- 1Jilin Ginseng Academy, Key Laboratory of Active Substances and Biological Mechanisms of Ginseng Efficacy, Ministry of Education, Jilin Provincial Key Laboratory of Bio-Macromolecules of Chinese Medicine, Changchun University of Chinese Medicine, Changchun, China
- 2Research Center of Traditional Chinese Medicine, The First Affiliated Hospital of Changchun University of Chinese Medicine, Changchun, China
- 3College of Acupuncture and Tuina, Changchun University of Chinese Medicine, Changchun, China
- 4Department of Endocrinology, The First Affiliated Hospital of Changchun University of Chinese Medicine, Changchun, China
Panax ginseng, as the king of Chinese herb, has significant therapeutic effects on obesity, type 2 diabetes mellitus, fatty liver disease, colitis, diarrhea, and many other diseases. This review systematically summarized recent findings, which show that ginseng plays its role by regulating gut microbiota diversity, and gut microbiota could also regulate the transformation of ginsenosides. We conclude the characteristics of ginseng in regulating gut microbiota, as the potential targets to prevent and treat metabolic diseases, colitis, neurological diseases, cancer, and other diseases. Ginseng treatment can increase some probiotics such as Bifidobacterium, Bacteroides, Verrucomicrobia, Akkermansia, and reduce pathogenic bacteria such as Deferribacters, Lactobacillus, Helicobacter against various diseases. Meanwhile, Bacteroides, Eubacterium, and Bifidobacterium were found to be the key bacteria for ginsenoside transformation in vivo. Overall, ginseng can regulate gut microbiome diversity, further affect the synthesis of secondary metabolites, as well as promote the transformation of ginsenosides for improving the absorptivity of ginsenosides. This review can provide better insight into the interaction of ginseng with gut microbiota in multiple disorders and ginsenoside transformation.
Introduction
Panax ginseng C. A. Meyer (ginseng), called the king of herbs, has become one of the most popular Chinese herbal medicines in the world. It is widely used to treat various diseases (Ratan et al., 2021) and regulate human health (Fan et al., 2020). Moreover, ginseng also has high production value. According to statistics in 2016, China’s Ginseng output reached 28,900 tons, creating an output value of $7.5 billion for the ginseng industry in Jilin Province, ranking the first position (Li et al., 2019). In recent years, a variety of active ingredients have been isolated and identified from ginseng, mainly ginsenoside, polysaccharide, volatile oil, amino acid, polyacetylene, alkaloid, as well as a small amount of salicylic acid amine, organic acid, non-saponin water-soluble glucoside, maltol and its derivative glucoside (Liu et al., 2020). Polysaccharides, as one of the main components of ginseng, have the functions of immunomodulation, anti-tumor and anti-diabetes (Zhao et al., 2019). Amino acids in ginseng can enhance immunity, promote cell growth, proliferation and angiogenesis (Sah et al., 2021). Polyacetylene can significantly inhibit the proliferation of cancer cells and lipid peroxidation, improve memory (Al-Hazmi et al., 2015) and anti-inflammation (Jin et al., 2021). Alkaloids have the functions of radiation protection, anti-diabetic (Chen et al., 2019) and anti-tumor (Ajebli et al., 2021; Xu et al., 2021). The volatile oil in ginseng has the effects of antioxidation and liver protection (Bak et al., 2012). Ginsenoside is the most extensively studied active ingredient at this stage and has been considered as the main components of pharmacological action (Guo et al., 2021), such as anti-tumor (Kim et al., 2016), anti-inflammatory (Han and Kim, 2020), antioxidant, inhibition of apoptosis (Zheng et al., 2018).There are other trace components in ginseng, but they are not the main active components.
At present, it has been found that ginseng contains a variety of non-widespread initial ginsenosides, however, it does not have good biological activity, but after the processing and transformation of gut microbiota, it is found that there are 289 kinds of ginsenosides (including free ginsenoside) and their biological activities were significantly improved (Li et al., 2022). Although ginseng has many biological effects, the mechanisms of the pharmacological effect and its metabolic process in vivo have not been fully explained. In recent years, multiple studies have shown that the effect of ginseng is closely related to the role of gut microbiota (Pan et al., 2019; Santangelo et al., 2019), and gut microbiota is also the main tool for ginsenoside transformation (Kim, 2018).
Human microbial community is a complex ecosystem, in which the microbial community in the intestine has the largest scale and the most species (Tan et al., 2020). There are as many as 100 trillion microorganisms in human intestine, which are composed of at least 1,500 genera and 50 different phyla (Robles-Alonso and Guarner, 2013). In the long process of evolution of gut microbiota, through individual adaptation and natural selection, the microbiota of different species, microbiota and host, microbiota and host environment are always in a state of dynamic balance, forming an interdependent system. More and more evidences show that gut microbiota plays a key role not only in the metabolism of nutrients and drugs and the absorption of fat in the diet, but also in the regulation of immunity, physiology, metabolism, and health maintenance (Xi et al., 2021; Zhang et al., 2021). Besides, intestinal microorganisms could affect multiple tissues and organs, such as intestinal cells, liver, adipose tissue, brain, and muscle. These extensive studies about intestinal microbiota have made great progress in both humans and animals. As an example, specific changes in microbiota composition are associated with various diseases (Cani et al., 2021). For example, the ratio of Firmicutes to Bacteroidetes is increased in obese patients, while it is decreased in enteritis patients (Stojanov et al., 2020). Meanwhile, many treatments have targeted gut microbiota, which have found that probiotics are a potential treatment option for different diseases, such as Alzheimer’s disease (Ji and Shen, 2021). Therefore, gut microbiota has been considered as potential targets for the prevention and treatment of various diseases.
Currently, there are a few reviews for summarizing the regulation of ginseng on gut microbiota in a series of diseases and the biotransformation of ginsenosides by gut microbiota. In our review, we searched and summarized the recent publications that ginseng could treat various diseases through the regulation of gut microbiota, including obesity, type 2 diabetes mellitus (T2DM), liver diseases, diarrhea, colitis, and others. Moreover, we summarized the gut microbiota affected by ginseng in the treatment process, and the transformation process of ginsenosides under the action of gut microbiota. By the relevant reports on ginseng and gut microbiota, it reveals new potential targets, intestinal microbiota for ginseng in the prevention and treatment of a variety of diseases and provides new insights into the future research direction, intestinal microorganisms as potential targets of ginseng.
Ginseng Treats Various Diseases by Regulating Gut Microbiota
Obesity
Obesity is a global health problem (Safaei et al., 2021). Many chronic diseases associated with obesity, such as diabetes, cardiovascular disease and non-alcoholic fatty liver disease which are the main causes of human death (Hu et al., 2017). At present, the studies have found that gut microbiota is a necessary condition for the progress of obesity. Transplanting the microbiota of obese mice into healthy mice can induce obesity in healthy mice. At the same time, the activation and accumulation of fat by gut microbiota have been gradually revealed (Crovesy et al., 2020; Liu et al., 2021). Ginseng extract could also induce Enterococcus faecalis to produce unsaturated long-chain fatty acids and myristic acid has good effect on activating brown fat, promoting the production of beige fat, and reducing the body weight of obese mice (Quan et al., 2020). Firmicutes can promote energy absorption and fat accumulation (Orbe-Orihuela et al., 2018), and Bacteroides can regulate bile acid metabolism, reduce the level of inflammation, and inhibit fat accumulation in obese hosts. The ratio of Firmicutes to Bacteroidetes also plays an important role in the process of obesity (Gibiino et al., 2018). At present, ginseng extract, red ginseng extract, and the combination of ginsenoside Rb1 with salvianolic acid B have been proved to reduce weight and lipid by decreasing the ratio of Firmicutes to Bacteroidetes (Zhou et al., 2020; Bai et al., 2021; Lee et al., 2021). Meanwhile, ginseng treatment for 28 days can also up-regulate Bacteroides, Parabacteroides, and Lactobacillus probiotics to reduce the abundance of bacteria that can induce obesity, such as Firmicutes, Deferribactes, Helicobacter in obese hosts. Monascus fermented ginseng can reduce the ratio of Firmicutes to Bacteroides in the intestinal tract of HFD rats, further to decrease the relative levels of sterol regulatory element binding protein-2 (SREBP-2) and hydroxymethylglutaryl-CoA (HMG-CoA) reductase, and increase the expression of CYP7A1 to improve lipid level of total cholesterol (TC) in blood and liver against metabolic disorder (Zhao et al., 2021). The microbiota structure in obese hosts might be the pathogenic factor, but ginseng can help the body return to the normal metabolic level and lose weight by improving this unbalanced microbiota structure. In obesity animal models, Firmicutes, Bacteroides, Lactobacillus, and Helicobacter may be potential targets for ginseng in the treatment of obesity (Table 1). Meanwhile, relative studies have found ginseng could reduce the body weight, blood lipid, blood glucose, and inflammatory level, as well as can significantly improve the structure of gut microbiota in obese patients (Table 2) (Song et al., 2014; Seong et al., 2021).
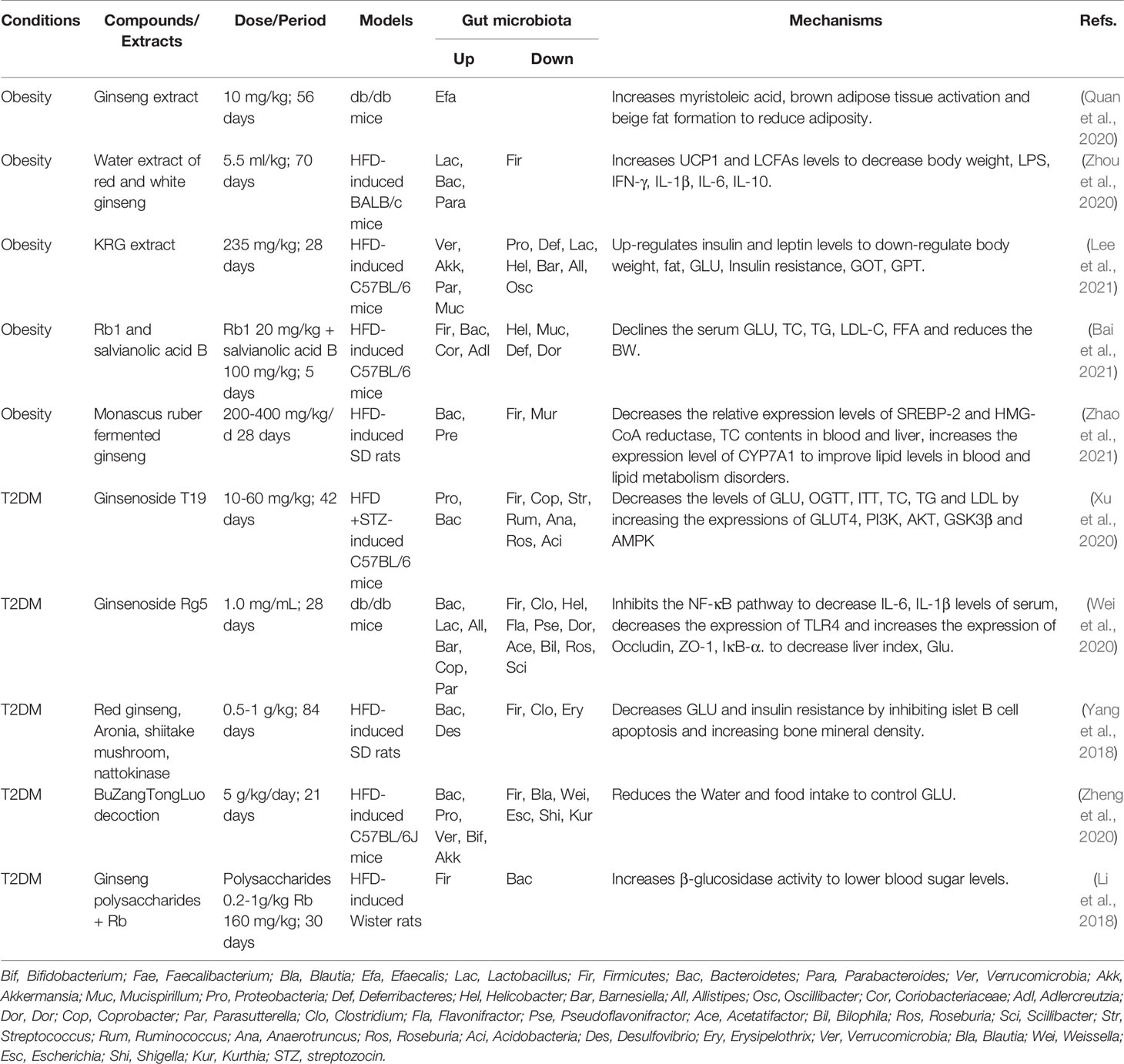
Table 1 Summary of anti-obesity activities of ginseng by regulating gut microbiota and related pathways.
Type 2 Diabetes Mellitus (T2DM)
T2DM is a complex disease, which is characterized by hyperglycemia and its complications mainly caused by insulin resistance (Del Prato and Chilton, 2018; Himanshu et al., 2020). In recent years, it has been found that the disorder of gut microbiota is an important factor in the occurrence and development of T2DM (Canfora et al., 2019). For example, the ratio of Firmicutes and Bacteroidetes is generally relatively high in the T2DM hosts (Perry et al., 2016). Current results have confirmed that ginseng plays a role in improving blood glucose level and insulin sensitivity in patients with T2DM (Gui et al., 2016). It proved that the treatment with ginsenoside T19 in C57BL/6 mice model induced by high-fat diet combined with streptozotocin could exert therapeutic effect for treating T2DM by reducing the relative abundance of pathogenic bacteria such as Coprobacillus and decreasing the ratio of Firmicutes and Bacteroidetes, as well as enhancing the relative abundance of probiotics such as Bacteroidetes (Xu et al., 2020). Ginsenoside Rg5 can also reverse the disordered gut microbiota, alleviate metabolic endotoxemia, repair intestinal barrier, inhibit nuclear factor kappaB (NF-κB) and other related inflammatory pathways to improve T2DM of Db/Db mice (Wei et al., 2020). Besides these ginsenosides, the combination of red ginseng, Aronia, Shiitake mushroom, and Nattokinase and Buzhong Tongluo decoction including Astragalus membranaceus, Dioscorea hemsleyi, Salvia miltiorrhiza, Scrophularia ningpoensis, Ophiopogon japonicus, Panax ginseng, Fritillariae cirrhosae, and Whitmania pigra Whitman have been proved to be able to up-regulate Bacteroides, Lactobacillus, Akkermansia probiotics, reduce the abundance of bacteria causing hyperglycemia, such as Helicobacter, Flavonifractor, and reduce the ratio of Firmicutes and Bacteroidetes, thus play their hypoglycemic effects (Yang et al., 2018; Zheng et al., 2020). In another disorder, metabolic syndrome, the powder from Korean red ginseng (KRG) has a good therapeutic effect, and its mechanism might be related to the improvement of the disorder of lipid metabolism (the reductions of TC and low-density lipoprotein, LDL) by increasing the relative abundance of Bacteroides and reducing the relative abundance of Firmicutes and Proteobacteria (Seong et al., 2021). Most studies show that ginseng can correct the abnormal metabolic level in the body by reversing the ratio of Firmicutes and Bacteroidetes. However, in the experiment of T2DM rat model treated with ginseng polysaccharide combined with Rb1, the ratio of Firmicutes and Bacteroidetes were increased after the treatment, which finally reduced the blood glucose level and increased β-glucosidase activity (Li et al., 2018). As same as most medical treatment, the ratio of Firmicutes and Bacteroidetes could be decreased, verse originally elevated in the pathological model. This change of gut microbiota could reduce the body’s energy intake and improve the level of glucose and lipid metabolism. However, this ratio was increased after treatment with polysaccharide and Rb1 in the above research. The original author did not make a targeted explanation. We speculate that it may be related to the state of the model, but the specific reason remains to be further revealed.
Therefore, the disordered gut microbiota is the inducer of T2DM, but ginseng can reverse the structures of disordered gut microbiota to restore the glucose metabolism and improve the disorder of glucose and lipid metabolism, ultimately inhibit the development of T2DM (Table 1).
Fatty Liver Diseases
Non-alcoholic fatty liver disease (NAFLD), a gradually serious health problem worldwide, is a chronic and multi-factorial cause of liver disease (Younossi et al., 2016), which is often observed in patients with obesity, dyslipidemia and diabetes, and manifesting as hepatic steatosis (Polyzos et al., 2019). Pathogenic causes of fatty liver diseases include insulin resistance, hepatic lipid metabolism disorder, inflammation, genetics, and other factors (Friedman et al., 2018). In addition, the studies have found that the gut microbiota is able to influence hepatic lipid metabolism as well as the balance of pro- inflammatory and anti-inflammatory factors in the liver. Therefore, the gut microbiota has been a potential target for the prevention and treatment of NAFLD (Kolodziejczyk et al., 2019). Ginseng is widely used in metabolic diseases and also has a significant effect on the improvement of NAFLD (Roh et al., 2020; Yoon et al., 2021; Gu et al., 2021). Another study of ginseng extract treatment for fatty liver mice induced by high-fat diet demonstrated that ginseng could reverse the structure of disturbed gut microbiota to inhibit the levels of sterol regulatory element binding protein-1c (SREBP-1c), fatty acid synthetase (FAS), and acetyl-coenzyme a carboxylase 1 (ACC-1), and increase carnitine palmityl transferase 1A (CPT-1a) expression to alleviate hepatic lipid accumulation and suppress NF-κB/I-kappa-B (IκB) inflammasome pathway (Liang et al., 2021).
Alcoholic fatty liver disease is also a highly harmful chronic liver disease. And its occurrence is confirmed to be associated with the gut microbiota (Liu et al., 2018). The researches have proved that fermented ginseng can up-regulate probiotics such as Bifidobacterium, Lactobacillus, Akkermansia and down-regulate pathogenic bacteria such as Peptostreptococcaceae, Colibacillus that lead to metabolic disorders, which can alleviate the level of inflammation and improve the situation of alcoholic liver injury (Fan et al., 2019). The further deterioration of non-alcoholic fatty liver disease and alcoholic fatty liver disease could lead to hepatitis and even liver cancer (Ocker, 2020). In a dimethylnitrosamine induced-cancer mice, ginsenoside Rg3 combined with Fe@Fe3O4 nanoparticles can reduce the number of cancerous cells and prolong the survival time of mice with liver cancer by elevating the ratio of Firmicutes and Bacteroidetes or reversing the pathogenic gut microbiota structure (Ren et al., 2020). In summary, ginseng can treat the animal liver diseases models by reversing the imbalanced gut microbiota, improving liver metabolism, and restoring host homeostasis (Table 3). Moreover, in NAFLD patients, KRG could decrease the ratio of Firmicutes and Bacteroidetes to improve liver lipid metabolism, decreass serum TC and total triglyceride (TG) (Table 2) (Hong et al., 2021).
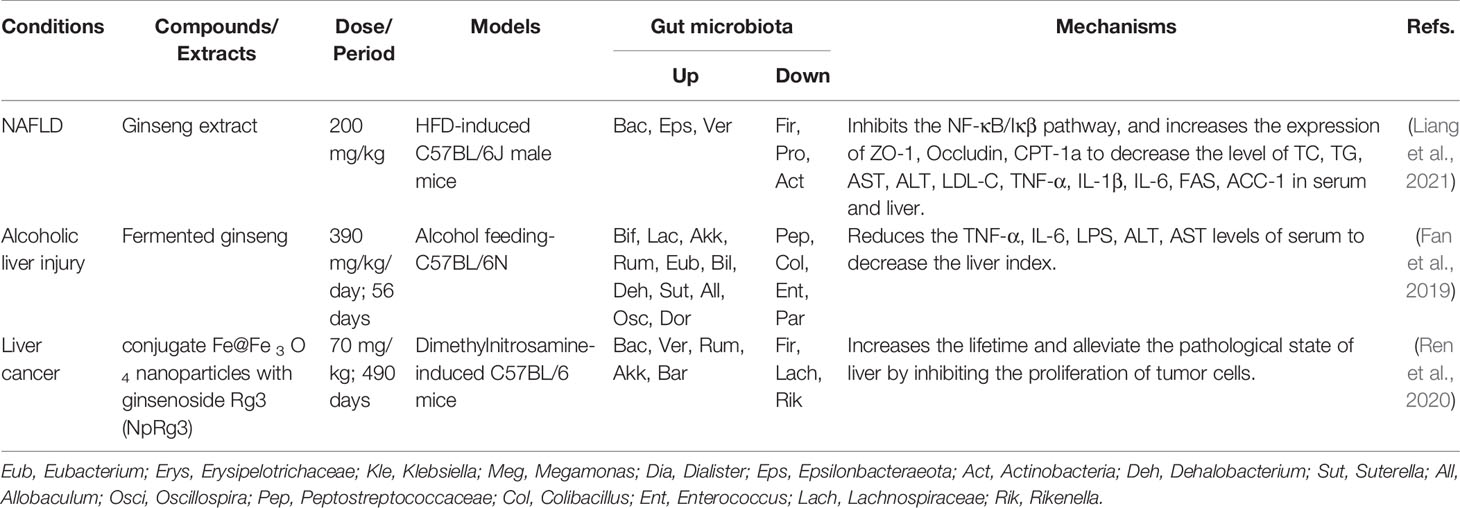
Table 3 Summary of anti-liver diseases activities of ginseng by regulating gut microbiota and related pathways.
Diarrhea
Diarrhea is one of the leading diseases with the highest morbidity and mortality worldwide which is characterized by acute and infectious (Shankar and Rosenbaum, 2020). Prolonged diarrhea can lead to the consequences such as hypovolemia, electrolyte imbalance, malnutrition, and skin damage (Reintam Blaser et al., 2015). There are many causes of diarrhea, and commonly there are the disorders of bile acid metabolism (Hughes et al., 2021), viruses (Becker-Dreps et al., 2020), and Escherichia coli infection (Ahmed et al., 2013). Furthermore, recent studies have found that gut microbiota is an important pathogenic factor leading to diarrhea (Fan and Pedersen, 2021). While the occurrence of diarrhea could be prevented and improved by restoring the structures of disorganized gut microbiota (Gallo et al., 2016). Ginseng can alleviate diarrheal symptoms by modulating the gut microbiota structure. In the Kunming mice induced by 5-fluorouracil, a combination of total ginsenoside and volatiles of Atractylodes Macrocephala can decrease the abundances of Bacteroides, Proteobacteria and other bacteria and increase the abundances of Firmicutes and Lactobacillus to restore gut microbiota homeostasis for the improvement of diarrheal situation (Wang et al., 2019a). Moreover, the combination of ginseng polysaccharides and volatilized oil from Atractylodes Macrocephala could reduce the diarrhea index and ameliorate colonic lesions by restoring the structures of gut microbiota in the disease state (Wang et al., 2019b). In addition, fermented ginseng and ginseng polysaccharides have also been proved to have therapeutic effects on diarrhea by up-regulating Firmicutes, Lactobacillus, the ratio of Firmicutes and Bacteroidetes, and down-regulating Proteobacteria, and Bacteroidetes (Qi et al., 2019; Qu et al., 2021). Taken together, the active components from ginseng can restore intestinal homeostatic balance and improve water and salt metabolism to alleviate diarrhea (Table 4).
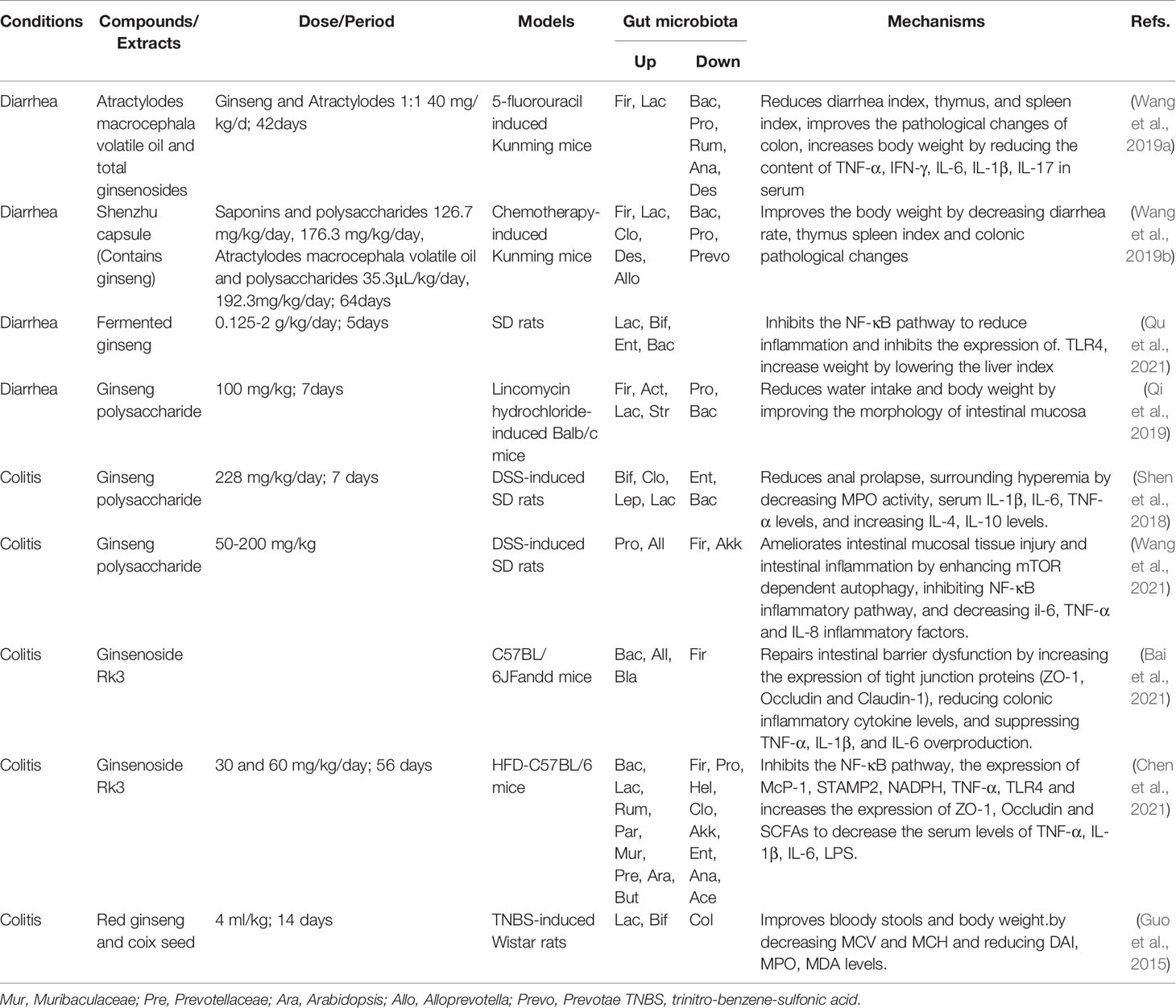
Table 4 Summary of anti-colitis and anti-diarrhea activities of ginseng by regulating gut microbiota and related pathways.
Colitis
Colitis is a chronic and persistent inflammatory environment leading to colon cancer, which is the third deadliest cancer of the world (Bocchetti et al., 2021). There are many factors leading to colitis, but the most fundamental is the gut microbiota. The changes in the composition and diversity of gut microbiota could lead to the damage of intestinal barrier and the immune function, and increase high levels of pro-inflammatory cytokines in the intestine and the incidence of erosion and ulcer (Hering et al., 2012). Studies have shown that probiotics supplementation can regulate bile acid metabolism and alleviate enteritis symptoms in mice (Liu and Wang, 2021). Probiotics can also restore unbalanced gut microbiota, enhance intestinal mucosal barrier function, improve intestinal immunity, and reduce gastrointestinal infection (Shen et al., 2018). Therefore, restoring the structure of gut microbiota is an important mechanism for the treatment of colitis. Ginseng has an anti-inflammatory and analgesic effects (Lee et al., 2019) and it could prevent the colitis and related cancer induced by dextran sodium sulfate (Shin et al., 2020). In the studies of dextran sodium sulfate (DSS)-induced SD rats, ginseng polysaccharides can significantly up-regulate the relative abundance of probiotics, reduce the relative abundance of pathogenic bacteria, and inhibit a variety of inflammatory signal pathways (Shen et al., 2018; Wang et al., 2021). Ginsenoside Rk3 can increase the abundance of probiotics such as Bacteroides and Lactobacillaceae and reduce the abundance of pathogenic bacteria that can promote the production of inflammatory factors such as Proteobacteria, Helicobacteraceae. At the same time, ginseng can decrease the ratio of Firmicutes and Bacteroidetes, increase the expressions of tight junction protein 1 (ZO-1) and occludin, improve the integrity of intestinal barrier and intestinal immune function through the inhibition of toll-like receptor 4 (TLR4)/NF-κB and other inflammatory pathways (Bai et al., 2021; Chen et al., 2021). The treatment of red ginseng and coix seed in the trinitrobenzene sulfonic acid-induced colitis rats is achieved by increasing the abundance of probiotics and reducing the abundance of pathogenic bacteria (Guo et al., 2015). In addition, ginsenoside Rg1 has been found could be transformed by gut microbiota in colitis model, this may the underling mechanism of anti-colitis effects of ginsenosides (Lee et al., 2015).Collectively, ginseng can inhibit the inflammation and improve colitis by reducing the relative abundance of pathogenic bacteria, such as Helicobacter, and increasing the relative abundance of Lactobacillus and Bacteroides probiotics (Table 4).
Other Diseases
Importantly, many studies have proved that ginseng, ginsenosides, and ginseng polysaccharides play potential effects on other diseases. For male Wister rats with spleen deficiency syndrome, the combination of ginseng and wild jujube could up-regulate the relative abundance of Firmicutes, Bacteroidetes, Lactobacillus, and Bifidobacterium, reduce the relative abundances of Actinobacteria, Proteobacteria, Streptococcus, Escherichia, Shigella, Veillonella, and Enterococcus, reverse the pathological state of the gut microbiota imbalance of the spleen deficiency syndrome, and improve the spleen deficiency syndrome (Li et al., 2020). For the fatigue, two studies showed that the extracts of ginseng and fermented ginseng leaf can recover from exercise-induced fatigue by improving the structure of gut microbiota and reducing the level of inflammation (Zheng et al., 2021; Zhou et al., 2021). For neurological diseases, ginseng extract, ginsenoside or the formula containing ginseng can regulate gut microbiota to play neuroprotective effect and improve memory impairment. In the rat ischemia/reperfusion model, ginsenoside Rb1 can increase the relative abundance of Lactobacillus and gamma-aminobutyric acid receptors to reduce the proinflammatory cytokines for the improvement of neuroprotection (Chen et al., 2020). In the rats induced by D-galactose, ginseng decoction called Dushen Tang showed good curative ability for memory impairment by up-regulating Bacteroidetes and down-regulating Lactobacillus (Wang et al., 2021). In the mice with Parkinson’s disease, the treatment with the extract from KRG can improve neuronal function and alleviate Parkinson’s symptoms by up-regulating the relative abundance of Eubacterium and down-regulating the relative abundances of Verrucomicrobia and Ruminococcus (Jeon et al., 2020). For Alzheimer’s disease, a formula named Qisheng Wan containing ginseng and ginsenoside Rg1 can restore the structures of disordered gut microbiota and reduce the levels of inflammatory factors to reduce the symptoms of Alzheimer’s disease (Wang et al., 2020; Xiong et al., 2022). In the ApcMin/+ mice during the development of colorectal cancer, ginsenoside Rb3 and Rd can promote the growth of beneficial bacteria (Bifidobacterium spp., Lactobacillus spp., Bacteroides acidifaciens, and Bacteroides xylanisolvens) and lower the abundance of cancer cachexia-related bacteria (Dysgonomonas spp., Helicobacter spp.) to reinstate mucosal architecture and improve mucosal immunity (Huang et al., 2017). Moreover, ginsenosides can facilitate the therapeutic effect of cyclophosphamide in the mice with mammary carcinoma, which may be related with the increases of anti-tumor cytokines and the production of gut probiotics (Akkermansia, Bifidobacterium, and Lactobacillus) (Zhu et al., 2021). In addition, ginseng polysaccharides at 200 mg/kg can potentiate anti-tumor effect of anti-programmed cell death 1/programmed cell death ligand 1 immunotherapy in non-small lung cancer by regulating the relative abundance of bacteria such as Escherichia, Rikenella, Parabacteroides distasonis, and Bacteroides vulgatus (Huang et al., 2021).
The products of red ginseng, including its water extract, 50% ethanol extract and bifidobacterial-fermentation of ethanol extract were compared to explore their mechanisms against ovalbumin-induced allergic rhinitis. It found that bifidobacteria-fermented extract of red ginseng can reduce the levels of IL-4, IL-5, and IL-13 in the colon and restore the populations of gut microbiota (Bacteroidetes, Actinobacteria, and Firmicutes) (Kim et al., 2019). Furthermore, the same research group reported that bifidobacteria-fermented extract of red ginseng and its main constituent, ginsenoside Rd, protopanaxatriol can ameliorate gut dysbiosis (Bacteroidetes and Proteobacteria populations) to mitigate anxiety/depression (Han et al., 2020). Additionally, the intake of ginseng extract for 34 weeks can decrease the abundance of Bifidobacteriaceae and Lactobacillus, and increase the abundance of Proteobacteria, Methylobacteriaceae, and Parasutterella, Sutterella, which suggest that it regulate the host-gut metabolism in the normal rats (Sun et al., 2018). Collectively, ginseng can up-regulate the relative abundance of probiotics, reduce the relative abundance of pathogenic bacteria, or restore the structure of unbalanced gut microbiota to prevent and treat various diseases, including fatigue, neurological diseases, cancer, allergic rhinitis, and depression (Table 5).
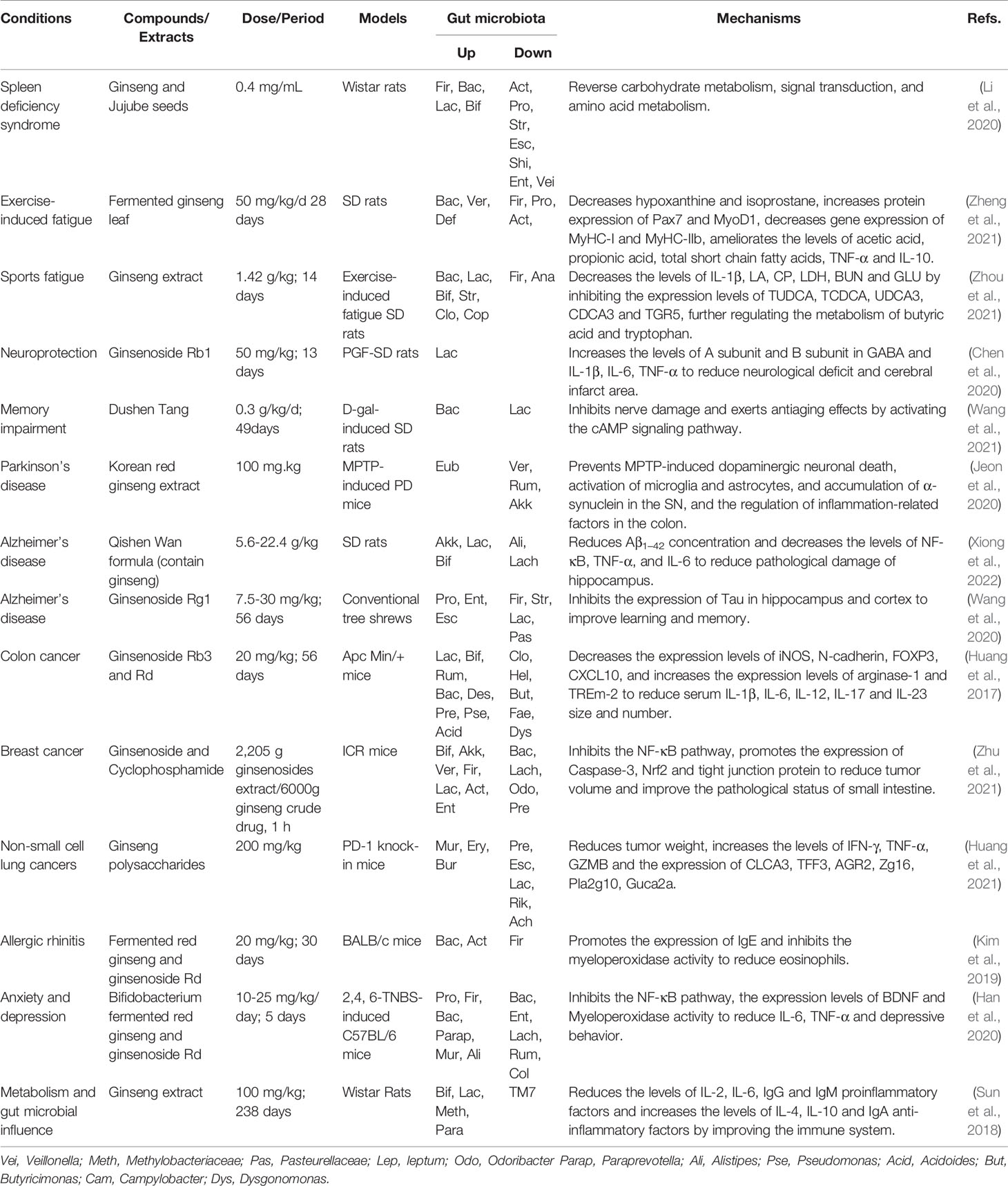
Table 5 Summary of anti-other diseases activities of ginseng by regulating gut microbiota and related pathways.
In summary, ginseng has significant therapeutic effects on a series of diseases, including obesity, T2DM, liver diseases, colitis, diarrhea, exercise-induced fatigue, Alzheimer’s disease, and cancer by regulation gut microbiota. Recent studies demonstrate that ginseng can further inhibits NF-κB and other inflammatory pathways to reduce the levels of proinflammatory factors (TNF-α and IL-1β) and increases the expressions of key proteins such as TLR4, ZO-1, and Occludin by regulating the species diversity and relative abundance of intestinal flora, thus maintaining the intestinal mucosal barrier homeostasis. The flora regulated by ginseng mainly includes Bacteroides, Bifidobacterium, Parabacteroides, Akkermansia, Helicobacter, Lactobacillus, and Proteobacteria during the process of different diseases. The detailed information for the compositions of gut microbiota and regulated signaling pathways in the therapeutic effect of ginseng against various diseases has been shown in Figure 1.
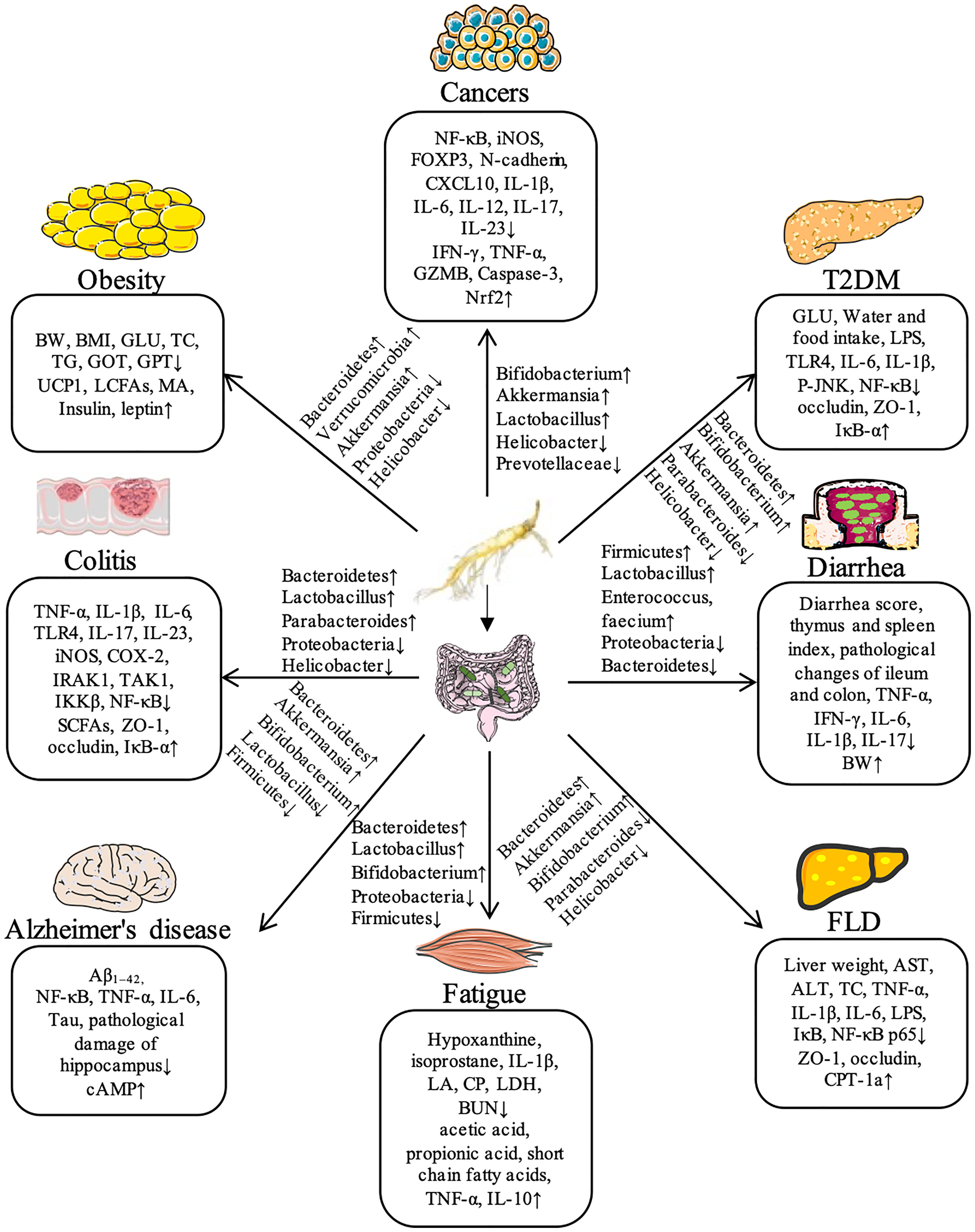
Figure 1 Ginseng shows its potential therapeutic effects on a variety of diseases through the regulation of gut microbiota in animal models.
Transformation and Modification of Ginsenosides by Gut Microbiota
Microbiota is an important core to host metabolism, could regulate the expression and function of enzymes involved in drug metabolism (Xu et al., 2017), it can also participate in the regulation of ginsenoside transformation by producing nitrate reductase and glucuronidase to hydrolyze glycosidic bonds (Yang et al., 2020). Besides, many important components such as folate, indole, gamma-aminobutyric acid, and short chain fatty acids (SCFAs) are produced by the metabolism of ingested ingredients by microbiota. These metabolites also are the raw materials for the synthesis of above-mentioned molecules. Therefore, gut microbiota plays an vital role in the pharmacokinetics and pharmacodynamics of natural products (Pan et al., 2019).
The oral utilization of ginsenosides is very low, and the activity of some ginsenosides is not good, hence they need to be transformed to improve their activity. Ginsenoside compound K (CK) is a kind of ginsenoside with good activity, which is transformed from a variety of ginsenosides through different processing. A large number of studies have found that ginsenoside CK has many pharmacological effects that it can induce apoptosis of colon cancer cells (Pak et al., 2020), treat lung cancer (Jin et al., 2018), and reduce the formation of atherosclerotic plaque (Zhou et al., 2016). Together, most studies have proved that CK has a significant therapeutic effect on inflammation and cancer. Structurally, CK has less Arabinofuranose (Araf), arabopyranose (Arap), and glucose (Glc) than the initial ginsenoside. Ginsenoside Rc can be transformed into Mb and Mc by removing Glc at C-3 under the action of Bacteroides and Fusobacterium, and then into CK by removing Araf at C-20 through Bacteroides, Eubacterium and Bifidobacterium (Choi et al., 2018). Ginsenoside Rb2 can first remove the Glc at C-3 through Bifidobacterium and Eubacterium, then remove the Araf at C-20 under the action of Bacteroides and Fusobacterium and convert it into F2, and finally transformed into CK under the action of Bacteroides and Fusobacterium (Kim et al., 2018). Ginsenoside Rb1 has only three more molecules of Glc than CK, which is transformed into CK under the action of Eubacterium, Lactobacillus and Bacteroides (Quan et al., 2013). In addition, Ginsenoside Rd and F2 are transferring states for CK transformation. Ginsenoside Rd is usually transformed from Rb1, Rb2 and Rc by removing redundant Araf and Glc under the action of Eubacterium and Bifidobacterium, while F2 is transformed from Mb, Co and Rd by removing Araf or Glc under the action of Eubacterium, Fusobacterium and Bacteroides (Zhang et al., 2021). Meanwhile, it found that ginsenoside which have Arap and Araf at C-20 preferentially removed Glc at C-3 during transformation. Bacteroides tends to preferentially remove the Glc at C-3. In addition, in the whole process of CK transformation, the enzymatic hydrolysis of Araf group mainly depends on Bifidobacterium, Bacteroides mainly guides the enzymatic hydrolysis of Glc, and the enzymatic hydrolysis of Arap is mainly mediated by Fusobacterium (Figure 2A).
Proto-ginsenoside of ginsenodiol type (PPD) has also been shown to have a significant inhibitory effect on breast cancer (Zhang et al., 2018), uterine cancer (Jo et al., 2021), prostate cancer (Cao et al., 2014), and metastasis of cancer cells (Lu et al., 2020). Although PPD has good pharmacological activity, it cannot be directly extracted from ginseng, it needs to be processed and transformed under the action of gut microbiota in vivo. Most of PPD is transformed from CK by removing the Glc at C-20 under the action of Bacteroides, Eubacterium and Bifidobacterium, and the other part is transformed from ginsenoside Rg3 by removing the two molecules of Glc at C-3 under the action of Bacteroides, Eubacterium and Bifidobacterium (Figure 2A). Proto-ginsenoside of ginsentriol type (PPT) is also a saponin with good activity, which has pharmacological effects such as reducing memory and cognitive impairment, improving Alzheimer’s disease (Lu et al., 2018), inhibiting septic shock and peritonitis (Jiang et al., 2020), improving cellular inflammation (Kim et al., 2015). PPT is not an initial saponin, which is transformed from a variety of ginsenoside under the action of gut microbiota in the intestine. In the whole transformation process of PPT, ginsenosides Re and Rf are the initial ginsenoside and ginsenoside Rh1 is the key secondary ginsenoside. During the transformation, ginsenoside Re can remove Glc or rhamnose (Rha) under the action of Fusobacterium, Bacteroides and Eubacterium, and then convert to F1, Rg1 and Rg2 (Bi et al., 2019). Ginsenoside Rh1, as a transferring style for PPT transformation, can be transformed from Rg1, Rg2, Rf by removing Glc, Rha and Glc under the action of Bacteroides, Eubacterium and Bifidobacterium, respectively. Under Bacteroides-mediated enzymatic hydrolysis, ginsenoside F1 and Rh1 can remove Glc at C-6 or C-20 to finally transformed into PPT (Chen et al., 2007). In addition, ginsenoside Rg1 can remove Glc, Rha at C-6 and then convert into F1 under the action of Fusobacterium and Bacteroides (Kim et al., 2011).
Summarily, we found that ginsenoside containing Rha such as Re, Rg2 could preferentially remove Rha when converted into PPT, and then hydrolyze other sugar groups. The hydrolysis of Rha for ginsenosides mainly depends on Eubacterium (Figure 2B). Besides, we concluded that Bacteroides, Bifidobacterium and Eubacterium are intestinal bacteria mainly involved in the transformation of ginsenoside. Among them, Bacteroides that degrade polysaccharides are most important bacteria. It has been found that Bacteroidetes could regulate thousands of enzymes, which can regulate specific carbohydrate degrading according to the structural information of the target glycan (Lapebie et al., 2019). As shown in Figure 3, we found that Bacteroides could induce the hydrolysis of glycosidic bonds at all possible positions. Besides, Bifidobacterium prefer to hydrolyze the glycosidic bond at position C-20, which mainly depends on its own glucosidase and glucanase, including β- glucan (Fernandez-Julia et al., 2021). Nowadays, it also has been approved that the hydrolysis by Eubacterium is closely related to β -glucosidase, but the further mechanism of ginsenoside transformation is unclear (Yang et al., 1995). According to the available evidence, Lactobacillus prefers to hydrolyze the glycosidic bond at position C-3, and the glycosidic bonds hydrolyzed by Microbacterium and Rhodanobacterium are more tended located at position C-20 (Figure 3). Collectively, Bifidobacterium and Bacteroides are the most beneficial bacteria and participate in the transformation process of ginsenosides in vivo. The therapeutic effect of ginseng depends on the further processing and modification of a variety of ginsenoside by the gut microbiota to enhance the absorption and the activity of ginsenoside in the body. At present, most researches of gut microbiota regulating ginsenoside transformation were still at an initial level, just showed the relationship between microbiota and transformation, but the internal mechanism and details need to be further clarified.
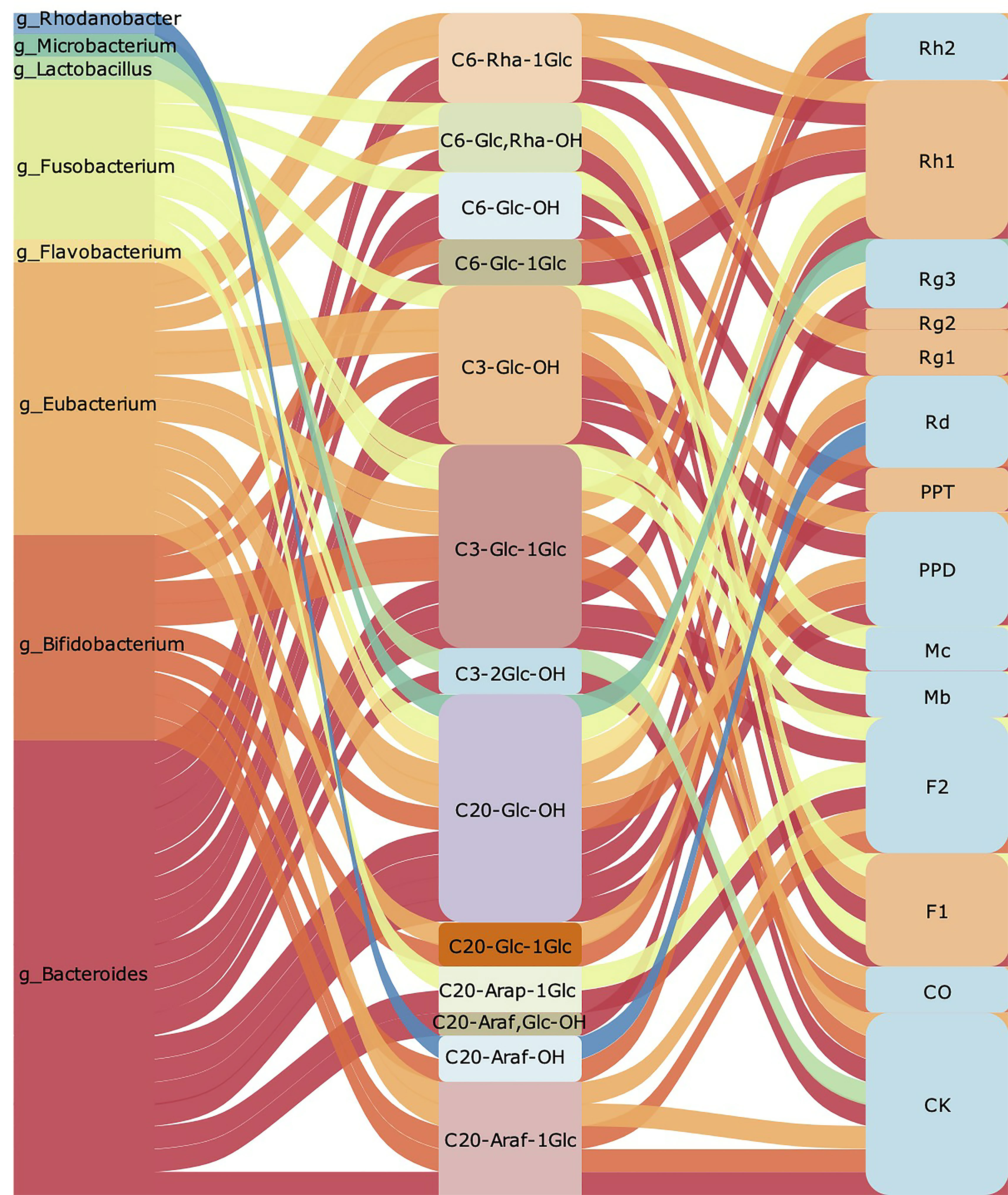
Figure 3 The summary of gut microbiota participates regulating ginsenoside transform. The left row is the gut microbiota involved in ginsenoside transformation. The middle part shows branched chain position of glycosidic bond hydrolysis, the removed sugar group, and the remaining functional groups respectively. The corresponding ginsenosides transformed are listed on the right row.
Conclusion and Future Perspective
In our review, we summarized the findings of many studies, which showed that ginseng can play a role in the prevention and treatment of obesity, diabetes, fatty liver, colitis, diarrhea, cancer, and other diseases by regulating the host microbiota. At the same time, microbiota is also the key medium of ginsenoside transformation in vivo. The pharmacological functions of ginseng against various diseases might be related with the abundance and the structure of gut microbiota, including increase the relative abundance of probiotics (Bacteroides, Lactobacillus, Bifidobacterium, Akkermansia) and reduce the relative abundance of pathogenic bacteria (Firmicutes, Helicobacter, Clostridium Proteobacteria). Ginsenosides could be transformed into CK, PPD or PPT for better activities under the actions of probiotics, including Bacteroides, Eubacterium, Bifidobacterium, and Fusobacterium. Therefore, we can use probiotics combined with ginseng to improve the pharmacodynamic value of ginseng to fully play its pharmacological effect in future. Based on the current findings, the network relationship of ginseng extract, formula containing ginseng, ginsenosides, and polysaccharides with different microbiota have summarized and shown in Figure 4.
The following aspects and suggestions about ginseng and gut microbiota should be considered, according to the research status: (1) The number of human experiments is relatively small, and most experiments use animal models, very few the studies were performed in human. (2) The detection methods for gut microbiota are mostly limited to 16S rDNA sequencing, and the changes of microbiota are not revealed deeply and specifically enough. (3) Most of the current research results only find the changes of microbiota, but there are few reports on the regulation mechanism of microbiota. (4) The detection method is single, only one detection method is used, the method of multi-omics combination is not used. (5) The ginseng used in the current studies lacks standardized and unified standards. Researchers rarely report the quality control results of ginseng, which cannot ensure the repeatability and stability of the experiment. Therefore, we suggest that future researches should focus on the species, types, and strain of gut microbiota, their metabolites, and related signaling pathways regulated by ginseng. This review can provide better insight into the interaction of ginseng with gut microbiota in multiple disorders and ginsenoside transformation.
Author Contributions
ZC and ZZ collected, analyzed, and reviewed the literature, wrote the main manuscript. JQL, HQ, QH, and JL assembled figures/tables. JQL, HQ, JL, JC, and QL added and checked references. ZZ, JM, and XL designed and supervised the manuscript. JM and XL revised the final version of the manuscript. All authors have read and agreed to the published version of the manuscript.
Funding
This study was supported by the National Natural Science Foundation of China (U19A2013, 81804013), National Key Research and Development Program of China (2017YFC1702103), the Science and Technology Development Plan Project of Jilin Province (20200404057YY, 2020YFC0845000, 202002053JC, 2020122228JC).
Conflict of Interest
The authors declare that the research was conducted in the absence of any commercial or financial relationships that could be construed as a potential conflict of interest.
Publisher’s Note
All claims expressed in this article are solely those of the authors and do not necessarily represent those of their affiliated organizations, or those of the publisher, the editors and the reviewers. Any product that may be evaluated in this article, or claim that may be made by its manufacturer, is not guaranteed or endorsed by the publisher.
Glossary
T2DM: type 2 diabetes mellitus
FLD: fatty liver disease
NF-κB: nuclear factor kappaB
NAFLD: Nonalcoholic fatty liver disease
TC: total cholesterol
TG: total triglyceride
SREBP-1c: sterol regulatory element binding protein-1c
FAS: fatty acid synthetase
ACC-1: acetyl-coenzyme A carboxylase 1
CPT-1a: carnitine palmityl transferase 1A
IκB: I-kappa-B
ZO-1: tight junction protein 1
TLR4: toll-like receptor 4
IL: interleukin
TNF-α: tumor necrosis factor-α
PPD: Proto-ginsenoside of ginsenodiol type
PPT: Proto-ginsenoside of ginsentriol type
Bif: Bifidobacterium
Fae: Faecalibacterium
Bla: Blautia
Efa: Efaecalis
Lac: Lactobacillus
Fir: Firmicutes
Bac: Bacteroidetes
Para: Parabacteroides
Ver: Verrucomicrobia
Akk: Akkermansia
Muc: Mucispirillum
Pro: Proteobacteria
Def: Deferribacteres
Hel: Helicobacter
Bar: Barnesiella
All: Allistipes
Osc: Oscillibacter
Cor: Coriobacteriaceae
Adl: Adlercreutzia
Dor: Dorea
Cop: Coprobacter
Par: Parasutterella
Clo: Clostridium
Fla: Flavonifractor
Pse: Pseudoflavonifractor
Ace: Acetatifactor
Bil: Bilophila
Ros: Roseburia
Sci: Scillibacter
Str: Streptococcus
Rum: Ruminococcus
Ana: Anaerotruncus
Ros: Roseburia
Aci: Acidobacteria
Des: Desulfovibrio
Ery: Erysipelothrix
Ver: Verrucomicrobia
Bla: Blautia
Wei: Weissella
Esc: Escherichia
Shi: Shigella
Kur: Kurthia
STZ: streptozocin
Eub: Eubacterium
Erys: Erysipelotrichaceae
Kle: Klebsiella
Meg: Megamonas
Dia: Dialister
Eps: Epsilonbacteraeota
Act: Actinobacteria
Deh: Dehalobacterium
Sut: Suterella
All: Allobaculum
Osci: Oscillospira
Pep: Peptostreptococcaceae
Col: Colibacillus
Ent: Enterococcus
Lach: Lachnospiraceae
Rik: Rikenella
Mur: Muribaculaceae
Pre: Prevotellaceae
Ara: Arabidopsis
But: Butyricicoccus
Allo: Alloprevotella
Prevo: Prevotae
TNBS: trinitro-benzene-sulfonic acid
Vei: Veillonella
Meth: Methylobacteriaceae
Pas: Pasteurellaceae
Lep: leptum
Odo: Odoribacter
Parap: Paraprevotella
Ali: Alistipes.
Pse: Pseudomonas
Acid: Acidoides
But: Butyricimonas
Cam: Campylobacter
Dys: Dysgonomonas
Bur: Burkholderiales
Ach: Acholeplasma
References
Ahmed, T., Bhuiyan, T. R., Zaman, K., Sinclair, D., Qadri, F. (2013). Vaccines for Preventing Enterotoxigenic Escherichia Coli (ETEC) Diarrhoea. Cochrane Database Syst. Rev. 2013 (7), CD009029. doi: 10.1002/14651858.CD009029.pub2
Ajebli, M., Khan, H., Eddouks, M. (2021). Natural Alkaloids and Diabetes Mellitus: A Review. Endocr. Metab. Immune Disord. Drug Targets 21, 111–130. doi: 10.2174/1871530320666200821124817
Al-Hazmi, M. A., Rawi, S. M., Arafa, N. M., Wagas, A., Montasser, A. O. (2015). The Potent Effects of Ginseng Root Extract and Memantine on Cognitive Dysfunction in Male Albino Rats. Toxicol. Ind. Health 31, 494–509. doi: 10.1177/0748233713475517
Bai, Y., Bao, X., Mu, Q., Fang, X., Zhu, R., Liu, C., et al. (2021). Ginsenoside Rb1, Salvianolic Acid B and Their Combination Modulate Gut Microbiota and Improve Glucolipid Metabolism in High-Fat Diet Induced Obese Mice. PeerJ 9, e10598. doi: 10.7717/peerj.10598
Bai, X., Fu, R., Duan, Z., Wang, P., Zhu, C., Fan, D. (2021). Ginsenoside Rk3 Alleviates Gut Microbiota Dysbiosis and Colonic Inflammation in Antibiotic-Treated Mice. Food Res. Int. 146, 110465. doi: 10.1016/j.foodres.2021.110465
Bak, M. J., Jun, M., Jeong, W. S. (2012). Antioxidant and Hepatoprotective Effects of the Red Ginseng Essential Oil in H(2)O(2)-Treated Hepg2 Cells and CCl (4)-Treated Mice. Int. J. Mol. Sci. 13, 2314–2330. doi: 10.3390/ijms13022314
Becker-Dreps, S., Gonzalez, F., Bucardo, F. (2020). Sapovirus: An Emerging Cause of Childhood Diarrhea. Curr. Opin. Infect. Dis. 33, 388–397. doi: 10.1097/QCO.0000000000000671
Bi, Y. F., Wang, X. Z., Jiang, S., Liu, J. S., Zheng, M. Z., Chen, P. (2019). Enzymatic Transformation of Ginsenosides Re, Rg1, and Rf to Ginsenosides Rg2 and Aglycon PPT by Using Beta-Glucosidase From Thermotoga Neapolitana. Biotechnol. Lett. 41, 613–623. doi: 10.1007/s10529-019-02665-7
Bocchetti, M., Ferraro, M. G., Ricciardiello, F., Ottaiano, A., Luce, A., Cossu, A. M., et al. (2021). The Role of microRNAs in Development of Colitis-Associated Colorectal Cancer. Int. J. Mol. Sci. 22 (8), 3967. doi: 10.3390/ijms22083967
Canfora, E. E., Meex, R. C. R., Venema, K., Blaak, E. E. (2019). Gut Microbial Metabolites in Obesity, NAFLD and T2DM. Nat. Rev. Endocrinol. 15, 261–273. doi: 10.1038/s41574-019-0156-z
Cani, P. D., Moens de Hase, E., Van Hul, M. (2021). Gut Microbiota and Host Metabolism: From Proof of Concept to Therapeutic Intervention. Microorganisms 9 (6), 1302. doi: 10.3390/microorganisms9061302
Cao, B., Qi, Y., Yang, Y., Liu, X., Xu, D., Guo, W., et al. (2014). 20(S)-Protopanaxadiol Inhibition of Progression and Growth of Castration-Resistant Prostate Cancer. PloS One 9, e111201. doi: 10.1371/journal.pone.0111201
Chen, W., Balan, P., Popovich, D. G. (2019). Review of Ginseng Anti-Diabetic Studies. Molecules 24 (24), 4501. doi: 10.3390/molecules24244501
Chen, H., Shen, J., Li, H., Zheng, X., Kang, D., Xu, Y., et al. (2020). Ginsenoside Rb1 Exerts Neuroprotective Effects Through Regulation of Lactobacillus Helveticus Abundance and GABAA Receptor Expression. J. Ginseng Res. 44, 86–95. doi: 10.1016/j.jgr.2018.09.002
Chen, H., Yang, H., Deng, J., Fan, D. (2021). Ginsenoside Rk3 Ameliorates Obesity-Induced Colitis by Regulating of Intestinal Flora and the TLR4/NF-kappaB Signaling Pathway in C57BL/6 Mice. J. Agric. Food Chem. 69, 3082–3093. doi: 10.1021/acs.jafc.0c07805
Chen, G., Yang, M., Lu, Z., Zhang, J., Huang, H., Liang, Y., et al. (2007). Microbial Transformation of 20(S)-Protopanaxatriol-Type Saponins by Absidia Coerulea. J. Nat. Prod. 70, 1203–1206. doi: 10.1021/np070053v
Choi, J. H., Shin, K. C., Oh, D. K. (2018). An L213A Variant of Beta-Glycosidase From Sulfolobus Solfataricus With Increased Alpha-L-Arabinofuranosidase Activity Converts Ginsenoside Rc to Compound K. PloS One 13 (1), e0191018. doi: 10.1371/journal.pone.0191018
Crovesy, L., Masterson, D., Rosado, E. L. (2020). Profile of the Gut Microbiota of Adults With Obesity: A Systematic Review. Eur. J. Clin. Nutr. 74, 1251–1262. doi: 10.1038/s41430-020-0607-6
Del Prato, S., Chilton, R. (2018). Practical Strategies for Improving Outcomes in T2DM: The Potential Role of Pioglitazone and DPP4 Inhibitors. Diabetes Obes. Metab. 20, 786–799. doi: 10.1111/dom.13169
Fan, Y., Pedersen, O. (2021). Gut Microbiota in Human Metabolic Health and Disease. Nat. Rev. Microbiol. 19, 55–71. doi: 10.1038/s41579-020-0433-9
Fan, J., Wang, Y., You, Y., Ai, Z., Dai, W., Piao, C., et al. (2019). Fermented Ginseng Improved Alcohol Liver Injury in Association With Changes in the Gut Microbiota of Mice. Food Funct. 10, 5566–5573. doi: 10.1039/C9FO01415B
Fan, S., Zhang, Z., Su, H., Xu, P., Qi, H., Zhao, D., et al. (2020). Panax Ginseng Clinical Trials: Current Status and Future Perspectives. BioMed. Pharmacother. 132, 110832. doi: 10.1016/j.biopha.2020.110832
Fernandez-Julia, P. J., Munoz-Munoz, J., van Sinderen, D. (2021). A Comprehensive Review on the Impact of Beta-Glucan Metabolism by Bacteroides and Bifidobacterium Species as Members of the Gut Microbiota. Int. J. Biol. Macromol. 181, 877–889. doi: 10.1016/j.ijbiomac.2021.04.069
Friedman, S. L., Neuschwander-Tetri, B. A., Rinella, M., Sanyal, A. J. (2018). Mechanisms of NAFLD Development and Therapeutic Strategies. Nat. Med. 24, 908–922. doi: 10.1038/s41591-018-0104-9
Gallo, A., Passaro, G., Gasbarrini, A., Landolfi, R., Montalto, M. (2016). Modulation of Microbiota as Treatment for Intestinal Inflammatory Disorders: An Uptodate. World J. Gastroenterol. 22, 7186–7202. doi: 10.3748/wjg.v22.i32.7186
Gibiino, G., Lopetuso, L. R., Scaldaferri, F., Rizzatti, G., Binda, C., Gasbarrini, A. (2018). Exploring Bacteroidetes: Metabolic Key Points and Immunological Tricks of Our Gut Commensals. Dig Liver Dis. 50, 635–639. doi: 10.1016/j.dld.2018.03.016
Gui, Q. F., Xu, Z. R., Xu, K. Y., Yang, Y. M. (2016). The Efficacy of Ginseng-Related Therapies in Type 2 Diabetes Mellitus: An Updated Systematic Review and Meta-Analysis. Med. (Baltimore) 95 (6), e2584. doi: 10.1097/MD.0000000000002584
Guo, M., Ding, S., Zhao, C., Gu, X., He, X., Huang, K., et al. (2015). Red Ginseng and Semen Coicis can Improve the Structure of Gut Microbiota and Relieve the Symptoms of Ulcerative Colitis. J. Ethnopharmacol. 162, 7–13. doi: 10.1016/j.jep.2014.12.029
Guo, M., Shao, S., Wang, D., Zhao, D., Wang, M. (2021). Recent Progress in Polysaccharides From Panax Ginseng C. A. Meyer. Food Funct. 12, 494–518. doi: 10.1039/D0FO01896A
Gu, D., Yi, H., Jiang, K., Fakhar, S. H., Shi, J., He, Y., et al. (2021). Transcriptome Analysis Reveals the Efficacy of Ginsenoside-Rg1 in the Treatment of Nonalcoholic Fatty Liver Disease. Life Sci. 267, 118986. doi: 10.1016/j.lfs.2020.118986
Han, S. K., Joo, M. K., Kim, J. K., Jeung, W., Kang, H., Kim, D. H. (2020). Bifidobacteria-Fermented Red Ginseng and Its Constituents Ginsenoside Rd and Protopanaxatriol Alleviate Anxiety/Depression in Mice by the Amelioration of Gut Dysbiosis. Nutrients 12 (4), 901. doi: 10.3390/nu12040901
Han, M. J., Kim, D. H. (2020). Effects of Red and Fermented Ginseng and Ginsenosides on Allergic Disorders. Biomolecules 10 (4), 634. doi: 10.3390/biom10040634
Hering, N. A., Fromm, M., Schulzke, J. D. (2012). Determinants of Colonic Barrier Function in Inflammatory Bowel Disease and Potential Therapeutics. J. Physiol. 590, 1035–1044. doi: 10.1113/jphysiol.2011.224568
Himanshu, D., Ali, W., Wamique, M. (2020). Type 2 Diabetes Mellitus: Pathogenesis and Genetic Diagnosis. J. Diabetes Metab. Disord. 19, 1959–1966. doi: 10.1007/s40200-020-00641-x
Hong, J. T., Lee, M. J., Yoon, S. J., Shin, S. P., Bang, C. S., Baik, G. H., et al. (2021). Effect of Korea Red Ginseng on Nonalcoholic Fatty Liver Disease: An Association of Gut Microbiota With Liver Function. J. Ginseng Res. 45, 316–324. doi: 10.1016/j.jgr.2020.07.004
Huang, G., Khan, I., Li, X., Chen, L., Leong, W., Ho, L. T., et al. (2017). Ginsenosides Rb3 and Rd Reduce Polyps Formation While Reinstate the Dysbiotic Gut Microbiota and the Intestinal Microenvironment in Apc(Min/+) Mice. Sci. Rep. 7, 12552. doi: 10.1038/s41598-017-12644-5
Huang, J., Liu, D., Wang, Y., Liu, L., Li, J., Yuan, J., et al. (2022). Ginseng Polysaccharides Alter the Gut Microbiota and Kynurenine/Tryptophan Ratio, Potentiating the Antitumour Effect of Antiprogrammed Cell Death 1/Programmed Cell Death Ligand 1 (Anti-PD-1/PD-L1) Immunotherapy. Gut 71 (4), 734. doi: 10.1136/gutjnl-2020-321031
Hughes, L. E., Ford, C., Brookes, M. J., Gama, R. (2021). Bile Acid Diarrhoea: Current and Potential Methods of Diagnosis. Ann. Clin. Biochem. 58, 22–28. doi: 10.1177/0004563220966139
Hu, L., Huang, X., You, C., Li, J., Hong, K., Li, P., et al. (2017). Prevalence of Overweight, Obesity, Abdominal Obesity and Obesity-Related Risk Factors in Southern China. PloS One 12, e0183934. doi: 10.1371/journal.pone.0183934
Jeon, H., Kim, H. Y., Bae, C. H., Lee, Y., Kim, S. (2020). Korean Red Ginseng Regulates Intestinal Tight Junction and Inflammation in the Colon of a Parkinson's Disease Mouse Model. J. Med. Food 23, 1231–1237. doi: 10.1089/jmf.2019.4640
Jiang, J., Sun, X., Akther, M., Lian, M. L., Quan, L. H., Koppula, S., et al. (2020). Ginsenoside Metabolite 20(S)-Protopanaxatriol From Panax Ginseng Attenuates Inflammation-Mediated NLRP3 Inflammasome Activation. J. Ethnopharmacol. 251, 112564. doi: 10.1016/j.jep.2020.112564
Jin, L., Zhou, W., Li, R., Jin, M., Jin, C., Sun, J., et al. (2021). A New Polyacetylene and Other Constituents With Anti-Inflammatory Activity From Artemisia Halodendron. Nat. Prod. Res. 35, 1010–1013. doi: 10.1080/14786419.2019.1610962
Jin, X., Zhou, J., Zhang, Z., Lv, H. (2018). The Combined Administration of Parthenolide and Ginsenoside CK in Long Circulation Liposomes With Targeted Tlyp-1 Ligand Induce Mitochondria-Mediated Lung Cancer Apoptosis. Artif. Cells Nanomed. Biotechnol. 46, S931–S942. doi: 10.1080/21691401.2018.1518913
Ji, H. F., Shen, L. (2021). Probiotics as Potential Therapeutic Options for Alzheimer's Disease. Appl. Microbiol. Biotechnol. 105, 7721–7730. doi: 10.1007/s00253-021-11607-1
Jo, H., Jang, D., Park, S. K., Lee, M. G., Cha, B., Park, C., et al. (2021). Ginsenoside 20(S)-Protopanaxadiol Induces Cell Death in Human Endometrial Cancer Cells via Apoptosis. J. Ginseng Res. 45, 126–133. doi: 10.1016/j.jgr.2020.02.002
Kim, D. H. (2018). Gut Microbiota-Mediated Pharmacokinetics of Ginseng Saponins. J. Ginseng Res. 42, 255–263. doi: 10.1016/j.jgr.2017.04.011
Kim, H. I., Kim, J. K., Kim, J. Y., Han, M. J., Kim, D. H. (2019). Fermented Red Ginseng and Ginsenoside Rd Alleviate Ovalbumin-Induced Allergic Rhinitis in Mice by Suppressing IgE, Interleukin-4, and Interleukin-5 Expression. J. Ginseng Res. 43, 635–644. doi: 10.1016/j.jgr.2019.02.006
Kim, H. S., Lim, J. M., Kim, J. Y., Kim, Y., Park, S., Sohn, J. (2016). Panaxydol, a Component of Panax Ginseng, Induces Apoptosis in Cancer Cells Through EGFR Activation and ER Stress and Inhibits Tumor Growth in Mouse Models. Int. J. Cancer 138, 1432–1441. doi: 10.1002/ijc.29879
Kim, D. Y., Ro, J. Y., Lee, C. H. (2015). 20(S)-Protopanaxatriol Inhibits Release of Inflammatory Mediators in Immunoglobulin E-Mediated Mast Cell Activation. J. Ginseng Res. 39, 189–198. doi: 10.1016/j.jgr.2014.11.001
Kim, M. J., Upadhyaya, J., Yoon, M. S., Ryu, N. S., Song, Y. E., Park, H. W., et al. (2018). Highly Regioselective Biotransformation of Ginsenoside Rb2 Into Compound Y and Compound K by Beta-Glycosidase Purified From Armillaria Mellea Mycelia. J. Ginseng Res. 42, 504–511. doi: 10.1016/j.jgr.2017.07.001
Kim, Y. S., Yoo, M. H., Lee, G. W., Choi, J. G., Kim, K. R., Oh, D. K. (2011). Ginsenoside F1 Production From Ginsenoside Rg1 by a Purified Beta-Glucosidase From Fusarium Moniliforme Var. Subglutinans. Biotechnol. Lett. 33, 2457–2461. doi: 10.1007/s10529-011-0719-0
Kolodziejczyk, A. A., Zheng, D., Shibolet, O., Elinav, E. (2019). The Role of the Microbiome in NAFLD and NASH. EMBO Mol. Med. 11 (2), e9302. doi: 10.15252/emmm.201809302
Lapebie, P., Lombard, V., Drula, E., Terrapon, N., Henrissat, B. (2019). Bacteroidetes Use Thousands of Enzyme Combinations to Break Down Glycans. Nat. Commun. 10, 2043. doi: 10.1038/s41467-019-10068-5
Lee, S. Y., Jeong, J. J., Eun, S. H., Kim, D. H. (2015). Anti-Inflammatory Effects of Ginsenoside Rg1 and its Metabolites Ginsenoside Rh1 and 20(S)-Protopanaxatriol in Mice With TNBS-Induced Colitis. Eur. J. Pharmacol. 762, 333–343. doi: 10.1016/j.ejphar.2015.06.011
Lee, Y. Y., Saba, E., Irfan, M., Kim, M., Chan, J. Y., Jeon, B. S., et al. (2019). The Anti-Inflammatory and Anti-Nociceptive Effects of Korean Black Ginseng. Phytomedicine 54, 169–181. doi: 10.1016/j.phymed.2018.09.186
Lee, S. Y., Yuk, H. G., Ko, S. G., Cho, S. G., Moon, G. S. (2021). Gut Microbiome Prolongs an Inhibitory Effect of Korean Red Ginseng on High-Fat-Diet-Induced Mouse Obesity. Nutrients 13 (3), 926. doi: 10.3390/nu13030926
Liang, W., Zhou, K., Jian, P., Chang, Z., Zhang, Q., Liu, Y., et al. (2021). Ginsenosides Improve Nonalcoholic Fatty Liver Disease via Integrated Regulation of Gut Microbiota, Inflammation and Energy Homeostasis. Front. Pharmacol. 12, 622841. doi: 10.3389/fphar.2021.622841
Li, J., Li, R., Li, N., Zheng, F., Dai, Y., Ge, Y., et al. (2018). Mechanism of Antidiabetic and Synergistic Effects of Ginseng Polysaccharide and Ginsenoside Rb1 on Diabetic Rat Model. J. Pharm. BioMed. Anal. 158, 451–460. doi: 10.1016/j.jpba.2018.06.024
Li, X., Liu, J., Zuo, T. T., Hu, Y., Li, Z., Wang, H. D., et al. (2022). Advances and Challenges in Ginseng Research From 2011 to 2020: The Phytochemistry, Quality Control, Metabolism, and Biosynthesis. Nat. Prod. Rep. doi: 10.1039/D1NP00071C
Li, X. Y., Sun, L. W., Zhao, D. Q. (2019). Current Status and Problem-Solving Strategies for Ginseng Industry. Chin. J. Integr. Med. 25, 883–886. doi: 10.1007/s11655-019-3046-2
Liu, H., Liu, M., Fu, X., Zhang, Z., Zhu, L., Zheng, X., et al. (2018). Astaxanthin Prevents Alcoholic Fatty Liver Disease by Modulating Mouse Gut Microbiota. Nutrients 10 (9), 1298. doi: 10.3390/nu10091298
Liu, B. N., Liu, X. T., Liang, Z. H., Wang, J. H. (2021). Gut Microbiota in Obesity. World J. Gastroenterol. 27, 3837–3850. doi: 10.3748/wjg.v27.i25.3837
Liu, H., Lu, X., Hu, Y., Fan, X. (2020). Chemical Constituents of Panax Ginseng and Panax Notoginseng Explain Why They Differ in Therapeutic Efficacy. Pharmacol. Res. 161, 105263. doi: 10.1016/j.phrs.2020.105263
Liu, Z., Wang, H. (2021). Probiotics Alleviate Inflammatory Bowel Disease in Mice by Regulating Intestinal Microorganisms-Bile Acid-NLRP3 Inflammasome Pathway. Acta Biochim. Pol 68 (4), 687–693. doi: 10.18388/abp.2020_5597
Li, F. T., Yang, D., Song, F. Y., Liu, M., Dai, Y. L., Zheng, F., et al. (2020). In Vitro Effects of Ginseng and the Seed of Zizyphus Jujuba Var Spinosa on Gut Microbiota of Rats With Spleen Deficiency. Chem. Biodivers 17(9), e2000199.. doi: 10.1002/cbdv.202000199
Lu, Z., Liu, H., Fu, W., Wang, Y., Geng, J., Wang, Y., et al. (2020). 20(S)-Protopanaxadiol Inhibits Epithelial-Mesenchymal Transition by Promoting Retinoid X Receptor Alpha in Human Colorectal Carcinoma Cells. J. Cell Mol. Med. 24, 14349–14365. doi: 10.1111/jcmm.16054
Lu, C., Lv, J., Dong, L., Jiang, N., Wang, Y., Wang, Q., et al. (2018). Neuroprotective Effects of 20(S)-Protopanaxatriol (PPT) on Scopolamine-Induced Cognitive Deficits in Mice. Phytother. Res. 32, 1056–1063. doi: 10.1002/ptr.6044
Ocker, M. (2020). Fibroblast Growth Factor Signaling in Non-Alcoholic Fatty Liver Disease and Non-Alcoholic Steatohepatitis: Paving the Way to Hepatocellular Carcinoma. World J. Gastroenterol. 26, 279–290. doi: 10.3748/wjg.v26.i3.279
Orbe-Orihuela, Y. C., Lagunas-Martinez, A., Bahena-Roman, M., Madrid-Marina, V., Torres-Poveda, K., Flores-Alfaro, E., et al. (2018). High Relative Abundance of Firmicutes and Increased TNF-Alpha Levels Correlate With Obesity in Children. Salud Publica Mex 60, 5–11. doi: 10.21149/8133
Pak, J. N., Jung, J. H., Park, J. E., Hwang, J., Lee, H. J., Shim, B. S., et al. (2020). P53 Dependent LGR5 Inhibition and Caspase 3 Activation are Critically Involved in Apoptotic Effect of Compound K and its Combination Therapy Potential in HCT116 Cells. Phytother. Res. 34, 2745–2755. doi: 10.1002/ptr.6717
Pan, C., Guo, Q., Lu, N. (2019). Role of Gut Microbiota in the Pharmacological Effects of Natural Products. Evid Based Complement. Alternat. Med. 2019, 2682748. doi: 10.1155/2019/2682748
Perry, R. J., Peng, L., Barry, N. A., Cline, G. W., Zhang, D., Cardone, R. L., et al. (2016). Acetate Mediates a Microbiome-Brain-Beta-Cell Axis to Promote Metabolic Syndrome. Nature 534, 213–217. doi: 10.1038/nature18309
Polyzos, S. A., Kountouras, J., Mantzoros, C. S. (2019). Obesity and Nonalcoholic Fatty Liver Disease: From Pathophysiology to Therapeutics. Metabolism 92, 82–97. doi: 10.1016/j.metabol.2018.11.014
Qi, Y. L., Li, S. S., Qu, D., Chen, L. X., Gong, R. Z., Gao, K., et al. (2019). Effects of Ginseng Neutral Polysaccharide on Gut Microbiota in Antibiotic-Associated Diarrhea Mice. Zhongguo Zhong Yao Za Zhi 44 (4), 811–818. doi: 10.19540/j.cnki.cjcmm.20181129.002
Quan, L. H., Kim, Y. J., Li, G. H., Choi, K. T., Yang, D. C. (2013). Microbial Transformation of Ginsenoside Rb1 to Compound K by Lactobacillus Paralimentarius. World J. Microbiol. Biotechnol. 29, 1001–1007. doi: 10.1007/s11274-013-1260-1
Quan, L. H., Zhang, C., Dong, M., Jiang, J., Xu, H., Yan, C., et al. (2020). Myristoleic Acid Produced by Enterococci Reduces Obesity Through Brown Adipose Tissue Activation. Gut 69, 1239–1247. doi: 10.1136/gutjnl-2019-319114
Qu, Q., Yang, F., Zhao, C., Liu, X., Yang, P., Li, Z., et al. (2021). Effects of Fermented Ginseng on the Gut Microbiota and Immunity of Rats With Antibiotic-Associated Diarrhea. J. Ethnopharmacol. 267, 113594. doi: 10.1016/j.jep.2020.113594
Ratan, Z. A., Haidere, M. F., Hong, Y. H., Park, S. H., Lee, J. O., Lee, J., et al. (2021). Pharmacological Potential of Ginseng and its Major Component Ginsenosides. J. Ginseng Res. 45, 199–210. doi: 10.1016/j.jgr.2020.02.004
Reintam Blaser, A., Deane, A. M., Fruhwald, S. (2015). Diarrhoea in the Critically Ill. Curr. Opin. Crit. Care 21, 142–153. doi: 10.1097/MCC.0000000000000188
Ren, Z., Chen, X., Hong, L., Zhao, X., Cui, G., Li, A., et al. (2020). Nanoparticle Conjugation of Ginsenoside Rg3 Inhibits Hepatocellular Carcinoma Development and Metastasis. Small 16, e1905233. doi: 10.1002/smll.201905233
Robles-Alonso, V., Guarner, F. (2013). Progress in the Knowledge of the Intestinal Human Microbiota. Nutr. Hosp. 28 (3), 553–557. doi: 10.3305/nh.2013.28.3.6601
Roh, E., Hwang, H. J., Kim, J. W., Hong, S. H., Kim, J. A., Lee, Y. B., et al. (2020). Ginsenoside Mc1 Improves Liver Steatosis and Insulin Resistance by Attenuating ER Stress. J. Ethnopharmacol. 259, 112927. doi: 10.1016/j.jep.2020.112927
Safaei, M., Sundararajan, E. A., Driss, M., Boulila, W., Shapi'i, A. (2021). A Systematic Literature Review on Obesity: Understanding the Causes & Consequences of Obesity and Reviewing Various Machine Learning Approaches Used to Predict Obesity. Comput. Biol. Med. 136, 104754. doi: 10.1016/j.compbiomed.2021.104754
Sah, N., Wu, G., Bazer, F. W. (2021). Regulation of Gene Expression by Amino Acids in Animal Cells. Adv. Exp. Med. Biol. 1332, 1–15. doi: 10.1007/978-3-030-74180-8_1
Santangelo, R., Silvestrini, A., Mancuso, C. (2019). Ginsenosides, Catechins, Quercetin and Gut Microbiota: Current Evidence of Challenging Interactions. Food Chem. Toxicol. 123, 42–49. doi: 10.1016/j.fct.2018.10.042
Seong, E., Bose, S., Han, S. Y., Song, E. J., Lee, M., Nam, Y. D., et al. (2021). Positive Influence of Gut Microbiota on the Effects of Korean Red Ginseng in Metabolic Syndrome: A Randomized, Double-Blind, Placebo-Controlled Clinical Trial. EPMA J. 12, 177–197. doi: 10.1007/s13167-021-00243-4
Shankar, S., Rosenbaum, J. (2020). Chronic Diarrhoea in Children: A Practical Algorithm-Based Approach. J. Paediatr. Child Health 56, 1029–1038. doi: 10.1111/jpc.14986
Shen, H., Gao, X. J., Li, T., Jing, W. H., Han, B. L., Jia, Y. M., et al. (2018). Ginseng Polysaccharides Enhanced Ginsenoside Rb1 and Microbial Metabolites Exposure Through Enhancing Intestinal Absorption and Affecting Gut Microbial Metabolism. J. Ethnopharmacol. 216, 47–56. doi: 10.1016/j.jep.2018.01.021
Shen, Z. H., Zhu, C. X., Quan, Y. S., Yang, Z. Y., Wu, S., Luo, W. W., et al. (2018). Relationship Between Intestinal Microbiota and Ulcerative Colitis: Mechanisms and Clinical Application of Probiotics and Fecal Microbiota Transplantation. World J. Gastroenterol. 24, 5–14. doi: 10.3748/wjg.v24.i1.5
Shin, H. J., Kim, D. H., Zhong, X., Yum, H. W., Kim, S. J., Chun, K. S., et al. (2020). Preventive Effects of Korean Red Ginseng on Experimentally Induced Colitis and Colon Carcinogenesis. J. Tradit. Complement. Med. 10, 198–206. doi: 10.1016/j.jtcme.2020.04.004
Song, M. Y., Kim, B. S., Kim, H. (2014). Influence of Panax Ginseng on Obesity and Gut Microbiota in Obese Middle-Aged Korean Women. J. Ginseng Res. 38, 106–115. doi: 10.1016/j.jgr.2013.12.004
Stojanov, S., Berlec, A., Strukelj, B. (2020). The Influence of Probiotics on the Firmicutes/Bacteroidetes Ratio in the Treatment of Obesity and Inflammatory Bowel Disease. Microorganisms 8 (11), 1715. doi: 10.3390/microorganisms8111715
Sun, Y., Chen, S., Wei, R., Xie, X., Wang, C., Fan, S., et al. (2018). Metabolome and Gut Microbiota Variation With Long-Term Intake of Panax Ginseng Extracts on Rats. Food Funct. 9, 3547–3556. doi: 10.1039/C8FO00025E
Tan, G. S. E., Tay, H. L., Tan, S. H., Lee, T. H., Ng, T. M., Lye, D. C. (2020). Gut Microbiota Modulation: Implications for Infection Control and Antimicrobial Stewardship. Adv. Ther. 37, 4054–4067. doi: 10.1007/s12325-020-01458-z
Wang, J., Feng, W., Zhang, S., Chen, L., Sheng, Y., Tang, F., et al. (2019a). Ameliorative Effect of Atractylodes Macrocephala Essential Oil Combined With Panax Ginseng Total Saponins on 5-Fluorouracil Induced Diarrhea is Associated With Gut Microbial Modulation. J. Ethnopharmacol. 238, 111887. doi: 10.1016/j.jep.2019.111887
Wang, J., Feng, W., Zhang, S., Chen, L., Tang, F., Sheng, Y., et al. (2019b). Gut Microbial Modulation in the Treatment of Chemotherapy-Induced Diarrhea With Shenzhu Capsule. BMC Complement. Altern. Med. 19, 126. doi: 10.1186/s12906-019-2548-y
Wang, J. F., He, M., Guo, W. J., Zhang, Y. H., Sui, X., Lin, J. A., et al. (2021b). Microbiome-Metabolomics Reveals Endogenous Alterations of Energy Metabolism by the Dushen Tang to Attenuate D-Galactose-Induced Memory Impairment in Rats. BioMed. Res. Int. 2021, 6649085. doi: 10.1155/2021/6649085
Wang, L., Lu, J., Zeng, Y., Guo, Y., Wu, C., Zhao, H., et al. (2020). Improving Alzheimer's Disease by Altering Gut Microbiota in Tree Shrews With Ginsenoside Rg1. FEMS Microbiol. Lett. 367(4), fnaa011. doi: 10.1093/femsle/fnaa011
Wang, D., Shao, S., Zhang, Y., Zhao, D., Wang, M. (2021). Insight Into Polysaccharides From Panax Ginseng C. A. Meyer in Improving Intestinal Inflammation: Modulating Intestinal Microbiota and Autophagy. Front. Immunol. 12, 683911. doi: 10.3389/fimmu.2021.683911
Wei, Y., Yang, H., Zhu, C., Deng, J., Fan, D. (2020). Hypoglycemic Effect of Ginsenoside Rg5 Mediated Partly by Modulating Gut Microbiota Dysbiosis in Diabetic Db/Db Mice. J. Agric. Food Chem. 68, 5107–5117. doi: 10.1021/acs.jafc.0c00605
Xiong, W., Zhao, X. Q., Xu, Q., Wei, G. H., Zhang, L. D., Fan, Y. Q., et al. (2022). Qisheng Wan Formula Ameliorates Cognitive Impairment of Alzheimer's Disease Rat via Inflammation Inhibition and Intestinal Microbiota Regulation. J. Ethnopharmacol. 282, 114598. doi: 10.1016/j.jep.2021.114598
Xi, L., Song, Y., Han, J., Qin, X. (2021). Microbiome Analysis Reveals the Significant Changes in Gut Microbiota of Diarrheic Baer's Pochards (Aythya Baeri). Microb. Pathog. 157, 105015. doi: 10.1016/j.micpath.2021.105015
Xu, J., Chen, H. B., Li, S. L. (2017). Understanding the Molecular Mechanisms of the Interplay Between Herbal Medicines and Gut Microbiota. Med. Res. Rev. 37, 1140–1185. doi: 10.1002/med.21431
Xu, J., Li, T., Xia, X., Fu, C., Wang, X., Zhao, Y. (2020). Dietary Ginsenoside T19 Supplementation Regulates Glucose and Lipid Metabolism via AMPK and PI3K Pathways and Its Effect on Intestinal Microbiota. J. Agric. Food Chem. 68, 14452–14462. doi: 10.1021/acs.jafc.0c04429
Xu, W., Wang, B., Gao, Y., Cai, Y., Zhang, J., Wu, Z., et al. (2021). Alkaloids Exhibit a Meaningful Function as Anticancer Agents by Restraining Cellular Signaling Pathways. Mini Rev. Med. Chem. doi: 10.2174/1389557521666211007114935
Yang, L., Akao, T., Kobashi, K. (1995). Purification and Characterization of a Geniposide-Hydrolyzing Beta-Glucosidase From Eubacterium SpA-44, a Strict Anaerobe From Human Feces. Biol. Pharm. Bull. 18, 1175–1178. doi: 10.1248/bpb.18.1175
Yang, H. J., Kim, M. J., Kwon, D. Y., Kim, D. S., Zhang, T., Ha, C., et al. (2018). Combination of Aronia, Red Ginseng, Shiitake Mushroom and Nattokinase Potentiated Insulin Secretion and Reduced Insulin Resistance With Improving Gut Microbiome Dysbiosis in Insulin Deficient Type 2 Diabetic Rats. Nutrients 10(7), 948. doi: 10.3390/nu10070948
Yang, L., Zou, H., Gao, Y., Luo, J., Xie, X., Meng, W., et al. (2020). Insights Into Gastrointestinal Microbiota-Generated Ginsenoside Metabolites and Their Bioactivities. Drug Metab. Rev. 52, 125–138. doi: 10.1080/03602532.2020.1714645
Yoon, S. J., Kim, S. K., Lee, N. Y., Choi, Y. R., Kim, H. S., Gupta, H., et al. (2021). Effect of Korean Red Ginseng on Metabolic Syndrome. J. Ginseng Res. 45, 380–389. doi: 10.1016/j.jgr.2020.11.002
Younossi, Z. M., Koenig, A. B., Abdelatif, D., Fazel, Y., Henry, L., Wymer, M. (2016). Global Epidemiology of Nonalcoholic Fatty Liver Disease-Meta-Analytic Assessment of Prevalence, Incidence, and Outcomes. Hepatology 64, 73–84. doi: 10.1002/hep.28431
Zhang, M., Wang, Y., Wu, Y., Li, F., Han, M., Dai, Y., et al. (2021). In Vitro Transformation of Protopanaxadiol Saponins in Human Intestinal Flora and Its Effect on Intestinal Flora. Evid Based Complement. Alternat. Med. 2021, 1735803. doi: 10.1155/2021/1735803
Zhang, H., Xu, H. L., Wang, Y. C., Lu, Z. Y., Yu, X. F., Sui, D. Y. (2018). 20(S)-Protopanaxadiol-Induced Apoptosis in MCF-7 Breast Cancer Cell Line Through the Inhibition of PI3K/AKT/mTOR Signaling Pathway. Int. J. Mol. Sci. 19 (4), 1053. doi: 10.3390/ijms19041053
Zhang, Z., Zhang, Y., Li, J., Fu, C., Zhang, X. (2021). The Neuroprotective Effect of Tea Polyphenols on the Regulation of Intestinal Flora. Molecules 26 (12), 3692. doi: 10.3390/molecules26123692
Zhao, B., Lv, C., Lu, J. (2019). Natural Occurring Polysaccharides From Panax Ginseng CA. Meyer: A Review of Isolation, Structures, and Bioactivities. Int. J. Biol. Macromol. 133, 324–336. doi: 10.1016/j.ijbiomac.2019.03.229
Zhao, C., Qu, Q., Yang, F., Li, Z., Yang, P., Han, L., et al. (2021). Monascus Ruber Fermented Panax Ginseng Ameliorates Lipid Metabolism Disorders and Modulate Gut Microbiota in Rats Fed a High-Fat Diet. J. Ethnopharmacol. 278, 114300. doi: 10.1016/j.jep.2021.114300
Zheng, J., Chen, M., Ye, C., Sun, X., Jiang, N., Zou, X., et al. (2020). BuZangTongLuo Decoction Improved Hindlimb Ischemia by Activating Angiogenesis and Regulating Gut Microbiota in Diabetic Mice. J. Ethnopharmacol. 248, 112330. doi: 10.1016/j.jep.2019.112330
Zheng, Z. Y., Xie, G., Liu, H. X., Tan, G. L., Li, L., Liu, W. L., et al. (2021). Fermented Ginseng Leaf Enriched With Rare Ginsenosides Relieves Exercise-Induced Fatigue via Regulating Metabolites of Muscular Interstitial Fluid, Satellite Cells-Mediated Muscle Repair and Gut Microbiota. J. Funct. Foods 83, 104509. doi: 10.1016/j.jff.2021.104509
Zheng, M., Xin, Y., Li, Y., Xu, F., Xi, X., Guo, H., et al. (2018). Ginsenosides: A Potential Neuroprotective Agent. BioMed. Res. Int. 2018, 8174345. doi: 10.1155/2018/8174345
Zhou, S. S., Auyeung, K. K., Yip, K. M., Ye, R., Zhao, Z. Z., Mao, Q., et al. (2020). Stronger Anti-Obesity Effect of White Ginseng Over Red Ginseng and the Potential Mechanisms Involving Chemically Structural/Compositional Specificity to Gut Microbiota. Phytomedicine 74, 152761. doi: 10.1016/j.phymed.2018.11.021
Zhou, L., Zheng, Y., Li, Z., Bao, L., Dou, Y., Tang, Y., et al. (2016). Compound K Attenuates the Development of Atherosclerosis in ApoE(-/-) Mice via LXRalpha Activation. Int. J. Mol. Sci. 17 (7), 1054. doi: 10.3390/ijms17071054
Zhou, S. S., Zhou, J., Xu, J. D., Shen, H., Kong, M., Yip, K. M., et al. (2021). Ginseng Ameliorates Exercise-Induced Fatigue Potentially by Regulating the Gut Microbiota. Food Funct. 12, 3954–3964. doi: 10.1039/D0FO03384G
Keywords: ginseng (Panax ginseng C.A. Meyer), gut microbiota, multiple disorders, gisenoside transformation, ginsenoside
Citation: Chen Z, Zhang Z, Liu J, Qi H, Li J, Chen J, Huang Q, Liu Q, Mi J and Li X (2022) Gut Microbiota: Therapeutic Targets of Ginseng Against Multiple Disorders and Ginsenoside Transformation. Front. Cell. Infect. Microbiol. 12:853981. doi: 10.3389/fcimb.2022.853981
Received: 13 January 2022; Accepted: 24 March 2022;
Published: 25 April 2022.
Edited by:
Rudolf Bauer, University of Graz, AustriaReviewed by:
Xiaobo Zhang, Zhejiang University, ChinaYolanda B. Lombardo, National University of the Littoral, Argentina
Copyright © 2022 Chen, Zhang, Liu, Qi, Li, Chen, Huang, Liu, Mi and Li. This is an open-access article distributed under the terms of the Creative Commons Attribution License (CC BY). The use, distribution or reproduction in other forums is permitted, provided the original author(s) and the copyright owner(s) are credited and that the original publication in this journal is cited, in accordance with accepted academic practice. No use, distribution or reproduction is permitted which does not comply with these terms.
*Correspondence: Jia Mi, bWlqaWE4MjAxQDEyNi5jb20=; Xiangyan Li, eGlhbmd5YW5fbGkxOTgxQDE2My5jb20=
†These authors have contributed equally to this work