- 1Department of Pharmacology and Toxicology, Egyptian Drug Authority [EDA; formerly The National Organization for Drug Control and Research (NODCAR)], Cairo, Egypt
- 2Pritzker School of Medicine, University of Chicago, Chicago, IL, United States
- 3Department of Obstetrics and Gynecology, University of Chicago, Chicago, IL, United States
- 4Department of Pathology, University of Illinois at Chicago, Chicago, IL, United States
Every year, millions of women are affected by genital tract disorders, such as bacterial vaginosis (BV), endometrial cancer, polycystic ovary syndrome (PCOS), endometriosis, and uterine fibroids (UFs). These disorders pose a significant economic burden on healthcare systems and have serious implications for health and fertility outcomes. This review explores the relationships between gut, vaginal, and uterine dysbiosis and the pathogenesis of various diseases of the female genital tract. In recent years, reproductive health clinicians and scientists have focused on the microbiome to investigate its role in the pathogenesis and prevention of such diseases. Recent studies of the gut, vaginal, and uterine microbiomes have identified patterns in bacterial composition and changes across individuals’ lives associated with specific healthy and diseased states, particularly regarding the effects of the estrogen–gut microbiome axis on estrogen-driven disorders (such as endometrial cancer, endometriosis, and UFs) and disorders associated with estrogen deficiency (such as PCOS). Furthermore, this review discusses the contribution of vitamin D deficiency to gut dysbiosis and altered estrogen metabolism as well as how these changes play key roles in the pathogenesis of UFs. More research on the microbiome influences on reproductive health and fertility is vital.
1. Introduction
Mounting evidence suggests that the microbiome has a critical role in maintaining human health in almost every organ system—from the skin to the gut to the male and female genital tracts (Rowe et al., 2020). The human microbiome refers to all of the bacteria, viruses, fungi, and archaea living on and within the human body, with the highest number being found in the gut. There is a complex relationship between the human host and its microbial inhabitants. Microbiota piqued scientific interest in many areas of pathological disorders, including tumor biology. Bacteria and their metabolites can control many signaling pathways (e.g., E-cadherin/β-catenin signaling), inducing DNA double-strand breaks and activation of the DNA damage checkpoint pathway, leading to cell death, and modifying cell differentiation, which helps to maintain the homeostasis of the body (Nougayrède et al., 2006; Rubinstein et al., 2013; Eslami-S et al., 2020). Dysbiosis refers to the imbalance of harmful microbes and has been linked to cancer and other diseases (Armstrong et al., 2018; Maynard, 2019; Lu et al., 2021).
Babies acquire their mother’s microbiome at birth through the vaginal canal or face the skin during a Cesarean delivery (Wang et al., 2017). Microbes colonize areas directly exposed to the air or surrounding environment, such as the gastrointestinal tract (including the mouth and stomach), urogenital tract, and skin. Their survival and growth depend on their environment, and each niche has a characteristic collection of microbes. Many factors influence the final bacterial composition within each organ system and among individuals. In particular, the adult microbiome is highly influenced by age, genetics, ethnicity, diet, exercise, chemotherapy, probiotics, prebiotics, smoking, stress, and exposure to endocrine-disrupting chemicals (EDCs), which are well-known factors affecting the populations of different microbes in the gut (Figure 1). The microbiome can in turn influence the overall health of the host (Evans et al., 2014; Singh et al., 2017; Hasan and Yang, 2019; Rinninella et al., 2019). The microbiome interacts with the host immune system and is also independently metabolically active, producing factors that alter the pH of the local environment, with consequences for nearby microbes and host tissues.
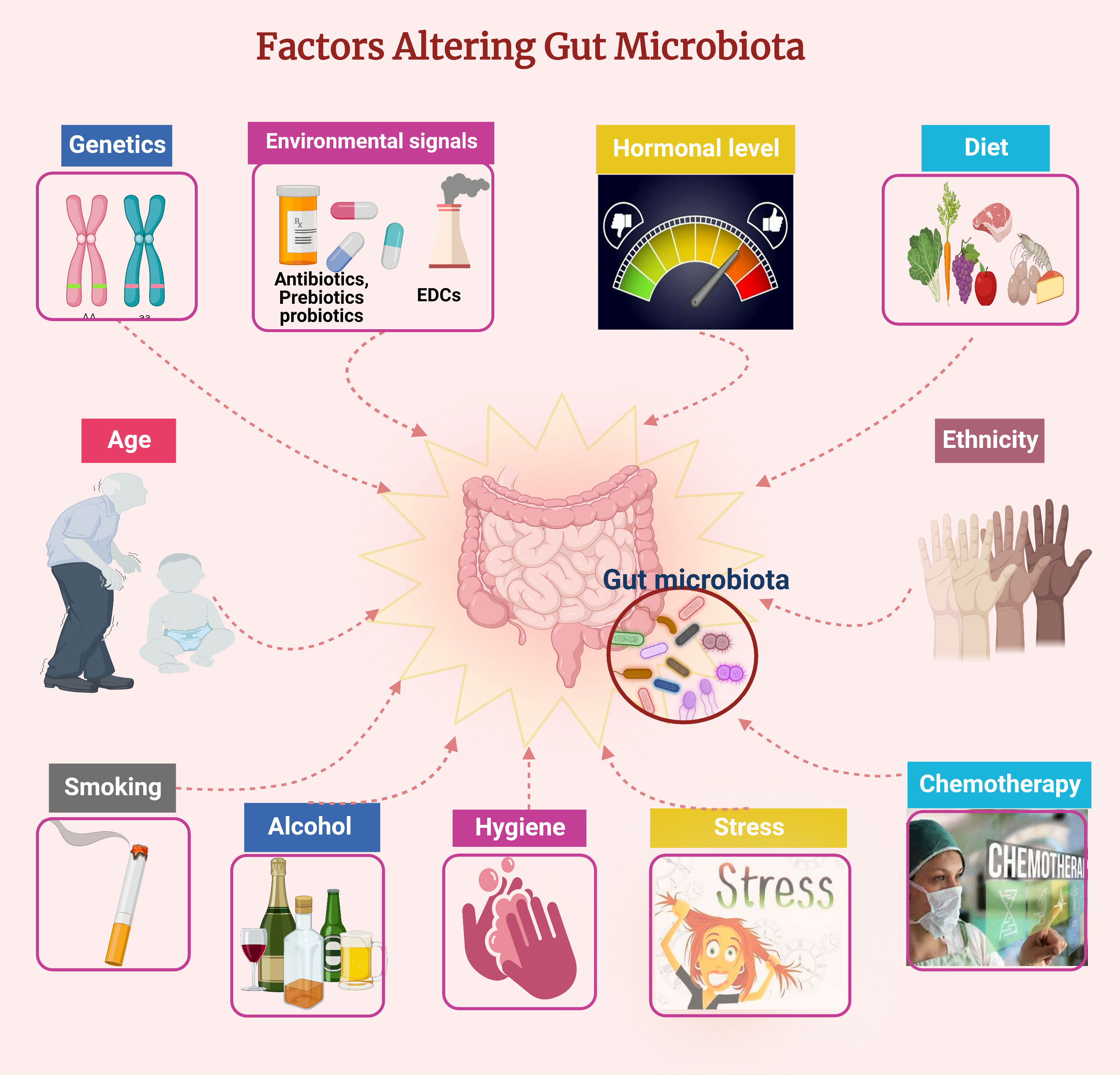
Figure 1 Factors affecting the gut microbiota composition. Figure created using BioRender.com.
Recent studies suggest a potential connection between certain gut bacteria and female reproductive tract disorders, such as bacterial vaginosis (BV), cervical and endometrial cancer, polycystic ovary syndrome (PCOS), postmenopausal syndrome, endometriosis, endometritis, and uterine fibroids (UFs) (Mirmonsef et al., 2011; Bodelon et al., 2014; Fuhrman et al., 2014; Guo et al., 2016; Yuan et al., 2018; Łaniewski et al., 2020; Qiu et al., 2022). An investigation into the delicate architecture of the human microbiome may yield insights to improve gynecological health. This review explores the relationships between the gut, vaginal, and uterine dysbiosis and the pathogenesis of various female reproductive tract diseases, such as BV, cervical and endometrial cancer, PCOS, postmenopausal syndrome, endometriosis, endometritis, and UFs. This review also discusses the effect of vitamin D deficiency on the diversity of the gut microbiota and circulated estrogen levels on UF development.
2. Female reproductive tract microbiomes
The female reproductive tract comprises the vulva, vagina, cervix, endometrium, fallopian tubes, and ovaries. It harbors a unique microbiome that accounts for approximately 9% of the body’s total microbial population (Moreno and Simon, 2019). The microbiome consists of bacteria (bacteriome), archaea (archaeome), fungi (fungome), viruses (virome), and a few protozoal parasites (Madere & Monaco, 2022). The female reproductive tract microbiome has an essential protective role in supporting vaginal health, reducing the likelihood of preterm birth, and controlling urogenital infections such as BV, yeast infections, and sexually transmitted infections (STIs), including human immunodeficiency virus (HIV) (Punzón-Jiménez and Labarta, 2021). Studies on the female reproductive tract microbiome have mainly focused on determining the bacterial composition and functions (Franasiak and Scott, 2015). At least five bacterial community groupings are represented in the female reproductive tract, distinguishable by the abundance of Lactobacillus spp. and the existence of more diverse anaerobes (Smith & Ravel, 2017; Madere and Monaco, 2022).
Upper versus lower reproductive tract microbiomes
The upper reproductive tract comprises the fallopian tubes, ovaries, and uterus, including the endocervix, and the lower tract consists of the ectocervix and vagina. The microbiome of the female genital tract varies between the lower and upper tracts, with the lower tract having a bacterial burden of 100–10,000-fold more than the upper tract (Chen et al., 2017; Baker et al., 2018). In 2011, Ravel et al. (2011) described five community state types (CSTs) in the vagina of healthy women, determined mainly by the dominant species of Lactobacillus and pH value, with CSTs I, II, III, and V involving dominant L. crispatus, L. gasseri, L. iners, and L. jensenii, respectively. CST IV has a low abundance of Lactobacillus and higher abundances of specific anaerobic genera (Atopobium, Dialister, Gardnerella, Prevotella, and Sneathia) (Ravel et al., 2011). Lactobacillus (i.e., L. crispatus and L. iners) was abundant in 92% of cervical specimens from healthy women (Ravel et al., 2011). Another study confirmed that the microbiome is strongly altered from the lower to the upper genital tract, with decreased Lactobacillus in the upper genital tract compared to that in the lower genital tract (Chen et al., 2017). The female reproductive tract microbiome is not fixed but changes with age, physiological conditions, lifestyle, and environmental factors.
2.1.1. Vaginal microbiome
The composition of the vaginal microbiome is important in reproductive health. Lactobacillus is one of the main bacteria colonizing the healthy vagina. Its production of lactic acid as a metabolic bioproduct inhibits the overgrowth of potentially pathogenic yeast, bacteria, and viruses, thus limiting phylogenetic diversity in the vaginal microbiome. Epidemiological research has linked the abundance of Lactobacillus to ethnicity, with Caucasian/Asian women having high levels of Lactobacillus compared to African/Hispanic women (Gupta et al., 2019). The mean pH varies from 4.0 ± 0.3 for CST I to 5.3 ± 0.6 for CST IV (Ravel et al., 2011; Punzón-Jiménez and Labarta, 2021). In the presence of estrogen, vaginal epithelial cells accumulate glycogen that can be metabolized by Lactobacillus into lactic acid (Miller et al., 2016), resulting in decreased vaginal pH and less colonization by pathogenic bacteria such as Chlamydia trachomatis, Neisseria gonorrhoeae, and Trichomonas vaginalis (Chen et al., 2017; Champer et al., 2018; Chambers et al., 2021).
The implications of vaginal dysbiosis are represented by the condition of BV. BV is a common cause of abnormal vaginal discharge, and sex hormones may play a role in BV pathogenesis. Estrogen increases vaginal epithelial activity, resulting in a condensed epithelium with more glycogen deposited in the superficial cells (Punzón-Jiménez and Labarta, 2021). This vaginal acidity is vital in preventing the overgrowth of BV-associated bacteria. Decreased estrogen is associated with vaginal dysbiosis, involving the replacement of regular hydrogen peroxide- and lactic acid-producing Lactobacillus in the vagina with a high abundance of anaerobic bacteria such as Gardnerella, Mycoplasma, and Prevotella, which increases the vaginal pH to >4.5 (Punzón-Jiménez and Labarta, 2021). Anaerobic bacteria associated with BV include Gardnerella vaginalis, which has virulence factors that allow it to bind to the host epithelium and generate a biofilm community that contributes to BV symptoms (Schwebke et al., 2014). Vaginal dysbiosis is made more likely by contraceptives, hormonal changes, obesity, and hysterectomy (Gupta et al., 2019).
2.1.2. Endometrial microbiome
The endometrium plays a critical role in the reproductive process in premenopausal women. It is associated with complex physiological changes influenced by estrogen and progesterone during the menstrual cycle and pregnancy. It is also affected by factors such as prostaglandins, integrins, angiogenesis, and immune-modulating cytokines. The first study on the bacterial composition of the uterus reported that real-time quantitative PCR identified 95% of 58 patients undergoing hysterectomy as having a uterine microbiota, with the most common species being L. iners (in 45%) and Prevotella (in 33%) (Mitchell et al., 2015). Several subsequent studies have found that Lactobacillus is the most prominent genus in the endometrium, with Lactobacillus being identified in both endometrial fluid and endometrial biopsies (Franasiak et al., 2016; Moreno et al., 2016; Miles et al., 2017; Moreno and Simon, 2019; Kiecka et al., 2021). It is unclear whether Lactobacillus is an actual endometrial colonizer or whether it ascended from the vagina. These results were consistent with another study on 70 women, with additional findings of Streptococcus, Staphylococcus, Corynebacterium, and Bifidobacterium (Tao et al., 2017). Moreno and Simon (2019) reported that among 35 endometrial samples from fertile healthy women, Lactobacillus was the most abundant genus (71.1%), followed by Gardnerella, Bifidobacterium, Streptococcus, and Prevotella (12.6%, 3.7%, 3.2%, and 0.9%, respectively) (Moreno & Simon, 2019). A low percentage of Lactobacillus in the female vaginal sample is less likely to have a successful embryo implantation, with an interplay between the uterine microenvironment and the host’s immune system (Moreno et al., 2022). This involves increased pro-inflammatory cytokine production in endometrial cells, including interleukin (IL)-1α, IL-1β, IL-17α, and tumor necrosis factor (TNF)-α, due to the estrogen-dependent nature of endometrial cell cytokine production (Sobstyl et al., 2022). However, other groups reported that Proteobacteria, Firmicutes, and Bacteroidetes were prevalent in endometrial samples, while Lactobacillus spp. was only found in a low percentage of endometrial samples (Verstraelen et al., 2016; Chen et al., 2017; Burac, 2018; Liu et al., 2019). Future endometrial microbiota studies need to focus on the effect of the microbiota on the female genital tract to potentially enhance the treatment of gynecological disorders. Although several studies have highlighted the predominance of Lactobacillus in the uterine microbiota, there remains uncertainty about the bacterial composition related to the healthy endometrium.
2.1.3. Fallopian tube and ovarian microbiomes
The fallopian tubes and ovaries harbor highly variable microbial species among different women. Additionally, a study found high intraindividual variability between fallopian tubes and ovaries, with Bacteroides, Corynebacterium, Lactobacillus, Coprococcus, and Hymenobacter being found in fallopian tube fluid specimens, and Lactobacillus, Corynebacterium, Escherichia, and Blautia being found in ovarian fluid specimens (Miles et al., 2017). Chen et al. (2017) reported that the fallopian tubes and ovaries had lower Lactobacillus than the vagina and cervix. In contrast, Pelzer et al. (2011) reported that fallopian tube specimens lacked Lactobacillus, but they did have at least some bacterial species. In addition, Pelzer et al. (2011) showed that, regarding the ovarian bacterial community, L. iners, Actinomyces spp., Corynebacterium aurimucosum, Fusobacterium spp., Prevotella, and Staphylococcus spp. were more dominant in the left ovarian follicular fluid than the right. Fallopian tubes and ovaries harbor a mixture of bacteria that thrive in mildly alkaline states, contrasting with the acidity of the vagina (Punzón-Jiménez and Labarta, 2021).
3. Gut microbiome and link to estrogen metabolism
3.1.. Overview of gut microbiome
The gut microbiome is a complex ecosystem of microbes acting upon and changing in response to the host environment (Gilbert et al., 2018; Chambers et al., 2021). The gut microbiome is dominated by five bacterial phyla: phylum Firmicutes (Clostridium, Lactobacillus, Eubacterium, Ruminococcus, Butyrivibrio, Anaerostipes, Roseburia, Faecalibacterium, etc.; Gram-positive species), phylum Bacteroidetes (Bacteroides, Porphyromonas, Prevotella, etc.; Gram-negative species), phylum Proteobacteria (Enterobacteriaceae, etc.; Gram-negative species), phylum Actinobacteria (Bifidobacterium; Gram-negative species), and phylum Verrucomicrobia (Akkermansia, etc.; Gram-negative species) (Doaa et al., 2016). Regarding the gut microbiota, almost 90% are Firmicutes and Bacteroidetes, while the rest are Actinobacteria and Proteobacteria. Verrucomicrobia is the least abundant (Yurtdaş and Akdevelioğlu, 2020; Sędzikowska and Szablewski, 2021). The gut microbiome’s structure is developed from birth and modulated by delivery mode and the maternal skin and gut microbiomes (Dominguez-Bello et al., 2010).
The gut microbiota influences the intestinal milieu, which acts on other organs and pathways. A healthy gut microbiota improves digestion, metabolism, and immune response; the symbiotic relationship between the host’s gut and the microbiota that maintains homeostasis is known as normobiosis (Rea et al., 2018).
Gut microbiomes are responsible for metabolizing estrogen; estrogens are metabolized by microbe-secreted β-glucuronidase, which converts estrogen from a conjugated form to a deconjugated form (Plottel and Blaser, 2011; Williams et al., 2020). Deconjugated and unbound (active and free) estrogens circulate in the bloodstream and bind to estrogen receptors alpha (ERα) and beta (ERβ) (Baker et al., 2017). Phytoestrogens are similarly metabolized and act via ERα and ERβ (Baker et al., 2017). The binding of estrogens and phytoestrogens to ERs initiates downstream signaling (Rietjens et al., 2017). This ultimately alters physiological conditioning, affecting reproductive health and neural development (Dewi et al., 2012).
3.2. Estrobolome and endobolome
The estrobolome refers to the gut microbiota genes involved in the metabolism of estrogen (Plottel and Blaser, 2011; Graham et al., 2021), while the endobolome refers to the gut microbiota genes and pathways involved in the metabolism of steroid hormones (including estrogen) and EDCs (Aguilera et al., 2020). EDCs have been defined by the US Environmental Protection Agency as “exogenous chemicals that interfere with the synthesis, secretion, metabolism, binding activity, or elimination of natural blood-borne hormones that are present in the body and are responsible for homeostasis, reproduction, and developmental function” (Diamanti-Kandarakis et al., 2009; Zoeller et al., 2016; Aguilera et al., 2020). EDCs include natural chemicals found in nature (phytoestrogens, genistein, and coumestrol) and artificial chemicals utilized in industrial solvents/lubricants and their by-products [polychlorinated biphenyls (PCBs), polybrominated biphenyls (PBBs), and dioxins], plasticizers (phthalates), pesticides [methoxychlor, chlorpyrifos, and dichlorodiphenyltrichloroethane (DDT)], antibacterials (triclosan), fungicides (vinclozolin), diethylstilbestrol (DES), and bisphenol A (BPA) (Diamanti-Kandarakis et al., 2009; Darbre, 2017; Aguilera et al., 2020). EDC exposure can cause marked gut dysbiosis, which can lead to disorders in many organ systems (Rosenfeld, 2017).
4. Gut dysbiosis and gynecological disorders
Estrogen–gut microbiome relations have physiological and clinical outcomes. Dysbiosis and a drop in gut microbiota abundance affect the estrobolome, leading to broad-range disorders (Baker et al., 2017). Dysbiosis and inflammation reduce the gut microbiota and reduce the β-glucuronidase activity. This reduction in the function of β-glucuronidase is followed by free estrogen reduction in the blood circulation. This is followed by altering estrogen receptor activations, leading to a hypoestrogenic pathologic status: obesity and metabolic syndrome, including PCOS. Furthermore, the estrobolome causes a hyperestrogenic pathologic status via a rise in the abundance of β-glucuronidase-producing bacteria, i.e., an increased Firmicutes/Bacteroidetes ratio, which boosts levels of free circulating estrogens and increases binding to ERα and ERβ, causing diseases such as endometrial cancer, breast cancer, endometriosis, and UFs to force disorders such as endometriosis and cancer (Plottel and Blaser, 2011; Dewi et al., 2012; Baker et al., 2017) (Figures 2A, B). Apparently, the gut microbiome regulates estrogen metabolism and implicates estrogen-related disorders (Flores et al., 2012; Sommer and Bäckhed, 2013; Liu et al., 2017). Moreover, EDC exposure in the developmental stage is linked to the development of UFs later in life. This is due to an altered estrogen balance and inhibition of DNA damage repair machinery, which lead to conversion of myometrium stem cells to tumor-initialing stem cells (Prusinski Fernung et al., 2018; Elkafas et al., 2019).
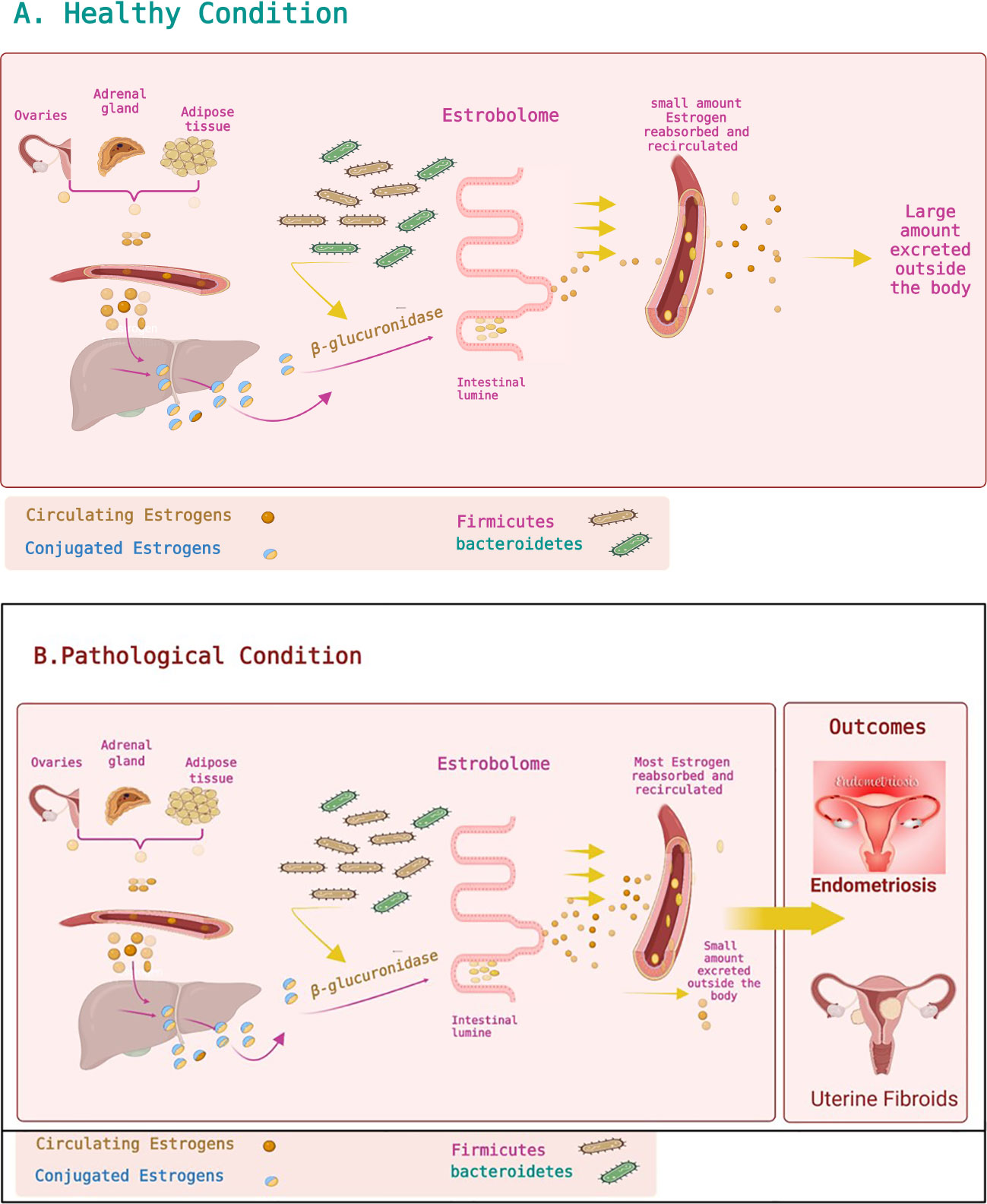
Figure 2 Estrobolome and estrogen metabolism. (A) Healthy condition. Estrogens are mainly produced by the ovaries, adipose tissue, and adrenal glands and distributed via the bloodstream in a free or protein-bound state. Estrogens and their metabolites can then be conjugated in the liver. Conjugated estrogens are excreted from the liver after metabolic transformation to water-soluble molecules and passed in the bile to the gut. The conjugated estrogens eliminated in the bile can be deconjugated by gut bacteria that have β-glucuronidase activity, leading to estrogen reabsorption into the bloodstream. Circulating estrogens impact target tissues, including the uterus, stimulating cell proliferation. The estrobolome affects estrogen elimination and circulation by regulating the enterohepatic circulation of estrogens. (B) Pathological condition. Altering the estrobolome by increasing the Firmicutes/Bacteroidetes ratio leads to more deconjugated estrogen and more circulating estrogen, impacting specific organs and contributing to multiple diseases such as endometriosis and uterine fibroids. Figures are created using BioRender.com.
4.1. Polycystic ovary syndrome
PCOS is a hormonal disease caused by an imbalance between estrogen and androgen. Tremellen and Pearce (2012) studied the role of gut dysbiosis in PCOS development due to poor diet. Dysbiosis increases gut mucosal permeability, resulting in the entry of lipopolysaccharide from Gram-negative colonic bacteria into the circulation. The resultant activation of the immune system interrupts insulin receptor function, increasing serum insulin levels, raising the ovaries’ production of androgens, and interfering with regular follicle production (Tremellen and Pearce, 2012). Studies have shown that lower circulating unconjugated estrogen and increased testosterone in PCOS patients may alter the gut microbiome (Tremellen and Pearce, 2012; Liu et al., 2017). Regarding the role of gut microbiota on PCOS, studies done by Guo et al. (2016) in 2016 showed that treatment of female Sprague–Dawley rats with letrozole (a nonsteroidal aromatase inhibitor) promoted PCOS and gut dysbiosis and altered the sex hormone levels, estrous cycles, and ovarian morphology. Lactobacillus, Ruminococcus, and Clostridium were downregulated while Prevotella was upregulated in PCOS rats compared to control rats (Guo et al., 2016). The same group showed that after treating PCOS rats with Lactobacillus and fecal microbiota transplantation (FMT) from healthy rats, the estrous cycles were enhanced in all animals in the FMT study group and 75% of rats in the Lactobacillus transplantation group had diminished androgen biosynthesis and normalized ovarian morphologies. The bacterial composition of gut microbiota was fixed in both FMT and Lactobacillus-treated groups, raising Lactobacillus and Clostridium and reducing Prevotella (Guo et al., 2016). A study indicated that the gut dysbiosis related to PCOS involved decreased bacterial diversity and an altered Firmicutes/Bacteroidetes ratio (Thackray, 2019). The role of the lower genital tract microbiota in PCOS has also been investigated. A study of vaginal and cervical biopsies showed that there were increased pathogens (such as Gardnerella vaginalis, Mycoplasma hominis, and Prevotella) and reduced Lactobacillus in both the vagina and cervix in PCOS patients compared to healthy controls (Tu et al., 2020). These findings were consistent with another study showing increased Mycoplasma and Prevotella and decreased L. crispatus in vaginal and cervical samples from PCOS patients compared to healthy controls (Hong et al., 2020). Thus, the estrobolome may affect sex hormone-driven disorders involving estrogen, such as PCOS.
4.2. Postmenopausal syndrome
Menopause is the permanent stop of menstrual cycle resulting in the loss of folliculogenesis and reduction in estrogen levels resulting in modifications impacting all body functions (Dalal and Agarwal, 2015). The gut microbiota may be important in regulating estrogen levels and estrogen metabolism during and after menopause. In postmenopausal women, gut microbiota diversity is linked to higher estrogen metabolite levels relative to parent estrogen levels in urine (Fuhrman et al., 2014). The gut microbiota also metabolizes estrogen-like compounds in food (such as soy isoflavones) and stimulates specific bacterial growth. Supplementation with soy isoflavones in postmenopausal women increases Bifidobacterium and reduces unclassified Clostridiaceae (Qi et al., 2021). Bifidobacterium has a beneficial role in stimulating absorption and immunity and inhibiting intestinal infection, while Clostridiaceae is involved in inflammatory diseases and is associated with obesity. These effects suggest that this host–microbiome estrogen-related interaction contributes to a broad spectrum of pathways influencing women’s health and illness (Nakatsu et al., 2014; Qi et al., 2021).
4.3. Cervical and endometrial cancer
During dysbiosis, invasion of the vagina by many anaerobic bacteria as the abundance of Lactobacillus decreases leads to inflammatory reactions that promote tumor growth, thus causing diseases such as cervical intraepithelial neoplasia, which can develop into cervical cancer (Champer et al., 2018). Endometrial cancer is the fourth most common cancer in women and the fifth most common cause of cancer death in the United States (Brooks et al., 2019). In endometrial cancer, estrogens are essential for increasing inflammation by upregulating the production of pro-inflammatory mediators such as IL-6 and TNF-α, which can in turn synergistically upregulate the expression of enzymes involved in ovarian steroidogenesis (aromatase, 17β-hydroxysteroid dehydrogenase, and estrone sulfatase), thus producing a feedback loop (Wallace et al., 2010; Borella et al., 2021). Moreover, high estrogen can change the vaginal microbiome, indirectly sustaining the development of endometrial cancer by interfering with the gut and vaginal microbiomes (Nakamura et al., 2019; Borella et al., 2021). A cross-sectional study analyzed the microbiomes of the vagina, cervix, fallopian tubes, and ovaries and found that the bacterial taxa in the genital tract of patients with endometrial cancer are characterized by the predominance of Firmicutes (Anaerostipes, Dialister, Peptoniphilus, Ruminococcus, and Anaerotruncus), Spirochaetes (such as Treponema), Actinobacteria (Atopobium), Bacteroidetes (Bacteroides and Porphyromonas), and Proteobacteria (Arthrospira) (Walther-António et al., 2016; Borella et al., 2021). Another study examined the influence of Atopobium vaginae and Porphyromonas somera on endometrial cells in vitro, revealing that these bacteria increase the release of inflammatory cytokines and chemokines (IL-1α, IL-1β, IL-17α, and TNF-α) by endometrial cells, indicating their role in maintaining inflammation and in neoplastic initiation (Caselli et al., 2019). This was supported by another study demonstrating a link between genital tract dysbiosis, high inflammatory cytokine expression, and endometrial cancer (Lu et al., 2021).
4.4. Endometriosis and endometritis
Endometriosis is characterized by chronic inflammation in which the endometriotic cells promote the synthesis of ILs, TNF-α, and vascular endothelial growth factor (VEGF), generating the conditions for tumor transformation (Kokcu, 2011; Jiang et al., 2021). Endometritis is an infectious and inflammatory disorder of the endometrium (Kitaya et al., 2018).
Estrogen promotes epithelial cell proliferation throughout the female genital tract, and estrogen dysregulation is associated with proliferative disorders such as endometriosis (Zhang et al., 2009; Anderson, 2019). Endometriosis is common in premenopausal women and is associated with a hyperproliferative state secondary to elevated estrogen levels (Laschke and Menger, 2016). In 2002, Bailey and Coe (2002) showed that monkeys with endometriosis had a low abundance of Lactobacillus and a high abundance of Gram-negative bacteria. Recently, research has shown that endometriosis is accompanied by gut microbial dysbiosis; however, the exact findings vary among studies. For instance, Ata et al. (2019) showed that patients with moderate-to-severe endometriosis (n = 14) have more Shigella/Escherichia in their colon than healthy individuals (n = 14). In contrast, Shan et al. (2021) showed that patients with stage 3–4 endometriosis (n = 12) had lower gut microbiota alpha diversity and a higher Firmicutes/Bacteroidetes ratio than healthy participants. A recent large study by Svensson et al. (2021) on stool samples reported that the abundance of 12 bacteria in the classes Bacilli, Bacteroidia, Clostridia, Coriobacteriia, and Gammaproteobacteria strongly varied between endometriosis patients (n = 66) and healthy individuals (n = 198). The discrepancy between these studies necessitates more extensive studies of how endometriosis stage, age, race, medical history, medication use, and diet affect the bacteria involved in endometriosis (Chadchan et al., 2022). An animal study reported that at 42 days after mice were intraperitoneally injected with endometrial tissue to trigger endometriosis, there was a significant elevation in the Firmicutes/Bacteroidetes ratio between the endometriotic and control mice (Yuan et al., 2018).
Several studies have examined the relationship between hormone dysregulation, the uterine microbiota, and gynecological disorders such as endometriosis (Khan et al., 2014; Walther-António et al., 2016; Hernandes et al., 2020; Schreurs et al., 2021). Investigated the intrauterine microbiome colonization and occurrence of endometritis in women with endometriosis and controls (fertile women with no evidence of endometriosis), without significant clinical differences between the two groups. A subset of women from both groups was treated with a gonadotropin-releasing hormone agonist (GnRHa) for 4–6 months before collecting uterine and vaginal samples. Both groups, with or without endometriosis, had 16 types of anaerobic and aerobic bacteria and yeast colonizing the vagina. However, notably, subclinical uterus infection was more common in endometriosis patients than controls and much more common in those treated with GnRHa than in those who did not receive GnRHa (Khan et al., 2014). This was attributed to the presumption that GnRHa alters the intrauterine environment, thus increasing uterine infection in women with endometriosis. There was a significant increase in the diagnosis of endometritis in the GnRHa-treated women than untreated women, with or without endometriosis, due to the increased endometrial colonization rate caused by GnRHa. Higher abundances of multiple bacteria (including Staphylococcaceae, Moraxellaceae, Lactobacillacae, Streptococcaceae, and Enterobacteriaceae) were detected in endometrial swabs from GnRHa-treated women than untreated women, with or without endometriosis. Streptococcaceae and Staphylococcaceae increased and Lactobacillacae decreased in GnRHa-treated compared to untreated endometriosis patients, indicating that low estrogen due to GnRHa increases intrauterine infection, thus causing endometrial dysbiosis (Khan et al., 2014). Microbes from the vaginal area can move to and colonize the endometrium (where they are usually exposed to a different environment) (Khan et al., 2014). The increased intrauterine colonization in endometriosis patients was hypothesized to be attributable to increased prostaglandin E2 (PGE2), which caused immunosuppression that enabled bacterial survival and replication. In support of this hypothesis, PGE2-mediated immunosuppression of lymphocytes is higher in women with endometriosis than those without endometriosis (Khan et al., 2014). Notably, the presence of pathogenic bacteria (such as Escherichia coli) and enhanced bacterial colonization in women with endometriosis caused subclinical endometritis and further promoted endometriosis severity and/or complications (Khan et al., 2014). As sex steroids (such as estrogen) modulate the number of antimicrobial agents [such as defensins and secretory leukocyte protease inhibitor (SLPI)] in the endometrium and fallopian tubes (Figure 3A) (Khan et al., 2014), altering estrogen levels can lead to intrauterine microbial infection and/or tissue inflammation, leading to dysbiosis (Figure 3B). These studies concluded that altering estrogen levels triggers inflammatory responses in the endometrial tissues, leading to microbial colonization of the uterus, endometriosis, and endometritis (Khan et al., 2014).
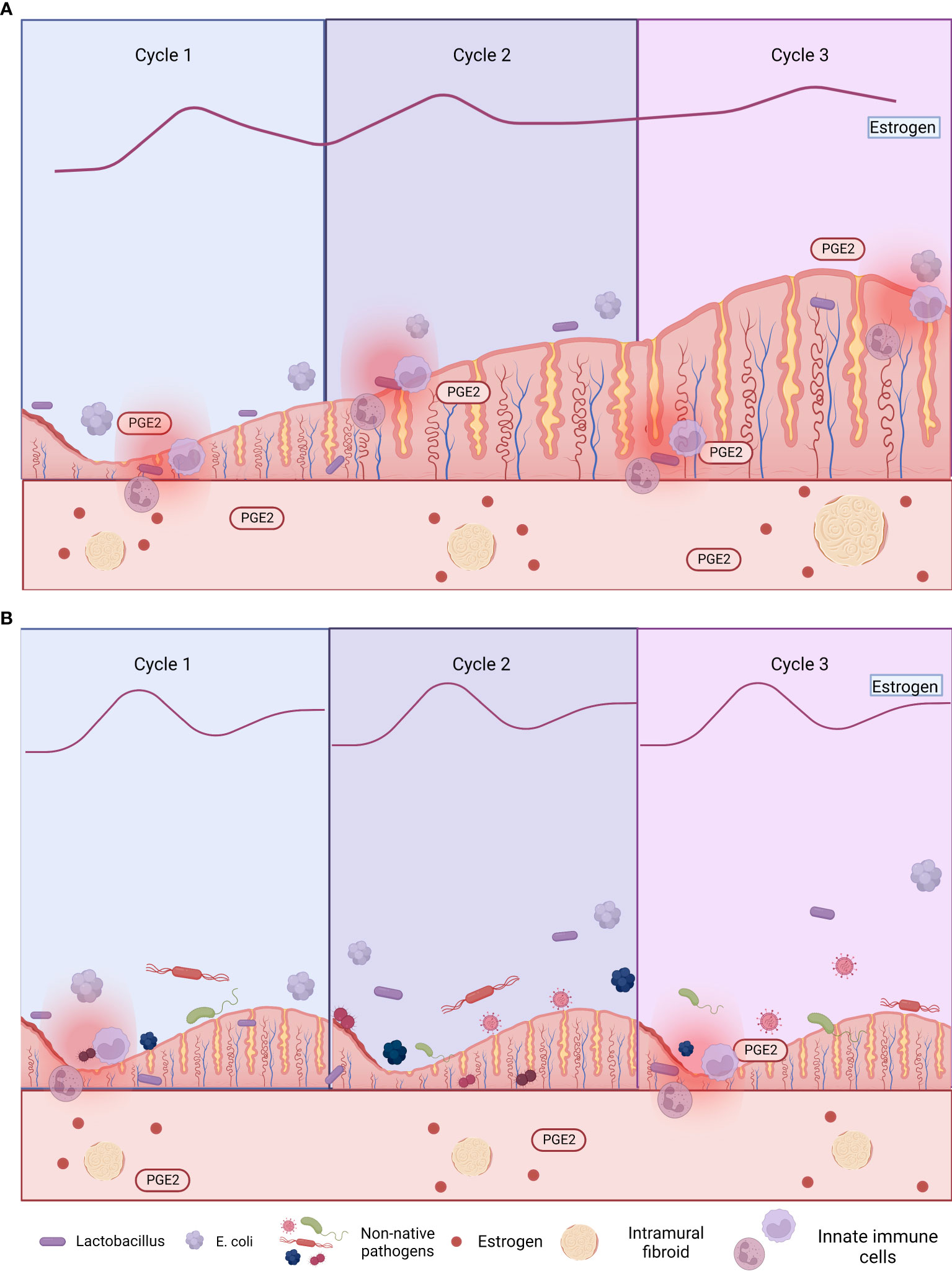
Figure 3 Comorbid endometriosis and uterine fibroids that are (A) untreated and (B) treated with gonadotropin-releasing hormone agonist (GnRHa). Excess estrogen exposure is associated with both endometriosis and uterine fibroids (UFs), so estrogen is a target of multiple treatments (e.g., GnRHa and aromatase inhibitors). However, these treatments have local immunosuppressive effects, increasing susceptibility to uterine dysbiosis and subsequent endometriosis (Khan et al., 2014; Qu et al., 2021). Figures are created using BioRender.com.
4.5. Uterine fibroids
UFs or leiomyomas are benign monoclonal tumors of the uterus derived from uterine smooth muscle (myometrium). They affect >70% of women of reproductive age worldwide, especially women of color (Wise and Laughlin-Tommaso, 2016; Al-Hendy et al., 2017). UFs significantly impact patients’ health, fertility, and economic status. In the United States, the annual financial burden of UF treatment is estimated to have reached $34.4 billion (Giuliani et al., 2020). There is a wide range of invasive and noninvasive treatment options, from short-term pharmacotherapies to laparoscopic or open myomectomy or hysterectomy (Istre, 2008; Yang et al., 2022). The treatment depends on the tumor size, tumor location, age, and reproductive plans. Epidemiological studies indicate that African American women develop UFs more frequently and at an earlier age, and the tumors tend to be more aggressive, growing larger and causing more significant symptoms than in Caucasian women (Al-Hendy and Salama, 2006; Eltoukhi et al., 2014; Stewart et al., 2016).
A characteristic feature of UFs is their dependence on the ovarian steroid hormones estrogen and progesterone (Elkafas et al., 2017). These hormones stimulate complex paracrine signals, which allow UF cells to secrete mitogenic stimuli and thereby influence adjacent immature UF cells, thus providing UF cells with undifferentiated cells that can support tumor growth (Ono et al., 2013; Reis et al., 2016). Estradiol increases the sensitivity of tissues to progesterone by increasing the availability of progesterone receptors (PRs) (McGuire et al., 1977; Mote et al., 2002; Elkafas et al., 2017). Selective PR modulators (SPRMs) are synthetic steroid ligands designed to modulate the PR site in a tissue-specific way; binding of SPRMs to PRs can cause agonistic effects, antagonistic effects [e.g., they can compete with agonists or enhance the effects of co-repressors to cause antagonistic effects (Islam et al., 2020)], or mixed effects. They can induce receptor dimerization and DNA binding and coordinate with co-activators and/or co-repressors (Madauss et al., 2007). Asoprisnil and ulipristal acetate are SPRMs, while raloxifene is a selective ER modulator that suppresses UF growth (ElKafas et al., 2018). Research using these compounds can help to study estrogen- and progesterone-dependent gynecological disorders.
In recent years, high-throughput sequencing has been used to study the gut microbiome diversity in UF patients. A case–control study examined the differences in follicle-stimulating hormone (FSH), estradiol (E2), and anti-Mullerian hormone (AMH) before and after transabdominal hysterectomy for UF patients and the effect of transabdominal hysterectomy on the gut microbiome (Wang et al., 2020). The results indicated that FSH increased after surgery, while E2 and AMH decreased. The ovaries are involved in hormone secretion and ovulation, and the study concluded that transabdominal hysterectomy altered ovary function, leading to decreased E2 and AMH and increased FSH. This decreased estrogen affected the gut microbiome diversity, with increased Proteobacteria and Firmicutes and decreased Bacteroidetes (Wang et al., 2020; Yang et al., 2022).
5. Vitamin D deficiency and its link to gut dysbiosis and uterine fibroids
Diet and nutrition are key factors that maintain microbiome homeostasis in the body. Vitamin D is an essential nutrient for microbiome homeostasis. Vitamin D receptors (VDRs) are widely expressed in the intestines, immune system (Liu et al., 2018), uterus, and liver (Zinser et al., 2005; Clark and Mach, 2016). Vitamin D levels not only affect the gut microbiome but also impact the airway microbiome (Robles-Vera et al., 2019).
According to the current UF pathogenesis theory, vitamin D is one of the most significant contributing factors to the development of this disease (Sharan et al., 2011; Ciebiera et al., 2018). Reduced serum vitamin D is a risk factor for the development of UFs (Brakta et al., 2015; Elkafas et al., 2021). Epidemiological studies show that African American women have lower vitamin D levels than Caucasian women (Laughlin et al., 2010). Among infertile patients with various ethnicities, UF patients had lower vitamin D than women without UFs, suggesting that vitamin D deficiency in UF patients can occur independently of ethnicity (Sabry et al., 2013). Vitamin D level is inversely proportional to UF volume, regardless of ethnicity (Sabry et al., 2013). A study on the effect of low VDR levels on the pathogenesis of UFs indicated that 60% of women with UFs had low VDR levels (Halder et al., 2013a). The authors noted that inadequate or loss of VDR is a risk factor for UF pathogenesis (Halder et al., 2013b).
The effect of vitamin D on gut microbiota diversity has been examined in several studies. A study reported that vitamin D supplementation increased the overall diversity of the gut microbiota, which involved increasing Bacteroidetes and decreasing Firmicutes (thereby improving the Firmicutes/Bacteroidetes ratio) and increasing the phyla Verrucomicrobia and Actinobacteria (Singh et al., 2020). Akkermansia muciniphila, which belongs to the phylum Verrucomicrobia, assists in maintaining host intestinal homeostasis by transforming mucin into beneficial by-products, thus maintaining gut homeostasis. The abundance of A. muciniphila is negatively associated with body mass, inflammation, and metabolic syndrome (Singh et al., 2020). In 2015, Wu et al. (2015) placed fecal communities into two enterotypes based on Prevotella and Bacteroides levels. They observed that vitamin D intake increased Bacteroides and decreased Prevotella (Wu et al., 2015), which was a previously reported benefit of vitamin D (Singh et al., 2020). It was noted in the studies by Wu et al. (2015) and Singh et al. (2020) that vitamin D is essential in maintaining gut microbiome homeostasis, and deficiency causes gut dysbiosis.
Vitamin D aids in gut microbiome homeostasis by activating VDRs in the proximal colon. VDRs are transcription factors that regulate the expression of over 1,000 host genes (Singh et al., 2020). These genes are involved in gut microbiome homeostasis, inflammation, prevention of pathogen invasion, and commensal bacterial colonization. The interactions between VDR signaling and the gut microbiome affect host immune responses and inflammation (Singh et al., 2019). Vitamin D has been considered a treatment option for UFs. Arjeh et al. (2020) conducted a randomized controlled trial on the effect of oral vitamin D supplementation on UF growth. They concluded that as vitamin D deficiency causes dysbiosis that leads to the pathogenesis of UFs, vitamin D supplementation could be a treatment option for UFs (Arjeh et al., 2020). The 12-week randomized controlled trial in UF patients showed no significant difference in the volume of fibroids in the oral vitamin D group despite an increase in the placebo group (Arjeh et al., 2020). It was concluded that vitamin D inhibits UF cell proliferation via its indirect effects on the gut microbiome (Arjeh et al., 2020).
VDRs inhibit bacteria-induced pro-inflammatory nuclear factor NF-κB activity in the gut (Wu et al., 2015). Supplementation with 25 µg/kg vitamin D in pregnant mice before a 100-µg/kg lipopolysaccharide injection activated VDR signaling, inhibited the pro-inflammatory NF-κB p65 pathway, and decreased the expression of the inflammatory cytokines TNF-α, IL-Iβ, and IL-6 (Clark and Mach, 2016). VDRs maintain the intestinal barrier function by decreasing intestinal permeability, dysbiosis, inflammation, and lack of immune tolerance in the gut (Cantorna et al., 2014). Accordingly, VDR-knockout mice are more sensitive to lipopolysaccharide-induced endotoxemia, with increased expression of inflammatory cytokines [e.g., TNF-α, interferon IFN-γ, IL-1a, IL-1β, IL-21, and IL-10] and more weight loss, bleeding, ulceration, septic shock, and death compared to control mice (Froicu and Cantorna, 2007). In autoimmune patients, vitamin D3 may directly interact with the gut microbiota and reduce dysbiosis (Ananthakrishnan et al., 2014). In a cohort of 3,188 inflammatory bowel disease patients, higher plasma vitamin D3 (27.1 ng/ml) was associated with a remarkably decreased risk of Clostridium difficile infection (Ananthakrishnan et al., 2014). A study of multiple sclerosis (MS) patients revealed that oral administration of 5,000 IU of vitamin D3 daily for 90 days increased Akkermansia, which stimulates immune tolerance, and increased Faecalibacterium and Coprococcus, which both generate butyrate, a short-chain fatty acid with anti-inflammatory, antiproliferative, and apoptotic effects (Cantarel et al., 2015). A case series of seven relapsing-remitting MS (RRMS) patients demonstrated that vitamin D supplementation increased Enterobacteria in both healthy controls and RRMS patients, with shifts in Firmicutes, Actinobacteria, and Proteobacteria in the RRMS patients (Mielcarz and Kasper, 2015). The vitamin D/VDR complex increases innate immune cells’ chemotactic and phagocytic capabilities and activates the transcription of antimicrobial peptides in several cell types, including colonic cells (Lagishetty et al., 2010). Vitamin D induced the expression of antibacterial peptides cathelicidin (hCAP18) and human β-defensin-2 (HBD-2), which are expressed in breast milk and the intestinal mucosa and are involved in innate immunity, promoting numerous functions such as chemotaxis of inflammatory immune cells and microbicidal action (Wampach et al., 2017).
Mice fed a vitamin D-deficient diet exhibited a pre-fibroid status in the myometrium marked by increased expression of sex steroid receptors and proliferation-related genes, high DNA damage, and fibrosis progression (ElHusseini et al., 2018). There was also higher inflammation and immunosuppression in the vitamin D-deficient mice (due to increased regulatory T cells in the myometrium) compared to the controls (ElHusseini et al., 2018). The same research group reported a preliminary study on Eker rats of the effect of early-life exposure to EDCs on myometrium stem cells. They found a significant increase in inflammatory mediators (IL-6, IL-17, IL-1β, IL-1α, TNF-α, and NF-κB) produced by the exposed MMSCs compared to unexposed myometrial stem cells (MMSCs) (Elkafas et al., 2019). Vitamin D reversed these inflammatory mediators (lowering IL-6, IL-17, IL-1β, IL-1α, TNF-α, and NF-κB) and enhanced the anti-inflammatory effect by increasing IL-4 and IL-10 (Elkafas et al., 2019). Concluding that, the gut microbiome may be involved in inflammation via the endobolome, and vitamin D deficiency decreases Akkermansia, which reduces immune tolerance, and increases Firmicutes, which increases free estrogen. Thus, vitamin D is an essential nutrient that maintains the homeostasis of the gut microbiome (Figure 4).
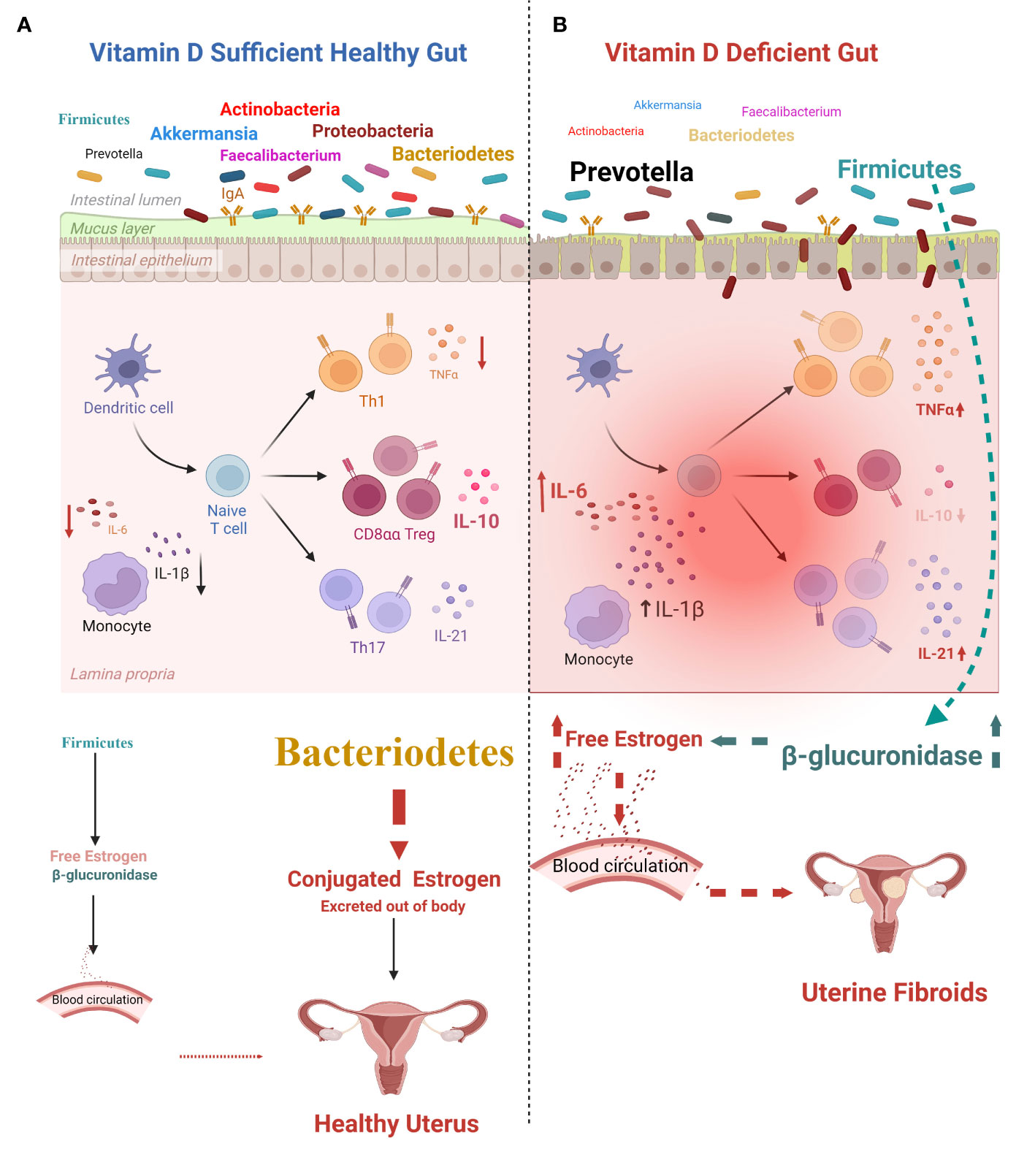
Figure 4 Effects of vitamin D on the gut and estrogen metabolism. (A) Effect of vitamin D sufficiency: healthy gut. Dominant gut bacteria (including Bacteroidetes) are anti-inflammatory, preserve gut homeostasis, and maintain estrogen metabolite levels, decreasing the incidence of uterine diseases. (B) Effect of vitamin D deficiency: pathological gut. Lower vitamin D level leads to gut inflammation with increased release of interleukin (IL)-1β, IL-6, tumor necrosis factor (TNF)-α, and IL-21, which causes gut dysbiosis (involving an increase in the Firmicutes/Bacteroidetes ratio) followed by an increase in the release of unbound estrogen into the circulation. This increases the incidence of uterine fibroids (UFs). Figure created using BioRender.com.
6. Conclusions
Conditions such as BV, cervical and endometrial cancer, PCOS, postmenopausal syndrome, endometriosis, endometritis, UFs, and bacterial infections negatively affect women’s reproductive health and quality of life. The human microbiota plays a vital role in maintaining women’s overall health and preventing these conditions. The human microbiome’s composition and function are critical in understanding the pathogenesis of these diseases. Several studies over the last 20 years have demonstrated a strong association between the microbiome and chronic gynecological disorders. These disorders have come to be better understood by investigating associated disturbances in the uterine, gut, and vaginal microbiome, and future studies may involve therapeutic manipulation of the human microbiome, as in the case of vitamin D supplementation for UF prevention and treatment. Further research is required to fully understand the role of the microbiome and its interactions with the human host in the prevention, development, and treatment of genital tract disorders.
Author contributions
NI and HE designed the scope and organization of the review. HE conducted literature reviews, constructed figures, and contributed to the writing of most of the manuscript. MW edited and summarized some sections, create one figure and write the abstract. NI and AA-H supervised the writing and critically edited and reviewed the complete manuscript and figures. All authors contributed to the article and approved the submitted version.
Funding
This manuscript was funded by NIH R01 HD094378-02 to AA-H.
Conflict of interest
The authors declare that the research was conducted in the absence of any commercial or financial relationships that could be construed as a potential conflict of interest.
Publisher’s note
All claims expressed in this article are solely those of the authors and do not necessarily represent those of their affiliated organizations, or those of the publisher, the editors and the reviewers. Any product that may be evaluated in this article, or claim that may be made by its manufacturer, is not guaranteed or endorsed by the publisher.
References
Aguilera, M., Gálvez-Ontiveros, Y., Rivas, A. (2020). Endobolome, a new concept for determining the influence of microbiota disrupting chemicals (MDC) in relation to specific endocrine pathogenesis. Front. Microbiol. 11, 578007. doi: 10.3389/fmicb.2020.578007
Al-Hendy, A., Myers, E. R., Stewart, E. (2017). Uterine fibroids: burden and unmet medical need. Semin. Reprod. Med. 35 (6), 473–480. doi: 10.1055/s-0037-1607264
Al-Hendy, A., Salama, S. A. (2006). Ethnic distribution of estrogen receptor-α polymorphism is associated with a higher prevalence of uterine leiomyomas in black americans. Fertil. Steril. 86 (3), 686–693. doi: 10.1016/j.fertnstert.2006.01.052
Ananthakrishnan, A. N., Cagan, A., Gainer, V. S., Cheng, S. C., Cai, T., Szolovits, P., et al. (2014). Higher plasma vitamin d is associated with reduced risk of Clostridium difficile infection in patients with inflammatory bowel diseases. Aliment. Pharmacol. Ther. 39 (10), 1136–1142. doi: 10.1111/apt.12706
Anderson, G. (2019). Endometriosis pathoetiology and pathophysiology: roles of vitamin a, estrogen, immunity, adipocytes, gut microbiome and melatonergic pathway on mitochondria regulation. Biomol. Concepts 10 (1), 133–149. doi: 10.1515/bmc-2019-0017
Arjeh, S., Darsareh, F., Asl, Z. A., Kutenaei, M. A. (2020). Effect of oral consumption of vitamin d on uterine fibroids: a randomized clinical trial. Complementary therapies Clin. Pract. 39, 101159. doi: 10.1016/j.ctcp.2020.101159
Armstrong, H., Bording-Jorgensen, M., Dijk, S., Wine, E. (2018). The complex interplay between chronic inflammation, the microbiome, and cancer: understanding disease progression and what we can do to prevent it. Cancers 10 (3), 83. doi: 10.3390/cancers10030083
Ata, B., Yildiz, S., Turkgeldi, E., Brocal, V. P., Dinleyici, E. C., Moya, A., et al. (2019). The endobiota study: comparison of vaginal, cervical, and gut microbiota between women with stage 3/4 endometriosis and healthy controls. Sci. Rep. 9 (1), 1–9. doi: 10.1038/s41598-019-39700-6
Bailey, M. T., Coe, C. L. (2002). Endometriosis is associated with an altered profile of intestinal microflora in female rhesus monkeys. Hum. Reprod. 17 (7), 1704–1708. doi: 10.1093/humrep/17.7.1704
Baker, J. M., Al-Nakkash, L., Herbst-Kralovetz, M. M. (2017). Estrogen–gut microbiome axis: physiological and clinical implications. Maturitas 103, 45–53. doi: 10.1016/j.maturitas.2017.06.025
Baker, J. M., Chase, D. M., Herbst-Kralovetz, M. M. (2018). Uterine microbiota: residents, tourists, or invaders? Frontiers in immunology, 9, 208.
Bodelon, C., Madeleine, M. M., Johnson, L. G., Du, Q., Galloway, D. A., Malkki, M., et al. (2014). Genetic variation in the TLR and NF-KB pathways and cervical and vulvar cancer risk: a population-based case-control study. Int. J. Cancer. 134 (2), 437–444. doi: 10.1002/ijc.28364
Borella, F., Carosso, A. R., Cosma, S., Preti, M., Collemi, G., Cassoni, P., et al. (2021). Gut microbiota and gynecological cancers: a summary of pathogenetic mechanisms and future directions. ACS Infect. Dis. 7 (5), 987–1009. doi: 10.1021/acsinfecdis.0c00839
Brakta, S., Diamond, J. S., Al-Hendy, A., Diamond, M. P., Halder, S. K. (2015). Role of vitamin d in uterine fibroid biology. Fertility sterility 104 (3), 698–706. doi: 10.1016/j.fertnstert.2015.05.031
Brooks, R. A., Fleming, G. F., Lastra, R. R., Lee, N. K., Moroney, J. W., Son, C. H., et al. (2019). Current recommendations and recent progress in endometrial cancer. CA: Cancer J. Clin. 69 (4), 258–279. doi: 10.3322/caac.21561
Burac, M. (2018). Characterization of the human endometrial microbiome and its risks associated in infertility patients: a systematic review. In: MedEsperaInternational Medical Congress for Students and Young Doctors. 7, 3-5 mai 2018, Chişinău. Chisinau, Republic of Moldova: 2018, pp. 74–75.
Cantarel, B. L., Waubant, E., Chehoud, C., Kuczynski, J., DeSantis, T. Z., Warrington, J., et al. (2015). Gut microbiota in multiple sclerosis: possible influence of immunomodulators. J. Invest. Med. 63 (5), 729–734. doi: 10.1097/JIM.0000000000000192
Cantorna, M. T., McDaniel, K., Bora, S., Chen, J., James, J. (2014). Vitamin d, immune regulation, the microbiota, and inflammatory bowel disease. Exp. Biol. Med. 239 (11), 1524–1530. doi: 10.1177/1535370214523890
Caselli, E., Soffritti, I., D’Accolti, M., Piva, I., Greco, P., Bonaccorsi, G. (2019). Atopobium vaginae and Porphyromonas somerae induce proinflammatory cytokines expression in endometrial cells: a possible implication for endometrial cancer? Cancer Manage. Res. 11, 8571. doi: 10.2147/CMAR.S217362
Chadchan, S. B., Singh, V., Kommagani, R. (2022). Female reproductive dysfunctions and the gut microbiota. J. Mol. Endocrinol. 69 (3), R81–R94. doi: 10.1530/JME-21-0238
Chambers, L. M., Bussies, P., Vargas, R., Esakov, E., Tewari, S., Reizes, O., et al. (2021). The microbiome and gynecologic cancer: current evidence and future opportunities. Curr. Oncol. Rep. 23 (8), 1–12. doi: 10.1007/s11912-021-01079-x
Champer, M., Wong, A. M., Champer, J., Brito, I. L., Messer, P. W., Hou, J. Y., et al. (2018). The role of the vaginal microbiome in gynaecological cancer. BJOG 125 (3), 309–315. doi: 10.1111/1471-0528.14631
Chen, C., Song, X., Wei, W., Zhong, H., Dai, J., Lan, Z., et al. (2017). The microbiota continuum along the female reproductive tract and its relation to uterine-related diseases. Nat. Commun. 8 (1), 1–11. doi: 10.1038/s41467-017-00901-0
Ciebiera, M., Włodarczyk, M., Ciebiera, M., Zaręba, K., Łukaszuk, K., Jakiel, G. (2018). Vitamin d and uterine fibroids–review of the literature and novel concepts. Int. J. Mol. Sci. 19 (7), 2051. doi: 10.3390/ijms19072051
Clark, A., Mach, N. (2016). Role of vitamin d in the hygiene hypothesis: the interplay between vitamin d, vitamin d receptors, gut microbiota, and immune response. Front. Immunol. 7, 627. doi: 10.3389/fimmu.2016.00627
Dalal, P. K., Agarwal, M. (2015). Postmenopausal syndrome. Indian J. Psychiatry 57 (Suppl 2), S222. doi: 10.4103/0019-5545.161483
Darbre, P. D. (2017). Endocrine disruptors and obesity. Curr. Obes. Rep. 6 (1), 18–27. doi: 10.1007/s13679-017-0240-4
Dewi, F. N., Wood, C. E., Lampe, J. W., Hullar, M. A., Franke, A. A., Golden, D. L., et al. (2012). Endogenous and exogenous equol are antiestrogenic in reproductive tissues of apolipoprotein e-null mice. J. Nutr. 142 (10), 1829–1835. doi: 10.3945/jn.112.161711
Diamanti-Kandarakis, E., Bourguignon, J. P., Giudice, L. C., Hauser, R., Prins, G. S., Soto, A. M., et al. (2009). Endocrine-disrupting chemicals: an endocrine society scientific statement. Endocrine Rev. 30 (4), 293–342. doi: 10.1210/er.2009-0002
Doaa, M., Dalia, M., Ahmed, F. S. (2016). Gut bacterial microbiota in psoriasis: a case control study. Afr. J. Microbiol. Res. 10 (33), 1337–1343. doi: 10.5897/AJMR2016.8046
Dominguez-Bello, M. G., Costello, E. K., Contreras, M., Magris, M., Hidalgo, G., Fierer, N., et al. (2010). Delivery mode shapes the acquisition and structure of the initial microbiota across multiple body habitats in newborns. Proc. Natl. Acad. Sci. 107 (26), 11971–11975. doi: 10.1073/pnas.1002601107
ElHusseini, H., Elkafas, H., Abdelaziz, M., Halder, S., Atabiekov, I., Eziba, N., et al. (2018). Diet-induced vitamin d deficiency triggers inflammation and DNA damage profile in murine myometrium. Int. J. women’s Health 10, 503. doi: 10.2147/IJWH.S163961
ElKafas, H., Ali, M., Al-Hendy, A. (2018). “Leiomyomas,” in Encyclopedia of reproduction, 2nd ed. Ed. Skinner, M. K. (Oxford: Academic Press), 101–105.
Elkafas, H. E., Badary, O. A., Elmorsy, E., Kamel, R., Al-Hendy, A., Yang, Q. (2019). Targeting activated pro-inflammatory pathway in primed myometrial stem cells with vitamin D3 and paricalcitol. Fertility Sterility 112 (3), e100–e101. doi: 10.1016/j.fertnstert.2019.07.382
Elkafas, H. E., Badary, O. A., Elmorsy, E., Kamel, R., Yang, Q., Al-Hendy, A. (2021). Endocrine-Disrupting Chemicals and Vitamin D Deficiency in the Pathogenesis of Uterine Fibroids. Journal of advanced pharmacy research, 5(2), 260–275.
Elkafas, H., Qiwei, Y., Al-Hendy, A. (2017). Origin of uterine fibroids: conversion of myometrial stem cells to tumor-initiating cells. Semin. Reprod. Med. 35 (6), 481–486. doi: 10.1055/s-0037-1607205
Eltoukhi, H. M., Modi, M. N., Weston, M., Armstrong, A. Y., Stewart, E. A. (2014). The health disparities of uterine fibroid tumors for African American women: a public health issue. Am. J. obstetrics gynecology 210 (3), 194–199. doi: 10.1016/j.ajog.2013.08.008
Eslami-S, Z., Majidzadeh-A, K., Halvaei, S., Babapirali, F., Esmaeili, R. (2020). Microbiome and breast cancer: new role for an ancient population. Front. Oncol. 10, 120. doi: 10.3389/fonc.2020.00120
Evans, C. C., LePard, K. J., Kwak, J. W., Stancukas, M. C., Laskowski, S., Dougherty, J., et al. (2014). Exercise prevents weight gain and alters the gut microbiota in a mouse model of high fat diet-induced obesity. PLos One 9 (3), e92193. doi: 10.1371/journal.pone.0092193
Flores, R., Shi, J., Gail, M. H., Gajer, P., Ravel, J., Goedert, J. J. (2012). Association of fecal microbial diversity and taxonomy with selected enzymatic functions. PLos One 7 (6), e39745. doi: 10.1371/journal.pone.0039745
Franasiak, J. M., Scott, R. T., Jr. (2015). Introduction: microbiome in human reproduction. Fertility sterility 104 (6), 1341–1343. doi: 10.1016/j.fertnstert.2015.10.021
Franasiak, J. M., Werner, M. D., Juneau, C. R., Tao, X., Landis, J. N., Zhan, Y., et al. (2016). Endometrial microbiome at the time of embryo transfer: next-generation sequencing of the 16S ribosomal subunit. J. assisted Reprod. Genet. 33 (1), 129–136. doi: 10.1007/s10815-015-0614-z
Froicu, M., Cantorna, M. T. (2007). Vitamin d and the vitamin d receptor are critical for control of the innate immune response to colonic injury. BMC Immunol. 8 (1), 1–11. doi: 10.1186/1471-2172-8-5
Fuhrman, B. J., Feigelson, H. S., Flores, R., Gail, M. H., Xu, X., Ravel, J., et al. (2014). Associations of the fecal microbiome with urinary estrogens and estrogen metabolites in postmenopausal women. J. Clin. Endocrinol. Metab. 99 (12), 4632–4640. doi: 10.1210/jc.2014-2222
Gilbert, J. A., Blaser, M. J., Caporaso, J. G., Jansson, J. K., Lynch, S. V., Knight, R. (2018). Current understanding of the human microbiome. Nat. Med. 24 (4), 392–400. doi: 10.1038/nm.4517
Giuliani, E., As-Sanie, S., Marsh, E. E. (2020). Epidemiology and management of uterine fibroids. Int. J. Gynecology Obstetrics. 149 (1), 3–9. doi: 10.1002/ijgo.13102
Graham, M. E., Herbert, W. G., Song, S. D., Raman, H. N., Zhu, J. E., Gonzalez, P. E., et al. (2021). Gut and vaginal microbiomes on steroids: implications for women’s health. Trends Endocrinol. Metab. 32 (8), 554–565. doi: 10.1016/j.tem.2021.04.014
Guo, Y., Qi, Y., Yang, X., Zhao, L., Wen, S., Liu, Y., et al. (2016). Association between polycystic ovary syndrome and gut microbiota. PLos One 11 (4), e0153196. doi: 10.1371/journal.pone.0153196
Gupta, S., Kakkar, V., Bhushan, I. (2019). Crosstalk between vaginal microbiome and female health: a review. Microbial pathogenesis 136, 103696. doi: 10.1016/j.micpath.2019.103696
Halder, S. K., Osteen, K. G., Al-Hendy, A. (2013a). 1, 25-dihydroxyvitamin d3 reduces extracellular matrix-associated protein expression in human uterine fibroid cells. Biol. Reprod. 89(6) 150, 151–113. doi: 10.1095/biolreprod.113.107714
Halder, S. K., Osteen, K. G., Al-Hendy, A. (2013b). Vitamin D3 inhibits expression and activities of matrix metalloproteinase-2 and-9 in human uterine fibroid cells. Hum. Reprod. 28 (9), 2407–2416. doi: 10.1093/humrep/det265
Hasan, N., Yang, H. (2019). Factors affecting the composition of the gut microbiota, and its modulation. PeerJ. 7, e7502. doi: 10.7717/peerj.7502
Hernandes, C., Silveira, P., Rodrigues Sereia, A. F., Christoff, A. P., Mendes, H., Valter de Oliveira, L. F., et al. (2020). Microbiome profile of deep endometriosis patients: comparison of vaginal fluid, endometrium and lesion. Diagnostics 10 (3), 163. doi: 10.3390/diagnostics10030163
Hong, X., Qin, P., Huang, K., Ding, X., Ma, J., Xuan, Y., et al. (2020). Association between polycystic ovary syndrome and the vaginal microbiome: a case-control study. Clin. Endocrinol. 93 (1), 52–60. doi: 10.1111/cen.14198
Islam, M. S., Afrin, S., Jones, S. I., Segars, J. (2020). Selective progesterone receptor modulators–mechanisms and therapeutic utility. Endocrine Rev. 41 (5), 643–694. doi: 10.1210/endrev/bnaa012
Istre, O. (2008). Management of symptomatic fibroids: conservative surgical treatment modalities other than abdominal or laparoscopic myomectomy. Best Pract. Res. Clin. Obstetrics Gynaecology 22 (4), 735–747. doi: 10.1016/j.bpobgyn.2008.01.010
Jiang, I., Yong, P. J., Allaire, C., Bedaiwy, M. A. (2021). Intricate connections between the microbiota and endometriosis. Int. J. Mol. Sci. 22 (11), 5644. doi: 10.3390/ijms22115644
Khan, K. N., Fujishita, A., Kitajima, M., Hiraki, K., Nakashima, M., Masuzaki, H. (2014). Intra-uterine microbial colonization and occurrence of endometritis in women with endometriosis. Hum. Reprod. 29 (11), 2446–2456. doi: 10.1093/humrep/deu222
Kiecka, A., Macura, B., Szczepanik, M. (2021). Can Lactobacillus spp. be a factor reducing the risk of miscarriage? Polish J. Microbiol. 70 (4), 431. doi: 10.33073/pjm-2021-043
Kitaya, K., Takeuchi, T., Mizuta, S., Matsubayashi, H., Ishikawa, T. (2018). Endometritis: new time, new concepts. Fertility sterility. 110 (3), 344–350. doi: 10.1016/j.fertnstert.2018.04.012
Kokcu, A. (2011). Relationship between endometriosis and cancer from current perspective. Arch. gynecology obstetrics. 284 (6), 1473–1479. doi: 10.1007/s00404-011-2047-y
Lagishetty, V., Chun, R. F., Liu, N. Q., Lisse, T. S., Adams, J. S., Hewison, M. (2010). 1α-hydroxylase and innate immune responses to 25-hydroxyvitamin d in colonic cell lines. J. Steroid Biochem. Mol. Biol. 121 (1-2), 228–233. doi: 10.1016/j.jsbmb.2010.02.004
Łaniewski, P., Ilhan, Z. E., Herbst-Kralovetz, M. M. (2020). The microbiome and gynaecological cancer development, prevention and therapy. Nat. Rev. Urol. 17 (4), 232–250. doi: 10.1038/s41585-020-0286-z
Laschke, M. W., Menger, M. D. (2016). The gut microbiota: a puppet master in the pathogenesis of endometriosis? Am. J. Obstet. Gynecol. 215 (1), 68.e1–68.e4. doi: 10.1016/j.ajog.2016.02.036
Laughlin, S. K., Schroeder, J. C., Baird, D. D. (2010). New directions in the epidemiology of uterine fibroids. Semin. Reprod. Med. 28 (3), 204–217. doi: 10.1055/s-0030-1251477
Liu, Y., Ko, E. Y. L., Wong, K. K. W., Chen, X., Cheung, W. C., Law, T. S. M., et al. (2019). Endometrial microbiota in infertile women with and without chronic endometritis as diagnosed using a quantitative and reference range-based method. Fertility Sterility. 112 (4), 707–717. doi: 10.1016/j.fertnstert.2019.05.015
Liu, R., Zhang, C., Shi, Y., Zhang, F., Li, L., Wang, X., et al. (2017). Dysbiosis of gut microbiota associated with clinical parameters in polycystic ovary syndrome. Front. Microbiol. 8, 324. doi: 10.3389/fmicb.2017.00324
Liu, W., Zhang, L., Xu, H. J., Li, Y., Hu, C. M., Yang, J. Y., et al. (2018). The anti-inflammatory effects of vitamin d in tumorigenesis. Int. J. Mol. Sci. 19 (9), 2736. doi: 10.3390/ijms19092736
Lu, W., He, F., Lin, Z., Liu, S., Tang, L., Huang, Y., et al. (2021). Dysbiosis of the endometrial microbiota and its association with inflammatory cytokines in endometrial cancer. Int. J. Cancer. 148 (7), 1708–1716. doi: 10.1002/ijc.33428
Madauss, K. P., Stewart, E. L., Williams, S. P. (2007). The evolution of progesterone receptor ligands. Medicinal Res. Rev. 27 (3), 374–400. doi: 10.1002/med.20083
Madere, F. S., Monaco, C. L. (2022). The female reproductive tract virome: understanding the dynamic role of viruses in gynecological health and disease. Curr. Opin. Virology. (Elsevier) 52, 15–23. doi: 10.1016/j.coviro.2021.10.010
Maynard, C. L. (2019). “The microbiota in immunity and inflammation,” in Clinical immunology. Eds. Rich, R. R., Fleisher, T. A., Shearer, W. T., Schroeder, H. W., Frew, A. J., Weyand. Elsevier, C. M., 207–219.
McGuire, W. L., Horwitz, K. B., Pearson, O. H., Segaloff, A. (1977). Current status of estrogen and progesterone receptors in breast cancer. Cancer 39 (6), 2934–2947. doi: 10.1002/1097-0142(197706)39:6<2934::AID-CNCR2820390680>3.0.CO;2-P
Mielcarz, D. W., Kasper, L. H. (2015). The gut microbiome in multiple sclerosis. Curr. Treat options Neurol. 17 (4), 1–10. doi: 10.1007/s11940-015-0344-7
Miles, S. M., Hardy, B. L., Merrell, D. S. (2017). Investigation of the microbiota of the reproductive tract in women undergoing a total hysterectomy and bilateral salpingo-oopherectomy. Fertility sterility 107 (3), 813–820. doi: 10.1016/j.fertnstert.2016.11.028
Miller, E. A., Beasley, D. E., Dunn, R. R., Archie, E. A. (2016). Lactobacilli dominance and vaginal pH: why is the human vaginal microbiome unique? Front. Microbiol. 7, 1936. doi: 10.3389/fmicb.2016.01936
Mirmonsef, P., Gilbert, D., Zariffard, M. R., Hamaker, B. R., Kaur, A., Landay, A. L., et al. (2011). The effects of commensal bacteria on innate immune responses in the female genital tract. Am. J. Reprod. Immunol. 65 (3), 190–195. doi: 10.1111/j.1600-0897.2010.00943.x
Mitchell, C. M., Haick, A., Nkwopara, E., Garcia, R., Rendi, M., Agnew, K., et al. (2015). Colonization of the upper genital tract by vaginal bacterial species in nonpregnant women. Am. J. obstetrics gynecology 212 (5), 611. e1. doi: 10.1016/j.ajog.2014.11.043
Moreno, I., Codoñer, F. M., Vilella, F., Valbuena, D., Martinez-Blanch, J. F., Jimenez-Almazán, J., et al. (2016). Evidence that the endometrial microbiota has an effect on implantation success or failure. Am. J. obstetrics gynecology 215 (6), 684–703. doi: 10.1016/j.ajog.2016.09.075
Moreno, I., Garcia-Grau, I., Perez-Villaroya, D., Gonzalez-Monfort, M., Bahçeci, M., Barrionuevo, M. J., et al. (2022). Endometrial microbiota composition is associated with reproductive outcome in infertile patients. Microbiome 10 (1), 1–17. doi: 10.1186/s40168-021-01184-w
Moreno, I., Simon, C. (2019). Deciphering the effect of reproductive tract microbiota on human reproduction. Reprod. Med. Biol. 18 (1), 40–50. doi: 10.1002/rmb2.12249
Mote, P. A., Bartow, S., Tran, N., Clarke, C. L. (2002). Loss of co-ordinate expression of progesterone receptors a and b is an early event in breast carcinogenesis. Breast Cancer Res. Treat 72 (2), 163–172. doi: 10.1023/A:1014820500738
Nakamura, A., Ooga, T., Matsumoto, M. (2019). Intestinal luminal putrescine is produced by collective biosynthetic pathways of the commensal microbiome. Gut Microbes 10 (2), 159–171. doi: 10.1080/19490976.2018.1494466
Nakatsu, C. H., Armstrong, A., Clavijo, A. P., Martin, B. R., Barnes, S., Weaver, C. M. (2014). Fecal bacterial community changes associated with isoflavone metabolites in postmenopausal women after soy bar consumption. PLos One 9 (10), e108924. doi: 10.1371/journal.pone.0108924
Nougayrède, J. P., Homburg, S., Taieb, F., Boury, M., Brzuszkiewicz, E., Gottschalk, G., et al. (2006). Escherichia coli induces DNA double-strand breaks in eukaryotic cells. Science 313 (5788), 848–851. doi: 10.1126/science.1127059
Ono, M., Yin, P., Navarro, A., Moravek, M. B., Coon, J. S., Druschitz, S. A., et al. (2013). Paracrine activation of WNT/β-catenin pathway in uterine leiomyoma stem cells promotes tumor growth. Proc. Natl. Acad. Sci. 110 (42), 17053–17058. doi: 10.1073/pnas.1313650110
Pelzer, E. S., Allan, J. A., Cunningham, K., Mengersen, K., Allan, J. M., Launchbury, T., et al. (2011). Microbial colonization of follicular fluid: alterations in cytokine expression and adverse assisted reproduction technology outcomes. Hum. Reprod. 26 (7), 1799–1812. doi: 10.1093/humrep/der108
Plottel, C. S., Blaser, M. J. (2011). Microbiome and malignancy. Cell Host Microbe 10 (4), 324–335. doi: 10.1016/j.chom.2011.10.003
Prusinski Fernung, L. E., Yang, Q., Sakamuro, D., Kumari, A., Mas, A., Al-Hendy, A. (2018). Endocrine disruptor exposure during development increases incidence of uterine fibroids by altering DNA repair in myometrial stem cells. Biol. Reprod. 99 (4), 735–748. doi: 10.1093/biolre/ioy097
Punzón-Jiménez, P., Labarta, E. (2021). The impact of the female genital tract microbiome in women health and reproduction: a review. J. Assisted Reprod. Genet. 38 (10), 2519–2541. doi: 10.1007/s10815-021-02247-5
Qiu, J. R., Yang, M. Y., Ma, Y. L., Yang, M. C. (2022). Effect of ejiao (Asini corii colla) and turtle carapace glue on gut microbiota in nude mice with uterine fibroids based on high-throughput sequencing of 16SrRNA gene. Evidence-Based Complementary Altern. Med. 2022, 9 doi: 10.1155/2022/3934877
Qi, X., Yun, C., Pang, Y., Qiao, J. (2021). The impact of the gut microbiota on the reproductive and metabolic endocrine system. Gut Microbes 13 (1), 1894070. doi: 10.1080/19490976.2021.1894070
Qu, D., Yang, M., Tong, L., Yu, X., Jing, Q., Yang, Y., et al. (2021). Combination of dydrogesterone and antibiotic versus antibiotic alone for chronic endometritis: a randomized controlled trial study. Reprod. Sci. 28 (11), 3073–3080. doi: 10.1007/s43032-021-00583-6
Ravel, J., Gajer, P., Abdo, Z., Schneider, G. M., Koenig, S. S., McCulle, S. L., et al. (2011). Vaginal microbiome of reproductive-age women. Proc. Natl. Acad. Sci. 108(Supplement 1), 4680–4687. doi: 10.1073/pnas.1002611107
Rea, D., Coppola, G., Palma, G., Barbieri, A., Luciano, A., Del Prete, P., et al. (2018). Microbiota effects on cancer: from risks to therapies. Oncotarget. 9 (25), 17915. doi: 10.18632/oncotarget.24681
Reis, F. M., Bloise, E., Ortiga-Carvalho, T. M. (2016). Hormones and pathogenesis of uterine fibroids. Best Pract. Res. Clin. Obstetrics Gynaecology 34, 13–24. doi: 10.1016/j.bpobgyn.2015.11.015
Rietjens, I. M., Louisse, J., Beekmann, K. (2017). The potential health effects of dietary phytoestrogens. Br. J. Pharmacol. 174 (11), 1263–1280. doi: 10.1111/bph.13622
Rinninella, E., Raoul, P., Cintoni, M., Franceschi, F., Miggiano, G. A. D., Gasbarrini, A., et al. (2019). What is the healthy gut microbiota composition? a changing ecosystem across age, environment, diet, and diseases. Microorganisms 7 (1), 14. doi: 10.3390/microorganisms7010014
Robles-Vera, I., Callejo, M., Ramos, R., Duarte, J., Perez-Vizcaino, F. (2019). Impact of vitamin d deficit on the rat gut microbiome. Nutrients 11 (11), 2564. doi: 10.3390/nu11112564
Rosenfeld, C. S. (2017). Gut dysbiosis in animals due to environmental chemical exposures. Front. Cell. Infection Microbiol. 7 (396). doi: 10.3389/fcimb.2017.00396
Rowe, M., Veerus, L., Trosvik, P., Buckling, A., Pizzari, T. (2020). The reproductive microbiome: an emerging driver of sexual selection, sexual conflict, mating systems, and reproductive isolation. Trends Ecol. Evol. 35 (3), 220–234. doi: 10.1016/j.tree.2019.11.004
Rubinstein, M. R., Wang, X., Liu, W., Hao, Y., Cai, G., Han, Y. W. (2013). Fusobacterium nucleatum promotes colorectal carcinogenesis by modulating e-cadherin/β-catenin signaling via its FadA adhesin. Cell Host Microbe 14 (2), 195–206. doi: 10.1016/j.chom.2013.07.012
Sabry, M., Halder, S. K., Allah, A. S. A., Roshdy, E., Rajaratnam, V., Al-Hendy, A. (2013). Serum vitamin D3 level inversely correlates with uterine fibroid volume in different ethnic groups: a cross-sectional observational study. Int. J. women’s Health 5, 93. doi: 10.2147/IJWH.S38800
Schreurs, M. P., de Vos van Steenwijk, P. J., Romano, A., Dieleman, S., Werner, H. M. (2021). How the gut microbiome links to menopause and obesity, with possible implications for endometrial cancer development. J. Clin. Med. 10 (13), 2916. doi: 10.3390/jcm10132916
Schwebke, J. R., Muzny, C. A., Josey, W. E. (2014). Role of Gardnerella vaginalis in the pathogenesis of bacterial vaginosis: a conceptual model. J. Infect. Dis. 210 (3), 338–343. doi: 10.1093/infdis/jiu089
Sędzikowska, A., Szablewski, L. (2021). Human gut microbiota in health and selected cancers. International Journal of Molecular Sciences, 22(24), 13440.
Shan, J., Ni, Z., Cheng, W., Zhou, L., Zhai, D., Sun, S., et al. (2021). Gut microbiota imbalance and its correlations with hormone and inflammatory factors in patients with stage 3/4 endometriosis. Arch. Gynecology Obstetrics. 304 (5), 1363–1373. doi: 10.1007/s00404-021-06057-z
Sharan, C., Halder, S. K., Thota, C., Jaleel, T., Nair, S., Al-Hendy, A. (2011). Vitamin d inhibits proliferation of human uterine leiomyoma cells via catechol-o-methyltransferase. Fertility sterility 95 (1), 247–253. doi: 10.1016/j.fertnstert.2010.07.1041
Singh, R. K., Chang, H. W., Yan, D. I., Lee, K. M., Ucmak, D., Wong, K., et al. (2017). Influence of diet on the gut microbiome and implications for human health. J. Trans. Med. 15 (1), 1–17. doi: 10.1186/s12967-017-1175-y
Singh, P., Kumar, M., Al Khodor, S. (2019). Vitamin d deficiency in the gulf cooperation council: exploring the triad of genetic predisposition, the gut microbiome and the immune system. Front. Immunol. 10, 1042. doi: 10.3389/fimmu.2019.01042
Singh, P., Rawat, A., Alwakeel, M., Sharif, E., Al Khodor, S. (2020). The potential role of vitamin d supplementation as a gut microbiota modifier in healthy individuals. Sci. Rep. 10 (1), 1–14. doi: 10.1038/s41598-020-77806-4
Smith, S. B., Ravel, J. (2017). The vaginal microbiota, host defence and reproductive physiology. J. Physiol. 595 (2), 451–463. doi: 10.1113/JP271694
Sobstyl, M., Brecht, P., Sobstyl, A., Mertowska, P., Grywalska, E. (2022). The role of microbiota in the immunopathogenesis of endometrial cancer. Int. J. Mol. Sci. 23 (10), 5756. doi: 10.3390/ijms23105756
Sommer, F., Bäckhed, F. (2013). The gut microbiota–masters of host development and physiology. Nat. Rev. Microbiol. 11 (4), 227–238. doi: 10.1038/nrmicro2974
Stewart, E. A., Laughlin-Tommaso, S. K., Catherino, W. H., Lalitkumar, S., Gupta, D., Vollenhoven, B. (2016). Uterine fibroids. Nat. Rev. Dis. Primers 2 (1), 1–18. doi: 10.1038/nrdp.2016.43
Svensson, A., Brunkwall, L., Roth, B., Orho-Melander, M., Ohlsson, B. (2021). Associations between endometriosis and gut microbiota. Reprod. Sci. 28, 2367–2377. doi: 10.1007/s43032-021-00506-5
Tao, X., Franasiak, J. M., Zhan, Y., Scott, R. T., III, Rajchel, J., Bedard, J., et al. (2017). Characterizing the endometrial microbiome by analyzing the ultra-low bacteria from embryo transfer catheter tips in IVF cycles: Next generation sequencing (NGS) analysis of the 16S ribosomal gene. Hum. Microbiome J. 3, 15–21. doi: 10.1016/j.humic.2017.01.004
Thackray, V. G. (2019). Sex, microbes, and polycystic ovary syndrome. Trends Endocrinol. Metab. 30 (1), 54–65. doi: 10.1016/j.tem.2018.11.001
Tremellen, K., Pearce, K. (2012). Dysbiosis of gut microbiota (DOGMA)–a novel theory for the development of polycystic ovarian syndrome. Med. Hypotheses 79 (1), 104–112. doi: 10.1016/j.mehy.2012.04.016
Tu, Y., Zheng, G., Ding, G., Wu, Y., Xi, J., Ge, Y., et al. (2020). Comparative analysis of lower genital tract microbiome between PCOS and healthy women. Front. Physiol. 11, 1108. doi: 10.3389/fphys.2020.01108
Verstraelen, H., Vilchez-Vargas, R., Desimpel, F., Jauregui, R., Vankeirsbilck, N., Weyers, S., et al (2016). Characterisation of the human uterine microbiome in non-pregnant women through deep sequencing of the V1-2 region of the 16S rRNA gene. PeerJ 4, e1602. doi: 10.771.7/peerj.1602
Wallace, A. E., Gibson, D. A., Saunders, P. T., Jabbour, H. N. (2010). Inflammatory events in endometrial adenocarcinoma. J. Endocrinol. 206 (2), 141–157. doi: 10.1677/JOE-10-0072
Walther-António, M. R., Chen, J., Multinu, F., Hokenstad, A., Distad, T. J., Cheek, E. H., et al. (2016). Potential contribution of the uterine microbiome in the development of endometrial cancer. Genome Med. 8 (1), 1–15. doi: 10.1186/s13073-016-0368-y
Wampach, L., Heintz-Buschart, A., Hogan, A., Muller, E. E., Narayanasamy, S., Laczny, C. C., et al. (2017). Colonization and succession within the human gut microbiome by archaea, bacteria, and microeukaryotes during the first year of life. Front. Microbiol. 8, 738. doi: 10.3389/fmicb.2017.00738
Wang, W., Li, Y., Wu, Q., Pan, X., He, X., Ma, X. (2020). High-throughput sequencing study of the effect of transabdominal hysterectomy on intestinal flora in patients with uterine fibroids. BMC Microbiol. 20 (1), 1–11. doi: 10.1186/s12866-020-01779-7
Wang, B., Yao, M., Lv, L., Ling, Z., Li, L. (2017). The human microbiota in health and disease. Engineering 3 (1), 71–82. doi: 10.1016/J.ENG.2017.01.008
Williams, C. L., Garcia-Reyero, N., Martyniuk, C. J., Tubbs, C. W., Bisesi, J. H., Jr. (2020). Regulation of endocrine systems by the microbiome: perspectives from comparative animal models. Gen. Comp. Endocrinol. 292, 113437. doi: 10.1016/j.ygcen.2020.113437
Wise, L. A., Laughlin-Tommaso, S. K. (2016). Epidemiology of uterine fibroids–from menarche to menopause. Clin. obstetrics gynecology 59 (1), 2. doi: 10.1097/GRF.0000000000000164
Wu, S., Zhang, Y. G., Lu, R., Xia, Y., Zhou, D., Petrof, E. O., et al. (2015). Intestinal epithelial vitamin d receptor deletion leads to defective autophagy in colitis. Gut 64 (7), 1082–1094. doi: 10.1136/gutjnl-2014-307436
Yang, Q., Ciebiera, M., Bariani, M. V., Ali, M., Elkafas, H., Boyer, T. G., et al. (2022). Comprehensive review of uterine fibroids: developmental origin, pathogenesis, and treatment. Endocrine Rev. 43 (4), 678–719. doi: 10.1210/endrev/bnab039
Yuan, M., Li, D., Zhang, Z., Sun, H., An, M., Wang, G. (2018). Endometriosis induces gut microbiota alterations in mice. Hum. Reprod. 33 (4), 607–616. doi: 10.1093/humrep/dex372
Yurtdaş, G., Akdevelioğlu, Y. (2020). A new approach to polycystic ovary syndrome: the gut microbiota. J. Am. Coll. Nutr. 39 (4), 371–382. doi: 10.1080/07315724.2019.1657515
Zhang, Q., Shen, Q., Celestino, J., Milam, M. R., Westin, S. N., Lacour, R. A., et al. (2009). Enhanced estrogen-induced proliferation in obese rat endometrium. Am. J. obstetrics gynecology 200 (2), 186. e1. doi: 10.1016/j.ajog.2008.08.064
Zinser, G. M., Suckow, M., Welsh, J. (2005). Vitamin d receptor (VDR) ablation alters carcinogen-induced tumorigenesis in mammary gland, epidermis and lymphoid tissues. J. Steroid Biochem. Mol. Biol. 97 (1-2), 153–164. doi: 10.1016/j.jsbmb.2005.06.024
Keywords: endometrium, fibroid, PCOS, vaginal microbiome, gut microbiome
Citation: Elkafas H, Walls M, Al-Hendy A and Ismail N (2022) Gut and genital tract microbiomes: Dysbiosis and link to gynecological disorders. Front. Cell. Infect. Microbiol. 12:1059825. doi: 10.3389/fcimb.2022.1059825
Received: 02 October 2022; Accepted: 18 November 2022;
Published: 16 December 2022.
Edited by:
Pallavi Singh, Northern Illinois University, United StatesReviewed by:
Sandeep Kondakala, National Center for Toxicological Research (FDA), United StatesVerima Pereira, University of California, San Francisco, United States
Chandni Talwar, Baylor College of Medicine, United States
Kathiresh Kumar, Texas A&M Health Science Center, United States
Copyright © 2022 Elkafas, Walls, Al-Hendy and Ismail. This is an open-access article distributed under the terms of the Creative Commons Attribution License (CC BY). The use, distribution or reproduction in other forums is permitted, provided the original author(s) and the copyright owner(s) are credited and that the original publication in this journal is cited, in accordance with accepted academic practice. No use, distribution or reproduction is permitted which does not comply with these terms.
*Correspondence: Nahed Ismail, aXNtYWlsN0B1aWMuZWR1