- 1School of Biotechnology and Biomolecular Sciences, University of New South Wales, Sydney, NSW, Australia
- 2Bioanalytical Mass Spectrometry Facility, University of New South Wales, Sydney, NSW, Australia
The Bordetella genus is divided into two groups: classical and non-classical. Bordetella pertussis, Bordetella bronchiseptica and Bordetella parapertussis are known as classical bordetellae, a group of important human pathogens causing whooping cough or whooping cough-like disease and hypothesized to have evolved from environmental non-classical bordetellae. Bordetella infections have increased globally driving the need to better understand these pathogens for the development of new treatments and vaccines. One unexplored component in Bordetella is the role of serine, threonine and tyrosine phosphorylation. Therefore, this study characterized the phosphoproteome of classical bordetellae and examined its potential role in Bordetella biology and virulence. Applying strict identification of localization criteria, this study identified 70 unique phosphorylated proteins in the classical bordetellae group with a high degree of conservation. Phosphorylation was a key regulator of Bordetella metabolism with proteins involved in gluconeogenesis, TCA cycle, amino acid and nucleotide synthesis significantly enriched. Three key virulence pathways were also phosphorylated including type III secretion system, alcaligin synthesis and the BvgAS master transcriptional regulatory system for virulence genes in Bordetella. Seven new phosphosites were identified in BvgA with 6 located in the DNA binding domain. Of the 7, 4 were not present in non-classical bordetellae. This suggests that serine/threonine phosphorylation may play an important role in stabilizing/destabilizing BvgA binding to DNA for fine-tuning of virulence gene expression and that BvgA phosphorylation may be an important factor separating classical from non-classical bordetellae. This study provides the first insight into the phosphoproteome of classical Bordetella species and the role that Ser/Thr/Tyr phosphorylation may play in Bordetella biology and virulence.
Introduction
The genus Bordetella contains both environmental Gram-negative bacterial species and human pathogens (Gross et al., 2008). Bordetella pertussis together with Bordetella bronchiseptica and Bordetella parapertussis, make up the classical bordetellae group which causes respiratory disease in mammals while non-classical bordetellae species include environmental (Bordetella petrii), avian (Bordetella avium and Bordetella hinzii) and opportunistic human pathogens (Bordetella holmseii, Bordetella trematum and Bordetella ansorpii). The pathogenic classical bordetellae group is hypothesized to have evolved out of free-living environmental non-classical Bordetella species (Gross et al., 2008).
Of the three classical species, B. bronchiseptica is the ancestral species to B. pertussis and B. parapertussis (Diavatopoulos et al., 2005). It can survive in the environment and infects a broad range of mammals including dogs, pigs, cats, rabbits and humans (Mattoo and Cherry, 2005). B. pertussis and B. parapertussis are both host restricted pathogens. B. pertussis is responsible for whooping cough (pertussis), a severe, human respiratory disease while B. parapertussis contains a human specific lineage which causes pertussis-like disease and an ovine specific lineage which causes pneumonia in sheep (Diavatopoulos et al., 2005).
Genomic studies revealed that B. pertussis and B. parapertussis both evolved independently from B. bronchiseptica and this was associated with large-scale gene-loss (Parkhill et al., 2003). Comparison of the core genome estimates that 51% of the genes are shared by all classical Bordetella strains with significant differences found in virulence and surface-protein encoding genes (Park et al., 2012). There are limited proteomic studies that have compared the proteomes of all three classical Bordetella species. A recent study by Oviedo et al. (2019) investigated proteomic differences between B. pertussis and B. parapertussis and identified key expression differences in stress response proteins which may lead to differences in persistence within the host between the two Bordetella pathogens.
Despite continued vaccinations with high coverage, whooping cough has re-emerged worldwide in the 21st century with an estimated ~16 million cases every year (World Health Organization, 2010; Xu et al., 2019). This has spurred the need to better understand the biology and virulence behind the causal organism in order to develop more effective vaccines and treatments. One important aspect of pertussis biology is protein phosphorylation, a key post-translational modification which affects a wide range of cellular functions including cell division, metabolism, stress response and virulence (Cummings et al., 2006). Protein phosphorylation in B. pertussis has been studied in relation to histidine and aspartate phosphorylation in two-component regulatory systems such as BvgAS (Cummings et al., 2006; Moon et al., 2017), RisAS (Coutte et al., 2016) and the recently described PlrSR (Bone et al., 2017). Two-component systems contain a sensor kinase (e.g. BvgS) and a response regulator (e.g. BvgA). Under specific environmental cues, a histidine residue on the sensor kinase is autophosphorylated and then passed onto an aspartate residue (Asp54) on the response regulator through the histidine-aspartate phosphorylation pathway. This response regulator then binds to DNA and regulates the expression of a number of genes (Boulanger et al., 2013).
In addition to histidine and aspartate phosphorylation in two-component systems, serine, threonine and tyrosine (Ser/Thr/Tyr) phosphorylation, once thought to be unique to eukaryotes, have also been found to occur in other bacterial species (Dworkin, 2015). Bacterial Ser/Thr/Tyr kinases can be divided into two groups: Hanks-type Ser/Thr kinases [or eukaryotic-like serine and threonine kinase (eSTK)] which share a common origin and homology to Ser/Thr kinases found in eukaryotes (Stancik et al., 2018), and bacterial tyrosine kinases (BY-kinases) which share little homology to eukaryotic tyrosine kinases and are typified by Walker’s A and B motifs (Bechet et al., 2009). Tyrosine phosphorylation has been implicated in bacterial virulence with pathogenic bacteria such as Klebsiella pneumonaie having a high proportion of tyrosine phosphorylation (25.8%) (Lin M.-H. et al., 2009). Ser/Thr phosphorylation has also been implicated in virulence but are more associated with metabolism and cell division (Pereira et al., 2011). Despite the importance of phosphorylation, the Ser/Thr/Tyr phosphoproteome has not been characterized in Bordetella species. Here, we aimed to explore the Ser/Thr/Tyr phosphoproteome of classical Bordetella species and examine its role in Bordetella biology and virulence.
Materials and Methods
Experimental Design and Statistical Rationale
For each Bordetella strain used, 3 biological replicates were prepared as described below. For each biological replicate, phosphopeptides were sequentially enriched with TiO2 followed by IMAC as described below and one sampling from each fractionation was performed for LC-MS/MS. Statistical differences in the number of phosphorylated serine, threonine and tyrosine residues between the three Bordetella strains were determined using Fisher’s exact test in Prism Graphpad (v8.0) with p values < 0.05 assigned as statistically significant. Statistical assessment of essential gene enrichment and enrichment of functional categories between the proteome and phosphoproteome was also determined using Fisher’s exact test and Benjamini-Hochberg adjustment for multiple hypothesis testing correction in Rstudio (v 0.99.903).
Bacterial Culture and Protein Extraction
Bordetella strains (L1423 (B. pertussis), RB50 (B. bronchiseptica) and 12822 (B. parapertussis)) were inoculated into 100 ml Thalen–IJessel (THIJS) media as previously described in Luu et al. (2018b) and incubated for 24 h to reach mid-exponential phase. After 24 h, the cultures were centrifuged, and the resulting cell pellet was washed three times with 25 mM NaHCO3. The cell pellet was then resuspended in disruption buffer (50 mM Tris-HCl, 2 mM EDTA and 0.4 mM PMSF) with phosphatase inhibitors (5 mM of each: NaF, β-glycerophosphate, Na Orthovanadate and Na pyrophosphate) and sonicated. The lysate was then centrifuged to remove cellular debris and concentrated using a 3 kDa ultra centrifugal unit (Amicon).
Protein Digestion and Sequential Enrichment of Phosphopeptides
For digestion, 500 µg of proteins were trypsin digested as described in Luu et al. (2017) and cleaned up with an Oasis HLB 1cc vac cartridge (Waters). After cleanup, phosphopeptides were sequentially enriched for monophosphorylated peptides followed by multi-phosphorylated peptides using TiO2 and IMAC, respectively (Figure 1) (Thingholm et al., 2008). First, TiO2 enrichment was performed using Titansphere Phos-TiO Spin Tip kit (3 mg/200 µl) (GL Sciences) according to manufacturer’s instructions. Any uncaptured phosphopeptides in the flow through were cleaned up with an Oasis HLB 1cc vac cartridge and further enriched in a second IMAC fraction using the high-select Fe-NTA Phosphopeptide enrichment kit (ThermoFisher) according to manufacturer’s instructions.
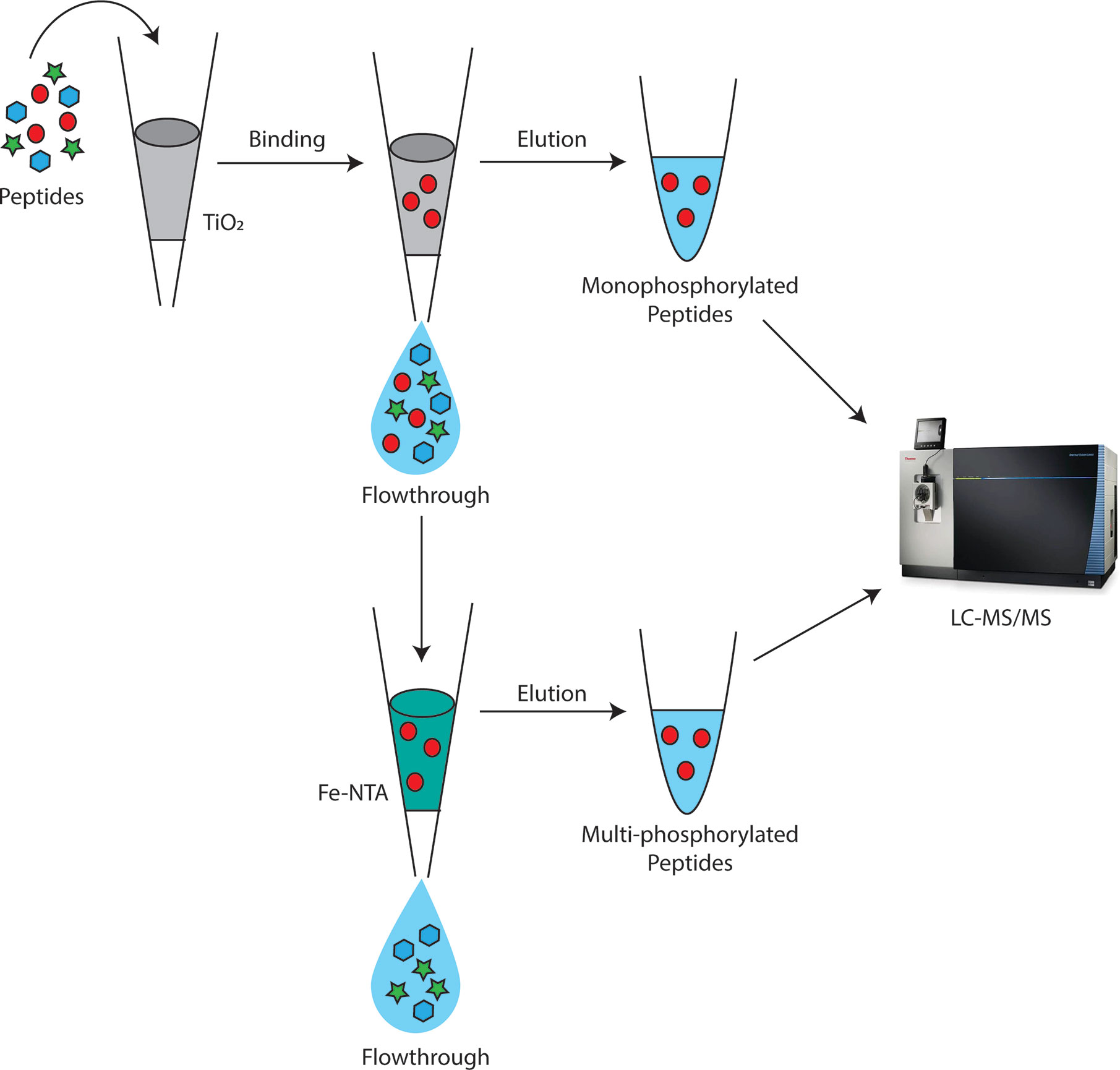
Figure 1 Depiction of workflow for sequential enrichment of Bordetella mono and multi-phosphorylated peptides (red circles). Monophosphorylated peptides were first enriched using TiO2 beads. The flowthrough from TiO2 was then applied to Fe-NTA columns to further separate multi-phosphorylated peptides from non-phosphorylated peptides with IMAC. The elution from TiO2 and IMAC were then analyzed using LC-MS/MS on the Fusion Lumos.
LC-MS/MS
TiO2 and Fe-NTA phosphopeptide enriched samples were each resuspended in 10 µl of 1% formic acid, 0.05% heptafluorobutyric acid and 2% acetonitrile. The samples (2.5 - 5 µl) were then loaded onto the Fusion Lumos (ThermoFisher) connected to an UltiMate nanoRSLC UPLC and autosampler system (Dionex).
The peptides were initially concentrated and desalted with H2O:CH3CN (98:2, 0.2% TFA) at 15 µl/min on a micro C18 precolumn (300 µm x 5 mm, Dionex). After a 4 min wash, the micro C18 precolumn was switched (Valco 10 port UPLC valve, Valco) into line to a fritless nano column (75µ x ~15cm), which contained C18AQ media (1.9µ, 120 Å Dr Maisch). Peptides were then separated on a linear gradient of H2O:CH3CN (98:2, 0.1% formic acid) to H2O:CH3CN (64:36, 0.1% formic acid) at 0.2 µl/min over 30 min. Positive ions were generated with electrospray ionization at 2000V. Data dependent acquisition (DDA) was performed with survey scan from m/z of 350 to 1750, resolution of 120,000 at m/z 200, accumulation target value of 400,000 ions and lockmass enabled (m/z 445.12003). A top-speed approach with a cycle time of 2s was used for data-dependent tandem MS analysis. Ions were fragmented by higher-energy collisional dissociation (HCD) with intensity threshold at 25,000. A mass tolerance of 10 ppm and dynamic exclusion of 20s was set.
Phosphoproteome Analysis
Raw data files were processed via Mascot Daemon (v2.5.1) for peak picking and identification with Oxidation (M), Carbamidomethyl (C), Phospho (ST) and Phospho (Y) set as variable modifications. The remaining search parameters were enzyme: Trypsin, max missed cleavage: 3, mass values: monoisotopic, peptide mass tolerance: 4.0 ppm, MS/MS tolerance 0.4 Da and instrument: ESI-TRAP. For B. pertussis, a search database consisting of B. pertussis strains: Tohama I (NC_002929.2, 3426 protein sequences), CS (NC_017223.1, 3456 protein sequences), B1917 (CP009751.1, 3458 protein sequences) and B1920 (CP009752.1, 3461 protein sequences) from Genbank were used, while for B. bronchiseptica and B. parapertussis searches, strains RB50 (NC_002927.3, 4994 protein sequences) and 12822 (NC_002928.3, 4129 protein sequences) were used.
The resulting Mascot files were loaded into Scaffold (v4.11.0) for verification using the ProteinProphet algorithm (Searle, 2010) with protein probability: 99%, peptide probability: 95% and minimum number of peptides: 1. The A-score algorithm (Vincent-Maloney et al., 2011) in Scaffold PTM (v3.3.0) was used to identify and localize phosphosites with the minimum localization confidence set at 95%. All PSM (peptide-spectral matches) for phosphorylation were then manually checked on Scaffold to ensure a prominent b and/or y ion series was present. Each spectrum is available for interpretation using Scaffold-Viewer (see Data availability section). In Supplementary Tables 1–3, the charge, m/z, MOWSE score for the highest confident PSM is shown. These strict criteria filtered out and eliminated all but the most high quality identification for subsequent analysis.
MPSite (Hasan et al., 2019) and NetPhosBac (v1.0) (Miller et al., 2009) were used to analyze whether serine/threonine phosphosites identified in this study were also bioinformatically predicted to be phosphorylated. Tyrosine prediction was not performed as no bacterial tyrosine phosphorylation prediction tool was available. Bordetella phosphosites were also searched against the database of phosphorylation sites in prokaryotes (dbPSP v2.0) (Shi et al., 2020) to identify homologous phosphorylation sites reported in other bacteria.
Protein cellular localization was predicted using PSORTb (v3.0) (Nancy et al., 2010) while functional categories was assigned according to Bart et al. (2010) and Parkhill et al. (2003). Functional enrichment network analysis was performed using STRING (v11.0) (Szklarczyk et al., 2019) for B. pertussis and B. bronchiseptica. For B. parapertussis, functional category enrichment was not performed as B. parapertussis was not available in the STRING (v11.0) database.
Results
The Phosphoproteome of Classical Bordetella
We compared the Ser/Thr/Tyr phosphoproteome of the three classical Bordetella species in this study. In B. pertussis, the largest number of unique phosphosites was identified with 54 sites (Supplementary Table 1). These phosphosites belonged to 53 non-redundant phosphopeptides and 45 proteins (1.3% of total proteins in the genome) (Table 1). Of the 54 phosphosites, 72% were located on serine residues (pSer), 17% were on threonine (pThr) and 11% were on tyrosine (pTyr). In B. parapertussis, we identified 50 phosphosites from 50 phosphopeptides and 42 proteins (1% of total proteins in the genome) with the distribution being 80% pSer, 12% pThr and 8% pTyr (Supplementary Table 2). In B. bronchiseptica, 29 phosphosites were identified belonging to 28 phosphopeptides and 23 proteins (0.5% of total proteins in the genome) (Supplementary Table 3). The distribution of phosphosites was 69% pSer, 21% pThr and 10% pTyr. The genome size of B. bronchiseptica is ~30% and ~17% larger than B. pertussis and B. parapertussis respectively, however the number of phosphoproteins identified did not reflect this (Parkhill et al., 2003). Additionally, there were no significant differences in the proportion of pSer/pThr/pTyr sites between the three classical bordetellae species.
All but one phosphorylated protein (BvgA) identified had 1 or 2 phosphosites. Most peptides were mono-phosphorylated with multi-phosphorylated peptides in classical Bordetella uncommon. There were only two phosphopeptides detected that were doubly phosphorylated (AlcA, alcaligin biosynthesis protein and BP3319, choloylglycine hydrolase). The maximum number of phosphorylated sites identified within a single protein in B. pertussis, B. parapertussis and B. bronchiseptica was 5 with BvgA, all of which were located on different mono-phosphorylated peptides.
Of the 120 Ser/Thr phosphosites identified in this study, 67 (56%) were predicted to be phosphorylated by MPSite (Hasan et al., 2019) and/or NetPhosBac (Miller et al., 2009) (Supplementary Tables 1–3). Of the 133 total phosphosites identified, 31 homologous phosphosites were identified in other bacteria using dbPSP (Shi et al., 2020), including 4 Tyr sites and 2 Ser/Thr sites not predicted by MPSite and NetPhosBac. Therefore, 55% of phosphosites reported in this study were predicted to be phosphorylated or previously identified as phosphosites in other species.
Common Phosphorylated Proteins in Classical Bordetella
In total, 70 phosphorylated proteins were detected across the 3 species. Of the 70 phosphorylated proteins, 29 (41%) were detected to be phosphorylated in two or more classical Bordetella species and 12 (17%) were phosphorylated in all three species (Figure 2). Of the 12 commonly phosphorylated proteins, 13 phosphopeptides and phosphosites were also shared (Supplementary Table 4). Common phosphorylated proteins between the classical Bordetella species included 7 metabolism-related proteins: PpsA, SucC, SucD and EftB which are involved in gluconeogenesis/phosphotransferase system for sugar uptake, the TCA cycle and oxidative phosphorylation; AroA, an enzyme in the shikimate pathway for aromatic amino acid biosynthesis; CorC, a magnesium and cobalt transport protein and GlnE which regulates cellular nitrogen levels. The phosphorylation of two proteins involved in stress response (HtpG and Ppk) and one protein in cell wall synthesis (Ddl) were also shared.
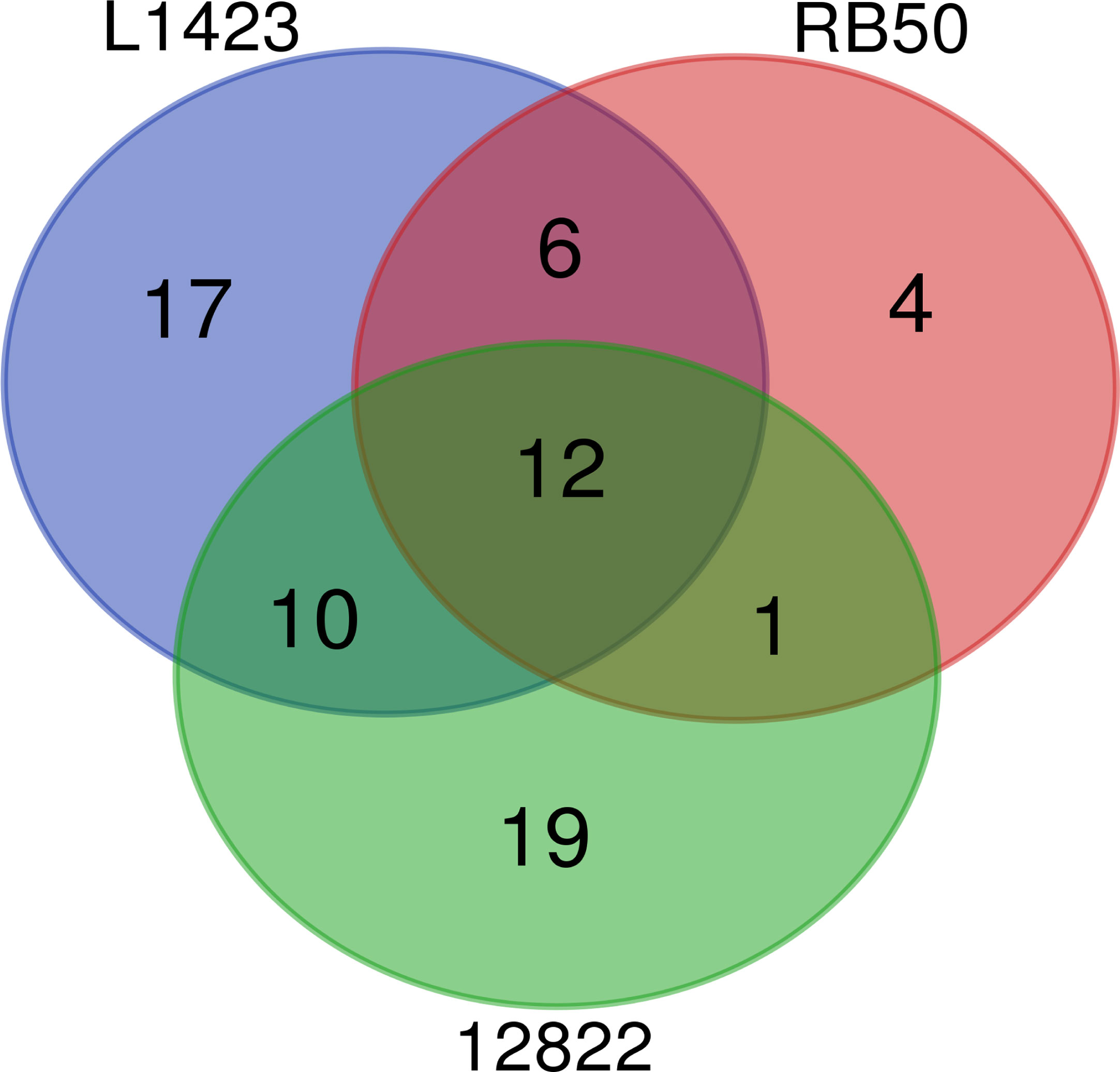
Figure 2 Comparison of the number of phosphorylated proteins identified in B. pertussis L1423, B. bronchiseptica RB50 and B. parapertussis 12822.
Most interestingly, BvgA, the BvgAS response regulator and master transcriptional activator for Bordetella virulence genes were also commonly phosphorylated in the classical bordetellae group. BvgA was found to be heavily phosphorylated with 7 different Ser/Thr phosphorylation sites identified (Figures 3 and 4), 3 of which were identified in all three species (Ser141, Ser143 and Ser 172). A fourth phosphorylated site, Thr144, was identified in B. pertussis and B. parapertussis while in B. bronchiseptica, this phosphorylated site was also identified in the spectra but the A-score localization probability was 93%, slightly lower than the 95% cutoff set. Manual inspection of the spectra helped confirm the phosphorylation of Thr144 in B. bronchiseptica. Additionally, different phosphopeptides with the same precursor mass can be readily separated by nanoLC and have different elution times (Santiago et al., 2019). Manual inspection of the extracted ion chromatography at m/z 686.3295 ± 2.5 ppm for B. bronchiseptica showed three prominent peaks corresponding to three BvgA peptides phosphorylated at Ser143, Thr144 and Ser147 (Figure 5). This provides further evidence that the BvgA peptide (SDSTLISVLSNR) is monophosphorylated at multiple sites. One phosphorylated site (Ser71) was only identified in two species (B. pertussis and B. bronchiseptica). There was also one unique phosphorylated site in B bronchiseptica (Ser147) while another phosphorylated site (Ser150) was unique to B. parapertussis. Interestingly, the majority of phosphorylated sites were located at the DNA binding helix-turn-helix luxR domain, toward the C-terminus.
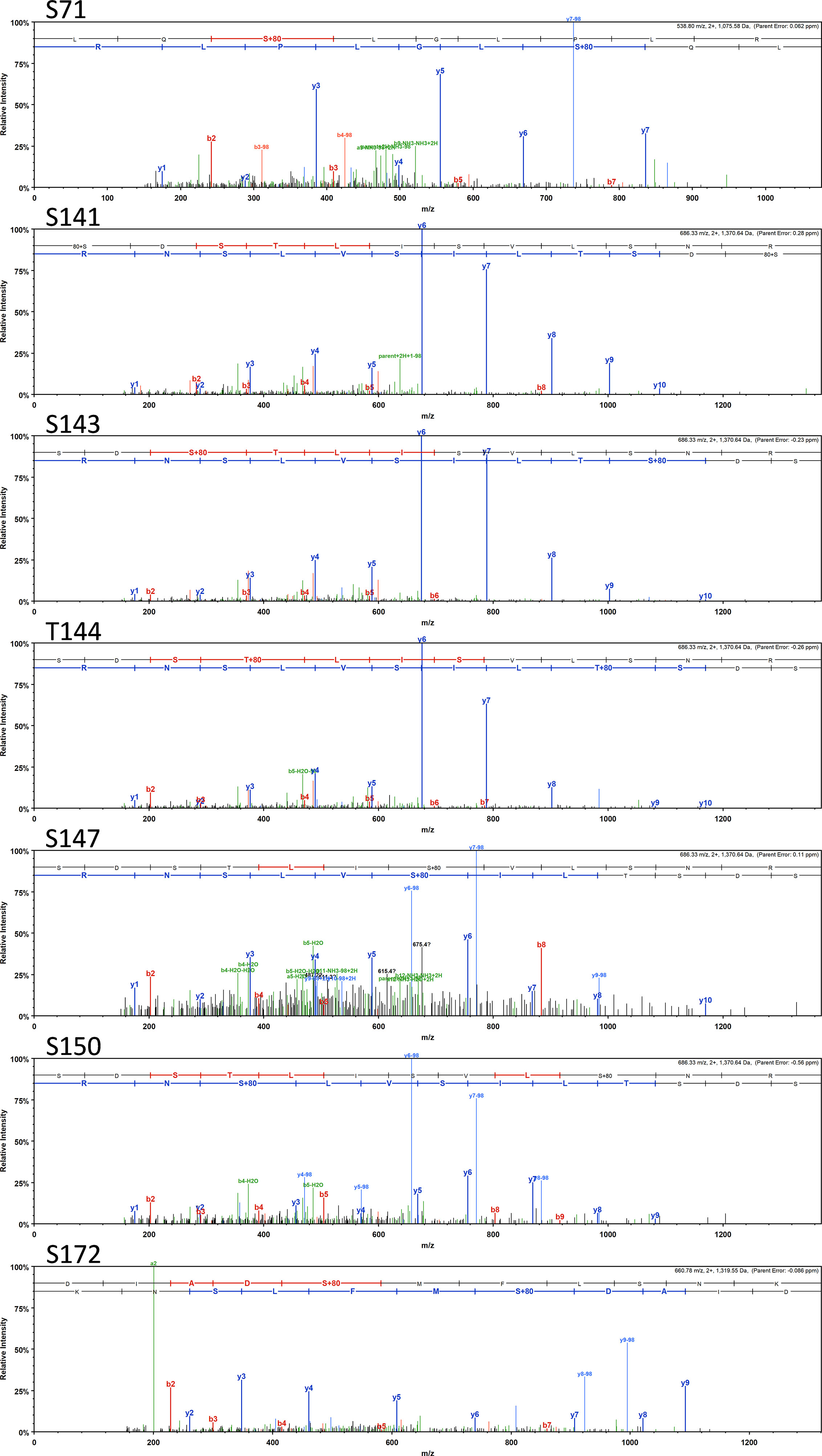
Figure 3 Annotated spectra of the 7 BvgA Ser/Thr sites (Ser71, Ser141, Ser143, Thr144, Ser150 and Ser 172) phosphorylated in classical Bordetellae. Annotated spectra for the highest confident PSM (based on A-score and MOWSE score) is shown.
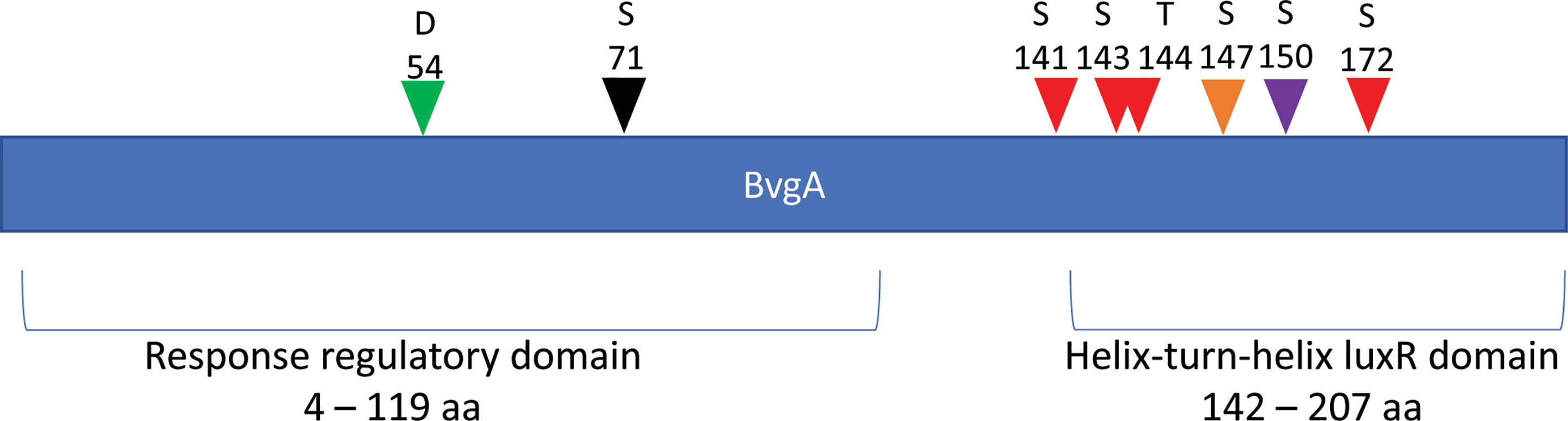
Figure 4 Distribution of phosphosites identified in BvgA. Green arrow denotes the known Asp54 site phosphorylated by BvgS. Red arrows depict Ser/Thr sites phosphorylated in all 3 Bordetella species. The black arrow shows a pSer site in B. pertussis and B. bronchiseptica and the purple arrow illustrates a unique pSer site in B. parapertussis. Finally, orange arrows show a unique pSer site in B. bronchiseptica.
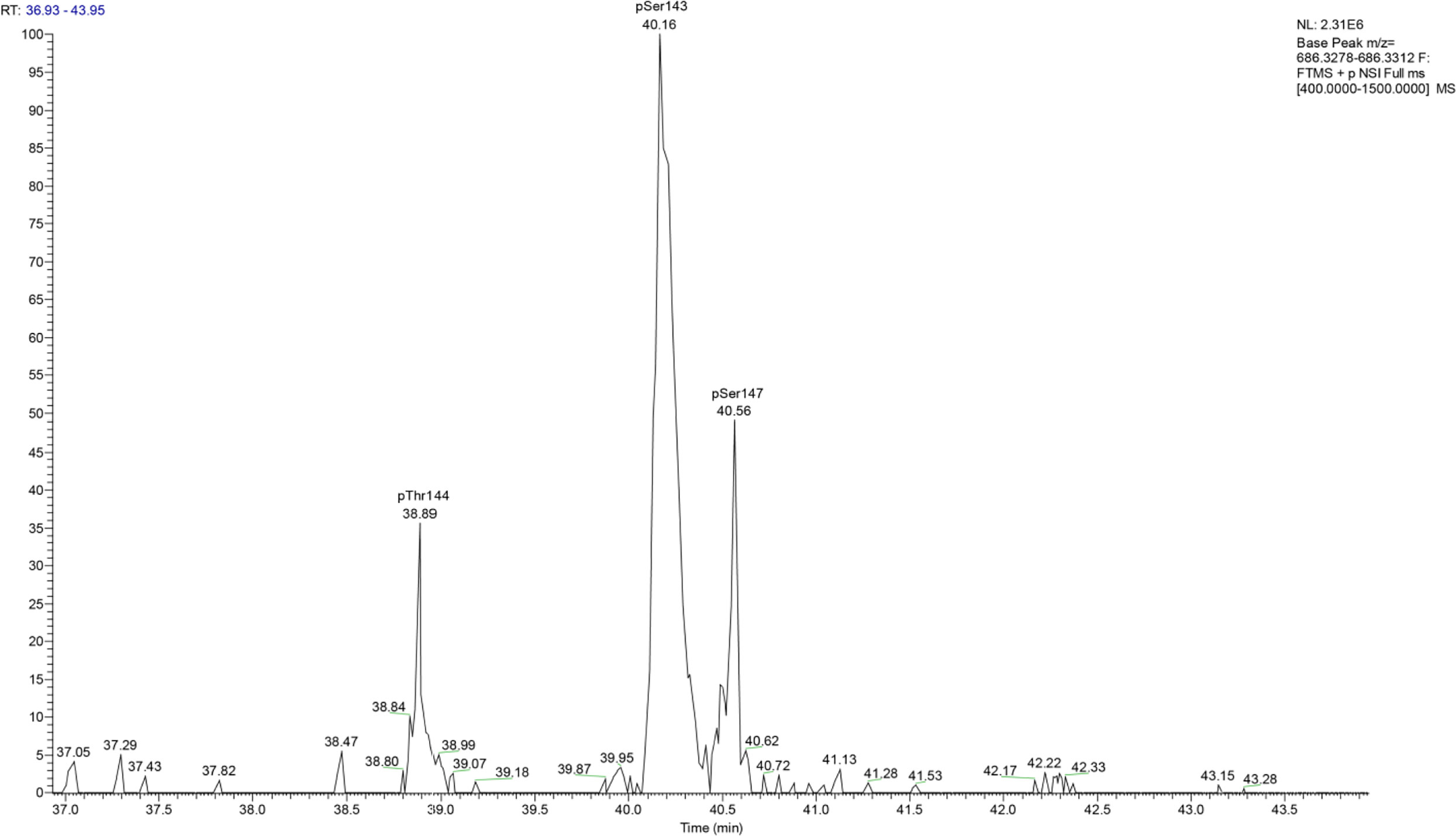
Figure 5 Extracted ion chromatography at m/z 686.3295 ± 2.5 ppm for B. bronchiseptica shows three prominent peaks at retention times 38.89, 40.16 and 40.56 which corresponded to BvgA phosphorylation at Thr144, Ser143 and Ser147, respectively.
For proteins that were exclusively phosphorylated between two Bordetella species (Supplementary Table 4), six proteins were commonly phosphorylated in B. pertussis and B. bronchiseptica. These included a histone protein (BpH1), deoxycytidine triphosphate deaminase (Dcd), two hypothetical proteins (BP2865 and BP1212) and two virulence-related proteins (BfrH and BtrV). BfrH is an iron siderophore receptor while BtrV is an anti-anti sigma factor involved in the regulation of type III secretion system (T3SS) and the only previously characterized serine phosphorylated protein in Bordetella (Kozak et al., 2005). Evidence of BtrV phosphorylation at Ser55 was also observed in B. parapertussis, however the localization probability A-score was 93%, lower than the 95% cutoff. Based on manual spectra inspection and a previous study, we concluded that BtrV is also phosphorylated in B. parapertussis. For B. pertussis and B. parapertussis, 10 proteins were commonly phosphorylated, many of which are involved in core cellular processes (GpmA, OdhB, PyrH, GyrB, RbfA and BP3130) and responses (GroES, BP0965, BP2757 and BP1012). Similar to BtrV, evidence of GroES phosphorylation at Ser23 was also observed with a localization probability of 88%, marginally lower than the cutoff for confident assignment. Finally, one protein, BP3296 (hydrolase), was commonly phosphorylated in B. bronchiseptica and B. parapertussis. Besides BtrV and OdhB, all proteins that were exclusively phosphorylated in two Bordetella species shared the same phosphopeptide and phosphosites.
Conservation of Phosphoproteins and Phosphorylation Sites in Classical Bordetella Species
Of the 70 phosphorylated proteins in the classical bordetellae group, only 1 gene (BPP4111) was absent in B. pertussis while another was a pseudogene (BP0021) and is not expressed. Therefore, there were 68 phosphorylated proteins (97%) that were classified as “core proteins” in classical Bordetella with the potential to be phosphorylated.
To determine whether phosphorylation sites identified in one Bordetella species were conserved in other classical Bordetella species, we mapped the phosphorylation site and 6 amino acids sequences on either side (termed: phosphosite sequence) to the corresponding homologous proteins in the remaining two species. The phosphorylation site and adjacent amino acid sequences were highly conserved with 96-100% of phosphosite sequences showing 100% identity between homologous genes in classical Bordetella species.
Finally, we also examined if phosphorylation differences between classical Bordetella species may be due to proteome differences. We compared whether the phosphoproteins identified in this study were detected in the proteomes from previous Bordetella studies (Kurushima et al., 2012; Luu et al., 2018a; Luu et al., 2018b; Oviedo et al., 2019). Most phosphoproteins (70-75%) identified in one Bordetella species have been previously detected in the proteome of other Bordetella species (Supplementary Table 5).
Essentiality of Phosphorylated Proteins
In a previous study, 609 genes out of 3624 genes (16.8%) in Tohama I were classified as essential when grown in vitro (Gonyar et al., 2019). We found 23 (51.1%) of the 45 phosphorylated proteins in B. pertussis fell into the essential genes, suggesting a higher proportion of essential genes that are phosphorylated (Fisher’s exact test, p < 0.0001). This finding highlights the importance of phosphorylation in B. pertussis.
Functional Category Analysis of Phosphorylated Proteins
Functional category distribution and cellular localization of phosphorylated proteins were similar between the 3 species. The majority of phosphorylated proteins were cytoplasmic (52%-74%), followed by cytoplasmic membrane (13%-14%) and outer membrane (0–4%) while the cellular localization for 12-30% of proteins were unknown. The primary functional categories identified for phosphorylated proteins were central/intermediary metabolism (12%-22%), energy metabolism (11%-17%), conserved hypothetical proteins (4%-11%), cell surface (2%-13%) and macromolecule synthesis/modification (0%-12%) (Figure 6). This was followed by transport/binding protein (4%-7%), cell process (4%-7%) regulation (2%-9%), and pathogenicity (2%-9%). Compared to whole cell proteins identified in our previous B. pertussis proteomic studies (Luu et al., 2018a; Luu et al., 2018b), there was a higher proportion of regulation and central/intermediary metabolism proteins in the B. pertussis phosphorylation dataset, although this was not statistically significant (Figure 6).
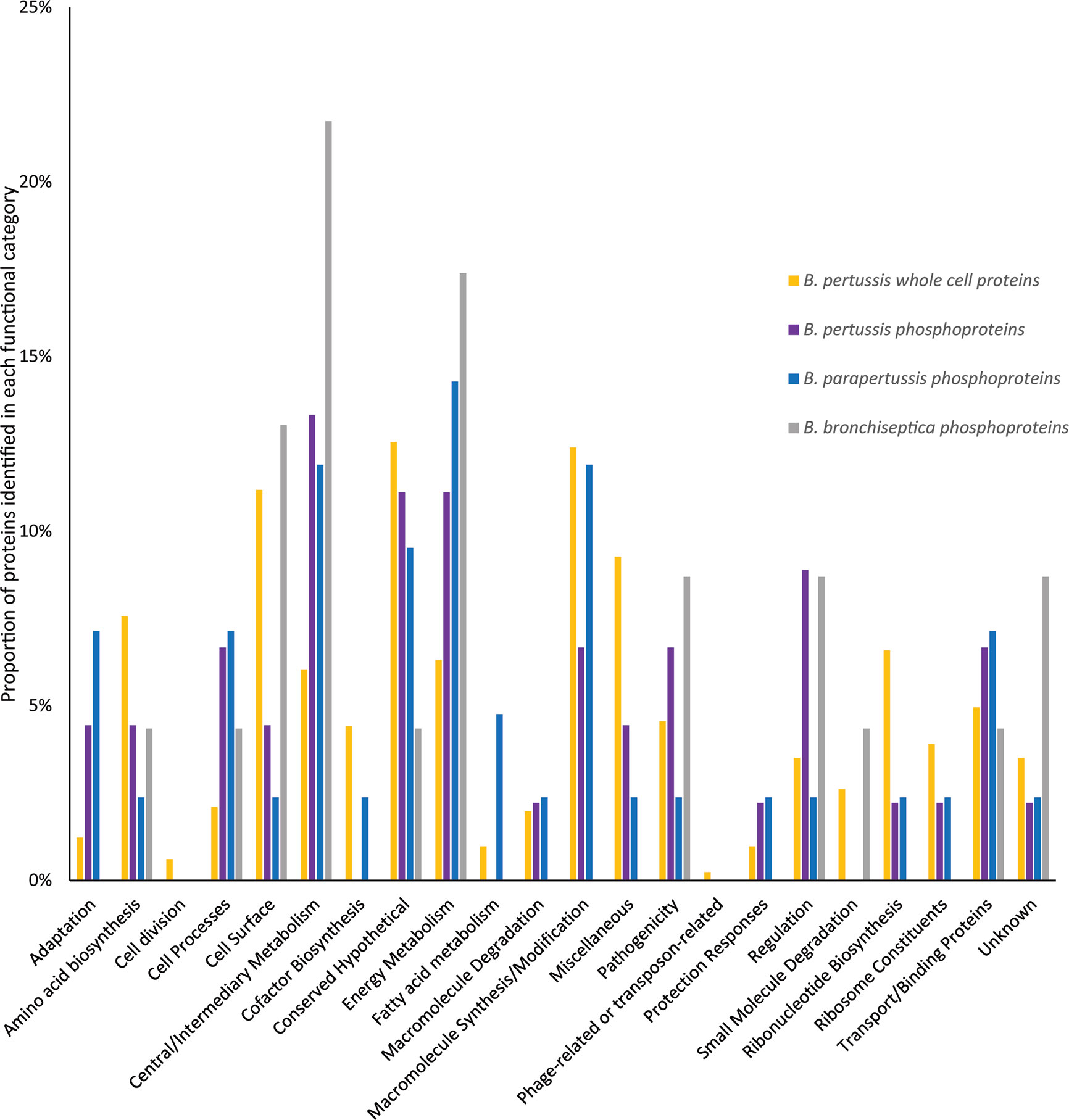
Figure 6 Comparison of the functional categories of phosphorylated proteins identified in B. pertussis (purple bars) and whole cell B. pertussis proteins identified in Luu et al. (2018a; 2018b) (blue bars).* denotes significant difference between phosphorylated and whole cell proteins.
A functional enrichment network analysis of phosphorylated proteins with STRING identified 12 and 11 significantly enriched molecular function GO terms in B. pertussis and B. bronchiseptica (Supplementary Table 6). Enriched GO terms were primarily related to binding including ATP/nucleotide binding, ion binding and protein/unfolded protein binding. Enriched local network clusters and enriched UniProt keywords in STRING also identified an enrichment of clusters/keywords related to carbon and nitrogen metabolism such as the TCA cycle and pentose phosphate pathway (Supplementary Table 6).
To further elucidate the role of phosphorylation in metabolism, the phosphoproteins identified in one or more Bordetella species were collated and mapped onto KEGG pathways (Figure 7). Of the Bordetella proteins involved in central carbon metabolism: four enzymes (Fba, GpmA, Eno and PpsA) in the glycolysis/gluconeogenesis pathway and four enzymes in the TCA cycle (OdhB, SucC, SucD and Mdh) were phosphorylated. PrsA, an enzyme that catalyzes synthesis of phosphoribosyl pyrophosphate (PRPP), was also phosphorylated. PRPP is an important intermediary metabolite required for histidine and nucleic acid biosynthesis.
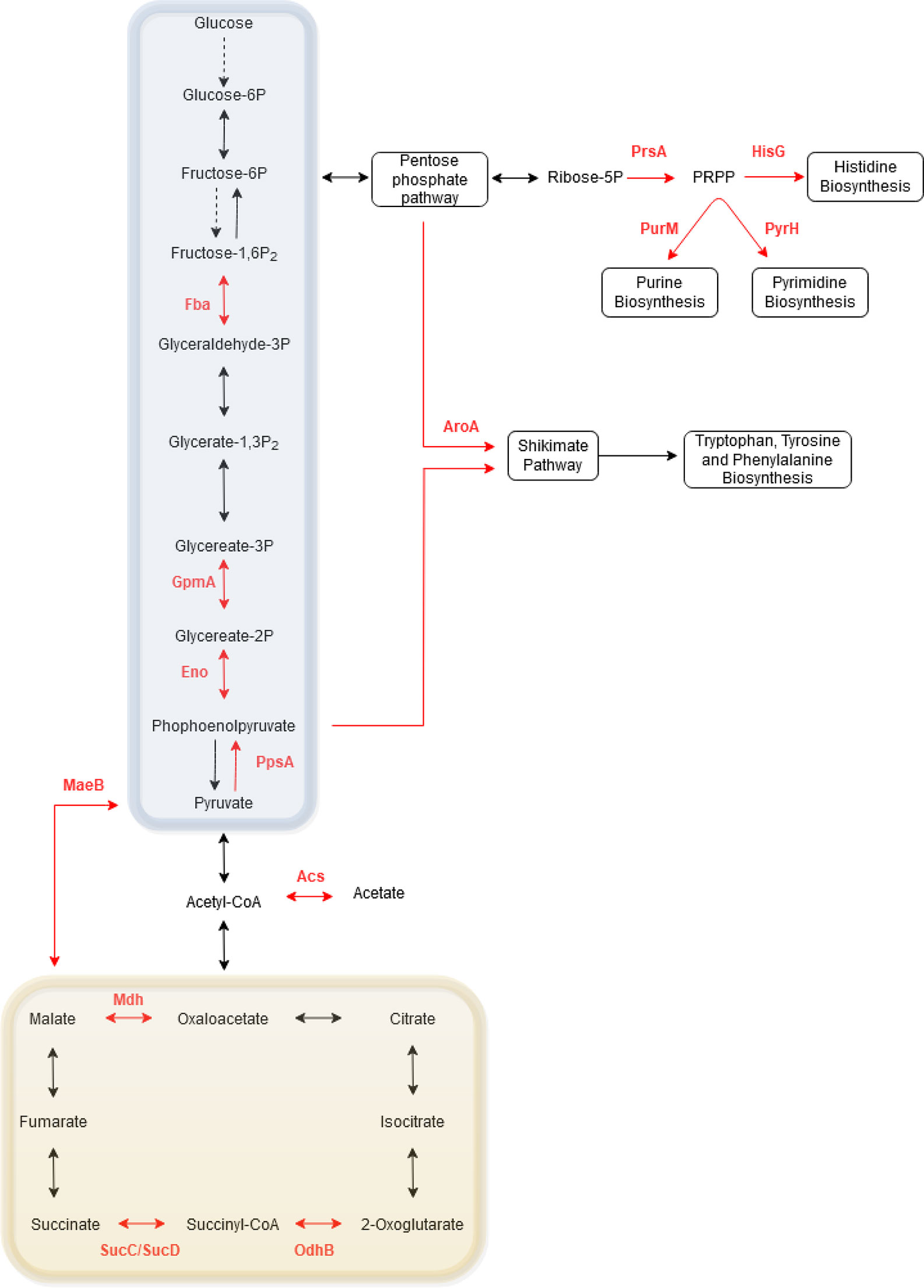
Figure 7 Schematic of the phosphorylated proteins involved in Bordetella central carbon and amino acid metabolism. Red arrows and text illustrate phosphorylated proteins and their enzymatic pathway in Bordetella. Dashed arrows illustrate enzymes that are absent in Bordetella. The glycolysis/gluoconeogenesis pathway is highlighted in the blue box while the TCA cycle is shown in the yellow box.
Two enzymes required for the biosynthesis of amino acids (HisG for histidine and AroA for tryptophan, tyrosine and phenylalanine) and two enzymes for nucleotide synthesis (PurM for purines and PyrH for pyrimidines) were also phosphorylated. Finally, one enzyme involved in acetate (Acs) and pyruvate (MaeB) metabolism pathway was phosphorylated.
Phosphorylated Virulence Factors in Bordetella
As stated above, BtrV (the T3SS anti-anti sigma factor) and BvgA (the virulence response regulator) were the only virulence factors commonly phosphorylated across all 3 Bordetella species (Table 2). In addition, four other virulence factors were also phosphorylated in one or more Bordetella species (Table 2). Three were involved in iron acquisition including BfrH, a siderophore receptor (Burgos et al., 2010), as well as AlcA and AlcC, both of which are involved in the synthesis of the alcaligin siderophore. BfrH was phosphorylated in both B. pertussis and B. bronchiseptica while AlcA and AlcC was only phosphorylated in B. pertussis and B. parapertussis respectively. The final phosphorylated virulence factor was BcrH2, a T3SS chaperonin protein for the pore forming proteins BopB-BopD (Nogawa et al., 2004). BcrH2 was phosphorylated only in B. pertussis.
To further compare the conservation of identified BvgA phosphosites in pathogenic classical and non-classical environmental/opportunistic Bordetella species, all available Bordetella BvgA amino acid sequences from UniProt (up to 1st of October 2020) were downloaded and aligned using MUSCLE (Edgar, 2004) and MEGA X (Kumar et al., 2018). Sequence alignment showed that the previously characterized aspartate phosphorylation site (Asp54) was conserved in 100% of Bordetella species (Figure 8). Of the 7 newly identified Ser/Thr sites in this study, only 1 site (Ser150) was also conserved in every Bordetella species. Two sites (Ser147 and Ser172) were present in all classical Bordetella and most non-classical Bordetella species, with Ser172 absent in B. petrii and B. genomospecies 2 and 7 while Ser147 was also absent in the aforementioned species and B. trematum. Interestingly, 4 phosphorylation sites (Ser71, Ser141, Ser143 and Thr144) were conserved and unique to all classical Bordetella species and absent in non-classical Bordetella (Figure 8).
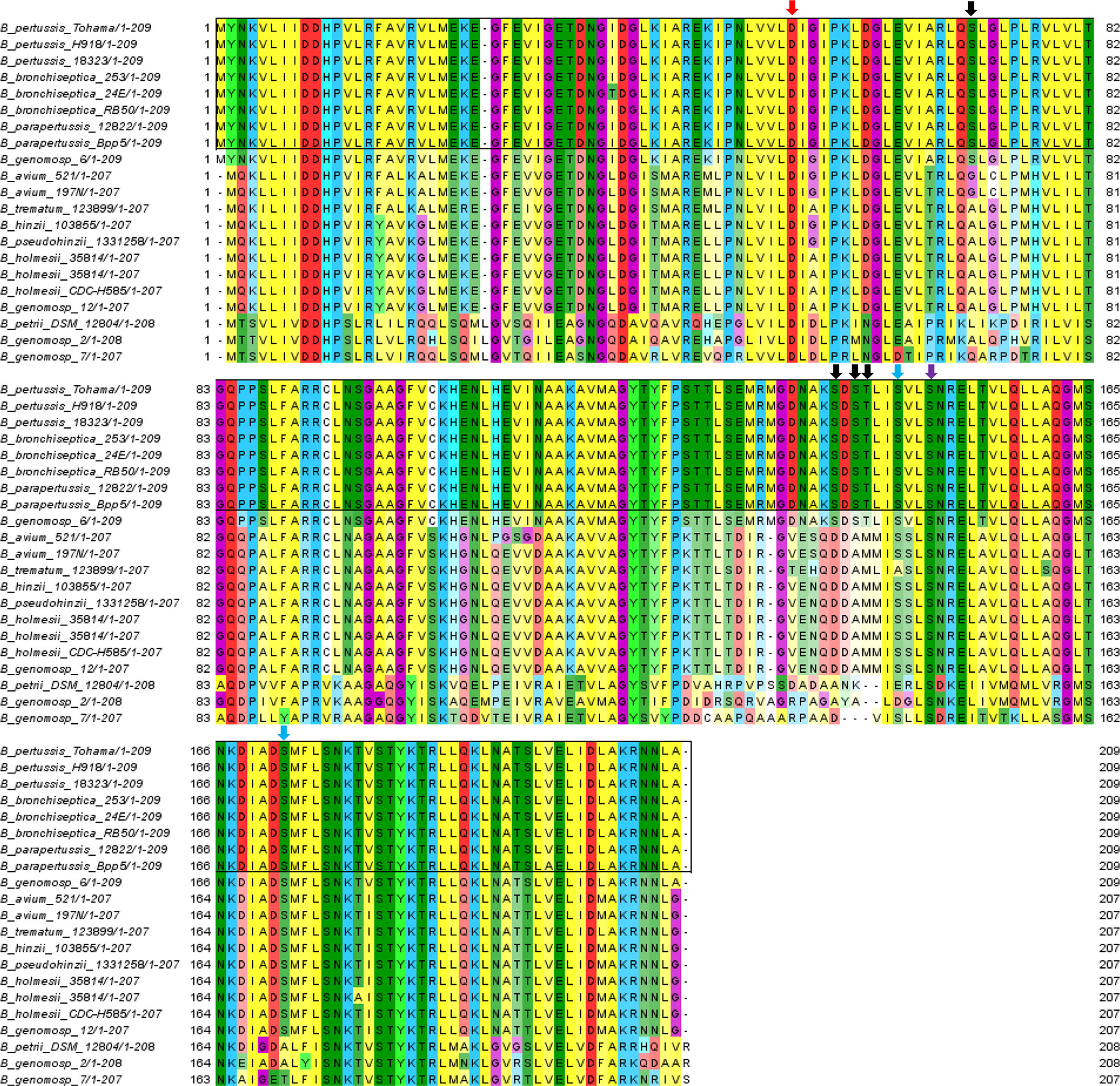
Figure 8 Multiple sequence alignment of BvgA sequences in classical and non-classical Bordetella species. The red and purple arrow shows the phosphorylated Asp54 and Ser150 site conserved in all Bordetella species, respectively. Black arrows illustrate pSer/pThr sites (Ser71, Ser141, Ser143 and Thr 147) conserved in classical and absent in non-classical Bordetella. Blue arrows illustrate pSer sites (Ser147 and Ser172) conserved in most Bordetella species. Classical Bordetella species are depicted at the top in the black outline while non-classical Bordetella species are below.
Potential Ser/Thr/Tyr Kinases in Bordetella
To identify potential Bordetella Hanks-type Ser/Thr kinases (BstK), we searched the SignalCensus database (Galperin et al., 2010) and NCBI conserved domain database [for the Pkc_like superfamily (cl21453)] (Marchler-Bauer et al., 2017). In the SignalCensus database, four potential Ser/Thr kinases were identified in B. bronchiseptica and B. parapertussis, and three in B. pertussis (Table 3). Two of the potential kinases identified in all 3 species were UbiB, a potential protein kinase regulator of UbiI which is involved in ubiquinone biosynthesis, and BP2349, a 3-deoxy-D-manno-octulosonic-acid kinase which may be involved in the LPS core biosynthesis pathway (White et al., 1999; Poon et al., 2000). Interestingly, BP0018 and BB0818 are uncharacterized membrane proteins with a Pkc_like domain, present in Ser/Thr kinases. BP0018 is present in all three species but BB0818|BPP0732 is only present in B. bronchiseptica and B. parapertussis. Both proteins may be potential BstK. In the NCBI conserved domain database (cl21453), a further three potential kinases were identified in all three species. BP2792 was a hypothetical protein while BP2342 and BP3672 both belonged to the subfamily: PhoP regulatory network protein YrbL which are important regulators of adaptation to low Mg2+ environments and virulence (Zwir et al., 2005).
To identify BY-kinases in Bordetella, the BYKdb (Jadeau et al., 2012) and NCBI conserved domain database [BY-kinase domain (cd05387)] (Marchler-Bauer et al., 2017) were searched. No bacterial tyrosine kinases (BY-kinase) were identified in the classical Bordetella group in BYKdb and in the NCBI conserved domain database entry for BY-kinase domain (cd05387). One potential BY-kinase (protein accession no: WP_066122743.1) in BYKdb was identified in some non-classical Bordetella species (B. ansorpii, B. bronchialis, B. flabilis, Bordetella sp. N, H567 and HZ20, Bordetella genomosp. 8, 9, 10, 11 and 13).
Discussion
Protein phosphorylation is an important post translation modification and a rapid signaling mechanism used by cells in response to different environmental changes. Most previous phosphoprotein studies of Bordetella species have predominantly focused on the aspartate and histidine phosphorylation of the two-component systems such as BvgAS (Cummings et al., 2006), RisAS (Coutte et al., 2016) and PlrSR (Bone et al., 2017). Although a previous bacterial phosphoproteomic study (Lai et al., 2017) was able to identify several pHis and pAsp sites, we could not confidently verify any spectra which supported pHis and pAsp sites when Phospho (H) and Phospho (D) were included as variable modifications in our Mascot searches. This was presumably because of the instability of these modifications in acidic buffers. Therefore, pHis and pAsp were not analyzed further. This study did identify 45, 42 and 23 Ser/Thr/Tyr phosphorylated proteins in B. pertussis, B. parapertussis and B. bronchiseptica, respectively. Functional categories of phosphorylated proteins were diverse and encompassed key regulatory, housekeeping and metabolic proteins. Most previous bacterial phosphoproteomic studies in non-Bordetella species used 1– 100 milligrams of protein and employed laborious SCX fractionation steps (separating up to 15 fractions), which greatly increases costs and run time (Supplementary Table 7) (Manteca et al., 2011). Our use of sequential phosphopeptide enrichment (Figure 1) coupled to a highly sensitive Fusion Lumos mass spectrometer enabled identification of similar numbers of phosphoproteins using 0.5 milligram of initial protein input. The advantage of requiring less input protein makes bacterial phosphoproteomics more feasible and accessible, especially for slow growing and fastidious bacteria such as B. pertussis.
Extent and Conservation of Ser/Thr/Tyr Phosphorylation in the Classical Bordetellae
This study found that Ser/Thr/Tyr phosphorylation was highly conserved across the classical bordetellae group and controlled similar core and essential metabolic and virulence pathways in different species. The evolution of Bordetella from environmental species to host adapted pathogen is associated with progressive genome reduction. However, despite significant gene loss in B. pertussis and B. parapertussis, phosphoproteins were highly conserved with only two phosphorylated proteins not found (absent/pseudogene) in all 3 classical species. The strong conservation despite gene loss suggests a strong selection pressure for phosphorylation and that Ser/Thr/Tyr phosphorylation play an important role in regulating fundamental cellular processes in Bordetella including transcription, translation, cell division, stress response, transport and metabolism.
Additionally, there was a high overlap of common phosphorylated proteins (41%) detected between two or more species and a similar proportion of pSer (~70%)/pThr (~20%)/pTyr (10%) sites. Almost all phosphosite sequences identified in one Bordetella species were conserved in other Bordetella species. Since only one hypothesized Ser/Thr/Tyr kinase was identified to be missing in B. pertussis (BB0818|BPP0732) (Table 3), this indicates that phosphosites identified in one Bordetella species also have the potential to be phosphorylated in other Bordetella species.
Remarkably, despite being distantly related, the proportion of phosphorylated pSer/pThr/pTyr sites in Bordetella were similar to those observed in other Gram-negative and Gram-positive model organisms such as E. coli K-12 and B. subtilis (Macek et al., 2007; Macek et al., 2008) (Supplementary Table 7). Many of the phosphoproteins identified in this study, especially those related to central carbon and amino acid metabolism (Fba, GlmM, Eno, SucC and AroA etc.) were also phosphorylated in other diverse bacterial species when these pathways were functional (Shi et al., 2020). This provides further evidence of the ubiquitous nature of Ser/Thr/Tyr protein phosphorylation in bacteria and the important role it plays in providing another layer of control to the cell to rapidly regulate metabolic and housekeeping proteins as phosphorylation is the fastest mode of regulation (Yang et al., 2019).
Many phosphoproteins were only detected in a single Bordetella species. It is possible that phosphosites identified in one Bordetella species may also have been phosphorylated in other Bordetella species but were missed due to limitations in instrument sensitivity to detect the phosphosite. It is also possible that the same phosphosite in different species may have different occupancy levels and hence were not detected in other species where the phosphosite occupancy may be lower. Finally, it is possible that the wide variation in phosphoproteins and sites identified in this study may reflect the highly dynamic nature of protein phosphorylation, the presence of other unknown regulators controlling kinases/phosphatases or the different growth stages of the three species.
Ser/Thr/Tyr Phosphorylation May Regulate Central Carbon and Nitrogen Metabolism
Bordetella proteins involved in central carbon and nitrogen metabolism were found to be significantly enriched for phosphorylation including 15 enzymes involved in glycolysis/gluconeogenesis, TCA cycle, amino acid and nucleotide synthesis (Figure 7). The classical bordetellae group does not contain a functional glycolysis pathway due to the absence of key genes (glucokinase, phosphofructokinase and fructose-1,6-bisphosphatase) (Parkhill et al., 2003), however it does contain a functional and essential gluconeogenesis pathway (Gonyar et al., 2019). Four enzymes in this pathway were found to be phosphorylated which suggests that the gluconeogenesis pathway in Bordetella may be under the control of Ser/Thr/Tyr phosphorylation. In addition to gluconeogenesis, several enzymes in the TCA cycle were also observed to be phosphorylated. Bordetella was long thought not to possess a complete functional TCA cycle due to its inability to metabolize acetyl-CoA and oxaloacetate to 2-oxoglutarate (Thalen et al., 1999). However recently, several studies have confirmed that Bordetella does contain a complete functional and essential TCA cycle (Izac et al., 2015; Gonyar et al., 2019). Although the Bordetella genomes contain all the necessary genes for the TCA cycle, not all strains were able to use citrate as the sole metabolite for growth (an indicator for a complete TCA cycle) (Dos Santos et al., 2017). Dos Santos et al. (2017) hypothesized that regulatory networks controlling the TCA cycle may explain the strain specific differences and discrepancies observed between studies over whether Bordetella has a functional TCA cycle. It is tempting to speculate that Ser/Thr/Tyr phosphorylation of the TCA cycle may be the regulator network responsible for these important metabolic differences as Ser/Thr/Tyr phosphorylation is known to be a key regulator of the TCA cycle in other bacteria and archaea (Macek et al., 2007; Macek et al., 2008; Esser et al., 2012), however further studies are required to confirm this.
Ser/Thr Phosphorylation of BvgA May Affect DNA Binding Capability and Play a Key Role in the Adaptive Evolution of Bordetella From Environmental Species to Human Pathogenic Species
BvgAS is the primary regulator for over 550 genes in Bordetella (Moon et al., 2017). It is present in all classical and non-classical Bordetella species except B. ansorpii (Linz et al., 2016). Through phase variation, BvgA controls almost all Bordetella virulence genes, several metabolic and stress/protection response pathways and further regulates >25 different transcriptional regulators (Moon et al., 2017). It is well known that BvgA is phosphorylated at Asp54 by BvgS, its cognate sensor kinase. Asp54 is highly conserved in all bacterial two-component response regulators. Previously, it was suggested that Asp54 is the only site phosphorylated on BvgA (Boulanger et al., 2013) using phos-tag gels and that phosphorylation of Asp54 is both necessary and sufficient for virulence gene expression. Significantly however we discovered for the first time that BvgA is also highly phosphorylated on multiple Ser/Thr residues. The discovery of multiple new phosphorylated sites previously missed by Boulanger et al. (2013) and others may be due to our use of highly sensitive LC-MS/MS which can specifically identify phosphorylated amino acid residues as opposed to phos-tag gels which can only identify the presence of phosphorylation (as indicated by a shift in band size) but not which or how many amino acid residues are phosphorylated. Interestingly, in Boulanger et al. (2013) the deletion of BvgS, the mutation of Asp54 and the presence of Bvg modulating factors (50 mM MgSO4) were all found to eliminate BvgA phosphorylation in the phos-tag gel. This may suggest that additional Ser/Thr phosphorylation in BvgA may be dependent on the initial phosphorylation of Asp54.
In contrast to eukaryotes, multi-phosphorylated proteins in bacteria are rare and most phosphoproteins were mono-phosphorylated in Bordetella. BvgA however was highly phosphorylated with 7 phosphosites detected, 6 of which were located in the helix-turn-helix luxR domain for DNA binding. Multi-phosphorylated proteins in eukaryotes are common and act as “molecular switchboards” for signal fine tuning (Wang et al., 2001). The multi-phosphorylation of BvgA in the DNA-binding domain suggests that Ser/Thr phosphorylation may play an important role in stabilizing or destabilizing BvgA binding to DNA for further fine-tuning of virulence gene expression. Other two-component response regulators that were phosphorylated include BP0991 and BP1092 in B. pertussis and BPP0020 in B. parapertussis. All were phosphorylated at sites located in the helix-turn-helix domain for protein-DNA binding. This suggests that Ser/Thr phosphorylation may be a general mechanism for bacteria to control the ability of response regulators to bind DNA.
Evidence for Ser/Thr/Tyr phosphorylation in controlling the DNA-binding capacity of two-component response regulators such as BvgA has been observed in other Gram-positive pathogens including CovR in Group A and B Streptococcus (GAS/GBS) (Lin W. J. et al., 2009; Horstmann et al., 2014), GraR and VraR in Staphylococcus aureus (Fridman et al., 2013; Canova et al., 2014), WalR in Bacillus subtilis (Libby et al., 2015) and DosR in Mycobacterium tuberculosis (Chao et al., 2010). These interactions between two-component response regulators and Ser/Thr/Tyr phosphorylation were found to be important for virulence. Ser/Thr/Tyr phosphorylation can either antagonize or amplify the activity of response regulators. For example, CovR is the major response regulator that represses gene expression in GAS and GBS when Asp53 is phosphorylated by its cognate sensor kinase, CovS. However, phosphorylation of CovR at Thr65 was found to antagonize Asp53 and led to increased virulence gene expression by reducing/eliminating CovR’s ability to bind DNA (Lin W. J. et al., 2009; Horstmann et al., 2014). In contrast, phosphorylation of WalR at Thr101 in B. subtilis enhanced WalR activity and expression of WalR cell wall synthesis regulon (Libby et al., 2015). In Gram-negatives, the role of Ser/Thr/Tyr phosphorylation of response regulators has not yet been characterized, however there is limited evidence that phosphorylation of two-component system response regulators also occurs with Ser77 and Ser80 phosphorylated on ActR in Sinorhizobium meliloti (Liu et al., 2015). Our results provide further evidence that Ser/Thr phosphorylation of two-component response regulators is an important and unexplored regulatory mechanism in Gram-negative bacteria.
Multiple sequence alignment between all available Bordetella BvgA sequences revealed 4 phosphorylation sites in the helix-turn-helix luxR DNA binding domain that were exclusively present in all classical Bordetella species (including Bordetella genomospecies 6 whose closest ancestor is B. bronchiseptica (Spilker et al., 2014)) but absent in non-classical species. While BvgA is functionally interchangeable between the 3 classical species (Martínez De Tejada et al., 1996), functional differences between classical and non-classical BvgA has been demonstrated with B. pertussis and B. holmesii (Gerlach et al., 2004). When B. holmseii BvgA was introduced into B. pertussis, it was found that B. holmesii BvgA could be phosphorylated by B. pertussis BvgS. However, despite being phosphorylated at Asp54, B. holmesii BvgA could not bind to the virulence protomers in B. pertussis or lead to the expression of virulence genes (Horvat and Gross, 2009). Interestingly, when Horvat and Gross (2009) created a hybrid BvgA by replacing the B. holmseii BvgA DNA-binding domain with the B. pertussis BvgA DNA binding domain, DNA binding and virulence gene expression was restored. The mutational substitution of amino acids (not found to be phosphorylated in this study) in B. homesii BvgA did not restore promoter binding. This suggests that functional differences between classical and non-classical BvgA may be due to the ability of BvgA to undergo Ser/Thr phosphorylation at the helix-turn-helix luxR DNA-binding domain in classical Bordetella species. It also suggests that Ser/Thr phosphorylation of the DNA-binding domain in classical BvgA is essential for virulence gene expression. The speciation and adaptation of Bordetella from free-living environmental/opportunistic pathogens (non-classical bordetellae) to host-adapted pathogens (classical bordetellae) has been associated with progressive gene loss (Cummings et al., 2004; Linz et al., 2016), however it is also possible that pathogenic adaptation may also be associated with phosphoproteome changes. The Ser/Thr phosphorylation of BvgA may be a key event in the acquisition of virulence and evolution of the Bordetella genus into a human pathogen. This study identified several potential Bordetella Ser/Thr/Tyr kinases (BstK) and ongoing work is currently being performed to identify the kinase involved in Ser/Thr phosphorylation of BvgA and its role in virulence gene expression.
Interestingly, in the evolution of BvgAS in classical Bordetella, BvgS varied remarkably between species especially at the Venus flytrap 1 domain (VFT1) while in contrast, BvgA was highly conserved (Herrou et al., 2009). The highly conserved nature of BvgA provides further evidence of the potential importance of Ser/Thr phosphorylation in classical Bordetella species and is potentially a result of selection pressure to maintain phosphorylation.
Ser/Thr/Tyr Phosphorylation of Key Virulence Factors and Its Role in Pathogenesis
Ser/Thr/Tyr phosphorylation was found on T3SS (BtrV and BcrH2) and iron acquisition (BfrH, AlcA and AlcC) proteins. The T3SS is an important virulence determinant used by bacterial pathogens to deliver effectors to host cells. The T3SS is present in all classical Bordetella species and absent in most non-classical Bordetella species (except B. ansorpii) (Linz et al., 2016). BtrV is an important anti-anti sigma factor involved in the regulation of T3SS and forms a partner switching complex with two other proteins, BtrU and BtrW. When BtrV is unphosphorylated (or dephosphorylated by BtrU), it binds to BtrW and inhibits type III secretion. Upon phosphorylation by BtrW, BtrV dissociates and type III secretion can occur (Kozak et al., 2005). BtrV was previously found by Kozak et al. (2005) to be phosphorylated at the conserved Ser55 site, however the authors could not rule out phosphorylation of the adjacent site, Ser54. In this study, we detected the monophosphorylation of BtrV at two sites, Ser55 and Ser54, with Ser55 being the predominant site. This study also detected the phosphorylation of BcrH2 at Ser148. BcrH2 is a chaperonin protein that transports the pore forming proteins BopB-BopD from the bacterial cytoplasm (Nogawa et al., 2004). The role of BcrH2 phosphorylation is currently unknown, however it may affect protein turnover or the stabilization of BcrH2 to BopB-BopD.
Iron acquisition is an essential trait for all pathogenic bacteria. This study found three proteins associated with iron acquisition to be phosphorylated including two (AlcA and AlcC) belonging to the alcaligin synthesis pathway. Alcaligin is the primary siderophore produced by Bordetella species. The pathway is present in all classical Bordetella species but absent in most non-classical Bordetella species (except B. holmesii) (Linz et al., 2016). Phosphorylation of key enzymes in this pathway may be an important regulator for iron uptake and virulence in the classical bordetellae group.
Finally, tyrosine phosphorylation has been previously linked to bacterial pathogenicity (Ge and Shan, 2011) with pathogens such as enterohemorrhagic E. coli (Hansen et al., 2013), Shigella flexneri (Standish et al., 2016), Helicobacter pylori (18.5% pTyr) (Ge et al., 2011), Pseudomonas aeruginosa (14.5% pTyr) (Ravichandran et al., 2009) and Klebsiella pneumoniae (25.8% pTyr) (Lin M.-H. et al., 2009) (Supplementary Table 7) having high abundances of pTyr sites. It was found that P. aeruginosa (14.5% had higher pTyr sites compared to its non-pathogenic counterpart P. putida (7.5%) (Ravichandran et al., 2009). However, this study did not identify widespread tyrosine phosphorylation or a difference in the proportion of pTyr sites between the 3 classical species despite B. pertussis considered to have a higher pathogenic potential compared to its ancestor B. bronchiseptica and causes greater disease severity compared to B. parapertussis. It is possible that differences in pTyr may exist between classical pathogenic and non-classical environmental/opportunistic Bordetella species. Additional enrichment of pTyr using specific antibodies may also be needed to completely elucidate the role of tyrosine phosphorylation in Bordetella virulence. This suggests that some of the potential Hanks-type Ser/Thr kinases identified in this study may be dual specificity protein kinases (DSPK) that can phosphorylate Ser/Thr and Tyr. Examples of DSPK have been identified in Bacillus anthracis and M. tuberculosis (Arora et al., 2012; Kusebauch et al., 2014).
Conclusion
This study analyzed the Ser/Thr/Tyr phosphoproteome of 3 classical Bordetella species and identified 70 unique phosphorylated proteins, of which, 41% were phosphorylated in two or more species. Core metabolic pathways in Bordetella were significantly enriched for phosphorylated proteins including gluconeogenesis, the TCA cycle, amino acid and nucleotide synthesis. This study found that serine/threonine phosphorylation of virulence pathways may be an important virulence determining factor separating classical from non-classical Bordetella species. Interestingly, BvgA was found to be heavily phosphorylated by a yet unknown Bstk, especially at the helix-turn-helix luxR domain with 7 different phosphosites identified. This indicates a potential role for Ser/Thr/Tyr phosphorylation to stabilize/destabilize BvgA binding to DNA for virulence regulation (Figure 9). Additional phosphorylation sites were also identified in BvgA-regulated proteins such as the T3SS, possibly providing another layer of control.
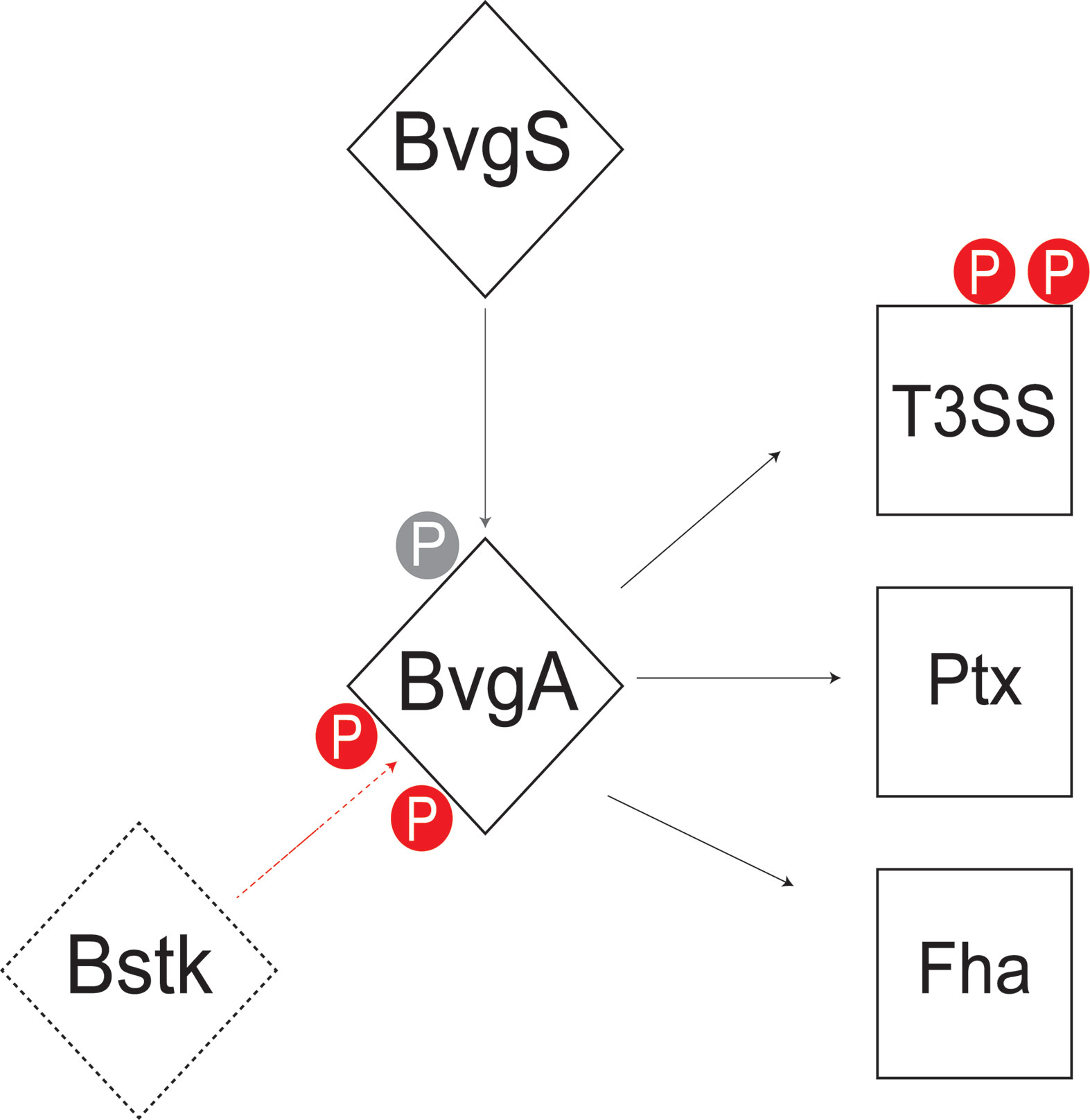
Figure 9 Summary of the Bvg phosphorylation model in Bordetella. BvgA is phosphorylated by BvgS at Asp54 (grey) and one or more unknown Bstk (red) during the Bvg+ phase. BvgA regulates the expression of several virulence factors including the type III secretion system (T3SS), pertussis toxin (Ptx) and filamentous hemagglutinin (Fha). Some of these BvgA-regulated virulence factors such as T3SS are also phosphorylated at Ser/Thr/Tyr sites (red).
As the aim of this study was to provide the first snapshot of the phosphoproteome in classical Bordetella species, it is likely that under different growth stages and conditions such as other Bvg phases, iron limitation and biofilm formation etc., other important phosphorylation events for biology and virulence will be identified. Further characterization of the phosphoproteome in non-classical bordetellae especially environmental associated Bordetella species will also uncover the role of phosphorylation in the evolution of the Bordetella genus toward a host-adapted pathogen. In conclusion, this study provides the first insight into the Ser/Thr/Tyr phosphoproteome of classical Bordetella species and its role in Bordetella biology and virulence.
Data Availability Statement
The datasets presented in this study can be found in online repositories. The names of the repository/repositories and accession number(s) can be found below: http://www.proteomexchange.org/, PXD020866.
Author Contributions
LL: conceptualization, methodology, investigation, formal analysis, writing—original draft preparation, and visualization. LZ: methodology, formal analysis, validation, and writing—review and editing. SK: software, formal analysis, and writing—review and editing. MR: methodology, formal analysis, validation, and writing—review and editing. RL: conceptualization, methodology, supervision, formal analysis, resources, funding acquisition, and writing—review and editing. All authors contributed to the article and approved the submitted version.
Funding
The funding for this project was provided by the National Health and Medical Research Council of Australia (NHMRC).
Conflict of Interest
The authors declare that the research was conducted in the absence of any commercial or financial relationships that could be construed as a potential conflict of interest.
Supplementary Material
The Supplementary Material for this article can be found online at: https://www.frontiersin.org/articles/10.3389/fcimb.2021.660280/full#supplementary-material
Supplementary Table 1 | List of Ser/Thr/Tyr phosphorylated proteins, peptides and sites identified in B. pertussis L1423 with a localization probability (A-score) ≥95%.
Supplementary Table 2 | List of Ser/Thr/Tyr phosphorylated proteins, peptides and sites identified in B. parapertussis 12822 with a localization probability (A-score) ≥95%.
Supplementary Table 3 | List of Ser/Thr/Tyr phosphorylated proteins, peptides and sites identified in B. bronchiseptica RB50 with a localization probability (A-score) ≥95%.
Supplementary Table 4 | Common phosphorylated proteins identified in two or more Bordetella species. Localization probability below the cut-off are indicated.
Supplementary Table 5 | List of unique phosphoproteins detected in Bordetella proteomic studies.
Supplementary Table 6 | List of significantly functionally enriched clusters of phosphorylated proteins identified with STRING v11.
Supplementary Table 7 | Comparison of the amount of starting proteins digested and the number of Ser/Thr/Tyr phosphoproteins detected in this study with previous bacterial phosphoproteomic studies.
References
Arora, G., Sajid, A., Arulanandh, M. D., Singhal, A., Mattoo, A. R., Pomerantsev, A. P., et al. (2012). Unveiling the novel dual specificity protein kinases in Bacillus anthracis identification of the first prokaryotic dual specificity tyrosine phosphorylation-regulated kinase (DYRK)-like kinase. J. Biol. Chem. 287, 26749–26763. doi: 10.1074/jbc.M112.351304
Bart, M. J., Van Gent, M., Van Der Heide, H. G., Boekhorst, J., Hermans, P., Parkhill, J., et al. (2010). Comparative genomics of prevaccination and modern Bordetella pertussis strains. BMC Genomics 11, 627. doi: 10.1186/1471-2164-11-627
Bechet, E., Guiral, S., Torres, S., Mijakovic, I., Cozzone, A.-J., Grangeasse, C. (2009). Tyrosine-kinases in bacteria: from a matter of controversy to the status of key regulatory enzymes. Amino Acids 37, 499. doi: 10.1007/s00726-009-0237-8
Bone, M. A., Wilk, A. J., Perault, A. I., Marlatt, S. A., Scheller, E. V., Anthouard, R., et al. (2017). Bordetella PlrSR regulatory system controls BvgAS activity and virulence in the lower respiratory tract. Proc. Natl. Acad. Sci. 114, E1519–E1527. doi: 10.1073/pnas.1609565114
Boulanger, A., Chen, Q., Hinton, D. M., Stibitz, S. (2013). In vivo phosphorylation dynamics of the B ordetella pertussis virulence-controlling response regulator BvgA. Mol. Microbiol. 88, 156–172. doi: 10.1111/mmi.12177
Burgos, J. M., King-Lyons, N. D., Connell, T. D. (2010). Expression of BfrH, a putative siderophore receptor of Bordetella bronchiseptica, is regulated by iron, Fur1, and the extracellular function sigma factor EcfI. Infect. Immun. 78, 1147–1162. doi: 10.1128/IAI.00961-09
Canova, M. J., Baronian, G., Brelle, S., Cohen-Gonsaud, M., Bischoff, M., Molle, V. (2014). A novel mode of regulation of the Staphylococcus aureus Vancomycin-resistance-associated response regulator VraR mediated by Stk1 protein phosphorylation. Biochem. Biophys. Res. Commun. 447, 165–171. doi: 10.1016/j.bbrc.2014.03.128
Chao, J. D., Papavinasasundaram, K. G., Zheng, X., Chávez-Steenbock, A., Wang, X., Lee, G. Q., et al. (2010). Convergence of Ser/Thr and two-component signaling to coordinate expression of the dormancy regulon in Mycobacterium tuberculosis. J. Biol. Chem. 285, 29239–29246. doi: 10.1074/jbc.M110.132894
Coutte, L., Huot, L., Antoine, R., Slupek, S., Merkel, T. J., Chen, Q., et al. (2016). The multifaceted RisA regulon of Bordetella pertussis. Sci. Rep. 6, 32774. doi: 10.1038/srep32774
Cummings, C., Brinig, M., Lepp, P., Van De Pas, S., Relman, D. (2004). Bordetella species are distinguished by patterns of substantial gene loss and host adaptation. J. Bacteriol. 186, 1484–1492. doi: 10.1128/JB.186.5.1484-1492.2004
Cummings, C., Bootsma, H., Relman, D., Miller, J. (2006). Species-and strain-specific control of a complex, flexible regulon by Bordetella BvgAS. J. Bacteriol. 188, 1775–1785. doi: 10.1128/JB.188.5.1775-1785.2006
Diavatopoulos, D. A., Cummings, C. A., Schouls, L. M., Brinig, M. M., Relman, D. A., Mooi, F. R. (2005). Bordetella pertussis, the causative agent of whooping cough, evolved from a distinct, human-associated lineage of B. bronchiseptica. PloS Pathog. 1, e45. doi: 10.1371/journal.ppat.0010045
Dos Santos, F. B., Olivier, B. G., Boele, J., Smessaert, V., De Rop, P., Krumpochova, P., et al. (2017). Probing the genome-scale metabolic landscape of Bordetella pertussis, the causative agent of whooping cough. Appl. Environ. Microbiol. 83, e01528–e01517. doi: 10.1128/AEM.01528-17
Dworkin, J. (2015). Ser/Thr phosphorylation as a regulatory mechanism in bacteria. Curr. Opin. Microbiol. 24, 47–52. doi: 10.1016/j.mib.2015.01.005
Edgar, R. C. (2004). MUSCLE: multiple sequence alignment with high accuracy and high throughput. J. Nucleic Acids Res. 32, 1792–1797. doi: 10.1093/nar/gkh340
Esser, D., Pham, T., Reimann, J., Albers, S., Siebers, B., Wright, P. (2012). Change of carbon source causes dramatic effects in the phospho-proteome of the archaeon Sulfolobus solfataricus. J. Proteome Res. 11, 4823–4833. doi: 10.1021/pr300190k
Fridman, M., Williams, G. D., Muzamal, U., Hunter, H., Siu, K. M., Golemi-Kotra, D. (2013). Two unique phosphorylation-driven signaling pathways crosstalk in Staphylococcus aureus to modulate the cell-wall charge: Stk1/Stp1 meets GraSR. Biochemistry 52, 7975–7986. doi: 10.1021/bi401177n
Galperin, M. Y., Higdon, R., Kolker, E. (2010). Interplay of heritage and habitat in the distribution of bacterial signal transduction systems. Mol. Biosyst. 6, 721–728. doi: 10.1039/b908047c
Ge, R., Sun, X., Xiao, C., Yin, X., Shan, W., Chen, Z., et al. (2011). Phosphoproteome analysis of the pathogenic bacterium Helicobacter pylori reveals over-representation of tyrosine phosphorylation and multiply phosphorylated proteins. Proteomics 11, 1449–1461. doi: 10.1002/pmic.201000649
Ge, R., Shan, W. (2011). Bacterial phosphoproteomic analysis reveals the correlation between protein phosphorylation and bacterial pathogenicity. Genomics Proteomics Bioinf. 9, 119–127. doi: 10.1016/S1672-0229(11)60015-6
Gerlach, G., Janzen, S., Beier, D., Gross, R. (2004). Functional characterization of the BvgAS two-component system of Bordetella holmesii. Microbiology 150, 3715–3729. doi: 10.1099/mic.0.27432-0
Gonyar, L. A., Gelbach, P. E., Mcduffie, D. G., Koeppel, A. F., Chen, Q., Lee, G., et al. (2019). In Vivo Gene Essentiality and Metabolism in Bordetella pertussis. mSphere 4, e00694–e00618. doi: 10.1128/mSphere.00694-18
Gross, R., Guzman, C. A., Sebaihia, M., Dos Santos, V., Pieper, D. H., Koebnik, R., et al. (2008). The missing link: Bordetella petrii is endowed with both the metabolic versatility of environmental bacteria and virulence traits of pathogenic Bordetellae. BMC Genomics 9, 449. doi: 10.1186/1471-2164-9-449
Hansen, A.-M., Chaerkady, R., Sharma, J., Díaz-Mejía, J. J., Tyagi, N., Renuse, S., et al. (2013). The Escherichia coli phosphotyrosine proteome relates to core pathways and virulence. PloS Pathog. 9, e1003403. doi: 10.1371/journal.ppat.1003403
Hasan, M. M., Rashid, M. M., Khatun, M. S., Kurata, H. (2019). Computational identification of microbial phosphorylation sites by the enhanced characteristics of sequence information. Sci. Rep. 9, 1–9. doi: 10.1038/s41598-019-44548-x
Herrou, J., Debrie, A.-S., Willery, E., Renaud-Mongenie, G., Locht, C., Mooi, F., et al. (2009). Molecular evolution of the two-component system BvgAS involved in virulence regulation in Bordetella. PloS One 4, e6996. doi: 10.1371/annotation/ae7f86ff-0aca-4411-aed3-3eeea9303abb
Horstmann, N., Saldaña, M., Sahasrabhojane, P., Yao, H., Su, X., Thompson, E., et al. (2014). Dual-site phosphorylation of the control of virulence regulator impacts group a streptococcal global gene expression and pathogenesis. PloS Pathog. 10, e1004088. doi: 10.1371/journal.ppat.1004088
Horvat, A., Gross, R. (2009). Molecular characterization of the BvgA response regulator of Bordetella holmesii. Microbiol. Res. 164, 243–252. doi: 10.1016/j.micres.2006.11.015
Izac, M., Garnier, D., Speck, D., Lindley, N. D. (2015). A functional tricarboxylic acid cycle operates during growth of Bordetella pertussis on amino acid mixtures as sole carbon substrates. PloS One 10, e0145251. doi: 10.1371/journal.pone.0145251
Jadeau, F., Grangeasse, C., Shi, L., Mijakovic, I., Deléage, G., Combet, C. (2012). BYKdb: the Bacterial protein tYrosine Kinase database. Nucleic Acids Res. 40, D321–D324. doi: 10.1093/nar/gkr915
Kozak, N. A., Mattoo, S., Foreman-Wykert, A. K., Whitelegge, J. P., Miller, J. F. (2005). Interactions between partner switcher orthologs BtrW and BtrV regulate type III secretion in Bordetella. J. Bacteriol. 187, 5665–5676. doi: 10.1128/JB.187.16.5665-5676.2005
Kumar, S., Stecher, G., Li, M., Knyaz, C., Tamura, K. (2018). MEGA X: molecular evolutionary genetics analysis across computing platforms. Mol. Biol. Evol. 35, 1547–1549. doi: 10.1093/molbev/msy096
Kurushima, J., Kuwae, A., Abe, A. (2012). The type III secreted protein BspR regulates the virulence genes in Bordetella bronchiseptica. PloS One 7, e38925. doi: 10.1371/journal.pone.0038925
Kusebauch, U., Ortega, C., Ollodart, A., Rogers, R. S., Sherman, D. R., Moritz, R. L., et al. (2014). Mycobacterium tuberculosis supports protein tyrosine phosphorylation. Proc. Natl. Acad. Sci. 111, 9265–9270. doi: 10.1073/pnas.1323894111
Lai, S.-J., Tu, I.-F., Wu, W.-L., Yang, J.-T., Luk, L. Y., Lai, M.-C., et al. (2017). Site-specific His/Asp phosphoproteomic analysis of prokaryotes reveals putative targets for drug resistance. BMC Microbiol. 17, 1–10. doi: 10.1186/s12866-017-1034-2
Libby, E. A., Goss, L. A., Dworkin, J. (2015). The eukaryotic-like Ser/Thr kinase PrkC regulates the essential WalRK two-component system in Bacillus subtilis. PloS Genet. 11, e1005275. doi: 10.1371/journal.pgen.1005275
Lin, M.-H., Hsu, T.-L., Lin, S.-Y., Pan, Y.-J., Jan, J.-T., Wang, J.-T., et al. (2009a). Phosphoproteomics of Klebsiella pneumoniae NTUH-K2044 reveals a tight link between tyrosine phosphorylation and virulence. Mol. Cell. Proteomics 8, 2613–2623. doi: 10.1074/mcp.M900276-MCP200
Lin, W. J., Walthers, D., Connelly, J. E., Burnside, K., Jewell, K. A., Kenney, L. J., et al. (2009b). Threonine phosphorylation prevents promoter DNA binding of the Group B Streptococcus response regulator CovR. Mol. Microbiol. 71, 1477–1495. doi: 10.1111/j.1365-2958.2009.06616.x
Linz, B., Ivanov, Y. V., Preston, A., Brinkac, L., Parkhill, J., Kim, M., et al. (2016). Acquisition and loss of virulence-associated factors during genome evolution and speciation in three clades of Bordetella species. BMC Genomics 17, 767. doi: 10.1186/s12864-016-3112-5
Liu, T., Tian, C. F., Chen, W. X. (2015). Site-specific Ser/Thr/Tyr phosphoproteome of Sinorhizobium meliloti at stationary phase. PloS One 10, e0139143. doi: 10.1145/2818302
Luu, L. D. W., Octavia, S., Zhong, L., Raftery, M., Sintchenko, V., Lan, R. (2017). Characterisation of the Bordetella pertussis secretome under different media. J. Proteomics 158, 43–51. doi: 10.1016/j.jprot.2017.02.010
Luu, L. D. W., Octavia, S., Zhong, L., Raftery, M. J., Sintchenko, V., Lan, R. (2018a). Comparison of the whole cell proteome and secretome of epidemic Bordetella pertussis strains from the 2008-2012 Australian epidemic under sulfate modulating conditions. Front. Microbiol. 9, 2851. doi: 10.3389/fmicb.2018.02851
Luu, L. D. W., Octavia, S., Zhong, L., Raftery, M. J., Sintchenko, V., Lan, R. (2018b). Proteomic Adaptation of Australian Epidemic Bordetella pertussis. Proteomics 18, 1700237. doi: 10.1002/pmic.201700237
Macek, B., Mijakovic, I., Olsen, J. V., Gnad, F., Kumar, C., Jensen, P. R., et al. (2007). The serine/threonine/tyrosine phosphoproteome of the model bacterium Bacillus subtilis. Mol. Cell. Proteomics 6, 697–707. doi: 10.1074/mcp.M600464-MCP200
Macek, B., Gnad, F., Soufi, B., Kumar, C., Olsen, J. V., Mijakovic, I., et al. (2008). Phosphoproteome analysis of E. coli reveals evolutionary conservation of bacterial Ser/Thr/Tyr phosphorylation. Mol. Cell. Proteomics 7, 299–307. doi: 10.1074/mcp.M700311-MCP200
Manteca, A., Ye, J., Sánchez, J., Jensen, O. N. (2011). Phosphoproteome analysis of Streptomyces development reveals extensive protein phosphorylation accompanying bacterial differentiation. J. Proteome Res. 10, 5481–5492. doi: 10.1021/pr200762y
Marchler-Bauer, A., Bo, Y., Han, L., He, J., Lanczycki, C. J., Lu, S., et al. (2017). CDD/SPARCLE: functional classification of proteins via subfamily domain architectures. Nucleic Acids Res. 45, D200–D203. doi: 10.1093/nar/gkw1129
Martínez De Tejada, G., Miller, J. F., Cotter, P. A. (1996). Comparative analysis of the virulence control systems of Bordetella pertussis and Bordetella bronchiseptica. Mol. Microbiol. 22, 895–908. doi: 10.1046/j.1365-2958.1996.01538.x
Mattoo, S., Cherry, J. D. (2005). Molecular pathogenesis, epidemiology, and clinical manifestations of respiratory infections due to Bordetella pertussis and other Bordetella subspecies. Clin. Microbiol. Rev. 18, 326–382. doi: 10.1128/CMR.18.2.326-382.2005
Miller, M. L., Soufi, B., Jers, C., Blom, N., Macek, B., Mijakovic, I. (2009). NetPhosBac–a predictor for Ser/Thr phosphorylation sites in bacterial proteins. Proteomics 9, 116–125. doi: 10.1002/pmic.200800285
Moon, K., Bonocora, R. P., Kim, D. D., Chen, Q., Wade, J. T., Stibitz, S., et al. (2017). The BvgAS Regulon of Bordetella pertussis. mBio 8, e01526–e01517. doi: 10.1128/mBio.01526-17
Nancy, Y. Y., Wagner, J. R., Laird, M. R., Melli, G., Rey, S., Lo, R., et al. (2010). PSORTb 3.0: improved protein subcellular localization prediction with refined localization subcategories and predictive capabilities for all prokaryotes. Bioinformatics 26, 1608–1615. doi: 10.1093/bioinformatics/btq249
Nogawa, H., Kuwae, A., Matsuzawa, T., Abe, A. (2004). The type III secreted protein BopD in Bordetella bronchiseptica is complexed with BopB for pore formation on the host plasma membrane. J. Bacteriol. 186, 3806–3813. doi: 10.1128/JB.186.12.3806-3813.2004
Oviedo, J. M., Surmann, K., Gorgojo, J. P., Valdez, H., Dhople, V. M., Lamberti, Y., et al. (2019). Shotgun proteomic analysis of Bordetella parapertussis provides insights into the physiological response to iron starvation and potential new virulence determinants absent in Bordetella pertussis. J. Proteomics 206, 103448. doi: 10.1016/j.jprot.2019.103448
Park, J., Zhang, Y., Buboltz, A. M., Zhang, X., Schuster, S. C., Ahuja, U., et al. (2012). Comparative genomics of the classical Bordetella subspecies: the evolution and exchange of virulence-associated diversity amongst closely related pathogens. BMC Genomics 13, 545. doi: 10.1186/1471-2164-13-545
Parkhill, J., Sebaihia, M., Preston, A., Murphy, L. D., Thomson, N., Harris, D. E., et al. (2003). Comparative analysis of the genome sequences of Bordetella pertussis, Bordetella parapertussis and Bordetella bronchiseptica. Nat. Genet. 35, 32–40. doi: 10.1038/ng1227
Pereira, S. F., Goss, L., Dworkin, J. (2011). Eukaryote-like serine/threonine kinases and phosphatases in bacteria. Microbiol. Mol. Biol. Rev. 75, 192–212. doi: 10.1128/MMBR.00042-10
Poon, W. W., Davis, D. E., Ha, H. T., Jonassen, T., Rather, P. N., Clarke, C. F. (2000). Identification of Escherichia coli ubiB, a gene required for the first monooxygenase step in ubiquinone biosynthesis. J. Bacteriol. 182, 5139–5146. doi: 10.1128/JB.182.18.5139-5146.2000
Ravichandran, A., Sugiyama, N., Tomita, M., Swarup, S., Ishihama, Y. (2009). Ser/Thr/Tyr phosphoproteome analysis of pathogenic and non-pathogenic Pseudomonas species. Proteomics 9, 2764–2775. doi: 10.1002/pmic.200800655
Santiago, F. S., Sanchez-Guerrero, E., Zhang, G., Zhong, L., Raftery, M. J., Khachigian, L. M. (2019). Extracellular signal-regulated kinase-1 phosphorylates early growth response-1 at serine 26. Biochem. Biophys. Res. Commun. 510, 345–351. doi: 10.1016/j.bbrc.2019.01.019
Searle, B. C. (2010). Scaffold: a bioinformatic tool for validating MS/MS-based proteomic studies. Proteomics 10, 1265–1269. doi: 10.1002/pmic.200900437
Shi, Y., Zhang, Y., Lin, S., Wang, C., Zhou, J., Peng, D., et al. (2020). dbPSP 2.0, an updated database of protein phosphorylation sites in prokaryotes. Sci. Data 7, 1–9. doi: 10.1038/s41597-020-0506-7
Spilker, T., Leber, A. L., Marcon, M. J., Newton, D. W., Darrah, R., Vandamme, P., et al. (2014). A simplified sequence-based identification scheme for Bordetella reveals several putative novel species. J. Clin. Microbiol. 52, 674–677. doi: 10.1128/JCM.02572-13
Stancik, I. A., Šestak, M. S., Ji, B., Axelson-Fisk, M., Franjevic, D., Jers, C., et al. (2018). Serine/Threonine protein kinases from bacteria, archaea and eukarya share a common evolutionary origin deeply rooted in the tree of life. J. Mol. Biol. 430, 27–32. doi: 10.1016/j.jmb.2017.11.004
Standish, A. J., Teh, M. Y., Tran, E. N. H., Doyle, M. T., Baker, P. J., Morona, R. (2016). Unprecedented abundance of protein tyrosine phosphorylation modulates Shigella flexneri virulence. J. Mol. Biol. 428, 4197–4208. doi: 10.1016/j.jmb.2016.06.016
Szklarczyk, D., Gable, A. L., Lyon, D., Junge, A., Wyder, S., Huerta-Cepas, J., et al. (2019). STRING v11: protein–protein association networks with increased coverage, supporting functional discovery in genome-wide experimental datasets. Nucleic Acids Res. 47, D607–D613. doi: 10.1093/nar/gky1131
Thalen, M., Van Den Ijssel, J., Jiskoot, W., Zomer, B., Roholl, P., De Gooijer, C., et al. (1999). Rational medium design for Bordetella pertussis: basic metabolism. J. Biotechnol. 75, 147–159. doi: 10.1016/S0168-1656(99)00155-8
Thingholm, T. E., Jensen, O. N., Robinson, P. J., Larsen, M. R. (2008). SIMAC (sequential elution from IMAC), a phosphoproteomics strategy for the rapid separation of monophosphorylated from multiply phosphorylated peptides. Mol. Cell. Proteomics 7, 661–671. doi: 10.1074/mcp.M700362-MCP200
Vincent-Maloney, N., Searle, B., Turner, M. (2011). Probabilistically assigning sites of protein modification with scaffold PTM. J. Biomol. Techniques: JBT 22, S36.
Wang, X., Paulin, F. E., Campbell, L. E., Gomez, E., O’brien, K., Morrice, N., et al. (2001). Eukaryotic initiation factor 2B: identification of multiple phosphorylation sites in the ϵ-subunit and their functions in vivo. EMBO J. 20, 4349–4359. doi: 10.1093/emboj/20.16.4349
White, K. A., Lin, S., Cotter, R. J., Raetz, C. R. (1999). A Haemophilus influenzae Gene That Encodes a Membrane Bound 3-Deoxy-d-manno-octulosonic Acid (Kdo) Kinase POSSIBLE INVOLVEMENT OF KDO PHOSPHORYLATION IN BACTERIAL VIRULENCE. J. Biol. Chem. 274, 31391–31400. doi: 10.1074/jbc.274.44.31391
World Health Organization (2010). WHO vaccine-preventable diseases: monitoring system: 2010 global summary.
Xu, Z., Octavia, S., Luu, L. D. W., Payne, M., Timms, V., Tay, C. Y., et al. (2019). Pertactin-Negative and Filamentous Hemagglutinin-Negative Bordetella pertussis, Australi–2017. Emerg. Infect. Dis. 25, 1196. doi: 10.3201/eid2506.180240
Yang, P., Humphrey, S. J., Cinghu, S., Pathania, R., Oldfield, A. J., Kumar, D., et al. (2019). Multi-omic profiling reveals dynamics of the phased progression of pluripotency. Cell Syst. 8, 427–445. e410. doi: 10.1016/j.cels.2019.03.012
Keywords: Bordetella pertussis, Bordetella, phosphoproteomics, BvgA, protein phosphorylation, Bordetella parapertussis, Bordetella bronchiseptica, serine/threonine/tyrosine kinase
Citation: Luu LDW, Zhong L, Kaur S, Raftery MJ and Lan R (2021) Comparative Phosphoproteomics of Classical Bordetellae Elucidates the Potential Role of Serine, Threonine and Tyrosine Phosphorylation in Bordetella Biology and Virulence. Front. Cell. Infect. Microbiol. 11:660280. doi: 10.3389/fcimb.2021.660280
Received: 03 February 2021; Accepted: 17 March 2021;
Published: 13 April 2021.
Edited by:
Vincenzo Scarlato, University of Bologna, ItalyReviewed by:
Maria Eugenia Rodriguez, CONICET Research and Development Center for Industrial Fermentations (CINDEFI), ArgentinaNelson da Cruz Soares, University of Sharjah, United Arab Emirates
Copyright © 2021 Luu, Zhong, Kaur, Raftery and Lan. This is an open-access article distributed under the terms of the Creative Commons Attribution License (CC BY). The use, distribution or reproduction in other forums is permitted, provided the original author(s) and the copyright owner(s) are credited and that the original publication in this journal is cited, in accordance with accepted academic practice. No use, distribution or reproduction is permitted which does not comply with these terms.
*Correspondence: Ruiting Lan, ci5sYW5AdW5zdy5lZHUuYXU=