Enterochromaffin Cells: Sentinels to Gut Microbiota in Hyperalgesia?
- 1Department of Anesthesiology, Tongji Hospital, Tongji Medical College, Huazhong University of Science and Technology, Wuhan, China
- 2Department of Anesthesiology, Huashan Hospital, Fudan University, Shanghai, China
In recent years, increasing studies have been conducted on the mechanism of gut microbiota in neuropsychiatric diseases and non-neuropsychiatric diseases. The academic community has also recognized the existence of the microbiota-gut-brain axis. Chronic pain has always been an urgent difficulty for human beings, which often causes anxiety, depression, and other mental symptoms, seriously affecting people’s quality of life. Hyperalgesia is one of the main adverse reactions of chronic pain. The mechanism of gut microbiota in hyperalgesia has been extensively studied, providing a new target for pain treatment. Enterochromaffin cells, as the chief sentinel for sensing gut microbiota and its metabolites, can play an important role in the interaction between the gut microbiota and hyperalgesia through paracrine or neural pathways. Therefore, this systematic review describes the role of gut microbiota in the pathological mechanism of hyperalgesia, learns about the role of enterochromaffin cell receptors and secretions in hyperalgesia, and provides a new strategy for pain treatment by targeting enterochromaffin cells through restoring disturbed gut microbiota or supplementing probiotics.
Introduction
Hyperalgesia refers to a decreased pain threshold and an increased response to harmful stimuli, which seriously affects patients’ health and quality of life (Nugraha et al., 2019). The central mechanisms of hyperalgesia mainly include glutamate/NMDA (N-methyl-D-aspartic acid) receptor-mediated sensitization, de-inhibition of inhibitory interneurons, and activation of microglia (Basbaum et al., 2009). Peripheral mechanisms include tissue damage and persistent inflammation (Treede et al., 1992). At present, many studies have proved that gut microbiota is involved in the peripheral regulation mechanism of chronic pain, and it is not only confined to visceral pain but also hyperalgesia induced by neuropathic and metabolic diseases (Pellegrini et al., 2018; Yang et al., 2019).
Diverse and stable gut microbiota is vital to the health of the host. The gut microbiota begins to establish soon after the host is born and is continuously affected by external factors, such as antibiotics, diet, stress, etc. The gut microbiota is a dynamic ecosystem that maintains a bidirectional connection with the host through the microbe-gut-organ axis and actively responds to various physiological and pathological conditions. Among them, studies on the mechanism of the microbe-gut-brain axis in neuropsychiatric diseases, including chronic pain and cognition, have attracted the most attention (Pellegrini et al., 2018; Zhan et al., 2018). Gut microbiota can affect the occurrence of visceral pain, inflammatory pain, neuropathic pain, headache, and opioid tolerance through peripheral and central mechanisms (Guo et al., 2019). Their regulatory role in chronic pain has opened up a new idea for pain treatment by restoring healthy gut microbiota (Xu et al., 2021).
The intestinal epithelium is composed of epithelial absorption cells, goblet cells, paneth cells, and enteroendocrine cells. Enterochromaffin cells (ECs) are the most abundant subtype of intestinal enteroendocrine cells in the colon, which can be directly contacted by gut microbiota on the side of the intestinal lumen and interact with the afferent and efferent nerve endings located in the lamina propria by synaptic connection. Although its accounts for less than 1% of intestinal epithelial cells, it can produce and release 90% of the serotonin in the body, which is essential for intestinal motility, platelet function, immune response, and bone development (Karsenty and Yadav, 2011; Matthes and Bader, 2018). In addition, ECs are electroexcitatory, similar to other primary sensory cells, expressing functional voltage-gated Na+ and Ca2+ channels (Bellono et al., 2017). These important characteristics enable ECs to act as bidirectional information transmitters among the intestinal lumen, intestinal epithelial cells, and specific primary afferent nerve fibers. In brief, ECs are considered the fundamental cells of microbiota-gut-brain interaction in cognition, depression, and chronic pain (Rhee et al., 2009).
Gut Microbiota and Hyperalgesia
Gut microbiota has been recognized as one of the key pain regulators, which can directly or indirectly mediate pain tolerance or sensitization through immune, metabolic, endocrine, and neural signaling pathways. Numerous clinical or pre-clinical studies on chronic pain-related diseases have reported changes in gut microbiota (Braundmeier-Fleming et al., 2016; Clos-Garcia et al., 2019). For example, the diversity of the gut microbiota in patients with fibromyalgia was reduced, and the abundance of Bifidobacterium and Eubacterium genera was significantly reduced (Clos-Garcia et al., 2019). Eggerthella sinensis, Colinsella aerofaciens, Faecalibacterium prasunitzii, Odoribacter splanchnicus, and Lactonifactor longoviformis decreased in patients with bladder pain syndrome (Braundmeier-Fleming et al., 2016). In addition, a large cohort study proved that the abundance of Streptococcus species was linked to the increase in knee joint pain (Boer et al., 2019). PubMed was searched, and we selected relevant studies before August 1st, 2021. The search keyword string included “hyperalgesia OR chronic pain” AND “gut microbiota OR intestinal flora.” To this end, 15 related studies were enrolled. The details of each study are presented in Table 1.
In studies related to gut microbiota, the use of antibiotics to construct pseudo-sterile mouse models is a conventional intervention (Yang et al., 2019; Zhang et al., 2019). The common measuring method of behavioral pain response included: mechanical sensitivity by von Frey method, cold sensitivity by cold plantar assay, heat sensitivity by Hargreaves test, or tail-flick test. The imbalance of the gut microbiota caused by antibiotics also impacts the occurrence of pain. However, the effects of antibiotic-induced changes in the gut microbiota on pain have been inconsistent in numerous studies (Shen et al., 2017; Yang et al., 2019). It has been suggested that the lack of gut microbiota may be a protective mechanism in terms of hyperalgesia for the host. Compared with wild mice, inflammatory stimuli, such as carrageenan, lipopolysaccharide (LPS), tumor necrosis factor (TNF)- alpha, interleukin (IL)-1beta, and chemokine (C-X-C motif) ligand 1 (CXCL1), cause relatively less noxious reactions in germ-free (GF) mice, while recolonization of the gut microbiota or administration of IL-10 antagonists can reverse this phenomenon in GF mice (Amaral et al., 2008). Similarly, mechanical hyperalgesia in neuropathic pain induced by chemotherapy drugs (paclitaxel, oxaliplatin) and chronic compressive nerve injury pain was reduced in GF mice or antibiotic-treated mice, while recovery of the gut microbiota can disrupt this protective effect and promote chemotherapy-induced mechanical hyperalgesia (Shen et al., 2017; Ramakrishna et al., 2019; Ding et al., 2021). Conversely, some studies have concluded that the interference of antibiotic administration leads to the imbalance of the gut microbiota in mice, which can induce mechanical tenderness and spontaneous pain, accompanied by anxiety, depression-like behaviors, and spatial memory impairment (Aguilera et al., 2015; Yang et al., 2019). Transplanting the gut microbiota from spared nerve injury model rats susceptible to anhedonia into antibiotic treatment rats further aggravated chronic pain and depression-like phenotypes (Yang et al., 2019). In addition, in mice with dysbacteriosis, the cannabinoid receptor (CB) 2 level was upregulated, while the CB1 and μ opioid receptors expressions were down-regulated (Aguilera et al., 2013; Aguilera et al., 2015), and large amounts of IL-10 could be produced (Souza et al., 2004), affecting the pain response. Although there are different opinions about the effect of gut microbiota imbalance on chronic pain, which may involve differences in the antibiotic compatibility, detection time, dosage, and even animal models, these experimental results are sufficient to prove that the symbiotic gut microbiota affects the production of hyperalgesia in the host.
Access to effective bacteria or prebiotics is one of the crucial research goals in treating various systemic diseases, and chronic pain is no exception. Lactobacillus paracasei and Bifidobacterium infantis 35624 can normalize pain threshold (Verdú et al., 2006; McKernan et al., 2010). Taking Lactobacillus acidophilus NCFM strain can induce the expression of μ opioid receptor and CB2 in intestinal epithelial cells, mediating the analgesic effects similar to morphine (Rousseaux et al., 2007). Intestinal microbial exopolysaccharides (EPSs) have been shown to play an active role in antioxidant, blood pressure and blood glucose regulation, apoptosis, and autophagy of cancer cell lines (Maeda et al., 2004; Di et al., 2017; Tang et al., 2017). Intraperitoneal injection of probiotic strain Lactobacillus paraplantarum BGCG11 (EPS CG11) producing high molecular weight EPSs can significantly reduce the mechanical hyperalgesia of inflammatory pain in Wistar rats by decreasing the expression of pro-inflammatory factors IL-1β and inducible nitric oxide synthase (iNOS) (Dinić et al., 2018) (Table 2)
In addition, the imbalance of gut microbiota can lead to structural and metabolic changes in the chronic pain-related brain areas. Anterior cingulate cortex (ACC) volume was decreased, periaqueductal grey volume was increased, and single nerve cells in the ACC also appeared noticeable dendrite changes in pseudo-sterile mice with visceral sensitization (Luczynski et al., 2017). The number of C-Fos immunoreactive neurons in the prefrontal cortex and hippocampus was decreased, while in ACC and insular cortex was increased in mice with dysbacteriosis (Wang et al., 2021a). The amygdala also plays a role in mood disorders, nerve regeneration diseases, and chronic pain. In particular, humans have labeled the laterocapsular division of the central nucleus as “pain-sensitive amygdala.” The gut microbiota can affect the morphology, activity, functional connection, and gene expression of the amygdala through the vagus nerve [including the enteric nervous system (ENS)], spinal cord transmission (especially visceral pain), regulation of tryptophan metabolism, and immune regulation (Cowan et al., 2018). The activation of microglia is also associated with the development of chronic pain. The microglia in the brain mainly initiate neuronal apoptosis, clearing dead cells and pruning synapses. However, microglia in antibiotic treatment or GF mice show immature and malformed phenotypes with significantly longer processes and increased numbers of segments, branching, and terminal points. In addition, mice deficient for the short-chain fatty acids (SCFAs) receptor, free fatty acid receptor (FFAR) 2, also showed an immature state of microglia in pseudo-sterile mice, indicating that the presence of gut microbiota is critical for the development, homeostasis, and functional status of microglia in the central nervous system (Erny et al., 2015). A considerable part of the metabolites in mammalian blood is derived from gut microbiota, and changes in the gut microbiota will also affect brain metabolites (Wikoff et al., 2009). Furthermore, GF mice were found to have lower tryptophan (a precursor of 5-HT), tyrosine (a precursor of dopamine and norepinephrine), and glutamine in the brain than mice rich in gut microbiota, in addition to lower energy production and consumption through glycolysis and the tricarboxylic acid cycle (Matsumoto et al., 2013). In GF mice, the blood-brain barrier (BBB) permeability was increased, and the expressions of tight junction proteins such as occludin and claudin-5 in the frontal cortex, striatum, hippocampus were decreased (Braniste et al., 2014). While Clostridium tyrobutyricum or Bacteroides thetaiotaomicron treatment can increase tight junction protein expression and restore BBB permeability in GF mice, which indicated that the gut microbiota was contributed to maintaining the integrity of the BBB, and the destruction of BBB caused by gut microbiota imbalance provided a structural basis for harmful intestinal substances to affect the host brain function (Braniste et al., 2014). The above suggested that the gut microbiota is inextricably linked to the host’s hyperalgesia (Table 3).
Gut Microbiota Medium or Metabolites and Hyperalgesia
The role of mediators or metabolites derived from gut microbiota in hyperalgesia is well known. Gut microbiota can release nervous system factors involved in the regulation of gut brain-axis communication, such as 5-HT released by genera Candida, Streptococcus, Escherichia and Enterococcus, dopamine or norepinephrine produced by genera Escherichia, Bacillus and Saccharomyces, acetylcholine generated by genus Lactobacillus, γ-aminobutyric acid produced by genera Lactobacillus and Bifidobacterium (Holzer and Farzi, 2014). Moreover, gut microbiota-derived mediators can activate Toll-like receptors (TLRs), GABA receptors, and transient receptor potentials (TRP), and so on, participating in the regulation of chronic pain (Santoni et al., 2021).
Pathogen-associated molecular patterns (PAMPs) derived from gut microbiota were significant contributors to peripheral sensitization under chronic pain conditions. PAMPs obtained from gut microbiota included LPS, flagellin, N-formyl peptides, lipoteichoic acid, peptidoglycan, β-glucan, etc. (Chiu, 2018). They can directly sensitize the primary neurons in the dorsal root ganglia or indirectly by activating immune cells to promote the release of cytokines and chemokines, mediating peripheral sensitization of pain. LPS can send signals to nociceptive dorsal root ganglion neurons in the colon of mice (Ochoa-Cortes et al., 2010). Bacterial flagella can be recognized by the host TLR5 to play a host defenses role (Hayashi et al., 2001) and block the sensitization of dorsal root ganglion A fiber sensory neurons, inhibiting the mechanical hyperalgesia caused by chemotherapy, nerve injury, and diabetic neuropathy (Xu et al., 2015). N-formyl peptide bound to the formyl peptide receptors on the host nociceptive dorsal root ganglia to induce mechanical hyperalgesia (Chiu et al., 2013).
In addition to PAMPs, gut microbiota metabolites are also involved in pain regulation. Microbiota-derived metabolites include SCFAs, bile acids, indole derivatives, vitamins, polyamines, and lipids (Hosseinkhani et al., 2021). Gut microbiota was considered the main producer of SCFAs, providing SCFAs to the host by decomposing fermented starch and dietary fiber. SCFAs included volatile fatty acids fermentation products such as isovaleric acid, isobutyric acid, and butyric acid. Several receptors of SCFAs have been identified as G-protein coupled receptor (GPR) 41/FFAR3, GPR43/FFAR2, GPR109A, and olfactory receptor (Olfr) 78, which mediate leukocyte recruitment, chemokine production, intestinal wall permeability, and BBB permeability changes (Braniste et al., 2014; Ohira et al., 2017). In chronic constriction injury, obesity-induced peripheral neuropathy pain, rheumatoid arthritis pain, changes in SCFAs and butyrate (a histone deacetylase inhibitor) for pain relief were shown. The mechanism involved alleviating the polarization of pro-inflammatory microglia in the spinal cord and hippocampus (Zhou et al., 2021) and increasing the serotonin metabolite 5-hydroxyindole-3-acetic acid, which in turn activated the aryl-hydrocarbon receptor and inhibited inflammation and pain in a Breg cell-dependent manner (Rosser et al., 2020), changing in the immune cell population of the peripheral nervous system (Bonomo et al., 2020).
The Interaction of Hyperalgesia-Related Receptors in ECs With Intestinal Flora and Its Metabolites
ECs, as intestinal epithelial chemoreceptors, can detect gastrointestinal symbiotic bacteria, infectious microorganisms, food intake, endogenous regulatory substances, etc. It has been found that allyl isothiocyanate, isovalerate, isobutyrate, butyrate, catecholamines, and son on can specifically and continuously activate ECs, trigger Ca2+ transients, and participate in a variety of pathophysiological states (Bellono et al., 2017). From the perspective of chronic pain-related receptors of ECs, the possible hyperalgesia targets of ECs interacting with gut microbiota are discussed below.
TRP Family
The TRP family is a kind of non-selective transmembrane cation channels superfamily, which can be divided into seven subfamilies according to different amino acid sequences: TRPC (canonical), TRPV (vanilloid), TRPM (melastatin), TRPP (polycystin), TRPML (mucolipin), TRPA (ankyrin) and TRPN (NOMPC like, or no mechanoreceptor potential C). They maintain the transmembrane transport of cell Na+, Ca2+ and Mg2+, and intracellular organelle homeostasis, participating in various pathophysiological processes such as neurodegenerative diseases and gastrointestinal peristalsis (Nilius and Owsianik, 2011; Blackshaw, 2014; Nilius and Szallasi, 2014). Among them, at least TRPV1-4, TRPM8 and TRPA1 are expressed in nociceptive sensory neurons, which transfer thermal, chemical, and mechanical stimulation signals, playing an important role in the occurrence and development of pathological pain perception (Dai, 2016). TRPV1 (Deng et al., 2021), TRPV5 and 6 (Hua et al., 2019), TRPM7 (Lv et al., 2020) and 8 (Wen et al., 2020), TRPA1 (Pagano et al., 2019), TRPP1 (Beer et al., 2019) have all been found to have some associations with the gut microbiota, but so far only the TRPA1 receptor has been studied in ECs (Nozawa et al., 2009).
TRPA1 is a chemoreceptor widely expressed in humans and animals, including dorsal root ganglia, bladder, gastrointestinal tract, skin, respiratory tract, blood vessels, etc. It helps to sense pain, temperature (<17°C), mechanical stimulation, and chemical stimulants. It has become a target for the development of analgesic and anti-inflammatory drugs. Its functional mutations were considered one of the pathogeneses of familial paroxysmal pain syndrome (Kremeyer et al., 2010). TRPA1 receptor is activated by allyl isothiocyanate, cinnamaldehyde, organic sulfur compounds in garlic and onions, and smoke bombs (Bautista et al., 2006). In the gastrointestinal tract, the TRPA1 receptor is mainly expressed in the visceral afferent nerves, which can sense exogenous dietary stimuli such as mustard and garlic and endogenous inflammatory factors such as prostaglandins and other lipid-derived metabolites (Logashina et al., 2019). As the main detector of luminal irritants on the intestinal mucosa, the TRPA1 receptor is necessary for the normal mechanical and chemical sensory functions of specific subsets of the vagus, viscera, and pelvic afferent nerve (Brierley et al., 2009). It has been demonstrated that TRPA1 is a molecular target of LPS, a toxic substance produced by bacterial lysis, that directly acts on nociceptive sensory neurons. This finding provides new insight into the mechanism of hyperalgesia during bacterial infection (Meseguer et al., 2014). IL-33 can be expressed and released by damaged tissues or necrotic barrier cells to participate in intestinal infections and type II immune responses. The IL-33/ST2 signaling pathway is an important signal to activate the dorsal horn ganglion and induce pain and itch (Han et al., 2013). IL-13/ST2 signaling pathway can mediate the rapid release of 5-HT from ECs dependent on the TRPA1 receptor and then cause the corresponding symptoms of 5-HT dysregulation (Chen et al., 2021b). In addition, cinnamaldehyde can also stimulate the QGP-1 cells of the human ECs model to release 5-HT in a dose-dependent manner by activating TRPA1 (Lieder et al., 2020).
Piezo1/2
Since piezo1/2 was identified in mammalian cells in 2010 (Coste et al., 2010), the research focus of piezo1/2 has expanded from its structure to activation mechanism and its role in physiology and pathology (Kim et al., 2012; Guo et al., 2021). Piezo1/2 is a new type of mechanical ion channel that can be expressed in neurons, endothelial cells, red blood cells, etc. It acts as a multi-functional mechanical sensor in the bladder, colon, kidney, lung, and skin. Mechanical stimulus signals, such as tension and pulsation, are converted into electrochemical signals, which play an important role in blood vessel development, bone formation, and somatosensory conduction (Ranade et al., 2014a; Ranade et al., 2014b; Syeda et al., 2016; Jiang et al., 2021). Piezo1 is predominantly expressed in non-sensory tissues, while piezo2 is mainly expressed in sensory tissues. Some early studies focused on the role of piezo1/2 in the trigeminal nervous system (Fernández-Trillo et al., 2020; Dolgorukova et al., 2021). For example, IL-6 can cause trigeminal neuralgia by activating piezo2 (Liu et al., 2021a). In addition, activating piezo1 receptor on trigeminal nerve nociceptive fibers can trigger the release of calcitonin gene -related peptide, a key mediator of migraine (Mikhailov et al., 2019). The mechanisms of epac1-piezo2 axis in bone cancer pain, inflammatory pain, and mechanical allodynia of neuropathic pain have also been investigated (Eijkelkamp et al., 2013; Singhmar et al., 2016; Ni et al., 2021). Piezo1/2 also exists in intestinal epithelial cells, and the expression level in the colon is higher than that in the small intestine. Piezo2 in the colon is significantly negatively correlated with the visceral sensitivity of irritable bowel syndrome (IBS) (Bai et al., 2017). Selectively and high expression of piezo2 in human and mouse ECs can sense mechanical stimulation and induce the release of 5-HT (Wang et al., 2017a). More interestingly, piezo1 can induce ECs to produce 5-HT by sensing the single-stranded RNA (ssRNA) of the gut microbiota (rather than protein and DNA). ssRNA-stimulated Piezo1 induced a significant calcium response to release 5-HT in a MyD88/TRIF-independent and canonical Wnt signaling-independent manner, promoting intestinal motility and reducing bone mass, etc., independently of the stimulation of mechanical intestinal peristalsis (Sugisawa et al., 2020). Although the mechanism by which ssRNA of the gut microbiota activates piezo1/2 has still not been fully explained, the groundbreaking discovery of the interaction between the gut microbiota and piezo1/2 in ECs could be a theoretical cornerstone for treating hyperalgesia with gut microbiota.
Olfactory Receptor (Olfr)
Although Olfrs are a type of GPR mainly responsible for the volatile odor signals transduction in olfactory neurons, they can be ectopically expressed in non-olfactory tissues, such as the heart, skin, lungs, and intestinal epithelium, etc., with both olfactory and non-olfactory functions (Braun et al., 2007; Chen et al., 2018). Olfr plays an important role in many physiological and pathological processes such as sensory perception, behavior and emotion regulation, immune system activity and inflammation regulation, tumor growth, and metastasis (Meijerink, 2021). Although there were few studies on its mechanism of action in hyperalgesia, the current research status suggests that it is closely related to hyperalgesia in certain diseases (Lee et al., 2019). The genome-wide association study of blood samples from breast cancer patients showed that the occurrence of pain after adjuvant radiotherapy was significantly associated with the activity of Olfr genes (OR52N1, OR4C12, OR4A47) (Lee et al., 2019). Whole-genome analysis of whole blood samples from patients with head and neck tumors showed that Olfr genes (OR13G1, OR6F1, OR14A2) were susceptibility genes for pretreatment pain (Reyes-Gibby et al., 2016). In addition, R-carvone-responsive Olfr OR1A1 has been used in a pre-clinical study to design cells controlled by peppermint aromatherapy to treat chronic pain (Wang et al., 2018). In recent years, Olfr78/OR51E2 and Olfr558/OR51E1, members of the olfactory GPR subfamily, have been identified as sensors for SCFAs and/or branched-chain fatty acids in the intestine. Olfr78, the SCFAs receptor on enteroendocrine cells, shows a specific affinity for acetic acid and propionic acid. It can promote the secretion of anorexigenic gut hormone peptide YY in mice intestinal enteroendocrine cells, regulate appetite, and maintain energy homeostasis (Nishida et al., 2021). Propionic acid can regulate blood pressure in mice by regulating renin release and vascular tension, proving a subtle relationship between gut microbial metabolites and Olfr78 on vascular smooth muscle cells in blood pressure control (Pluznick et al., 2013; Pluznick, 2014). In addition, acetic acid and propionic acid can also act on the Olfr OR51E2 (human-derived Olfr78) on airway smooth muscle cells to slow down the remodeling of cytoskeleton and the proliferation of airway smooth muscle cells, becoming specific receptors targeting the intestine-lung axis to treat asthma (Aisenberg et al., 2016). These suggest that certain specific connections between the gut microbiota with its metabolites and Olfrs play a role in the physiological and pathological processes. In addition, the intestinal odors can stimulate the release of 5-HT through the Olfrs localized at the apical side of ECs (Braun et al., 2007) to participate in the occurrence of gastrointestinal diseases such as IBS. Recently, it has been further discovered that isovaleric acid can act as a ligand for Olfr558, which can activate Gαolf/s-adenylyl cyclase signaling in ECs, induce 5-HT3 secretion involved in the occurrence of visceral sensitivity (Bellono et al., 2017). Therefore, the excavation of the mechanism of Olfrs on ECs will be more conducive to understanding the relationship between the gut microbiota and various pain-related diseases.
α2Aadrenoreceptor
Research on the role of gut microbiota and its metabolites acting through adrenergic receptors is mainly manifested in lipid metabolism and cardiovascular aspects, such as promoting platelet thrombosis (Huynh, 2020). Changes in the gut microbiota will also affect the host catecholamine hormone levels. It was found that the level of free catecholamines in the intestine of pseudo-sterile mice was lower than that of specific pathogen-free mice, and most of the catecholamines in the intestines of pseudo-sterile mice were non-biologically active conjugated forms. In contrast, catecholamines in the intestines of specific pathogen-free mice are biologically active free form (Asano et al., 2012). The levels of catecholamines in the intestines, especially norepinephrine, fluctuate with infection, inflammation, or sympathetic tone. Norepinephrine is an effective bacterial stimulator that can upregulate the proliferation, toxicity, and adhesion of bacteria. However, long-term infection and injury can cause chronic visceral sensitization. Norepinephrine can increase the growth rate, vitality, and invasion of Campylobacter jejuni, destroying the tight junctions of the intestinal epithelium (Cogan et al., 2007). Norepinephrine, exudated from the noradrenergic nerve terminal or the capillaries in the intestinal wall, can activate the adrenergic-like QseC receptor on the surface of the bacteria in the intestinal lumen, altering the virulence of the microbiota via autoinducer 3-mediated signaling pathways. Simultaneously, the autoinducer three released by gut microbiota into the intestinal lumen can activate intestinal epithelium’s adrenergic receptors, reduce intestinal epithelial cell fluid secretion, and impair the host’s ability to expel pathogens (Rhee et al., 2009). Among the adrenergic receptors, only α2A adrenergic receptors are expressed in ECs, located on the basolateral side, and receiving sympathetic excitatory stimulation. Adrα2A-TRPC4 mediates the catecholamine sensitivity of ECs through a Gαi-dependent signaling cascade (Bellono et al., 2017). The destruction of the gut microbiota induced by antibiotic vancomycin in the neonatal period resulted in visceral allergy in adult male rats, accompanied by decreased mRNA expression of α-2A adrenergic receptor and TRPV1 receptor in the lumbosacral spinal cord (O’Mahony et al., 2014), so α2A adrenergic receptors may act as a chronic pain target for gut microbiota.
Serotonin Signaling by ECs
Part of the 5-HT secreted by ECs can be used as neurotransmitters for synaptic connection with primary afferent nerve fibers. In contrast, the other part can be circulated to other tissues, including the brain, through blood circulation to participate in osteogenesis, learning, memory, emotional regulation, pain tolerance, etc. It is a crucial signal molecule in the bidirectional communication system between the brain and the gut (Crowell and Wessinger, 2007; Gershon, 2013). A variety of intestinal stimulants, such as mechanical stimulation, diet, gastric acid, bacterial metabolites, viruses, and drugs, can trigger ECs to release 5-HT to intestinal mast cells, spinal afferent nerves, and neurons in the ENS, eventually causing afferent nociceptive and mechanical sensitivity terminal receptor sensitivity, resulting in hyperalgesia (Akiba et al., 2017; Wood, 2020). Under normal physiological conditions, the release of 5-HT can stimulate the intestinal epithelium and ENS, maintain the contraction of intestinal smooth muscle, and facilitate the elimination of harmful bacteria in the intestinal lumen. However, the proliferation of ECs and increased availability of 5-HT are involved in the development of visceral sensitization and peripheral mechanical hyperalgesia in IBS (Cremon et al., 2011; Qin et al., 2019; Nascimento et al., 2021). For example, stimulating FFAR2 on ECs can enhance the defense function of the duodenal mucosa by increasing HCO3- secretion and regulating 5-HT biosynthesis. However, excessive activation of FFAR2, which drives the excessive release of 5-HT, can cause mucosal damage by reducing mucosal blood flow (Akiba et al., 2017). The presence of serotonin transporter knockouts in female mice can also exhibit visceral sensitization and gastrointestinal motility disorders, such as low pain pressure threshold and increased fecal output, accompanied by the increased relative proportion of ECs and colon 5-HT concentration (Bi et al., 2021). The mechanism by which ECs release 5-HT is also related to adenosine triphosphate (ATP) and its metabolites. ATP and its breakdown products are a purinergic transmitter in the ENS, which can initiate enteric nerve reflex or activate afferent nerve endings to transmit pain to the brain. Through BON cells or EC cells isolated from human intestinal surgery specimens, it is verified that ECs can respond to ATP, uridine triphosphate (UTP) and uridine diphosphate (UDP), mainly by activating P2X3, P2Y4R and PLC/IP3/IP3R/SERCA Ca2+ signaling pathways, to involve in visceral sensitization and pain production (Xu et al., 2008; Liñán-Rico et al., 2017). In addition, metabolites of norepinephrine and isovalerate activate ECs to release 5-HT (Bellono et al., 2017). And 5-HT can also activate TRPV4 to mediate visceral hypersensitivity through protein kinase C (PKC), phospholipase Cbeta (PLCbeta), mitogen-activated protein kinase kinase (MAPKK) and phospholipase A2 (PLA2)-dependent mechanisms (Cenac et al., 2010).
Gut Microbiota-ECs-Vagal Afferent Nerves Signaling
A large number of studies have suggested that the vagus nerve is one of the key pathways in the mechanism of the gut-brain axis (O’Mahony et al., 2011; Xu et al., 2020). The gastrointestinal tract not only contains a huge microbial ecosystem but also includes ENS composed of tens of thousands of sensory afferent neurons. The enteric vagus nerve originates from the neural crest cells of the vagus nerve and is comprised of nerve plexus embedded in the intestinal wall. Due to its autonomy, neurotransmitter diversity, and complex cell structure, ENS enjoys the “second brain” reputation (Fattahi et al., 2016). ENS is connected with the central autonomic neural network of the brain through the parasympathetic nerves and sympathetic nerves. These sympathetic and parasympathetic nerves can regulate ENS through afferent and efferent activities, thereby forming the bidirectional activity of the gut-brain axis. The intestinal vagus nerve regulates the contraction of gastrointestinal smooth muscle and the secretion of glands, innervates the gastrointestinal mucosal mechanical receptors, chemoreceptors, and tension receptors, and transmits sensations to the nucleus tractus solitarius, and then projects to the central nervous system, such as the amygdala, thalamus, and the locus coeruleus, etc. The colonization of the gut microbiota will also affect the development, excitability, and plasticity of the ENS (Collins et al., 2014; Hyland and Cryan, 2016). The excitability of endogenous primary afferent neurons was reduced, and nitrergic neurons were increased, and calbindin positive neurons and glial cells were decreased in the colon and ileum muscle of GF mice. While the colonization of normal gut microbiota can restore neurons excitability and the number of calbindin positive neurons (McVey Neufeld et al., 2013; Hyland and Cryan, 2016). In addition, colonization of Bacteroides thetaiotaomicron restored the growth of neurites and glial cells and the expression of nitric oxide synthase, substance P, and other neurotransmitters (Aktar et al., 2020). The probiotic Saccharomyces boulardii reduced the calcium-binding protein intermuscular neurons in pig jejunum (Kamm et al., 2004). Pediococcus acidilactici treatment increased galanin and calcitonin gene-related peptide-immunoreactive neurons and glial fibrillary acidic protein-positive enteric glial cells in submucosal ileal ganglion of piglets (di Giancamillo et al., 2010). All the above proved that the gut microbiota can selectively affect the growth and stability of the ENS. Hence, ECs was considered one of those mediators promoting the communication between the gut microbiota and ENS (Bohórquez et al., 2015; Yano et al., 2015). ECs releases 5-HT to regulate the growth, maintenance, and nerve reflex of the intestinal mucosa and ENS by stimulating 5-HT receptors on submucosal primary afferent neurons (Pan and Gershon, 2000; Gross et al., 2012). Clinical data showed that the increased number of ECs was present in the colonic mucosa of IBS patients, and the increased 5-HT released from the mucosa was related to the severity of discomfort such as abdominal pain. Perfusion of colonic mucosal supernatant in IBS patients resulted in significant activation of mesenteric sensory neurons, inhibited by 5-HT3 receptor antagonists, indicating that 5-HT released by ECs can affect the ENS in hyperalgesia (Cremon et al., 2011) (Figure 1).
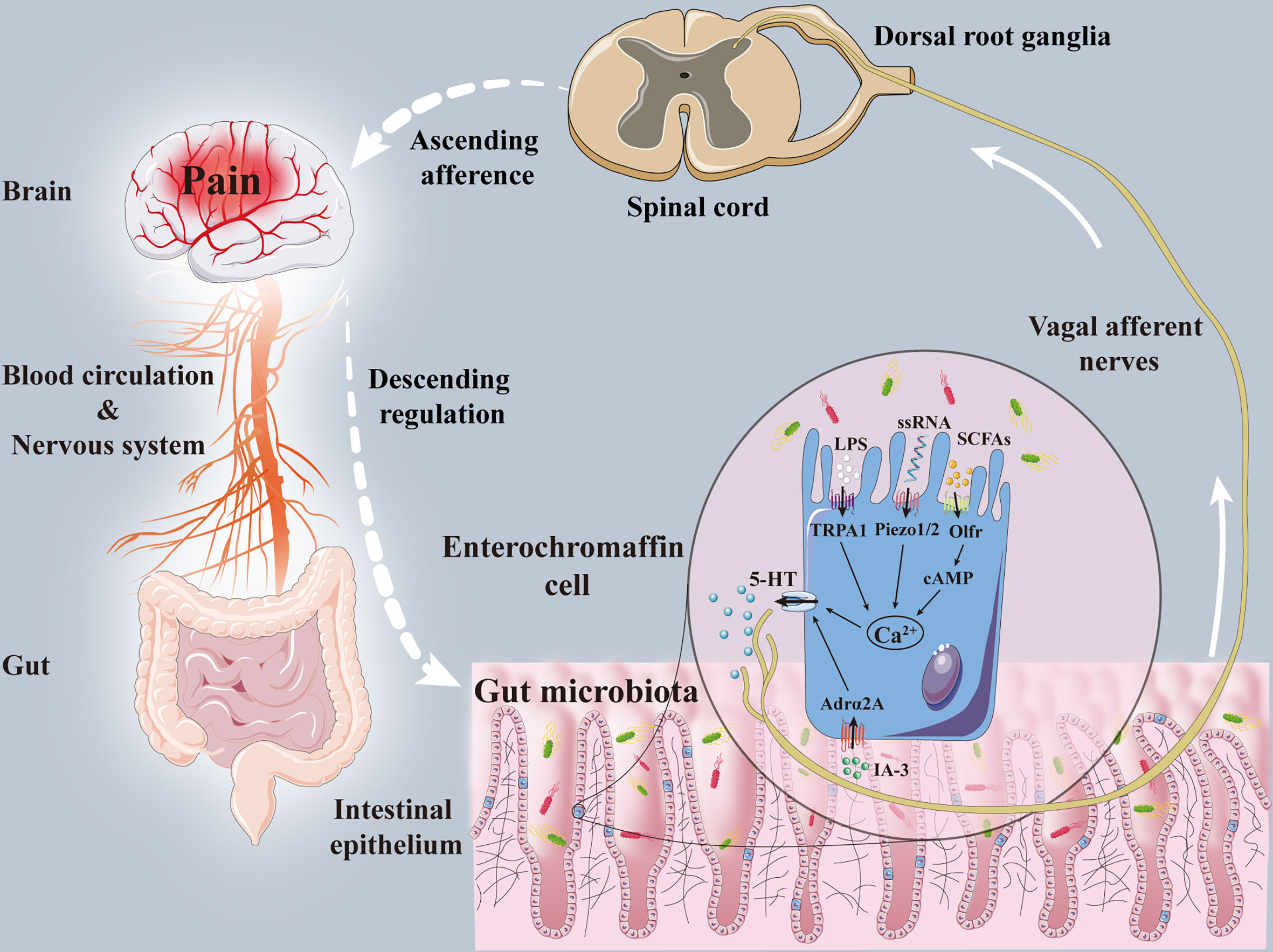
Figure 1 The mechanisms of enterochromaffin cells in the interaction between gut microbiota and pain. Gut microbiota and its metabolites, including IA-3, ssRNA, SCFAs and LPS, acted on enterochromaffin cells receptors (Adrα2A, piezo1/2, cAMP, Olfr, TRPA1) to induce the release of 5-HT. Part of 5-HT transferred hyperalgesia signals to dorsal root ganglia and spinal dorsal horn through the vagal afferent nerve. Pain signals are then transmitted to the brain through the spinal-thalamic tract and other ascending afferent tracts, and eventually integrated through the cerebral cortex and limbic system. After that, the brain can regulate pain sensation through descending regulation and neuromediators. Adrα2A, α2A adrenoreceptor; cAMP, cyclic adenosine monophosphate; IA-3, autoinducer 3; LPS, lipopolysaccharide; Olfr, olfactory receptor; SCFAs, short-chain fatty acids; ssRNA, single-stranded RNA; TRPA1, transient receptor potential A1; 5-HT, 5-hydroxytryptamine.
Throughout the gastrointestinal tract, there are other enteroendocrine cells except ECs, there are interactions between these cells and ECs, such as hormone stimulation, and so on (Yu et al., 2016). as a limitation, this review discussed only the role of ECs in hyperalgesia, ignoring the effects of other enteroendocrine cells influenced by the ECs after the disturbance of the gut microbiota. Therefore, it is necessary to further investigate the effects of enteroendocrine cells influenced by the ECs. In addition, different gut microbiota may have different effects on ECs, which need to be further studied.
Conclusion
By sensing different gut microbiota and its metabolites, ECs can activate pain-related receptors and induce the release of 5-HT to transmits pain signals to the brain through the vagus nerve. ECs become sentinels for sensing the gut microbiota to participate in the occurrence of hyperalgesia. Although the characteristics and functions of ECs in vivo still need to be further understood, the existing studies have laid a theoretical foundation for the effect of ECs receptors and 5-HT secreted by ECs on the gut microbiota and hyperalgesia. To continue to explore the underlying mechanism of ECs will be an indispensable and promising challenge in the search for pain treatment strategies in the future.
Author Contributions
XX wrote the manuscript of this review. All authors critically reviewed and approved the final version of the paper.
Funding
This study was supported by a grant from the National Natural Science Foundation of China (Grant No. 81341034).
Conflict of Interest
The authors declare that the research was conducted in the absence of any commercial or financial relationships that could be construed as a potential conflict of interest.
Publisher’s Note
All claims expressed in this article are solely those of the authors and do not necessarily represent those of their affiliated organizations, or those of the publisher, the editors and the reviewers. Any product that may be evaluated in this article, or claim that may be made by its manufacturer, is not guaranteed or endorsed by the publisher.
Abbreviations
ACC, anterior cingulate cortex; ATP, adenosine triphosphate; BBB, blood-brain barrier; CB, cannabinoid receptor; ECs, enterochromaffin cells; ENS, enteric nervous system; EPSs, exopolysaccharides; FFAR, free fatty acid receptor; GF, germ-free; GPR, G-protein coupled receptor; IBS, irritable bowel syndrome; IL, interleukin; LPS, lipopolysaccharide; Olfr, olfactory receptor; PAMPs, pathogen-associated molecular patterns; SCFAs, short-chain fatty acids; ssRNA, single-stranded RNA; TLR, Toll-like receptors; TRP, transient receptor potentials.
References
Aguilera, M., Cerdà-Cuéllar, M., Martínez, V. (2015). Antibiotic-Induced Dysbiosis Alters Host-Bacterial Interactions and Leads to Colonic Sensory and Motor Changes in Mice. Gut Microbes. 6, 10–23. doi: 10.4161/19490976.2014.990790
Aguilera, M., Vergara, P., Martínez, V. (2013). Stress and Antibiotics Alter Luminal and Wall-Adhered Microbiota and Enhance the Local Expression of Visceral Sensory-Related Systems in Mice. Neurogastroenterol. Motil. 25, e515–e529. doi: 10.1111/nmo.12154
Aisenberg, W. H., Huang, J., Zhu, W., Rajkumar, P., Cruz, R., Santhanam, L., et al. (2016). Defining an Olfactory Receptor Function in Airway Smooth Muscle Cells. Sci. Rep. 6, 38231. doi: 10.1038/srep38231
Akiba, Y., Maruta, K., Narimatsu, K., Said, H., Kaji, I., Kuri, A., et al. (2017). FFA2 Activation Combined With Ulcerogenic COX Inhibition Induces Duodenal Mucosal Injury via the 5-HT Pathway in Rats. Am. J. Physiol. Gastrointest. Liver Physiol. 313, G117–g128. doi: 10.1152/ajpgi.00041.2017
Aktar, R., Parkar, N., Stentz, R., Baumard, L., Parker, A., Goldson, A., et al. (2020). Human Resident Gut Microbe Bacteroides Thetaiotaomicron Regulates Colonic Neuronal Innervation and Neurogenic Function. Gut Microbes 11, 1745–1757. doi: 10.1080/19490976.2020.1766936
Amaral, F. A., Sachs, D., Costa, V. V., Fagundes, C. T., Cisalpino, D., Cunha, T. M., et al. (2008). Commensal Microbiota Is Fundamental for the Development of Inflammatory Pain. Proc. Natl. Acad. Sci. U. S. A. 105, 2193–2197. doi: 10.1073/pnas.0711891105
Asano, Y., Hiramoto, T., Nishino, R., Aiba, Y., Kimura, T., Yoshihara, K., et al. (2012). Critical Role of Gut Microbiota in the Production of Biologically Active, Free Catecholamines in the Gut Lumen of Mice. Am. J. Physiol. Gastrointest. Liver Physiol. 303, G1288–G1295. doi: 10.1152/ajpgi.00341.2012
Bai, T., Li, Y., Xia, J., Jiang, Y., Zhang, L., Wang, H., et al. (2017). Piezo2: A Candidate Biomarker for Visceral Hypersensitivity in Irritable Bowel Syndrome? J. Neurogastroenterol. Motil. 23, 453–463. doi: 10.5056/jnm16114
Basbaum, A. I., Bautista, D. M., Scherrer, G., Julius, D. (2009). Cellular and Molecular Mechanisms of Pain. Cell 139, 267–284. doi: 10.1016/j.cell.2009.09.028
Bautista, D. M., Jordt, S. E., Nikai, T., Tsuruda, P. R., Read, A. J., Poblete, J., et al. (2006). TRPA1 Mediates the Inflammatory Actions of Environmental Irritants and Proalgesic Agents. Cell 124, 1269–1282. doi: 10.1016/j.cell.2006.02.023
Beer, F., Urbat, F., Franz, C., Huch, M., Kulling, S. E., Bunzel, M., et al. (2019). The Human Fecal Microbiota Metabolizes Foodborne Heterocyclic Aromatic Amines by Reuterin Conjugation and Further Transformations. Mol. Nutr. Food Res. 63, e1801177. doi: 10.1002/mnfr.201801177
Bellono, N. W., Bayrer, J. R., Leitch, D. B., Castro, J., Zhang, C., O’donnell, T. A., et al. (2017). Enterochromaffin Cells Are Gut Chemosensors That Couple to Sensory Neural Pathways. Cell 170, 185–198.e116. doi: 10.1016/j.cell.2017.05.034
Bi, Z., Zhang, S., Meng, Y., Feng, Y., Wang, Y., Wang, E., et al. (2021). Female Serotonin Transporter-Knockout Rat: A Potential Model of Irritable Bowel Syndrome. FASEB J. 35, e21701. doi: 10.1096/fj.202000007RRR
Blackshaw, L. A. (2014). Transient Receptor Potential Cation Channels in Visceral Sensory Pathways. Br. J. Pharmacol. 171, 2528–2536. doi: 10.1111/bph.12641
Boer, C. G., Radjabzadeh, D., Medina-Gomez, C., Garmaeva, S., Schiphof, D., Arp, P., et al. (2019). Intestinal Microbiome Composition and Its Relation to Joint Pain and Inflammation. Nat. Commun. 10, 4881. doi: 10.1038/s41467-019-12873-4
Bohórquez, D. V., Shahid, R. A., Erdmann, A., Kreger, A. M., Wang, Y., Calakos, N., et al. (2015). Neuroepithelial Circuit Formed by Innervation of Sensory Enteroendocrine Cells. J. Clin. Invest. 125, 782–786. doi: 10.1172/jci78361
Bonomo, R. R., Cook, T. M., Gavini, C. K., White, C. R., Jones, J. R., Bovo, E., et al. (2020). Fecal Transplantation and Butyrate Improve Neuropathic Pain, Modify Immune Cell Profile, and Gene Expression in the PNS of Obese Mice. Proc. Natl. Acad. Sci. U. S. A. 117, 26482–26493. doi: 10.1073/pnas.2006065117
Brandon-Mong, G. J., Shaw, G. T., Chen, W. H., Chen, C. C., Wang, D. (2020). A Network Approach to Investigating the Key Microbes and Stability of Gut Microbial Communities in a Mouse Neuropathic Pain Model. BMC Microbiol. 20, 295. doi: 10.1186/s12866-020-01981-7
Braniste, V., Al-Asmakh, M., Kowal, C., Anuar, F., Abbaspour, A., Tóth, M., et al. (2014). The Gut Microbiota Influences Blood-Brain Barrier Permeability in Mice. Sci. Transl. Med. 6, 263ra158. doi: 10.1126/scitranslmed.3009759
Braundmeier-Fleming, A., Russell, N. T., Yang, W., Nas, M. Y., Yaggie, R. E., Berry, M., et al. (2016). Stool-Based Biomarkers of Interstitial Cystitis/Bladder Pain Syndrome. Sci. Rep. 6, 26083. doi: 10.1038/srep26083
Braun, T., Voland, P., Kunz, L., Prinz, C., Gratzl, M. (2007). Enterochromaffin Cells of the Human Gut: Sensors for Spices and Odorants. Gastroenterology 132, 1890–1901. doi: 10.1053/j.gastro.2007.02.036
Brierley, S. M., Hughes, P. A., Page, A. J., Kwan, K. Y., Martin, C. M., O’donnell, T. A., et al. (2009). The Ion Channel TRPA1 Is Required for Normal Mechanosensation and Is Modulated by Algesic Stimuli. Gastroenterology 137, 2084–2095.e2083. doi: 10.1053/j.gastro.2009.07.048
Cenac, N., Altier, C., Motta, J. P., D’aldebert, E., Galeano, S., Zamponi, G. W., et al. (2010). Potentiation of TRPV4 Signalling by Histamine and Serotonin: An Important Mechanism for Visceral Hypersensitivity. Gut 59, 481–488. doi: 10.1136/gut.2009.192567
Chen, Z., Luo, J., Li, J., Kim, G., Stewart, A., Urban, J. F., Jr., et al. (2021b). Interleukin-33 Promotes Serotonin Release From Enterochromaffin Cells for Intestinal Homeostasis. Immunity 54, 151–163.e156. doi: 10.1016/j.immuni.2020.10.014
Chen, P., Wang, C., Ren, Y. N., Ye, Z. J., Jiang, C., Wu, Z. B. (2021a). Alterations in the Gut Microbiota and Metabolite Profiles in the Context of Neuropathic Pain. Mol. Brain 14, 50. doi: 10.1186/s13041-021-00765-y
Chen, Z., Zhao, H., Fu, N., Chen, L. (2018). The Diversified Function and Potential Therapy of Ectopic Olfactory Receptors in Non-Olfactory Tissues. J. Cell Physiol. 233, 2104–2115. doi: 10.1002/jcp.25929
Chiu, I. M. (2018). Infection, Pain, and Itch. Neurosci. Bull. 34, 109–119. doi: 10.1007/s12264-017-0098-1
Chiu, I. M., Heesters, B. A., Ghasemlou, N., Von Hehn, C. A., Zhao, F., Tran, J., et al. (2013). Bacteria Activate Sensory Neurons That Modulate Pain and Inflammation. Nature 501, 52–57. doi: 10.1038/nature12479
Clos-Garcia, M., Andrés-Marin, N., Fernández-Eulate, G., Abecia, L., Lavín, J. L., Van Liempd, S., et al. (2019). Gut Microbiome and Serum Metabolome Analyses Identify Molecular Biomarkers and Altered Glutamate Metabolism in Fibromyalgia. EBioMedicine 46, 499–511. doi: 10.1016/j.ebiom.2019.07.031
Cogan, T. A., Thomas, A. O., Rees, L. E., Taylor, A. H., Jepson, M. A., Williams, P. H., et al. (2007). Norepinephrine Increases the Pathogenic Potential of Campylobacter Jejuni. Gut 56, 1060–1065. doi: 10.1136/gut.2006.114926
Collins, J., Borojevic, R., Verdu, E. F., Huizinga, J. D., Ratcliffe, E. M. (2014). Intestinal Microbiota Influence the Early Postnatal Development of the Enteric Nervous System. Neurogastroenterol. Motil. 26, 98–107. doi: 10.1111/nmo.12236
Coste, B., Mathur, J., Schmidt, M., Earley, T. J., Ranade, S., Petrus, M. J., et al. (2010). Piezo1 and Piezo2 Are Essential Components of Distinct Mechanically Activated Cation Channels. Science 330, 55–60. doi: 10.1126/science.1193270
Cowan, C. S. M., Hoban, A. E., Ventura-Silva, A. P., Dinan, T. G., Clarke, G., Cryan, J. F. (2018). Gutsy Moves: The Amygdala as a Critical Node in Microbiota to Brain Signaling. Bioessays 40, 1. doi: 10.1002/bies.201700172
Cremon, C., Carini, G., Wang, B., Vasina, V., Cogliandro, R. F., De Giorgio, R., et al. (2011). Intestinal Serotonin Release, Sensory Neuron Activation, and Abdominal Pain in Irritable Bowel Syndrome. Am. J. Gastroenterol. 106, 1290–1298. doi: 10.1038/ajg.2011.86
Crowell, M. D., Wessinger, S. B. (2007). 5-HT and the Brain-Gut Axis: Opportunities for Pharmacologic Intervention. Expert Opin. Investig. Drugs 16, 761–765. doi: 10.1517/13543784.16.6.761
Cuozzo, M., Castelli, V., Avagliano, C., Cimini, A., D’angelo, M., Cristiano, C., et al. (2021). Effects of Chronic Oral Probiotic Treatment in Paclitaxel-Induced Neuropathic Pain. Biomedicines 9, 346. doi: 10.3390/biomedicines9040346
Dekker Nitert, M., Mousa, A., Barrett, H. L., Naderpoor, N., De Courten, B. (2020). Altered Gut Microbiota Composition Is Associated With Back Pain in Overweight and Obese Individuals. Front. Endocrinol. (Lausanne) 11, 605. doi: 10.3389/fendo.2020.00605
Deng, F., Zhao, B. C., Yang, X., Lin, Z. B., Sun, Q. S., Wang, Y. F., et al. (2021). The Gut Microbiota Metabolite Capsiate Promotes Gpx4 Expression by Activating TRPV1 to Inhibit Intestinal Ischemia Reperfusion-Induced Ferroptosis. Gut Microbes 13, 1–21. doi: 10.1080/19490976.2021.1902719
Diaz Heijtz, R., Wang, S., Anuar, F., Qian, Y., Björkholm, B., Samuelsson, A., et al. (2011). Normal Gut Microbiota Modulates Brain Development and Behavior. Proc. Natl. Acad. Sci. U. S. A. 108, 3047–3052. doi: 10.1073/pnas.1010529108
Di Giancamillo, A., Vitari, F., Bosi, G., Savoini, G., Domeneghini, C. (2010). The Chemical Code of Porcine Enteric Neurons and the Number of Enteric Glial Cells Are Altered by Dietary Probiotics. Neurogastroenterol. Motil. 22, e271–e278. doi: 10.1111/j.1365-2982.2010.01529.x
Ding, W., You, Z., Chen, Q., Yang, L., Doheny, J., Zhou, X., et al. (2021). Gut Microbiota Influences Neuropathic Pain Through Modulating Proinflammatory and Anti-Inflammatory T Cells. Anesth. Analg. 132, 1146–1155. doi: 10.1213/ane.0000000000005155
Dinić, M., Pecikoza, U., Djokić, J., Stepanović-Petrović, R., Milenković, M., Stevanović, M., et al. (2018). Exopolysaccharide Produced by Probiotic Strain Lactobacillus Paraplantarum BGCG11 Reduces Inflammatory Hyperalgesia in Rats. Front. Pharmacol. 9, 1. doi: 10.3389/fphar.2018.00001
Distrutti, E., Cipriani, S., Mencarelli, A., Renga, B., Fiorucci, S. (2013). Probiotics VSL3 Protect Against Development of Visceral Pain in Murine Model of Irritable Bowel Syndrome. PloS One 8, e63893. doi: 10.1371/journal.pone.0063893
Di, W., Zhang, L., Wang, S., Yi, H., Han, X., Fan, R., et al. (2017). Physicochemical Characterization and Antitumour Activity of Exopolysaccharides Produced by Lactobacillus Casei SB27 From Yak Milk. Carbohydr. Polym. 171, 307–315. doi: 10.1016/j.carbpol.2017.03.018
Dolgorukova, A., Isaeva, J. E., Verbitskaya, E., Lyubashina, O. A., Giniatullin, R., Sokolov, A. Y. (2021). Differential Effects of the Piezo1 Agonist Yoda1 in the Trigeminovascular System: An Electrophysiological and Intravital Microscopy Study in Rats. Exp. Neurol. 339, 113634. doi: 10.1016/j.expneurol.2021.113634
Duncker, S. C., Wang, L., Hols, P., Bienenstock, J. (2008). The D-Alanine Content of Lipoteichoic Acid Is Crucial for Lactobacillus Plantarum-Mediated Protection From Visceral Pain Perception in a Rat Colorectal Distension Model. Neurogastroenterol. Motil. 20, 843–850. doi: 10.1111/j.1365-2982.2008.01085.x
Eijkelkamp, N., Linley, J. E., Torres, J. M., Bee, L., Dickenson, A. H., Gringhuis, M., et al. (2013). A Role for Piezo2 in EPAC1-Dependent Mechanical Allodynia. Nat. Commun. 4, 1682. doi: 10.1038/ncomms2673
Erny, D., Hrabě De Angelis, A. L., Jaitin, D., Wieghofer, P., Staszewski, O., David, E., et al. (2015). Host Microbiota Constantly Control Maturation and Function of Microglia in the CNS. Nat. Neurosci. 18, 965–977. doi: 10.1038/nn.4030
Fattahi, F., Steinbeck, J. A., Kriks, S., Tchieu, J., Zimmer, B., Kishinevsky, S., et al. (2016). Deriving Human ENS Lineages for Cell Therapy and Drug Discovery in Hirschsprung Disease. Nature 531, 105–109. doi: 10.1038/nature16951
Fernández-Trillo, J., Florez-Paz, D., Íñigo-Portugués, A., González-González, O., Del Campo, A. G., González, A., et al. (2020). Piezo2 Mediates Low-Threshold Mechanically Evoked Pain in the Cornea. J. Neurosci. 40, 8976–8993. doi: 10.1523/jneurosci.0247-20.2020
Freidin, M. B., Stalteri, M. A., Wells, P. M., Lachance, G., Baleanu, A. F., Bowyer, R. C. E., et al. (2021). An Association Between Chronic Widespread Pain and the Gut Microbiome. Rheumatol. (Oxford) 60, 3727–3737. doi: 10.1093/rheumatology/keaa847
Gao, J., Gillilland, M. G., 3rd, Owyang, C. (2014). Rifaximin, Gut Microbes and Mucosal Inflammation: Unraveling a Complex Relationship. Gut Microbes 5, 571–575. doi: 10.4161/gmic.32130
Gershon, M. D. (2013). 5-Hydroxytryptamine (Serotonin) in the Gastrointestinal Tract. Curr. Opin. Endocrinol. Diabetes Obes. 20, 14–21. doi: 10.1097/MED.0b013e32835bc703
Gross, E. R., Gershon, M. D., Margolis, K. G., Gertsberg, Z. V., Li, Z., Cowles, R. A. (2012). Neuronal Serotonin Regulates Growth of the Intestinal Mucosa in Mice. Gastroenterology 143, 408–417.e402. doi: 10.1053/j.gastro.2012.05.007
Guglielmetti, S., Mora, D., Gschwender, M., Popp, K. (2011). Randomised Clinical Trial: Bifidobacterium Bifidum Mimbb75 Significantly Alleviates Irritable Bowel Syndrome and Improves Quality of Life–A Double-Blind, Placebo-Controlled Study. Aliment Pharmacol. Ther. 33, 1123–1132. doi: 10.1111/j.1365-2036.2011.04633.x
Guida, F., Boccella, S., Belardo, C., Iannotta, M., Piscitelli, F., De Filippis, F., et al. (2020). Altered Gut Microbiota and Endocannabinoid System Tone in Vitamin D Deficiency-Mediated Chronic Pain. Brain Behav. Immun. 85, 128–141. doi: 10.1016/j.bbi.2019.04.006
Guo, R., Chen, L. H., Xing, C., Liu, T. (2019). Pain Regulation by Gut Microbiota: Molecular Mechanisms and Therapeutic Potential. Br. J. Anaesth. 123, 637–654. doi: 10.1016/j.bja.2019.07.026
Guo, J., Gu, D., Zhao, T., Zhao, Z., Xiong, Y., Sun, M., et al. (2021). Trends in Piezo Channel Research Over the Past Decade: A Bibliometric Analysis. Front. Pharmacol. 12, 668714. doi: 10.3389/fphar.2021.668714
Han, P., Zhao, J., Liu, S. B., Yang, C. J., Wang, Y. Q., Wu, G. C., et al. (2013). Interleukin-33 Mediates Formalin-Induced Inflammatory Pain in Mice. Neuroscience 241, 59–66. doi: 10.1016/j.neuroscience.2013.03.019
Hayashi, F., Smith, K. D., Ozinsky, A., Hawn, T. R., Yi, E. C., Goodlett, D. R., et al. (2001). The Innate Immune Response to Bacterial Flagellin Is Mediated by Toll-Like Receptor 5. Nature 410, 1099–1103. doi: 10.1038/35074106
Hegde, S., Lin, Y. M., Fu, Y., Savidge, T., Shi, X. Z. (2020). Precision Lactobacillus Reuteri Therapy Attenuates Luminal Distension-Associated Visceral Hypersensitivity by Inducing Peripheral Opioid Receptors in the Colon. Pain 161, 2737–2749. doi: 10.1097/j.pain.0000000000001967
Hoban, A. E., Stilling, R. M., Moloney, G. M., Moloney, R. D., Shanahan, F., Dinan, T. G., et al. (2017). Microbial Regulation of Microrna Expression in the Amygdala and Prefrontal Cortex. Microbiome 5, 102. doi: 10.1186/s40168-017-0321-3
Holzer, P., Farzi, A. (2014). Neuropeptides and the Microbiota-Gut-Brain Axis. Adv. Exp. Med. Biol. 817, 195–219. doi: 10.1007/978-1-4939-0897-4_9
Hosseinifard, E. S., Morshedi, M., Bavafa-Valenlia, K., Saghafi-Asl, M. (2019). The Novel Insight Into Anti-Inflammatory and Anxiolytic Effects of Psychobiotics in Diabetic Rats: Possible Link Between Gut Microbiota and Brain Regions. Eur. J. Nutr. 58, 3361–3375. doi: 10.1007/s00394-019-01924-7
Hosseinkhani, F., Heinken, A., Thiele, I., Lindenburg, P. W., Harms, A. C., Hankemeier, T. (2021). The Contribution of Gut Bacterial Metabolites in the Human Immune Signaling Pathway of Non-Communicable Diseases. Gut Microbes 13, 1–22. doi: 10.1080/19490976.2021.1882927
Hua, P., Xiong, Y., Yu, Z., Liu, B., Zhao, L. (2019). Effect of Chlorella Pyrenoidosa Protein Hydrolysate-Calcium Chelate on Calcium Absorption Metabolism and Gut Microbiota Composition in Low-Calcium Diet-Fed Rats. Mar Drugs 17, 348. doi: 10.3390/md17060348
Hun, L. (2009). Bacillus Coagulans Significantly Improved Abdominal Pain and Bloating in Patients With IBS. Postgrad. Med. 121, 119–124. doi: 10.3810/pgm.2009.03.1984
Huynh, K. (2020). Novel Gut Microbiota-Derived Metabolite Promotes Platelet Thrombosis via Adrenergic Receptor Signalling. Nat. Rev. Cardiol. 17, 265. doi: 10.1038/s41569-020-0367-y
Hyland, N. P., Cryan, J. F. (2016). Microbe-Host Interactions: Influence of the Gut Microbiota on the Enteric Nervous System. Dev. Biol. 417, 182–187. doi: 10.1016/j.ydbio.2016.06.027
Jadrešin, O., Hojsak, I., Mišak, Z., Kekez, A. J., Trbojević, T., Ivković, L., et al. (2017). Lactobacillus Reuteri DSM 17938 in the Treatment of Functional Abdominal Pain in Children: RCT Study. J. Pediatr. Gastroenterol. Nutr. 64, 925–929. doi: 10.1097/mpg.0000000000001478
Jadrešin, O., Sila, S., Trivić, I., Mišak, Z., Kolaček, S., Hojsak, I. (2020). Lactobacillus Reuteri DSM 17938 Is Effective in the Treatment of Functional Abdominal Pain in Children: Results of the Double-Blind Randomized Study. Clin. Nutr. 39, 3645–3651. doi: 10.1016/j.clnu.2020.04.019
Jhun, J., Cho, K. H., Lee, D. H., Kwon, J. Y., Woo, J. S., Kim, J., et al. (2021). Oral Administration of Lactobacillus Rhamnosus Ameliorates the Progression of Osteoarthritis by Inhibiting Joint Pain and Inflammation. Cells 10, 1057. doi: 10.3390/cells10051057
Jiang, Y., Yang, X., Jiang, J., Xiao, B. (2021). Structural Designs and Mechanogating Mechanisms of the Mechanosensitive Piezo Channels. Trends Biochem. Sci. 46, 472–488. doi: 10.1016/j.tibs.2021.01.008
Kamm, K., Hoppe, S., Breves, G., Schröder, B., Schemann, M. (2004). Effects of the Probiotic Yeast Saccharomyces Boulardii on the Neurochemistry of Myenteric Neurones in Pig Jejunum. Neurogastroenterol. Motil. 16, 53–60. doi: 10.1046/j.1365-2982.2003.00458.x
Karsenty, G., Yadav, V. K. (2011). Regulation of Bone Mass by Serotonin: Molecular Biology and Therapeutic Implications. Annu. Rev. Med. 62, 323–331. doi: 10.1146/annurev-med-090710-133426
Kawase, T., Nagasawa, M., Ikeda, H., Yasuo, S., Koga, Y., Furuse, M. (2017). Gut Microbiota of Mice Putatively Modifies Amino Acid Metabolism in the Host Brain. Br. J. Nutr. 117, 775–783. doi: 10.1017/s0007114517000678
Kim, S. E., Coste, B., Chadha, A., Cook, B., Patapoutian, A. (2012). The Role of Drosophila Piezo in Mechanical Nociception. Nature 483, 209–212. doi: 10.1038/nature10801
Kremeyer, B., Lopera, F., Cox, J. J., Momin, A., Rugiero, F., Marsh, S., et al. (2010). A Gain-of-Function Mutation in TRPA1 Causes Familial Episodic Pain Syndrome. Neuron 66, 671–680. doi: 10.1016/j.neuron.2010.04.030
Lee, E., Takita, C., Wright, J. L., Slifer, S. H., Martin, E. R., Urbanic, J. J., et al. (2019). Genome-Wide Enriched Pathway Analysis of Acute Post-Radiotherapy Pain in Breast Cancer Patients: A Prospective Cohort Study. Hum. Genomics 13, 28. doi: 10.1186/s40246-019-0212-8
Lian, N., Shen, M., Zhang, K., Pan, J., Jiang, Y., Yu, Y., et al. (2021). Drinking Hydrogen-Rich Water Alleviates Chemotherapy-Induced Neuropathic Pain Through the Regulation of Gut Microbiota. J. Pain Res. 14, 681–691. doi: 10.2147/jpr.s288289
Lieder, B., Hoi, J., Burian, N., Hans, J., Holik, A. K., Beltran Marquez, L. R., et al. (2020). Structure-Dependent Effects of Cinnamaldehyde Derivatives on TRPA1-Induced Serotonin Release in Human Intestinal Cell Models. J. Agric. Food Chem. 68, 3924–3932. doi: 10.1021/acs.jafc.9b08163
Liñán-Rico, A., Ochoa-Cortes, F., Zuleta-Alarcon, A., Alhaj, M., Tili, E., Enneking, J., et al. (2017). UTP - Gated Signaling Pathways of 5-HT Release From BON Cells as a Model of Human Enterochromaffin Cells. Front. Pharmacol. 8, 429. doi: 10.3389/fphar.2017.00429
Liu, Y., Li, W., Yang, H., Zhang, X., Wang, W., Jia, S., et al. (2021b). Leveraging 16S Rrna Microbiome Sequencing Data to Identify Bacterial Signatures for Irritable Bowel Syndrome. Front. Cell Infect. Microbiol. 11, 645951. doi: 10.3389/fcimb.2021.645951
Liu, M., Li, Y., Zhong, J., Xia, L., Dou, N. (2021a). The Effect of IL-6/Piezo2 on the Trigeminal Neuropathic Pain. Aging (Albany NY) 13, 13615–13625. doi: 10.18632/aging.202887
Liu, Y. W., Wang, Y. P., Yen, H. F., Liu, P. Y., Tzeng, W. J., Tsai, C. F., et al. (2020). Lactobacillus Plantarum PS128 Ameliorated Visceral Hypersensitivity in Rats Through the Gut-Brain Axis. Probiotics Antimicrob. Proteins 12, 980–993. doi: 10.1007/s12602-019-09595-w
Logashina, Y. A., Korolkova, Y. V., Kozlov, S. A., Andreev, Y. A. (2019). TRPA1 Channel as a Regulator of Neurogenic Inflammation and Pain: Structure, Function, Role in Pathophysiology, and Therapeutic Potential of Ligands. Biochem. (Mosc) 84, 101–118. doi: 10.1134/s0006297919020020
Luczynski, P., Tramullas, M., Viola, M., Shanahan, F., Clarke, G., O’mahony, S., et al. (2017). Microbiota Regulates Visceral Pain in the Mouse. Elife 6, e25887. doi: 10.7554/eLife.25887
Lu, J., Synowiec, S., Lu, L., Yu, Y., Bretherick, T., Takada, S., et al. (2018). Microbiota Influence the Development of the Brain and Behaviors in C57BL/6J Mice. PloS One 13, e0201829. doi: 10.1371/journal.pone.0201829
Lv, W., Graves, D. T., He, L., Shi, Y., Deng, X., Zhao, Y., et al. (2020). Depletion of the Diabetic Gut Microbiota Resistance Enhances Stem Cells Therapy in Type 1 Diabetes Mellitus. Theranostics 10, 6500–6516. doi: 10.7150/thno.44113
Maeda, H., Zhu, X., Omura, K., Suzuki, S., Kitamura, S. (2004). Effects of an Exopolysaccharide (Kefiran) on Lipids, Blood Pressure, Blood Glucose, and Constipation. Biofactors 22, 197–200. doi: 10.1002/biof.5520220141
Ma, Y., Liu, S., Shu, H., Crawford, J., Xing, Y., Tao, F. (2020). Resveratrol Alleviates Temporomandibular Joint Inflammatory Pain by Recovering Disturbed Gut Microbiota. Brain Behav. Immun. 87, 455–464. doi: 10.1016/j.bbi.2020.01.016
Matsumoto, M., Kibe, R., Ooga, T., Aiba, Y., Sawaki, E., Koga, Y., et al. (2013). Cerebral Low-Molecular Metabolites Influenced by Intestinal Microbiota: A Pilot Study. Front. Syst. Neurosci. 7, 9. doi: 10.3389/fnsys.2013.00009
Matthes, S., Bader, M. (2018). Peripheral Serotonin Synthesis as a New Drug Target. Trends Pharmacol. Sci. 39, 560–572. doi: 10.1016/j.tips.2018.03.004
Mckernan, D. P., Fitzgerald, P., Dinan, T. G., Cryan, J. F. (2010). The Probiotic Bifidobacterium Infantis 35624 Displays Visceral Antinociceptive Effects in the Rat. Neurogastroenterol. Motil. 22, 1029–1035, e1268. doi: 10.1111/j.1365-2982.2010.01520.x
Mcvey Neufeld, K. A., Mao, Y. K., Bienenstock, J., Foster, J. A., Kunze, W. A. (2013). The Microbiome Is Essential for Normal Gut Intrinsic Primary Afferent Neuron Excitability in the Mouse. Neurogastroenterol. Motil. 25, 183–e188. doi: 10.1111/nmo.12049
Meijerink, J. (2021). The Intestinal Fatty Acid-Enteroendocrine Interplay, Emerging Roles for Olfactory Signaling and Serotonin Conjugates. Molecules 26, 1416. doi: 10.3390/molecules26051416
Meseguer, V., Alpizar, Y. A., Luis, E., Tajada, S., Denlinger, B., Fajardo, O., et al. (2014). TRPA1 Channels Mediate Acute Neurogenic Inflammation and Pain Produced by Bacterial Endotoxins. Nat. Commun. 5, 3125. doi: 10.1038/ncomms4125
Mikhailov, N., Leskinen, J., Fagerlund, I., Poguzhelskaya, E., Giniatullina, R., Gafurov, O., et al. (2019). Mechanosensitive Meningeal Nociception via Piezo Channels: Implications for Pulsatile Pain in Migraine? Neuropharmacology 149, 113–123. doi: 10.1016/j.neuropharm.2019.02.015
Nascimento, E. B., Jr., Romero, T. R. L., Dutra, M., Fiebich, B. L., Duarte, I. D. G., Coelho, M. M. (2021). Role of Peripheral 5-HT(1D), 5-HT(3) and 5-HT(7) Receptors in the Mechanical Allodynia Induced by Serotonin in Mice. BioMed. Pharmacother. 135, 111210. doi: 10.1016/j.biopha.2020.111210
Nilius, B., Owsianik, G. (2011). The Transient Receptor Potential Family of Ion Channels. Genome Biol. 12, 218. doi: 10.1186/gb-2011-12-3-218
Nilius, B., Szallasi, A. (2014). Transient Receptor Potential Channels as Drug Targets: From the Science of Basic Research to the Art of Medicine. Pharmacol. Rev. 66, 676–814. doi: 10.1124/pr.113.008268
Nishida, A., Miyamoto, J., Shimizu, H., Kimura, I. (2021). Gut Microbial Short-Chain Fatty Acids-Mediated Olfactory Receptor 78 Stimulation Promotes Anorexigenic Gut Hormone Peptide YY Secretion in Mice. Biochem. Biophys. Res. Commun. 557, 48–54. doi: 10.1016/j.bbrc.2021.03.167
Ni, K., Zhang, W., Ni, Y., Mao, Y. T., Wang, Y., Gu, X. P., et al. (2021). Dorsal Root Ganglia NR2B-Mediated Epac1-Piezo2 Signaling Pathway Contributes to Mechanical Allodynia of Bone Cancer Pain. Oncol. Lett. 21, 338. doi: 10.3892/ol.2021.12599
Nozawa, K., Kawabata-Shoda, E., Doihara, H., Kojima, R., Okada, H., Mochizuki, S., et al. (2009). TRPA1 Regulates Gastrointestinal Motility Through Serotonin Release From Enterochromaffin Cells. Proc. Natl. Acad. Sci. U. S. A. 106, 3408–3413. doi: 10.1073/pnas.0805323106
Nugraha, B., Gutenbrunner, C., Barke, A., Karst, M., Schiller, J., Schäfer, P., et al. (2019). The IASP Classification of Chronic Pain for ICD-11: Functioning Properties of Chronic Pain. Pain 160, 88–94. doi: 10.1097/j.pain.0000000000001433
Ochoa-Cortes, F., Ramos-Lomas, T., Miranda-Morales, M., Spreadbury, I., Ibeakanma, C., Barajas-Lopez, C., et al. (2010). Bacterial Cell Products Signal to Mouse Colonic Nociceptive Dorsal Root Ganglia Neurons. Am. J. Physiol. Gastrointest. Liver Physiol. 299, G723–G732. doi: 10.1152/ajpgi.00494.2009
Ohira, H., Tsutsui, W., Fujioka, Y. (2017). Are Short Chain Fatty Acids in Gut Microbiota Defensive Players for Inflammation and Atherosclerosis? J. Atheroscler. Thromb. 24, 660–672. doi: 10.5551/jat.RV17006
O’mahony, S. M., Felice, V. D., Nally, K., Savignac, H. M., Claesson, M. J., Scully, P., et al. (2014). Disturbance of the Gut Microbiota in Early-Life Selectively Affects Visceral Pain in Adulthood Without Impacting Cognitive or Anxiety-Related Behaviors in Male Rats. Neuroscience 277, 885–901. doi: 10.1016/j.neuroscience.2014.07.054
O’mahony, S. M., Hyland, N. P., Dinan, T. G., Cryan, J. F. (2011). Maternal Separation as a Model of Brain-Gut Axis Dysfunction. Psychopharmacol. (Berl) 214, 71–88. doi: 10.1007/s00213-010-2010-9
Pagano, E., Romano, B., Iannotti, F. A., Parisi, O. A., D’armiento, M., Pignatiello, S., et al. (2019). The Non-Euphoric Phytocannabinoid Cannabidivarin Counteracts Intestinal Inflammation in Mice and Cytokine Expression in Biopsies From UC Pediatric Patients. Pharmacol. Res. 149, 104464. doi: 10.1016/j.phrs.2019.104464
Pan, H., Gershon, M.D. (2000). Activation of Intrinsic Afferent Pathways in Submucosal Ganglia of the Guinea Pig Small Intestine. J. Neurosci. 20, 3295–3309. doi: 10.1523/jneurosci.20-09-03295.2000
Pellegrini, C., Antonioli, L., Colucci, R., Blandizzi, C., Fornai, M. (2018). Interplay Among Gut Microbiota, Intestinal Mucosal Barrier and Enteric Neuro-Immune System: A Common Path to Neurodegenerative Diseases? Acta Neuropathol. 136, 345–361. doi: 10.1007/s00401-018-1856-5
Philip, V., Newton, D. F., Oh, H., Collins, S. M., Bercik, P., Sibille, E. (2021). Transcriptional Markers of Excitation-Inhibition Balance in Germ-Free Mice Show Region-Specific Dysregulation and Rescue After Bacterial Colonization. J. Psychiatr. Res. 135, 248–255. doi: 10.1016/j.jpsychires.2021.01.021
Pluznick, J. (2014). A Novel SCFA Receptor, the Microbiota, and Blood Pressure Regulation. Gut Microbes 5, 202–207. doi: 10.4161/gmic.27492
Pluznick, J. L., Protzko, R. J., Gevorgyan, H., Peterlin, Z., Sipos, A., Han, J., et al. (2013). Olfactory Receptor Responding to Gut Microbiota-Derived Signals Plays a Role in Renin Secretion and Blood Pressure Regulation. Proc. Natl. Acad. Sci. U. S. A. 110, 4410–4415. doi: 10.1073/pnas.1215927110
Qin, H. Y., Zang, K. H., Zuo, X., Wu, X. A., Bian, Z. X. (2019). Quercetin Attenuates Visceral Hypersensitivity and 5-Hydroxytryptamine Availability in Postinflammatory Irritable Bowel Syndrome Rats: Role of Enterochromaffin Cells in the Colon. J. Med. Food 22, 663–671. doi: 10.1089/jmf.2018.4264
Ramakrishna, C., Corleto, J., Ruegger, P. M., Logan, G. D., Peacock, B. B., Mendonca, S., et al. (2019). Dominant Role of the Gut Microbiota in Chemotherapy Induced Neuropathic Pain. Sci. Rep. 9, 20324. doi: 10.1038/s41598-019-56832-x
Ranade, S. S., Qiu, Z., Woo, S. H., Hur, S. S., Murthy, S. E., Cahalan, S. M., et al. (2014a). Piezo1, a Mechanically Activated Ion Channel, Is Required for Vascular Development in Mice. Proc. Natl. Acad. Sci. U. S. A. 111, 10347–10352. doi: 10.1073/pnas.1409233111
Ranade, S. S., Woo, S. H., Dubin, A. E., Moshourab, R. A., Wetzel, C., Petrus, M., et al. (2014b). Piezo2 Is the Major Transducer of Mechanical Forces for Touch Sensation in Mice. Nature 516, 121–125. doi: 10.1038/nature13980
Reyes-Gibby, C. C., Wang, J., Silvas, M. R., Yu, R. K., Hanna, E. Y., Shete, S. (2016). Genome-Wide Association Study Suggests Common Variants Within RP11-634B7.4 Gene Influencing Severe Pre-Treatment Pain in Head and Neck Cancer Patients. Sci. Rep. 6, 34206. doi: 10.1038/srep34206
Rhee, S. H., Pothoulakis, C., Mayer, E. A. (2009). Principles and Clinical Implications of the Brain-Gut-Enteric Microbiota Axis. Nat. Rev. Gastroenterol. Hepatol. 6, 306–314. doi: 10.1038/nrgastro.2009.35
Ringel-Kulka, T., Goldsmith, J. R., Carroll, I. M., Barros, S. P., Palsson, O., Jobin, C., et al. (2014). Lactobacillus Acidophilus NCFM Affects Colonic Mucosal Opioid Receptor Expression in Patients With Functional Abdominal Pain - A Randomised Clinical Study. Aliment Pharmacol. Ther. 40, 200–207. doi: 10.1111/apt.12800
Romano, C., Ferrau, V., Cavataio, F., Iacono, G., Spina, M., Lionetti, E., et al. (2014). Lactobacillus Reuteri in Children With Functional Abdominal Pain (FAP). J. Paediatr. Child Health 50, E68–E71. doi: 10.1111/j.1440-1754.2010.01797.x
Rosser, E. C., Piper, C. J. M., Matei, D. E., Blair, P. A., Rendeiro, A. F., Orford, M., et al. (2020). Microbiota-Derived Metabolites Suppress Arthritis by Amplifying Aryl-Hydrocarbon Receptor Activation in Regulatory B Cells. Cell Metab. 31, 837–851.e810. doi: 10.1016/j.cmet.2020.03.003
Rousseaux, C., Thuru, X., Gelot, A., Barnich, N., Neut, C., Dubuquoy, L., et al. (2007). Lactobacillus Acidophilus Modulates Intestinal Pain and Induces Opioid and Cannabinoid Receptors. Nat. Med. 13, 35–37. doi: 10.1038/nm1521
Santoni, M., Miccini, F., Battelli, N. (2021). Gut Microbiota, Immunity and Pain. Immunol. Lett. 229, 44–47. doi: 10.1016/j.imlet.2020.11.010
Saulnier, D. M., Riehle, K., Mistretta, T. A., Diaz, M. A., Mandal, D., Raza, S., et al. (2011). Gastrointestinal Microbiome Signatures of Pediatric Patients With Irritable Bowel Syndrome. Gastroenterology 141, 1782–1791. doi: 10.1053/j.gastro.2011.06.072
Shen, S., Lim, G., You, Z., Ding, W., Huang, P., Ran, C., et al. (2017). Gut Microbiota Is Critical for the Induction of Chemotherapy-Induced Pain. Nat. Neurosci. 20, 1213–1216. doi: 10.1038/nn.4606
Singhmar, P., Huo, X., Eijkelkamp, N., Berciano, S. R., Baameur, F., Mei, F. C., et al. (2016). Critical Role for Epac1 in Inflammatory Pain Controlled by GRK2-Mediated Phosphorylation of Epac1. Proc. Natl. Acad. Sci. U. S. A. 113, 3036–3041. doi: 10.1073/pnas.1516036113
Souza, D. G., Vieira, A. T., Soares, A. C., Pinho, V., Nicoli, J. R., Vieira, L. Q., et al. (2004). The Essential Role of the Intestinal Microbiota in Facilitating Acute Inflammatory Responses. J. Immunol. 173, 4137–4146. doi: 10.4049/jimmunol.173.6.4137
Spiller, R., Pélerin, F., Cayzeele Decherf, A., Maudet, C., Housez, B., Cazaubiel, M., et al. (2016). Randomized Double Blind Placebo-Controlled Trial of Saccharomyces Cerevisiae CNCM I-3856 in Irritable Bowel Syndrome: Improvement in Abdominal Pain and Bloating in Those With Predominant Constipation. United Eur. Gastroenterol. J. 4, 353–362. doi: 10.1177/2050640615602571
Sugisawa, E., Takayama, Y., Takemura, N., Kondo, T., Hatakeyama, S., Kumagai, Y., et al. (2020). RNA Sensing by Gut Piezo1 Is Essential for Systemic Serotonin Synthesis. Cell 182, 609–624.e621. doi: 10.1016/j.cell.2020.06.022
Sun, N., Hu, H., Wang, F., Li, L., Zhu, W., Shen, Y., et al. (2021). Antibiotic-Induced Microbiome Depletion in Adult Mice Disrupts Blood-Brain Barrier and Facilitates Brain Infiltration of Monocytes After Bone-Marrow Transplantation. Brain Behav. Immun. 92, 102–114. doi: 10.1016/j.bbi.2020.11.032
Syeda, R., Florendo, M. N., Cox, C. D., Kefauver, J. M., Santos, J. S., Martinac, B., et al. (2016). Piezo1 Channels Are Inherently Mechanosensitive. Cell Rep. 17, 1739–1746. doi: 10.1016/j.celrep.2016.10.033
Tang, W., Dong, M., Wang, W., Han, S., Rui, X., Chen, X., et al. (2017). Structural Characterization and Antioxidant Property of Released Exopolysaccharides From Lactobacillus Delbrueckii Ssp. Bulgaricus SRFM-1. Carbohydr. Polym. 173, 654–664. doi: 10.1016/j.carbpol.2017.06.039
Tillisch, K., Labus, J., Kilpatrick, L., Jiang, Z., Stains, J., Ebrat, B., et al. (2013). Consumption of Fermented Milk Product With Probiotic Modulates Brain Activity. Gastroenterology 144, 1394–1401, 1401.e1391–1394. doi: 10.1053/j.gastro.2013.02.043
Treede, R. D., Meyer, R. A., Raja, S. N., Campbell, J. N. (1992). Peripheral and Central Mechanisms of Cutaneous Hyperalgesia. Prog. Neurobiol. 38, 397–421. doi: 10.1016/0301-0082(92)90027-c
Verdú, E. F., Bercik, P., Verma-Gandhu, M., Huang, X. X., Blennerhassett, P., Jackson, W., et al. (2006). Specific Probiotic Therapy Attenuates Antibiotic Induced Visceral Hypersensitivity in Mice. Gut 55, 182–190. doi: 10.1136/gut.2005.066100
Wang, F., Knutson, K., Alcaino, C., Linden, D. R., Gibbons, S. J., Kashyap, P., et al. (2017a). Mechanosensitive Ion Channel Piezo2 Is Important for Enterochromaffin Cell Response to Mechanical Forces. J. Physiol. 595, 79–91. doi: 10.1113/jp272718
Wang, P., Tu, K., Cao, P., Yang, Y., Zhang, H., Qiu, X. T., et al. (2021a). Antibiotics-Induced Intestinal Dysbacteriosis Caused Behavioral Alternations and Neuronal Activation in Different Brain Regions in Mice. Mol. Brain 14, 49. doi: 10.1186/s13041-021-00759-w
Wang, P., Tu, K., Cao, P., Yang, Y., Zhang, H., Qiu, X. T., et al. (2021b). Correction to: Antibiotics-Induced Intestinal Dysbacteriosis Caused Behavioral Alternations and Neuronal Activation in Different Brain Regions in Mice. Mol. Brain 14, 69. doi: 10.1186/s13041-021-00781-y
Wang, H., Xie, M., Charpin-El Hamri, G., Ye, H., Fussenegger, M. (2018). Treatment of Chronic Pain by Designer Cells Controlled by Spearmint Aromatherapy. Nat. BioMed. Eng. 2, 114–123. doi: 10.1038/s41551-018-0192-3
Wang, W., Xin, H., Fang, X., Dou, H., Liu, F., Huang, D., et al. (2017b). Isomalto-Oligosaccharides Ameliorate Visceral Hyperalgesia With Repair Damage of Ileal Epithelial Ultrastructure in Rats. PloS One 12, e0175276. doi: 10.1371/journal.pone.0175276
Weizman, Z., Abu-Abed, J., Binsztok, M. (2016). Lactobacillus Reuteri DSM 17938 for the Management of Functional Abdominal Pain in Childhood: A Randomized, Double-Blind, Placebo-Controlled Trial. J. Pediatr. 174, 160–164.e161. doi: 10.1016/j.jpeds.2016.04.003
Wen, J., Bo, T., Zhang, X., Wang, Z., Wang, D. (2020). Thermo-Trps and Gut Microbiota Are Involved in Thermogenesis and Energy Metabolism During Low Temperature Exposure of Obese Mice. J. Exp. Biol. 223, jeb218974. doi: 10.1242/jeb.218974
Wikoff, W. R., Anfora, A. T., Liu, J., Schultz, P. G., Lesley, S. A., Peters, E. C., et al. (2009). Metabolomics Analysis Reveals Large Effects of Gut Microflora on Mammalian Blood Metabolites. Proc. Natl. Acad. Sci. U. S. A. 106, 3698–3703. doi: 10.1073/pnas.0812874106
Wood, J. D. (2020). Serotonergic Integration in the Intestinal Mucosa. Curr. Pharm. Des. 26, 3010–3014. doi: 10.2174/1381612826666200612161542
Xu, D., Gao, J., Gillilland, M., 3rd, Wu, X., Song, I., Kao, J. Y., et al. (2014). Rifaximin Alters Intestinal Bacteria and Prevents Stress-Induced Gut Inflammation and Visceral Hyperalgesia in Rats. Gastroenterology 146, 484–496.e484. doi: 10.1053/j.gastro.2013.10.026
Xu, H. M., Huang, H. L., Zhou, Y. L., Zhao, H. L., Xu, J., Shou, D. W., et al. (2021). Fecal Microbiota Transplantation: A New Therapeutic Attempt From the Gut to the Brain. Gastroenterol. Res. Pract. 2021, 6699268. doi: 10.1155/2021/6699268
Xu, X., Hu, Y., Yan, E., Zhan, G., Liu, C., Yang, C. (2020). Perioperative Neurocognitive Dysfunction: Thinking From the Gut? Aging (Albany NY) 12, 15797–15817. doi: 10.18632/aging.103738
Xu, Z. Z., Kim, Y. H., Bang, S., Zhang, Y., Berta, T., Wang, F., et al. (2015). Inhibition of Mechanical Allodynia in Neuropathic Pain by TLR5-Mediated a-Fiber Blockade. Nat. Med. 21, 1326–1331. doi: 10.1038/nm.3978
Xu, G. Y., Shenoy, M., Winston, J. H., Mittal, S., Pasricha, P. J. (2008). P2X Receptor-Mediated Visceral Hyperalgesia in a Rat Model of Chronic Visceral Hypersensitivity. Gut 57, 1230–1237. doi: 10.1136/gut.2007.134221
Yang, C., Fang, X., Zhan, G., Huang, N., Li, S., Bi, J., et al. (2019). Key Role of Gut Microbiota in Anhedonia-Like Phenotype in Rodents With Neuropathic Pain. Transl. Psychiatry 9, 57. doi: 10.1038/s41398-019-0379-8
Yano, J. M., Yu, K., Donaldson, G. P., Shastri, G. G., Ann, P., Ma, L., et al. (2015). Indigenous Bacteria From the Gut Microbiota Regulate Host Serotonin Biosynthesis. Cell 161, 264–276. doi: 10.1016/j.cell.2015.02.047
Yoon, J. S., Sohn, W., Lee, O. Y., Lee, S. P., Lee, K. N., Jun, D. W., et al. (2014). Effect of Multispecies Probiotics on Irritable Bowel Syndrome: A Randomized, Double-Blind, Placebo-Controlled Trial. J. Gastroenterol. Hepatol. 29, 52–59. doi: 10.1111/jgh.12322
Yu, Y., Daly, D. M., Adam, I. J., Kitsanta, P., Hill, C. J., Wild, J., et al. (2016). Interplay Between Mast Cells, Enterochromaffin Cells, and Sensory Signaling in the Aging Human Bowel. Neurogastroenterol. Motil. 28, 1465–1479. doi: 10.1111/nmo.12842
Zhang, J., Bi, J. J., Guo, G. J., Yang, L., Zhu, B., Zhan, G. F., et al. (2019). Abnormal Composition of Gut Microbiota Contributes to Delirium-Like Behaviors After Abdominal Surgery in Mice. CNS Neurosci. Ther. 25, 685–696. doi: 10.1111/cns.13103
Zhan, G., Yang, N., Li, S., Huang, N., Fang, X., Zhang, J., et al. (2018). Abnormal Gut Microbiota Composition Contributes to Cognitive Dysfunction in SAMP8 Mice. Aging (Albany NY) 10, 1257–1267. doi: 10.18632/aging.101464
Zhou, F., Wang, X., Han, B., Tang, X., Liu, R., Ji, Q., et al. (2021). Short-Chain Fatty Acids Contribute to Neuropathic Pain via Regulating Microglia Activation and Polarization. Mol. Pain 17, 1744806921996520. doi: 10.1177/1744806921996520
Keywords: enterochromaffin cells, gut microbiota, hyperalgesia, chronic pain, microbiota-gut-brain axis
Citation: Xu X, Chen R, Zhan G, Wang D, Tan X and Xu H (2021) Enterochromaffin Cells: Sentinels to Gut Microbiota in Hyperalgesia? Front. Cell. Infect. Microbiol. 11:760076. doi: 10.3389/fcimb.2021.760076
Received: 17 August 2021; Accepted: 29 September 2021;
Published: 14 October 2021.
Edited by:
Frederic Antonio Carvalho, INSERM U1107 Douleur et Biophysique Neurosensorielle (Neuro-Dol), FranceReviewed by:
Parisa Gazerani, Oslo Metropolitan University, NorwaySaartjie Roux, Nelson Mandela University, South Africa
Peter Nagy, Cornell University, United States
Ivan Bonet, University of California, San Francisco, United States
Copyright © 2021 Xu, Chen, Zhan, Wang, Tan and Xu. This is an open-access article distributed under the terms of the Creative Commons Attribution License (CC BY). The use, distribution or reproduction in other forums is permitted, provided the original author(s) and the copyright owner(s) are credited and that the original publication in this journal is cited, in accordance with accepted academic practice. No use, distribution or reproduction is permitted which does not comply with these terms.
*Correspondence: Hui Xu, huixu@tjh.tjmu.edu.cn