Interactions Between Specific Immune Status of Pregnant Women and SARS-CoV-2 Infection
- 1Department of Anesthesiology, Guangdong Provincial People’s Hospital, Guangdong Academy of Medical Sciences, Guangzhou, China
- 2The Second School of Clinical Medicine, Southern Medical University, Guangzhou, China
- 3Department of Gynaecology and Obstetrics, Guangdong Provincial People’s Hospital, Guangdong Academy of Medical Sciences, Guangzhou, China
- 4Department of Cardiology, Guangdong Cardiovascular Institute, Guangzhou, China
Severe acute respiratory syndrome coronavirus 2 (SARS-CoV-2) is the pathogen responsible for the Coronavirus Disease 2019 (COVID-19) global pandemic. Because it is a new and highly contagious coronavirus, most people, especially pregnant women, lack immunity. It is therefore important to understand the interaction between why pregnant women are susceptible to SARS-CoV-2 and the specific immune systems of pregnant women. Here, we provide an overview of the changes that occur in the immune system during pregnancy, the activation and response of the immune system in pregnant women with COVID-19, adverse pregnancy outcomes in pregnant women with COVID-19, and the treatment and prevention of COVID-19 in this population.
Introduction
Coronavirus Disease 2019 (COVID-19) is an acute respiratory infectious disease caused by severe acute respiratory syndrome coronavirus 2 (SARS-CoV-2), which has become a public health emergency of international concern in recent times. According to past epidemiological data, pregnant women are significantly more susceptible to influenza viruses and have higher morbidity from these infections than non-pregnant women (Zhao et al., 2020). With further expansion of the pandemic, pregnant women have gradually been found to be susceptible to SARS-CoV-2, which is mainly related to the special immune states of women during pregnancy (Zhu et al., 2020). Although pregnant women are at an increased risk for SARS-CoV-2 infection, the majority of pregnant women with COVID-19 have mild symptoms; about one-fifth of them develop moderate or severe disease (Andrikopoulou et al., 2020; Islam et al., 2020). The results of a meta-analysis of pregnant women and COVID-19 (n=236) showed that 51% of pregnant women infected with COVID-19 had fever (vs. 91% non-pregnant patients) and 31% had cough (vs. 67% non-pregnant patients) (Gao et al., 2020). On the other hand, studies have shown COVID-19 combined with pregnancy can lead to premature delivery, fetal distress, fetal vascular perfusion, premature rupture of membranes, and other adverse pregnancy events (Chen H. et al., 2020). Although the treatments for COVID-19 are evolving, health care providers do not have specific treatments for pregnant women. Understanding the changes that occur in the immune system during pregnancy and the interaction between the immune system and COVID-19 is extremely important, because each key link is expected to become a potential target of COVID-19, and provides new methods and ideas for the treatment of COVID-19 in pregnant women.
Major Changes in the Immune System in Pregnant Women
Compared to non-pregnant women, pregnant women have a unique state of immunity. This status can affect how pregnant women respond to viral infections (Silasi et al., 2015), and SARS-CoV-2 is no exception. The main changes are summarized below (Table 1):
The CD3+/CD4+ and CD3+/CD8+ peripheral blood T lymphocyte and specific antiviral serum antibody counts were low in the pregnant patient (Chan et al., 2010). In addition to a decrease in the number of T cells in the blood during pregnancy, these cells are significantly less active when stimulated (Vazquez et al., 2015).
Transferring CD4+ T cell populations to T helper cell 2 (Th2) phenotypes during pregnancy (a response that promotes humoral rather than cellular immune responses) (Piccinni et al., 2000), reduced T helper cell 1 (Th1) reactivity may lead to reduced clearance of infected cells, increasing susceptibility to COVID-19 (Veenstra Van Nieuwenhoven et al., 2003). B cells are also affected by pregnancy, as their production is reduced during pregnancy (Medina et al., 1993).
Circulating natural killer (NK) cells decrease during pregnancy (Veenstra Van Nieuwenhoven et al., 2003). Since NK cells make up one fifth of the lymphocytes in the lung parenchyma, their role may provide a strong and extensive innate immune response to SARS-CoV-2 infection. Therefore, the decrease of NK cells is closely related to viral infection (Bozzano et al., 2021)..
Dendritic cells (DCs) are antigen-presenting cells that play a critical role in antiviral immunity (Liu, 2005). DCs are divided into two major types known as plasmacytoid cells (pDCs) and myeloid cells (mDCs) (Merad et al., 2013). Most studies have shown that the proportion of mDC/pDC is higher in pregnant women than in non-pregnant women (Darmochwal-Kolarz et al., 2003; Shin et al., 2009). During pregnancy, the production of interferon alpha (IFN-α) increases due to stimulation by toll-like receptors (TLRs), while the occurrence frequency of pDC decreases slightly, which may reduce the initiation of adaptive antiviral immune responses (Cordeau et al., 2012).
The phagocytic function of neutrophils and monocytes was significantly reduced in pregnant compared to non-pregnant women (Lampé et al., 2015).
The levels of interferon-γ (IFN-γ), vascular endothelial growth factor (VEGF), interleukin (IL)-1α, IL-1β, IL-6, IL-12, IL-17, IL-2, TNF-α (tumor necrosis factor α), and chemokines were decreased (Elenkov et al., 2001; Dashraath et al., 2020). On the contrary, IL-4, IL-10, IL-13, and transforming growth factor-β (TGF-β) levels were increased (Elenkov et al., 2001; Dashraath et al., 2020). Their changes during pregnancy are associated with a reduction in symptoms found in patients with COVID-19 (Pazos et al., 2012; Dashraath et al., 2020).
Circulating progesterone (P4) and steroid 17β-estradiol (E2) levels are elevated during pregnancy. The anti-inflammatory effects of E2 in innate immunity include inhibition of the production of pro-inflammatory cytokines such as IL-6 and IL-1β, tumor necrosis factor-α, monocytes, and macrophages which is a major factor in the COVID-19 cytokine storm (Chen R. et al., 2021) and powerful inhibition of monocyte chemoattractant protein-1 (MCP-1), thereby preventing innate immune cells, especially neutrophils and monocytes, from migrating to inflammatory areas. E2 stimulates CD4+ T helper cells to produce anti-inflammatory cytokines such as interleukin-4 (IL-4) and IL-10. In general, high E2 concentrations favor helper T cell type 2 (Th2) anti-inflammatory responses. E2 reduces production of interleukin-17 (IL-17) by pro-inflammatory T helper cell 17 (Th17 cells). E2 enhances the expansion of regulatory T cells (Treg), thereby promoting immune tolerance. E2 also stimulates the production of antibodies by B cells (Straub, 2007). P4 facilitates the CD4+ T-helper cell response from Th1 toward Th2 and the production of anti-inflammatory IL-4 and IL-10 cytokines (Szekeres-Bartho et al., 1996; Szekeres-Bartho and Wegmann, 1996).
Activation and Response of the Immune System in Pregnant Women With COVID-19
Th immune status of pregnant women with COVID-19 is unique which makes it easier for the virus to invade, and the rate of SARS-CoV-2 infection is higher in pregnant women than non-pregnant women. However, after SARS-CoV-2 invades a pregnant woman, her immune system confers a certain protective effect, and the serious consequences caused by the virus are likely reduced (Wei et al., 2020). Pregnant women hospitalized for COVID-19 are less likely to develop symptoms than non-pregnant women of similar age (Allotey et al., 2020) (Figure 1).
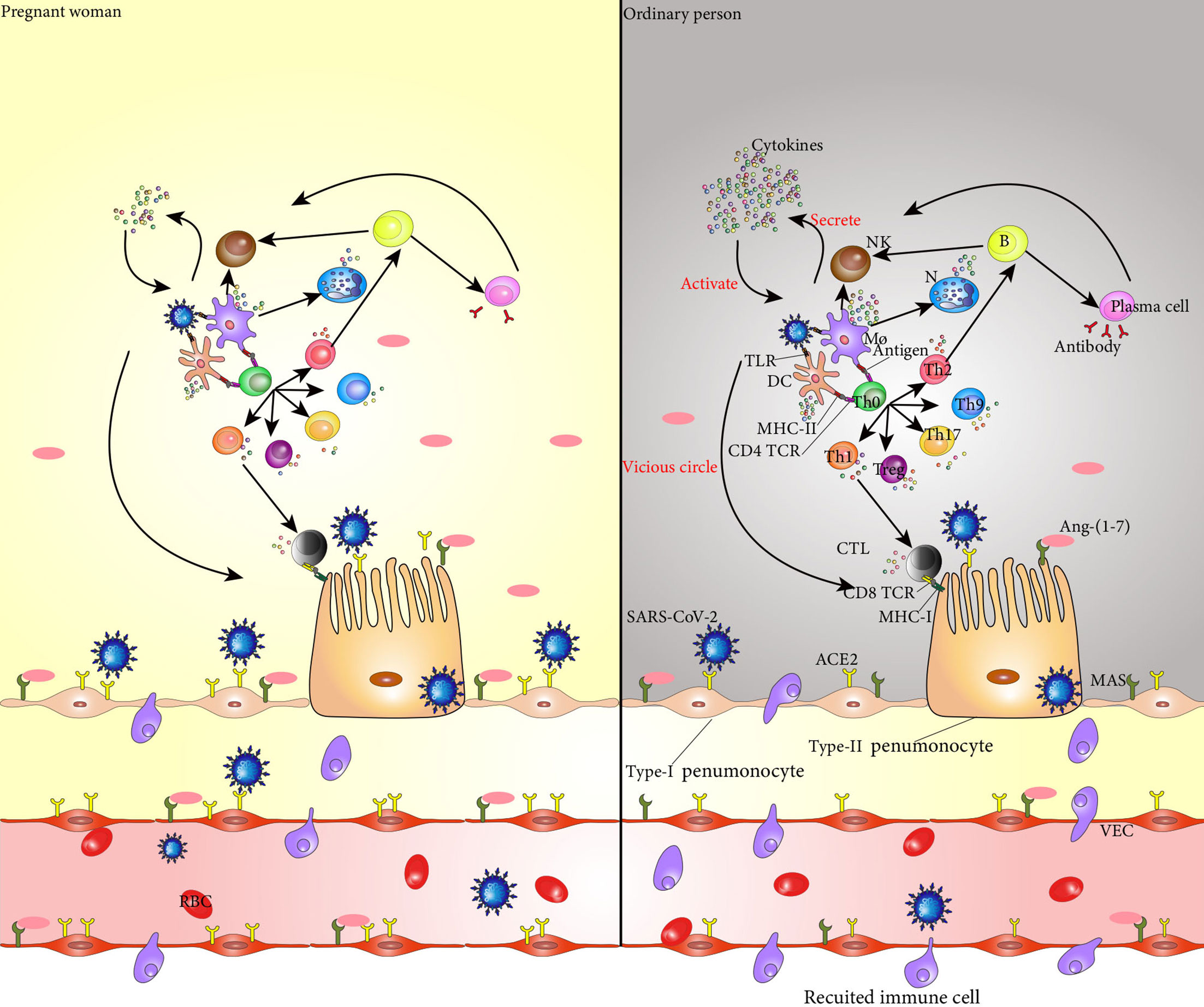
Figure 1 SARS-CoV-2 binds to ACE2 in the alveolar epithelial cells and invades these cells. Entry of SARS-CoV-2 into the body activates dendritic cells and macrophages, which secrete cytokines to activate NK cells. These NK cells kill the target cells infected by SARS-CoV-2. TLRs on dendritic cells and macrophages recognize viruses and secrete cytokines that mediate the inflammatory response. The CD4 TCR on the surface of Th0 cell membrane specifically binds to the antigen-peptide MHC II molecular complex on the surface of dendritic cells and macrophages, promoting the differentiation of Th0 cells into Th1, Th2, Treg, Th9, and Th17 cells, and the cytokines secreted by these cells promote the inflammatory response. Th1 cells secrete cytokines to activate CTL, and CD8 TCR on the surface of CTL recognizes and binds to MHC-I on the surface of cells infected with SARS-CoV-2 to kill target cells. Th2 cells secrete cytokines that assist the activation of B cells, which are activated into plasma cells that produce neutralizing antibodies to clear SARS-CoV-2. At the same time, B cells secrete cytokines to promote the killing activity of NK cells. The cytokines secreted by each immune cell in the immune response act on the immune cells themselves and promote their secretion of cytokines, forming a vicious cycle, finally resulting in a cytokine storm and aggravating the inflammatory response. In addition, Ang 1-7 produced by hydrolysis of ACE2 specifically binds to the PI3K/Akt and ERK signaling pathways regulated by MAS receptors on the cell surface, and plays an anti-inflammatory and cellular protective role. The increase of ACE2 levels in pregnant women makes invasion by SARS-CoV-2 easier; however, at the same time, the level of Ang 1-7 also increases, and the protective effect on the alveolar surface epithelial cells is stronger than that of non-pregnant women. During pregnancy, the immune system changes, and the cytokines secreted by dendritic cells and macrophages are reduced, and the activation of Th0 cells and NK cells is weakened, resulting in less cytokine. SARS-CoV-2, severe acute respiratory syndrome coronavirus 2; NK cell, natural killer cell; TLR, Toll-like receptors; Th0 cell, T helper cell 0; Th1 cell, T helper cell 2; Th2 cell, T helper cell 2; Treg, regulatory cells; Th9 cell, T helper cell 9; Th17 cell, T helper cell 17; CTL, cytotoxic T lymphocyte; MHC-I, major histocompatibility complex-I; Ang-(1-7), angiotensin-(1-7); ACE2, angiotensin-converting enzyme 2; PI3K, phosphoinositide 3-kinase; ERK, extracellularsignal-regulated kinase;.
The angiotensin-converting enzyme 2 (ACE2) is a key receptor for coronavirus invasion. Similar to the way other coronaviruses invade the body, studies have shown that SARS-CoV-2 uses the spike glycoprotein (S) proteins to bind to its receptors on target cells (Wan et al., 2020; Zhou et al., 2020). ACE2 expression and activity are enhanced during pregnancy (Levy et al., 2008). Compared with non-pregnant women, pregnant women showed a two-fold increase in ACE2 receptor expression in different organs including the placenta, kidneys, and uterus (Brosnihan et al., 2004). This automatically makes pregnant women more susceptible to SARS-CoV-2 than non-pregnant women.
SARS-CoV-2 infects alveolar epithelial cells by recognizing the ACE2 receptor. Following cell invasion, the virus replicates in large quantities, which activates immune cells. Interestingly, ACE2 has a lung-protective effect (Ziegler et al., 2020), and elevated ACE2 levels in pregnant women make their lungs more protective than those of non-pregnant women, possibly reducing lung inflammation. In addition, angiopoietin 1-7 (Ang 1-7) produced by ACE2 degradation of Ang II was higher in pregnant women than in non-pregnant women, and the anti-inflammatory effect of phosphoinositide 3-kinase (PI3K)/Akt and extracellular signal-regulated kinase (ERK) signaling pathways regulated by MAS receptors specifically bound to Ang 1-7 was also stronger than that in non-pregnant women (Passos-Silva et al., 2013). This results in less severe COVID-19 symptoms in pregnant women than in non-pregnant women, fewer cytokine storms, and less progression towards acute respiratory distress syndrome (ARDS), multiple organ dysfunction, and death.
The pattern recognition receptors (PRRs) expressed by lung epithelial cells, macrophages, and DCs bind to damage-associated molecular patterns (DAMPs) produced by virus-infected epithelial cells and pathogen-associated molecular patterns (PAMPs) of the virus itself, which then activate nuclear factor-k-gene binding (NF-kB) and several mitogen-activated protein kinases (MAPKs), triggering a cascade of inflammatory responses and the release of chemokines. Inflammation is less severe in pregnant women and cytokine storms are less likely to occur. This is mainly caused by the following mechanisms. A physiological shift in pregnant women to a TH2 environment conducive to the expression of anti-inflammatory cytokines (IL-4 and IL-10). The levels of IFN-γ, VEGF, IL-1α, IL-1β, IL-6, IL-12, IL-17, and chemokines were decreased in pregnant woman, so the cascade of inflammatory cytokines was weaker than that of non-pregnant women. DCs activated by SARS-CoV-2 release IFN and IL-7 to promote immune inflammatory response and they trigger a direct antiviral response and manipulate the activation of NK cells and CTL (Hassanzadeh-Kiabi et al., 2017; Jamilloux et al., 2020). During pregnancy, the occurrence frequency of pDC decrease. Thus, DC-mediated inflammatory response was reduced in pregnant women. The phagocytic function of neutrophils and monocytes is significantly reduced during pregnancy and also releases fewer cytokines. NK cells help clear virus-infected cells through a variety of mechanisms including direct contact, cytokine or chemokine secretion, and indirect influence on lateral and downstream adaptive immune responses by affecting dendritic cells and T cells (Moretta, 2002; Mavilio et al., 2006; Vivier et al., 2011). The decrease of NK cells in pregnant women results in a significantly reduced inflammatory response in the body. The anti-inflammatory effects of E2 and P4 also play a role in reducing inflammatory response. As a result, the expression of pro-inflammatory and anti-inflammatory cytokines was relatively low in pregnant women with COVID-19. In particular, macrophage chemokines, referred to as major components of the cytokine storm during COVID-19 (Jafarzadeh et al., 2020), including macrophage inflammatory protein 1-alpha (MIP1α), CTACK, RANTES, eotaxin, growth-related oncogene alpha (GRO-α), and TNF were found at significantly low levels in pregnant patients. At the same time, basic fibroblast growth factor (FGF), leukemia inhibitory factor (LIF), granulocyte colony-stimulating factor (G-CSF), platelet-derived growth factor subunit B (PDGF-BB), and other growth factors were also significantly lower in COVID-19-positive pregnant women than pregnant women without COVID-19 (Chen G. et al., 2021).
CD4+ and CD8+ T cells not only eliminate the virus, but also stimulate hypermacrophage syndrome, eventually causing a cytokine storm in SARS-CoV-2 patients (Wang et al., 2020). The CD3+/CD4+ and CD3+/CD8+ peripheral blood T lymphocytes were low, which will reduce clearance of infected cells and the appearance of cytokine storm. Patients with COVID-19 show both a B cell immune response and a follicle-assisted T cell response (Thevarajan et al., 2020). In addition to producing antibodies, activated B cells also secrete IL-1, IL-6, IL-8, TNF, lymphotoxin-α (LT-A), G-CSF, granulocyte-macrophage colony-stimulating factor (GM-CSF), macrophage-colony stimulating factor (M-CSF), IL-7, and other cytokines that can aggravate the cytokine storm (Vazquez et al., 2015). Production of B cell lymphocytes is reduced during pregnancy, which alleviate the occurrence of cytokine storm.
A reduced inflammatory response also leads to delayed clearance of the virus and increased infection rates. This can lead to adverse obstetric outcomes.
Adverse Pregnancy Outcomes due to COVID-19
Adverse obstetric outcomes of COVID-19 include miscarriage, intrauterine growth restriction, premature rupture of membranes, intrauterine/fetal distress, preeclampsia, vertical transmission, and preterm delivery (Dashraath et al., 2020; Schwartz, 2020) (Table 2).
Endothelial cell dysfunction is the core mechanism that leads to preeclampsia in pregnant women (Burton et al., 2019). Endothelial dysfunction after SARS-CoV-2 infection is the key to progression of COVID-19, so pregnant women infected with COVID-19 are at an increased risk of developing preeclampsia (Bonaventura et al., 2021).
The delicate balance between Treg and Th17 cells mediates the mother’s tolerance to the fetus (Aluvihare et al., 2004; Lee J. H. et al., 2011). In patients with COVID-19, the level of Treg cells (CD3+CD4+CD25+ CD127low+) decreases (Wang et al., 2020; Qin et al., 2020), the level of Th17 cells (CCR6+ Th17) increases, and the ratio of Treg/Th17 cells decreases (Wu and Yang, 2020). Reduced Treg cell count and increased Th17 cell percentage are associated with pregnancy complications such as miscarriage, preeclampsia, and preterm delivery (Sasaki et al., 2007; Xiong et al., 2010; Fu et al., 2014; Koucký et al., 2014; Eghbal-Fard et al., 2019).
IFN-γ, TNF, IL-6, and IL-8 levels were significantly increased in COVID-19 patients (Huang et al., 2020), causing pregnant women with COVID-19 to be more prone to miscarriage and premature rupture of membranes. The secretion of TNF-α, IL-8, and IL-6 by macrophages in the process of inflammation can lead to abortion, premature rupture of membranes, and preterm labor (Lee S. Y. et al., 2011; Roncari et al., 2013; Li et al., 2015; Li et al., 2020).
SARS-CoV-2 not only binds to ACE2 but also causes it to be downregulated, so Ang 1-7) also decreases (Glowacka et al., 2010). Preeclampsia is associated with reduced plasma Ang 1-7) levels in the mother (Roncari et al., 2013). COVID-19 increases the risk of preeclampsia in pregnant women.
Patients with COVID-19 have a higher incidence of thromboembolic complications (Knight et al., 2020), and healthy pregnant women have higher levels of circulating clotting and fibrinolytic factors (such as fibrinolytic enzyme) (Di Renzo and Giardina, 2020). Alterations in clotting and fibrinolysis are thought to play an important role in the pathogenesis of preeclampsia (Schjetlein et al., 1997). Pregnant women with COVID-19 may have additive or co-operative risk factors for the onset of preeclampsia.
Studies have shown that that although vertical transmission of SARS-CoV-2 in utero is low, it is possible and appears to occur in a minority of pregnant women with COVID-19 in the third trimester (Fenizia et al., 2020; Kotlyar et al., 2021).
Treatment
There are several treatments that hold promise for preventing and treating pregnant women with COVID-19 (Table 3).
Bamlanivimab and etesevimab are recombinant human immunoglobulin G1 antibodies, which is a therapy that has proven to be more effective. A randomized clinical trial showed the treatment to be effective (Gottlieb et al., 2021). Phase III data on bamlanivimab plus etesevimab in COVID-19 patients showed that among non-hospitalized patients with mild-to-moderate COVID-19 disease, bamlanivimab plus etesevimab led to a lower incidence of COVID-19-related hospitalization and death than the placebo and accelerated the decline in the SARS-CoV-2 viral load (Dougan et al., 2021). The study population included high-risk groups such as immunosuppressed states, chronic diseases, immunodeficiency, and obesity. All these patients benefited from the treatment. Although there is still no data showing that it is safe for pregnant women, we believe this is a very promising treatment.
Torzumab is an IL-6 receptor antagonist and a meta-analysis of torzumab use and COVID-19 inpatient mortality showed that torzumab reduced all-cause mortality in COVID-19 inpatients within 28 days (Shankar-Hari et al., 2021). In addition, studies have shown that torzumab is effective in patients with severe COVID-19 during pregnancy and is a treatment option for COVID-19 during pregnancy (Naqvi et al., 2020).
There is some evidence that IFN can show anti-SARS-CoV-2 effect (Busnadiego et al., 2020; Monk et al., 2021), especially as inhaled nebulized interferon beta-1a (SNG001). Studies have shown that none of the patients who received IFN during pregnancy experienced stillbirth or delivered babies with severe malformations, and IFN did not significantly increase the risk of malformation, miscarriage, stillbirth, or preterm birth. Therefore, IFN is likely effective and safe in terms of COVID-19 during pregnancy (Yazdani Brojeni et al., 2012).
Remdesivir, a novel broad-spectrum antiviral nucleotide prodrug, has been shown to inhibit the replication of SARS coronavirus in vitro. Published results have suggested that the use of remdesivir may reduce clinical recovery time in patients with COVID-19 (Beigel et al., 2020). The manufacturer safety data of remdesivir indicate no reproductive developmental toxicity in animals at clinically relevant doses; furthermore, embryonic toxicity was only noted when systemically toxic doses were administered to female animals before conception (Maldarelli et al., 2020). Studies of the use of remdesivir did not document specific adverse outcomes in pregnant women (Mulangu et al., 2019; Burwick et al., 2020). These data provide patients and clinicians with reliable information about the safety of remdesivir in the treatment of pregnant women with COVID-19. Remdesivir is expected to be beneficial for COVID-19 therapy.
Dexamethasone is a common anti-inflammatory drug. It is known that glucocorticoids inhibit inflammation through non-genetic mechanisms, such as binding to glucocorticoid receptors on the T-cell membrane, resulting in disorder of receptor signals and immune response, and interaction with calcium and sodium across the cell membrane, leading to rapid resolution of inflammation (Langarizadeh et al., 2021). Hence, it may play a vital role in the treatment of COVID-19. The administration of prenatal corticosteroids to women at risk of preterm birth has been shown to have significant benefits in neonatal morbidity and mortality (Kemp et al., 2016), which make it reasonable for COVID-19 patients to use dexamethasone during pregnancy.
There is evidence for pregnant women with COVID-19 that appropriate use of magnesium sulfate, aspirin, metformin, and anticoagulants can help cure the disease and reduce adverse outcomes such as preeclampsia, premature birth, and miscarriage (Chen X. et al., 2020; D'Souza et al., 2021).
Vitamin D is an immunomodulatory hormone that has been established to be effective against a variety of upper respiratory tract infections. Vitamin D prevents excessive inflammatory response and accelerates the healing process in affected areas, primarily in lung tissue (Mohan et al., 2020). Moreover, Treg/Th17 imbalance can be corrected with vitamin D supplementation in vivo (Ji et al., 2019). The imbalance of Treg/Th17 in COVID-19 patients not only leads to uncontrolled cytokine release and increased inflammatory response in COVID-19 patients but also leads to adverse obstetric outcomes in pregnant patients. Vitamin D therefore holds promise as a complementary treatment.
High-dose inhaled nitric oxide (160–200 ppm) has shown antimicrobial effects against bacteria and viruses (including SARS-CoV), and is used as an adjunct treatment for ARDS and pulmonary hypertension. A case series of pregnant patients with severe COVID-19 treated with high-dose nitric oxide demonstrated improvement in hypoxemia and tachypnea with no adverse neonatal effects (Safaee Fakhr et al., 2020). Hence, it also holds promise as a complementary treatment.
We wish to highlight the need for close interdepartmental collaboration in caring for pregnant women presenting with COVID-19.
Prevention
For the influenza virus, studies have shown significant reductions in low birth weight and preterm birth in vaccinated women compared to unvaccinated women (Heath et al., 2020), while other studies have not shown any association between vaccination and spontaneous abortion, preterm birth, birth defects, or small gestational age babies. Vaccination is one of the most promising preventive measures against COVID-19. Because pregnant women are more susceptible to SARS-CoV-2, vaccination during pregnancy is the most optimal strategy for preventing maternal and neonatal disease (Andreani et al., 2020; Heath et al., 2020). A preliminary finding of mRNA safety of COVID-19 vaccine in pregnant women showed that vaccination is safe in the third trimester of pregnancy (Shimabukuro et al., 2021). In addition to vaccination protecting women against COVID-19 and its complications during pregnancy, emerging evidence has shown transplacental transfer of SARS-CoV-2 antibodies after maternal COVID-19 vaccination during the third trimester, which suggests that maternal vaccination might provide some level of protection to the neonate (Gill and Jones, 2021; Gray et al., 2021; Rottenstreich et al., 2021). To date, there is still little information on the efficacy and safety of vaccinating pregnant women. More extensive research is required regarding vaccinating pregnant women.
Studies (Cheng et al., 2021) have shown that wearing a surgical mask can be a very cost-effective method to prevent the spread of virus. Because the vaccine does not provide 100% protection against COVID-19, mask-wearing is especially important for pregnant women who are more vulnerable to infection.
Conclusion
COVID-19-related immune system changes in pregnant women involve multiple cytokines, cells, and receptors. The unique immune status of pregnant women makes them more susceptible to SARS-CoV-2 invasion. However, after SARS-CoV-2 invades a pregnant woman, the immune system of the body has a certain protective effect, which reduces the serious consequences caused by the virus. However, changes in the immune system caused by the virus can also lead to poor pregnancy outcomes. Therefore, it is of great significance to accurately identify COVID-19 inflammatory pathways and therapeutic targets in pregnant women. The efficacy of the current COVID-19 treatment for pregnant women is still not satisfactory. Many clinical studies of drugs and vaccines have excluded pregnant women. The treatment of pregnant women has become a challenging problem, and more studies are needed to address these issues.
Author Contributions
HS and HY conceived and designed the study. RC, SZ, and SS performed the literature search and drafted the manuscript. All authors contributed to the article and approved the submitted version.
Funding
This project was supported by Medical Affairs Department and Scientific Research department of Guangdong Provincial People’s Hospital and the Scientific Research Initial Funding of Guangdong Provincial People’s Hospital awarded to HS (KJ012019529).
Conflict of Interest
The authors declare that the research was conducted in the absence of any commercial or financial relationships that could be construed as a potential conflict of interest.
Publisher’s Note
All claims expressed in this article are solely those of the authors and do not necessarily represent those of their affiliated organizations, or those of the publisher, the editors and the reviewers. Any product that may be evaluated in this article, or claim that may be made by its manufacturer, is not guaranteed or endorsed by the publisher.
References
Allotey, J., Stallings, E., Bonet, M., Yap, M., Chatterjee, S., Kew, T., et al. (2020). Clinical Manifestations, Risk Factors, and Maternal and Perinatal Outcomes of Coronavirus Disease 2019 in Pregnancy: Living Systematic Review and Meta-Analysis. BMJ (Clinical Res. ed.) 370, m3320. doi: 10.1136/bmj.m3320
Alspach, E., Lussier, D. M., Schreiber, R. D. (2019). Interferon γ and Its Important Roles in Promoting and Inhibiting Spontaneous and Therapeutic Cancer Immunity. Csh Perspect. Biol. 11 (3), a028480. doi: 10.1101/cshperspect.a028480
Aluvihare, V. R., Kallikourdis, M., Betz, A. G. (2004). Regulatory T Cells Mediate Maternal Tolerance to the Fetus. Nat. Immunol. 5 (3), 266–271. doi: 10.1038/ni1037
Amatya, N., Garg, A. V., Gaffen, S. L. (2017). IL-17 Signaling: The Yin and the Yang. Trends Immunol. 38 (5), 310–322. doi: 10.1016/j.it.2017.01.006
Andreani, J., Le Bideau, M., Duflot, I., Jardot, P., Rolland, C., Boxberger, M., et al. (2020). In Vitro Testing of Combined Hydroxychloroquine and Azithromycin on SARS-CoV-2 Shows Synergistic Effect. Microb. Pathogenesis 145, 104228. doi: 10.1016/j.micpath.2020.104228
Andrikopoulou, M., Madden, N., Wen, T., Aubey, J., Aziz, J., Aleha, C. D., et al. (2020). Symptoms and Critical Illness Among Obstetric Patients With Coronavirus Disease 2019 (COVID-19) Infection. Obstet. Gynecol. 136 (2), 291–299. doi: 10.1097/AOG.0000000000003996
Beigel, J. H., Tomashek, K. M., Dodd, L. E., Mehta, A. K., Zingman, B. S., Kalil, A. C., et al. (2020). Remdesivir for the Treatment of Covid-19 - Final Report. New Engl. J. Med. 383 (19), 1813–1826. doi: 10.1056/NEJMoa2007764
Bonaventura, A., Vecchié, A., Dagna, L., Martinod, K., Dixon, D. L., Tassell, B. W. V., et al. (2021). Endothelial Dysfunction and Immunothrombosis as Key Pathogenic Mechanisms in COVID-19. Nat. Rev. Immunol. 21 (5), 319–329. doi: 10.1038/s41577-021-00536-9
Bozzano, F., Dentone, C., Perrone, C., Biagio, A. D., Fenoglio, D., Parodi, A., et al. (2021). Extensive Activation, Tissue Trafficking, Turnover and Functional Impairment of NK Cells in COVID-19 Patients at Disease Onset Associates With Subsequent Disease Severity. PloS Pathog. 17 (4), e1009448. doi: 10.1371/journal.ppat.1009448
Brenner, D., Blaser, H., Mak, T. W. (2015). Regulation of Tumour Necrosis Factor Signalling: Live or Let Die. Nat. Rev. Immunol. 15 (6), 362–374. doi: 10.1038/nri3834
Brosnihan, K. B., Neves, L. A., Anton, L., Joyner, J., Valdes, G., Merrill, D. C. (2004). Enhanced Expression of Ang-(1-7) During Pregnancy. Braz. J. Med. Biol. Res. = Rev. Bras. Pesquisas Medicas e Biologicas. 37 (8), 1255–1262. doi: 10.1590/S0100-879X2004000800017
Burton, G. J., Redman, C. W., Roberts, J. M., Moffett, A. (2019). Pre-Eclampsia: Pathophysiology and Clinical Implications. BMJ (Clinical Res. ed.) 366, l2381. doi: 10.1136/bmj.l2381
Burwick, R. M., Yawetz, S., Stephenson, K. E., Collier, A. Y., Sen, P., Blackburn, B. G., et al. (2020). Compassionate Use of Remdesivir in Pregnant Women With Severe Covid-19. Clin. Infect. Dis. an Off. Publ. Infect. Dis. Soc. A. 1–9. doi: 10.1093/cid/ciaa1466
Busnadiego, I., Fernbach, S., Pohl, M. O., Karakus, U., Huber, M., Trkola, A, et al. (2020). Antiviral Activity of Type I, II, and III Interferons Counterbalances ACE2 Inducibility and Restricts SARS-CoV-2. Mbio 11 (5), e01928-20. doi: 10.1128/mBio.01928-20
Chan, K. H., Zhang, A. J., To, K. K., Chan, C. C. S., Poon, V. K. K., Guo, K., et al. (2010). Wild Type and Mutant 2009 Pandemic Influenza A (H1N1) Viruses Cause More Severe Disease and Higher Mortality in Pregnant BALB/c Mice. PloS One 5 (10), e13757. doi: 10.1371/journal.pone.0013757
Cheng, Y., Ma, N., Witt, C., Rapp, S., Wild, P. S., Andreae, M. O., et al. (2021). Face Masks Effectively Limit the Probability of SARS-CoV-2 Transmission. Sci. (New York N.Y.) 372 (6549), 1439–1443. doi: 10.1126/science.abg6296
Chen, X., Guo, H., Qiu, L., Zhang, C., Deng, Q., Leng, Q. (2020). Immunomodulatory and Antiviral Activity of Metformin and Its Potential Implications in Treating Coronavirus Disease 2019 and Lung Injury. Front. Immunol. 11, 2056. doi: 10.3389/fimmu.2020.02056
Chen, H., Guo, J., Wang, C., Luo, F., Yu, X., Zhang, W., et al. (2020). Clinical Characteristics and Intrauterine Vertical Transmission Potential of COVID-19 Infection in Nine Pregnant Women: A Retrospective Review of Medical Records. Lancet (London England) 395 (10226), 809–815. doi: 10.1016/S0140-6736(20)30360-3
Chen, R., Lan, Z., Ye, J., Pang, L., Liu, Y., Wu, W., et al. (2021). Cytokine Storm: The Primary Determinant for the Pathophysiological Evolution of COVID-19 Deterioration. Front. Immunol. 12, 589095. doi: 10.3389/fimmu.2021.589095
Chen, G., Liao, Q., Ai, J., Yang, B., Bai, H., Chen, J., et al. (2021). Immune Response to COVID-19 During Pregnancy. Front. Immunol. 12, 675476. doi: 10.3389/fimmu.2021.675476
Chen, J., Zhao, Y., Chu, X., Lu, Y., Wang, S., Yi, Q. (2016). Dectin-1-Activated Dendritic Cells: A Potent Th9 Cell Inducer for Tumor Immunotherapy. Oncoimmunology 5 (11), e1238558. doi: 10.1080/2162402X.2016.1238558
Cordeau, M., Herblot, S., Charrier, E., Audibert, F., Cordeiro, P., Harnois, M., et al. (2012). Defects in CD54 and CD86 Up-Regulation by Plasmacytoid Dendritic Cells During Pregnancy. Immunol. Invest. 41 (5), 497–506. doi: 10.3109/08820139.2012.682243
D'Souza, R., Ashraf, R., Rowe, H., Zipursky, J., Clarfield, L., Maxwell, C., et al. (2021). Pregnancy and COVID-19: Pharmacologic Considerations. Ultrasound Obstet. Gynecol. Off. J. Int. Soc. Ultrasound Obstet. Gynecol. 57 (2), 195–203. doi: 10.1002/uog.23116
Darmochwal-Kolarz, D., Rolinski, J., Tabarkiewicz, J., Leszczynska-Gorzelak, B., Buczkowski, J., Wojas, K., et al. (2003). Myeloid and Lymphoid Dendritic Cells in Normal Pregnancy and Pre-Eclampsia. Clin. Exp. Immunol. 132 (2), 339–344. doi: 10.1046/j.1365-2249.2003.02136.x
Dashraath, P., Wong, J., Lim, M., Lim, L., Li, S., Biswas, A., et al. (2020). Coronavirus Disease 2019 (COVID-19) Pandemic and Pregnancy. Am. J. Obstet. Gynecol. 222 (6), 521–531. doi: 10.1016/j.ajog.2020.03.021
Di Renzo, G. C., Giardina, I. (2020). Coronavirus Disease 2019 in Pregnancy: Consider Thromboembolic Disorders and Thromboprophylaxis. Am. J. Obstet. Gynecol. 223 (1), 135. doi: 10.1016/j.ajog.2020.04.017
Dougan, M., Nirula, A., Azizad, M., Mocherla, B., Gottlieb, R. L., Chen, P., et al. (2021). Bamlanivimab Plus Etesevimab in Mild or Moderate Covid-19. New Engl. J. Med. 2102685. doi: 10.1056/NEJMoa2102685
Eghbal-Fard, S., Yousefi, M., Heydarlou, H., Ahmadi, M., Taghavi, S., Movasaghpour, A., et al. (2019). The Imbalance of Th17/Treg Axis Involved in the Pathogenesis of Preeclampsia. J. Cell Physiol. 234 (4), 5106–5116. doi: 10.1002/jcp.27315
Elenkov, I. J., Wilder, R. L., Bakalov, V. K., Link, A. A., Dimitrov, M. A., Fisher, S., et al. (2001). IL-12, TNF-Alpha, and Hormonal Changes During Late Pregnancy and Early Postpartum: Implications for Autoimmune Disease Activity During These Times. J. Clin. Endocrinol. Metab. 86 (10), 4933–4938. doi: 10.1210/jcem.86.10.7905
Fenizia, C., Biasin, M., Cetin, I., Vergani, P., Mileto, D., Spinillo, A., et al. (2020). Analysis of SARS-CoV-2 Vertical Transmission During Pregnancy. Nat. Commun. 11 (1), 5128. doi: 10.1038/s41467-020-18933-4
Franke, F., Kirchenbaum, G. A., Kuerten, S., Lehmann, P. V. (2020). IL-21 in Conjunction With Anti-CD40 and IL-4 Constitutes a Potent Polyclonal B Cell Stimulator for Monitoring Antigen-Specific Memory B Cells. Cells-Basel 9 (2), 433. doi: 10.3390/cells9020433
Furue, K., Ito, T., Tsuji, G., Ulzii, D., Vu, Y. H., Kido-Nakahara, M., et al. (2019). The IL-13-OVOL1-FLG Axis in Atopic Dermatitis. Immunology 158 (4), 281–286. doi: 10.1111/imm.13120
Fu, B., Tian, Z., Wei, H. (2014). TH17 Cells in Human Recurrent Pregnancy Loss and Pre-Eclampsia. Cell Mol. Immunol. 11 (6), 564–570. doi: 10.1038/cmi.2014.54
Gao, Y. J., Ye, L., Zhang, J. S., Yin, Y. X., Liu, M., Yu, H. B., et al. (2020). Clinical Features and Outcomes of Pregnant Women With COVID-19: A Systematic Review and Meta-Analysis. BMC Infect. Dis. 20 (1), 564. doi: 10.1186/s12879-020-05274-2
Gill, L., Jones, C. W. (2021). Severe Acute Respiratory Syndrome Coronavirus 2 (SARS-CoV-2) Antibodies in Neonatal Cord Blood After Vaccination in Pregnancy. Obstet. Gynecol. 137 (5), 894–896. doi: 10.1097/AOG.0000000000004367
Glowacka, I., Bertram, S., Herzog, P., Pfefferle, S., Steffen, I., Muench, M. O., et al. (2010). Differential Downregulation of ACE2 by the Spike Proteins of Severe Acute Respiratory Syndrome Coronavirus and Human Coronavirus NL63. J. Virol. 84 (2), 1198–1205. doi: 10.1128/JVI.01248-09
Gobert, M., Lafaille, J. J. (2012). Maternal-Fetal Immune Tolerance, Block by Block. Cell 150 (1), 7–9. doi: 10.1016/j.cell.2012.06.020
Gottlieb, R. L., Nirula, A., Chen, P., Boscia, J., Heller, B., Morris, J., et al. (2021). Effect of Bamlanivimab as Monotherapy or in Combination With Etesevimab on Viral Load in Patients With Mild to Moderate COVID-19: A Randomized Clinical Trial. JAMA 325 (7), 632–644. doi: 10.1001/jama.2021.0202
Gray, K. J., Bordt, E. A., Atyeo, C., Deriso, E., Akinwunmi, B., Young, N., et al. (2021). COVID-19 Vaccine Response in Pregnant and Lactating Women: A Cohort Study. medRxiv Preprint Server Health Sci. doi: 10.1101/2021.03.07.21253094
Grunwell, J. R., Stephenson, S. T., Tirouvanziam, R., Brown, L., Brown, M. R., Fitzpatrick, A. M. (2019). Children With Neutrophil-Predominant Severe Asthma Have Proinflammatory Neutrophils With Enhanced Survival and Impaired Clearance. J. Allergy Clin. Immunol. In Pract. 7 (2), 516–525. doi: 10.1016/j.jaip.2018.08.024
Guilliams, M., Mildner, A., Yona, S. (2018). Developmental and Functional Heterogeneity of Monocytes. Immunity 49 (4), 595–613. doi: 10.1016/j.immuni.2018.10.005
Haep, L., Britzen-Laurent, N., Weber, T. G., Naschberger, E., Schaefer, A., Kremmer, E., et al. (2015). Interferon Gamma Counteracts the Angiogenic Switch and Induces Vascular Permeability in Dextran Sulfate Sodium Colitis in Mice. Inflammation Bowel Dis. 21 (10), 2360–2371. doi: 10.1097/MIB.0000000000000490
Hart, P. H., Bonder, C. S., Balogh, J., Dickensheets, H. L., Donnelly, R. P., Finlay-Jones, J. J. (1999). Differential Responses of Human Monocytes and Macrophages to IL-4 and IL-13. J. Leukocyte Biol. 66 (4), 575–578. doi: 10.1002/jlb.66.4.575
Hassanzadeh-Kiabi, N., Yáñez, A., Dang, I., Martins, G. A., Underhill, D. M., Goodridge, H. S. (2017). Autocrine Type I IFN Signaling in Dendritic Cells Stimulated With Fungal β-Glucans or Lipopolysaccharide Promotes CD8 T Cell Activation. J. Immunol. (Baltimore Md. 1950) 198 (1), 375–382. doi: 10.4049/jimmunol.1601143
Heath, P. T., Le Doare, K., Khalil, A. (2020). Inclusion of Pregnant Women in COVID-19 Vaccine Development. Lancet Infect. Diseases 20 (9), 1007–1008. doi: 10.1016/S1473-3099(20)30638-1
Hirano, T. (2021). IL-6 in Inflammation, Autoimmunity and Cancer. Int. Immunol. 33 (3), 127–148. doi: 10.1093/intimm/dxaa078
Holmes, K., Roberts, O. L., Thomas, A. M., Cross, M. J. (2007). Vascular Endothelial Growth Factor Receptor-2: Structure, Function, Intracellular Signalling and Therapeutic Inhibition. Cell Signal. 19 (10), 2003–2012. doi: 10.1016/j.cellsig.2007.05.013
Horkova, V., Drobek, A., Mueller, D., Gubser, C., Niederlova, V., Wyss, L., et al. (2020). Dynamics of the Coreceptor-LCK Interactions During T Cell Development Shape the Self-Reactivity of Peripheral CD4 and CD8 T Cells. Cell Rep. 30 (5), 1504–1514. doi: 10.1016/j.celrep.2020.01.008
Huang, C., Wang, Y., Li, X., Ren, L., Zhao, J., Hu, Y., et al. (2020). Clinical Features of Patients Infected With 2019 Novel Coronavirus in Wuhan, China. Lancet (London England) 395 (10223), 497–506. doi: 10.1016/S0140-6736(20)30183-5
Huntington, N. D., Cursons, J., Rautela, J. (2020). The Cancer-Natural Killer Cell Immunity Cycle. Nat. Rev. Cancer 20 (8), 437–454. doi: 10.1038/s41568-020-0272-z
Islam, M. M., Poly, T. N., Walther, B. A., Yang, H. C., Wang, C. W., Hsieh, W. S., et al. (2020). Clinical Characteristics and Neonatal Outcomes of Pregnant Patients With COVID-19: A Systematic Review. Front. Med. 7, 573468. doi: 10.3389/fmed.2020.573468
Jafarzadeh, A., Chauhan, P., Saha, B., Jafarzadeh, S., Nemati, M. (2020). Contribution of Monocytes and Macrophages to the Local Tissue Inflammation and Cytokine Storm in COVID-19: Lessons From SARS and MERS, and Potential Therapeutic Interventions. Life Sci. 257, 118102. doi: 10.1016/j.lfs.2020.118102
Jaillon, S., Ponzetta, A., Di Mitri, D., Santoni, A., Bonecchi, R., Mantovani, A. (2020). Neutrophil Diversity and Plasticity in Tumour Progression and Therapy. Nat. Rev. Cancer 20 (9), 485–503. doi: 10.1038/s41568-020-0281-y
Jamilloux, Y., Henry, T., Belot, A., Viel, S., Fautr, M., Jammal, T. E., et al. (2020). Should We Stimulate or Suppress Immune Responses in COVID-19? Cytokine and Anti-Cytokine Interventions. Autoimmun. Rev. 19 (7), 102567. doi: 10.1016/j.autrev.2020.102567
Ji, J., Zhai, H., Zhou, H., Song, S., Mor, G., Liao, A. (2019). The Role and Mechanism of Vitamin D-Mediated Regulation of Treg/Th17 Balance in Recurrent Pregnancy Loss. Am. J. Reprod. Immunol. (New York N.Y. 1989) 81 (6), e13112. doi: 10.1111/aji.13112
Junttila, I. S. (2018). Tuning the Cytokine Responses: An Update on Interleukin (IL)-4 and IL-13 Receptor Complexes. Front. Immunol. 9, 888. doi: 10.3389/fimmu.2018.00888
Kemp, M. W., Newnham, J. P., Challis, J. G., Jobe, A. H., Stock, S. J. (2016). The Clinical Use of Corticosteroids in Pregnancy. Hum. Reprod. Update 22 (2), 240–259. doi: 10.1093/humupd/dmv047
Kimura, A., Kishimoto, T. (2010). IL-6: Regulator of Treg/Th17 Balance. Eur. J. Immunol. 40 (7), 1830–1835. doi: 10.1002/eji.201040391
Knight, M., Bunch, K., Vousden, N., Morris, E., Simpson, N., Gale, C., et al. (2020). Characteristics and Outcomes of Pregnant Women Admitted to Hospital With Confirmed SARS-CoV-2 Infection in UK: National Population Based Cohort Study. BMJ (Clinical Res. ed.) 369, m2107. doi: 10.1136/bmj.m2107
Kotlyar, A. M., Grechukhina, O., Chen, A., Popkhadze, S., Grimshaw, A., Tal, O., et al. (2021). Vertical Transmission of Coronavirus Disease 2019: A Systematic Review and Meta-Analysis. Am. J. Obstet. Gynecol. 224 (1), 35–53. doi: 10.1016/j.ajog.2020.07.049
Koucký, M., Malíčková, K., Cindrová-Davies, T., Germanova, A., Parizek, A., Kalousova, M., et al. (2014). Low Levels of Circulating T-Regulatory Lymphocytes and Short Cervical Length are Associated With Preterm Labor. J. Reprod. Immunol. 106, 110–117. doi: 10.1016/j.jri.2014.04.001
Lamb, Y. N. (2020). Remdesivir: First Approval. Drugs 80 (13), 1355–1363. doi: 10.1007/s40265-020-01378-w
Lampé, R., Kövér, Á., Szűcs, S., Pal, L., Arnyas, E., Adany, R., et al. (2015). Phagocytic Index of Neutrophil Granulocytes and Monocytes in Healthy and Preeclamptic Pregnancy. J. Reprod. Immunol. 107, 26–30. doi: 10.1016/j.jri.2014.11.001
Langarizadeh, M. A., Ranjbar Tavakoli, M., Abiri, A., Ghasempour, A., Rezaei, M. (2021). Ameri A. A Review on Function and Side Effects of Systemic Corticosteroids Used in High-Grade COVID-19 to Prevent Cytokine Storms. Excli. J. 20, 339–365. doi: 10.17179/excli2020-3196
Laurence, A., Tato, C. M., Davidson, T. S., Kanno, Y., Chen, Z., Yao, Z., et al. (2007). Interleukin-2 Signaling via STAT5 Constrains T Helper 17 Cell Generation. Immunity 26 (3), 371–381. doi: 10.1016/j.immuni.2007.02.009
Lee, S. Y., Buhimschi, I. A., Dulay, A. T., Ali, U. A., Zhao, G., Abdel-Razeq, S. S., et al. (2011). IL-6 Trans-Signaling System in Intra-Amniotic Inflammation, Preterm Birth, and Preterm Premature Rupture of the Membranes. J. Immunol. (Baltimore Md. 1950) 186 (5), 3226–3236. doi: 10.4049/jimmunol.1003587
Lee, J. H., Ulrich, B., Cho, J., Park, J., Kim, C. H. (2011). Progesterone Promotes Differentiation of Human Cord Blood Fetal T Cells Into T Regulatory Cells But Suppresses Their Differentiation Into Th17 Cells. J. Immunol. (Baltimore Md. 1950) 187 (4), 1778–1787. doi: 10.4049/jimmunol.1003919
Levy, A., Yagil, Y., Bursztyn, M., Barkalifa, R., Scharf, S., Yagil, C. (2008). ACE2 Expression and Activity are Enhanced During Pregnancy. Am. J. Physiol. Regul. Integr. Comp. Physiol. 295 (6), R1953–R1961. doi: 10.1152/ajpregu.90592.2008
Li Jeon, N., Baskaran, H., Dertinger, S. K., Whitesides, G. M., Van de Water, L., Toner, M. (2002). Neutrophil Chemotaxis in Linear and Complex Gradients of Interleukin-8 Formed in a Microfabricated Device. Nat. Biotechnol. 20 (8), 826–830. doi: 10.1038/nbt712
Lin, Q., Zhao, L., Jing, R., Trexler, C., Wang, H., Li, Y., et al. (2019). Inositol 1,4,5-Trisphosphate Receptors in Endothelial Cells Play an Essential Role in Vasodilation and Blood Pressure Regulation. J. Am. Heart Assoc. 8 (4), e11704. doi: 10.1161/JAHA.118.011704
Li, Z. Y., Song, Z. H., Meng, C. Y., Yang, D. D., Yang, Y., Peng, J. P. (2015). IFN-γ Modulates Ly-49 Receptors on NK Cells in IFN-γ-Induced Pregnancy Failure. Sci. Rep. Uk. 5, 18159. doi: 10.1038/srep18159
Liu, Y. J. (2005). IPC: Professional Type 1 Interferon-Producing Cells and Plasmacytoid Dendritic Cell Precursors. Annu. Rev. Immunol. 23, 275–306. doi: 10.1146/annurev.immunol.23.021704.115633
Li, W., Zhao, X., Li, S., Chen, X., Cui, H., Chang, Y., et al. (2020). Upregulation of TNF-α and IL-6 Induces Preterm Premature Rupture of Membranes by Activation of ADAMTS-9 in Embryonic Membrane Cells. Life Sci. 260, 118237. doi: 10.1016/j.lfs.2020.118237
Maldarelli, G. A., Savage, M., Mazur, S., Oxford-Horrey, C., Salvatore, M., Marks, K. M. (2020). Remdesivir Treatment for Severe COVID-19 in Third-Trimester Pregnancy: Case Report and Management Discussion. Open Forum Infect. Di. 7 (9), a345. doi: 10.1093/ofid/ofaa345
Mantovani, A., Dinarello, C. A., Molgora, M., Garlanda, C. (2019). Interleukin-1 and Related Cytokines in the Regulation of Inflammation and Immunity. Immunity 50 (4), 778–795. doi: 10.1016/j.immuni.2019.03.012
Mavilio, D., Lombardo, G., Kinter, A., Fogli, M., Sala, A. L., Ortolano, S., et al. (2006). Characterization of the Defective Interaction Between a Subset of Natural Killer Cells and Dendritic Cells in HIV-1 Infection. J. Exp. Med. 203 (10), 2339–2350. doi: 10.1084/jem.20060894
Medina, K. L., Smithson, G., Kincade, P. W. (1993). Suppression of B Lymphopoiesis During Normal Pregnancy. J. Exp. Med. 178 (5), 1507–1515. doi: 10.1084/jem.178.5.1507
Merad, M., Sathe, P., Helft, J., Miller, J., Mortha, A. (2013). The Dendritic Cell Lineage: Ontogeny and Function of Dendritic Cells and Their Subsets in the Steady State and the Inflamed Setting. Annu. Rev. Immunol. 31, 563–604. doi: 10.1146/annurev-immunol-020711-074950
Mercier, O., Arthur Ataam, J., Langer, N. B., Dorfmuller, P., Lamrani, L., Lecerf, F., et al. (2017). Abnormal Pulmonary Endothelial Cells may Underlie the Enigmatic Pathogenesis of Chronic Thromboembolic Pulmonary Hypertension. J. Heart Lung Transplant. Off. Publ. Int. Soc. Heart Transpl. 36 (3), 305–314. doi: 10.1016/j.healun.2016.08.012
Mesner, D., Hotter, D., Kirchhoff, F., Jolly, C. (2020). Loss of Nef-Mediated CD3 Down-Regulation in the HIV-1 Lineage Increases Viral Infectivity and Spread. P Natl. Acad. Sci. U.S.A. 117 (13), 7382–7391. doi: 10.1073/pnas.1921135117
Mohan, M., Cherian, J. J., Sharma, A. (2020). Exploring Links Between Vitamin D Deficiency and COVID-19. PloS Pathog. 16 (9), e1008874. doi: 10.1371/journal.ppat.1008874
Monk, P. D., Marsden, R. J., Tear, V. J., Brookes, J., Batten, T. N., Mankowski, M., et al. (2021). Safety and Efficacy of Inhaled Nebulised Interferon Beta-1a (SNG001) for Treatment of SARS-CoV-2 Infection: A Randomised, Double-Blind, Placebo-Controlled, Phase 2 Trial. Lancet Respir. Med. 9 (2), 196–206. doi: 10.1016/S2213-2600(20)30511-7
Moretta, A. (2002). Natural Killer Cells and Dendritic Cells: Rendezvous in Abused Tissues. Nat. Rev. Immunol. 2 (12), 957–964. doi: 10.1038/nri956
Mulangu, S., Dodd, L. E., Davey, R. T., Mbaya, O. T., Proschan, M., Mukadi, D., et al (2019). A Randomized, Controlled Trial of Ebola Virus Disease Therapeutics. New Engl. J. Med. 381 (24), 2293–2303. doi: 10.1056/NEJMoa1910993
Naqvi, M., Zakowski, P., Glucksman, L., Smithson, S., Burwick, R. M. (2020). Tocilizumab and Remdesivir in a Pregnant Patient With Coronavirus Disease 2019 (COVID-19). Obstet. Gynecol. 136 (5), 1025–1029. doi: 10.1097/AOG.0000000000004050
Passos-Silva, D. G., Verano-Braga, T., Santos, R. A. (2013). Angiotensin-(1-7): Beyond the Cardio-Renal Actions. Clin. Sci. (London Engl. 1979). 124 (7), 443–456. doi: 10.1042/CS20120461
Patel, V. B., Zhong, J. C., Grant, M. B., Oudit, G. Y. (2016). Role of the ACE2/Angiotensin 1-7 Axis of the Renin-Angiotensin System in Heart Failure. Circ. Res. 118 (8), 1313–1326. doi: 10.1161/CIRCRESAHA.116.307708
Pazos, M., Sperling, R. S., Moran, T. M., Kraus, T. A. (2012). The Influence of Pregnancy on Systemic Immunity. Immunol. Res. 54, 254–261. doi: 10.1007/s12026-012-8303-9
Piccinni, M. P., Maggi, E., Romagnani, S. (2000). Role of Hormone-Controlled T-Cell Cytokines in the Maintenance of Pregnancy. Biochem. Soc. T. 28 (2), 212–215. doi: 10.1042/bst0280212
Qin, C., Zhou, L., Hu, Z., Zhang, S., Yang, S., Tao, Y., et al. (2020). Dysregulation of Immune Response in Patients With Coronavirus 2019 (COVID-19) in Wuhan, China. Clin. Infect. Dis. An Off. Publ. Infect. Dis. Soc. A. 71 (15), 762–768. doi: 10.1093/cid/ciaa248
Roncari, D., Politch, J. A., Sonalkar, S., Finneseth, M., Borgatta, L. (2013). Inflammation or Infection at the Time of Second Trimester Induced Abortion. Contraception 87 (1), 67–70. doi: 10.1016/j.contraception.2012.09.016
Rottenstreich, A., Zarbiv, G., Oiknine-Djian, E., Zigron, R., Wolf, D. G., Porat, S. (2021). Efficient Maternofetal Transplacental Transfer of Anti- SARS-CoV-2 Spike Antibodies After Antenatal SARS-CoV-2 BNT162b2 mRNA Vaccination. Clin. Infect. Dis. An Off. Publ. Infect. Dis. Soc. A. ciab266. doi: 10.1093/cid/ciab266
Safaee Fakhr, B., Wiegand, S. B., Pinciroli, R., Gianni, S., Morais, C. C. A., Ikeda, T., et al. (2020). High Concentrations of Nitric Oxide Inhalation Therapy in Pregnant Patients With Severe Coronavirus Disease 2019 (COVID-19). Obstet. Gynecol. 136 (6), 1109–1113. doi: 10.1097/AOG.0000000000004128
Sasaki, Y., Darmochwal-Kolarz, D., Suzuki, D., Sakai, M., Ito, M., Shima, T., et al. (2007). Proportion of Peripheral Blood and Decidual CD4(+) CD25(bright) Regulatory T Cells in Pre-Eclampsia. Clin. Exp. Immunol. 149 (1), 139–145. doi: 10.1111/j.1365-2249.2007.03397.x
Schjetlein, R., Haugen, G., Wisløff, F. (1997). Markers of Intravascular Coagulation and Fibrinolysis in Preeclampsia: Association With Intrauterine Growth Retardation. Acta Obstet. Gyn. Scan. 76 (6), 541–546. doi: 10.3109/00016349709024580
Schwartz, D. A. (2020). An Analysis of 38 Pregnant Women With COVID-19, Their Newborn Infants, and Maternal-Fetal Transmission of SARS-CoV-2: Maternal Coronavirus Infections and Pregnancy Outcomes. Arch. Pathol. Lab. Med. 144 (7), 799–805. doi: 10.5858/arpa.2020-0901-SA
Shankar-Hari, M., Vale, C. L., Godolphin, P. J., et al. (2021). Association Between Administration of IL-6 Antagonists and Mortality Among Patients Hospitalized for COVID-19: A Meta-Analysis. JAMA. doi: 10.1001/jama.2021.11330
Shimabukuro, T. T., Kim, S. Y., Myers, T. R., Moro, P. L., Oduyebo, T., Panagiotakopoulos, L., et al. (2021). Preliminary Findings of mRNA Covid-19 Vaccine Safety in Pregnant Persons. N. Engl. J. Med. 384 (24), 2273–2282. doi: 10.1056/NEJMoa2104983
Shin, S., Jang, J. Y., Roh, E. Y., Yoon, J. H., Kim, J. S., Han, K. S., et al. (2009). Differences in Circulating Dendritic Cell Subtypes in Pregnant Women, Cord Blood and Healthy Adult Women. J. Korean Med. Sci. 24 (5), 853–859. doi: 10.3346/jkms.2009.24.5.853
Silasi, M., Cardenas, I., Kwon, J. Y., Racicot, K., Aldo, P., Mor, G. (2015). Viral Infections During Pregnancy. Am. J. Reprod. Immunol. (New York N.Y. 1989) 73 (3), 199–213. doi: 10.1111/aji.12355
Straub, R. H. (2007). The Complex Role of Estrogens in Inflammation. Endocr. Rev. 28 (5), 521–574. doi: 10.1210/er.2007-0001
Szekeres-Bartho, J., Faust, Z., Varga, P., Szereday, L., Kelemen, K. (1996). The Immunological Pregnancy Protective Effect of Progesterone is Manifested via Controlling Cytokine Production. Am. J. Reprod. Immunol. (New York N.Y. 1989) 35 (4), 348–351. doi: 10.1111/j.1600-0897.1996.tb00492.x
Szekeres-Bartho, J., Wegmann, T. G. (1996). A Progesterone-Dependent Immunomodulatory Protein Alters the Th1/Th2 Balance. J. Reprod. Immunol. 31, 81–95. doi: 10.1016/0165-0378(96)00964-3
Thevarajan, I., Nguyen, T., Koutsakos, M., Druce, J., Caly, L., van de Sandt, C. E., et al. (2020). Breadth of Concomitant Immune Responses Prior to Patient Recovery: A Case Report of non-Severe COVID-19. Nat. Med. 26 (4), 453–455. doi: 10.1038/s41591-020-0819-2
Travis, M. A., Sheppard, D. (2014). TGF-β Activation and Function in Immunity. Annu. Rev. Immunol. 32, 51–82. doi: 10.1146/annurev-immunol-032713-120257
Trinchieri, G. (2003). Interleukin-12 and the Regulation of Innate Resistance and Adaptive Immunity. Nat. Rev. Immunol. 3 (2), 133–146. doi: 10.1038/nri1001
Vazquez, M. I., Catalan-Dibene, J., Zlotnik, A. (2015). B Cells Responses and Cytokine Production are Regulated by Their Immune Microenvironment. Cytokine 74 (2), 318–326. doi: 10.1016/j.cyto.2015.02.007
Veenstra Van Nieuwenhoven, A. L., Heineman, M. J., Faas, M. M. (2003). The Immunology of Successful Pregnancy. Hum. Reprod. Update 9 (4), 347–357. doi: 10.1093/humupd/dmg026
Virdis, A., Santini, F., Colucci, R., Duranti, E., Salvetti, G., Rugani, I., et al. (2011). Vascular Generation of Tumor Necrosis Factor-α Reduces Nitric Oxide Availability in Small Arteries From Visceral Fat of Obese Patients. J. Am. Coll. Cardiol. 58 (3), 238–247. doi: 10.1016/j.jacc.2011.01.050
Vivier, E., Raulet, D. H., Moretta, A., Caligiuri, M. A., Zitvogel, L., Lanier, L. L., et al. (2011). Innate or Adaptive Immunity? The Example of Natural Killer Cells. Sci. (New York N.Y.) 331 (6013), 44–49. doi: 10.1126/science.1198687
Walter, M. R. (2014). The Molecular Basis of IL-10 Function: From Receptor Structure to the Onset of Signaling. Curr. Top. Microbiol. 380, 191–212. doi: 10.1007/978-3-662-43492-5_9
Wang, F., Hou, H., Luo, Y., Tang, G., Wu, S., Huang, M., et al. (2020). The Laboratory Tests and Host Immunity of COVID-19 Patients With Different Severity of Illness. JCI Insight 5 (10), e137799. doi: 10.1172/jci.insight.137799
Wan, Y., Shang, J., Graham, R., Baric, R. S., Li, F. (2020). Receptor Recognition by the Novel Coronavirus From Wuhan: An Analysis Based on Decade-Long Structural Studies of SARS Coronavirus. J. Virol. 94 (7), e00127–20. doi: 10.1128/JVI.00127-20
Watson, J., Aarden, L. A., Shaw, J., Paetkau, V. (1979). Molecular and Quantitative Analysis of Helper T Cell-Replacing Factors on the Induction of Antigen-Sensitive B and T Lymphocytes. J. Immunol. (Baltimore Md. 1950) 122 (5), 1633–1638.
Wei, L., Gao, X., Chen, S., Zeng, W., Wu, J., Lin, X., et al. (2020). Clinical Characteristics and Outcomes of Childbearing-Age Women With COVID-19 in Wuhan: Retrospective, Single-Center Study. J. Med. Internet Res. 22 (8), e19642. doi: 10.2196/19642
Wilson, E. B., Livingstone, A. M. (2008). Cutting Edge: CD4+ T Cell-Derived IL-2 is Essential for Help-Dependent Primary CD8+ T Cell Responses. J. Immunol. (Baltimore Md. 1950) 181 (11), 7445–7448. doi: 10.4049/jimmunol.181.11.7445
Wu, D., Yang, X. O. (2020). TH17 Responses in Cytokine Storm of COVID-19: An Emerging Target of JAK2 Inhibitor Fedratinib. J. Microbiol. Immunol. Infect. = Wei mian yu gan ran za zhi 53 (3), 368–370. doi: 10.1016/j.jmii.2020.03.005
Xiong, H., Zhou, C., Qi, G. (2010). Proportional Changes of CD4+CD25+Foxp3+ Regulatory T Cells in Maternal Peripheral Blood During Pregnancy and Labor at Term and Preterm. Clin. Invest. Med. Medecine clinique experimentale 33 (6), E422. doi: 10.25011/cim.v33i6.14594
Yang, L., Wang, J., Hui, P., Yarovinsky, T. O., Badeti, S., Pham, K., et al. (2021). Potential Role of IFN-α in COVID-19 Patients and its Underlying Treatment Options. Appl. Microbiol. Biot. 105 (10), 4005–4015. doi: 10.1007/s00253-021-11319-6
Yazdani Brojeni, P., Matok, I., Garcia Bournissen, F., Koren, G. (2012). A Systematic Review of the Fetal Safety of Interferon Alpha. Reprod. Toxicol. (Elmsford N.Y.) 33 (3), 265–268. doi: 10.1016/j.reprotox.2011.11.003
Zhang, Y., Liu, Z., Tian, M., Hu, X., Wang, L., Ji, J., et al. (2018). The Altered PD-1/PD-L1 Pathway Delivers the 'One-Two Punch' Effects to Promote the Treg/Th17 Imbalance in Pre-Eclampsia. Cell Mol. Immunol. 15 (7), 710–723. doi: 10.1038/cmi.2017.70
Zhao, X., Jiang, Y., Zhao, Y., Xi, H., Liu, C., Qu, F., et al. (2020). Analysis of the Susceptibility to COVID-19 in Pregnancy and Recommendations on Potential Drug Screening. Eur. J. Clin. Microbiol. Infect. Dis. Off. Publ. Eur. Soc. Clin. Microbiol. 39 (7), 1209–1220. doi: 10.1007/s10096-020-03897-6
Zhou, P., Yang, X. L., Wang, X. G., Hu, B., Zhang, L., Zhang, W., et al. (2020). A Pneumonia Outbreak Associated With a New Coronavirus of Probable Bat Origin. Nature 579 (7798), 270–273. doi: 10.1038/s41586-020-2012-7
Zhu, H., Wang, L., Fang, C., Peng, S., Zhang, L., Chang, G., et al. (2020). Clinical Analysis of 10 Neonates Born to Mothers With 2019-Ncov Pneumonia. Trans. Pediatr. 9 (1), 51–60. doi: 10.21037/tp.2020.02.06
Ziegler, C., Allon, S. J., Nyquist, S. K., Mbano, I. M., Miao, V. N., Tzouanas, C. N., et al. (2020). SARS-CoV-2 Receptor ACE2 Is an Interferon-Stimulated Gene in Human Airway Epithelial Cells and Is Detected in Specific Cell Subsets Across Tissues. Cell 181 (5), 1016–1035. doi: 10.1016/j.cell.2020.04.035
Keywords: SARS-CoV-2, COVID-19, pregnant women, immune system, treatment
Citation: Chen R, Zhang S, Su S, Ye H and Shu H (2021) Interactions Between Specific Immune Status of Pregnant Women and SARS-CoV-2 Infection. Front. Cell. Infect. Microbiol. 11:721309. doi: 10.3389/fcimb.2021.721309
Received: 06 June 2021; Accepted: 29 July 2021;
Published: 12 August 2021.
Edited by:
Karen E Racicot, Michigan State University, United StatesReviewed by:
Sonia Zuñiga, Centro Nacional de Biotecnología (CSIC), SpainJian Chen, Fudan University, China
Copyright © 2021 Chen, Zhang, Su, Ye and Shu. This is an open-access article distributed under the terms of the Creative Commons Attribution License (CC BY). The use, distribution or reproduction in other forums is permitted, provided the original author(s) and the copyright owner(s) are credited and that the original publication in this journal is cited, in accordance with accepted academic practice. No use, distribution or reproduction is permitted which does not comply with these terms.
*Correspondence: Haihua Shu, shuhaihua@hotmail.com; Haiyan Ye, leafboat1998@126.com
†These authors share first authorship