- 1Center for Translational Immunology, Institute for Biomedical Sciences, Georgia State University, Atlanta, GA, United States
- 2Institute for Glycomics, Griffith University, Gold Coast, QLD, Australia
Neisseria gonorrhoeae and Neisseria meningitidis are human-specific pathogens in the Neisseriaceae family that can cause devastating diseases. Although both species inhabit mucosal surfaces, they cause dramatically different diseases. Despite this, they have evolved similar mechanisms to survive and thrive in a metal-restricted host. The human host restricts, or overloads, the bacterial metal nutrient supply within host cell niches to limit pathogenesis and disease progression. Thus, the pathogenic Neisseria require appropriate metal homeostasis mechanisms to acclimate to such a hostile and ever-changing host environment. This review discusses the mechanisms by which the host allocates and alters zinc, manganese, and copper levels and the ability of the pathogenic Neisseria to sense and respond to such alterations. This review will also discuss integrated metal homeostasis in N. gonorrhoeae and the significance of investigating metal interplay.
Introduction - Pathogenic Neisseriae Cause Devastating Yet Distinct Diseases to the Human Host
Neisseria gonorrhoeae and Neisseria meningitidis are human-specific pathogens of significant public health concern. Despite high DNA and amino acid sequence identity, N. gonorrhoeae and N. meningitidis cause significantly different diseases (Tinsley and Nassif, 1996; Perrin et al., 2002).
N. gonorrhoeae is the causative agent of the second most common reportable infectious disease in the United States, gonorrhea, and predominantly colonizes the genital mucosal epithelium and oropharynx (CDC, 2019b). Symptomatic gonococcal infection presents as urethritis, cervicitis, salpingitis, pharyngitis or conjunctivitis (CDC, 2019b). However, gonorrhea can present asymptomatically as well. Asymptomatic infection in women is of great concern as it enables the pathogen to ascend to the upper reproductive tract, where it can cause pelvic inflammatory disease. Pelvic inflammatory disease can lead to ectopic pregnancy, scarring, infertility, and chronic pelvic pain. In 2018, the incidence of gonococcal disease was 179.1 cases per 100,000, correlating with a total of 583,404 reported cases in the United States (CDC, 2019b).
N. meningitidis can inhabit the nasopharynx without eliciting symptoms; this carrier state can be found in 5-10% of the US population (CDC, 2019c). The carrier state can transition to symptomatic disseminated infection, sometimes referred to as invasive meningococcal disease (IMD), which is characterized by nausea, vomiting, rash, stiffness of neck, fever, and diarrhea (CDC, 2019c). While the incidence of meningococcal disease has decreased dramatically from ~1.50 per 100,000 in 1980 to ~0.2 per 100,000 in 2018, IMD remains a severe threat to infants. Incidence of IMD in children younger than 1 year has averaged around 1.20 per 100,000 in the past 10 years (CDC, 2019a). About 12% of infections result in death, and some survivors experience permanent brain damage, loss of limbs or hearing loss (Candrilli, 2019; CDC, 2019a).
While the pathogenic Neisseriae pose a direct threat to human health, they also represent a substantial economic burden in the United States. In 2018, gonorrhea infections resulted in an estimated $85 million in direct medical costs in the United States (Kumar et al., 2021). The estimated total cost of the response to an IMD outbreak at the University of Oregon Hospital (7 cases) and the Oregon State University Hospitals (6 cases) was $12.3 million (Candrilli, 2019).
During neisserial infection, the host limits bacterial proliferation by modulating metal availability through two related mechanisms: nutritional immunity and metal intoxication. Nutritional immunity is characterized by host sequestration of free metals from the bacterial nutrient supply, limiting metals required for enzymatic and metabolic functions (Hennigar and McClung, 2016). Metal intoxication is the process by which the host overloads pathogens with toxic metal concentrations (Becker and Skaar, 2014). Metal overload in bacteria contributes to reactive oxygen species (ROS) and reactive nitrogen species (RNS) cycling (Djoko et al., 2012), protein mismetallation (Veyrier et al., 2011), and subsequent stalling of respiration (Djoko and McEwan, 2013). Pathogenic bacteria have evolved mechanisms to access restricted metals as well as limit metal overload.
Metal availability within a biological niche dictates pathogen survival and the extent of pathogenesis. Although causing distinct disease presentations, pathogenic Neisseria share many mechanisms of responding to metal scarcity and overload to ensure survival and maintain virulence. This review aims to provide an overview of the neisserial response to nutritional immunity and metal intoxication with respect to zinc, manganese, and copper.
Zinc, Manganese, and Copper Are Allocated to Specific Host Niches
Transition metals, such as zinc, manganese, and copper, are essential to many host processes, including oxidative stress resistance (Girotto et al., 2014; Jarosz et al., 2017; Ganini et al., 2018), cell signaling and metabolism (Maares et al., 2018), immune modulation (Hu Frisk et al., 2017), post-translational modifications (Braiterman et al., 2015; Tidball et al., 2015), and structural maintenance and enzymatic processing (Tidball et al., 2015; Ganini et al., 2018). These metals play similar roles in pathogens including Neisseria meningitidis (Persson et al., 2001; Pawlik et al., 2012; Hecel et al., 2018) and Escherichia coli (Kaur et al., 2017).
Zinc within the human body is primarily localized to the bone and skeletal muscle, with moderate concentrations found in the kidneys and liver (Jackson, 1989). Most zinc, however, is metabolically unavailable to the host due to slow zinc turnover with the exception being zinc found in the sperm (Baer and King, 1984). High levels of zinc-binding metallothioneins (Suzuki et al., 1994), which maintain zinc and copper homeostasis and limit heavy toxicity in host cells (Rahman and Karim, 2018), can be found in male secretions (Suzuki et al., 1992). It is feasible that zinc-binding metallothioneins help create a zinc limited environment for the gonococcus during male urethral infection. The majority of zinc within the host is not easily accessible to pathogens due to a limited pool of labile zinc (Brown et al., 2001), which can be further restricted during infection by production of calprotectin and other S100 proteins that act to sequester free zinc away from the pathogen (Yadav et al., 2020). Calprotectin makes up 45% of the protein content in neutrophils (Edgeworth et al., 1991) and is released following neutrophil death (Voganatsi et al., 2001) and Neutrophil Extracellular Trap (NET) formation (Urban et al., 2009). The zinc sequestering protein, S100A7, is enriched in lower genital tract epithelial cells (Mildner et al., 2010). In the case of mucosal infection by N. gonorrhoeae, a robust Th17 response results in the influx of neutrophils (Liu and Russell, 2011). Thus, S100A7 and calprotectin, which has been released by neutrophils, create a zinc limited environment for N. gonorrhoeae (Zackular et al., 2015). The remaining zinc is dispersed among the reproductive (Baer and King, 1984) and immune systems (Brown et al., 2001).
Within the human host, manganese exists as Mn2+ and Mn3+ (O'Neal and Zheng, 2015). Mn2+ is found in the blood bound to albumin, ß-globulin, bicarbonate, and citrate, and in the cytosolic content of neutrophils bound to calprotectin (O'Neal and Zheng, 2015; Zackular et al., 2015). Within the cell, Mn2+ is found at the highest concentrations in the endoplasmic reticulum and mitochondria, where it plays an antioxidant role through Mn-dependent superoxide dismutase (MnSOD) (Maynard and Cotzias, 1955; Ganini et al., 2018). In neurons, Mn2+ is required for signal transduction and enzymatic function (Gunter et al., 2013; Tidball et al., 2015). Excess Mn2+ accumulates in the liver, kidneys, bone, and pancreas, with higher levels bound to regulatory proteins in the brain and cerebrospinal fluid. Mn3+ can be bound to transferrin, which transports Mn3+ to neuronal cells in a mechanism similar to Fe3+ transport (Chen et al., 2001; Gunter et al., 2013). Many Gram-negative pathogens utilize Mn2+ (i.e. Acinetobacter baumanii, Salmonella enterica, E. coli, Helicobacter pylori, and N. gonorrhoeae), in the face of nutritional immunity, for oxidative stress resistance and metabolism (Tseng et al., 2001; Kehres et al., 2002b; Anjem et al., 2009; Lee et al., 2010; Diaz-Ochoa et al., 2016; Juttukonda et al., 2016). During gonococcal infection of macrophages, the manganese transport protein Natural resistance-associated macrophage protein 1 (NRAMP1) (Jabado et al., 2000), is upregulated on the phagosomal membrane (Forbes and Gros, 2003; Zughaier et al., 2014). NRAMP1 shuttles manganese from the phagosomal compartment to the cell cytosol to restrict pathogen access to manganese. Consequently N. gonorrhoeae may experience manganese limitation in the endolysosomal compartment following phagocytosis by macrophages (Ivanov et al., 2022).
Copper is found primarily in the liver and plasma in free and ceruloplasmin-bound forms (Dunn et al., 1991). Ceruloplasmin facilitates copper transport through the vasculature and possesses the ferroxidase activity necessary for the oxidation of Fe2+ to Fe3+ and subsequent iron loading of transferrin (Ramos et al., 2016; Hyre et al., 2017). Ceruloplasmin is also transported to the urinary tract during human infection (Hyre et al., 2017). Thus, N. gonorrhoeae may experience ceruloplasmin-dependent copper limitation in addition to S100 protein-dependent zinc limitation at the mucosal surface. Copper can also be found within the cytosol of neutrophils, which are recruited to the gonococcal infection site (Johnson and Criss, 2011; Sintsova et al., 2014), following import by CTR1 on the neutrophil membrane (Zhou and Gitschier, 1997; Cichon et al., 2020).
Metal Acquisition by the Pathogenic Neisseriae in Metal-Restricted Niches Requires Highly Specific Metal Import
Metal import poses a particular challenge to Gram-negative bacteria, as it requires transport across a two-component cell wall. Outer membrane transport utilizes the proton motive force and requires energy transduction, via the Ton system, from the cytoplasmic membrane. Scarce metals (i.e., zinc, manganese, and copper) are transported into the cytoplasm in an ATP-driven mechanism, which is often tightly regulated to avoid metal overload, protein mismetallation, and oxidative stress. Highly specific metal transport is required in a host that uses metal sequestration to restrict microbial growth and pathogenesis.
In the human host, which allocates metals to specific niches, the pathogenic Neisseria, N. gonorrhoeae and N. meningitidis, have evolved mechanisms to acquire zinc, manganese, and copper in specific environments. Gonococcal TdfH and TdfJ are zinc-specific TonB-dependent transporters that pirate zinc from calprotectin (Kammerman et al., 2020) and S100A7 (Maurakis et al., 2019), respectively, to transport that zinc across the outer membrane to the periplasm (Figure 1). Gonococcal TdfH binds calprotectin through a high-affinity bimodal interaction. TdfH interacts with a tetramer of calprotectin, which itself is a heterodimer of S100A8 and S100A9 (Bera et al., 2022). Interestingly, gonococcal growth when calprotectin is the sole zinc source requires the presence of zinc in site 1 of calprotectin, the preferred zinc utilization site by gonococcal TdfH. Mutant calprotectin unable to bind zinc at site 2 fully supports gonococcal growth (Kammerman et al., 2020). The cryoEM structure of the calprotectin:TdfH complex has been determined by Bera et al. (Bera et al., 2022). Since site 1 of calprotectin is capable of binding both zinc and manganese (Gagnon et al., 2015) and gonococcal TdfH is able to bind manganese-loaded calprotectin (Bera et al., 2022), it is feasible that TdfH may also be a manganese importer. The meningococcal TdfH homolog was renamed calprotectin-binding protein A (CbpA); this protein has been shown to bind to zinc- or manganese-loaded calprotectin (Table 1) with higher affinity than it does to unloaded calprotectin, demonstrating a preference for metalated calprotectin over the apo form (Figure 1) (Stork et al., 2013). Gonococcal TdfJ was shown to bind S100A7 with high specificity and to use the human protein as a zinc source (Maurakis et al., 2019). Similarly, the meningococcal homolog of TdfJ is a zinc-specific importer required for zinc import during zinc limitation (Stork et al., 2010).
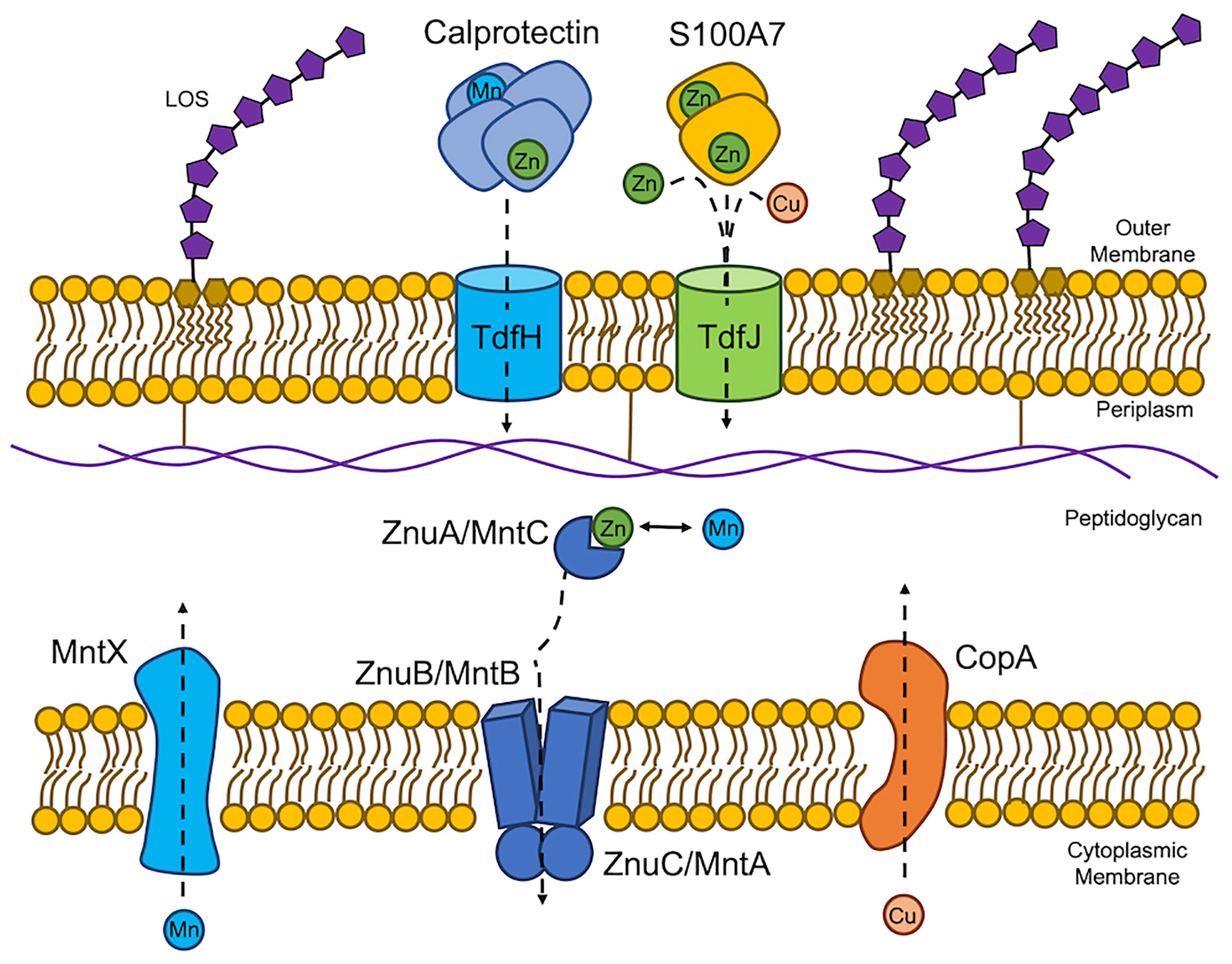
Figure 1 Neisseria gonorrhoeae and Neisseria meningitidis experience host-driven nutritional immunity and metal intoxication. The host exerts nutritional immunity on the pathogenic Neisseriae by exposing the bacteria to calprotectin and S100A7. Calprotectin sequesters both zinc (Zn) and manganese (Mn) from the extracellular environment to limit Zn and Mn availability. N. gonorrhoeae in turn expresses TdfH, which has been shown to strip Zn from calprotectin and subsequently import the ion. TdfH is able bind Mn-loaded calprotectin suggesting a role for Mn import across the outer membrane. TdfH is referred to as CbpA in N. meningitidis. S100A7 also sequesters Zn from the extracellular environment. Gonococcal TdfJ binds S100A7 to pirate and import the Zn payload. Lipooligosaccharide (LOS) is shown in the outer membrane for reference. Once in the periplasm, Zn and Mn are chaperoned by the periplasmic binding protein, ZnuA (MntC) to the permease in the cytoplasmic membrane, ZnuB (MntB). Transport across the cytoplasmic membrane is energized by the ATPase, ZnuC (MntA). The host also exerts metal intoxication, specifically copper (Cu) intoxication on N. gonorrhoeae and Mn intoxication in N. meningitidis through unknown mechanisms. In response, these efflux proteins export excess cytoplasmic Cu or Mn from to the periplasmic space.
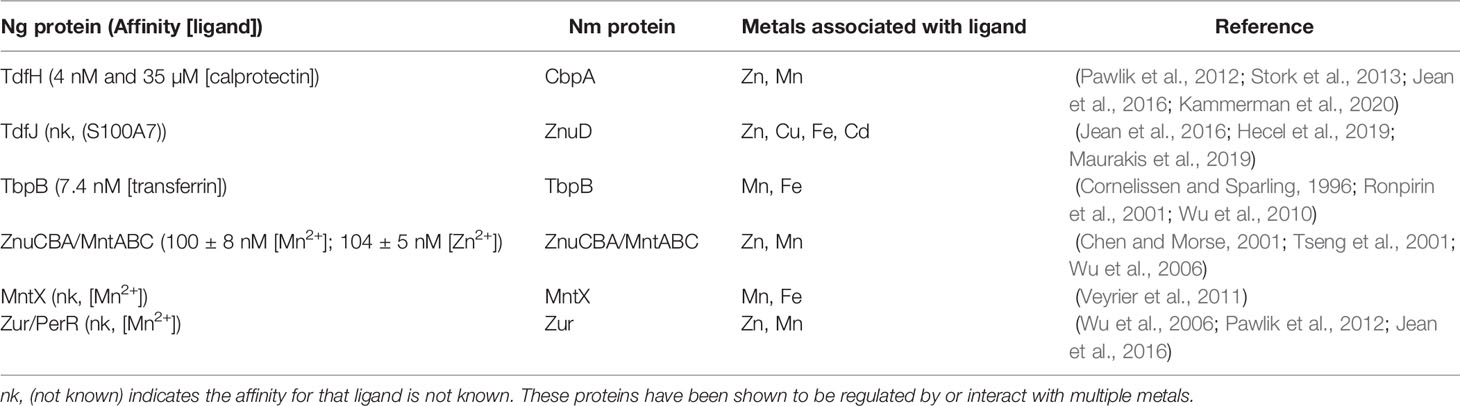
Table 1 Neisseria gonorrhoeae (Ng) and Neisseria meningitidis (Nm) express proteins which are potentially involved in integrated metal homeostasis.
After crossing the outer membrane, metals must be escorted across the periplasm to transporters in the cytoplasmic membrane. Metal chaperoning across the periplasm is often accomplished by periplasmic metal-binding proteins (PBP) of the Cluster A-I substrate-binding protein family. PBP transport precedes the ATP-dependent transport step through the cytoplasmic membrane (Dintilhac and Claverys, 1997; Berntsson et al., 2010). Precise metal transport across the periplasm is required for acclimation to the specific metal environments encountered by Gram-negative pathogens (Lewis et al., 1999; Ammendola et al., 2007; Davies and Walker, 2007; Lim et al., 2008; Davis et al., 2009; Desrosiers et al., 2010; Pederick et al., 2015). PBPs deliver specific metals to permeases in the cytoplasmic membrane, where an ATPase then hydrolyzes ATP to energize metal transport into the cytoplasm.
N. gonorrhoeae express a zinc import system encoded by znuCBA (NGO_0170-_0168) (accession number NC_002946) where the gene products, ZnuC, ZnuB, and ZnuA are the ATPase, permease, and PBP, respectively (Figure 2 and Table 1). ZnuCBA transports zinc through the periplasm and across the cytoplasmic membrane. A znuA mutant was growth deficient in the presence of all supplemental metals (i.e. Mg2+, Mn2+, Cu2+, Ni2+, Fe2+, Fe3+, Ca2+, and Cd2+) except Zn2+, demonstrating the specificity of this importer for zinc, over other metals, under these conditions (Chen and Morse, 2001). Growth only with supplemental zinc suggests that the gonococcus requires specific zinc import via ZnuA for cellular processes that cannot be completed with substituting metals under these conditions.
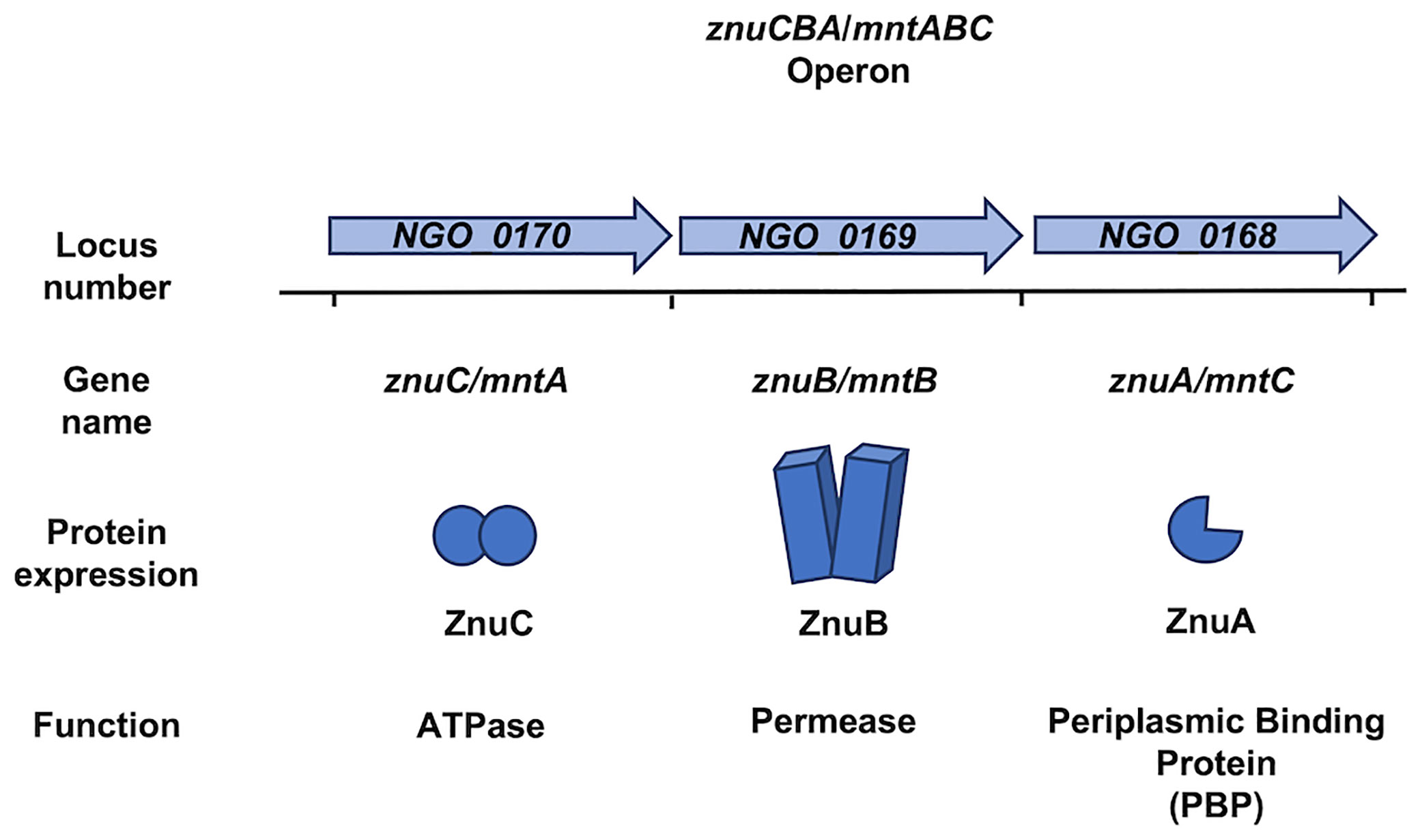
Figure 2 The genome of Neisseria gonorrhoeae and Neisseria meningitidis encodes the znuCBA/mntABC operon. The znuCBA operon in N. gonorrhoeae is indicated by the locus numbers NGO_0170, NGO_0169, and NGO_0168. The operon is named in order of predicted transcriptional direction. NGO_0170 encodes an ATPase named ZnuC which provides energy from zinc (Zn) transport into the cytoplasm. NGO_0169 encodes a permease named ZnuB which serves as a channel for transporting Zn through the cytoplasmic membrane. NGO_0160 encodes ZnuA, which chaperones Zn from the periplasm and delivers it to ZnuB. A simplified image of the znuCBA gene products is depicted. The gene products of the NGO_0170, NGO_0169, and NGO_0168 have been implicated in both Zn and manganese (Mn) binding and transport. Thus, znuCBA is also referred to as mntABC in reference to Mn transport. znuCBA and mntABC are two different names to identify the same genetic sequence indicated by the locus numbers NGO_0170, NGO_0169, and NGO_0168. The znuCBA operon including the intergenic regions in N. gonorrhoeae is 92% identical to that in N. meningitidis (Accession number AE002098.2), in which znuCBA is also responsible for Zn and Mn import.
A manganese-specific outer membrane importer has not been identified in pathogenic Neisseriae despite the requirement for manganese, rather than zinc, cobalt, or magnesium, to resist oxidative killing (Tseng et al., 2001). However, the ability of TdfH to bind to manganese-loaded calprotectin suggests the possibility for highly specific manganese import across the outer membrane in the pathogenic Neisseriae (Table 1) (Bera et al., 2022).
The znuCBA operon is also referred to as mntABC in the context of manganese transport through the periplasm and across the cytoplasmic membrane (Table 1). MntA, MntB, and MntC are the ATPase, permease, and PBP, respectively. znuCBA and mntABC are different names for the same operon. znuCBA was used to describe the operon when the gene products were demonstrated to be involved in zinc import; somewhat confusingly, mntABC was deployed as the term to describe the operon when the gene products were involved in manganese import (Figure 2). The gene locus is the same for both systems and presumably encodes the proteins required for both manganese and zinc import.
ZnuA (Figure 2), from Ngo strain FA1090 (accession number NC_002946) shares 96% amino acid identity with a zinc ABC transport PBP encoded by N. meningitidis. This high sequence similarity suggests that N. meningitidis also requires a PBP for zinc and manganese transport through the periplasm. A gonococcal mntC mutant imports 500-fold less manganese than the wild type, demonstrating a dual metal-binding capacity (Tseng et al., 2001). MntC binds manganese and zinc with nearly equal affinity (100 ± 8 nM for Mn2+ and 104 ± 5 nM for Zn2+), suggesting that the gonococcus occupies niches during infection that are limited in both metals (Chen and Morse, 2001; Lim et al., 2008). This import system may be required for growth and pathogenesis in N. meningitidis much like it is in N. gonorrhoeae.
Calmettes et al. showed that the meningococcal TdfJ homolog, ZnuD, crystalizes with zinc and cadmium (Table 1) at distinct binding sites in the absence of a chelator, suggesting the ability to bind both ions in their free form (Calmettes et al., 2015). Hecel et al. defined the metal binding specificity of the flexible loop responsible for ion capture by ZnuD (Hecel et al., 2019), noting that this flexible loop binds copper (Figure 1) with higher affinity and stability than it does zinc and that the loop undergoes a substantial conformational change upon copper binding (Hecel et al., 2019). The ability of ZnuD/TdfJ to bind copper suggests that this transporter may import copper in addition to zinc (Figure 1, Table 1).
A periplasmic copper chaperone has not yet been identified in the pathogenic Neisseriae. However, the ability of ZnuD to bind free copper suggests that copper could be imported through the outer membrane to the periplasm and may require a chaperone for delivery to the cytoplasmic membrane.
Metal Availability Sensors Regulate Metal Import Genes
Metal-dependent regulation in bacteria is often accomplished by the ferric uptake regulator (Fur) -family proteins (Taylor-Robinson et al., 1990). Fur-family metalloregulators include Fur, PerR, Mur, Nur, Zur, and Irr, which are responsible for regulating iron uptake, peroxide stress sensing, manganese uptake, nickel uptake, zinc uptake, and heme-dependent iron uptake (Fillat, 2014). These Fur-family regulators are responsible for sensing metal availability within microenvironments inhabited by pathogens and subsequently coordinating a transcriptional response.
Pathogenic Neisseriae express two well-characterized metal dependent regulators: Fur and Zur (Zur has also previously been called PerR) (Wu et al., 2006; Pawlik et al., 2012; Jean, 2015; Jean et al., 2016). In N. gonorrhoeae, Zur is hypothesized to repress zinc and manganese import genes in the presence of these metals and to de-repress zinc and manganese import genes in the absence of these metals (Table 1) (Chen and Morse, 2001; Wu et al., 2006). Production of gonococcal proteins TdfJ and TdfH is zinc-repressed in a Zur-depending manner (Jean et al., 2016). In the context of infection at mucosal surfaces and in neutrophils, N. gonorrhoeae requires a zinc sensor, such as Zur, to mount a transcriptional response to calprotectin- and S100A7- mediated zinc limitation. Zur de-represses expression of high-affinity metal importers, including znuCBA, tdfJ, and tdfH, so that the gonococcus can effectively and efficiently import Zn. Meningococcal Zur specifically binds to the promoter of znuD (PznuD) in the presence of Zn2+, but not Ca2+, Co2+, Cu2+, Fe2+, Mg2+, Mn2+, or Ni2+. Zinc-dependent binding of Zur to PznuD is abrogated with the addition of a zinc-specific chelator, N,N,N′,N′-tetrakis (2-pyridinylmethyl)-1,2-ethanediamine (TPEN) (Pawlik et al., 2012), suggesting that Zur is responsible for sensing zinc availability and coordinating a transcriptional response that allows for zinc acquisition. Microarray and RT-qPCR analyses also showed zinc-dependent regulation of 11 other genes, including cbpA, znuCBA, the high-affinity zinc ABC importer, and genes encoding multiple ribosomal proteins, nitrosative stress resistance proteins, and metabolic proteins (Pawlik et al., 2012; Stork et al., 2013).
Manganese-dependent regulation by gonococcal Zur (also referred to as PerR) was demonstrated by Tseng et al. and Wu et al. (Table 1) (Tseng et al., 2001; Seib et al., 2006; Wu et al., 2006). Wu et al. established that znuCBA (mntABC) is manganese-repressed in a Zur-dependent manner. Manganese has also been shown to upregulate many ribosomal proteins, pilus assembly proteins, adhesion proteins, outer membrane proteins, the multidrug efflux pump protein channel, MtrE, and many metabolic proteins (Wu et al., 2010). Interestingly, the iron-repressed transferrin-binding protein A (TbpA) and the transport protein ExbB were also manganese-repressed (Ronpirin et al., 2001; Wu et al., 2010). These data suggest that gonococcal Zur senses manganese limitation during infection where calprotectin and potentially other manganese-binding proteins sequester manganese.
Copper sensing and regulation in bacteria are often accomplished by CueR, which is absent from the gonococcal genome (accession number NC_002946; Arguello et al., 2013). The genome of N. meningitidis encodes a putative CueR regulator (accession number MBF1297094.1) that is 47.62% identical to that found in E. coli (accession number NP_415020). Although, it has not yet been empirically characterized as a copper-dependent regulator. Neisserial MisR (accession number WP_002214312.1), is homologous to CueR. MisR is the response regulator of theMisR-MisS two-component regulatory system and is known to be involved in cationic antimicrobial peptide resistance (Kandler et al., 2016). Interestingly, MisR is 36% identical and 58% similar to Pseudomonas aeruginosa CopR, which is involved in regulation of copper homeostasis (Novoa-Aponte et al., 2020).
Much work is needed to characterize the ability of pathogenic Neisseriae to sense and regulate copper, considering that ceruloplasmin is found in the serum, which is a meningococcal infection site (Osaki et al., 1966). Ceruloplasmin concentrations in the cerebrospinal fluid (0.8-2.2 µg/mL) are 100-500-fold lower than that in the serum (Irani, 2008). The gonococcus may also need to sense copper levels, considering the potential for copper to fluctuate following CTR1 protein expression within neutrophils.
Transition Metals Are Required for Survival and Virulence
Bacteria utilize scarce metals for several mechanisms related to survival and virulence, such as resistance to reactive oxygen species (ROS) and reactive nitrogen species (RNS), metabolism, maintenance of cell structural integrity, and proper protein structure and function.
Zn contributes to the function of biosynthetic pathways and virulence in N. gonorrhoeae and N. meningitidis as a cofactor for enzymes, enabling survival and virulence. For example, in both species, biosynthesis of lipid A, a potent immune activator (Mandrell et al., 1988; Steimle et al., 2016), involves a putative zinc-dependent metalloamidase, UDP-3-O-(R-3- hydroxymyristoyl)-N-acetylglucosamine deacetylase (LpxC) (Barb and Zhou, 2008; Mochalkin et al., 2008; John et al., 2018). Lipid A anchors LOS into the bacterial membrane and can activate the immune system after its release from the bacterial cell wall (Steimle et al., 2016). Additionally, it can be directly recognized by host Lipid-A binding protein (LPB), which plays a role in sensing of pathogenic (Knapp et al., 2003) and commensal species (Steimle et al., 2016). Thus, LOS is a key virulence factor in N. gonorrhoeae and N. meningitidis. Similarly, the N. meningitidis protein, Ght, a zinc binding protein involved in LOS biogenesis, (Putker et al., 2014) is involved in LOS expression and outer membrane integrity (Putker et al., 2014). These observations implicate zinc in virulence and survival.
Manganese contributes to oxidative stress resistance in the pathogenic Neisseriae and thus contributes to survival during infection at highly oxidative sites (Tseng et al., 2001; Wu et al., 2006). Manganese in bacteria cycles between the Mn2+ and Mn3+ states during MnSOD processing of reactive oxygen species (Abreu and Cabelli, 2010). Interestingly, the pathogenic Neisseria do not express a MnSOD and instead use manganese directly as an ROS quencher (Seib et al., 2006). Wu et al. demonstrated a role for gonococcal mntC in the oxidative stress response under anaerobic rather than aerobic conditions (Wu et al., 2009). The vagina and cervix are normally oxygen-depleted, making anaerobic gonococcal growth conditions highly relevant (Hill et al., 2005). In vitro, growth of an mntC mutant under anaerobic conditions was inhibited by paraquat, an intracellular inducer of ROS, to an extent similar to that of the wild type (Wu et al., 2009). However, the mntC mutant was less competitive than the wild type during in vivo infection, which is characterized by both anaerobic and highly oxidative conditions (Wu et al., 2009). Reduced competition by the mntC mutant under anaerobic and oxidative conditions suggests that manganese is critical to gonococcal growth within the cervical niche. In contrast to the gonococcus, growth of the meningococcus in the presence of manganese does not enhance oxidative stress resistance (Seib et al., 2004).
N. meningitidis is able to grow on manganese concentrations 50-100 times higher (>100 mmol/L) than N. gonorrhoeae, which suggests that manganese homeostasis differs between these species (Tseng et al., 2001; Seib et al., 2004). Despite the non-restorative effect of manganese during oxidative conditions in the meningococcus, manganese is a vital cofactor in biosynthetic pathways within the bacterium. Meningococcal sialic acid synthase, NeuB, was shown to crystalize best with the addition of manganese, suggesting that this enzyme also requires a manganese cofactor (Tseng et al., 2001; Gunawan et al., 2005). NeuB is involved in sialylated capsule formation (Gunawan et al., 2005), and the sialylated surface of N. meningitidis has been shown to protect the bacterium from complement deposition (van Emmerik et al., 1994) through molecular mimicry of host cell surface proteins (Mandrell et al., 1988). Due to the potential involvement of manganese in NeuB activity, and consequently capsule formation, the metal may play a role in protection from host complement deposition and thus in immune evasion. This mechanism of innate immune evasion is particularly useful to the pathogen during infection of the vasculature, a niche that is complement is enriched.
In the pathogenic Neisseria, copper plays a role in resistance to extracellular RNS (Mellies et al., 1997; Boulanger and Murphy, 2002; Seib et al., 2006). N. gonorrhoeae (Gotschlich and Seiff, 1987) and N. meningitidis (Woods et al., 1989) possess a surface-exposed lipid-modified azurin (Desrosiers et al. 2010), which is a putative electron donor to peroxidases (Seib et al., 2006). This biological function is particularly relevant to macrophage infection because they are known to increase the expression of nitric oxide synthase (NOS) upon stimulation with LOS (Blondiau et al., 1994; Iovine et al., 2008). N. gonorrhoeae can survive in the harsh environment of macrophage phagosomes, potentially through a mechanism involving copper-bound Laz (Château and Seifert, 2016; Quillin and Seifert, 2018). Interestingly, the gonococcal genome encodes a putative peptidase with a PepSy domain (Accession number WP_003702955.1). This peptidase is 34% identical and 51% similar to that produced by P. aeruginosa (NCBI Reference Sequence: NP_252478.1). The peptidase in P. aeruginosa has been shown to be copper-repressed (Quintana et al., 2017). A similar putative peptidase is predicted to be produced by the meningococcus (Accession number WP_079889394.1) and is 36% identical and 50% similar to that from P. aeruginosa. The role of copper in the regulation and function of this peptidase in N. gonorrhoeae and N. meningitidis is a potential focus of future investigation.
The Response to Metal Overload Requires Sensing and Export of Intoxicating Metals
The host applies metal intoxication strategies to limit bacterial growth and survival. Metal intoxication is the process by which the host floods the bacterial nutrient supply with metals. The consequences of metal intoxication for bacteria include electron transport chain (ETC) inhibition, protein mismetallation, and ROS and RNS accumulation (Chandrangsu et al., 2017). To limit these consequences, bacteria utilize mechanisms that store or export excess metal and repress metal import systems (Chandrangsu et al., 2017). Metal toxicity in N. gonorrhoeae has also been shown in reference to Mn2+, Co2+, Ni2+, and Zn2+ (Odugbemi et al., 1978; Veyrier et al., 2011). However, specific responses to overload of each metal remain poorly characterized.
While macrophages have not been shown to exert metal intoxication upon the pathogenic Neisseria species, these immune cells have demonstrated the ability to increase the concentration of zinc in the cytosol and the phagocytic vacuole via the SLC39A transporters (Begum et al., 2002; Stafford et al., 2013; Chandrangsu et al., 2017) suggesting that host-induced zinc toxicity may be relevant to pathogenic Neisseria infection. Macrophages have been shown to increase phagosomal zinc concentrations to apply metal stress on invading Mycobacterium species (Wagner et al., 2005; Lefrançois et al., 2019). In Streptococcus pneumoniae, excess zinc competes with manganese for binding to pneumococcal surface antigen A (PsaA), resulting in reduced manganese uptake, reduced oxidative stress resistance, and reduced resistance to PMN killing (McDevitt et al., 2011). In E. coli, high levels of manganese correlate with reduced levels of Fe2+, iron-containing enzymes in the ETC and TCA cycle (iron-sulfur clusters and heme-containing enzymes), and consequently, reduced levels of NADH and ATP (Kaur et al., 2017).
Although not empirically tested in the gonococcus, manganese intoxication has been tested and shown to be relevant to meningococcal growth. Excess manganese in N. meningitidis results in protein mismetalation and subsequent dysregulation of Fur-regulated genes (Table 1) (Veyrier et al., 2011). Under excess manganese conditions, the meningococcus expresses mntX, the gene encoding a manganese export protein, which is critical to survival under high manganese conditions. MntX contains predicted transmembrane domains suggesting that this protein transports manganese from the cytoplasm to the periplasm (Veyrier et al., 2011). The mntX mutant exhibited a reduced ability to survive in the blood of infected mice relative to the wild-type strain (Veyrier et al., 2011). Additionally, the mntX mutant showed reduced resistance to human serum (Veyrier et al., 2011). Taken together, these data suggest that the meningococcus senses high manganese during septicemic infection and responds by expressing mntX. It is also feasible that the meningococcus requires a manganese exporter during infection in the cerebrospinal fluid. The blood and cerebrospinal fluid are body sites that are manganese-enriched and may be a hostile environment for a pathogen lacking a Mn exporter. Metal toxicity in N. meningitidis has also been shown in reference to Cu2+, Co2+, Ni2+, and Zn2+ (Odugbemi et al., 1978). Conversely, mntX is frameshifted in 66% of sequenced N. gonorrhoeae strains. A N. gonorrhoeae strain, which was sensitive to manganese in this way, could be rescued through complementation with meningococcal mntX (Veyrier et al., 2011). Expression of mntX in certain gonococcal strains may enable dissemination to the blood and meninges.
Copper intoxication is used by the host to limit bacterial infection. For example, copper influx into the phagosomal compartment of macrophages results in increased killing of an E. coli strain deficient in a copper efflux protein, CopA, relative to the wild type (White et al., 2009). The gonococcus also produces a copper efflux protein, CopA, in the cytoplasmic membrane (Djoko et al., 2012). CopA likely transports copper from the cytoplasm to the periplasm. A copA mutant exhibited higher concentrations of internal copper and was growth impaired under high copper conditions (Djoko et al., 2012). Following copper supplementation, the copA mutant was limited in its ability to associate with and invade primary human cervical epithelial cells; it was also less resistant to killing by nitrite and S-nitrosoglutathione (GSNO), a nitric oxide generator (Djoko et al., 2012). This data suggest N. gonorrhoeae experiences copper overload within cervical epithelial cells. Djoko and McEwan showed that high levels of copper increase gonococcal sensitivity to the RNS generator, sodium nitrite and suggested that copper-dependent inactivation of hemoproteins involved in intracellular RNS detoxification results in RNS-dependent killing of N. gonorrhoeae (Djoko and McEwan, 2013).
While the concept of nutritional immunity has been thoroughly studied, the concept of metal intoxication requires further exploration. Expanded application of this concept to pathogenic Neisseriae infection will broaden our understanding of metal homeostasis and its role in pathogenesis.
Next Steps - Comprehensive Characterization of Nutritional Immunity and Metal Intoxication Requires Insight Into Integrated Metal Homeostasis
Pathogens possess mechanisms of defense against metal starvation and metal intoxication exerted by the host, as these metals play integral roles in metabolism, maintenance of cell structural integrity, and ROS and RNS resistance. Metal involvement in these processes is often studied in isolation, meaning only one metal (i.e., zinc, manganese, or copper) is considered at a time. However, it is improbable that pathogens encounter a single type of metal depletion or stress during infection of a host whose metal allocations and concentrations are heterogeneous.
One of the most thoroughly investigated examples of metal interplay is that between manganese and zinc in S. pneumoniae. Cell-associated manganese in wild-type S. pneumoniae is substantially reduced in the presence of excess zinc, and zinc depletion of cellular manganese could be restored by the addition of excess manganese (Jacobsen et al., 2011). Under high zinc conditions, zinc competes with and inhibits manganese binding to the manganese importer, PsaA, resulting in manganese starvation and zinc toxicity (Jacobsen et al., 2011; Eijkelkamp et al., 2014). Zinc-induced manganese starvation leads to increased sensitivity to oxidative stress (Eijkelkamp et al., 2014). Another example of metal interplay has been investigated in S.enterica Serovar Typhimurium. In this bacterium, mntH encodes a manganese importer that is iron-repressed in a Fur-dependent manner and manganese-repressed in a manganese transport repressor (MntR) -dependent manner (Kehres et al., 2002a). Kehres et al. hypothesized that co-regulation of mntH maintains an equilibrated Mn2+/Fe2+ ratio in Salmonella (Kehres et al., 2002a). The genomes of N. gonorrhoeae and N. meningitidis encode a MntH homolog; however, the functions of this Fe2+/Mn2+ symporter in manganese or iron import have yet to be tested in the Neisseriae. Helicobacter pylori expresses a metal efflux system, CznABC, which interacts with cadmium, zinc, and nickel and confers resistance to intoxicating levels of all three metals (Stähler et al., 2006). Acinetobacter baumannii, when grown in the presence of calprotectin that is able to simultaneously chelate zinc and manganese, exhibits reduced intracellular manganese and zinc but increased iron levels (Hood et al., 2012). In this case, calprotectin treatment not only resulted in altered zinc, manganese, and iron homeostasis but also in reduced growth (Hood et al., 2012). In Klebsiella pneumoniae, the zinc efflux protein, ZntA, is responsible for exporting zinc from the cytoplasm (Maunders Eve et al., 2022). The zntA mutant was shown to accumulate more manganese in addition to zinc and less iron than the wild type when subject to high zinc conditions, demonstrating integrated zinc, manganese, and iron homeostasis in wild type K. pneumoniae (Maunders Eve et al., 2022).
Pathogenic Neisseriae sense metal concentrations in the environment and respond by altering the expression of metal import or export systems and allocating these metals to metabolic and biosynthetic processes to allow for survival and virulence. N. gonorrhoeae and N. meningitidis are similar pathogens, which respond similarly to metal limitation and overload within the same host, despite causing different physiological symptoms. It is unlikely that the pathogenic Neisseria experience zinc, manganese, and copper starvation or intoxication in isolation from other metals. Interaction with multiple metals by neisserial proteins suggests the need for complex and integrated metal homeostasis (Table 1). This is evidenced by the ZnuCBA manganese import system in N. gonorrhoeae, which is manganese-regulated in a Zur-dependent manner but is also responsible for zinc import (Table 1) (Chen and Morse, 2001; Tseng et al., 2001; Wu et al., 2006). More work needs to be done to characterize Zur metal sensing when zinc and manganese are present together. Gonococcal TdfJ is zinc-repressed in a Zur-dependent manner and is iron-induced (Jean et al., 2016). The zinc to iron ratio required for optimal TdfJ expression in the presence of S100A7 should be addressed in future studies. Gonococcal TbpA (Table 1) is both iron- (Ronpirin et al., 2001; Vélez Acevedo et al., 2014) and manganese-repressed (Wu et al., 2010). TbpA is an iron-repressed (Ronpirin et al., 2001; Vélez Acevedo et al., 2014) TonB-dependent transporter that pirates iron from human transferrin and transports it across the outer membrane (Noto and Cornelissen, 2008; Cash et al., 2015). Considering that TbpA is also manganese-repressed and that transferrin can bind manganese in a manner similar to iron, it would be interesting to determine whether TbpA can pirate manganese from manganese-loaded transferrin. Additionally, studies regarding the application of this potential manganese transport system to a host niche would be informative. The manganese export protein, MntX, in N. meningitidis is required for survival under high manganese and low iron conditions, and the absence of the gene encoding this system results in mis-regulation of iron-regulated genes (Table 1) (Veyrier et al., 2011). It would be informative to discern the exact manganese to iron ratio required for optimal mntX expression and consequent serum resistance in N. meningitidis. In-frame MntX is only present in a subset of gonococcal strains (Veyrier et al., 2011).
The evolution of complex metal regulatory and import mechanisms suggests that the pathogenic Neisseriae possess a need for multifactorial metal homeostasis. Instances of overlap in different metal-related processes imply that the pathogenic Neisseriae may specifically require the integration of manganese and iron homeostasis and zinc and copper homeostasis. The exact mechanisms of integrated metal homeostasis and the host conditions under which they are relevant have not yet been fully deciphered. Further investigation into the complex metal environment sensed by the bacteria in the host could broaden our understanding of mixed metal homeostasis and the neisserial response to nutritional immunity and metal intoxication.
Author Contributions
AHB completed the literature review and manuscript drafting and editing based on JLS, KSL, and CNC comments. JLS reviewed and proofread the manuscript multiples times before review by KLS. CNC then reviewed and proofread the manuscript and acquired funding. All authors contributed to the article and approved the submitted version.
Funding
This work was funded by the National Institute of Allergy and Infectious Diseases, award numbers U19AI144182, R01AI127793, and R01AI125421 to CC. The funder had no role in data collection, synthesis, analysis, interpretation, or management of the data presented in this review. The funder had no role in review generation and revision, or in the decision to submit this review for publication.
Conflict of Interest
The authors declare that the research was conducted in the absence of any commercial or financial relationships that could be construed as a potential conflict of interest.
Publisher’s Note
All claims expressed in this article are solely those of the authors and do not necessarily represent those of their affiliated organizations, or those of the publisher, the editors and the reviewers. Any product that may be evaluated in this article, or claim that may be made by its manufacturer, is not guaranteed or endorsed by the publisher.
References
Abreu, I. A., Cabelli, D. E. (2010). Superoxide Dismutases—A Review of the Metal-Associated Mechanistic Variations. Biochim. Biophys. Acta (BBA) - Proteins Proteomics 1804 (2), 263–274. doi: 10.1016/j.bbapap.2009.11.005
Ammendola, S., Pasquali, P., Pistoia, C., Petrucci, P., Petrarca, P., Rotilio, G., et al. (2007). High-Affinity Zn2+ Uptake System ZnuABC Is Required for Bacterial Zinc Homeostasis in Intracellular Environments and Contributes to the Virulence of Salmonella Enterica. Infect. Immun. 75 (12), 5867. doi: 10.1128/IAI.00559-07
Anjem, A., Varghese, S., Imlay, J. A. (2009). Manganese Import is a Key Element of the OxyR Response to Hydrogen Peroxide in Escherichia Coli. Mol. Microbiol. 72 (4), 844–858. doi: 10.1111/j.1365-2958.2009.06699.x
Arguello, J., Raimunda, D., Padilla-Benavides, T. (2013). Mechanisms of Copper Homeostasis in Bacteria. Front. Cell. Infect. Microbiol. 3 (73). doi: 10.3389/fcimb.2013.00073
Baer, M. T., King, J. C. (1984). Tissue Zinc Levels and Zinc Excretion During Experimental Zinc Depletion in Young Men. Am. J. Clin. Nutr. 39 (4), 556–570. doi: 10.1093/ajcn/39.4.556
Barb, A. W., Zhou, P. (2008). Mechanism and Inhibition of LpxC: An Essential Zinc-Dependent Deacetylase of Bacterial Lipid A Synthesis. Curr. Pharm. Biotechnol. 9 (1), 9–15. doi: 10.2174/138920108783497668
Becker, K. W., Skaar, E. P. (2014). Metal Limitation and Toxicity at the Interface Between Host and Pathogen. FEMS Microbiol. Rev. 38 (6), 1235–1249. doi: 10.1111/1574-6976.12087
Begum, N. A., Kobayashi, M., Moriwaki, Y., Matsumoto, M., Toyoshima, K., Seya, T. (2002). Mycobacterium Bovis BCG Cell Wall and Lipopolysaccharide Induce a Novel Gene, BIGM103, Encoding a 7-TM Protein: Identification of a New Protein Family Having Zn-Transporter and Zn-Metalloprotease Signatures. Genomics 80 (6), 630–645. doi: 10.1006/geno.2002.7000
Bera, A. K., Wu, R., Harrison, S., Cornelissen, C. N., Chazin, W. J., Noinaj, N. (2022). TdfH Selectively Binds Metal-Loaded Tetrameric Calprotectin for Zinc Import. Commun. Biol. 5 (1), 103. doi: 10.1038/s42003-022-03039-y
Berntsson, R. P. A., Smits, S. H. J., Schmitt, L., Slotboom, D.-J., Poolman, B. (2010). A Structural Classification of Substrate-Binding Proteins. FEBS Lett. 584 (12), 2606–2617. doi: 10.1016/j.febslet.2010.04.043
Blondiau, C., Lagadec, P., Lejeune, P., Onier, N., Cavaillon, J.-M., Jeannin, J.-F. (1994). Correlation Between the Capacity to Activate Macrophages In Vitro and the Antitumor Activity In Vivo of Lipopolysaccharides From Different Bacterial Species. Immunobiology 190 (3), 243–254. doi: 10.1016/S0171-2985(11)80272-X
Boulanger, M. J., Murphy, M. E. P. (2002). Crystal Structure of the Soluble Domain of the Major Anaerobically Induced Outer Membrane Protein (AniA) From Pathogenic Neisseria: A New Class of Copper-Containing Nitrite Reductases. J. Mol. Biol. 315 (5), 1111–1127. doi: 10.1006/jmbi.2001.5251
Braiterman, L. T., Gupta, A., Chaerkady, R., Cole, R. N., Hubbard, A. L. (2015). Communication Between the N and C Termini is Required for Copper-Stimulated Ser/Thr Phosphorylation of Cu(I)-ATPase (ATP7B). J. Biol. Chem. 290 (14), 8803–8819. doi: 10.1074/jbc.M114.627414
Brown, K. H., Wuehler, S. E., Peerson, J. M. (2001). The Importance of Zinc in Human Nutrition and Estimation of the Global Prevalence of Zinc Deficiency. Food Nutr. Bull. 22 (2), 113–125. doi: 10.1177/156482650102200201
Calmettes, C., Ing, C., Buckwalter, C. M., El Bakkouri, M., Chieh-Lin Lai, C., Pogoutse, A., et al. (2015). The Molecular Mechanism of Zinc Acquisition by the Neisserial Outer-Membrane Transporter ZnuD. Nat. Commun. 6 (1), 7996. doi: 10.1038/ncomms8996
Candrilli, S., Kurosky, S. (2019). The Response to and Cost of Meningococcal Disease Outbreaks in University Campus Settings: A Case Study in Oregon, United States. Res. Triangle Park (NC): RTI Press). doi: 10.3768/rtipress.2019.rr.0034.1910
Cash, D. R., Noinaj, N., Buchanan, S. K., Cornelissen, C. N. (2015). Beyond the Crystal Structure: Insight Into the Function and Vaccine Potential of TbpA Expressed by Neisseria Gonorrhoeae. Infect. Immun. 83 (11), 4438–4449. doi: 10.1128/IAI.00762-15
CDC. (2019a). Enhanced Meningococcal Disease Surveillance Report, 2018. Atlanta, GA: National Center for Immunization and Respiratory Diseases Office of Infectious Disease.
CDC. (2019b). Sexually transmitted disease surveillance 2018. Atlanta, GA: U.S. Department of Health and Human Services Centers for Disease Control and Prevention National Center for HIV/AIDS, Viral Hepatitis, STD, and TB Prevention Division of STD.
CDC. (2019c). VPD Surveillance Manual 8 Meningococcal Disease: Chapter 8.1. Atlanta, GA: National Center for Immunization and Respiratory Diseases Office of Infectious Disease.
Chandrangsu, P., Rensing, C., Helmann, J. D. (2017). Metal Homeostasis and Resistance in Bacteria. Nat. Rev. Microbiol. 15 (6), 338–350. doi: 10.1038/nrmicro.2017.15
Château, A., Seifert, H. S. (2016). Neisseria Gonorrhoeae Survives Within and Modulates Apoptosis and Inflammatory Cytokine Production of Human Macrophages. Cell. Microbiol. 18 (4), 546–560. doi: 10.1111/cmi.12529
Chen, C.-Y., Morse, S. A. (2001). Identification and Characterization of a High-Affinity Zinc Uptake System in Neisseria Gonorrhoeae. FEMS Microbiol. Lett. 202 (1), 67–71. doi: 10.1111/j.1574-6968.2001.tb10781.x
Chen, J. Y., Tsao, G. C., Zhao, Q., Zheng, W. (2001). Differential Cytotoxicity of Mn(II) and Mn(III): Special Reference to Mitochondrial [Fe-S] Containing Enzymes. Toxicol. Appl. Pharmacol. 175 (2), 160–168. doi: 10.1006/taap.2001.9245
Cichon, I., Ortmann, W., Bednarz, A., Lenartowicz, M., Kolaczkowska, E. (2020). Reduced Neutrophil Extracellular Trap (NET) Formation During Systemic Inflammation in Mice With Menkes Disease and Wilson Disease: Copper Requirement for NET Release. Front. Immunol. 10 (3021). doi: 10.3389/fimmu.2019.03021
Cornelissen, C. N., Sparling, P. F. (1996). Binding and Surface Exposure Characteristics of the Gonococcal Transferrin Receptor are Dependent on Both Transferrin-Binding Proteins. J. bacteriol. 178 (5), 1437–1444. doi: 10.1128/jb.178.5.1437-1444.1996
Davies, B. W., Walker, G. C. (2007). Disruption of sitA Compromises Sinorhizobium Meliloti for Manganese Uptake Required for Protection Against Oxidative Stress. J. Bacteriol. 189 (5), 2101. doi: 10.1128/JB.01377-06
Davis, L. M., Kakuda, T., DiRita, V. J. (2009). A Campylobacter Jejuni znuA Orthologue Is Essential for Growth in Low-Zinc Environments and Chick Colonization. J. Bacteriol. 191 (5), 1631. doi: 10.1128/JB.01394-08
Desrosiers, D. C., Bearden, S. W., Mier, I., Abney, J., Paulley, J. T., Fetherston, J. D., et al. (2010). Znu Is the Predominant Zinc Importer in Yersinia Pestis During In Vitro Growth But Is Not Essential for Virulence. Infect. Immun. 78 (12), 5163. doi: 10.1128/IAI.00732-10
Diaz-Ochoa, V. E., Lam, D., Lee, C. S., Klaus, S., Behnsen, J., Liu, J. Z., et al. (2016). Salmonella Mitigates Oxidative Stress and Thrives in the Inflamed Gut by Evading Calprotectin-Mediated Manganese Sequestration. Cell Host Microbe 19 (6), 814–825. doi: 10.1016/j.chom.2016.05.005
Dintilhac, A., Claverys, J. P. (1997). The Adc Locus, Which Affects Competence for Genetic Transformation in Streptococcus Pneumoniae, Encodes an ABC Transporter With a Putative Lipoprotein Homologous to a Family of Streptococcal Adhesins. Res. Microbiol. 148 (2), 119–131. doi: 10.1016/S0923-2508(97)87643-7
Djoko, K. Y., Franiek, J. A., Edwards, J. L., Falsetta, M. L., Kidd, S. P., Potter, A. J., et al. (2012). Phenotypic Characterization of a copA Mutant of Neisseria Gonorrhoeae Identifies a Link Between Copper and Nitrosative Stress. Infect. Immun. 80 (3), 1065–1071. doi: 10.1128/IAI.06163-11
Djoko, K. Y., McEwan, A. G. (2013). Antimicrobial Action of Copper Is Amplified via Inhibition of Heme Biosynthesis. ACS Chem. Biol. 8 (10), 2217–2223. doi: 10.1021/cb4002443
Dunn, M. A., Green, M. H., Leach, R. M., Jr (1991). Kinetics of Copper Metabolism in Rats: A Compartmental Model. Am. J. Physiol. 261 (1 Pt 1), E115–E125. doi: 10.1152/ajpendo.1991.261.1.E115
Edgeworth, J., Gorman, M., Bennett, R., Freemont, P., Hogg, N. (1991). Identification of P8,14 as a Highly Abundant Heterodimeric Calcium Binding Protein Complex of Myeloid Cells. J. Biol. Chem. 266 (12), 7706–7713. doi: 10.1016/S0021-9258(20)89506-4
Eijkelkamp, B. A., Morey, J. R., Ween, M. P., Ong, C.-l., McEwan, A. G., Paton, J. C., et al. (2014). Extracellular Zinc Competitively Inhibits Manganese Uptake and Compromises Oxidative Stress Management in Streptococcus Pneumoniae. PLos One 9 (2), e89427. doi: 10.1371/journal.pone.0089427
Fillat, M. F. (2014). The FUR (Ferric Uptake Regulator) Superfamily: Diversity and Versatility of Key Transcriptional Regulators. Arch. Biochem. Biophys. 546, 41–52. doi: 10.1016/j.abb.2014.01.029
Forbes, J. R., Gros, P. (2003). Iron, Manganese, and Cobalt Transport by Nramp1 (Slc11a1) and Nramp2 (Slc11a2) Expressed at the Plasma Membrane. Blood 102 (5), 1884–1892. doi: 10.1182/blood-2003-02-0425
Gagnon, D. M., Brophy, M. B., Bowman, S. E. J., Stich, T. A., Drennan, C. L., Britt, R. D., et al. (2015). Manganese Binding Properties of Human Calprotectin Under Conditions of High and Low Calcium: X-Ray Crystallographic and Advanced Electron Paramagnetic Resonance Spectroscopic Analysis. J. Am. Chem. Soc. 137 (8), 3004–3016. doi: 10.1021/ja512204s
Ganini, D., Santos, J. H., Bonini, M. G., Mason, R. P. (2018). Switch of Mitochondrial Superoxide Dismutase Into a Prooxidant Peroxidase in Manganese-Deficient Cells and Mice. Cell Chem. Biol. 25 (4), 413–425.e416. doi: 10.1016/j.chembiol.2018.01.007
Girotto, S., Cendron, L., Bisaglia, M., Tessari, I., Mammi, S., Zanotti, G., et al. (2014). DJ-1 is a Copper Chaperone Acting on SOD1 Activation. J. Biol. Chem. 289 (15), 10887–10899. doi: 10.1074/jbc.M113.535112
Gotschlich, E. C., Seiff, M. E. (1987). Identification and Gene Structure of an Azurin-Like Protein With a Lipoprotein Signal Peptide in Neisseria Gonorrhoeae. FEMS Microbiol. Lett. 43 (3), 253–255. doi: 10.1111/j.1574-6968.1987.tb02153.x
Gunawan, J., Simard, D., Gilbert, M., Lovering, A. L., Wakarchuk, W. W., Tanner, M. E., et al. (2005). Structural and Mechanistic Analysis of Sialic Acid Synthase NeuB From Neisseria Meningitidis in Complex With Mn2+, Phosphoenolpyruvate, and N-Acetylmannosaminitol. J. Biol. Chem. 280 (5), 3555–3563. doi: 10.1074/jbc.M411942200
Gunter, T. E., Gerstner, B., Gunter, K. K., Malecki, J., Gelein, R., Valentine, W. M., et al. (2013). Manganese Transport via the Transferrin Mechanism. Neurotoxicology 34, 118–127. doi: 10.1016/j.neuro.2012.10.018
Hecel, A., Rowińska-Żyrek, M., Kozłowski, H. (2019). Copper(II)-Induced Restructuring of ZnuD, A Zinc(II) Transporter From Neisseria Meningitidis. Inorganic Chem. 58 (9), 5932–5942. doi: 10.1021/acs.inorgchem.9b00265
Hecel, A., Watly, J., Rowinska-Zyrek, M., Swiatek-Kozlowska, J., Kozlowski, H. (2018). Histidine Tracts in Human Transcription Factors: Insight Into Metal Ion Coordination Ability. JBIC J. Biol. Inorg. Chem. 23 (1), 81. doi: 10.1007/s00775-017-1512-x
Hennigar, S. R., McClung, J. P. (2016). Nutritional Immunity: Starving Pathogens of Trace Minerals. Am. J. lifestyle Med. 10 (3), 170–173. doi: 10.1177/1559827616629117
Hill, D. R., Brunner, M. E., Schmitz, D. C., Davis, C. C., Flood, J. A., Schlievert, P. M., et al. (2005). In Vivo Assessment of Human Vaginal Oxygen and Carbon Dioxide Levels During and Post Menses. J. Appl. Physiol. 99 (4), 1582–1591. doi: 10.1152/japplphysiol.01422.2004
Hood, M. I., Mortensen, B. L., Moore, J. L., Zhang, Y., Kehl-Fie, T. E., Sugitani, N., et al. (2012). Identification of an Acinetobacter Baumannii Zinc Acquisition System That Facilitates Resistance to Calprotectin-Mediated Zinc Sequestration. PLos Pathog. 8 (12), e1003068. doi: 10.1371/journal.ppat.1003068
Hu Frisk, J. M., Kjellén, L., Kaler, S. G., Pejler, G., Öhrvik, H. (2017). Copper Regulates Maturation and Expression of an MITF:Tryptase Axis in Mast Cells. J. Immunol. 199 (12), 4132–4141. doi: 10.4049/jimmunol.1700786
Hyre, A. N., Kavanagh, K., Kock, N. D., Donati, G. L., Subashchandrabose, S. (2017). Copper Is a Host Effector Mobilized to Urine During Urinary Tract Infection To Impair Bacterial Colonization. Infect. Immun. 85 (3), e01041–e01016. doi: 10.1128/IAI.01041-16
Iovine, N. M., Pursnani, S., Voldman, A., Wasserman, G., Blaser, M. J., Weinrauch, Y. (2008). Reactive Nitrogen Species Contribute to Innate Host Defense Against Campylobacter Jejuni. Infect. Immun. 76 (3), 986. doi: 10.1128/IAI.01063-07
Ivanov, S. S., Castore, R., Juarez Rodriguez, M. D., Circu, M., Dragoi, A.-M. (2022). Neisseria Gonorrhoeae Subverts Formin-Dependent Actin Polymerization to Colonize Human Macrophages. PLos Pathog. 17 (12), e1010184. doi: 10.1371/journal.ppat.1010184
Jabado, N., Jankowski, A., Dougaparsad, S., Picard, V., Grinstein, S., Gros, P. (2000). Natural Resistance to Intracellular Infections: Natural Resistance-Associated Macrophage Protein 1 (Nramp1) Functions as a pH-Dependent Manganese Transporter at the Phagosomal Membrane. J. Exp. Med. 192 (9), 1237–1248. doi: 10.1084/jem.192.9.1237
Jackson, M. J. (1989). "Physiology of Zinc: General Aspects," in Zinc in Human Biology, Ed (C.F. Mills: London: Springer London), 1–14.
Jacobsen, F. E., Kazmierczak, K. M., Lisher, J. P., Winkler, M. E., Giedroc, D. P. (2011). Interplay Between Manganese and Zinc Homeostasis in the Human Pathogen Streptococcus Pneumoniae. Metallomics 3 (1), 38–41. doi: 10.1039/c0mt00050g
Jarosz, M., Olbert, M., Wyszogrodzka, G., Młyniec, K., Librowski, T. (2017). Antioxidant and Anti-Inflammatory Effects of Zinc. Zinc-Dependent NF-κb Signaling. Inflammopharmacology 25 (1), 11–24. doi: 10.1007/s10787-017-0309-4
Jean, S. (2015). Characterizarion of the Regulation and Function of Neisseria Gonorrhoeae TonB-Dependent Transporters: TdfG, TdfH and TdfJ (Virginia Commonwealth University: Doctor of Philosophy).
Jean, S., Juneau, R. A., Criss, A. K., Cornelissen, C. N. (2016). Neisseria Gonorrhoeae Evades Calprotectin-Mediated Nutritional Immunity and Survives Neutrophil Extracellular Traps by Production of TdfH. Infect. Immun. 84 (10), 2982–2994. doi: 10.1128/IAI.00319-16
John, C. M., Feng, D., Jarvis, G. A. (2018). Treatment of Human Challenge and MDR Strains of Neisseria Gonorrhoeae With LpxC Inhibitors. J. Antimicrob. Chemother. 73 (8), 2064–2071. doi: 10.1093/jac/dky151
Johnson, M. B., Criss, A. K. (2011). Resistance of Neisseria Gonorrhoeae to Neutrophils. Front. Microbiol. 2. doi: 10.3389/fmicb.2011.00077
Juttukonda, L. J., Chazin, W. J., Skaar, E. P. (2016). Acinetobacter Baumannii Coordinates Urea Metabolism With Metal Import To Resist Host-Mediated Metal Limitation. mBio 7 (5), e01475–e01416. doi: 10.1128/mBio.01475-16
Kammerman, M. T., Bera, A., Wu, R., Harrison, S. A., Maxwell, C. N., Lundquist, K., et al. (2020). Molecular Insight Into TdfH-Mediated Zinc Piracy From Human Calprotectin by Neisseria Gonorrhoeae. mBio 11 (3), e00949–e00920. doi: 10.1128/mBio.00949-20
Kandler, J. L., Holley, C. L., Reimche, J. L., Dhulipala, V., Balthazar, J. T., Muszyński, A., et al. (2016). The MisR Response Regulator Is Necessary for Intrinsic Cationic Antimicrobial Peptide and Aminoglycoside Resistance in Neisseria Gonorrhoeae. Antimicrob. Agents chemother. 60 (8), 4690–4700. doi: 10.1128/AAC.00823-16
Kaur, G., Kumar, V., Arora, A., Tomar, A., Sur, R., Dutta, D., et al. (2017). Affected Energy Metabolism Under Manganese Stress Governs Cellular Toxicity. Sci. Rep. 7 (1), 11645–11645. doi: 10.1038/s41598-017-12004-3
Kehres, D. G., Janakiraman, A., Slauch, J. M., Maguire, M. E. (2002a). Regulation of Salmonella Enterica Serovar Typhimurium mntH Transcription by H2O2, Fe2+, and Mn2+. J. Bacteriol. 184 (12), 3151–3158. doi: 10.1128/jb.184.12.3151-3158.2002
Kehres, D. G., Janakiraman, A., Slauch, J. M., Maguire, M. E. (2002b). SitABCD is the Alkaline Mn2+ Transporter of Salmonella Enterica Serovar Typhimurium. J. bacteriol. 184 (12), 3159–3166. doi: 10.1128/jb.184.12.3159-3166.2002
Knapp, S., de Vos Alex, F., Florquin, S., Golenbock Douglas, T., van der Poll, T. (2003). Lipopolysaccharide Binding Protein Is an Essential Component of the Innate Immune Response to Escherichia Coli Peritonitis in Mice. Infect. Immun. 71 (12), 6747–6753. doi: 10.1128/IAI.71.12.6747-6753.2003
Kumar, S., Chesson, H., Gift, T. L. (2021). Estimating the Direct Medical Costs and Productivity Loss of Outpatient Chlamydia and Gonorrhea Treatment. Sex Transm. Dis. 48 (2), e18–e21. doi: 10.1097/olq.0000000000001240
Lee, M.-J., Chien-Liang, L., Tsai, J.-Y., Sue, W.-T., Hsia, W.-S., Huang, H. (2010). Identification and Biochemical Characterization of a Unique Mn2+-Dependent UMP Kinase From Helicobacter Pylori. Arch. Microbiol. 192 (9), 739–746. doi: 10.1007/s00203-010-0600-x
Lefrançois, L. H., Kalinina, V., Cardenal-Muñoz, E., Hanna, N., Koliwer-Brandl, H., Appiah, J., et al. (2019). Zn2+ Intoxication of Mycobacterium Marinum During Dictyostelium Discoideum Infection Is Counteracted by Induction of the Pathogen Zn2+ Exporter CtpC. bioRxiv 575217. doi: 10.1101/575217
Lewis, D. A., Klesney-Tait, J., Lumbley, S. R., Ward, C. K., Latimer, J. L., Ison, C. A., et al. (1999). Identification of the znuA-Encoded Periplasmic Zinc Transport Protein of Haemophilus Ducreyi. Infect. Immun. 67 (10), 5060–5068. doi: 10.1128/IAI.67.10.5060-5068.1999
Lim, K. H. L., Jones, C. E., vanden Hoven, R. N., Edwards, J. L., Falsetta, M. L., Apicella, M. A., et al. (2008). Metal Binding Specificity of the MntABC Permease of Neisseria Gonorrhoeae and Its Influence on Bacterial Growth and Interaction With Cervical Epithelial Cells. Am. Soc. Microbiol. J. Infect. Immun. doi: 10.1128/IAI.01725-07
Liu, Y., Russell, M. W. (2011). Diversion of the Immune Response to Neisseria Gonorrhoeae From Th17 to Th1/Th2 by Treatment With Anti-Transforming Growth Factor β Antibody Generates Immunological Memory and Protective Immunity. mBio 2 (3), e00095–e00011. doi: 10.1128/mBio.00095-11
Maares, M., Keil, C., Koza, J., Straubing, S., Schwerdtle, T., Haase, H. (2018). In Vitro Studies on Zinc Binding and Buffering by Intestinal Mucins. Int. J. Mol. Sci. 19 (9), 2662. doi: 10.3390/ijms19092662
Mandrell, R. E., Griffiss, J. M., Macher, B. A. (1988). Lipooligosaccharides (LOS) of Neisseria Gonorrhoeae and Neisseria Meningitidis Have Components That are Immunochemically Similar to Precursors of Human Blood Group Antigens. Carbohydrate Sequence Specificity of the Mouse Monoclonal Antibodies That Recognize Crossreacting Antigens on LOS and Human Erythrocytes. J. Exp. Med. 168 (1), 107–126. doi: 10.1084/jem.168.1.107
Maunders Eve, A., Ganio, K., Hayes Andrew, J., Neville Stephanie, L., Davies Mark, R., Strugnell Richard, A., et al. (2022). The Role of ZntA in Klebsiella Pneumoniae Zinc Homeostasis. Microbiol. Spectr. 10 (1), e01773–e01721. doi: 10.1128/spectrum.01773-21
Maurakis, S., Keller, K., Maxwell, C. N., Pereira, K., Chazin, W. J., Criss, A. K., et al. (2019). The Novel Interaction Between Neisseria Gonorrhoeae TdfJ and Human S100A7 Allows Gonococci to Subvert Host Zinc Restriction. PLos Pathog. 15 (8), e1007937–e1007937. doi: 10.1371/journal.ppat.1007937
Maynard, L. S., Cotzias, G. C. (1955). The Partition of Manganese Among Organs and Intracellular Organelles of the Rat. J. Biol. Chem. 214 (1), 489–495. doi: 10.1016/S0021-9258(18)70986-1
McDevitt, C. A., Ogunniyi, A. D., Valkov, E., Lawrence, M. C., Kobe, B., McEwan, A. G., et al. (2011). A Molecular Mechanism for Bacterial Susceptibility to Zinc. PLos Pathog. 7 (11), e1002357–e1002357. doi: 10.1371/journal.ppat.1002357
Mellies, J., Jose, J., Meyer, T. F. (1997). The Neisseria Gonorrhoeae Gene aniA Encodes an Inducible Nitrite Reductase. Mol. Gen. Genet. 256 (5), 525–532. doi: 10.1007/s004380050597
Mildner, M., Stichenwirth, M., Abtin, A., Eckhart, L., Sam, C., Gläser, R., et al. (2010). Psoriasin (S100A7) is a Major Escherichia Coli-Cidal Factor of the Female Genital Tract. Mucosal Immunol. 3 (6), 602–609. doi: 10.1038/mi.2010.37
Mochalkin, I., Knafels, J. D., Lightle, S. (2008). Crystal Structure of LpxC From Pseudomonas Aeruginosa Complexed With the Potent BB-78485 Inhibitor. Protein Sci. 17 (3), 450–457. doi: 10.1110/ps.073324108
Noto, J. M., Cornelissen, C. N. (2008). Identification of TbpA Residues Required for Transferrin-Iron Utilization by Neisseria Gonorrhoeae. Infect. Immun. 76 (5), 1960–1969. doi: 10.1128/IAI.00020-08
Novoa-Aponte, L., Xu, C., Soncini, F. C., Argüello, J. M. (2020). The Two-Component System CopRS Maintains Subfemtomolar Levels of Free Copper in the Periplasm of Pseudomonas Aeruginosa Using a Phosphatase-Based Mechanism. mSphere 5 (6), e01193–e01120. doi: 10.1128/mSphere.01193-20
O'Neal, S. L., Zheng, W. (2015). Manganese Toxicity Upon Overexposure: A Decade in Review. Curr. Environ. Health Rep. 2 (3), 315–328. doi: 10.1007/s40572-015-0056-x
Odugbemi, T., McEntegart, M., Hafiz, S. (1978). Effects of Various Divalent Cations on the Survival of Neisseria Gonorrhoeae in Liquid Media. Br. J. Vener Dis. 54 (4), 239–242. doi: 10.1136/sti.54.4.239
Osaki, S., Johnson, D. A., Frieden, E. (1966). The Possible Significance of the Ferrous Oxidase Activity of Ceruloplasmin in Normal Human Serum. J. Biol. Chem. 241 (12), 2746–2751. doi: 10.1016/S0021-9258(18)96527-0
Pawlik, M.-C., Hubert, K., Joseph, B., Claus, H., Schoen, C., Vogel, U. (2012). The Zinc-Responsive Regulon of Neisseria Meningitidis Comprises 17 Genes Under Control of a Zur Element. J. Bacteriol. 194 (23), 6594 LP–6603. doi: 10.1128/JB.01091-12
Pederick, V. G., Eijkelkamp, B. A., Begg, S. L., Ween, M. P., McAllister, L. J., Paton, J. C., et al. (2015). ZnuA and Zinc Homeostasis in Pseudomonas Aeruginosa. Sci. Rep. 5 (1), 13139. doi: 10.1038/srep13139
Perrin, A., Bonacorsi, S., Carbonnelle, E., Talibi, D., Dessen, P., Nassif, X., et al. (2002). Comparative Genomics Identifies the Genetic Islands That Distinguish Neisseria Meningitidis, the Agent of Cerebrospinal Meningitis, From Other Neisseria Species. Infect. Immun. 70 (12), 7063. doi: 10.1128/IAI.70.12.7063-7072.2002
Persson, K., Ly, H. D., Dieckelmann, M., Wakarchuk, W. W., Withers, S. G., Strynadka, N. C. (2001). Crystal Structure of the Retaining Galactosyltransferase LgtC From Neisseria Meningitidis in Complex With Donor and Acceptor Sugar Analogs. Nat. Struct. Biol. 8 (2), 166–175. doi: 10.1038/84168
Putker, F., Grutsch, A., Tommassen, J., Bos, M. P. (2014). Ght Protein of Neisseria Meningitidis Is Involved in the Regulation of Lipopolysaccharide Biosynthesis. J. Bacteriol. 196 (4), 780. doi: 10.1128/JB.00943-13
Quillin, S., Seifert, H. (2018). Neisseria Gonorrhoeae Host Adaptation and Pathogenesis. Springer Nat. 16, 226–240. doi: 10.1038/nrmicro.2017.169
Quintana, J., Novoa-Aponte, L., Argüello, J. M. (2017). Copper Homeostasis Networks in the Bacterium Pseudomonas Aeruginosa. J. Biol. Chem. 292 (38), 15691–15704. doi: 10.1074/jbc.M117.804492
Rahman, M. T., Karim, M. M. (2018). Metallothionein: A Potential Link in the Regulation of Zinc in Nutritional Immunity. Biol. Trace Element Res. 182 (1), 1–13. doi: 10.1007/s12011-017-1061-8
Ramos, D., Mar, D., Ishida, M., Vargas, R., Gaite, M., Montgomery, A., et al. (2016). Mechanism of Copper Uptake From Blood Plasma Ceruloplasmin by Mammalian Cells. PLos One 11 (3), e0149516–e0149516. doi: 10.1371/journal.pone.0149516
Ronpirin, C., Jerse, A. E., Cornelissen, C. N. (2001). Gonococcal Genes Encoding Transferrin-Binding Proteins A and B are Arranged in a Bicistronic Operon But are Subject to Differential Expression. Infect. Immun. 69 (10), 6336–6347. doi: 10.1128/IAI.69.10.6336-6347.2001
Seib, K. L., Tseng, H.-J., McEwan, A. G., Apicella, M. A., Jennings, M. P. (2004). Defenses Against Oxidative Stress in Neisseria Gonorrhoeae and Neisseria Meningitidis: Distinctive Systems for Different Lifestyles. J. Infect. Dis. 190 (1), 136–147. doi: 10.1086/421299
Seib, K. L., Wu, H.-J., Kidd, S. P., Apicella, M. A., Jennings, M. P., McEwan, A. G. (2006). Defenses Against Oxidative Stress in Neisseria Gonorrhoeae: A System Tailored for a Challenging Environment. Microbiol. Mol. Biol. Rev. 70 (2), 344–361. doi: 10.1128/MMBR.00044-05
Sintsova, A., Sarantis, H., Islam, E. A., Sun, C. X., Amin, M., Chan, C. H. F., et al. (2014). Global Analysis of Neutrophil Responses to Neisseria Gonorrhoeae Reveals a Self-Propagating Inflammatory Program. PLos Pathog. 10 (9), e1004341–e1004341. doi: 10.1371/journal.ppat.1004341
Stafford, S. L., Bokil, N. J., Achard, M. E. S., Kapetanovic, R., Schembri, M. A., McEwan, A. G., et al. (2013). Metal Ions in Macrophage Antimicrobial Pathways: Emerging Roles for Zinc and Copper. Biosci. Rep. 33 (4). doi: 10.1042/BSR20130014
Stähler, F. N., Odenbreit, S., Haas, R., Wilrich, J., Van Vliet, A. H. M., Kusters, J. G., et al. (2006). The Novel Helicobacter Pylori CznABC Metal Efflux Pump Is Required for Cadmium, Zinc, and Nickel Resistance, Urease Modulation, and Gastric Colonization. Infect. Immun. 74 (7), 3845–3852. doi: 10.1128/IAI.02025-05
Steimle, A., Autenrieth, I. B., Frick, J.-S. (2016). Structure and Function: Lipid A Modifications in Commensals and Pathogens. Int. J. Med. Microbiol. 306 (5), 290–301. doi: 10.1016/j.ijmm.2016.03.001
Stork, M., Bos, M. P., Jongerius, I., de Kok, N., Schilders, I., Weynants, V. E., et al. (2010). An Outer Membrane Receptor of Neisseria Meningitidis Involved in Zinc Acquisition With Vaccine Potential. PLos Pathog. 6 (7), e1000969. doi: 10.1371/journal.ppat.1000969
Stork, M., Grijpstra, J., Bos, M. P., Mañas Torres, C., Devos, N., Poolman, J. T., et al. (2013). Zinc Piracy as a Mechanism of Neisseria Meningitidis for Evasion of Nutritional Immunity. PLos Pathog. 9 (10), e1003733–e1003733. doi: 10.1371/journal.ppat.1003733
Suzuki, T., Suzuki, K., Nakajima, K., Otaki, N., Yamanaka, H. (1994). Metallothionein in Human Seminal Plasma. Int. J. Urol. 1 (4), 345–348. doi: 10.1111/j.1442-2042.1994.tb00062.x
Suzuki, T., Yamanaka, H., Nakajima, K., Suzuki, K., Kanatani, K., Kimura, M., et al. (1992). Immunohistochemical Study of Metallothionein in Human Seminal Vesicles. Tohoku J. Exp. Med. 167 (2), 127–134. doi: 10.1620/tjem.167.127
Taylor-Robinson, D., Furr, P. M., Hetherington, C. M. (1990). Neisseria Gonorrhoeae Colonises the Genital Tract of Oestradiol-Treated Germ-Free Female Mice. Microbial. Pathogen. 9 (5), 369–373. doi: 10.1016/0882-4010(90)90071-W
Tidball, A. M., Bryan, M. R., Uhouse, M. A., Kumar, K. K., Aboud, A. A., Feist, J. E., et al. (2015). A Novel Manganese-Dependent ATM-P53 Signaling Pathway is Selectively Impaired in Patient-Based Neuroprogenitor and Murine Striatal Models of Huntington's Disease. Hum. Mol. Genet. 24 (7), 1929–1944. doi: 10.1093/hmg/ddu609
Tinsley, C. R., Nassif, X. (1996). Analysis of the Genetic Differences Between Neisseria Meningitidis and Neisseria Gonorrhoeae: Two Closely Related Bacteria Expressing Two Different Pathogenicities. Proc. Natl. Acad. Sci. U. S. A. 93 (20), 11109–11114. doi: 10.1073/pnas.93.20.11109
Tseng, H. J., Srikhanta, Y., McEwan, A. G., Jennings, M. P. (2001). Accumulation of Manganese in Neisseria Gonorrhoeae Correlates With Resistance to Oxidative Killing by Superoxide Anion and is Independent of Superoxide Dismutase Activity. Mol. Biol. 40 (5), 1175–1186. doi: 10.1046/j.1365-2958.2001.02460.x
Urban, C. F., Ermert, D., Schmid, M., Abu-Abed, U., Goosmann, C., Nacken, W., et al. (2009). Neutrophil Extracellular Traps Contain Calprotectin, a Cytosolic Protein Complex Involved in Host Defense Against Candida Albicans. PLos Pathog. 5 (10), e1000639. doi: 10.1371/journal.ppat.1000639
van Emmerik, L. C., Kuijper, E. J., Fijen, C. A., Dankert, J., Thiel, S. (1994). Binding of Mannan-Binding Protein to Various Bacterial Pathogens of Meningitis. Clin. Exp. Immunol. 97 (3), 411–416. doi: 10.1111/j.1365-2249.1994.tb06103.x
Vélez Acevedo, R. N., Ronpirin, C., Kandler, J. L., Shafer, W. M., Cornelissen, C. N. (2014). Identification of Regulatory Elements That Control Expression of the tbpBA Operon in Neisseria Gonorrhoeae. J. Bacteriol. 196 (15), 2762–2774. doi: 10.1128/JB.01693-14
Veyrier, F. J., Boneca, I. G., Cellier, M. F., Taha, M.-K. (2011). A Novel Metal Transporter Mediating Manganese Export (MntX) Regulates the Mn to Fe Intracellular Ratio and Neisseria Meningitidis Virulence. PLos Pathog. 7 (9), e1002261–e1002261. doi: 10.1371/journal.ppat.1002261
Voganatsi, A., Panyutich, A., Miyasaki, K. T., Murthy, R. K. (2001). Mechanism of Extracellular Release of Human Neutrophil Calprotectin Complex. J. Leukocyte Biol. 70 (1), 130–134. doi: 10.1189/jlb.70.1.130
Wagner, D., Maser, J., Lai, B., Cai, Z., Barry, C. E., Höner zu Bentrup, K., et al. (2005). Elemental Analysis of Mycobacterium Avium, Mycobacterium Tuberculosis, and Mycobacterium Smegmatis Containing Phagosomes Indicates Pathogen-Induced Microenvironments Within the Host Cell’s Endosomal System. J. Immunol. 174 (3), 1491. doi: 10.4049/jimmunol.174.3.1491
White, C., Lee, J., Kambe, T., Fritsche, K., Petris, M. J. (2009). A Role for the ATP7A Copper-Transporting ATPase in Macrophage Bactericidal Activity. J. Biol. Chem. 284 (49), 33949–33956. doi: 10.1074/jbc.M109.070201
Woods, J. P., Dempsey, J. F., Kawula, T. H., Barritt, D. S., Cannon, J. G. (1989). Characterization of the Neisserial Lipid-Modified Azurin Bearing the H.8 Epitope. Mol. Microbiol. 3 (5), 583–591. doi: 10.1111/j.1365-2958.1989.tb00205.x
Wu, H.-J., Seib, K. L., Srikhanta, Y. N., Edwards, J., Kidd, S. P., Maguire, T. L., et al. (2010). Manganese Regulation of Virulence Factors and Oxidative Stress Resistance in Neisseria Gonorrhoeae. J. Proteomics 73 (5), 899–916. doi: 10.1016/j.jprot.2009.12.001
Wu, H.-J., Seib, K., Srikhanta, Y., Kidd, S., Edwards, J., Maguire, T., et al. (2006). PerR Controls Mn-Dependent Resistance to Oxidative Stress in Neisseria Gonorrhoeae. Mol. Biol. 60 (2), 401–416. doi: 10.1111/j.1365-2958.2006.05079.x
Wu, H., Soler-García, A. A., Jerse, A. E. (2009). A Strain-Specific Catalase Mutation and Mutation of the Metal-Binding Transporter Gene mntC Attenuate Neisseria Gonorrhoeae In Vivo But Not by Increasing Susceptibility to Oxidative Killing by Phagocytes. Infect. Immun. 77 (3), 1091–1102. doi: 10.1128/IAI.00825-08
Yadav, R., Noinaj, N., Ostan, N., Moraes, T., Stoudenmire, J., Maurakis, S., et al. (2020). Structural Basis for Evasion of Nutritional Immunity by the Pathogenic Neisseriae. Front. Microbiol. 10. doi: 10.3389/fmicb.2019.02981
Zackular, J. P., Chazin, W. J., Skaar, E. P. (2015). Nutritional Immunity: S100 Proteins at the Host-Pathogen Interface. J. Biol. Chem. 290 (31), 18991–18998. doi: 10.1074/jbc.R115.645085
Zhou, B., Gitschier, J. (1997). Hctr1: A Human Gene for Copper Uptake Identified by Complementation in Yeast. Proc. Natl. Acad. Sci. 94 (14), 7481. doi: 10.1073/pnas.94.14.7481
Keywords: metal intoxication, nutritional immunity, Neisseria gonorrhoeae, Neisseria meningitidis, zinc, manganese, copper, integrated metal homeostasis
Citation: Branch AH, Stoudenmire JL, Seib KL and Cornelissen CN (2022) Acclimation to Nutritional Immunity and Metal Intoxication Requires Zinc, Manganese, and Copper Homeostasis in the Pathogenic Neisseriae. Front. Cell. Infect. Microbiol. 12:909888. doi: 10.3389/fcimb.2022.909888
Received: 31 March 2022; Accepted: 18 May 2022;
Published: 30 June 2022.
Edited by:
Mauricio H. Pontes, The Pennsylvania State University, United StatesReviewed by:
Michael D. L. Johnson, University of Arizona, United StatesJacob P. Bitoun, Tulane University, United States
Copyright © 2022 Branch, Stoudenmire, Seib and Cornelissen. This is an open-access article distributed under the terms of the Creative Commons Attribution License (CC BY). The use, distribution or reproduction in other forums is permitted, provided the original author(s) and the copyright owner(s) are credited and that the original publication in this journal is cited, in accordance with accepted academic practice. No use, distribution or reproduction is permitted which does not comply with these terms.
*Correspondence: Cynthia Nau Cornelissen, ccornelissen@gsu.edu