The mechanism of berberine alleviating metabolic disorder based on gut microbiome
- 1School of Chinese Materia Medica, Beijing University of Chinese Medicine, Beijing, China
- 2Guang’anmen Hospital, China Academy of Chinese Medical Sciences, Beijing, China
- 3Shenzhen Hospital (Futian), Guangzhou University of Chinese Medicine, Shenzhen, China
With socioeconomic advances and improved living standards, metabolic syndrome has increasingly come into the attention. In recent decades, a growing number of studies have shown that the gut microbiome and its metabolites are closely related to the occurrence and development of many metabolic diseases, and play an important role that cannot be ignored, for instance, obesity, type 2 diabetes (T2DM), non-alcoholic fatty liver disease (NAFLD), cardiovascular disease and others. The correlation between gut microbiota and metabolic disorder has been widely recognized. Metabolic disorder could cause imbalance in gut microbiota, and disturbance of gut microbiota could aggravate metabolic disorder as well. Berberine (BBR), as a natural ingredient, plays an important role in the treatment of metabolic disorder. Studies have shown that BBR can alleviate the pathological conditions of metabolic disorders, and the mechanism is related to the regulation of gut microbiota: gut microbiota could regulate the absorption and utilization of berberine in the body; meanwhile, the structure and function of gut microbiota also changed after intervention by berberine. Therefore, we summarize relevant mechanism research, including the expressions of nitroreductases-producing bacteria to promote the absorption and utilization of berberine, strengthening intestinal barrier function, ameliorating inflammation regulating bile acid signal pathway and axis of bacteria-gut-brain. The aim of our study is to clarify the therapeutic characteristics of berberine further and provide the theoretical basis for the regulation of metabolic disorder from the perspective of gut microbiota.
1. Introduction
Metabolic disorders are a complex group of multifactorial disorders formed by one or more causes of glucose metabolism, lipid metabolism, purine metabolism and so on, including central obesity, insulin resistance, abnormal glucose metabolism, lipid metabolism disorders, non-alcoholic fatty liver disease(NFLD), and metabolic hypertension, among others (Kassi et al., 2011). With the improvement of living standards, the incidence of metabolic disorder is also increasing. The existence of multiple metabolic disorders not only increases the incidence rate of diabetes directly, but also greatly increases the risk of atherosclerotic cardiovascular disease, brings enormous burden to the global medical and health field (2001). Insulin resistance is the core factor of metabolic syndrome, it is also a common pathological manifestation of metabolic disorders such as diabetes and obesity (Balkau and Charles, 1999; Huang, 2009). The current situation shows that a large proportion of metabolic syndrome patients suffer from impaired glucose tolerance or diabetes (Shinkov et al., 2018).
The relationship between the occurrence of metabolic disorders with the gut microbiota is one of the main research directions to explore the mechanism of metabolic disorders at present (Meijnikman et al., 2018). The human gut flora is a very complex system of a great variety, according to current reports, more than 1000 kinds of microorganisms in the human gastrointestinal tract are known, which belong to five phyla: Bacteroidetes, Firmicutes, Actinobacteria, Proteobacteria, and Verrucomicrobia (Wu et al., 2021). Among which, anaerobic Bacteroides and Firmicutes are the two dominant species, accounting for more than 90% of all bacterial species (Qin et al., 2010). Numerous studies have shown that alterations in the composition of the gut microbiota have been associated with metabolic disorders (Festi et al., 2014; Ussar et al., 2015), including obesity (Gomes et al., 2018), DM (Baothman et al., 2016; Yang et al., 2021), high fat diet (Yin et al., 2018; Leeming et al., 2019; Schoeler and Caesar, 2019), antibiotics (Ianiro et al., 2016; Ramirez et al., 2020), and other factors can lead to gut microbiota dysbiosis (Jin et al., 2017; Greenhill, 2018; Guerre, 2020; Philippe, 2020), and at the same time, the gut microbiota can be mediated by drugs that regulate the body’s metabolism and play a role in improving metabolic disorders (Feng et al., 2020).
Consistently, as a natural medicine, berberine plays an important role in the treatment of metabolic disorders. Its mechanism of utility has also been extensively and intensively studied (Caliceti et al., 2016), a large number of experimental studies have shown that berberine alleviates body metabolism and insulin resistance through a variety of mechanisms, such as amelioration of oxidative stress, inhibition of macrophage inflammatory response, adenosine monophosphate-activated protein kinas (AMPK) activation and Phosphorylation of acetyl-CoA carboxylase (ACC), regulating mitochondria-related pathways (Zhang et al., 2018), inducing peroxisome proliferator-activated receptors (PPARs) increase6Modulating transcriptional programs of transcription factors, among others (Wang et al., 2020). In recent years, the mechanism of berberine in treating metabolic disorders by modulating gut microbiota has been increasingly discovered and recognized. In this paper, the author focuses on the mechanism of BBR ameliorate metabolic disorder, so as to further clarify the therapeutic characteristics of BBR, providing a theoretical basis for regulating and alleviating metabolic disorder from the perspective of gut microbiome.
2.Berberine for metabolic disorders
Berberine (BBR) is a quaternary ammonium salt from the group of bioactive isoquinoline alkaloids (Imenshahidi and Hosseinzadeh, 2016), which is the major active component of traditional Chinese herb Coptis chinensis (Tabeshpour et al., 2017). In addition, BBR also exist in various medicinal plants such as Berberis aristata, B. petiolaris, B. aquifolium, B. vulgaris, B. thunbergia and many others (Cicero and Baggioni, 2016; Liu et al., 2019). Its chemical structure is represented in Figure 1. In recent years, a large number of research confirmed that BBR has extensive pharmacological properties, such as anti-inflammatory (Kuo et al., 2004), pain‐relieving (Hashemzaei and Rezaee, 2021), anti-infective (Aswathanarayan and Vittal, 2018), antitumor (Liu et al., 2019),neuroprotective (Luo et al., 2012) and modulate energy metabolism, which can be used to treat cancer (Zhang et al., 2020), digestive (Zou et al., 2017), metabolic (Xu et al., 2021), cardiovascular (Martini et al., 2020), and neurological diseases (Kulkarni and Dhir, 2010). Formulas containing traditional herbal Coptis chinensis, has been used for thousands of years in traditional Chinese Medicine. Most of these formulas possess the efficacy of clearing heat, eliminating dampness, dissipating fire and detoxifying (Wang et al., 2017), similar to what we now considered as anti-infective and anti-inflammatory functions.
Accumulating clinical studies have shown that BBR has a good role in the treatment of metabolic diseases such as diabetes, obesity, non-alcoholic fatty liver disease and hyperlipidemia. BBR could ameliorate insulin resistance in patients with diabetes, lower blood sugar and hemoglobin A1c (HbA1c) in patients with T2DM (Yin et al., 2008; Zhang et al., 2010). Obesity is closely related to an increased risk of T2DM, BBR could successfully decrease body weight, body mass index, waist circumference in obese patients with T2DM (Xu et al., 2021). In addition, BBR can improve the hepatic fat content, apolipoprotein B (Apo B), alanine aminotransferase (ALT), and aspartate aminotransferase (AST) in patients with NAFLD (Yan et al., 2015). Furthermore, BBR treatment can substantial reduction in TC, TG, and LDL-C levels in patients with Hyperlipidemia (Kong et al., 2004).
In addition to clinical studies, berberine has been extensively explored in the treatment of metabolic syndrome in terms of mechanisms. Berberine has many extensive and intensive studies in the field of metabolic diseases. It can regulate and ameliorate glycolipid metabolism (Ma et al., 2018; Yu et al., 2019; Bertuccioli et al., 2020), lose weight (Neyrinck et al., 2021) and alleviation of insulin resistance (Memon et al., 2018), especially in the treatment of combined multiple metabolic disorders, the efficacy of BBR is outstanding (Yu-Guang et al., 2017). Meanwhile, numerous experimental studies have shown that BBR ameliorate body metabolic dysfunction through multiple mechanisms (Yin et al., 2012; Ilyas et al., 2020). In terms of glucose metabolism, BBR strengthened autophagy and protected from high glucose-related injury in podocytes by promoting the AMPK activation, at the same time, the bidirectional regulation of AMPK activity can reduce the risk of hypoglycemia caused by berberine (Xiao et al., 2018). BBR also through TLR4/MyD88/NF-κB signaling pathway improves gut-derived hormones (Gong et al., 2017a), alleviate inflammation by reducing the exogenous antigen load in the host (Zhang et al., 2012). In the regulation of blood lipids, BBR can decrease triglyceride expression in HepG2 cell lines (Cao et al., 2018), modulation of bile acids (He et al., 2016a) and upregulation of Trib1 mRNA levels to reduce lipid levels (Singh and Liu, 2019). With further in-depth studies scholars have found that modulation of gut microbiome may be another novel mechanism of action for berberine to ameliorate metabolic disorders (Hvistendahl, 2012; Feng et al., 2015).
3. Impact of gut microbiota on metabolic disorders
Earlier studies have shown that alterations in the gut microbiota are environmental factors that influence host susceptibility to obesity and increase adiposity (Backhed et al., 2004). When feces from obese and lean pairs of twins were transplanted separately into germ free mice, mice transplanted with feces from obese recipients gained weight and exhibited an obesity associated metabolic phenotype, whereas mice transplanted with feces from lean recipients showed no significant metabolic phenotype (Ridaura et al., 2013). Therefore, gut microbiota is strongly associated with the occurrence of metabolic disorders.
On the one hand, the findings suggest that metabolic disorders significantly affect the structure and function of the gut microbiota. On the other hand, gut microbiota can produce metabolites by fermenting dietary fiber, for instance, short-chain fatty acids (SCFAs) and bile acids (BAs), have important metabolic functions (Canfora et al., 2019), can significantly affect body metabolism function. The Firmicutes/Bacteroidetes (F/B) ratio is widely accepted to have an important influence in maintaining normal intestinal homeostasis, the increase of F/B will lead to obesity (Stojanov et al., 2020). Numerous studies have confirmed that, humans or animals with obesity/insulin resistance or diabetes have an elevated abundance of opportunistic pathogens (such as sulfate reducing bacteria) and a decreased abundance of beneficial bacteria (as F.prausnitzii (Xu et al., 2020a), A.muciniphila, R.intestinalis, Bifidobacterium and Akkermansia muciniphila.) in the gut (Everard et al., 2011; Qin et al., 2012; Tilg and Moschen, 2014; Feng et al., 2015; Cani, 2019). Further studies revealed that certain specific bacteria have important effects on body metabolism, such as the endotoxin producing bacterium E.clocae isolated from obese patients, which can cause obesity and insulin resistance in axenic mice (Fei and Zhao, 2013). It follows that the metabolic function of the organism interacts with the gut microbiome, and modulation of the gut microbiota can improves metabolic function.
In recent years, a large number of studies have focused on the relationship between berberine and gut microbiota. Research shows can ameliorate metabolic abnormalities by modulating the flora to enhance bioavailability (Liu et al., 2016), regulating the bacteria-brain-gut axis (Sun et al., 2016; Sun et al., 2017), strengthening intestinal barrier function (Zhang et al., 2012; Gong et al., 2017a), alleviating metabolic endotoxemia (Gao et al., 2017), increasing of short-chain fatty acid (SCFA)-producing bacteria (Mcnabney and Henagan, 2017) and other ways. Now, these mechanisms will be systematically summarized and elaborated in this review.
4. Mechanism of berberine ameliorates metabolic disorders based on gut microbiome
Berberine can induce cell death of harmful intestinal bacteria and increase the number and species of beneficial bacteria (Habtemariam, 2020). From administration to absorption, BBR can be metabolized by gut microbiota to improve its therapeutic effects on metabolism related diseases such as diabetes, hyperlipidemia, or directly affect gut microbiota to regulate and ameliorate metabolic disorders (Cui et al., 2018). The gut microbiota cuts down BBR to the absorbable form of DhBBR, which converts to BBR and enters the blood after absorption in intestinal tissue, so as to improve the bioavailability of BBR (Feng et al., 2015). Studies have shown that BBR can increase its oral availability and relieve metabolic disorder by reversing the changes in the quantity, structure and composition of gut microbiota under the pathological conditions (Table 1). At the same time, BBR improves intestinal barrier function and reduces the inflammation of metabolism related diseases by regulating gut microbiota. In addition, BBR achieves energy balance by regulating gut microbiota dependent metabolites (such as LPS, SCFAs, BAs) and related downstream pathways. What’s more, it can improve gastrointestinal hormones and metabolic disorders by regulating bacterial-brain-gut axis (Figure 2).
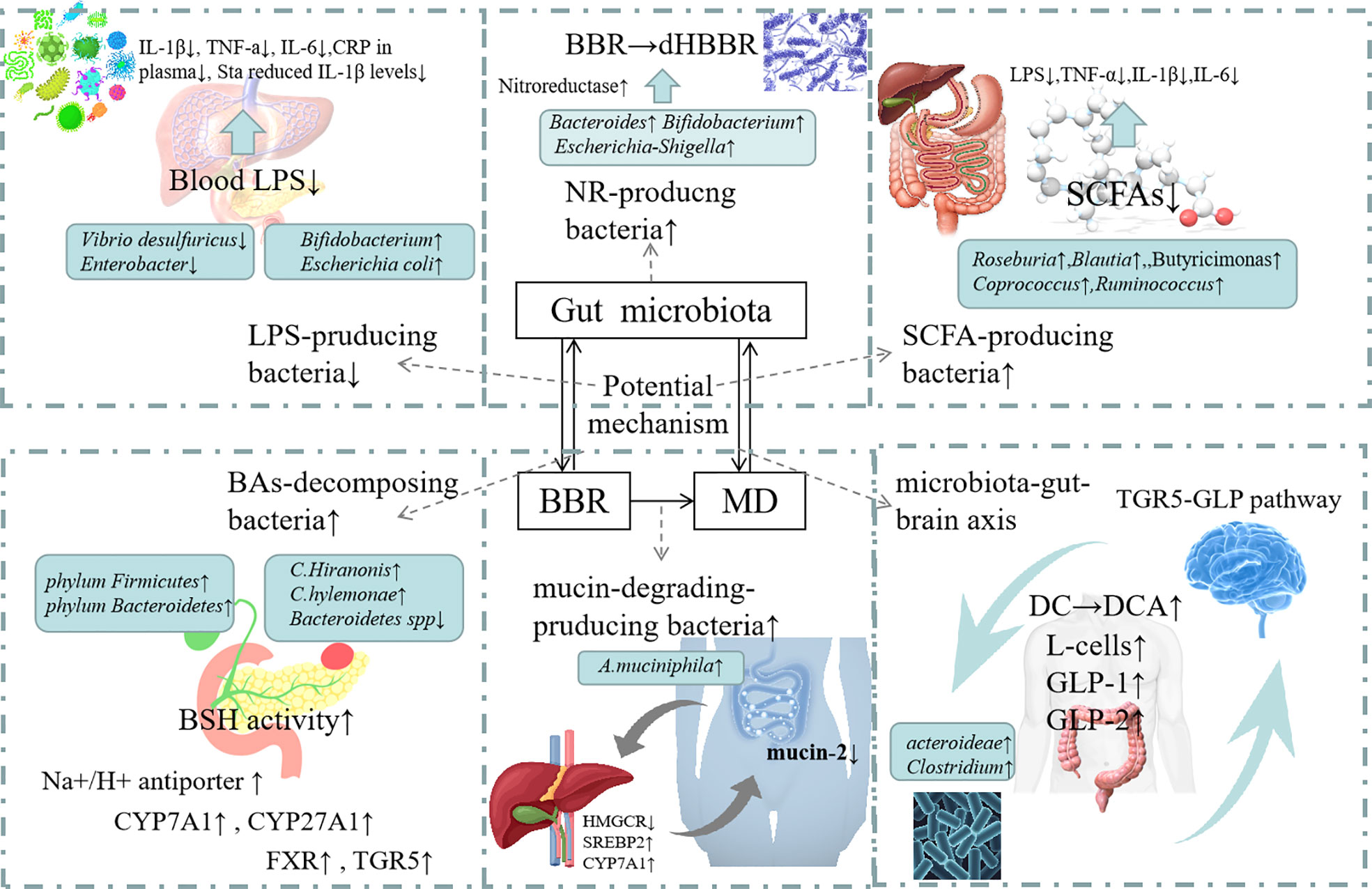
Figure 2 Mechanism of action of Berberine in modulating Gut Microbioata. Increasing of the NR-producing bacteria: BBR can increase th abundance of Bacteroides, Escherichia-Shigella and Bifidobacterium which can produc NRs. Nrs converts BBR into its absorbable form of DhBBR, which has highly polar and easily absorbed into the blood. Increasing of mucin-degrading-producing bacteria: BBR can increase the abundance of A.muciniphila. The increased abundance of A.muciniphila may lead to the reduction in mucin-2 expression in ileum. BBR seemed to protect the intestinal barrier integrity through modulating HMGCT, SREBP2 and CYP7A1 expressions. Decreasing of LPS-producing bacteria: BBR reduced the level of Vibrio desulfuricus and Enterobacter to inhibit the production of LPS. So that inflammatory factors (IL-1β, TNF-a IL-6, CRP in plasma Sta reduced IL-1β levels) were decreased. Increasing of SCFA-producing bacteria: BBR increased the number of SCFA producing bacteria (such as Butyricimonas, Coprococcus, Ruminococcus and Roseburia), raised the expression of pro-inflammatory cytokines, including LPS, TNF-α IL-1β and IL-6. Increasing of BAs-decomposing bacteria: BBR increased the number of BAs-decomposing bacteria (such as phylum Firmicutes, phylum Bacteroidetes, C. scindens and C. hylemonae) and reduced the level of C. hiranosis, decreased the activity of BSH. The possible mechanism is to Up-regulate Na+/H+ antiporter, up-regulate colonic TGR5 expression and GLP secretion and to increase CYP7A1 and CYP27A1 expression. Regulating microbiota-gut-brain axis: BBR increases the propotion of Bacteroidetes and Firmicutes, increases the expression of serum GLP-1, GLP-2, increase of the number of L.cells.
4.1 Increasing of NR-producing bacteria to promote the utilization of BBR
The oral bioavailability of Berberine is low (< 1%) as a result of poor aqueous solubility, the molecular structure of berberine prevents its rapid absorption from the intestine (Habtemariam, 2020). The extremely low plasma concentrations following oral administration of BBR in experimental or clinical settings are not sufficient to achieve the effects observed in in vitro experiments, which presents challenges in explaining its excellent and diverse pharmacological effects in clinical trials (Tan et al., 2013; Liu et al., 2016). Consequently, the investigators try to find out solutions in order to solve the problem of poor bioavailability (Liu et al., 2010). Previous researches have shown that gut microbiota participates in the metabolism of most oral drugs in vivo both directly and indirectly (Zhang et al., 2020). Some chemical components have been metabolized before the first-pass effect of liver, and the activity or toxicity of drugs can be significantly changed, affecting the exertion of drug efficacy (Sousa et al., 2008; Possemiers et al., 2011). Efficacy of intestinal microbiota in drug action remains underappreciated as before. There is increasing evidence that gut microbiota plays an important role in treatment through interaction with drugs. It contains a variety of metabolic enzymes, which can trigger a series of drug metabolic reactions and embodied the important role of gut bacteria for oral absorption of BBR (Xu et al., 2017).
Nitroreductases (NRs) are bacterial enzymes that reduce nitro-containing compounds which play key roles in BBR intestinal absorption. It has been found that various types of nitroreductase producing bacteria, such as Enterobacter, can produce nitroreductase to catalyze the reduction reaction of BBR to Dihydroberberine (DhBBR), which is a key bacterium to promote the intestinal absorption of BBR,NRs turns BBR into its absorbable form (DhBBR), which has highly polar and easily absorbed into the blood, and it is rapidly converted into BBR by oxidation after entering the blood. Studies have confirmed that the absorption rate of DhBBR is 5-fold correspond to BBR in animals (Direct, 2002). Some studies have also shown that after the increase of NR producing bacteria, the oral utilization and clinical efficacy of BBR can be improved. The experiment has shown that increasing fecal NR activity by approximately 10% in the gut microbiota can increase blood BBR concentrations by 65-70% in both animal experiments and human studies, suggesting that bacterial NR has a highly efficient function in converting BBR to DhBBR (Wang et al., 2017a).
Previous studies have shown that the elevation of fecal NR caused by HFD was due to an increased proportion of NR-producing bacteria in the gut or their increased activity. The bioavailability of BBR (100 mg/kg/d) in HFD-fed hamsters was higher than in normal chow-fed hamsters when administered orally. HFD caused increased mortality in the abundance of Enterobacter spp in mice, and BBR decreased blood lipids (such as TC, TG, LDL) in the HFD-fed hamsters, but did not play a role in those who keep with general diet (Wang et al., 2017a). In contrast, another researcher used antibiotics to reduce gut microbiota as a control group, inhibitory effect of oral treatment on gut bacteria in mice with type 2 diabetes decreased the BBR-to-DhBBR conversion and the concentration of BBR in the blood, at the same time, the lipid-lowering and hypoglycemic effects of BBR were reduced (Feng et al., 2015).Clinical study showed that NR activity in feces was higher in hyperlipidemia patients than in healthy subjects. Correlation analysis also showed that blood BBR was positively correlated with fecal NR activity. Therefore, it is reasonable to suggest that fecal NR activity is one of the effective biomarkers of BBR in the treatment of hyperlipidemia (Wang et al., 2017a).
Therefore, it is believed that HFD increases the proportion of NR producers in the gut bacterial community and/or the NR activity in bacteria. Studies showed an increase in the NR-producing bacteria in the gut microbiota community with Bacteroides (Schapiro et al., 2004), Escherichia-Shigella (Fu et al., 2007), and Bifidobacterium (Kinouchi et al., 1982), whose growth rate were the the highest among the top 50 bacterial strains. Moreover, Ru Feng et al. (2018) studied the pharmacokinetics of gut microbiota modulating BBR (50 mg/kg/d) and active metabolites in Beagle Dogs, as a result, an increase in the number of bacteria producing nitroreductase was observed in Escherichia–Shigella and Bacteroides.
Increasing of NR-producing bacteria was speculated to be one of the mechanisms of BBR improving metabolism. Nitroreductase activity is not only a biomarker to predict the therapeutic effect of BBR, but also an important target to improve the efficacy of BBR.
4.2 Increasing of mucin-degrading-producing bacteria to strengthen the intestinal barrier function
Intestinal barrier function is closely related to the occurrence and development of metabolic diseases (Scheithauer et al., 2020). T2DM is well known as a metabolic disease with low level chronic inflammation. Mucin-2 is a large glycoprotein that maintains intestinal balance by forming a physical barrier between the intestinal contents and the epithelium which is a very important glycoprotein (Paone and Cani, 2020). Thass CA (Thaiss et al., 2018) firmly believed glucose as an orchestrator of intestinal barrier function. Hyperglycemia destroys the integrity and balance of intestinal epithelial cells, interferes with the function of tight junction protein and adhesion protein between intestinal epithelial cells, leads to intestinal barrier dysfunction, increases the spread of intestinal infection, and then aggravates metabolic disorder. BBR can ameliorate endoplasmic‐reticulum stress and reduce apoptosis of goblet cells by reducing the expression of mucin‐2. Therefore, BBR has the effect of strengthening intestinal barrier.
Akkermansia muciniphila (A.muciniphila) has been authenticated as a mucin-degrading in the mucus layer, which has been proved to prevent the development of obesity and associated complications (Plovier et al., 2017). A.muciniphila treatment reversed metabolic disturbances caused by taking a high-fat diet, such as metabolic endotoxemia, adipose tissue inflammation, increased fat mass and insulin resistance in humans (Everard et al., 2013). Surprisingly, this bacterium is called a mucus degrader, and adding A.muciniphila increases the number of goblet cells and the production of antimicrobial peptides, which stimulated mucus production (Paone and Cani, 2020). A.muciniphila plays an active role in improving host metabolism and its abundance is often inversely correlated with metabolic disturbances in most preclinical and clinical studies (Zhou and Zhang, 2019), some studies made known a negative correlation among A. muciniphila and markers associated with metabolic disorders (Remely et al., 2015). A study has also shown that he decrease of circulating endotoxin levels mediated by A muciniphila can be attributed to the induction of intestinal expression of tight junction proteins (occult band protein-1 and occludin), thus reversing the increase of intestinal permeability induced by Western diet (Li et al., 2016). After BBR intervention, the abundance of A.muciniphila in B6 mice induced by high-fat diet was 19.1 times higher than that in the control group (He et al., 2016a). A feature of Diabetic rats fed with high fat and high-carbonhydrate diet were proinflammatory intestinal changes, altered gut-derived hormones, and what’s more, Intestinal permeability can be increased by 2.77-fold than rats on a regular diet. However, BBR treatment can significantly reverse the above changes, reduce the inflammatory changes of intestinal immune system and reduce the damage of intestinal barrier. Besides, high concentration of BBR treatment can reduce the intestinal permeability of diabetic rats by 27.5%. The researchers speculate that BBR seems to protect the integrity of intestinal barrier by regulating the expression of ZO-1 and OCLN (He et al., 2016a). Also, BBR can significantly increasing the abundance of bacteria such as phylum Verrucomicrobia, especially A.muciniphila. To decrease the FBG levels, improve the impaired oral glucose tolerance, and ameliorate the balance of α‐ and β‐cells in diabetic db/db mice, The increased abundance of A.muciniphila may lead to the reduction in mucin‐2 expression in ileum (Li et al., 2020). Atherosclerosis (AS) is the main co-morbidity of metabolic syndrome, research lends credence to a possible indirect role of IL-17 in determining or favoring early (Tarantino et al., 2014). This beneficial effect of BBR was associated with the regulation of gut microbiota, particularly with increased abundance of Akkermansia (Yang et al., 2021).
Interestingly, Gavage of BBR multiplied the abundance of A.muciniphila in rats. However, it did not stimulate A.muciniphila growth in direct incubation, which can be seen that BBR may promote A.muciniphila in a host-dependent way (Dong et al., 2021). How BBR stimulates A.muciniphila remains further research. But it can be confirmed that BBR may regulate tight junction protein and protect the integrity of intestinal barrier by increasing mucin degrading bacteria.
4.3 Decreasing of LPS-producing bacteria to improve metabolic endotoxemia
Studies (Cani et al., 2007a; Cani et al., 2008) proved that metabolic endotoxemia leads to inflammatory reaction which can causes weight gain and diabetes. Endotoxin plasma lipopolysaccharide (LPS) is one of the strong virulence factors of Gram-negative bacterial species, released upon lysis by LPS producing bacteria. It plays an important role in both acute infections and chronic infections (Kallio et al., 2008). It is a major active component in the generation of toxic effects from endotoxin and an initiating factor in metabolic endotoxemia. This “metabolic endotoxemia” has been widely recognized as an important reason for triggering or promoting obesity, insulin resistance, metabolic syndrome, and ultimately leading to diabetes (Cani et al., 2007b). A high-fat diet leads to an increase in the abundance of intestinal Gram-negative bacteria, such as Proteus. A large amount of LPS produced by Proteus enters the blood because of the increase of intestinal permeability. Binding of lipopolysaccharide to the complex of CD14 and toll-like receptor 4 on the surface of innate immune cells, LPS can induce systemic inflammation, which ultimately impairs insulin sensitivity and induces insulin resistance-related metabolic disorders (Pussinen et al., 2011).
Proteobacteria belongs to unstable potential pro-inflammatory flora. Enterobacter, Vibrio desulfuricus and Enterobacter cloacae increase significantly in the state of metabolic disorder, and then cause metabolic diseases (Bai et al., 2016). The abundances of Desulfovibrio genus were rised in obesity and T2DM (Van Hul et al., 2018). Desulfovibrio is supposed to an opportunistic pathogen that can produce endotoxins. The researchers found that BBR reversed these increases and reduced the level of Vibrio desulfuricus, in addition, they discovered that BBR treatment reduced inflammation of KKAy mice at least in part by regulating TLR4, ERK, and p38MAPK pathways (Cao et al., 2020). Some other researches showed that BBR could reduce the abundance of Vibrio desulfuricus (Zou et al., 2015) and Enterobacter (Weglarz et al., 2007) cloacae and inhibit the production of LPS. To investigate the role of BBR-mediated modulation of gut microbiota in reducing host inflammation and ameliorating insulin resistance-related metabolic abnormalities, reviewers (Zhang et al., 2012) measured the serum concentration of LBP which is a biomarker of circulating LPS. they found that HFD induced a significant increase in serum LBP level in rats, which co-administration with BBR essentially prevented, suggesting a potential role for gut microbiota antigens in this pharmacological process. Another study (Zhang et al., 2011) showed that BBR significantly reduced intestinal injury induced by LPS injury rat model and decreased serum levels of downstream inflammatory cytokines. What’s more, it is also one of the results of this study that the combined administration of BBR can significantly prevent HFD induced systemic inflammation. A Long-term HFD altered the gut microbiota composition by reducing protective bacteria like Bifidobacterium and increasing gram negative bacteria like Escherichia coli, resulting in increased release of LPS into plasma. A study showed that BBR set-back these effects and restrained LPS-induced TLR4/TNF-α activation, leading to increased insulin receptor and insulin receptor substrate-1 expression in the liver and reduce insulin resistance (Liu et al., 2018).
Gut microbial Dysbiosis affects the integrity of the intestinal epithelium and increases blood levels of LPS (Cani and Delzenne, 2009; Tagliabue and Elli, 2013). Meanwhile, HFD induces high levels of circulating LPS, which promotes metabolic inflammation and insulin resistance (Cani et al., 2007a; Cani et al., 2007b). Demonstrated that lacking the IL-17 cytokine receptor (IL-17RA−/−) mice in the HFD group exhibited increased intestinal permeability, with increased levels of LPS in vat of IL-17RA−/− compared with C57BL/6 wild-type (WT) mice, suggesting that LPS may negatively affect insulin signaling and aggravate insulin resistance in these mice. Through a series of experimental findings, the investigators showed the importance of the IL-17/IL-17R axis in the metabolic and immunological alterations associated with the development of obesity and metabolic syndrome, by driving intestinal neutrophil migration, limiting intestinal Dysbiosis and attenuating LPS translocation to visceral adipose tissue (VAT) (Perez et al., 2019).
An up-regulation of Th17-pattern-related cytokines such as IL-6, TNFa, IL-17 and IL-22 in the intestine and increased Th17 cell. Reported data reveal that BBR can directly suppress functions and differentiation of pro-inflammatory Th1 and Th17 cells, and indirectly decrease Th cell-mediated inflammation through modulating or suppressing other cells assisting autoreactive inflammation, such as CD4+ Foxp3+ T regulatory (Tregs), dendritic cells (DCs) and macrophages (Ehteshamfar et al., 2020). Therefore, berberine may also treat metabolic syndrome by regulating intestinal permeability, alleviating inflammatory factors entering the bloodstream, and thereby attenuating insulin resistance.
In conclusion, BBR can significantly reduce the abundance of Proteobacteria, such as Desulfovibrio, Enterobacter cloacae, and inhibit LPS production, effectively prevent serum LBP elevation (Zhang et al., 2012), regulating intestinal permeability, attenuating insulin resistance and improve metabolic endotoxemia.
4.4 Increasing of short-chain fatty acid (SCFA)-producing bacteria to regulate inflammatory response
To a certain extent, the occurrence and development of metabolic disorders are closely related to changes in the composition of the gut microbiota and its metabolites, such as short chain fatty acids (SCFA), can significantly affect glycolipid and energy metabolism. SCFAs, mainly produced by gut bacteria ferment carbohydrates and degrade aromatic compounds, including acetic, propionic, butyric among others, have the functions of promoting regeneration of epithelial cells (Mcnabney and Henagan, 2017), strengthening intestinal barrier function, suppressing part of the inflammatory response induced by LPS, and regulating of cells in skeletal muscle, liver, and fat to Alleviating glycemic homeostasis and insulin sensitivity (Park et al., 2015). Both animal and human researches have shown that the increase of SCFAs concentration in feces is related to weight gain, fat accumulation and insulin resistance, which may be due to the increase of SCFAs production and the decrease of SCFAs absorption (Sircana et al., 2018). SCFAs work as a mediator between gut microbiota, they have the potential to improve glucose homeostasis and insulin sensitivity in patients with T2DM, and in the setting of pancreatic dysfunction, they can regulate pancreatic insulin and glucagon secretion through GLP1 augmentation in pancreatic dysfunction (Mandaliya and Seshadri, 2019). Among these, the main energy of colon cells comes from butyric acid, increasing satiety and reducing food intake (Archer et al., 2004), and is also a regulator of inflammation, modulating chronic inflammation by activating anti-inflammatory Treg cells and inhibiting pro-inflammatory cytokine and chemokine response pathways, while modulating tight junction protein expression to adjust epithelial barrier function and intestinal permeability (Wei et al., 2018).However, a high-fat diet caused dysregulation of SCFAs producing bacteria structure and decreased abundance of SCFAs producing bacteria, reducing the distribution of SCFAs in the intestine and inhibiting them from exerting normal physiological functions, result in the body occurrence a series of metabolic related diseases (Zhang et al., 2015).
Reports indicated that BBR was provided with an effect on anti-diabetic by regulating short-chain fatty acids (SCFAs). BBR intervention changed the intestinal microflora of db/db mice, increased the number of SCFA producing bacteria (such as Butyricimonas, Coprococcus, Ruminococcus), reduced body weight, blood sugar level and intestinal inflammation in db/db mice (Zhang et al., 2019).Regarding the way BBR works in the body, current research believes that there are two aspects: one is the direct effect of circulating BBR, and the other is the indirect effect of butyrate through the intestinal microorganism, increased abundance of Escherichia-Shigella, Clostridium sensu stricto 1, and Bacteroides may be one of the reasons for the increase of butyrate production (Wang et al., 2017b). The anti-atherosclerotic effect of BBR is also related to changes in composition and functions of gut microbiota. Wu, M et al. (Wu et al., 2020) studied the effects of berberine on atherosclerosis and gut microbiota regulation in ApoE (-/-) mice fed a high-fat diet, after treatment with BBR, atherosclerotic lesions was decreased and it significantly reduces total cholesterol, and very low-density lipoprotein cholesterol levels. In detail, BBR enriched the abundance of Turicibacter, Alistipes, Roseburia, Allobaculum, and Blautia, and changed the abundance of Bilophila. These microbiota showed good anti-inflammatory effects, which are related to the production of SCFAs and significantly reduce pro-inflammatory cytokines (including TNF)- α6 IL-1 β And IL-6), they believe that reducing inflammation may be an important mechanism for the reduction of atherosclerosis in HFD-fed mice treated with BBR. After treated with BBR, Xu, X., et al (Xu et al., 2020) found Clostridium XIVa, Faecalibacterium, Ruminococcus2, Coprococcus, Dorea, Butyricicoccus, and Roseburia were markedly enriched in the gut microbiota. These bacteria are butyric acid producing bacteria and play a beneficial role in the host (Koh et al., 2016). In addition, increased production of fecal SCFAs was also detected in Xu’s study. Butyricoccus, Allobaculum, Phascolarctobacterium, Blautia and Bacteriodes were markedly increased by BBR in the high-fat diet-induced rats as bacteria that can produce SCFAs (Zhang et al., 2015). The efficacy was evaluated after 7 days of BBR treatment in beagle dogs, Feng, R et al. (Feng et al., 2018) found that the abundance of seven butyrate-producing genera increased than before. Escherichia-Shigella, Clostridium sensu stricto 1, Megamonas, Bacteroides, Ruminococcus and Blautia could produce butyate. That is to say BBR regulates metabolism by increasing the abundance of butyric acid producing bacteria. However, experiments in humans have also shown that BBR reduces acetate and propionate production but has no effect on butyrate levels, and that, at the same time, the use of BBR increases the abundance of Faecalibacterium and decreases the abundance of Bifidobacterium, Streptococcus and Enterococcus (Fu et al., 2022).Treatment with berberine in combination with Bifidobacterium could increase the abundance of beneficial bacteria in the intestine of diabetic patients and achieve better glucose lowering effect because of the inhibitory effect of berberine on Bifidobacterium (Ming et al., 2021).
4.5 Increasing of BAs-decomposing bacteria to regulate BAs-signal
Bile acids (BAs) are well known to be important metabolic and inflammatory signaling molecules that modulate lipid and energy-related nuclear hormone receptors, including farnesoid X receptor (FXR) and transmembrane G protein-coupled receptor 5 (TGR5 or GPBAR1) (Fiorucci et al., 2010). Bile acid synthesis is the main pathway of cholesterol excretion in vivo. Bile acids can activate TGR5 and FXR receptors, regulate blood glucose, increase glycogen synthesis, inhibit liver glycogen synthesis, protect islet cell function and maintain blood glucose homeostasis (Li and Chiang, 2014). BBR can increase the abundance of bacteria that promote the decomposition of bound bile acids and enhance the expression of bile acid receptors FXR and TGR5. BBR is considered to be an agonist of FXR and TGR5 and regulate bile acid signal and function (Han et al., 2017). In addition, bile acids have antibacterial properties, inhibit the growth of bacteria in the intestine and form a strong selective pressure on the intestinal flora. At the same time, bile acids are modified and regulated by microorganisms in the intestine (Fiorucci and Distrutti, 2015).
Research showed that BBR can significantly affect cholesterol metabolism and/or bile acid biosynthesis, suggesting that the circulating of bile acids is related to the lipid-lowering function of BBR. Previous reports have indicated that BBR treatment can increase the transforming of cholesterol to bile acid and reduce the level of cholesterol in the blood (Sun et al., 2017), and it affects the metabolism of hepatic lipids and cholesterol by increasing bile acid excretion into the large intestine (Mcdonald et al., 2012). In vitro studies on bile acid metabolism in the gut also showed that BBR could significantly reduce bile acid catabolism by gut microbiota in HFD hamsters. Meanwhile, Researchers predicted that BBR significantly increased CYP7A1 and CYP27A1 expression. The regulation of adipose genetic expression by insulin and fatty acids is mainly mediated by transcriptional factor, for instance SREBPs (Debose-Boyd and Ye, 2018). CYP7A1 is considered to be the classical rate-limiting enzyme for the conversion of cholesterol to bile acids. Meanwhile, this study found that BBR treatment can promote the expression of CYP7A1 in the liver of obese mice. It can be seen that the hypolipidemic effect of BBR may be related to its up-regulation of SREBP2 and CYP7A1 expression and promotion of bile acid metabolism (Feng et al., 2018).. Guo Y. et al. (Guo et al., 2016) found that BBR can significantly improve the content of BAs in serum, which is realized as an increase in primary BAs but a decrease in secondary BAs. Bacteroides were also observed to be enriched in the terminal ileum and large intestine of BBR-treated mice. A study showed that berberine compound increased the relative abundance of Proteobacteria and decreased Firmicutes and TM7 enrichment, which accelerate conversion of primary bile acid cholic acid (CA) into secondary bile acid deoxycholic acid (DCA) in ob/ob mice, and DCA upregulated colonic TGR5 expression and GLP secretion, thus acting as a hypoglycemic agent (Li et al., 2020). A randomized, double-blind, placebo-controlled clinical trial in 20 medical centers in China showed that BBR is mediated by the inhibition of DCA biotransformation by Ruminococcus bromii (Zhang et al., 2020).
The phylum Firmicutes have higher bile salt hydrolase (BSH) activity than the phylum Bacteroidetes in the intestinal microbiota, and the latter is only active against taurine-conjugated bile acids. The study distinctly shows that BBR remarkably increased the Firmicutes/Bacteroidetes ratio and suggests that this is one of the mechanisms of its induced serum free bile acid increase and lipid lowering effect (Gu et al., 2015).. Furthermore, there are other studies showing that bile acids induce transcriptional changes in low-abundance bile acid metabolizing bacteria, including C. scindens (Devendran et al., 2019), C. hylemonae, and C. hiranonis that are capable of turning taurine-conjugated bile acids into unconjugated bile acids and secondary bile acids such as ursodeoxycholic acid and lithocholic acid (Ridlon et al., 2020). BBR significantly alter gut microbial-bile acid metabolite interactions. Wolf, P.G., et al. (Wolf et al., 2021) found that sulfated bile acids were strongly associated with C. hiranonis, C. hylemonae and Bacteroidetes spp, which is positively correlated with the first two and negatively correlated with the last one. Also, BBR treatment increased cecal bile acid concentrations and up-regulated Na+/H+ antiporter, cell wall synthesis/repair, promote carbohydrate and amino acid metabolism. Studies have shown that the level of circulating bile acids in patients with metabolic disorders increases. BBR can increase some beneficial bacteria with benzene sulfonyl hydrazine activity, for instance Bacteroides, Bifidobacterium, Lactobacillus and Clostridium, promote the decomposition of bound bile acids and strengthen their excretion through the intestine. Lactobacillus converts primary bile acids into secondary bile acids through decarboxylation (Vincent et al., 2013). Overall, the mechanisms by which BBR alters the gut microbiome are related to its choleretic effects and are dose related.
4.6 Regulating microbiota-gut-brain axis to improve gastrointestinal hormone expression
The complex interaction between the brain and the gut is called the brain-gut axis, reflects the bidirectional interaction between the brain and the gut. And it is regulated by the gut microbiota, as well as mediated by brain-derived and gut derived hormones and peptides. microbiota-gut-brain axis involving many important factors such as hormones, nutrients and afferent/efferent regulatory autonomous neural pathways. A variety of physiological processes are involved at the same time, including satiety, regulation of metabolism, hormone secretion and sensitivity (especially insulin sensitivity), and bone metabolism (Romijn et al., 2008).. Recently, researchers have found that the disturbance of the gut-brain axis is closely related to the occurrence and development of metabolic diseases and exerts its effects through the hormone signaling pathway (Burokas et al., 2015). Studies have demonstrated that gastrointestinal hormones, such as ghrelin, orexin, glucagon-like peptide-1 (GLP-1), and leptin, can regulate feeding behavior, energy homeostasis, etc. (Cameron and Doucet, 2007; De Silva and Bloom, 2012).Numerous studies have shown that GLP-1 receptor agonists have good clinical efficacy in the treatment of diabetes and obesity (Han et al., 2017). Studies have shown that GLP-1 promotes beta-cell neogenesis and satiety, reduces glucagon secretion, delays gastric emptying, and increases peripheral glucose disposal (Donnelly, 2012). Drugs activating the GLP-1 receptor are also beneficial in the management of another current epidemic, namely nonalcoholic fatty liver disease (NAFLD) (Paternoster et al., 2019). Changes in gut hormones, containing increases in GLP-1 might have a role in induction and long-term maintenance of weight loss (Finelli et al., 2014). Consequently, the microbiota-gut-brain axis is a potential target for metabolic disease treatment and one of the hotspots for future research.
Studies have shown that BBR decreases the variousness of intestinal flora, increases the proportion of Bacteroidetes and Firmicutes, further increases the expression of serum GLP-1, GLP-2 (Wang et al., 2021), PYY, GIP and ghrelin (Sun et al., 2016), provides evidence that BBR treatment can inhibit microbiota diversity, elevate plasma GLP-1 and orexin-a, and upregulate hypothalamic GLP-1 receptor expression, which has beneficial effects on various metabolic disorders such as insulin resistance, obesity and obesity, thereby Induces regulation of the gut-brain axis of the microbiota (Sun et al., 2016). Previous studies (Lu et al., 2009; Yu et al., 2010) showed that that BBR increased the number of L-cells and the mRNA expression levels of proglucagon in the ileum, while promoting GLP-1 secretion in normal and diabetic rats. Other studies have also shown (Xu et al., 2017) BBR significantly increased the levels of plasma GLP-1 and GLP-2 in portal vein, as well as the number of L-cells in proximal colon and the mRNA expression level of preluganin. Some studies have linked the intestinal microbiota to the intestinal endocrine system (Cani et al., 2013). Butyrate may improve metabolism via gut-brain axis signaling (De Vadder et al., 2014). The results showed that after BBR intervention, the number of L-cells was positively correlated with the abundance of acteria, anaerobes, cholephila and oscillibacter. Studies indicated that Akkermansia muciniphila could significantly increase GLP-1 release from colonic L cells (Everard et al., 2011; Hansen et al., 2011), this study confirmed that BBR increased the abundance of akman bacteria and decreased the abundance of Lactobacillus, which seems to be related to the increase of the number of L cells and the intestinal endocrine peptides secreted by L cells. In summary, regulation of glucose and gut hormone levels by BBR has a lot to do with modulating the composition of the gut microbiome. There are few studies on BBR in the treatment of metabolic diseases by regulating microbiota-gut-brain axis, for its potential effectiveness, this mechanism is worthy of further study.
5. Discussion
Previous studies have shown that metabolic disorders significantly affect the structure and function of gut microbiome. In addition, gut microbiome can affect body metabolism by producing metabolites such as short chain fatty acids and succinic acid, which increases the abundance of beneficial bacteria and decreases the abundance of pathogenic bacteria. Therefore, this study focused on the abundance of beneficial and pathogenic bacteria after BBR intervention, and summarized the mechanism of BBR on metabolic diseases. In recent years, with the continuous unraveling of the interactions between gut microbiome and organismal metabolism, relevant intervention mechanisms including the regulation of short chain fatty acids, bile acid metabolic pathways, LPS/TLR4 signaling have been gradually studied (Harsch and Konturek, 2018). However, based on fecal microbiota transplant (FMT), metagene Association, and other techniques, it remains to be explored from dissecting the overall structural changes in gut microbiome to the specific effects of a single genus on organism metabolism. In addition, because the complexity of the interaction mechanism between gut microbiome and body metabolism leads to a causality still not fully clear and needs to be elucidated in more rational experimental designs and research approaches (Fischbach, 2018), it is hoped that gut microbiome will become more widely used in clinical practice as a therapeutic target for metabolic diseases.
Numerous studies have shown that BBR modulates the gut microbiome, and its effects on modulating metabolic function are influenced by the gut microbiota. For example, BBR improves lipid levels in HFD-fed rats, whereas it does not exert a fat regulating effect in normal rats. Further study revealed that the abundance of Nitroreductases producing bacteria increased under the metabolic disorder state, which can produce more Nitroreductases, catalyze the conversion of BBR to Dihydroberberine and promote the intestinal absorption of BBR. So that the metabolic effect of BBR improvement is dependent on the structure and function of gut microbiota. This also reveals, to some extent, the biological basis for the interindividual differences in the efficacy of BBR in regulating body metabolism in the clinic (Wang et al., 2017a).
After extensive literature reading and summarizing, we believe that in the exploration of BBR based on the mechanism related to gut microbiome regulating organism metabolism, it still needs to be combined with individual differences in gut microbiome and well-established experimental protocols to seek the best intervention mode and intervention dosage for clinical application. Then, different conclusions remain to be reported about the structural changes of intestinal flora caused by Berberine: Some believe that the abundance of Akkermansia increased after BBR intervention, But while others believe that BBR could not increase the abundance of Akkermansia alone. In addition, some of the mechanisms by which berberine regulates the structural changes of the flora remain to be explored, for instance, how BBR stimulates A.muciniphila remains further research. Therefore, not only the results of the pre-existing studies need to be validated, but the reasons leading to the discrepancy of the results should be further analyzed.
This review summarizes the mechanism of berberine improving metabolic disorder based on gut microbiome through a combing of literatures. It was found that BBR had a clear effect on altering the abundance of specific bacteria in the gut and modulating the structural function of the gut microbiome as a whole. Through strengthening intestinal barrier function, attenuating metabolic endotoxemia, modulating systemic inflammatory responses, bile acid signaling, and the Microbiota-Gut-Brain axis, among other pathways, in order to exert its effect of improving metabolic disorders. As more studies clarified the mechanisms and characteristics of BBR in ameliorating metabolic disorders by regulating gut microbiome, it provided a new target and research direction for the diagnosis and treatment practice of metabolic diseases in the future clinic. It is worth mentioning that BBR, as a widely used compound in clinic, both in animal experiments and clinical studies, more and more studies have focused on the effects of different doses of BBR on the gut microbiome, and on the basis of confirming its efficacy, dose studies will provide guidance for further exploring the mechanism of action of BBR.
Author contributions
HW and QZ designed and drafted the manuscript. HZ and ZG conceived the manuscript. CG edited and added valuable insights into the manuscript. All authors approved the final manuscript and agreed to be accountable for all aspects of the work.
Funding
This work was supported by the National Natural Science Foundation of China (Grant Nos. 81973837, 81704067 and 81430097), China. And the Strategic Priority Research Program of Chinese Academy of Sciences (Grant No. XDB29020000), China.
Conflict of interest
The authors declare that the research was conducted in the absence of any commercial or financial relationships that could be construed as a potential conflict of interest.
Publisher’s note
All claims expressed in this article are solely those of the authors and do not necessarily represent those of their affiliated organizations, or those of the publisher, the editors and the reviewers. Any product that may be evaluated in this article, or claim that may be made by its manufacturer, is not guaranteed or endorsed by the publisher.
References
Archer, B. J., Johnson, S. K., Devereux, H. M., Baxter, A. L. (2004). Effect of fat replacement by inulin or lupin-kernel fibre on sausage patty acceptability, post-meal perceptions of satiety and food intake in men. Br. J. Nutr. 91 (4), 591–599. doi: 10.1079/BJN20031088
Aswathanarayan, J. B., Vittal, R. R. (2018). Inhibition of biofilm formation and quorum sensing mediated phenotypes by berberine in Pseudomonas aeruginosa and Salmonella typhimurium. RSC Adv. 8 (63), 36133–36141. doi: 10.1039/c8ra06413j
(2001). Executive Summary of The Third Report of The National Cholesterol Education Program (NCEP) Expert Panel on Detection, Evaluation, And Treatment of High Blood Cholesterol In Adults (Adult Treatment Panel III). JAMA 285 (19), 2486–2497. doi: 10.1001/jama.285.19.2486
Backhed, F., Ding, H., Wang, T., Hooper, L. V., Koh, G. Y., Nagy, A., et al. (2004). The gut microbiota as an environmental factor that regulates fat storage. Proc. Natl. Acad. Sci. U S A. 101 (44), 15718–15723. doi: 10.1073/pnas.0407076101
Bai, J., Zhu, Y., Dong, Y. (2016). Response of gut microbiota and inflammatory status to bitter melon (Momordica charantia L.) in high fat diet induced obese rats. J. Ethnopharmacol. 194, 717–726. doi: 10.1016/j.jep.2016.10.043
Balkau, B., Charles, M. A. (1999). Comment on the provisional report from the WHO consultation. European Group for the Study of Insulin Resistance (EGIR). Diabetes Med. 16 (5), 442–443. doi: 10.1046/j.1464-5491.1999.00059.x
Baothman, O. A., Zamzami, M. A., Taher, I., Abubaker, J., Abu-Farha, M. (2016). The role of Gut Microbiota in the development of obesity and Diabetes. Lipids Health Dis. 15, 108. doi: 10.1186/s12944-016-0278-4
Bassis, C. M. (2019). Live and Diet by Your Gut Microbiota. mBio. 10 (5). doi: 10.1128/mBio.02335-19
Bertuccioli, A., Moricoli, S., Amatori, S., Rocchi, M., Vici, G., Sisti, D. (2020). Berberine and Dyslipidemia: Different Applications and Biopharmaceutical Formulations Without Statin-Like Molecules-A Meta-Analysis. J. Med. Food. 23 (2), 101–113. doi: 10.1089/jmf.2019.0088
Burokas, A., Moloney, R. D., Dinan, T. G., Cryan, J. F. (2015). Microbiota regulation of the Mammalian gut-brain axis. Adv. Appl. Microbiol. 91, 1–62. doi: 10.1016/bs.aambs.2015.02.001
Caliceti, C., Franco, P., Spinozzi, S., Roda, A., Cicero, A. F. (2016). Berberine: New Insights from Pharmacological Aspects to Clinical Evidences in the Management of Metabolic Disorders. Curr. Med. Chem. 23 (14), 1460–1476. doi: 10.2174/0929867323666160411143314
Cameron, J., Doucet, E. (2007). Getting to the bottom of feeding behaviour: who's on top? Appl. Physiol. Nutr. Metab. 32 (2). doi: 10.1139/h06-072
Canfora, E. E., Meex, R., Venema, K., Blaak, E. E. (2019). Gut microbial metabolites in obesity, NAFLD and T2DM. Nat. Rev. Endocrinol. 15 (5), 261–273. doi: 10.1038/s41574-019-0156-z
Cani, P. D. (2019). Microbiota and metabolites in metabolic diseases. Nat. Rev. Endocrinol. 15 (2), 69–70. doi: 10.1038/s41574-018-0143-9
Cani, P. D., Amar, J., Iglesias, M. A., Poggi, M., Knauf, C., Bastelica, D., et al. (2007a). Metabolic endotoxemia initiates obesity and insulin resistance. Diabetes 56 (7), 1761–1772. doi: 10.2337/db06-1491
Cani, P. D., Bibiloni, R., Knauf, C., Waget, A., Neyrinck, A. M., Delzenne, N. M., et al. (2008). Changes in gut microbiota control metabolic endotoxemia-induced inflammation in high-fat diet-induced obesity and diabetes in mice. Diabetes 57 (6), 1470–1481. doi: 10.2337/db07-1403
Cani, P. D., Delzenne, N. M. (2009). Interplay between obesity and associated metabolic disorders: new insights into the gut microbiota. Curr. Opin. Pharmacol. 9 (6), 737–743. doi: 10.1016/j.coph.2009.06.016
Cani, P. D., Everard, A., Duparc, T. (2013). Gut microbiota, enteroendocrine functions and metabolism. Curr. Opin. Pharmacol. 13 (6), 935–940. doi: 10.1016/j.coph.2013.09.008
Cani, P. D., Neyrinck, A. M., Fava, F., Knauf, C., Burcelin, R. G., Tuohy, K. M., et al. (2007b). Selective increases of bifidobacteria in gut microflora improve high-fat-diet-induced diabetes in mice through a mechanism associated with endotoxaemia. Diabetologia 50 (11), 2374–2383. doi: 10.1007/s00125-007-0791-0
Cao, H., Li, C., Lei, L., Wang, X., Liu, S., Liu, Q., et al. (2020). Stachyose Improves the Effects of Berberine on Glucose Metabolism by Regulating Intestinal Microbiota and Short-Chain Fatty Acids in Spontaneous Type 2 Diabetic KKAy Mice. Front. Pharmacol. 11, 578943. doi: 10.3389/fphar.2020.578943
Cao, S., Yu, S., Cheng, L., Yan, J., Zhu, Y., Deng, Y., et al. (2018). 9-O-benzoyl-substituted berberine exerts a triglyceride-lowering effect through AMPK signaling pathway in human hepatoma HepG2 cells. Environ. Toxicol. Pharmacol. 64, 11–17. doi: 10.1016/j.etap.2018.09.007
Cicero, A. F., Baggioni, A. (2016). Berberine and Its Role in Chronic Disease. Adv. Exp. Med. Biol. 928, 27–45. doi: 10.1007/978-3-319-41334-1_2
Cui, H. X., Hu, Y. N., Li, J. W., Yuan, K., Guo, Y. (2018). Preparation and Evaluation of Antidiabetic Agents of Berberine Organic Acid Salts for Enhancing the Bioavailability. Molecules 24 (1). doi: 10.3390/molecules24010103
Debose-Boyd, R. A., Ye, J. (2018). SREBPs in Lipid Metabolism, Insulin Signaling, and Beyond. Trends Biochem. Sci. 43 (5), 358–368. doi: 10.1016/j.tibs.2018.01.005
De Silva, A., Bloom, S. R. (2012). Gut Hormones and Appetite Control: A Focus on PYY and GLP-1 as Therapeutic Targets in Obesity. Gut Liver. 6 (1), 10–20. doi: 10.5009/gnl.2012.6.1.10
De Vadder, F., Kovatcheva-Datchary, P., Goncalves, D., Vinera, J., Zitoun, C., Duchampt, A., et al. (2014). Microbiota-generated metabolites promote metabolic benefits via gut-brain neural circuits. Cell. 156 (1-2), 84–96. doi: 10.1016/j.cell.2013.12.016
Devendran, S., Shrestha, R., Alves, J., Wolf, P. G., Ly, L., Hernandez, A. G., et al. (2019). Clostridium scindens ATCC 35704: Integration of Nutritional Requirements, the Complete Genome Sequence, and Global Transcriptional Responses to Bile Acids. Appl. Environ. Microbiol. 85 (7). doi: 10.1128/AEM.00052-19
Direct, T. I. T. C. (2002). Direct thrombin inhibitors in acute coronary syndromes: principal results of a meta-analysis based on individual patients' data. Lancet (London, England).
Dong, C., Yu, J., Yang, Y., Zhang, F., Su, W., Fan, Q., et al. (2021). Berberine, a potential prebiotic to indirectly promote Akkermansia growth through stimulating gut mucin secretion. Biomed. Pharmacother. 139, 111595. doi: 10.1016/j.biopha.2021.111595
Donnelly, D. (2012). The structure and function of the glucagon-like peptide-1 receptor and its ligands. Br. J. Pharmacol. 166 (1), 27–41. doi: 10.1111/j.1476-5381.2011.01687.x
Ehteshamfar, S. M., Akhbari, M., Afshari, J. T., Seyedi, M., Nikfar, B., Shapouri-Moghaddam, A., et al. (2020). Anti-inflammatory and immune-modulatory impacts of berberine on activation of autoreactive T cells in autoimmune inflammation. J. Cell. Mol. Med. 24 (23), 13573–13588. doi: 10.1111/jcmm.16049
Everard, A., Belzer, C., Geurts, L., Ouwerkerk, J. P., Druart, C., Bindels, L. B., et al. (2013). Cross-talk between Akkermansia muciniphila and intestinal epithelium controls diet-induced obesity. Proc. Natl. Acad. Sci. U. S. A. 110 (22), 9066–9071. doi: 10.1073/pnas.1219451110
Everard, A., Lazarevic, V., Derrien, M., Girard, M., Muccioli, G. G., Neyrinck, A. M., et al. (2011). Responses of gut microbiota and glucose and lipid metabolism to prebiotics in genetic obese and diet-induced leptin-resistant mice. Diabetes 60 (11), 2775–2786. doi: 10.2337/db11-0227
Fei, N., Zhao, L. (2013). An opportunistic pathogen isolated from the gut of an obese human causes obesity in germfree mice. ISME J. 7 (4), 880–884. doi: 10.1038/ismej.2012.153
Feng, W., Liu, J., Ao, H., Yue, S., Peng, C. (2020). Targeting gut microbiota for precision medicine: Focusing on the efficacy and toxicity of drugs. Theranostics 10 (24), 11278–11301. doi: 10.7150/thno.47289
Feng, R., Shou, J. W., Zhao, Z. X., He, C. Y., Ma, C., Huang, M., et al. (2015). Transforming berberine into its intestine-absorbable form by the gut microbiota. Sci. Rep. 5, 12155. doi: 10.1038/srep12155
Feng, R., Zhao, Z. X., Ma, S. R., Guo, F., Wang, Y., Jiang, J. D. (2018). Gut Microbiota-Regulated Pharmacokinetics of Berberine and Active Metabolites in Beagle Dogs After Oral Administration. Front. Pharmacol. 9. doi: 10.3389/fphar.2018.00214
Festi, D., Schiumerini, R., Eusebi, L. H., Marasco, G., Taddia, M., Colecchia, A. (2014). Gut microbiota and metabolic syndrome. World J. Gastroenterol. 20 (43), 16079–16094. doi: 10.3748/wjg.v20.i43.16079
Finelli, C., Padula, M. C., Martelli, G., Tarantino, G. (2014). Could the improvement of obesity-related co-morbidities depend on modified gut hormones secretion? World J. Gastroenterol. 20 (44), 16649–16664. doi: 10.3748/wjg.v20.i44.16649
Fiorucci, S., Cipriani, S., Mencarelli, A., Renga, B., Distrutti, E., Baldelli, F. (2010). Counter-regulatory role of bile acid activated receptors in immunity and inflammation. Curr. Mol. Med. 10 (6), 579–595. doi: 10.2174/1566524011009060579
Fiorucci, S., Distrutti, E. (2015). Bile Acid-Activated Receptors, Intestinal Microbiota, and the Treatment of Metabolic Disorders. Trends Mol. Med. 21 (11), 702–714. doi: 10.1016/j.molmed.2015.09.001
Fischbach, M. A. (2018). Microbiome: Focus on Causation and Mechanism. Cell 174 (4), 785–790. doi: 10.1016/j.cell.2018.07.038
Fu, H., Leng, W., Wang, J., Zhang, W., Peng, J., Wang, L., et al. (2007). Transcriptional profile induced by furazolidone treatment of Shigella flexneri. Appl. Microbiol. Biotechnol. 77 (3), 657–667. doi: 10.1007/s00253-007-1180-9
Fu, Y., Wang, Y., Wang, X., Sun, Y., Ren, J., Fang, B. (2022). Responses of human gut microbiota abundance and amino acid metabolism in vitro to berberine. Food Funct. 13 (11), 6329–6337. doi: 10.1039/d1fo04003k
Gao, Z., Li, Q., Wu, X., Zhao, X., Zhao, L., Tong, X. (2017). New Insights into the Mechanisms of Chinese Herbal Products on Diabetes: A Focus on the "Bacteria-Mucosal Immunity-Inflammation-Diabetes" Axis. J. Immunol. Res. 2017, 1813086. doi: 10.1155/2017/1813086
Gomes, A. C., Hoffmann, C., Mota, J. F. (2018). The human gut microbiota: Metabolism and perspective in obesity. Gut Microbes 9 (4), 308–325. doi: 10.1080/19490976.2018.1465157
Gong, J., Hu, M., Huang, Z., Fang, K., Wang, D., Chen, Q., et al. (2017a). Berberine Attenuates Intestinal Mucosal Barrier Dysfunction in Type 2 Diabetic Rats. Front. Pharmacol. 8. doi: 10.3389/fphar.2017.00042
Gong, J., Hu, M., Huang, Z., Fang, K., Wang, D., Chen, Q., et al. (2017b). Berberine Attenuates Intestinal Mucosal Barrier Dysfunction in Type 2 Diabetic Rats. Front. Pharmacol. 8. doi: 10.3389/fphar.2017.00042
Greenhill, C. (2018). Exercise affects gut microbiota and bone. Nat. Rev. Endocrinol. 14 (6), 322. doi: 10.1038/s41574-018-0014-4
Gu, S., Cao, B., Sun, R., Tang, Y., Paletta, J. L., Wu, X., et al. (2015). A metabolomic and pharmacokinetic study on the mechanism underlying the lipid-lowering effect of orally administered berberine. Mol. Biosyst. 11 (2), 463–474. doi: 10.1039/c4mb00500g
Guerre, P. (2020). Mycotoxin and Gut Microbiota Interactions. Toxins (Basel). 12 (12). doi: 10.3390/toxins12120769
Guo, Y., Zhang, Y., Huang, W., Selwyn, F. P., Klaassen, C. D. (2016). Dose-response effect of berberine on bile acid profile and gut microbiota in mice. BMC Complement Altern. Med. 16 (1), 394. doi: 10.1186/s12906-016-1367-7
Habtemariam, S. (2020). Berberine pharmacology and the gut microbiota: A hidden therapeutic link. Pharmacol. Res. 155, 104722. doi: 10.1016/j.phrs.2020.104722
Han, K., Bose, S., Wang, J. H., Lim, S. K., Chin, Y. W., Kim, Y. M., et al. (2017). In vivo therapeutic effect of combination treatment with metformin and Scutellaria baicalensis on maintaining bile acid homeostasis. PloS One 12 (9), e0182467. doi: 10.1371/journal.pone.0182467
Hansen, K. B., Rosenkilde, M. M., Knop, F. K., Wellner, N., Diep, T. A., Rehfeld, J. F., et al. (2011). 2-Oleoyl glycerol is a GPR119 agonist and signals GLP-1 release in humans. J. Clin. Endocrinol. Metab. 96 (9), E1409–E1417. doi: 10.1210/jc.2011-0647
Harsch, I. A., Konturek, P. C. (2018). The Role of Gut Microbiota in Obesity and Type 2 and Type 1 Diabetes Mellitus: New Insights into "Old" Diseases. Med. Sci. (Basel) 6 (2). doi: 10.3390/medsci6020032
Hashemzaei, M., Rezaee, R. (2021). A review on pain-relieving activity of berberine. Phytother. Res. 35 (6), 2846–2853. doi: 10.1002/ptr.6984
He, K., Hu, Y., Ma, H., Zou, Z., Xiao, Y., Yang, Y., et al. (2016a). Rhizoma Coptidis alkaloids alleviate hyperlipidemia in B6 mice by modulating gut microbiota and bile acid pathways. Biochim. Biophys. Acta 1862 (9), 1696–1709. doi: 10.1016/j.bbadis.2016.06.006
He, K., Hu, Y., Ma, H., Zou, Z., Xiao, Y., Yang, Y., et al. (2016b). Rhizoma Coptidis alkaloids alleviate hyperlipidemia in B6 mice by modulating gut microbiota and bile acid pathways. Biochim. Biophys. Acta 1862 (9), 1696–1709. doi: 10.1016/j.bbadis.2016.06.006
Huang, P. L. (2009). A comprehensive definition for metabolic syndrome. Dis. Model. Mech. 2 (5-6), 231–237. doi: 10.1242/dmm.001180
Hvistendahl, M. (2012). My microbiome and me. Science 336 (6086), 1248–1250. doi: 10.1126/science.336.6086.1248
Ianiro, G., Tilg, H., Gasbarrini, A. (2016). Antibiotics as deep modulators of gut microbiota: between good and evil. Gut. 65 (11), 1906–1915. doi: 10.1136/gutjnl-2016-312297
Ilyas, Z., Perna, S., Al-Thawadi, S., Alalwan, T. A., Riva, A., Petrangolini, G., et al. (2020). The effect of Berberine on weight loss in order to prevent obesity: A systematic review. Biomed. Pharmacother. 127, 110137. doi: 10.1016/j.biopha.2020.110137
Imenshahidi, M., Hosseinzadeh, H. (2016). Berberis Vulgaris and Berberine: An Update Review. Phytother. Res. 30 (11), 1745–1764. doi: 10.1002/ptr.5693
Jin, Y., Wu, S., Zeng, Z., Fu, Z. (2017). Effects of environmental pollutants on gut microbiota. Environ. Pollut. 222, 1–9. doi: 10.1016/j.envpol.2016.11.045
Kallio, K. A., Buhlin, K., Jauhiainen, M., Keva, R., Tuomainen, A. M., Klinge, B., et al. (2008). Lipopolysaccharide associates with pro-atherogenic lipoproteins in periodontitis patients. Innate Immun. 14 (4), 247–253. doi: 10.1177/1753425908095130
Kassi, E., Pervanidou, P., Kaltsas, G., Chrousos, G. (2011). Metabolic syndrome: definitions and controversies. BMC Med. 9, 48. doi: 10.1186/1741-7015-9-48
Kinouchi, T., Manabe, Y., Wakisaka, K., Ohnishi, Y. (1982). Biotransformation of 1-nitropyrene in intestinal anaerobic bacteria. Microbiol. Immunol. 26 (11), 993–1005. doi: 10.1111/j.1348-0421.1982.tb00249.x
Koh, A., De Vadder, F., Kovatcheva-Datchary, P., Backhed, F. (2016). From Dietary Fiber to Host Physiology: Short-Chain Fatty Acids as Key Bacterial Metabolites. Cell. 165 (6), 1332–1345. doi: 10.1016/j.cell.2016.05.041
Kong, W., Wei, J., Abidi, P., Lin, M., Inaba, S., Li, C., et al. (2004). Berberine is a novel cholesterol-lowering drug working through a unique mechanism distinct from statins. Nat. Med. 10 (12), 1344–1351. doi: 10.1038/nm1135
Kulkarni, S. K., Dhir, A. (2010). Berberine: a plant alkaloid with therapeutic potential for central nervous system disorders. Phytother. Res. 24 (3), 317–324. doi: 10.1002/ptr.2968
Kuo, C. L., Chi, C. W., Liu, T. Y. (2004). The anti-inflammatory potential of berberine in vitro and in vivo. Cancer Lett. 203 (2), 127–137. doi: 10.1016/j.canlet.2003.09.002
Leeming, E. R., Johnson, A. J., Spector, T. D., Le Roy, C. I. (2019). Effect of Diet on the Gut Microbiota: Rethinking Intervention Duration. Nutrients. 11 (12). doi: 10.3390/nu11122862
Li, T., Chiang, J. Y. (2014). Bile acid signaling in metabolic disease and drug therapy. Pharmacol. Rev. 66 (4), 948–983. doi: 10.1124/pr.113.008201
Li, J., Lin, S., Vanhoutte, P. M., Woo, C. W., Xu, A. (2016). Akkermansia Muciniphila Protects Against Atherosclerosis by Preventing Metabolic Endotoxemia-Induced Inflammation in Apoe-/- Mice. Circulation. 133 (24), 2434–2446. doi: 10.1161/CIRCULATIONAHA.115.019645
Liu, Y. T., Hao, H. P., Xie, H. G., Lai, L., Wang, Q., Liu, C. X., et al. (2010). Extensive intestinal first-pass elimination and predominant hepatic distribution of berberine explain its low plasma levels in rats. Drug Metab. Dispos. 38 (10), 1779–1784. doi: 10.1124/dmd.110.033936
Liu, D., Meng, X., Wu, D., Qiu, Z., Luo, H. (2019). A Natural Isoquinoline Alkaloid With Antitumor Activity: Studies of the Biological Activities of Berberine. Front. Pharmacol. 10. doi: 10.3389/fphar.2019.00009
Liu, D., Zhang, Y., Liu, Y., Hou, L., Li, S., Tian, H. (2018). Berberine Modulates Gut Microbiota and Reduces Insulin Resistance via the TLR4 Signaling Pathway. Exp. Clin. Endocrinol. Diabetes 126 (8), 513–520. doi: 10.1055/s-0043-125066
Liu, C. S., Zheng, Y. R., Zhang, Y. F., Long, X. Y. (2016). Research progress on berberine with a special focus on its oral bioavailability. Fitoterapia. 109, 274–282. doi: 10.1016/j.fitote.2016.02.001
Li, C. N., Wang, X., Lei, L., Liu, M. Z., Li, R. C., Sun, S. J. (2020). Berberine combined with stachyose induces better glycometabolism than berberine alone through modulating gut microbiota and fecal metabolomics in diabetic mice. Phytother. Res. 34 (5), 1166–1174. doi: 10.1002/ptr.6588
Li, M., Zhou, W., Dang, Y., Li, C., Ji, G., Zhang, L. (2020). Berberine compounds improves hyperglycemia via microbiome mediated colonic TGR5-GLP pathway in db/db mice. Biomed. Pharmacother. 132, 110953. doi: 10.1016/j.biopha.2020.110953
Luo, T., Jiang, W., Kong, Y., Li, S., He, F., Xu, J., et al. (2012). The protective effects of jatrorrhizine on beta-amyloid (25-35)-induced neurotoxicity in rat cortical neurons. CNS Neurol. Disord. Drug Targets 11 (8), 1030–1037. doi: 10.2174/1871527311211080013
Lu, S. S., Yu, Y. L., Zhu, H. J., Liu, X. D., Liu, L., Liu, Y. W., et al. (2009). Berberine promotes glucagon-like peptide-1 (7-36) amide secretion in streptozotocin-induced diabetic rats. J. Endocrinol. 200 (2), 159–165. doi: 10.1677/JOE-08-0419
Ma, X., Chen, Z., Wang, L., Wang, G., Wang, Z., Dong, X., et al. (2018). The Pathogenesis of Diabetes Mellitus by Oxidative Stress and Inflammation: Its Inhibition by Berberine. Front. Pharmacol. 9. doi: 10.3389/fphar.2018.00782
Ma, Y. G., Liang, L., Zhang, Y. B., Wang, B. F., Bai, Y. G., Dai, Z. J., et al. (2017). Berberine reduced blood pressure and improved vasodilation in diabetic rats. J. Mol. Endocrinol. 59 (3), 191–204. doi: 10.1530/JME-17-0014
Mandaliya, D. K., Seshadri, S. (2019). ). Short Chain Fatty Acids, pancreatic dysfunction and type 2 diabetes. Pancreatology 19 (2), 280–284. doi: 10.1016/j.pan.2019.01.021
Martini, D., Pucci, C., Gabellini, C., Pellegrino, M., Andreazzoli, M. (2020). Exposure to the natural alkaloid Berberine affects cardiovascular system morphogenesis and functionality during zebrafish development. Sci. Rep. 10 (1), 17358. doi: 10.1038/s41598-020-73661-5
Mcdonald, D., Price, M. N., Goodrich, J., Nawrocki, E. P., Desantis, T. Z., Probst, A., et al. (2012). An improved Greengenes taxonomy with explicit ranks for ecological and evolutionary analyses of bacteria and archaea. ISME J.
Mcnabney, S. M., Henagan, T. M. (2017). Short Chain Fatty Acids in the Colon and Peripheral Tissues: A Focus on Butyrate, Colon Cancer, Obesity and Insulin Resistance. Nutrients. 9 (12). doi: 10.3390/nu9121348
Meijnikman, A. S., Gerdes, V. E., Nieuwdorp, M., Herrema, H. (2018). Evaluating Causality of Gut Microbiota in Obesity and Diabetes in Humans. Endocr. Rev. 39 (2), 133–153. doi: 10.1210/er.2017-00192
Memon, M. A., Khan, R. N., Riaz, S., Ain, Q. U., Ahmed, M., Kumar, N. (2018). Methylglyoxal and insulin resistance in berberine-treated type 2 diabetic patients. J. Res. Med. Sci. 23, 110. doi: 10.4103/jrms.JRMS_1078_17
Ming, J., Yu, X., Xu, X., Wang, L., Ding, C., Wang, Z., et al. (2021). Effectiveness and safety of Bifidobacterium and berberine in human hyperglycemia and their regulatory effect on the gut microbiota: a multi-center, double-blind, randomized, parallel-controlled study. Genome Med. 13 (1), 125. doi: 10.1186/s13073-021-00942-7
Neyrinck, A. M., Sanchez, C. R., Rodriguez, J., Cani, P. D., Bindels, L. B., Delzenne, N. M. (2021). Prebiotic Effect of Berberine and Curcumin Is Associated with the Improvement of Obesity in Mice. Nutrients. 13 (5). doi: 10.3390/nu13051436
Paone, P., Cani, P. D. (2020). Mucus barrier, mucins and gut microbiota: the expected slimy partners? Gut. 69 (12), 2232–2243. doi: 10.1136/gutjnl-2020-322260
Park, J., Kim, M., Kang, S. G., Jannasch, A. H., Cooper, B., Patterson, J., et al. (2015). Short-chain fatty acids induce both effector and regulatory T cells by suppression of histone deacetylases and regulation of the mTOR-S6K pathway. Mucosal Immunol. 8 (1), 80–93. doi: 10.1038/mi.2014.44
Paternoster, S., Keating, D., Falasca, M. (2019). ditorial: Gastrointestinal Hormones. Front. Endocrinol. (Lausanne) 10. doi: 10.3389/fendo.2019.00498
Perez, M. M., Martins, L., Dias, M. S., Pereira, C. A., Leite, J. A., Goncalves, E., et al. (2019). Interleukin-17/interleukin-17 receptor axis elicits intestinal neutrophil migration, restrains gut dysbiosis and lipopolysaccharide translocation in high-fat diet-induced metabolic syndrome model. Immunology. 156 (4), 339–355. doi: 10.1111/imm.13028
Plovier, H., Everard, A., Druart, C., Depommier, C., Van Hul, M., Geurts, L., et al. (2017). A purified membrane protein from Akkermansia muciniphila or the pasteurized bacterium improves metabolism in obese and diabetic mice. Nat. Med. 23 (1), 107–113. doi: 10.1038/nm.4236
Possemiers, S., Bolca, S., Verstraete, W., Heyerick, A. (2011). The intestinal microbiome: a separate organ inside the body with the metabolic potential to influence the bioactivity of botanicals. Fitoterapia. 82 (1), 53–66. doi: 10.1016/j.fitote.2010.07.012
Pussinen, P. J., Havulinna, A. S., Lehto, M., Sundvall, J., Salomaa, V. (2011). Endotoxemia is associated with an increased risk of incident diabetes. Diabetes Care 34 (2), 392–397. doi: 10.2337/dc10-1676
Qin, J., Li, Y., Cai, Z., Li, S., Zhu, J., Zhang, F., et al. (2012). A metagenome-wide association study of gut microbiota in type 2 diabetes. Nature. 490, 55–60. doi: 10.1038/nature11450
Qin, J., Li, R., Raes, J., Arumugam, M., Burgdorf, K. S., Manichanh, C., et al. (2010). A human gut microbial gene catalogue established by metagenomic sequencing. Nature. 464, 59–65. doi: 10.1038/nature08821
Ramirez, J., Guarner, F., Bustos, F. L., Maruy, A., Sdepanian, V. L., Cohen, H. (2020). Antibiotics as Major Disruptors of Gut Microbiota. Front. Cell Infect. Microbiol. 10. doi: 10.3389/fcimb.2020.572912
Remely, M., Tesar, I., Hippe, B., Gnauer, S., Rust, P., Haslberger, A. G. (2015). Gut microbiota composition correlates with changes in body fat content due to weight loss. Benef. Microbes 6 (4), 431–439. doi: 10.3920/BM2014.0104
Ridaura, V. K., Faith, J. J., Rey, F. E., Cheng, J., Duncan, A. E., Kau, A. L., et al. (2013). Gut microbiota from twins discordant for obesity modulate metabolism in mice. Science 341 (6150), 1241214. doi: 10.1126/science.1241214
Ridlon, J. M., Devendran, S., Alves, J. M., Doden, H., Wolf, P. G., Pereira, G. V., et al. (2020). The 'in vivo lifestyle' of bile acid 7alpha-dehydroxylating bacteria: comparative genomics, metatranscriptomic, and bile acid metabolomics analysis of a defined microbial community in gnotobiotic mice. Gut Microbes 11 (3), 381–404. doi: 10.1080/19490976.2019.1618173
Romijn, J. A., Corssmit, E. P., Havekes, L. M., Pijl, H. (2008). Gut-brain axis. Curr. Opin. Clin. Nutr. Metab. Care 11 (4), 518–521. doi: 10.1097/MCO.0b013e328302c9b0
Schapiro, J. M., Gupta, R., Stefansson, E., Fang, F. C., Limaye, A. P. (2004). Isolation of metronidazole-resistant Bacteroides fragilis carrying the nimA nitroreductase gene from a patient in Washington State. J. Clin. Microbiol. 42 (9), 4127–4129. doi: 10.1128/JCM.42.9.4127-4129.2004
Scheithauer, T., Rampanelli, E., Nieuwdorp, M., Vallance, B. A., Verchere, C. B., van Raalte, D. H., et al. (2020). Gut Microbiota as a Trigger for Metabolic Inflammation in Obesity and Type 2 Diabetes. Front. Immunol. 11, 571731. doi: 10.3389/fimmu.2020.571731
Schoeler, M., Caesar, R. (2019). Dietary lipids, gut microbiota and lipid metabolism. Rev. Endocr. Metab. Disord. 20 (4), 461–472. doi: 10.1007/s11154-019-09512-0
Shinkov, A., Borissova, A. M., Kovatcheva, R., Vlahov, J., Dakovska, L., Atanassova, I., et al. (2018). Increased prevalence of depression and anxiety among subjects with metabolic syndrome and known type 2 diabetes mellitus - a population-based study. Postgrad. Med. 130 (2), 251–257. doi: 10.1080/00325481.2018.1410054
Singh, A. B., Liu, J. (2019). Berberine decreases plasma triglyceride levels and upregulates hepatic TRIB1 in LDLR wild type mice and in LDLR deficient mice. Sci. Rep. 9 (1), 15641. doi: 10.1038/s41598-019-52253-y
Sircana, A., Framarin, L., Leone, N., Berrutti, M., Castellino, F., Parente, R., et al. (2018). Altered Gut Microbiota in Type 2 Diabetes: Just a Coincidence? Curr. Diabetes Rep. 18 (10), 98. doi: 10.1007/s11892-018-1057-6
Sousa, T., Paterson, R., Moore, V., Carlsson, A., Abrahamsson, B., Basit, A. W. (2008). The gastrointestinal microbiota as a site for the biotransformation of drugs. Int. J. Pharm. 363 (1-2), 1–25. doi: 10.1016/j.ijpharm.2008.07.009
Stojanov, S., Berlec, A., Strukelj, B. (2020). The Influence of Probiotics on the Firmicutes/Bacteroidetes Ratio in the Treatment of Obesity and Inflammatory Bowel disease. Microorganisms 8 (11). doi: 10.3390/microorganisms8111715
Sun, H., Wang, N., Cang, Z., Zhu, C., Zhao, L., Nie, X., et al. (2016). Modulation of Microbiota-Gut-Brain Axis by Berberine Resulting in Improved Metabolic Status in High-Fat Diet-Fed Rats. Obes. Facts. 9 (6), 365–378. doi: 10.1159/000449507
Sun, R., Yang, N., Kong, B., Cao, B., Feng, D., Yu, X., et al. (2017). Orally Administered Berberine Modulates Hepatic Lipid Metabolism by Altering Microbial Bile Acid Metabolism and the Intestinal FXR Signaling Pathway. Mol. Pharmacol. 91 (2), 110–122. doi: 10.1124/mol.116.106617
Tabeshpour, J., Imenshahidi, M., Hosseinzadeh, H. (2017). A review of the effects of Berberis vulgaris and its major component, berberine, in metabolic syndrome. Iran. J. Basic Med. Sci. 20 (5), 557–568. doi: 10.22038/IJBMS.2017.8682
Tagliabue, A., Elli, M. (2013). The role of gut microbiota in human obesity: recent findings and future perspectives. Nutr. Metab. Cardiovasc. Dis. 23 (3), 160–168. doi: 10.1016/j.numecd.2012.09.002
Tan, X. S., Ma, J. Y., Feng, R., Ma, C., Chen, W. J., Sun, Y. P., et al. (2013). Tissue distribution of berberine and its metabolites after oral administration in rats. PloS One 8 (10), e77969. doi: 10.1371/journal.pone.0077969
Tarantino, G., Costantini, S., Finelli, C., Capone, F., Guerriero, E., La Sala, N., et al. (2014). Is serum Interleukin-17 associated with early atherosclerosis in obese patients? J. Transl. Med. 12, 214. doi: 10.1186/s12967-014-0214-1
Thaiss, C. A., Levy, M., Grosheva, I., Zheng, D., Soffer, E., Blacher, E., et al. (2018). Hyperglycemia drives intestinal barrier dysfunction and risk for enteric infection. Science 359 (6382), 1376–1383. doi: 10.1126/science.aar3318
Tilg, H., Moschen, A. R. (2014). Microbiota and diabetes: an evolving relationship. Gut. 63 (9), 1513–1521. doi: 10.1136/gutjnl-2014-306928
Ussar, S., Griffin, N. W., Bezy, O., Fujisaka, S., Vienberg, S., Softic, S., et al. (2015). Interactions between Gut Microbiota, Host Genetics and Diet Modulate the Predisposition to Obesity and Metabolic Syndrome. Cell Metab. 22 (3), 516–530. doi: 10.1016/j.cmet.2015.07.007
Van Hul, M., Geurts, L., Plovier, H., Druart, C., Everard, A., Stahlman, M., et al. (2018). Reduced obesity, diabetes, and steatosis upon cinnamon and grape pomace are associated with changes in gut microbiota and markers of gut barrier. Am. J. Physiol. Endocrinol. Metab. 314 (4), E334–E352. doi: 10.1152/ajpendo.00107.2017
Vincent, R. P., Omar, S., Ghozlan, S., Taylor, D. R., Cross, G., Sherwood, R. A., et al. (2013). Higher circulating bile acid concentrations in obese patients with type 2 diabetes. Ann. Clin. Biochem. 50 (Pt 4), 360–364. doi: 10.1177/0004563212473450
Wang, K., Feng, X., Chai, L., Cao, S., Qiu, F. (2017). The metabolism of berberine and its contribution to the pharmacological effects. Drug Metab. Rev. 49 (2), 139–157. doi: 10.1080/03602532.2017.1306544
Wang, Y., Liu, H., Zheng, M., Yang, Y., Ren, H., Kong, Y., et al. (2021). Berberine Slows the Progression of Prediabetes to Diabetes in Zucker Diabetic Fatty Rats by Enhancing Intestinal Secretion of Glucagon-Like Peptide-2 and Improving the Gut Microbiota. Front. Endocrinol. (Lausanne) 12. doi: 10.3389/fendo.2021.609134
Wang, Y., Shou, J. W., Li, X. Y., Zhao, Z. X., Fu, J., He, C. Y., et al. (2017a). Berberine-induced bioactive metabolites of the gut microbiota improve energy metabolism. Metabolism 70, 72–84. doi: 10.1016/j.metabol.2017.02.003
Wang, Y., Tong, Q., Shou, J. W., Zhao, Z. X., Li, X. Y., Zhang, X. F., et al. (2017b). Gut Microbiota-Mediated Personalized Treatment of Hyperlipidemia Using Berberine. Theranostics. 7 (9), 2443–2451. doi: 10.7150/thno.18290
Wang, S., Xu, Z., Cai, B., Chen, Q. (2021). Berberine as a Potential Multi-Target Agent for Metabolic Diseases: A Review of Investigations for Berberine. Endocr. Metab. Immune Disord. Drug Targets. 21 (6), 971–979. doi: 10.2174/1871530320666200910105612
Weglarz, L., Wawszczyk, J., Orchel, A., Jaworska-Kik, M., Dzierzewicz, Z. (2007). Phytic acid modulates in vitro IL-8 and IL-6 release from colonic epithelial cells stimulated with LPS and IL-1beta. Dig Dis. Sci. 52 (1), 93–102. doi: 10.1007/s10620-006-9320-0
Wei, X., Tao, J., Xiao, S., Jiang, S., Shang, E., Zhu, Z., et al. (2018). Xiexin Tang improves the symptom of type 2 diabetic rats by modulation of the gut microbiota. Sci. Rep. 8 (1), 3685. doi: 10.1038/s41598-018-22094-2
Wolf, P. G., Devendran, S., Doden, H. L., Ly, L. K., Moore, T., Takei, H., et al. (2021). Berberine alters gut microbial function through modulation of bile acids. BMC Microbiol. 21 (1), 24. doi: 10.1186/s12866-020-02020-1
Wu, J., Wang, K., Wang, X., Pang, Y., Jiang, C. (2021). The role of the gut microbiome and its metabolites in metabolic diseases. Protein Cell. 12 (5), 360–373. doi: 10.1007/s13238-020-00814-7
Wu, M., Yang, S., Wang, S., Cao, Y., Zhao, R., Li, X., et al. (2020). Effect of Berberine on Atherosclerosis and Gut Microbiota Modulation and Their Correlation in High-Fat Diet-Fed ApoE-/- Mice. Front. Pharmacol. 11. doi: 10.3389/fphar.2020.00223
Xiao, Y., Xu, M., Alimujiang, M., Bao, Y., Wei, L., Yin, J. (2018). Bidirectional regulation of adenosine 5'-monophosphate-activated protein kinase activity by berberine and metformin in response to changes in ambient glucose concentration. J. Cell. Biochem. 119 (12), 9910–9920. doi: 10.1002/jcb.27312
Xu, J., Chen, H. B., Li, S. L. (2017). Understanding the Molecular Mechanisms of the Interplay Between Herbal Medicines and Gut Microbiota. Med. Res. Rev. 37 (5), 1140–1185. doi: 10.1002/med.21431
Xu, X., Gao, Z., Yang, F., Yang, Y., Chen, L., Han, ,. L., et al. (2020a). Antidiabetic Effects of Gegen Qinlian Decoction via the Gut Microbiota Are Attributable to Its Key Ingredient Berberine. Genomics Proteomics Bioinf. 18 (6), 721–736. doi: 10.1016/j.gpb.2019.09.007
Xu, X., Gao, Z., Yang, F., Yang, Y., Chen, L., Han, L., et al. (2020b). Antidiabetic Effects of Gegen Qinlian Decoction via the Gut Microbiota Are Attributable to Its Key Ingredient Berberine. Genomics Proteomics Bioinf. 18 (6), 721–736. doi: 10.1016/j.gpb.2019.09.007
Xu, J. H., Liu, X. Z., Pan, W., Zou, D. J. (2017). Berberine protects against diet-induced obesity through regulating metabolic endotoxemia and gut hormone levels. Mol. Med. Rep. 15 (5), 2765–2787. doi: 10.3892/mmr.2017.6321
Xu, X., Yi, H., Wu, J., Kuang, T., Zhang, J., Li, Q., et al. (2021). Therapeutic effect of berberine on metabolic diseases: Both pharmacological data and clinical evidence. Biomed. Pharmacother. 133, 110984. doi: 10.1016/j.biopha.2020.110984
Yang, S., Li, D., Yu, Z., Li, Y., Wu, M. (2021). Multi-Pharmacology of Berberine in Atherosclerosis and Metabolic Diseases: Potential Contribution of Gut Microbiota. Front. Pharmacol. 12. doi: 10.3389/fphar.2021.709629
Yang, G., Wei, J., Liu, P., Zhang, Q., Tian, Y., Hou, G., et al. (2021) (154712). doi: 10.1016/j.metabol.2021.154712
Yan, H. M., Xia, M. F., Wang, Y., Chang, X. X., Yao, X. Z., Rao, S. X., et al. (2015). Efficacy of Berberine in Patients with Non-Alcoholic Fatty Liver Disease. PloS One 10 (8), e0134172. doi: 10.1371/journal.pone.0134172
Yin, J., Li, Y., Han, H., Chen, S., Gao, J., Liu, G., et al. (2018). Melatonin reprogramming of gut microbiota improves lipid dysmetabolism in high-fat diet-fed mice. J. Pineal Res. 65 (4), e12524. doi: 10.1111/jpi.12524
Yin, J., Xing, H., Ye, J. (2008). Efficacy of berberine in patients with type 2 diabetes mellitus. Metabolism. 57 (5), 712–717. doi: 10.1016/j.metabol.2008.01.013
Yin, J., Ye, J., Jia, W. (2012). Effects and mechanisms of berberine in diabetes treatment. Acta Pharm. Sin. B. 2 (4), 327–334. doi: 10.1016/j.apsb.2012.06.003
Yu, M., Alimujiang, M., Yin, J. (2019). 611-P: Berberine Prevented High-Fat Diet-Induced Metabolic Disorders via Inhibition of Whole Lipid Metabolism. Diabetes 68 (Supplement_1), 611–61P. doi: 10.2337/db19-611-P
Yu, Y., Liu, L., Wang, X., Liu, X., Liu, X., Xie, L., et al. (2010). Modulation of glucagon-like peptide-1 release by berberine: in vivo and in vitro studies. Biochem. Pharmacol. 79 (7), 1000–1006. doi: 10.1016/j.bcp.2009.11.017
Zhang, R., Gao, X., Bai, H., Ning, K. (2020). Traditional Chinese Medicine and Gut Microbiome: Their Respective and Concert Effects on Healthcare. Front. Pharmacol. 11. doi: 10.3389/fphar.2020.00538
Zhang, Y., Gu, Y., Ren, H., Wang, S., Zhong, H., Zhao, X., et al. (2020). Gut microbiome-related effects of berberine and probiotics on type 2 diabetes (the PREMOTE study). Nat. Commun. 11 (1), 5015. doi: 10.1038/s41467-020-18414-8
Zhang, B., Pan, Y., Xu, L., Tang, D., Dorfman, R. G., Zhou, Q., et al. (2018). Berberine promotes glucose uptake and inhibits gluconeogenesis by inhibiting deacetylase SIRT3. Endocrine. 62 (3), 576–587. doi: 10.1007/s12020-018-1689-y
Zhang, Q., Piao, X. L., Piao, X. S., Lu, T., Wang, D., Kim, S. W. (2011). Preventive effect of Coptis chinensis and berberine on intestinal injury in rats challenged with lipopolysaccharides. Food Chem. Toxicol. 49 (1), 61–69. doi: 10.1016/j.fct.2010.09.032
Zhang, C., Sheng, J., Li, G., Zhao, L., Wang, Y., Yang, W., et al. (2019). Effects of Berberine and Its Derivatives on Cancer: A Systems Pharmacology Review. Front. Pharmacol. 10. doi: 10.3389/fphar.2019.01461
Zhang, H., Wei, J., Xue, R., Wu, J. D., Zhao, W., Wang, Z. Z., et al. (2010). Berberine lowers blood glucose in type 2 diabetes mellitus patients through increasing insulin receptor expression. Metabolism. 59 (2), 285–292. doi: 10.1016/j.metabol.2009.07.029
Zhang, W., Xu, J. H., Yu, T., Chen, Q. K. (2019). Effects of berberine and metformin on intestinal inflammation and gut microbiome composition in db/db mice. Biomed. Pharmacother. 118, 109131. doi: 10.1016/j.biopha.2019.109131
Zhang, X., Zhao, Y., Xu, J., Xue, Z., Zhang, M., Pang, X., et al. (2015). Modulation of gut microbiota by berberine and metformin during the treatment of high-fat diet-induced obesity in rats. Sci. Rep. 5, 14405. doi: 10.1038/srep14405
Zhang, X., Zhao, Y., Zhang, M., Pang, X., Xu, J., Kang, C., et al. (2012). Structural changes of gut microbiota during berberine-mediated prevention of obesity and insulin resistance in high-fat diet-fed rats. PloS One 7 (8), e42529. doi: 10.1371/journal.pone.0042529
Zhou, J. C., Zhang, X. W. (2019). Akkermansia muciniphila: a promising target for the therapy of metabolic syndrome and related diseases. Chin. J. Nat. Med. 17 (11), 835–841. doi: 10.1016/S1875-5364(19)30101-3
Zou, Z. Y., Hu, Y. R., Ma, H., Wang, Y. Z., He, K., Xia, S., et al. (2015). Coptisine attenuates obesity-related inflammation through LPS/TLR-4-mediated signaling pathway in Syrian golden hamsters. Fitoterapia. 105, 139–146. doi: 10.1016/j.fitote.2015.06.005
Keywords: gut microbiota, metabolic disorder, diabetes, obesity, berberine
Citation: Wang H, Zhang H, Gao Z, Zhang Q and Gu C (2022) The mechanism of berberine alleviating metabolic disorder based on gut microbiome. Front. Cell. Infect. Microbiol. 12:854885. doi: 10.3389/fcimb.2022.854885
Received: 14 January 2022; Accepted: 01 August 2022;
Published: 25 August 2022.
Edited by:
Qin Feng, Shanghai University of Traditional Chinese Medicine, ChinaReviewed by:
Almagul Kushugulova, Nazarbayev University, KazakhstanGiovanni Tarantino, University of Naples Federico II, Italy
Houkai Li, Shanghai University of Traditional Chinese Medicine, China
Copyright © 2022 Wang, Zhang, Gao, Zhang and Gu. This is an open-access article distributed under the terms of the Creative Commons Attribution License (CC BY). The use, distribution or reproduction in other forums is permitted, provided the original author(s) and the copyright owner(s) are credited and that the original publication in this journal is cited, in accordance with accepted academic practice. No use, distribution or reproduction is permitted which does not comply with these terms.
*Correspondence: Chengjuan Gu, guchengjuan1116@163.com