- 1Department of Colorectal Surgery, Zhejiang Provincial People's Hospital, Affiliated People's Hospital of Hangzhou Medical College, Hangzhou, Zhejiang, China
- 2The Second Affiliated College of Zhejiang Chinese Medical University, Hangzhou, Zhejiang, China
It is well-established that there are trillions of gut microbiota (GM) in the human gut. GM and its metabolites can reportedly cause cancer by causing abnormal immune responses. With the development of sequencing technology and the application of germ-free models in recent years, significant inroads have been achieved in research on GM and microbiota-related metabolites. Accordingly, the role and mechanism of GM in colorectal cancer (CRC) development have been gradually revealed. Traditional Chinese medicine (TCM) represents an important source of natural medicines and herbal products, with huge potential as anti-CRC agents. The potential application of TCM to target gut microbes for the treatment of colorectal cancer represents an exciting area of investigation.
Introduction
Colorectal cancer (CRC) results from malignant transformation of intestinal epithelial mucosa mediated by various pathogenic factors. With lifestyle changes associated with the fast pace of living nowadays, the morbidity and mortality rates attributed to CRC have been increasing worldwide. Although the exact cause of CRC remains unclear, CRC development and progression have been linked to inheritance, immunity, environment, dietary habits, and lifestyle. Studies have shown that obesity, smoking and depression are risk factors for the development of CRC. In addition, individuals with inflammatory bowel disease (IBD) are associated with an increased incidence of colitis-associated cancer (CAC). Chronic inflammation is considered to be an essential initiating factor for the occurrence of CRC. The homeostasis of the intestinal microenvironment is essential to maintain the normal function of intestinal epithelial mucosa and inhibit the occurrence and development of CRC. Therefore, exploring the abnormal molecular mechanisms in the microenvironment of CRC and finding effective therapeutic targets are significant for preventing and treating this patient population.
With the rapid development and wide application of microbiota sequencing technology and metabolomics technology, researchers can detect microbes and their metabolites in the gut to understand the distribution of microbiota and the changes of metabolites in the gut microenvironment. Accordingly, we have gained a deeper understanding of gut microbiota. Importantly, the gut microbiota and its metabolites play a key role in maintaining intestinal homeostasis and inhibiting the occurrence of CRC. According to a recent study, the intestinal microbiota might act as the driving factor for the development of intestinal cancer. A “driver-passenger” pattern was also proposed, whereby the original intestinal bacteria (driving factor) attack the intestine, leading to DNA damage to cause CRC. Next, tumors cause changes in the intestinal microecology, which is conducive to the proliferation of conditioned bacteria (bacterial passengers) (Marchesi et al., 2016). A study transplanted gut microbiota (GM) from CRC patients and healthy humans into antibiotic-treated mice, and the mice were molded with azoxymethane (AOM). The results showed that significantly higher proportions of conventional mice that were fed stools from CRC individuals developed high-grade dysplasia (P<0.05) and macroscopic polyps (P<0.01) compared to mice fed stools from healthy controls. In addition, real-time Polymerase Chain Reaction (PCR) revealed the upregulation of genes involved in cell proliferation, stemness, apoptosis, angiogenesis, invasiveness, and metastasis in mice fed stools from CRC patients. Recent data suggest that in addition to the GM itself, its metabolites, such as short-chain fatty acids (SCFAs) such as acetate, propionate, and butyrate, can suppress inflammation and cancer, while secondary bile acids promote cancer. Dietary intake yields an important effect on the gut environment, much of which is mediated by the metabolic activities of the intestinal microbiota on dietary compounds. Indeed, different microbial metabolites can potentially promote and protect against CRC.
Herbal medicine has been in use for thousands of years in China and reportedly exerts a good therapeutic effect on many diseases via its multi-targeting properties. Although the effectiveness of herbal medicine has been established, the mechanism underlying its effects on the human body remains unclear. Orally administered herbal medicine decoction can interact with the GM and play an important role in maintaining intestinal barrier integrity and intestinal microenvironment homeostasis. Herbal medicine is widely thought to have beneficial biogenic effects on GM, playing an important therapeutic role by regulating the population, distribution and metabolites of intestinal microbiota to promote its probiotic function. The potential value of TCM in CRC warrants further investigation. In this review, we aimed to provide a plausible mechanistic basis for the interactions between herbal medicines and GM and the therapeutic benefits of this interaction in CRC.
Relationship between GM and CRC
It is estimated that the total amount of GM genes is 100 times that of human genes (Komaroff, 2018). GM serves several functions in host metabolism, such as promoting immunity and protecting the intestinal barrier (Shin and Kim, 2018; Bender, 2020). Intestinal dysbiosis reduces the function of the intestinal mucosal barrier and enhances bacterial translocation, leading to inflammation and infection. Since the 1970s, germ-free mice have been used to demonstrate that alterations in the gut microbiota play an important role in the development of CRC (Reddy et al., 1976; Drewes et al., 2022; Zhao et al., 2022). Recent studies have shown that GM is a critical environmental factor in regulating body metabolism, contributing to the occurrence and development of CRC. During the early stages of CRC, the microbiota is characterized by decreased abundance of commensal bacteria that produce anti-inflammatory SCFAs, such as Lactobacillus, Bifidobacterium, and Clostridium, and markers of intestinal epithelial damage (such as diamine oxide, D-lactic acid and LPS). In addition, studies have shown that Fusobacterium nucleatum (F. nucleatum) and Escherichia coli (Escherichia coli pks+) are positively correlated with metabolites such as diamine oxide, D-lactic acid, and LPS (Liu et al., 2020).
Alterations in GM in CRC can be divided into three different patterns. in that first model, the abundance of some pro-inflammatory bacteria, such as Fusobacterium nucleatum, Peptostreptococcus anaerobius and Peptostreptococcus stomatis, increase continuously from stage 0 to progressive stage. In CRC stage I/II and stage III/IV, Parvimonas micra., P. anaerobius, P. stomatis, and P. micra, while their levels decreased after tumor resection, suggesting that these species may simply adapt to the cancer microenvironment but not participate in the occurrence of CRC. In the second pattern, the abundance of Atopobium parvulum and Actinomyces odontolyticus increased significantly in stage 0 of multiple polypoid adenomas, but did not increase in advanced and advanced stages. The third pattern, anti-inflammatory micro-organisms or certain probiotics, such as butyrate-producing bacteria (Lachnospira multipara and Eubacterium eligens) and Bifidobacterium longum, decreases as CRC progresses. The changes of intestinal microbiota composition in different stages of CRC are also different.
Potential causative bacteria and their metabolic pathways in CRC
Following the discovery that Helicobacter pylori increase the risk of gastric cancer (Caruso and Fucci, 1990), other species colonizing the gastrointestinal tract have been investigated for potential cancer-inducing properties. In a recent study, germ-free mice and conventional mice with AOM were fed stool samples from patients with CRC and healthy individuals. It was found that stool from patients with CRC increased the number of polyps, intestinal dysplasia and proliferation, markers of inflammation, and proportions of Th1 and Th17 cells in the colon, compared with stool from individuals without CRC. This study provides evidence that the fecal microbiota from patients with CRC can promote tumorigenesis in germ-free mice and mice (Wong et al., 2017).
Escherichia coli is an opportunistic pathogen that participates in the digestion and synthesis of vitamin K2 under normal circumstances but becomes pathogenic when the body’s immunity declines. Strains of Escherichia coli (E. coli) have been identified as a potential risk factor for CRC. E. coli was found to colonize cancer lesions and neighboring epithelium, suggesting they are the only cultivable organisms in close contact with the diseased site. Analysis of mucosa-associated E. coli from colon cancer showed that 66% of biopsies from CRC were positive for E. coli strains by bacterial culture and B2 E. coli strain 11G5 isolated from colon cancer can persist in the gut, and induce colon inflammation, epithelial damage and cell proliferation (Raisch et al., 2014). In another study, IL-10 double knockout mice were treated with the carcinogen AOM, and the animals were raised germ-free before they were mono-colonized with E. coli, suggesting the carcinogenic effect of the E. Coli strain NC101 (Arthur et al., 2012). In a mouse model of CRC, colibactin-producing pks+ E. coli was found to induce DNA double-strand breaks and mutations and promote tumor development. Recent research using mouse colon organoids demonstrated that pks+E. coli could promote the malignant transformation of colon organoids in vitro, emphasizing the relevance of copy number variation in driving the early development and progression of colon cancer (Iftekhar et al., 2021).
Recent studies have reported that the anaerobic gram‐negative oral commensal bacterium, F. nucleatum, plays a significant role in CRC. Cytochrome P450 monooxygenase (mainly CYP2J2) and its mediated product 12,13-EpOME were upregulated in tumor cells from CRC patients and mouse models of CRC, which activated epithelial-mesenchymal transition (EMT) in vitro and promoted CRC cell invasion and migration in vivo. Fecal F. nucleatum levels were positively correlated with serum 12,13-EpOME levels in CRC patients. F. nucleatum may promote CYP2J2 transcription by activating TLR4/AKT signaling, downregulating Keap1 and increasing NRF2 (Kong et al., 2021). Another research suggested that F. nucleatum binds to the T-cell inhibitory immune receptor of natural killer cells (NK) through another adhesin, Fap2 (Gur et al., 2015). F. nucleatum has also been shown to activate the cellular survival mechanism, autophagy, through TLR4 receptors located on CRC cell surfaces in response to oxaliplatin chemotherapy (Yu et al., 2017). In addition, F. nucleatum was found to yield an antagonistic effect on probiotics and was involved in establishing a multispecies microbial community in the large intestine. The extracellular protein secreted by F. nucleatum exhibited strong bacteriostatic activity against the probiotics F. nucleatum, Bifidobacterium, and Lactobacillus. Inhibiting the anti-inflammatory effect of probiotics might be a new reason for the Faecalibacterium prausnitzii being involved in CRC. A combination of F. nucleatum/Bifidobacterium and F. nucleatum/Faecalibacterium prausnitzii had superior sensitivity and specificity in detecting stage I CRC. Indeed, developing microbial ratio detection approaches has huge value for large-scale screening of early CRC (Guo et al., 2018).
There are 2 classes of Bacteroides fragilis distinguished by their ability to secrete a zinc-dependent metalloprotease toxin, Bacteroides fragilis toxin (BFT). Strains that do not secrete BFT are called nontoxigenic Bacteroides fragilis (NTBF), and those that do are referred to as enterotoxigenic Bacteroides fragilis (ETBF) (Wu et al., 2007). The ETBF can reportedly cause gastrointestinal (GI) inflammation and inhabit biofilms coating human CRC. Intestinal inflammation is mediated by BFT, the only known virulence factor of ETBF. BFT-induced increased barrier permeability has been reported to correlate with cleavage of the intercellular adhesion protein of the zonula adherens, E-cadherin. E-cadherin cleavage yields multiple potentially procarcinogenic triggers, including Wnt signaling, CEC proliferation, and epithelial barrier disruption that promote mucosal inflammation (Grivennikov et al., 2012). Recent experimental evidence demonstrating that ETBF-driven colitis promotes colon tumorigenesis has generated interest in the potential contribution of ETBF to human colon carcinogenesis. Other studies revealed additional mechanisms; BFT induces the antiapoptotic protein cIAP2 and the polyamine catalyst spermine oxidase, which triggers reactive oxygen species production, DNA damage, and cell proliferation (Kim et al., 2008). BFT also induces rapid DNA damage in colon epithelial cells in vivo, as detected by activation of the H2A histone family, member X, an initiator of DNA repair (Goodwin et al., 2011). A recent study showed that in multiple intestinal neoplasia (Min) mice, ETBF-induced inflammatory colitis leads to the development of colon cancer within 4 weeks. To sum up, cleavage of E-cadherin, activation of NF-κB (with antiapoptotic effects), increased polyamine metabolism, and induction of DNA damage within the colonic epithelium are key BFT oncogenic mechanisms identified to date in studies.
Enterococcus faecalis is the first colonizer of the human GI tract and has a major impact on intestinal immune development in the very early stages of life. Enterococcus faecalis produces substantial extracellular superoxide and derivative reactive oxygen species and hydroxyl radicals through autoxidation of membrane-associated demethylmenaquinone. These oxidants may cause chromosomal instability associated with sporadic adenomatous polyps and CRC. Enterococcus faecalis has also been demonstrated to produce metalloprotease that can directly compromise the intestinal epithelial barrier and induce inflammation (Huycke et al., 2002). In contrast, in another study, 5-week-old male and female Apc mutant Min mice were fed diets containing heat-killed Enterococcus faecalis strain EC-12 (EC-12) for 8 weeks. A 4.3% decrease in the number of polyps was observed in males vs. 30.9% in females. Moreover, heat-killed EC-12 reduced c-Myc and cyclin D1 mRNA expression levels in intestinal polyps (Miyamoto et al., 2017). Enterococcus faecalis reportedly become pathogenic only during major environmental alterations, such as during the appearance of cytokines and mucins or changes in oxygen tension (de Almeida et al., 2018).
A study showed that compared with healthy subjects, the feces and colon tissue of CRC patients were significantly enriched in P. micromonas (P. micra), which was associated with poor prognosis of CRC patients. In addition, oral administration of P. micra promoted colorectal tumorigenesis in Apcmin/+ mice and colonic epithelial cell proliferation in C57BL/6 mice and germ-free mice. In another microflora analysis, isolation and culture combined with experimental data showed that the toxin-producing strains of Clostridium difficile in the mucosal sera of CRC patients could induce colon tumors in mice, and its tumor-promoting effect depended on the continuous colonization of the strains and the production of the toxin TcdB. An increasing body of evidence suggests that Peptostreptococcus anaerobius (Tsoi et al., 2017), Parvimonas micra (Zhao et al., 2022), Salmonella (Mughini-Gras et al., 2018) and other gut microbiota members can also influence the development of CRC.
Potential beneficial bacteria and their metabolic pathways in CRC
Interestingly, some gut microbiota members yield a pathogenic effect in CRC, while others play a preventive role. Probiotic bacteria confer a health benefit on the host when administered adequately. A review retrieved 21 clinical trials involving 1831 patients undergoing elective colorectal surgery. According to the study results, probiotics could significantly decrease inflammatory factors, chemotherapy side effects, severe diarrhea, postoperative infectious complications, and duration of antibiotic therapy (Darbandi et al., 2020).
Some reports have indicated that lactic acid bacteria (LAB) isolated from different sources possess certain anticancer activity and properties. Intriguingly, lactic acid bacteria have antitumor properties that inactivate or inhibit carcinogenic compounds in the gastrointestinal tract, stimulate the immune response, and reduce the enzymatic activity of β-glucuronidase, azoreductase, and nitroreductase. A pivotal study on a murine model of CRC induced by AOM and Dextran Sulfate Sodium (DSS) revealed significantly alleviated inflammation and tumor development in mice treated with Lactobacillus compared to the CRC mice. Lactobacillus species inhibited tumor growth, and its anticancer activity was at least partially mediated by suppressing the Wnt/β-catenin pathway (Ghanavati et al., 2020). Another research used faecal shotgun metagenomic sequencing to show the depletion of Lactobacillus gallinarum in patients with CRC. In addition, it was suggested that Lactobacillus gallinarum protects against intestinal tumorigenesis by producing protective metabolites (indole-3-lactic acid) that can promote apoptosis of CRC cells (Sugimura et al., 2021). Lactobacillus casei BL23(L. casei BL23) is a probiotic strain known for its anti-inflammatory and anticancer properties. L. casei BL23 significantly protected mice against CRC development and reduced histological scores and proliferative index values. L. casei BL23 may exert an antitumor effect mediated through the downregulation of IL-22 cytokine and upregulation of caspase-7, caspase-9, and Bik (Jacouton et al., 2017). Lactobacillus paracasei (L. paracasei) is a lactic acid bacteria strain isolated from feta-type cheese that has huge prospects for application for CRC treatment. Current evidence suggests that L. paracasei induce CRC cells apoptosis and inhibit their proliferation by regulating the expression of specific Bcl-2 family proteins, producing reactive oxygen species (ROS), inducing the translocation of calreticulin (CRT) and changing the cell cycle (Chondrou et al., 2018; Nozari et al., 2019). Another research showed that the extracellular vesicles of L. paracasei (LpEVs) were taken up by CRC cells and inhibited their proliferation, migration, invasion and apoptosis. Transcriptome sequencing analysis revealed that LpEVs significantly inhibited the phosphorylation level of 3-phosphoinositide-dependent protein kinase-1 (PDK1) and AKT in CRC cells and reduced the expression of Bcl-2 protein (Shi et al., 2021).
Gianotti et al. conducted a randomized placebo-controlled clinical trial demonstrating the beneficial effects of two probiotic LAB strains, Bifidobacterium longum BB536 and Lactobacillus johnsonii La1, in this patient group (Gianotti et al., 2010). B. bifidum has been reported to yield anti-proliferative and protective effects against preneoplastic lesions in animal models of colorectal carcinogenesis (Mohania et al., 2014). Interestingly, some species of Bifidobacteria can decrease carcinogen-induced DNA damage, preneoplastic lesions, and tumors in the colon of rats (Oberreuther-Moschner et al., 2004). It has been reported that whole peptidoglycan, a metabolite produced by Bifidobacterium, could activate macrophages to produce large amounts of cytotoxic molecules, including TNF-α, IL-6, and IL-12 (Wang et al., 2001). Another study investigated the effects of secretion metabolites from different Bifidobacteria species on CRC cell lines. The results showed that the anticancer activity of Bifidobacteria was mediated by the downregulation and upregulation of antiapoptotic and proapoptotic genes, respectively (Faghfoori et al., 2021).
Recent research found that Streptococcus thermophilus (S thermophilus) was depleted in patients with CRC. The antitumor effects of S thermophilus were assessed in 2 murine models of intestinal tumorigenesis. Oral gavage of S thermophilus significantly reduced tumor formation in Apcmin/+ and azoxymethane-injected mice. The tumor-suppressive effect of S thermophilus was likely mediated by the secretion of β-galactosidase (Li et al., 2021).
A recent study used an ex vivo model of the human colon to investigate the impact of the bacteriocin-producing S. salivarius DPC6993 on F. nucleatum, a gut pathogen associated with CRC. Results indicated that S. salivarius DPC6993 could suppress the growth of the CRC-associated bacteria within the gut environment and exert minimal impact on the surrounding GM. As biotherapeutics for suppressing the growth of F. nucleatum in the human gut, these bacteriocin-producing strains can potentially reduce the risk of CRC development. A marked reduction in butyrate-producing bacteria has been observed in patients with CRC. A study found that Clostridium butyricum (C. butyricum) (one of the commonly used butyrate-producing bacteria) significantly inhibited high-fat diet (HFD)-induced intestinal tumor development in Apcmin/+ mice. Moreover, intestinal tumor cells treated with C. butyricum exhibited decreased proliferation and increased apoptosis. It was concluded that C. butyricum could inhibit intestinal tumor development by modulating Wnt signaling and GM (Chen et al., 2020).
Another study examined the effects of butyrate-producing Butyricicoccus pullicaecorum (B. pullicaecorum) on mice with 1,2-dimethylhydrazine (DMH)-induced CRC. Administration of B. pullicaecorum reduced symptoms of CRC by increasing levels of expression of proteins and mRNAs in the 5-limb solute carrier family 8 and the G 43 protein-coupled receptor (Chang et al., 2020).
Microbiota-associated metabolites and CRC
GM can ferment food residues and produce many metabolites, which regulate biological functions in the human body. The main fermentation products in healthy adults are gases and organic acids, especially the three SCFAs acetic, propionic and butyric acids. SCFAs are by-products of dietary fiber in the gut during fermentation and are the most important energy source of the colonic epithelial lining.
Short-chain fatty acids
The GM of CRC patients can increase pathogenic bacterial abundance and reduce SCFA-producing bacteria and SCFA production. The most common SCFA-producing probiotics are Clostridium butyricum, Bifidobacterium, Lactobacillus rhamnosus, Streptococcus thermophilus, Lactobacillus reuteri, Lactobacillus casei, and Lactobacillus acidophilus. SCFAs promote intestinal homeostasis and epithelial integrity by improving intestinal mucosal growth, colonic blood flow, and water and electrolyte absorption (Macia et al., 2015). In vitro and in vivo studies have shown that SCFAs can ameliorate the LPS-induced intestinal barrier disruption by suppressing NLRP3 inflammasome and autophagy by inhibiting ROS production (Feng et al., 2018). SCFAs are key regulators of immune function with immunomodulatory capabilities on various immune cells, including Treg cells, macrophages, and B cells. The host immune system mainly senses and recognizes gut bacterial metabolites by activating SCFA-GPCRs or inhibiting histone deacetylase (HDAC), thereby influencing the host immune response. Propionate in SCFAs promotes its inhibitory activity by inhibiting HDAC through the GPR43 signaling pathway, preventing the occurrence of T cell-induced colitis (Smith et al., 2013).
Bile acid
Epidemiological data suggest that the incidence of CRC is higher in patients with higher fecal bile acid concentrations. It should be borne in mind that not all bile acids promote CRC. Many experiments have shown that high concentrations of secondary bile acids (SBAs) play a role in tumor promotion during the development of CRC. A study revealed that the concentrations of deoxycholic acid (DCA), lithocholic acid (LCA), ursodeoxycholic acid (UDCA), and other indicators in the feces of CRC patients were higher than in the normal control group, while primary bile acids (cholalic acid
, chenodeoxycholine acid) levels were not different from the control group (Rider et al., 1984). It has been established that bile acids exert strong antimicrobial activities, as they damage bacterial cell membranes owing to their amphipathic properties and are, therefore, likely to modify the composition of the GM. Rats fed a diet supplemented with deoxycholic acid showed decreased production of SCFAs and major changes in the microbiota composition, with a relative increase in Gamma-proteobacteria and certain Firmicutes at the expense of Bacteroidetes.
Animal experiments substantiated that SBAs have more potent effects than primary bile acids (PBAs), especially in promoting tumorigenesis and as auxiliary carcinogens (Imray et al., 1992). A high-fat diet and gallbladder disease can increase the content of SBAs in the intestinal cavity, especially DCA levels, thereby promoting the occurrence and development of CRC. The molecular mechanisms that mediate the cytotoxic effects of bile acids are complex. SBAs are more hydrophobic and thus more potent at disrupting cell membranes, which is likely to lead to the generation of ROS via the activation of membrane-associated proteins such as NAD(P)H oxidases and phospholipase A2 (Majer et al., 2014). In contrast, some bile acids seem to counteract the cytotoxic effects of others. In this respect, ursodeoxycholic acid can inhibit the production of ROS and protect cells from the cytotoxic effects of deoxycholic acid (Lee et al., 2013).
Polyamines
Polyamines are derived from endogenous synthesis and GM metabolism of amino acids (Tofalo et al., 2019). Altered polyamine levels are related to cancer. In cancer cells, polyamine metabolism is frequently dysregulated primarily through the upregulation of the polyamine biosynthetic enzymes, leading to elevated polyamine levels necessary for malignant transformation and tumor progression. Polyamine levels were found to increase throughout tumor development in an APCMin/+ murine CRC model. Additionally, biofilm formation in colon cancer increases polyamine metabolites, suggesting polyamines produced by biofilm bacteria or the host enhance tumor development (Holbert et al., 2022). Recent research demonstrated elevated amino acids and polyamines levels in CRC patients, especially cadaverine and putrescine (Hanus et al., 2021). Spermine synthase (SMS), a polyamine biosynthetic enzyme, is reportedly overexpressed in CRC. In a recent study, disruption of the SMS gene in CRC cells was found to alter polyamine metabolism by dramatically reducing the levels of spermine and putrescine but producing excessive levels of spermidine (Guo et al., 2020). These studies indicate that SMS overexpression is required to balance spermidine levels to facilitate CRC cell growth. SMS cooperates with MYC to maintain CRC cell survival via distinct pathways that converge to repress the expression of the proapoptotic protein Bim. Combined inhibition of SMS and MYC signaling induces synergistic apoptosis and tumor regression.
In addition to the above-mentioned metabolite, other metabolites metabolized by intestinal flora, such as glycerophospholipids, tryptophan, trimethylamine oxide, etc., also contribute to CRC via their proinflammatory properties or stimulation of immune responses.
Herbal extract, GM and CRC
Herbal medicine has been in use for thousands of years in China and has a good therapeutic effect against many diseases via its multi-targeting effects. Although the effectiveness of herbal medicine has been established, the mechanism underlying its effects on the human body remains unclear. Oral and enema herbal medicine can significantly affect the distribution and number of GM. Herbal medicine might have beneficial biogenic effects on GM, and it plays an important therapeutic role by regulating the population, distribution and metabolites of intestinal microbiota to promote its probiotic function in CRC. Both these compound formulas and a variety of products of these main constituent herbs, such as extracts, fractions, or single compounds, have demonstrated therapeutic benefits in CRC. In this section we will focus on herbal extracts.
The isoquinoline alkaloid berberine extracted from the TCM, Coptis chinensis, has been documented to have specific antibacterial activity. It has been shown that berberine significantly ameliorates CRC progression in Apcmin/+ mice fed with an HFD, with reduced numbers of tumors and decreased inflammation in colon tissues (Wang et al., 2018). Treatment with berberine reversed the imbalance in intestinal microbiota caused by F. nucleatum colonization and was characterized by an increase in Tenericutes and Verrucomicrobia. At the genus level, some SCFA-producing bacteria increased in abundance (Zhang et al., 2013). SCFAs administration prevented tumor development and attenuated the colonic inflammation in a mouse model of CAC. It is widely acknowledged that GM produces SCFAs by fermentation of dietary fiber (Tian et al., 2018).
GM also ferments the non-digestible plant polysaccharides. Interestingly, polysaccharides, the predominant component of jujube fruit, have been shown to inhibit carcinogenesis in animal models. Research showed jujube polysaccharides (JP) could ward off colon cancer by ameliorating colitis cancer-induced gut dysbiosis. There was a significant decrease in Firmicutes/Bacteroidetes post-JP treatment. Jujube could restore the GM profile altered by AOM/DSS, indicating the potential of jujube polysaccharides as promising prebiotic candidates for the prevention and treatment of CRC (Ji et al., 2020). Another research showed Zizyphus jujuba cv. Muzao polysaccharides (ZMP) consumption prevented CRC mouse colon shortening, decreased their mortality, reduced proinflammatory cytokines, and increased the concentration of total SCFAs and the abundance of Bifidobacterium, Bacteroides and Lactobacillus (Ji et al., 2019).
Carboxymethylated pachyman (CMP) is a polysaccharide modified from the structure of pachyman isolated from Poria cocos. Recent studies have shown that CMP exhibits immune regulatory, anti-inflammatory and antioxidant activities. CMP combined with 5-fluorouracil (5-FU) reversed intestinal shortening (p < 0.01) and alleviated 5-FU-induced colon injury (p < 0.001) via suppression of ROS production. Besides, an increase in the levels of CAT, GSH-Px and GSH and a decrease in NF-κB, p-p38 and Bax expression were observed. More importantly, CMP had a significant impact against GM disorders produced by 5-fluoro-2, 4(1H,3H)pyrimidinedione (5-FU) by increasing the proportion of Bacteroidetes, Lactobacilli, butyric acid-producing and acetic acid-producing bacteria and restoring the GM diversity (Wang et al., 2018). 5-FU plays an important role in palliative and adjuvant systemic chemotherapy for CRC. Over the past decades, various regulatory strategies, such as the implementation of 5-FU-based combination regimens and 5-FU pro-drugs, have been developed and tested to enhance antineoplastic activity and reduce clinical resistance (Vodenkova et al., 2020). Emerging evidence suggests combining conventional chemotherapy with some natural dietary polyphenols for CRC treatment can significantly enhance the chemotherapeutic effect. In addition to CMP, resveratrol and curcumin enhanced the efficacy of 5-FU (Shakibaei et al., 2014; Buhrmann et al., 2015; McFadden et al., 2015). Moreover, resveratrol can be used with other chemotherapeutic agents to overcome drug resistance since it downregulates multidrug resistance protein 1 by blocking the activation of the NF-κB signaling pathway and inhibiting the transcriptional activity of cAMP response elements (Wang et al., 2015). Another study revealed the efficacy of polysaccharides isolated from Nostoc commune Vaucher (NVPS) against CAC tumorigenesis in mice treated with AOM/DSS. The treatment with NVPS significantly decreased the number and size of tumors and reduced the incidence of intestinal tumors. Moreover, the abundance of SCFA-producing genera, including butyric acid-producing genera (Butyricicoccus, Butyrivibrio and Butyricimonas) and acetic acid-producing genera (Lachnospiraceae UCG 001, Lachnospiraceae UCG 006, and Blautia), was significantly enriched following the NVPS treatment. These compositional alterations induced by NVPS were associated with suppressed colonic inflammation and carcinogenesis (Guo and Li, 2019). The study on the regulation of the intestinal microbiota and the inhibition of tumor cell proliferation by algae polysaccharides is of great importance to further explore the antitumor mechanism of algae polysaccharides. Research has shown that Nostoc flagelliforme capsule polysaccharides decreased the abundance of pathogenic bacteria and increased the abundance of probiotics positively correlated with SCFAs. Enteromorpha clathrata dietary polysaccharide and placine polysaccharides can promote tumor cell apoptosis while increasing the abundance of lactobacilli in the intestine, providing a theoretical basis for the future direction of regulating intestinal microbiota with seaweed polysaccharides in the treatment of tumors (Shang et al., 2018; Li et al., 2022).
A meta-analysis revealed that a high intake of certain dietary flavonoids is associated with decreased colon and rectal cancer risk. It has been shown that members of the GM significantly contribute to the metabolism of flavonoids, and flavonoids can alter the composition of the gut microbiome, highlighting that the microbiota plays a central role in the bioactivity of flavonoids (Li et al., 2018). Isoliquiritigenin (ISL), a flavonoid extracted from licorice, has shown antitumor efficacy. The levels of Bacteroidetes decreased and Firmicutes increased during CAC development. ISL reversed the imbalance at the phylum level, and the abundance of Helicobacteraceae increased after treatment with high-dose ISL, while Lachnospiraceae and Rikenellaceae decreased. At the genus level, ISL reduced the abundance of opportunistic pathogens (Escherichia and Enterococcus) and increased the levels of probiotics, particularly butyrate-producing bacteria (Butyricicoccus, Clostridium, and Ruminococcus). These results suggest a synergistic anticancer effect between ISL and GM (Wu et al., 2016).
The flavonoid is a common component found in many medicinal herbs. Apigenin can reportedly exhibit tumor suppression in vitro and in vivo. In a recent study, the size and number of tumors were reduced significantly in the apigenin treatment group. Fecal transplantation provided further evidence of apigenin-modulated GM exerting antitumor effects since Apigenin could not reduce the number or size of tumors when GM was depleted (Bian et al., 2020). Flavanols constitute a very complex group of flavonoids. Catechins, known as the major building blocks of tannins, are the most important representatives of flavanols. The structure of the GM of mice interfered with green tea extract epigallocatechin gallate (EGCG) remained relatively stable. Probiotics such as Lactobacillus and Bifidobacterium were increased in the EGCG+AOM/DSS group, and the relative abundance of Lactobacillus was significantly increased after long-term EGCG intervention. Its main mechanisms may include optimizing the combined GM to inhibit the growth of carcinogenic bacteria and the production of antitumor active substances (Wang et al., 2017). Berries are known to be a rich source of flavonoid compounds, especially anthocyanins. A recent research, six commonly consumed berries and their phenolic compounds, such as anthocyanins, flavonols, flavanols, ellagitannins, gallotannins, proanthocyanidins, and phenolic acids, were tested in vitro to evaluate their anticancer effects in HT-29/HCT116 colon cancer cells. Black raspberry and strawberry extracts showed the most significant proapoptotic effects against human cancer cells (Bouyahya et al., 2022). Another research showed that black raspberry (BRB) powder anthocyanins could modulate the composition of gut commensal microbiota, increase the abundance of probiotics such as Eubacterium rectale, Faecalibacterium prausnitzii and Lactobacillus, and changes in inflammation and the methylation status of the SFRP2 gene may play a central role in the chemoprevention of CRC (Chen et al., 2018).
Similar to other classes of flavonoids, the antitumor activity of the flavonol quercetin has been demonstrated against colon cancer cells both in vitro and in vivo. Quercetin can be metabolized and degraded into the SCFAs acetate and butyrate, associated with cytoprotective effects in the intestinal epithelium and protection against colon tumorigenesis (Li et al., 2018). Quercetin treatment reduced the abundance of Proteobacteria, which commonly blooms in colitis and during inflammation and in patients with IBD (Hughes et al., 2017). To date, no studies have demonstrated a positive effect of quercetin supplements on CRC risk in humans. In the polyp prevention trial, a high intake of flavonols, including quercetin, was associated with a significantly reduced risk of late-stage adenoma recurrence, while quercetin alone was not associated with adenoma recurrence (Bobe et al., 2008).
Gynostemma pentaphyllum (Gp) is a dietary herbal medicine. Gp saponins (GpS) were found to exhibit prebiotic and cancer-preventive properties through the modulation of GM in ApcMin/+ mice. One of the beneficial effects of GpS is its effectiveness in suppressing potentially harmful bacteria, such as sulfur-reducing bacteria and promoting SCFA-producing bacteria. It was found that inoculation with fecal materials from the GpS-treated ApcMin/+ mice effectively reduced the polyp burden in the untreated ApcMin/+ mice. Besides, a marked increase in the beneficial bacteria was detected in the feces of the FMT recipient ApcMin/+ mice. These bacteria include Lactobacillus, Bifidobacterium and Clostridium cluster IV, well-known probiotics (Liao et al., 2021).
Another research reported two of the most abundant compounds of GpS(ginsenosides Rb3 and Rd) for anti-CRC properties in ApcMin/+ mice. The anti-CRC properties correlated with the growth of beneficial bacteria such as Bifidobacterium, Lactobacillus and Bacteroides acidifaciens. In contrast, the abundance of cancer cachexia-associated bacteria, such as Dysgonomonas and Helicobacter, was significantly lower in Rb3/Rd-treated mice. Treatments of ginsenosides Rb3 and Rd exerted anticancer effects by holistically reinstating mucosal architecture, improving mucosal immunity, promoting beneficial bacteria, and downregulating cancer-cachexia-associated bacteria (Huang et al., 2017).
Panax quinquefolius L. is a commonly used herbal medicine, and ginsenosides are considered to be its bioactive constituents. The oral administration of ginseng (15 and 30 mg/kg/day) significantly suppressed AOM/DSS-induced colitis, as demonstrated by the disease activity index and colon tissue histology during the acute phase. Ginseng significantly suppressed AOM/DSS-induced tumor multiplicity during the chronic phase. Serum metabolomics data and the 16S rRNA data showed that after AOM/DSS, the microbiome community and serum metabolomics in the model group was significantly changed, and ginseng inhibited these changes. Oral ginseng significantly decreased AOM/DSS-induced colitis and colon carcinogenesis by inhibiting inflammatory cytokines, increasing the glutamine level and decreasing Bacteroidetes and Verrucomicrobia (Wang et al., 2016). In another example, zerumbone, the main component of Zingiber zerumbet, has been reported to have antibacterial, anti-inflammatory and antitumor activities and can reportedly reduce ETBF-induced, intestinal inflammation-related CRC by altering the IL-17, β-catenin, Stat3, and NF-κB pathways (Hwang et al., 2020).
Traditional Chinese medicine compounds, GM and CRC
Chinese compounds are the material basis of TCM for the treatment of diseases. Each Chinese compounds contains hundreds of chemical molecules, and one compound is a combination of thousands of chemical molecules. How these molecules with diverse chemical structures interact with the complex biological regulatory network of the body, so as to realize the role of strengthening the body and strengthening the capital, exorcising evil spirits and dispelling diseases, has always been the scientific frontier and important issue constantly explored by modern Chinese pharmacists. Chinese compound formula is mainly taken orally. After the complex Chinese compound formula reach the intestinal tract, they will interact with the GM. Analysis the effects and relationship between the active molecules of natural medicine, GM and host will provide new evidence and ideas for the study of the efficacy and mechanism of Chinese medicine, and help to clarify the function and molecular mechanism of GM. Previous studies indicated that TCM yields potential anticancer effects in improving quality of life and therapeutic effect. However, little is known about the mechanism of the TCM formula in cancer prevention.
Gegen Qinlian decoction (GQD), a classical TCM formula, has been proven effective in treating ulcerative colitis (UC). A systemic pharmacological study revealed that combination therapy with GQD and anti-mouse PD-1 potently inhibited the growth of CT26 tumors in a xenograft model. GM analysis revealed that combination therapy with GQD and anti-mouse PD-1 significantly enriched for Bacteroides_acidifaciens and Bacteroidales_S24-7_group. Direct treatment with GQD and anti-mouse PD-1 increased the expression of IFN-γ, which is a critical factor in antitumor immunotherapy. The Chinese medicinal formula GQD, combined with PD-1 blockade-based immunotherapy, represents a novel therapeutic strategy for CRC patients (Lv et al., 2019). It has been reported that Yi-Yi-Fu-Zi-Bai-Jiang-San (YYFZBJS) treatment blocked tumor initiation and progression in ApcMin/+ mice with less change of body weight and increased immune function. The result determined the effect of YYFZBJS on the mRNA expression of T-bet, Gata3, ROR-γt, and Foxp3, which are considered as the master regulator of Helper T lymphocytes development and function in the immune system of mice. Moreover, diversity analysis of fecal samples demonstrated that YYFZBJS regulated animal’s natural GM, including Bacteroides fragilis, Lachnospiraceae, etc. Intestinal tumors from conventional and germ-free mice fed with stool from YYFZBJS volunteers were decreased. Overall, YYFZBJS represents a new potential drug option for treating CRC (Sui et al., 2020). In addition, Wu Mei Wan (WMW) has been used for clinically treating colitis with remarkable efficacy. Our previous research investigates the underlying mechanism of WMW in preventing CAC. WMW attenuates CAC by regulating the balance between “tumor-promoting bacteria “(Bacteroidales_s24-7_group) and “tumor-suppressing bacteria”(Lachnospiraceae).
Taken together, the available data suggest that herbal medicine can modulate dysregulated microbiota during the development of CRC (Table 1). Herbal medicine exerted prebiotic-like effects and restored the intestinal flora diversity or mitigated gut dysbiosis compared with control groups (Figure 1).
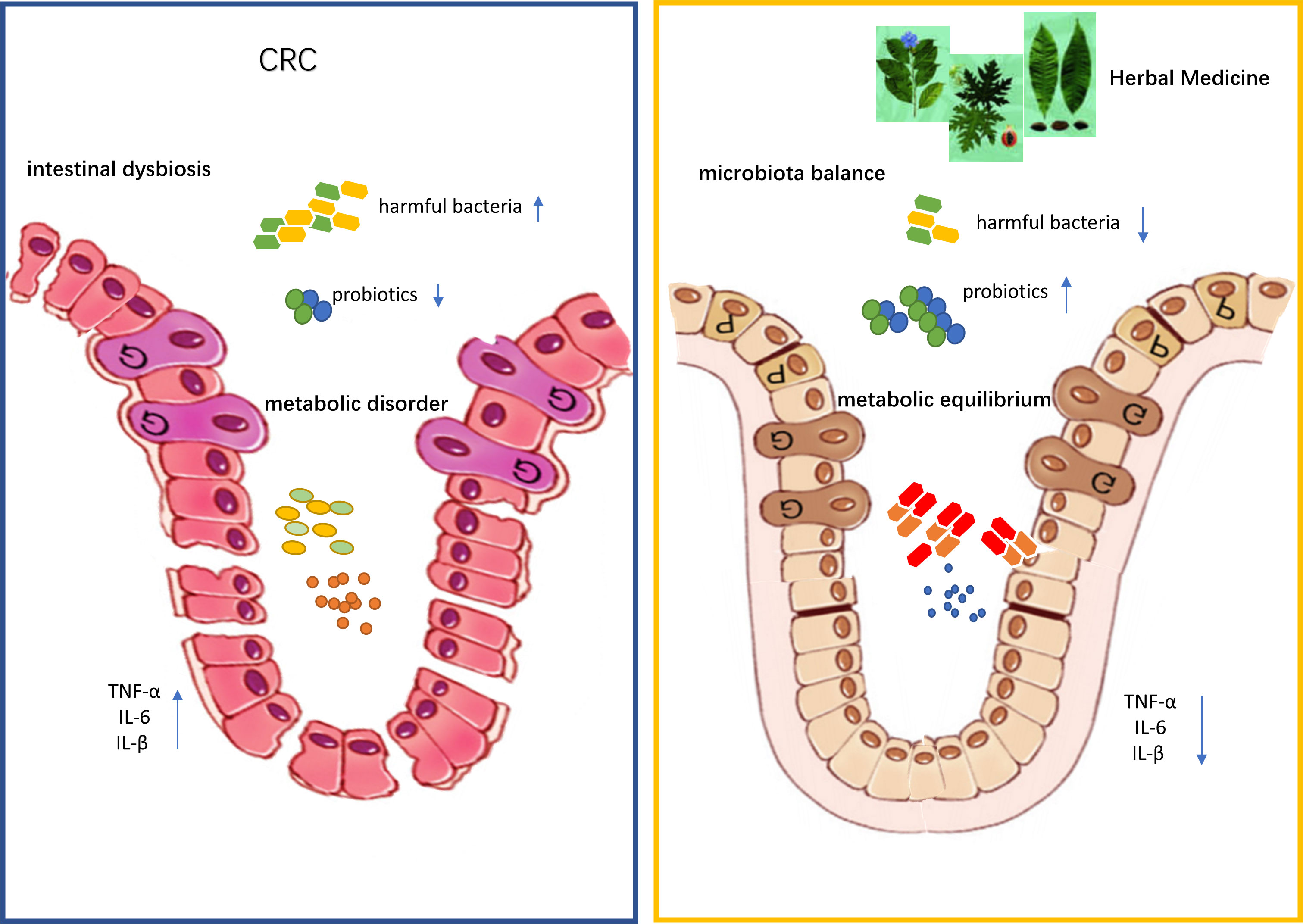
Figure 1 Traditional Chinese medicine improves colon cancer symptoms by regulating gut microbiota and its metabolites.
Future perspectives
In recent decades, GM’s role in mediating the therapeutic efficacy of Chinese medicine has been uncovered. This review discusses and provides a comprehensive overview of the existing evidence and research progress. There is an increasing consensus that GM is closely related to the occurrence and development of CRC and represents a new drug target for treating CRC. The above studies overlap in their assertion that GM and its metabolism play a key role in CRC mediated by immune system stress, amino acid metabolism, protein expression, and gene regulation.
TCM plays an important role in the treatment of several diseases. TCM plants contain various classes of secondary plant metabolites, which are poorly absorbed in the upper GI tract due to their high polarity and molecular weight. Therefore, it is highly conceivable that they interact with the gut microbiome, thereby modulating GM and its metabolism. It has been documented that Chinese medicine regulates the composition of GM and metabolic activities through the GM to treat CRC. Certain compound classes, such as polysaccharides, have been shown to exert prebiotic effects. Terms such as flavobiotics have been used to refer to phytochemical constituents conferring health benefits on the host by positively influencing the gut microbiome. Moreover, some of the plant constituents can be metabolized by GM into pharmacologically active compounds and other postbiotics such as SCFAs, BAs, and polyamines metabolites that can either have a local effect in the gut or be absorbed by the epithelial cells and provide other health benefits to the host via different pathways. TCM can work synergistically with chemotherapeutic drugs to treat CRC, increase their efficacy, reduce their side effects and yield better curative effects.
Most of the existing studies focus on the efficacy of TCM in treating diseases and the regulation of intestinal flora, and lack of further experimental verification and in-depth discussion of the mechanism. In addition, the components of TCM are complex, so it is difficult to explore the molecular mechanism of its specific treatment of diseases. The separation and purification of the components of TCM may contribute to the exploration of the mechanism. Further research on how the Chinese medicine compound affects GM and its metabolism in vivo is warranted. Emphasis should be paid to herbal medicines to better understand the relationship between Chinese medicine and GM, providing the foothold for personalized GM management and treatment with TCM.
Author contributions
FB and YT wrote an original draft, while ST and ZW reviewed the draft. All authors contributed to the article and approved the submitted version.
Funding
This work was supported by the Natural Science Foundation of Zhejiang Province (NO.LY18H160041 and NO. LQ21H160042). This work was also supported by the Seed Foundation of Zhejiang Provincial People’s Hospital (ZRY2022J008).
Acknowledgments
We thank Home for Researchers editorial team (www.home-for-researchers.com) for language editing service.
Conflict of interest
The authors declare that the research was conducted in the absence of any commercial or financial relationships that could be construed as a potential conflict of interest.
Publisher’s note
All claims expressed in this article are solely those of the authors and do not necessarily represent those of their affiliated organizations, or those of the publisher, the editors and the reviewers. Any product that may be evaluated in this article, or claim that may be made by its manufacturer, is not guaranteed or endorsed by the publisher.
References
Arthur, J. C., Perez-Chanona, E., Mühlbauer, M., Tomkovich, S., Uronis, J. M., Fan, T. J., et al. (2012). Intestinal inflammation targets cancer-inducing activity of the microbiota. Science 338 (6103), 120–123. doi: 10.1126/science.1224820
Bender, E. (2020). Could a bacteria-stuffed pill cure autoimmune diseases? Nature 577 (7792), S12–S13. doi: 10.1038/d41586-020-00197-z
Bian, S., Wan, H., Liao, X., Wang, W. (2020). Inhibitory effects of apigenin on tumor carcinogenesis by altering the gut microbiota. Mediators Inflammation 2020, 7141970. doi: 10.1155/2020/7141970
Bobe, G., Sansbury, L. B., Albert, P. S., Sansbury, L. B., Lanza, E., Schatzkin, A., et al. (2008). Dietary flavonoids and colorectal adenoma recurrence in the polyp prevention trial. Cancer Epidemiol. Biomarkers Prev. 17 (6), 1344–1353. doi: 10.1158/1055-9965.EPI-07-0747
Bouyahya, A., Omari, N. E., El Hachlafi, N., Jemly, M. E., Hakkour, M., Balahbib, A., et al. (2022). Chemical compounds of berry-derived polyphenols and their effects on gut microbiota, inflammation, and cancer. Molecules 27 (10), 3286. doi: 10.3390/molecules27103286
Buhrmann, C., Shayan, P., Kraehe, P., Popper, B., Goel, A., Shakibaei, M. (2015). Resveratrol induces chemosensitization to 5-fluorouracil through up-regulation of intercellular junctions, epithelial-to-mesenchymal transition and apoptosis in colorectal cancer. Biochem. Pharmacol. 98 (1), 51–68. doi: 10.1016/j.bcp.2015.08.105
Caruso, M. L., Fucci, L. (1990). Histological identification of helicobacter pylori in early and advanced gastric cancer. J. Clin. Gastroenterol. 12 (5), 601–602.
Chang, S. C., Shen, M. H., Liu, C. Y., Pu, C. M., Hu, J. M., Huang, C. J. (2020). A gut butyrate-producing bacterium butyricicoccus pullicaecorum regulates short-chain fatty acid transporter and receptor to reduce the progression of 1,2-dimethylhydrazine-associated colorectal cancer. Oncol. Lett. 20 (6), 327. doi: 10.3892/ol.2020.12190
Chen, L., Jiang, B., Zhong, C., Guo, J., Zhang, L., Mu, T., et al. (2018). Chemoprevention of colorectal cancer by black raspberry anthocyanins involved the modulation of gut microbiota and SFRP2 demethylation. Carcinogenesis 39 (3), 471–481. doi: 10.1093/carcin/bgy009
Chen, D., Jin, D., Huang, S., Wu, J., Xu, M., Liu, T., et al. (2020). Clostridium butyricum, a butyrate-producing probiotic, inhibits intestinal tumor development through modulating wnt signaling and gut microbiota. Cancer Lett. 469, 456–467. doi: 10.1016/j.canlet.2019.11.019
Chondrou, P., Karapetsas, A., Kiousi, D. E., Tsela, D., Tiptiri-Kourpeti, A., Anestopoulos, I., et al. (2018). Lactobacillus paracasei K5 displays adhesion, anti-proliferative activity and apoptotic effects in human colon cancer cells. Benef Microbes 9 (6), 975–983. doi: 10.3920/BM2017.0183
Darbandi, A., Mirshekar, M., Shariati, A., Moghadam, M. T., Lohrasbi, V., Asadolahi, P., et al. (2020). The effects of probiotics on reducing the colorectal cancer surgery complications: a periodic review during 2007-2017. Clin. Nutr. 39 (8), 2358–2367. doi: 10.1016/j.clnu.2019.11.008
de Almeida, C. V., Taddei, A., Amedei, A. (2018). The controversial role of enterococcus faecalis in colorectal cancer. Therap Adv. Gastroenterol. 11, 1756284818783606. doi: 10.1177/1756284818783606
Drewes, J. L., Chen, J., Markham, N. O., Knippel, R. J., Domingue, J. C., Tam, A. J., et al. (2022). Human colon cancer-derived clostridioides difficile strains drive colonic tumorigenesis in mice. Cancer Discovery 12 (8), 1873–1885. doi: 10.1158/2159-8290.CD-21-1273
Faghfoori, Z., Faghfoori, M. H., Saber, A., Izadi, A., Yari Khosroushahi, A. (2021). Anticancer effects of bifidobacteria on colon cancer cell lines. Cancer Cell Int. 21 (1), 258. doi: 10.1186/s12935-021-01971-3
Feng, Y., Wang, Y., Wang, P., Huang, Y., Wang, F. (2018). Short-chain fatty acids manifest stimulative and protective effects on intestinal barrier function through the inhibition of NLRP3 inflammasome and autophagy. Cell Physiol. Biochem. 49 (1), 190–205. doi: 10.1159/000492853
Ghanavati, R., Akbari, A., Mohammadi, F., Asadollahi, P., Javadi, A., Talebi, M., et al. (2020). Lactobacillus species inhibitory effect on colorectal cancer progression through modulating the wnt/beta-catenin signaling pathway. Mol. Cell Biochem. 470 (1-2), 1–13. doi: 10.1007/s11010-020-03740-8
Gianotti, L., Morelli, L., Galbiati, F., Rocchetti, S., Coppola, S., Beneduce, A., et al. (2010). A randomized double-blind trial on perioperative administration of probiotics in colorectal cancer patients. World J. Gastroenterol. 16 (2), 167–175. doi: 10.3748/wjg.v16.i2.167
Goodwin, A. C., Destefano, S. C., Wu, S., Huso, D. L., Wu, X., Murray-Stewart, T. R., et al. (2011). Polyamine catabolism contributes to enterotoxigenic bacteroides fragilis-induced colon tumorigenesis. Proc. Natl. Acad. Sci. U.S.A. 108 (37), 15354–15359. doi: 10.1073/pnas.1010203108
Grivennikov, S. I., Wang, K., Mucida, D., Stewart, C. A., Schnabl, B., Jauch, D., et al. (2012). Adenoma-linked barrier defects and microbial products drive IL-23/IL-17-mediated tumour growth. Nature 491 (7423), 254–258. doi: 10.1038/nature11465
Guo, M., Li, Z. (2019). Polysaccharides isolated from nostoc commune vaucher inhibit colitis-associated colon tumorigenesis in mice and modulate gut microbiota. Food Funct. 10 (10), 6873–6881. doi: 10.1039/C9FO00296K
Guo, S., Li, L., Xu, B., Li, M., Zeng, Q., Xiao, H., et al. (2018). A simple and novel fecal biomarker for colorectal cancer: ratio of fusobacterium nucleatum to probiotics populations, based on their antagonistic effect. Clin. Chem. 64 (9), 1327–1337. doi: 10.1373/clinchem.2018.289728
Guo, Y., Ye, Q., Deng, P., Cao, Y., He, D., Zhou, Z., et al. (2020). Spermine synthase and MYC cooperate to maintain colorectal cancer cell survival by repressing bim expression. Nat. Commun. 11 (1), 3243. doi: 10.1038/s41467-020-17067-x
Gur, C., Ibrahim, Y., Isaacson, B., Yamin, R., Abed, J., Gamliel, M., et al. (2015). Binding of the Fap2 protein of fusobacterium nucleatum to human inhibitory receptor TIGIT protects tumors from immune cell attack. Immunity 42 (2), 344–355. doi: 10.1016/j.immuni.2015.01.010
Hanus, M., Parada-Venegas, D., Landskron, G., Wielandt, A. M., Hurtado, C., Alvarez, K., et al. (2021). Immune system, microbiota, and microbial metabolites: the unresolved triad in colorectal cancer microenvironment. Front. Immunol. 12, 612826. doi: 10.3389/fimmu.2021.612826
Holbert, C. E., Cullen, M. T., Casero, R. A., Stewart, T. M. (2022). Polyamines in cancer: integrating organismal metabolism and antitumour immunity. Nat. Rev. Cancer 22 (8), 467–480. doi: 10.1038/s41568-022-00473-2
Huang, G., Khan, I., Li, X., Chen, L., Leong, W., Ho, L. T., et al. (2017). Ginsenosides Rb3 and Rd reduce polyps formation while reinstate the dysbiotic gut microbiota and the intestinal microenvironment in Apc(Min/+) mice. Sci. Rep. 7 (1), 12552. doi: 10.1038/s41598-017-12644-5
Hughes, E. R., Winter, M. G., Duerkop, B. A., Spiga, L., Furtado de Carvalho, T., Zhu, W., et al. (2017). Microbial respiration and formate oxidation as metabolic signatures of inflammation-associated dysbiosis. Cell Host Microbe 21 (2), 208–219. doi: 10.1016/j.chom.2017.01.005
Huycke, M. M., Abrams, V., Moore, D. R. (2002). Enterococcus faecalis produces extracellular superoxide and hydrogen peroxide that damages colonic epithelial cell DNA. Carcinogenesis 23 (3), 529–536. doi: 10.1093/carcin/23.3.529
Hwang, S., Jo, M., Hong, J. E., Park, C. O., Lee, C. G., Rhee, K. J. (2020). Protective effects of zerumbone on colonic tumorigenesis in enterotoxigenic bacteroides fragilis (ETBF)-colonized AOM/DSS BALB/c mice. Int. J. Mol. Sci. 21 (3), 857. doi: 10.3390/ijms21030857
Iftekhar, A., Berger, H., Bouznad, N., Heuberger, J., Boccellato, F., Dobrindt, U., et al. (2021). Genomic aberrations after short-term exposure to colibactin-producing e. coli transform primary colon epithelial cells. Nat. Commun. 12 (1), 1003. doi: 10.1038/s41467-021-21162-y
Imray, C. H., Radley, S., Davis, A., Barker, G., Hendrickse, C. W., Donovan, I. A., et al. (1992). Faecal unconjugated bile acids in patients with colorectal cancer or polyps. Gut 33 (9), 1239–1245. doi: 10.1136/gut.33.9.1239
Jacouton, E., Chain, F., Sokol, H., Langella, P., Bermúdez-Humarán, L. G. (2017). Probiotic strain lactobacillus casei BL23 prevents colitis-associated colorectal cancer. Front. Immunol. 8, 1553. doi: 10.3389/fimmu.2017.01553
Ji, X., Hou, C., Zhang, X., Han, L., Yin, S., Peng, Q., et al. (2019). Microbiome-metabolomic analysis of the impact of zizyphus jujuba cv. muzao polysaccharides consumption on colorectal cancer mice fecal microbiota and metabolites. Int. J. Biol. Macromol 131, 1067–1076. doi: 10.1016/j.ijbiomac.2019.03.175
Ji, X., Hou, C., Gao, Y., Xue, Y., Yan, Y., Guo, X. (2020). Metagenomic analysis of gut microbiota modulatory effects of jujube (Ziziphus jujuba mill.) polysaccharides in a colorectal cancer mouse model. Food Funct. 11 (1), 163–173. doi: 10.1039/c9fo02171j
Kim, J. M., Lee, J. Y., Kim, Y. J. (2008). Inhibition of apoptosis in bacteroides fragilis enterotoxin-stimulated intestinal epithelial cells through the induction of c-IAP-2. Eur. J. Immunol. 38 (8), 2190–2199. doi: 10.1002/eji.200838191
Komaroff, A. L. (2018). The microbiome and risk for atherosclerosis. JAMA 319 (23), 2381–2382. doi: 10.1001/jama.2018.5240
Kong, C., Yan, X., Zhu, Y., Zhu, H., Luo, Y., Liu, P., et al. (2021). Fusobacterium nucleatum promotes the development of colorectal cancer by activating a cytochrome P450/Epoxyoctadecenoic acid axis via TLR4/Keap1/NRF2 signaling. Cancer Res. 81 (17), 4485–4498. doi: 10.1158/0008-5472.CAN-21-0453
Lee, J. Y., Arai, H., Nakamura, Y., Fukiya, S., Wada, M., Yokota, A. (2013). Contribution of the 7beta-hydroxysteroid dehydrogenase from ruminococcus gnavus N53 to ursodeoxycholic acid formation in the human colon. J. Lipid Res. 54 (11), 3062–3069. doi: 10.1194/jlr.M039834
Li, Q., Hu, W., Liu, W. X., Zhao, L. Y., Huang, D., Liu, X. D., et al. (2021). Streptococcus thermophilus inhibits colorectal tumorigenesis through secreting beta-galactosidase. Gastroenterology 160 (4), 1179–1193.e14. doi: 10.1053/j.gastro.2020.09.003
Li, J., Shen, S. G., Han, C. F., Liu, S. T., Zhang, L. L., Chen, N., et al. (2022). Nostoc flagelliforme capsular polysaccharides from different culture conditions improve hyperlipidemia and regulate intestinal flora in C57BL/6J mice to varying degrees. Int. J. Biol. Macromol 202, 224–233. doi: 10.1016/j.ijbiomac.2022.01.034
Li, Y., Zhang, T., Chen, G. Y. (2018). Flavonoids and colorectal cancer prevention. Antioxid (Basel) 7 (12), 187. doi: 10.3390/antiox7120187
Liao, W., Khan, I., Huang, G., Chen, S., Liu, L., Leong, W. K., et al. (2021). Bifidobacterium animalis: the missing link for the cancer-preventive effect of gynostemma pentaphyllum. Gut Microbes 13 (1), 1847629. doi: 10.1080/19490976.2020.1847629
Liu, X., Cheng, Y., Shao, L., Ling, Z. (2020). Alterations of the predominant fecal microbiota and disruption of the gut mucosal barrier in patients with early-stage colorectal cancer. BioMed. Res. Int. 2020, 2948282. doi: 10.1155/2020/2948282
Lv, J., Jia, Y., Li, J., Kuai, W., Li, Y., Guo, F., et al. (2019). Gegen qinlian decoction enhances the effect of PD-1 blockade in colorectal cancer with microsatellite stability by remodelling the gut microbiota and the tumour microenvironment. Cell Death Dis. 10 (6), 415. doi: 10.1038/s41419-019-1638-6
Macia, L., Tan, J., Vieira, A. T., Leach, K., Stanley, D., Luong, S., et al. (2015). Metabolite-sensing receptors GPR43 and GPR109A facilitate dietary fibre-induced gut homeostasis through regulation of the inflammasome. Nat. Commun. 6, 6734. doi: 10.1038/ncomms7734
Majer, F., Sharma, R., Mullins, C., Keogh, L., Phipps, S., Duggan, S., et al. (2014). New highly toxic bile acids derived from deoxycholic acid, chenodeoxycholic acid and lithocholic acid. Bioorg Med. Chem. 22 (1), 256–268. doi: 10.1016/j.bmc.2013.11.029
Marchesi, J. R., Adams, D. H., Fava, F., Hermes, G. D., Hirschfield, G. M., Hold, G., et al. (2016). The gut microbiota and host health: a new clinical frontier. Gut 65 (2), 330–339. doi: 10.1136/gutjnl-2015-309990
McFadden, R. M., Larmonier, C. B., Shehab, K. W., Midura-Kiela, M., Ramalingam, R., Harrison, C. A., et al. (2015). The role of curcumin in modulating colonic microbiota during colitis and colon cancer prevention. Inflammation Bowel Dis. 21 (11), 2483–2494. doi: 10.1097/MIB.0000000000000522
Miyamoto, S., Komiya, M., Fujii, G., Hamoya, T., Nakanishi, R., Fujimoto, K., et al. (2017). Preventive effects of heat-killed enterococcus faecalis strain EC-12 on mouse intestinal tumor development. Int. J. Mol. Sci. 18, (4). doi: 10.3390/ijms18040826
Mohania, D., Kansal, V. K., Kruzliak, P., Kumari, A. (2014). Probiotic dahi containing lactobacillus acidophilus and bifidobacterium bifidum modulates the formation of aberrant crypt foci, mucin-depleted foci, and cell proliferation on 1,2-dimethylhydrazine-induced colorectal carcinogenesis in wistar rats. Rejuvenation Res. 17 (4), 325–333. doi: 10.1089/rej.2013.1537
Mughini-Gras, L., Schaapveld, M., Kramers, J., Mooij, S., Neefjes-Borst, E. A., Pelt, W. V., et al. (2018). Increased colon cancer risk after severe salmonella infection. PloS One 13 (1), e0189721. doi: 10.1371/journal.pone.0189721
Nozari, S., Faridvand, Y., Etesami, A., Ahmad Khan Beiki, M., Miresmaeili Mazrakhondi, S. A., Abdolalizadeh, J. (2019). Potential anticancer effects of cell wall protein fractions from lactobacillus paracasei on human intestinal caco-2 cell line. Lett. Appl. Microbiol. 69 (3), 148–154. doi: 10.1111/lam.13198
Oberreuther-Moschner, D. L., Jahreis, G., Rechkemmer, G., Pool-Zobel, B. L. (2004). Dietary intervention with the probiotics lactobacillus acidophilus 145 and bifidobacterium longum 913 modulates the potential of human faecal water to induce damage in HT29clone19A cells. Br. J. Nutr. 91 (6), 925–932. doi: 10.1079/BJN20041108
Raisch, J., Buc, E., Bonnet, M., Sauvanet, P., Vazeille, E., de Vallée, A., et al. (2014). Colon cancer-associated B2 escherichia coli colonize gut mucosa and promote cell proliferation. World J. Gastroenterol. 20 (21), 6560–6572. doi: 10.3748/wjg.v20.i21.6560
Reddy, B. S., Narisawa, T., Weisburger, J. H. (1976). Colon carcinogenesis in germ-free rats with intrarectal 1,2-dimethylhydrazine and subcutaneous azoxymethane. Cancer Res. 36 (8), 2874–2876.
Rider, A. A., Calkins, B. M., Arthur, R. S., Nair, P. P. (1984). Diet, nutrition intake, and metabolism in populations at high and low risk for colon cancer. concordance of nutrient information obtained by different methods. Am. J. Clin. Nutr. 40 (4 Suppl), 906–913. doi: 10.1093/ajcn/40.4.880
Shakibaei, M., Buhrmann, C., Kraehe, P., Shayan, P., Lueders, C., Goel, A. (2014). Curcumin chemosensitizes 5-fluorouracil resistant MMR-deficient human colon cancer cells in high density cultures. PloS One 9 (1), e85397. doi: 10.1371/journal.pone.0085397
Shang, Q., Wang, Y., Pan, L., Niu, Q., Li, C., Jiang, H., et al. (2018). Dietary polysaccharide from enteromorpha clathrata modulates gut microbiota and promotes the growth of akkermansia muciniphila, bifidobacterium spp. and lactobacillus spp. Mar. Drugs 16 (5), 167. doi: 10.3390/md16050167
Shi, Y., Meng, L., Zhang, C., Zhang, F., Fang, Y. (2021). Extracellular vesicles of lacticaseibacillus paracasei PC-H1 induce colorectal cancer cells apoptosis via PDK1/AKT/Bcl-2 signaling pathway. Microbiol. Res. 255, 126921. doi: 10.1016/j.micres.2021.126921
Shin, W., Kim, H. J. (2018). Intestinal barrier dysfunction orchestrates the onset of inflammatory host-microbiome cross-talk in a human gut inflammation-on-a-chip. Proc. Natl. Acad. Sci. U.S.A. 115 (45), E10539–E10547. doi: 10.1073/pnas.1810819115
Smith, P. M., Howitt, M. R., Panikov, N., Michaud, M., Gallini, C. A., Bohlooly-Y, M., et al. (2013). The microbial metabolites, short-chain fatty acids, regulate colonic treg cell homeostasis. Science 341 (6145), 569–573. doi: 10.1126/science.1241165
Sugimura, N., Li, Q., Chu, E. S.H., Lau, H. C.H., Fong, W., Liu, W., et al. (2021). Lactobacillus gallinarum modulates the gut microbiota and produces anti-cancer metabolites to protect against colorectal tumourigenesis. Gut. 71 (10), 2011–2021. doi: 10.1136/gutjnl-2020-323951
Sui, H., Zhang, L., Gu, K., Chai, N., Ji, Q., Zhou, L., et al. (2020). YYFZBJS ameliorates colorectal cancer progression in Apc(Min/+) mice by remodeling gut microbiota and inhibiting regulatory T-cell generation. Cell Commun. Signal 18 (1), 113. doi: 10.1186/s12964-020-00596-9
Tian, Y., Xu, Q., Sun, L., Ye, Y., Ji, G. (2018). Short-chain fatty acids administration is protective in colitis-associated colorectal cancer development. J. Nutr. Biochem. 57, 103–109. doi: 10.1016/j.jnutbio.2018.03.007
Tofalo, R., Cocchi, S., Suzzi, G. (2019). Polyamines and gut microbiota. Front. Nutr. 6, 16. doi: 10.3389/fnut.2019.00016
Tsoi, H., Chu, E. S. H., Zhang, X., Sheng, J., Nakatsu, G., Ng, S. C., et al. (2017). Peptostreptococcus anaerobius induces intracellular cholesterol biosynthesis in colon cells to induce proliferation and causes dysplasia in mice. Gastroenterology 152 (6), 1419–1433.e5. doi: 10.1053/j.gastro.2017.01.009
Vodenkova, S., Buchler, T., Cervena, K., Veskrnova, V., Vodicka, P., Vymetalkova, V. (2020). 5-fluorouracil and other fluoropyrimidines in colorectal cancer: past, present and future. Pharmacol. Ther. 206, 107447. doi: 10.1016/j.pharmthera.2019.107447
Wang, H., Guan, L., Li, J., Lai, M., Wen, X. (2018). The effects of berberine on the gut microbiota in apc (min/+) mice fed with a high fat diet. Molecules 23 (9). doi: 10.3390/molecules23092298
Wang, C., Yang, S., Gao, L., Wang, L., Cao, L. (2018). Carboxymethyl pachyman (CMP) reduces intestinal mucositis and regulates the intestinal microflora in 5-fluorouracil-treated CT26 tumour-bearing mice. Food Funct. 9 (5), 2695–2704. doi: 10.1039/C7FO01886J
Wang, X., Ye, T., Chen, W. J., Lv, Y., Hao, Z., Chen, J., et al. (2017). Structural shift of gut microbiota during chemo-preventive effects of epigallocatechin gallate on colorectal carcinogenesis in mice. World J. Gastroenterol. 23 (46), 8128–8139. doi: 10.3748/wjg.v23.i46.8128
Wang, C. Z., Yu, C., Wen, X. D., Chen, L., Zhang, C. F., Calway, T., et al. (2016). American Ginseng attenuates colitis-associated colon carcinogenesis in mice: impact on gut microbiota and metabolomics. Cancer Prev. Res. (Phila) 9 (10), 803–811. doi: 10.1158/1940-6207.CAPR-15-0372
Wang, Z., Zhang, L., Ni, Z., Sun, J., Gao, H., Cheng, Z., et al. (2015). Resveratrol induces AMPK-dependent MDR1 inhibition in colorectal cancer HCT116/L-OHP cells by preventing activation of NF-kappaB signaling and suppressing cAMP-responsive element transcriptional activity. Tumour Biol. 36 (12), 9499–9510. doi: 10.1007/s13277-015-3636-3
Wang, L. S., Zhu, H. M., Zhou, D. Y., Wang, Y. L., Zhang, W. D. (2001). Influence of whole peptidoglycan of bifidobacterium on cytotoxic effectors produced by mouse peritoneal macrophages. World J. Gastroenterol. 7 (3), 440–443. doi: 10.3748/wjg.v7.i3.440
Wong, S. H., Zhao, L., Zhang, X., Nakatsu, G., Han, J., Xu, W., et al. (2017). Gavage of fecal samples from patients with colorectal cancer promotes intestinal carcinogenesis in germ-free and conventional mice. Gastroenterology 153 (6), 1621–1633.e6. doi: 10.1053/j.gastro.2017.08.022
Wu, S., Rhee, K. J., Zhang, M., Franco, A., Sears, C. L. (2007). Bacteroides fragilis toxin stimulates intestinal epithelial cell shedding and gamma-secretase-dependent e-cadherin cleavage. J. Cell Sci. 120 (Pt 11), 1944–1952. doi: 10.1242/jcs.03455
Wu, M., Wu, Y., Deng, B., Li, J., Cao, H., Qu, Y., et al. (2016). Isoliquiritigenin decreases the incidence of colitis-associated colorectal cancer by modulating the intestinal microbiota. Oncotarget 7 (51), 85318–85331. doi: 10.18632/oncotarget.13347
Yu, T., Guo, F., Yu, Y., Sun, T., Ma, D., Han, J., et al. (2017). Fusobacterium nucleatum promotes chemoresistance to colorectal cancer by modulating autophagy. Cell 170 (3), 548–563.e16. doi: 10.1016/j.cell.2017.07.008
Zhang, J., Cao, H., Zhang, B., Cao, H., Xu, X., Ruan, H., et al. (2013). Berberine potently attenuates intestinal polyps growth in ApcMin mice and familial adenomatous polyposis patients through inhibition of wnt signalling. J. Cell Mol. Med. 17 (11), 1484–1493. doi: 10.1111/jcmm.12119
Keywords: microbial metabolism, gut microbiota, herbal medicine, colorectal cancer, bile acids
Citation: Bu F, Tu Y, Wan Z and Tu S (2023) Herbal medicine and its impact on the gut microbiota in colorectal cancer. Front. Cell. Infect. Microbiol. 13:1096008. doi: 10.3389/fcimb.2023.1096008
Received: 11 November 2022; Accepted: 13 June 2023;
Published: 04 July 2023.
Edited by:
Xingmin Sun, University of South Florida, United StatesReviewed by:
Qiong Wang, Affiliated Hospital of Nanjing University of Chinese Medicine, ChinaJian Li, Tianjin University of Science and Technology, China
Copyright © 2023 Bu, Tu, Wan and Tu. This is an open-access article distributed under the terms of the Creative Commons Attribution License (CC BY). The use, distribution or reproduction in other forums is permitted, provided the original author(s) and the copyright owner(s) are credited and that the original publication in this journal is cited, in accordance with accepted academic practice. No use, distribution or reproduction is permitted which does not comply with these terms.
*Correspondence: Shiliang Tu, dHVzaGlsaWFuZ0AxMjYuY29t; Ziang Wan, amVycnl3YW4wMUAxMjYuY29t