019.04.010
McDevitt E., Faidad K., Scasny A., Thompson C. D., Eichenbaum Z., McDaniel L. S., et al. (2020). Hydrogen Peroxide Production by Streptococcus pneumoniae Results in Alpha-hemolysis by Oxidation of Oxy-hemoglobin to Met-hemoglobin. mSphere 5, e01117–e01120. doi: 10.1128/mSphere.01117-20
Mosser J. L., Tomasz A. (1970). Choline-containing teichoic acid as a structural component of pneumococcal cell wall and its role in sensitivity to lysis by an autolytic enzyme. J. Biol. Chem. 245 (2), 287–298. doi: 10.1016/S0021-9258(18)63393-9
Nagaoka K., Yamashita Y., Kimura H., Suzuki M., Konno S., Fukumoto T., et al. (2019). Effects of Anaerobic Culturing on Pathogenicity and Virulence-Related Gene Expression in Pneumococcal Pneumonia. J. Infect. Dis. 219 (10), 1545–1553. doi: 10.1093/infdis/jiy718
Nelson A. L., Roche A. M., Gould J. M., Chim K., Ratner A. J., Weiser J. N. (2007). Capsule Enhances Pneumococcal Colonization by Limiting Mucus-Mediated Clearance. Infect. Immunity 75 (1), 83–90. doi: 10.1128/IAI.01475-06
Nolan R. A., Nolan W. G. (1972). Elemental Analysis of Vitamin-Free Casamino Acids. Appl. Microbiol. 24 (2), 290–291. doi: 10.1128/AM.24.2.290-291.1972
Ottolenghi E., Hotchkiss R. D. (1962). Release of genetic transforming agent from pneumococcal cultures during growth and disintegration. J. Exp. Med. 116 (4), 491–519. doi: 10.1084/jem.116.4.491
Pandya U., Sinha M., Luxon B. A., Watson D. A., Niesel D. W. (2009). Global transcription profiling and virulence potential of Streptococcus pneumoniae after serial passage. Gene 443, 22–31. doi: 10.1016/j.gene.2009.04.014
Pasteur L. (1881). Bulletin de l"Académie nationale de médecine (Paris: Masson and Académie Nationale de Médecine and Issy-les-Moulineaux: Elsevier Masson).
Rajput A., Poudel S., Tsunemoto H., Meehan M., Szubin R., Olson C. A., et al. (2019). Profiling the effect of nafcillin on HA-MRSA D712 using bacteriological and physiological media. Sci. Data 6, 322. doi: 10.1038/s41597-019-0331-z
Rane L., Subbarow Y. (1940). Nutritional Requirements of the Pneumococcus: I. Growth Factors for Types I, II, V, VII, VIII. J. Bacteriol. 40, 695–704. doi: 10.1128/JB.40.5.695-704.1940
Ruiz V., Rodríguez-Cerrato V., Huelves L., Del Prado G., Naves P., Ponte C., et al. (2011). Adherence of Streptococcus pneumoniae to polystyrene plates and epithelial cells and the antiadhesive potential of albumin and xylitol. Pediatr. Res. 69 (1), 23–27. doi: 10.1203/PDR.0b013e3181fed2b0
Salazar M. J., Machado H., Dillon N. A., Tsunemoto H., Szubin R., Dahesh S., et al. (2020). Genetic Determinants Enabling Medium-Dependent Adaptation to Nafcillin in Methicillin-Resistant Staphylococcus aureus. mSystems 5. doi: 10.1128/mSystems.00828-19
Schulz C., Gierok P., Petruschka L., Lalk M., Mader U., Hammerschmidt S. (2014). Regulation of the arginine deiminase system by ArgR2 interferes with arginine metabolism and fitness of Streptococcus pneumoniae. mBio 5. doi: 10.1128/mBio.01858-14
Sicard A. M. (1964). A New Synthetic Medium for Diplococcus Pneumoniae, and Its Use for the Study of Reciprocal Transformations at the Amia Locus. Genetics 50, 31–44. doi: 10.1093/genetics/50.1.31
Siegel S. J., Roche A. M., Weiser J. N. (2014). Influenza promotes pneumococcal growth during co-infection by providing host sialylated substrates as a nutrient source. Cell Host Microbe 16 (1), 55–67. doi: 10.1016/j.chom.2014.06.005
Smith K. S., Ferry J. G. (2000). Prokaryotic carbonic anhydrases. FEMS Microbiol. Rev. 24, 335–366. doi: 10.1111/j.1574-6976.2000.tb00546.x
Sorg R. A., Lin L., Van Doorn G. S., Sorg M., Olson J., Nizet V., et al. (2016). Collective Resistance in Microbial Communities by Intracellular Antibiotic Deactivation. PLoS Biol. 14, e2000631. doi: 10.1371/journal.pbio.2000631
Stedman T. L. (1900). “An International Encyclopedia of Modern Medical Science,” in Volume XIX Malaria and Microorganisms (William Wood and Company).
Sternberg G. (1881). “Special Report to the National Board of Health–Experimental Investigation Relating to the Etiology of the Malarial Fevers” In: Annual report National Board of Health. p. 65–91. Available at: https://hdl.handle.net/2027/iau.31858045161712
Sternberg G. M. (1896). A Text-book of Bacteriology - George Miller Sternberg - Google Books (William Wood and Company).
Stokes J. L., Gunness M., Foster J. W. (1944). Vitamin Content of Ingredients of Microbiological Culture Media. J. Bacteriol. 47, 293–299. doi: 10.1128/JB.47.3.293-299.1944
Suárez N., Texeira E. (2019). Optimal Conditions for Streptococcus pneumoniae Culture: In Solid and Liquid Media. Methods Mol. Biol. 1968, 3–10. doi: 10.1007/978-1-4939-9199-0_1
Syed S., Hakala P., Singh A. K., Lapatto H. A. K., King S. J., Meri S., et al. (2019). Role of pneumococcal nana neuraminidase activity in peripheral blood. Front. Cell Infect. Microbiol. 9, 218. doi: 10.3389/fcimb.2019.00218
Tai S. S., Lee C. J., Winter R. E. (1993). Hemin utilization is related to virulence of Streptococcus pneumoniae. Infect. Immun. 61 (12), 5401–5405. doi: 10.1128/IAI.61.12.5401-5405.1993
Talamon C. H., Broca A. A. D. T., Proust R. A. D. T., Feindel E. A. D. T., Texte S. (1883). Bulletins de la Société anatomique de Paris (Paris: V. Masson and G. Steinheil).
Terzaghi B. E., Sandine A. W. E. (1975). Improved Medium for Lactic Streptococci and Their Bacteriophages. Appl. Microbiol. 29 (6), 807–813. doi: 10.1128/AM.29.6.807-813.1975
Tettelin H., Nelson K. E., Paulsen I. T., Eisen J. A., Read T. D., Peterson S., et al. (2001). Complete genome sequence of a virulent isolate of Streptococcus pneumoniae. Science 293, 498–506. doi: 10.1126/science.1061217
Tikhomirova T. S., Ievlev R. S., Suvorina M., Bobyleva L. G., Vikhlyantsev I. M., Surin A. K., et al. (2018). Search for functionally significant motifs and amino acid residues of actin. Mol. Biol. 52, 118–135. doi: 10.1134/S0026893318010193
Todd E. W., Hewitt L. F. (1932). A new culture medium for the production of antigenic streptococcal hæmolysin. J. Pathol. 35, 973–974. doi: 10.1002/path.1700350614
Tomasz A., Zanati E., Ziegler R. (1971). DNA uptake during genetic transformation and the growing zone of the cell envelope. Proc. Natl. Acad. Sci. U. S. A. 68 (8), 1848–1852. doi: 10.1073/pnas.68.8.1848
Tomasz A. (1968). Biological consequences of the replacement of choline by ethanolamine in the cell wall of pneumococcus: chain formation, loss of transformability, and loss of autolysis. Proc. Natl. Acad. Sci. 59, 86–93. doi: 10.1073/pnas.59.1.86
Tothpal A., Desobry K., Joshi S. S., Wyllie A. L., Weinberger D. M. (2019). Variation of growth characteristics of pneumococcus with environmental conditions. BMC Microbiol. 19, 304. doi: 10.1186/s12866-019-1671-8
Trappetti C., Potter A. J., Paton A. W., Oggioni M. R., Paton J. C. (2011). LuxS mediates iron-dependent biofilm formation, competence, and fratricide in Streptococcus pneumoniae. Infect. Immun. 79, 4550–4558. doi: 10.1128/IAI.05644-11
Troxler L. J., Werren J. P., Schaffner T. O., Mostacci N., Vermathen P., Vermathen M., et al. (2019). Carbon source regulates polysaccharide capsule biosynthesis in Streptococcus pneumoniae. J. Biol. Chem. 294, 17224–17238. doi: 10.1074/jbc.RA119.010764
Updyke E. L., Nickle, Margaret I. (1953). Comparison of dehydrated media with fresh Todd-Hewitt broth for typing group A beta hemolytic streptococci. Appl. Microbiol. 2 (2), 117–118.
van Beek L. F., Surmann K., van den Berg van Saparoea H. B., Houben D., Jong W., Hentschker C., et al. (2020). Exploring metal availability in the natural niche of Streptococcus pneumoniae to discover potential vaccine antigens. Virulence 11 (1), 1310–1328. doi: 10.1080/21505594.2020.1825908
Van De Rijn I., Kessler R. E. (1980). Growth Characteristics of Group A Streptococci in a New Chemically Defined Medium. Infect. Immun. 27 (2), 444–448. doi: 10.1128/IAI.27.2.444-448.1980
Vera H. D. (1950). Relation of peptones and other culture media ingredients to the accuracy of fermentation tests. Am. J. Public Health Nations Health 40, 1267–1272. doi: 10.2105/AJPH.40.10.1267
Weimer K. E., Juneau R. A., Murrah K. A., Pang B., Armbruster C. E., Richardson S. H., et al. (2011). Divergent mechanisms for passive pneumococcal resistance to beta-lactam antibiotics in the presence of Haemophilus influenzae. J. Infect. Dis. 203, 549–555. doi: 10.1093/infdis/jiq087
Welch W. H. (1892). RE: Bulletin of the Johns Hopkins Hospital. v.3 1892. - Full View | HathiTrust Digital Library | HathiTrust Digital Library (Baltimore).
Wu S. C., Trask L. M., Phee R. E. (1980). Comparison of media and culture techniques for detection of Streptococcus pneumoniae in respiratory secretions. J. Clin. Microbiol. 12, 772–775. doi: 10.1128/JCM.12.6.772-775.1980
Yesilkaya H., Manco S., Kadioglu A., Terra V. S., Andrew P. W. (2008). The ability to utilize mucin affects the regulation of virulence gene expression in Streptococcus pneumoniae. FEMS Microbiol. Lett. 278, 231–235. doi: 10.1111/j.1574-6968.2007.01003.x
Keywords: Streptococcus pneumoniae (pneumococcus), media, metabolism, growth, experimental rigor
Citation: Sanchez-Rosario Y and Johnson MDL (2021) Media Matters, Examining Historical and Modern Streptococcus pneumoniae Growth Media and the Experiments They Affect. Front. Cell. Infect. Microbiol. 11:613623. doi: 10.3389/fcimb.2021.613623
Received: 02 October 2020; Accepted: 02 March 2021;
Published: 23 March 2021.
Edited by:
Victor Nizet, University of California, San Diego, United StatesReviewed by:
Aruni Wilson, Loma Linda University, United StatesRamesh Vemulapalli, Texas A&M University, United States
Copyright © 2021 Sanchez-Rosario and Johnson. This is an open-access article distributed under the terms of the Creative Commons Attribution License (CC BY). The use, distribution or reproduction in other forums is permitted, provided the original author(s) and the copyright owner(s) are credited and that the original publication in this journal is cited, in accordance with accepted academic practice. No use, distribution or reproduction is permitted which does not comply with these terms.
*Correspondence: Michael D. L. Johnson, bWRsam9obnNvbkBhcml6b25hLmVkdQ==