- 1Laboratory Medicine Centre, Nanfang Hospital, Southern Medical University, Guangzhou, China
- 2Department of Infectious Diseases, Shenzhen Children’s Hospital, Shenzhen, China
- 3School of Medicine, Southern University of Science and Technology, ShenZhen, Guangdong, China
- 4Center for Clinical Laboratory, Zhujiang Hospital, Southern Medical University, Guangzhou, China
Introduction: Samonella is 1 of 4 key global causes of diarrhoeal diseases, sometimes it can be serious, especially for yong children. Due to the extensive resistance of salmonella serotypes to conventional first-line drugs, macrolides (such as azithromycin) have been designated as the most important antibiotics for the treatment of salmonella. Antimicrobial resistance is a major public health problem in the world, and the mechanism of azithromycin resistance is rarely studied.
Methods: This study determined the azithromycin resistance and plasmids of Salmonella enterica isolates from children attending the Shenzhen Children’s Hospital. The susceptibility of ampicillin (AMP), ciprofloxacin (CIP), ceftriaxone (CRO), sulfamethoxazole (SMZ), chloramphenicol (CL), and azithromycin (AZM) were detected and the genes and plasmids from azithromycin-resistant Salmonella were detected by Illumina hi-seq and Nanopore MinIone whole genome sequencing (WGS) using a map-based method, and the genomic background of these factors was evaluated using various bioinformatics tools.
Results: In total, 15 strains of nontyphoid Salmonella strains that were isolated (including S. typhimurium, S.London, S. Goldcoast, and S.Stanley) demonstrated resistance to azithromycin (minimum inhibitory concentration,MIC from 32 to >256 µg/mL), and the resistance rate was 3.08% (15/487). The sensitivity test to other antibiotics demonstrated 100% resistance to AMP, and the resistance to SMZ and CL was 86.7% and 80.0%, respectively. Through WGS analysis, all isolates were positive for a plasmid-encoded mphA gene. Plasmid incompatibility typing identified five IncFIB(K), five IncHI2/HI2A/Q1, two IncC, one IncHI2/HI2A/N, one IncR, one IncFII and one IncHI2/HI2A plasmids. Sequence analyses of plasmids revealed extensive homology to various plasmids or transposons in regions involved in plasmid replication/maintenance functions and/or in antibiotic resistance gene clusters.
Conclusion: mphA is the main gene involved in azithromycin, a macrolide, and resistance to Salmonella. It is usually located on plasmids and easily spreads, hence posing a great threat to the current treatment of Salmonella infection. The plasmid sequence similarities suggest that the plasmids acquired resistance genes from a variety of enterica bacteria and underscore the importance of a further understanding of horizontal gene transfer among enterica bacteria.
1 Introduction
Salmonella is a gram negative rods belonging to the Enterobacteriaceae family and are divided into serotypes according to the structures of H and O antigens on their surface. The two species of Salmonella are S.bongori and S.enterica, S.enterica including more than 2,600 serotypes have been shown to be main sources of infections in human. These serogroups include S.Typhi,S.Paratyphi, S.Typhimurium, S.Enteritidis, S.Choleraesuis, and so on, which can be grouped into typhoidal and nontyphoidal Salmonella (NTS) serovars (Gilchrist et al., 2015).
Samonella is 1 of 4 key global causes of diarrhoeal diseases, most of salmonellosis is mild, diarrhea, fever and stomach cramps are the main symptoms, people should not take antibiotics and recover in 4 to 7 days (World Health Organization, 2018). Sometimes it can be serious, especially for yong children, it was reported by WHO that 550 million people falling ill and 220 million chidlren under the age of 5-year-old each year (World Health Organization, 2018). Current recommendations are that antibiotics be reserved for patients with severe disease or patients who are at a high risk for invasive disease (Guarino et al., 2014). Children with suspected or confirmed invasive infections, including infants younger than three months of age with immune deficiency, chronic basic diseases, and severe enteritis need antibiotics (Nair et al., 2021). However, with the wide application of antibiotics, the drug resistance rate is increasing yearly, which poses a severe challenge to treating Salmonella infection. The rational selection and use of antibiotics have become important issues worldwide.
Ampicillin, chloramphenicol and cotrimoxazole were the first-line antibiotics for the treatment of salmonellosis, resistance to first-line antibiotics used to treat infections caused by Salmonella is increasing. The emergence and spread of multi-drug resistance (MDR) pose a major threat to the effective treatment and control of Salmonellosis, macrolides(such as azithromycin) and carbapenems have been designated as the most important antibiotics for the treatment of Salmonella disease (Carey et al., 2021).
Azithromycin is the only remaining oral drug for the treatment of extensively drug resistant (XDR) Salmonella infection (Plumb et al., 2019). Particularly noteworthy is the emerging resistance to azithromycin, which will cause people to worry about incurable infection. It is necessary to monitor and diagnose azithromycin resistance to guide rational use and prevent the prevalence and expansion of drug resistance.It was reported that the azithromycin resistance rate of NTS isolates from Taiwan (3.1%) is much higher than that of NTS isolates from European countries and the United States (Chiou et al., 2023).Antimicrobial resistance is a major public health problem in the world, and the mechanism of azithromycin resistance is rarely studied.
This study aimed to determine the azithromycin resistance genes and plasmids of Salmonella enterica isolates from children attending the Shenzhen Children’s Hospital by susceptibility testing and whole genome sequencing (WGS), and provide information of monitoring and periodic review of sensitivity data to ensure the adequacy of treatment guidelines.
2 Materials and methods
2.1 Ethics approval and consent to participate
The data were approved by the ethics committee of Shenzhen Children’s Hospital under document number SEY0132407.
2.2 Bacterial collection
Salmonella enterica strains were isolated from clinical blood and fecal culture samples collected from the Shenzhen Children’s Hospital between January 2014 and December 2021. The data, including information of children and isolations were collected from Clinical Microbiology Laboratory, Department of clinical Laboratory, We excluded data on contaminated bacteria and duplicate strains detected from the same patient.
2.3 Bacterial culture and identification
A BACTEC™ FX-200 automatic blood culture instrument (BD Diagnostic Systems, Sparks, MD, USA) and BacT/Alert 3D blood culture system (BTA3D; bioMerieux, Marcy lEtoile, France) were used for blood culture, 1~10 ml of blood specimens were subjected to blood culture bottles and incubated at 37°C in automated system. Bottles that positive alerts were detected would be removed and samples would be cultured on Columbia blood agar plates and chocolate agar plates. Other samples were cultured and all isolates were identified according to methods of Manual of Clinical Microbiology [11th edition] (James et al., 2015). The bacteria were identified to the genus level using a VITEK 2 COMPACT automatic microbial identification drug sensitivity instrument (Biomerieux, France) and a mass spectrometry system (MALDI-TOF MS, Merier, France), and the serotypes were divided using the Danish Statens Serum Institut diagnostic serum according to structures of somatic O and flagellar H antigens(the Kauffman-White classification).
2.4 Drug sensitivity test
The susceptibility of five antimicrobial agents (Oxoid, UK), including ampicillin (AMP), ceftriaxone (CRO), chloramphenicol (CL), trimethoprim-sulfamethoxazole (SXT), ciprofloxacin (CIP), and azithromycin (AZM), was determined using the disk diffusion method to screen azithromycin resistant strains. The MIC value of azithromycin resistant stains were calculated using the E-test method (Biomerieux,France). The results, evaluated according to the judgment results of the breaking point standards recommended by the Clinical and Laboratory Standards Institute (CLSI) M100 2021 (Clinical and Laboratory Standards Institute[CLSI], 2021), were divided into sensitivity, mediation, and drug resistance. Since there is no definite azithromycin CLSI break point for any Salmonella serotype except S.Typhi, the azithromycin resistance standard of S. Typhi was used, i.e., inhibition zone ≤ 12 mm and MIC ≥ 32 mg/mL was determined as drug resistance.
2.5 Whole-genome sequencing
The S. enterica isolates for azithromycin resistance were further subjected to whole-genome sequencing. Genomic DNA was extracted from overnight cultures using a QIAamp DNA mini kit (Qiagen) according to the manufacturer’s instructions. DNA quality was assessed using Nanodrop spectrophotometry (Thermo Fisher), and quantity was assessed using the Qubit 4.0 system (Thermo Fisher). The DNA libraries were constructed with 150-bp paired-end whole-genome sequencing using the Illumina HiSeq 2500 system (Huada, Shenzhen, China) (Ma et al., 2020). The obtained paired-end Illumina reads were assembled de novo using SPAdes v3.6.2 (default parameters except -careful and -k 21,33,55,77,99,127). In addition, to obtain long read sequences, selected strains were further sequenced using Oxford Nanopore MinION flowcell R9.4 (Li et al., 2018). De novo hybrid assembly was performed using a combined Illumina HiSeq and Nanopore sequencing approach (Nextomics). Genome assembly was performed with Unicycler version 0.4.1 using a combination of short and long reads, followed by error correction with Pilon version1.12 (Wick et al., 2017) (Walker et al., 2014).
2.6 Data analysis
An in silico multilocus sequence typing (MLST) scheme was used to subtype the isolates using mlst software (version 2.19.0) (Larsen et al., 2012). The chromosome and plasmid sequences were annotated using the prokaryotic gene prediction tool Prokka (Seemann, 2014). The plasmid incompatibility type was searched using the online tool PlasmidFinder (https://cge.cbs.dtu.dk//services/PlasmidFinder/) (Carattoli et al., 2014). Antibiotic resistance genes were identified using both the Comprehensive Antibiotic Resistance Database (CARD) database (Alcock et al., 2020). Comparative plasmid illustration was implemented by BRIG (http://brig.sourceforge.net) (Alikhan et al., 2011). BLAST (https://blast.ncbi.nlm.nih.gov/Blast.cgi) was used for comparative analysis through coverage and identities (Camacho et al., 2009).
Genomic sequences and the associated metadata of 10561 Salmonella strains stored in the NCBI GenBank database were obtained. Bacterial core genome single nucleotide polymorphism (cgSNP) analysis between 15 azithromycin-resistant clinical isolates and 10561 complete or draft genomic sequences of Salmonella enterica strains was performed to construct a maximum likelihood phylogenetic tree using Parsnp software (Kaas et al., 2014).This analysis was performed using the default parameters, and S. enterica subsp. enterica serovar Typhimurium str. LT2 (RefSeq ID: NC_003197.2) as the reference genome. Phylogenetic trees were visualized and annotated by the Interactive Tree of Life (iTOL) V5 web server (Letunic and Bork, 2021).
2.7 Statistical analysis
We adopted WHONET 5.6 software for data analysis, and the comparison of rates adopts χ (2) inspections.
3 Results
3.1 Clinical informations
A total of 15 Salmonella strains were detected in 13 children. If the time interval between the detection of Salmonella in a child exceeded three days, it was collected as a new strain and tested accordingly. The clinical, demographic, and laboratory characteristics of the 13 patients with azithromycin-resistant Salmonella infections are displayed in Table 1.
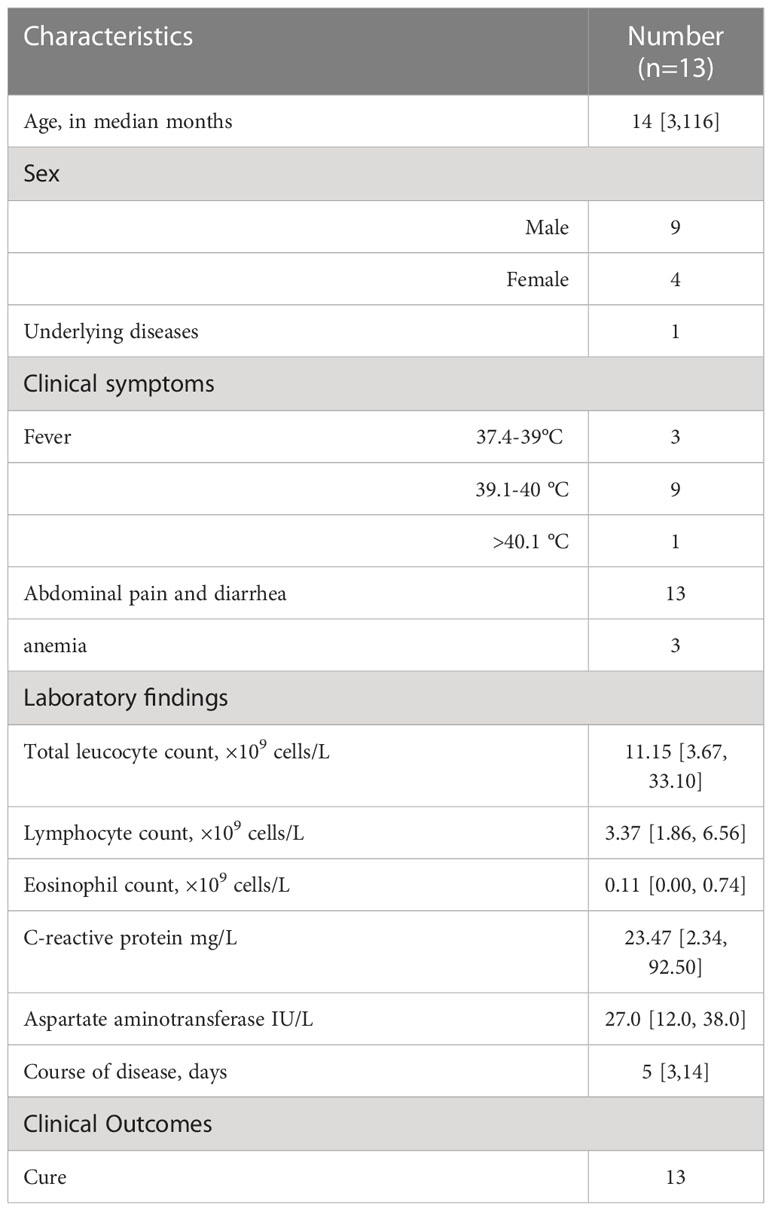
Table 1 General information and clinical features of pediatric patients with azithromycin-resistant Salmonella enteric.
3.2 Serotypes of azithromycin-resistant S. enterica
After routine drug sensitivity test screening of 487 retained S. enterica strains, 15 azithromycin-resistant S. enteric strains were detected. The serotype distribution was identified using traditional Salmonella serum, and the results of the data analysis after WGS and assembly are displayed in Figure 1. It was demonstrated four serotypes in the 15 Salmonella strains, including S.Stanley, S.London, S.Goldcoast or Brikama and S.I 4,[5],12:i:-.
3.3 Antimicrobial susceptibility profiles
Testing of the susceptibility of 15 Salmonella isolates (Table 2) to 6 antibiotics. Among these isolates, 100% (16/16) were resistant to AZM, while all isolates were also resistant to AMP. The resistance rates against SMZ, CL, CRO, and CIP were 87.3%, 80.0%, 46.7%, and 20.0%, respectively. For AZM, 26.67% of the isolates showed the highest MICs of 256 µg/mL, 20.0% showed MICs of 64 µg/mL, and 53.33% showed MICs of 32 µg/mL.
3.4 Phylogenetic analyses
In silico MLST analysis indicated that 15 isolates represented four sequence types, which were assigned to ST 29 (1/16), ST 34 (6/15), ST 155 (5/15), and ST 2529 (3/16) (Figure 1). We performed phylogenetic analysis of 15 S. enterica isolates and generated a phylogenetic tree with 68 strains and 3,067 SNP loci (Figure 2). The phylogenetic tree showed that the 15 self-tested strains clustered and formed four independent branches. The predominant ST type of the clade containing five samples is ST34; however, sample 1 is the rare ST type ST 29. The ST types of clades containing four and three samples were ST 155 and ST 2529, respectively. According to the results of phylogenetic analysis, the closest relative of ST34 isolates was identified in 2007 from a fecal sample in Australia, and the closest relative of ST34 isolates was identified in 2010 from a poultry small intenstine in Nigeria.
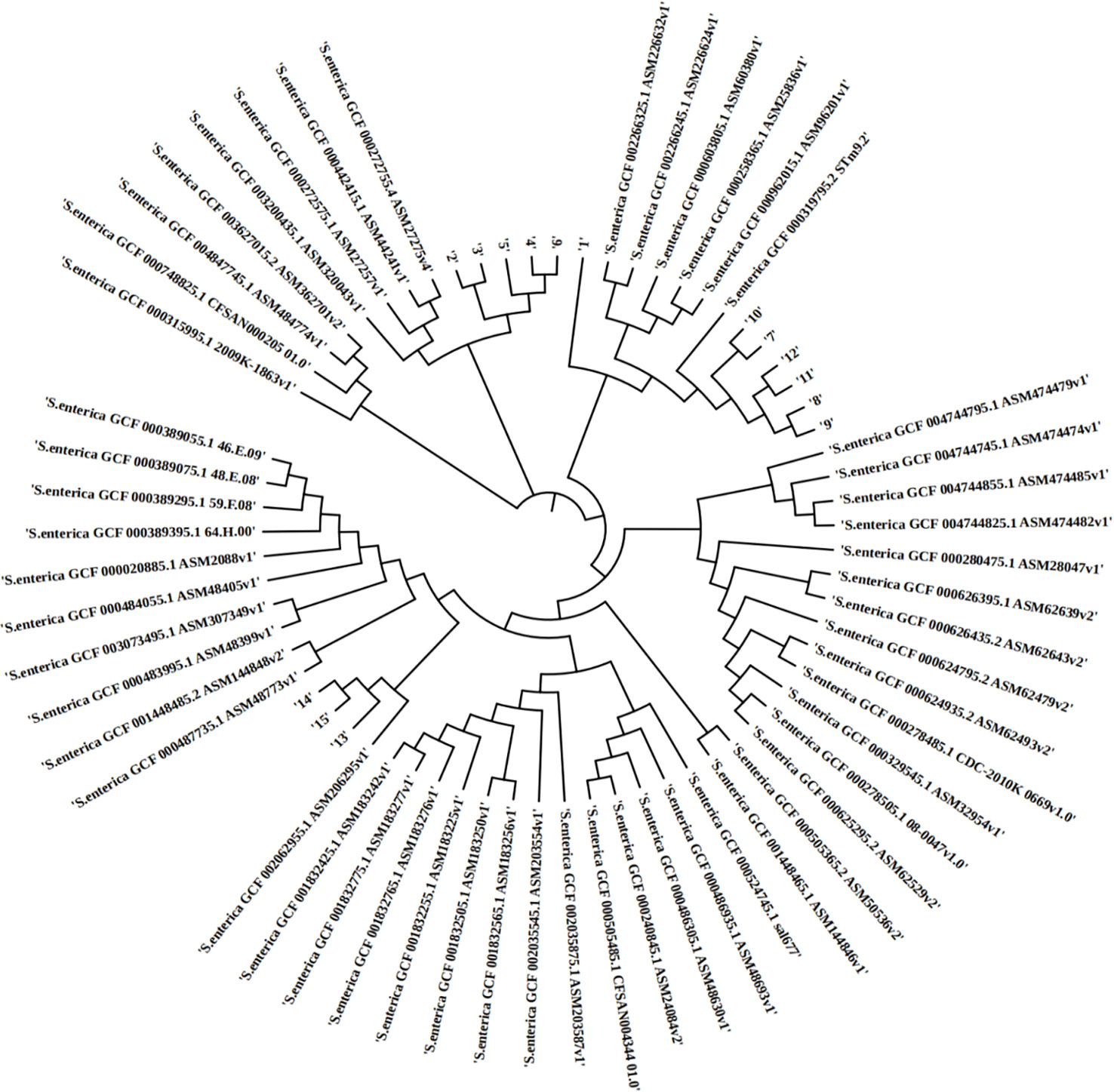
Figure 2 The phylopene relationship between 15 Salmonella enterica isolates and a total of 68 Salmonella enterica strains currently deposited in the NCBI GenBank database. The distance of SNPSs is represented by the branch length.
3.5 Genotypic characterization of antimicrobial resistance
We performed antimicrobial resistance gene analysis on 15 strains, which were extracted from whole genome sequencing (WGS) analysis. A total of 91 ARGs were found in the 15 isolates (Figures 3, 4), of which 39 ARGs were shared by the 15 isolates (Figure 3). The results shown in Figure 3 indicate that the 15 self-tested azithromycin-resistant strains all carried the azithromycin resistance gene mphA (Figure 3B), and the S. enterica_1 isolate carried two mphA genes. S. enterica_7 also carried the other azithromycin resistance gene ErmB. In addition, some CTX-M-type extended-spectrum beta-lactamase (ESBL) genes (blaCTX-M-14, blaCTX-M-55) and aminoglycoside resistance genes (APH(3’)-Ia, APH(3’’)-Ib, and APH(6)-Id) were identified among these isolates. Additional AMR genes (emr family genes, dfrA family genes and sul family) were also identified among these isolates. Overall, phenotypic resistance was highly correlated with the presence of known resistance determinants.
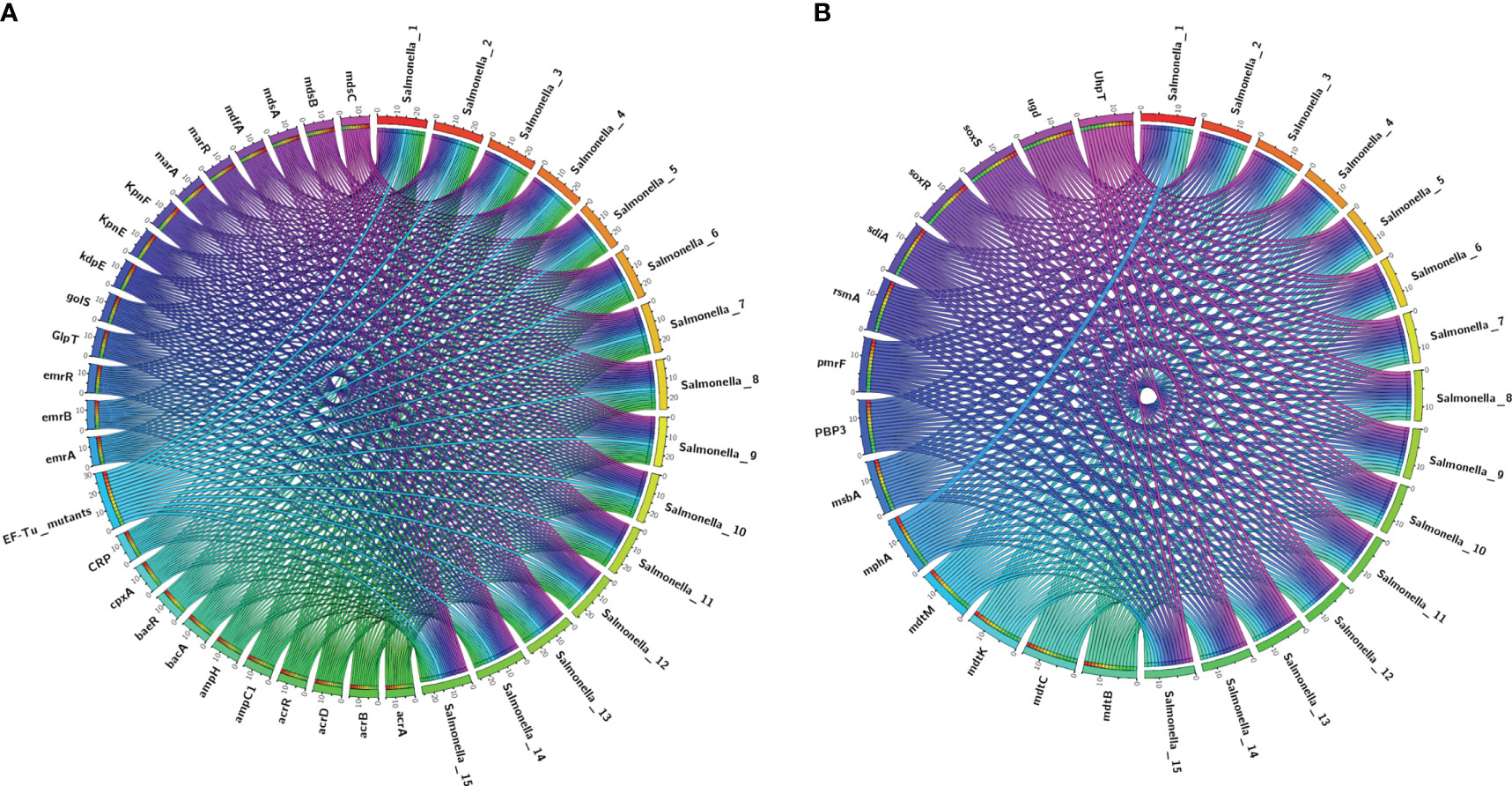
Figure 3 Detection of ARGs over the 15 plates. (A, B) Chord diagram illustrating the correlations between ARGs and the ARG-carrying pathogenic species. Thickness of the lines represents the number of samples observing such correlation. A total of 39 ARGs were shared by the 15 isolates.
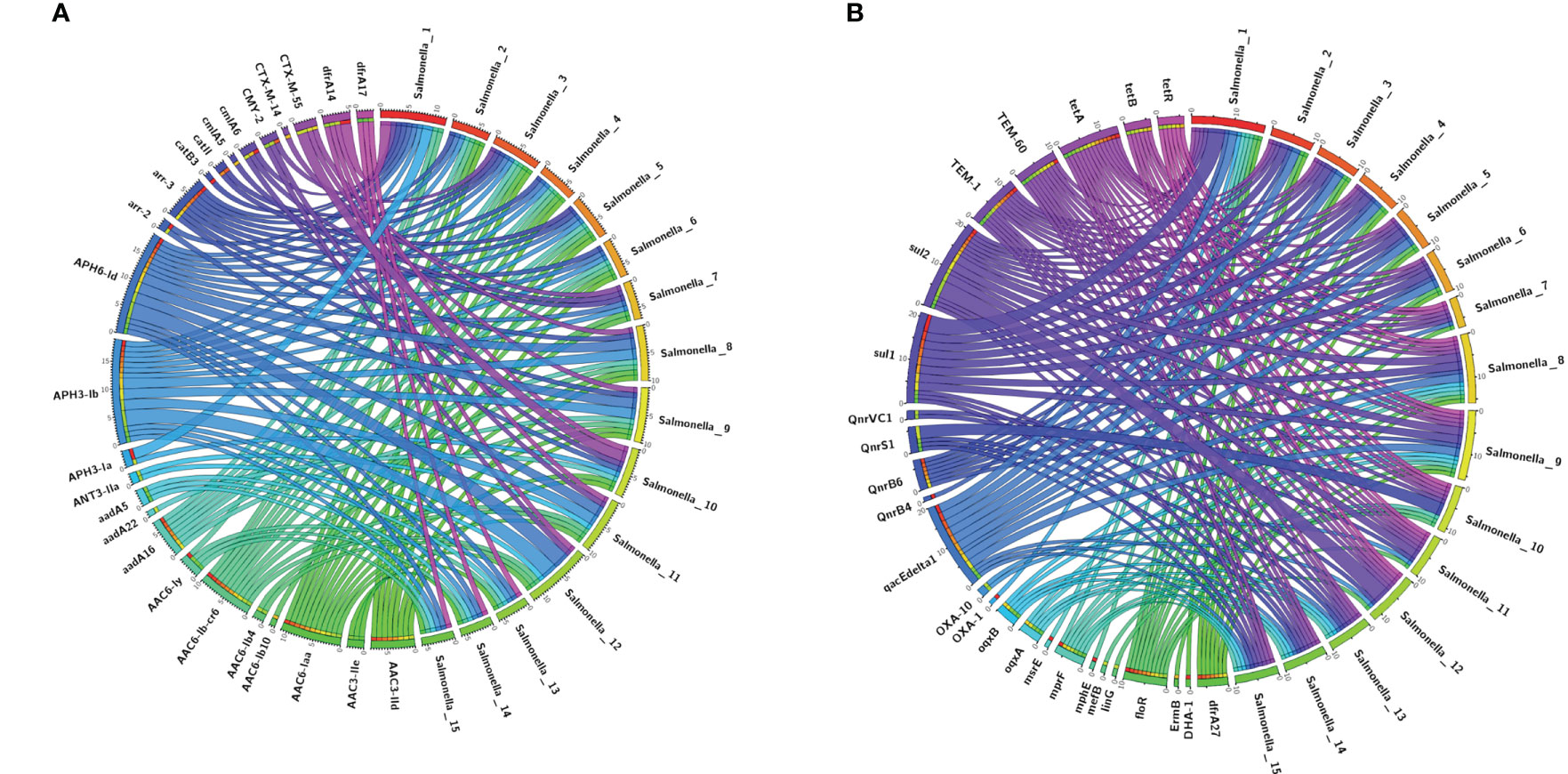
Figure 4 The upstream and downstream parts of the mphA resitance gene fragments shared the same backbone sequence containing genes.
3.6 Genetic characterization of mphA-carrying plasmid
Among the 15 isolates (S1-S15) with complete genome sequences, mphA was positive in all isolates and was located on several different plasmids。The upstream and downstream parts of the mphA resistance gene fragments shared the same backbone sequence containing genes for 2 mobile element protein genes, transcriptional regulator (TetR family), transcriptional regulator NanR, and the genetic structure of the mphA gene (Figure 5). Blast alignment showed that stable sequences containing mphA appeared in multiple plasmid structures, and the sequence identity was 100%.
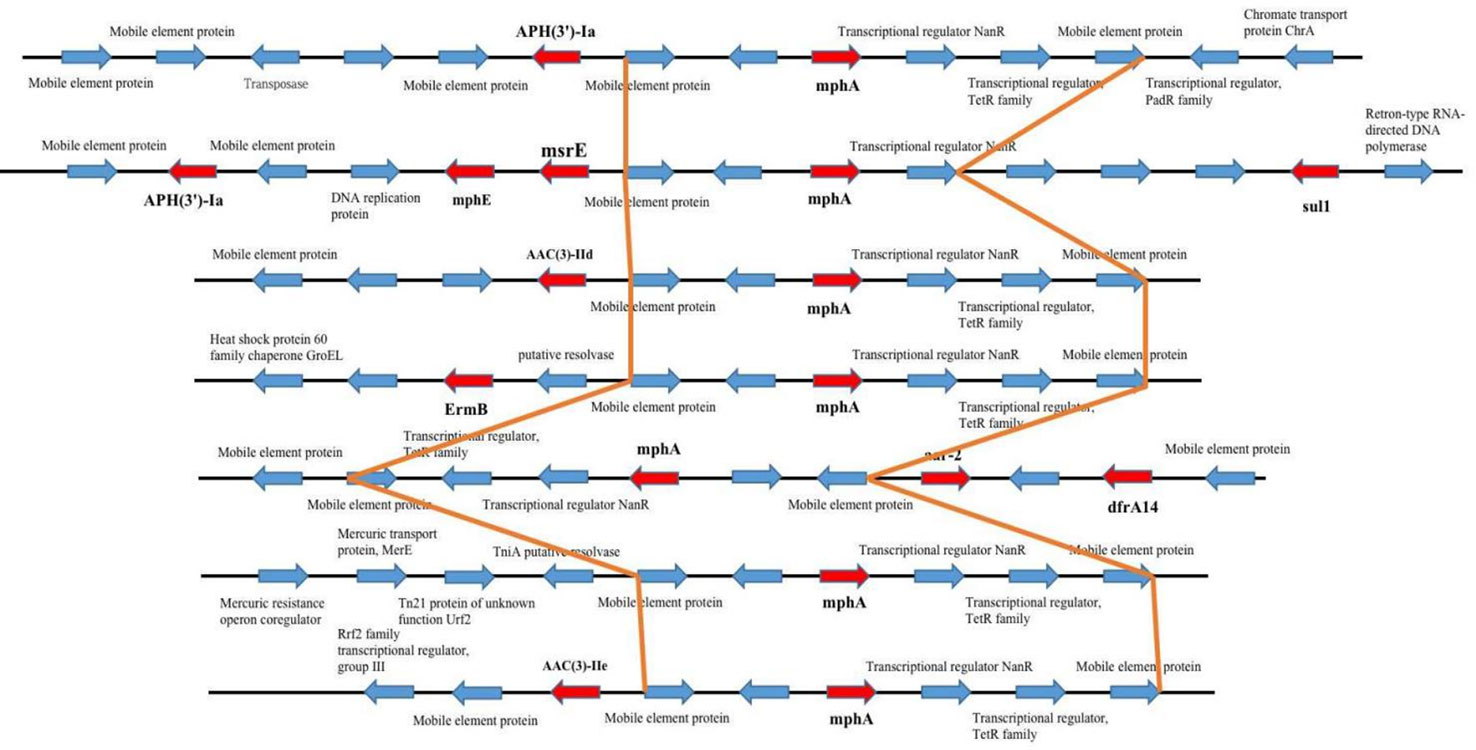
Figure 5 mph(A) were located on two different plasmids in S. enteric-7. (A) The large plasmid. (B) The small plasmid.
We used Blastn to compare the plasmid sequence in this study with that on the NCBI website, to find the closest homologous plasmid, the results are shown in the Table 3. Because the plasmid is not species-specific, lead to cannot form a complete phylogenetic tree containing all the plasmids in this study. Among the 15 isolates (S1-S15), we detected 8 diffrenet plasmids, and found cloest homologous plasmids to these eight plasmids(>82% coverage, >99.9% nucleotide sequence identity). 4 plasmids are homologous with plasmids from Salmonella spp., 2 plasmids are homologous with plasmids from Escherichia coli, 1 plasmid is homologous with plasmid from Klebsiella pneumoniae, and 1 plasmid is homologous with plasmid from Shigella flexneri. The plasmid carried is closely related to the phylogenetic aggregation of the strains. Strains with close branches carry the same plasmid, such as, strain S2-S6, strain S8-S9, strain S11-S12, strain S13-S15 (Figures 6–9).
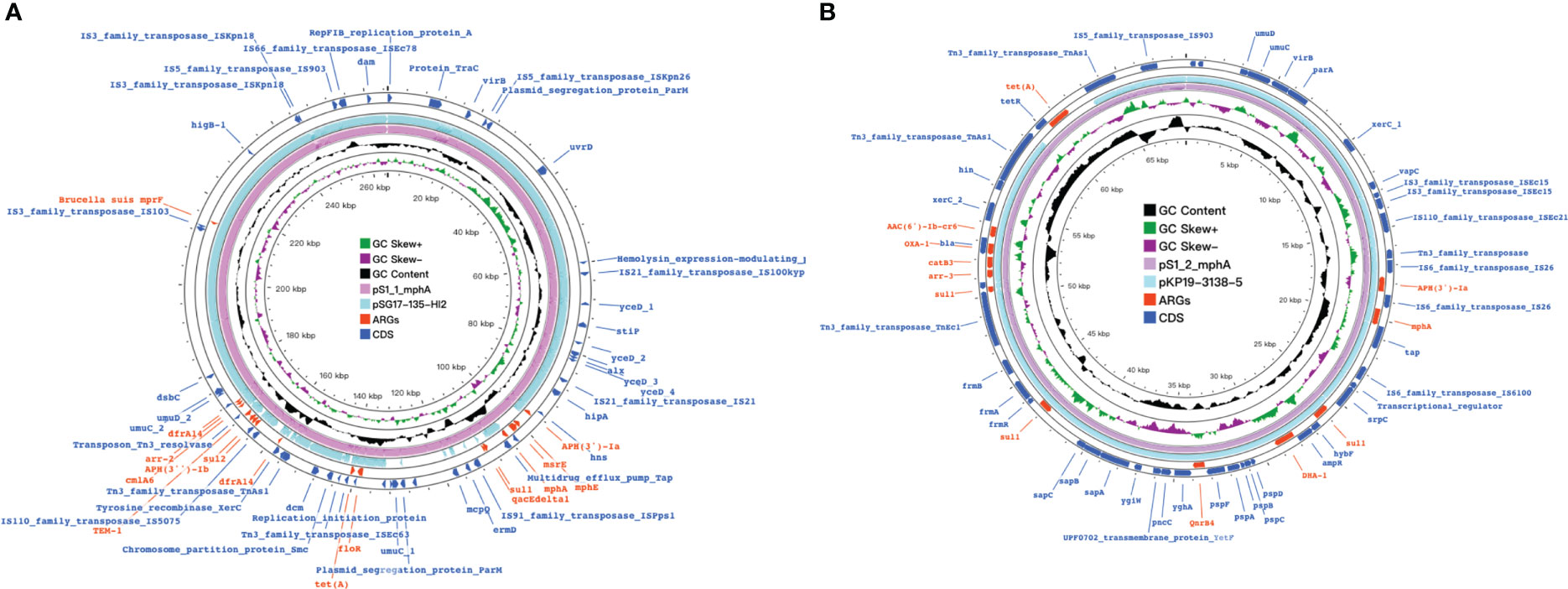
Figure 6 mph(A) were located on two different plasmids in S. enteric-2 (also include S.enteric-3, 4, 5, 6) and S. enteric-7. (A) The plasmid in S.enteric-2 (also include S.enteric-3, 4, 5, 6) (B) The plasmid in S.enteric-7.
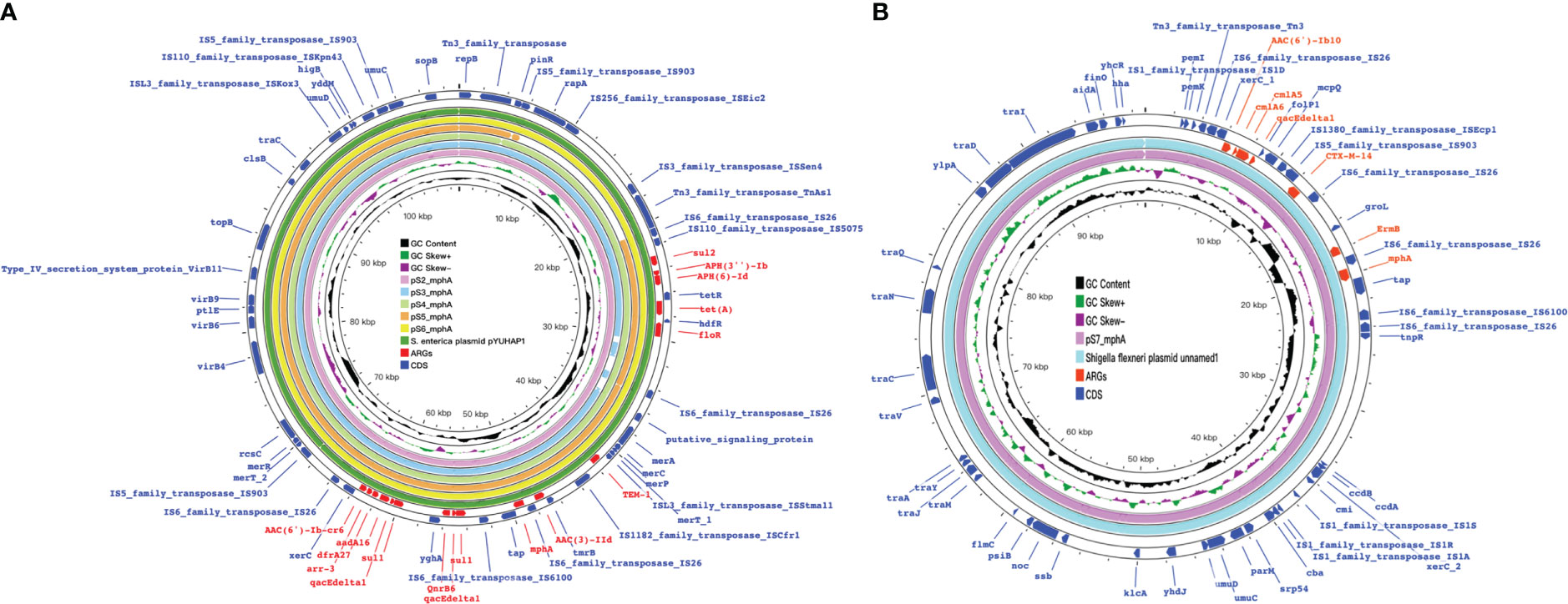
Figure 7 mph(A) were located on two different plasmids in S. enteric-8(also include S.enteric-9) and S.enteric-10. (A) The plasmid in S. enteric-8 (also include S. enteric-9) (B) The plasmid in S.enteric-10.
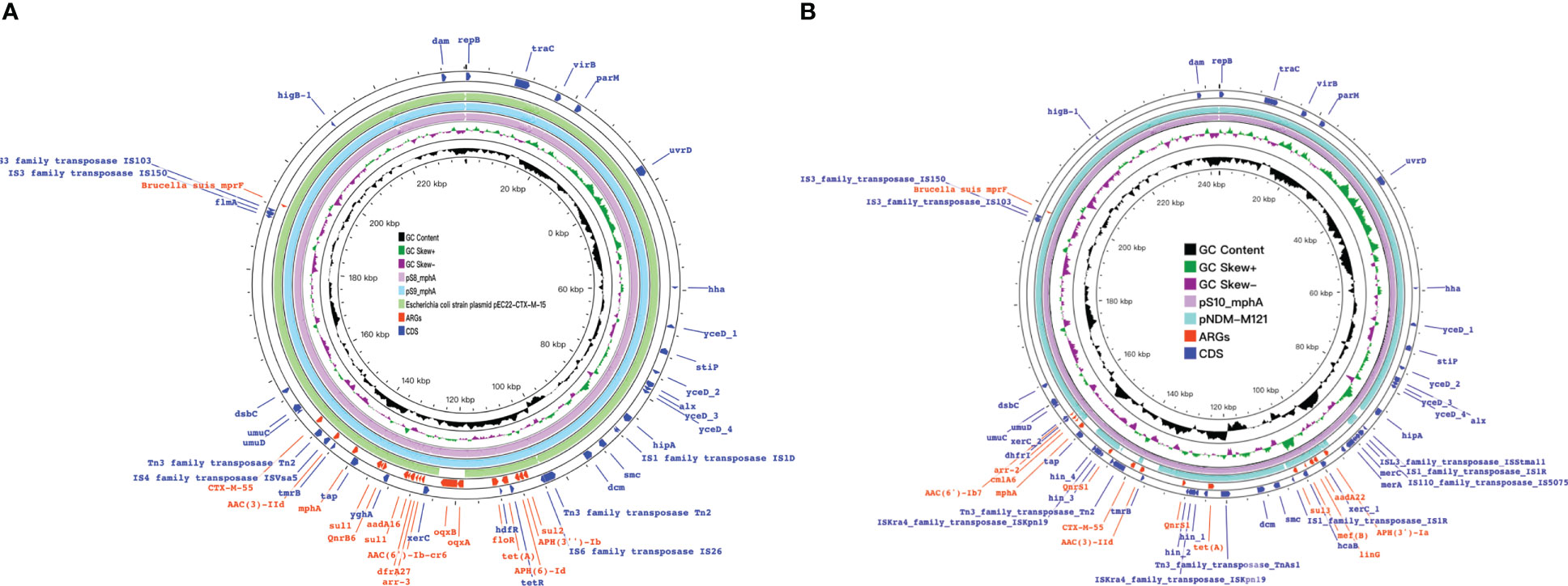
Figure 8 Detection of ARGs over the 15 isplates. (A, B) Chord diagram illustrating the correlations between ARGs and the ARG-carying pathogenic species. Thickness of the lines represents the number of samples observing such correlation. A total of 52 ARGs were not shared by the 15 isolates.
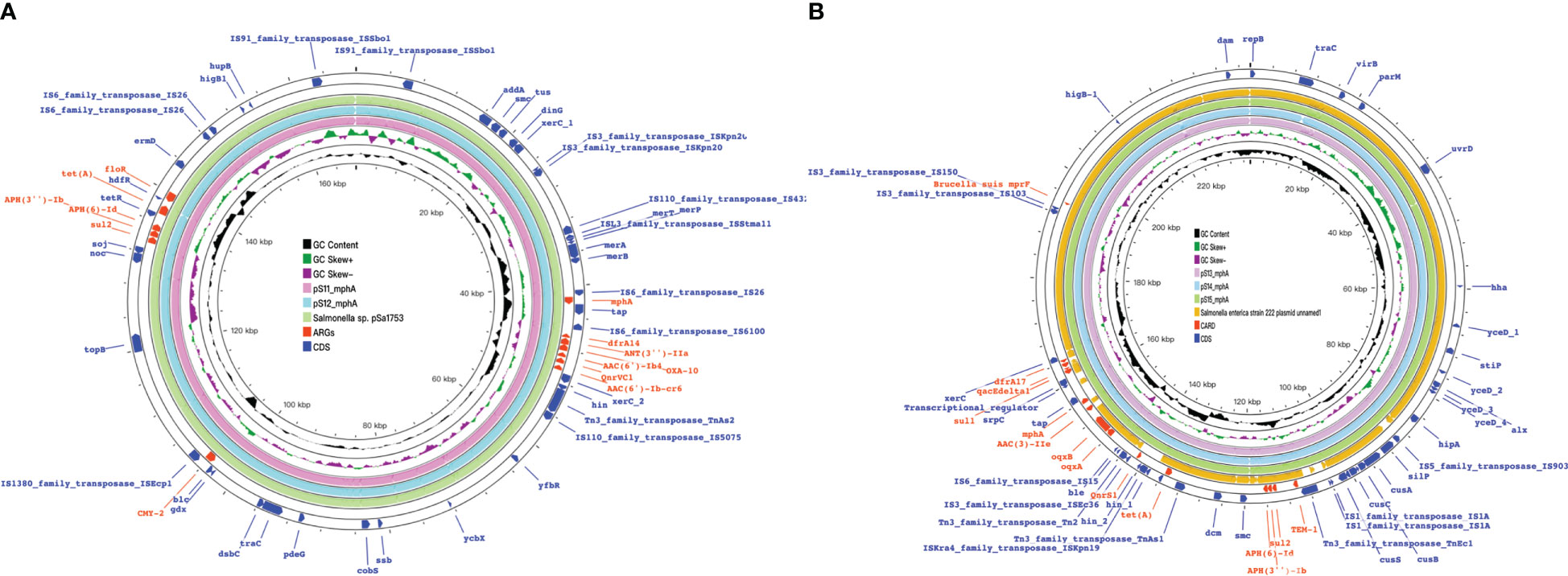
Figure 9 mph(A) were located on two different plasmids in S. enteric-11(also include S. enteric-12) and S.enteric-13(also include S.enteric-14, 15) (A) The plasmid in S.enteric-11(also include S.enteric-12) (B) S.enteric-13(also include S.enteric-14, 15).
The plasmids integrating multiple functional mobile elements and azithromycin resistance genes, which can be transferred without antibiotic selection pressure. Our plasmids sequence analysis indicates that the mphA-bearing IncX1 plasmids were hypothetically mobilizable and could move into the chromosome via insertion sequences such as IS21/IS26/ISVsa5/IS15. The typical IS26-mphA-tap transposition unit was embedded in the IncX1 plasmid and other MDR plasmids, such as IncHI2 (Figures 6, 7, 9). This highlights the pivotal role of IS26 and tap in the transmission of mphA among plasmids and chromosomes. Detailed analysis of mphA-bearing contigs in the 15 mphA-positive isolates showed that the core structure IS-mphA-tap (n = 15) and seven additional core structures were prevalent among these isolates (Figures 6–9). However, the complete structures around IS-mphA-tap were identified because of short and long fragmented assembled contigs based on Illumina short-read data and Nanopore long-read data.
4 Discussion
Azithromycin was discovered in 1980 by the Yugoslav pharmaceutical company Pliva and was on the List of Essential Medicines of World Health Organization’s List (Wang et al., 2020).
Similar to other macrolides, azithromycin mainly targets the P site of the ribosomal 50S subunit, one of the most conserved biomolecules in bacteria. At present, research on the mechanism of azithromycin resistance is increasing. Various reports on azithromycin-resistant Salmonella associated with mphA found that macrolide-2’-phosphotransferase encoded by mphA can mediate the increased resistance of NTS to azithromycin (Centers for Disease Control and Prevention(CDC)). In 2016, Nair Satheesh used WGS and drug resistance phenotype identification to determine the potential mechanism of azithromycin resistance of S.enteritidis isolates in the UK. Among 685 strains, 15 were resistant to azithromycin, and 12 encoded mphA (Nair et al., 2016). mphA-mediated azithromycin resistance has been reported in China.Among the 32 strains of azithromycin-resistant Salmonella typhimurium collected in Shanghai by Wang J et al (Wang et al, 2017).15 strains had MIC ≥ 128 μg/mL, from which mphA was detected.Hong et al. in Taiwan found that mphA existed in azithromycin-resistant Salmonella Typhi by identifying the drug resistance genes of serotypes of five strains from humans, pigs, and chickens (Hong et al., 2018). The emergence of mphA and its horizontal transmission ability therefore threaten the use of azithromycin for Salmonella infection. However, the mechanism of mphA level transfer is still unclear and needs to be further clarified using molecular epidemiology and comparative genomics.
This study demonstrated that mphA was detected in 15 azithromycin-resistant Salmonella strains, with no other azithromycin-related drug resistance gene detected, suggesting that the drug resistance gene is prevalent in Shenzhen Children’s Hospital. Among the 15 azithromycin-resistant Salmonella strains detected in 13 children, only one child had the basic disease (after neuroblastoma surgery) and was treated with special grade antibiotics (meropenem). One case was complicated with adenovirus infection with severe diarrhea symptoms. The antibiotics used were all third-generation cephalosporins. The treatment effect was good, and the patient was cured and discharged.
In this study, the correlation of the MIC with a resistance gene showed that the MIC ranged between 32 and 256 µg/mL among the study isolates and that the mphA gene was found in all S. enterica isolates. There are also reports of E. coli isolates with an MIC of ≥256 µg/mL carrying the mphA gene, followed by ermB and mphB in isolates with an MIC of >1024 and 128 µg/mL, respectively (Phuc Nguyen et al., 2009).
Generally, azithromycin-resistance genes such as mphA and ermB were reported to be carried in plasmids (Darton et al., 2018).Remarkably, in this study, the plasmid carrying the mphA gene was found to be carried in 8 different plasmids, which might be possible due to the presence of various insertion sequences and other mobile elements in S. enterica. Apart from this unique finding, genome analysis revealed the presence of multiple resistance genes that were expected. The genome also contained various mobile genetic elements that are reported to play a significant role in AMR dissemination in S. enterica (Yu et al., 2012).
An earlier study by Cho S showed that E. coli acts as a reservoir for macrolide-resistance genes from which resistant S. enterica might have emerged through horizontal gene transfer (Cho et al., 2019). This phenomenon has been previously demonstrated with E. coli donating mphA to S. sonnei (Phuc Nguyen et al., 2009). In this study, we looked for the occurrence of a similar event among the studied isolates. We compared the plasmid profile carried by S. enterica and E. coli carrying the macrolide resistance gene to identify the backbone similarity. Although the analysis revealed several genes in common, the S. enterica plasmid harbored an additional tra operon compared to E.coli, which might have been acquired due to evolution over time. The tra genes have always been the only genomic factors that make the plasmid conjugative. Interestingly, Benz et al. showed that plasmid transfer is mainly based on functional tra (transfer) genes rather than plasmid types (Benz et al., 2021). Furthermore, earlier studies have shown that plasmids carrying required functional tra genes can spread even without antibiotic selection pressure. These results highlight the potential risk of plasmids with resistance genes carrying functional tra genes being transferred by natural conjugation.
The widespread emergence of MDR S. enterica with changing AMR patterns has also been reported. Generally, in S. enterica, acquired resistance is more common. β-Lactam resistance is mainly due to the presence of OXA-type β-lactamases, followed by TEM and CTX-M. Trimethoprim/sulfamethoxazole resistance is encoded by the dhfr1A and sul genes. Quinolone resistance involves the accumulation of mutations in QRDR and plasmid-mediated quinolone resistance (PMQR) genes. Furthermore, resistance to tetracycline, chloramphenicol and streptomycin has been shown to be due to the presence of tetA/B, catB3 and aadA genes or both (Bustamante and Iredell, 2017; Wang et al, 2017; Wang et al., 2018). These results show the ability of species to acquire AMR determinants.
In conclusion, as the extensive resistance of salmonella serotypes to conventional first-line drugs, azithromycin have been designated as the most important antibiotics for the treatment of salmonella, the novel finding of an integrated plasmid in this study indicates the potential risk of S. enterica isolates becoming resistant to azithromycin in the future. Our study highlights the significance of the hybrid assembly approach in complete genome analysis. These findings suggest that it is imperative to monitor S. enterica susceptibility and to study the resistance mechanism of S. enterica against azithromycin, considering azithromycin is the only remaining oral drug for the treatment of XDR Salmonella infection.
Data availability statement
All clinical isolates sequence data used in the present study has been deposited in the NCBI database under project ID PRJNA879416.
Author contributions
QW and JD designed the experiments. HW, HC and BH performed the experiments. HW, HC, XH and YC analyzed the data. HW and HC wrote the manuscript. QW, JD, LY and LZ critically commented and revised the manuscript. All authors contributed to the article and approved the submitted version.
Funding
This work was supported by the Shenzhen Fund for Guangdong Provincial Highlevel Clinical Key Specialties (No. SZGSP012), the Guangdong Engineering Technology Research Center for accurate diagnosis of children’s infection (Platform funding, 2021B295), the Science Technology and Innovation Committee of Shenzhen (JCYJ20210324115408023), and the Science Technology and Innovation Committee of Shenzhen (JCJY20180302144721183).
Conflict of interest
The authors declare that the research was conducted in the absence of any commercial or financial relationships that could be construed as a potential conflict of interest.
Publisher’s note
All claims expressed in this article are solely those of the authors and do not necessarily represent those of their affiliated organizations, or those of the publisher, the editors and the reviewers. Any product that may be evaluated in this article, or claim that may be made by its manufacturer, is not guaranteed or endorsed by the publisher.
References
Alcock, B. P., Raphenya, A. R., Lau, T., Tsang, K. K., Bouchard, M., Edalatmand, A., et al. (2020). CARD 2020: Antibiotic resistome surveillance with the comprehensive antibiotic resistance database. Nucleic Acids Res. 48, D517–517D525. doi: 10.1093/nar/gkz935
Alikhan, N. F., Petty, N. K., Ben Zakour, N. L., Beatson, S. A. (2011). BLAST ring image generator (BRIG): Simple prokaryote genome comparisons. BMC Genomics 12, 402. doi: 10.1186/1471-2164-12-402
Centers for Disease Control and Prevention (CDC). (2013). Notes from the field: Multistate outbreak of human salmonella typhimurium infections linked to contact with pet hedgehogs - united states, 2011-2013. MMWR Morb Mortal Wkly Rep. 62, 73.
Benz, F., Huisman, J. S., Bakkeren, E., Herter, J. A., Stadler, T., Ackermann, M., et al. (2021). Plasmid- and strain-specific factors drive variation in ESBL-plasmid spread. Vitro vivo ISME J. 15, 862–878. doi: 10.1038/s41396-020-00819-4
Bustamante, P., Iredell, J. R. (2017). Carriage of type II toxin-antitoxin systems by the growing group of IncX plasmids. Plasmid. 91, 19–27. doi: 10.1016/j.plasmid.2017.02.006
Camacho, C., Coulouris, G., Avagyan, V., Ma, N., Papadopoulos, J., Bealer, K., et al. (2009). BLAST+: Architecture and applications. BMC Bioinf. 10, 421. doi: 10.1186/1471-2105-10-421
Carattoli, A., Zankari, E., García-Fernández, A., Voldby Larsen, M., Lund, O., Villa, L., et al. (2014). In silico detection and typing of plasmids using PlasmidFinder and plasmid multilocus sequence typing. Antimicrob. Agents Chemother. 58, 3895–3903. doi: 10.1128/AAC.02412-14
Carey, M. E., Jain, R., Yousuf, M., Maes, M., Dyson, Z. A., Thu, T., et al. (2021). Spontaneous emergence of azithromycin resistance in independent lineages of salmonella typhi in northern India. Clin. Infect. Dis. 72, e120–120e127. doi: 10.1093/cid/ciaa1773
Chiou, C. S., Hong, Y. P., Wang, Y. W., Chen, B. H., Teng, R. H., Song, H. Y., et al. (2023). Antimicrobial resistance and mechanisms of azithromycin resistance in nontyphoidal salmonella isolates in Taiwan, 2017 to 2018. Microbiol. Spectr. 11, e0336422. doi: 10.1128/spectrum.03364-22
Cho, S., Nguyen, H., McDonald, J. M., Woodley, T. A., Hiott, L. M., Barrett, J. B., et al. (2019). Genetic characterization of antimicrobial-resistant escherichia coli isolated from a mixed-use watershed in northeast Georgia, USA. Int. J. Environ. Res. Public Health 16, 3761. doi: 10.3390/ijerph16193761
Clinical and Laboratory Standards Institute[CLSI] (2021). Performance standads for antimicrobial susceptibility testing. 31st edn (Wayne, PA: Clinical and Laboratory Standards Institute).
Darton, T. C., Tuyen, H. T., The, H. C., Newton, P. N., Dance, D., Phetsouvanh, R., et al. (2018). Azithromycin resistance in shigella spp. Southeast Asia Antimicrob. Agents Chemother. 62, e01748–17. doi: 10.1128/AAC.01748-17
Gilchrist, J. J., MacLennan, C. A., Hill, A. V. (2015). Genetic susceptibility to invasive salmonella disease. Nat. Rev. Immunol. 15, 452–463. doi: 10.1038/nri3858
Guarino, A., Ashkenazi, S., Gendrel, D., Lo Vecchio, A., Shamir, R., Szajewska, H. (2014). European Society for pediatric gastroenterology, hepatology, and Nutrition/European society for pediatric infectious diseases evidence-based guidelines for the management of acute gastroenteritis in children in Europe: update 2014. J. Pediatr. Gastroenterol. Nutr. 59, 132–152. doi: 10.1097/MPG.0000000000000375
Hong, Y. P., Wang, Y. W., Huang, I. H., Liao, Y. C., Kuo, H. C., Liu, Y. Y., et al. (2018). Genetic relationships among multidrug-resistant salmonella enterica serovar typhimurium strains from humans and animals. Antimicrob. Agents Chemother. 62, e00213–18. doi: 10.1128/AAC.00213-18
James, H., Pfaller, M. A., Karen, C., Carroll, K. (2015). Manual of clinical microbiology. 11thedition (USA: ASM Press). Jorgensen.
Kaas, R. S., Leekitcharoenphon, P., Aarestrup, F. M., Lund, O. (2014). Solving the problem of comparing whole bacterial genomes across different sequencing platforms. PloS One 9, e104984. doi: 10.1371/journal.pone.0104984
Larsen, M. V., Cosentino, S., Rasmussen, S., Friis, C., Hasman, H., Marvig, R. L., et al. (2012). Multilocus sequence typing of total-genome-sequenced bacteria. J. Clin. Microbiol. 50, 1355–1361. doi: 10.1128/JCM.06094-11
Letunic, I., Bork, P. (2021). Interactive tree of life (iTOL) v5: an online tool for phylogenetic tree display and annotation. Nucleic Acids Res. 49, W293–293W296. doi: 10.1093/nar/gkab301
Li, R., Xie, M., Dong, N., Lin, D., Yang, X., Wong, M., et al. (2018). Efficient generation of complete sequences of MDR-encoding plasmids by rapid assembly of MinION barcoding sequencing data. Gigascience. 7, 1–9. doi: 10.1093/gigascience/gix132
Ma, K., Feng, Y., Liu, L., Yao, Z., Zong, Z. (2020). A cluster of colistin- and carbapenem-resistant klebsiella pneumoniae carrying blaNDM-1 and mcr-8.2. J. Infect. Dis. 221, S237–237S242. doi: 10.1093/infdis/jiz519
Nair, S., Ashton, P., Doumith, M., Connell, S., Painset, A., Mwaigwisya, S., et al. (2016). WGS for surveillance of antimicrobial resistance: A pilot study to detect the prevalence and mechanism of resistance to azithromycin in a UK population of non-typhoidal salmonella. J. Antimicrob. Chemother. 71, 3400–3408. doi: 10.1093/jac/dkw318
Nair, S., Chattaway, M., Langridge, G. C., Gentle, A., Day, M., Ainsworth, E. V., et al. (2021). ESBL-producing strains isolated from imported cases of enteric fever in England and Wales reveal multiple chromosomal integrations of blaCTX-M-15 in XDR salmonella typhi. J. Antimicrob. Chemother. 76, 1459–1466. doi: 10.1093/jac/dkab049
Phuc Nguyen, M. C., Woerther, P. L., Bouvet, M., Andremont, A., Leclercq, R., Canu, A. (2009). Escherichia coli as reservoir for macrolide resistance genes. Emerg. Infect. Dis. 15, 1648–1650. doi: 10.3201/eid1510.090696
Plumb, I. D., Schwensohn, C. A., Gieraltowski, L., Tecle, S., Schneider, Z. D., Freiman, J., et al. (2019). Outbreak of salmonella Newport infections with decreased susceptibility to azithromycin linked to beef obtained in the united states and soft cheese obtained in Mexico - united states, 2018-2019. MMWR Morb Mortal Wkly Rep. 68, 713–717. doi: 10.15585/mmwr.mm6833a1
Seemann, T. (2014). Prokka: rapid prokaryotic genome annotation. Bioinformatics. 30, 2068–2069. doi: 10.1093/bioinformatics/btu153
Walker, B. J., Abeel, T., Shea, T., Priest, M., Abouelliel, A., Sakthikumar, S., et al. (2014). Pilon: an integrated tool for comprehensive microbial variant detection and genome assembly improvement. PloS One 9, e112963. doi: 10.1371/journal.pone.0112963
Wang, C. N., Huttner, B. D., Magrini, N., Cheng, Y., Tong, J., Li, S., et al. (2020). Pediatric antibiotic prescribing in China according to the 2019 world health organization access, watch, and reserve (AWaRe) antibiotic categories. J. Pediatr. 220, 125–31.e5. doi: 10.1016/j.jpeds.2020.01.044
Wang, J., Li, Y., Xu, X., Liang, B., Wu, F., Yang, X., et al. (2017). Antimicrobial resistance of salmonella enterica serovar typhimurium in shanghai, China. Front. Microbiol. 8. doi: 10.3389/fmicb.2017.00510
Wang, Y., Tian, G. B., Zhang, R., Shen, Y., Tyrrell, J. M., Huang, X., et al. (2017). Prevalence, risk factors, outcomes, and molecular epidemiology of mcr-1-positive enterobacteriaceae in patients and healthy adults from China: an epidemiological and clinical study. Lancet Infect. Dis. 17, 390–399. doi: 10.1016/S1473-3099(16)30527-8
Wang, Y., Tong, M. K., Chow, K. H., Cheng, V. C., Tse, C. W., Wu, A. K., et al. (2018). Occurrence of highly conjugative IncX3 epidemic plasmid carrying bla (NDM) in enterobacteriaceae isolates in geographically widespread areas. Front. Microbiol. 9. doi: 10.3389/fmicb.2018.02272
Wick, R. R., Judd, L. M., Gorrie, C. L., Holt, K. E. (2017). Unicycler: Resolving bacterial genome assemblies from short and long sequencing reads. PloS Comput. Biol. 13, e1005595. doi: 10.1371/journal.pcbi.1005595
World Health Organization (2018) Salmonella(non-typhoidal). Available at: http://www.int/news-room/fact-sheets/detail/salmonella-(non-typhoidal).
Keywords: azithromycin, mphA gene, plasmid, children, Salmonella enterica
Citation: Wang H, Cheng H, Huang B, Hu X, Chen Y, Zheng L, Yang L, Deng J and Wang Q (2023) Characterization of resistance genes and plasmids from sick children caused by Salmonella enterica resistance to azithromycin in Shenzhen, China. Front. Cell. Infect. Microbiol. 13:1116172. doi: 10.3389/fcimb.2023.1116172
Received: 05 December 2022; Accepted: 15 March 2023;
Published: 29 March 2023.
Edited by:
Yonghong Yang, Beijing Children’s Hospital, Capital Medical University, ChinaReviewed by:
Ben Pascoe, University of Oxford, United KingdomWenen Liu, Xiangya Hospital, Central South University, China
Copyright © 2023 Wang, Cheng, Huang, Hu, Chen, Zheng, Yang, Deng and Wang. This is an open-access article distributed under the terms of the Creative Commons Attribution License (CC BY). The use, distribution or reproduction in other forums is permitted, provided the original author(s) and the copyright owner(s) are credited and that the original publication in this journal is cited, in accordance with accepted academic practice. No use, distribution or reproduction is permitted which does not comply with these terms.
*Correspondence: Qian Wang, d2FuZ3FpYW5femp5eUAxMjYuY29t; Jikui Deng, c3pzZXR5eWRlbmdAc2luYS5jb20=; Liang Yang, eWFuZ2xAc3VzdGVjaC5lZHUuY24=; Lei Zheng, bmZ5eXpsQDE2My5jb20=
†These authors share first authorship