- Department of Dermatology, Zhongnan Hospital of Wuhan University, Wuhan, Hubei, China
Cryptococcus is the causal agent of cryptococcosis, a disease with high mortality mainly related to HIV immunosuppression and usually manifests with pneumonia and/or meningoencephalitis. There are very few therapeutic options; thus, innovative approaches are required. Herein, We examined the interaction of everolimus (EVL) with amphotericin B (AmB) and azoles [fluconazole (FLU), posaconazole (POS), voriconazole (VOR), itraconazole (ITR)] against Cryptococcus. Eighteen Cryptococcus neoforman clinical isolates were analyzed. Following the guidelines of the Clinical and Laboratory Standards Institute (CLSI) M27-A4, we conducted a broth microdilution experiment to determine the minimum inhibitory concentrations (MICs) of azoles, EVL, and AmB for assessing antifungal susceptibility. A fractional inhibitory concentration index (FICI) of less than and equal to 0.5 indicated synergy, with a range of 0.5 to 4.0 indicated indifference and a value more than 4.0 indicated antagonism. These experiments revealed that EVL had antifungal activity against C. neoforman. Moreover, EVL, POS, AmB, FLU, ITR, and VOR exhibited MIC values ranging from 0.5-2 μg/mL, 0.03125-2 μg/mL, 0.25-4 μg/mL, 0.5-32μg/mL, 0.0625-4μg/mL and 0.03125-2μg/mL, respectively. The combination of EVL with AmB and azoles (POS, FLU, ITR, and VOR) exhibited synergistic antifungal effects against 16 (88.9%), 9 (50%), 11 (61.1%), 10 (55.6%) or 6 (33.3%) of analyzed Cryptococcus strains. In the presence of EVL, the MIC values of AmB and azoles were significantly lowered. No antagonism was observed. Subsequently, in vivo analyses conducted using the G. mellonella model further confirmed that combination EVL+ POS, EVL+ FLU, and EVL+ITR treatment were associated with significantly improved larval survival following Cryptococcus spp. infection. These findings provide the first published evidence suggesting that a combination of EVL and AmB or azoles exhibit a synergistic effect and may be an effective antifungal disease treatment strategy for infections caused by Cryptococcus spp.
Introduction
Cryptococcosis generally occurs as an opportunistic infection in immunocompromised hosts. Patients with acquired immune deficiency syndrome (AIDS) or other immune deficiencies are especially at risk (Pappas et al., 2001; Singh and Forrest, 2009; DiNardo et al., 2015; Henao-Martínez and Beckham, 2015). Cryptococcus, as the causative agent of cryptococcosis, is found in various environmental sources, including contaminated milk, birds droppings, and soil. Pneumonia, meningitis, skin, soft tissue, bone, and joint infections (Cho et al., 2021) are typical cryptococcal symptoms; however, the infection may spread to other organs via the lymphatic system or the circulatory system.
Globally, cryptococcosis is one of the deadliest invasive mycoses due to its high morbidity and death rate (Perfect et al., 2010). About 200,000 individuals a year are killed by pathogenic species of Cryptococcus (Fonseca et al., 2019). Standard therapy is a typically an aggressive intravenous injection of an antifungal drug, followed by suppressive treatment taken orally for a period that varies depending on the patient’s condition (Cho et al., 2021). Amphotericin B (AmB) (typically lipid formulations) plus 5-fluorocytosine (5FC) for induction treatment for 2 weeks, followed by fluconazole as suppressive therapy, is the recommended treatment (Perfect et al., 2010; Cho et al., 2021). However, the high dosages needed for these infections, the severe toxicities of AmB have been a limiting factor in its use (Thakur and Revankar, 2011). Furthermore, in regions with higher disease load and death rates, the availability of 5-FC is limited (Maziarz and Perfect, 2016). Cryptococcosis remains a challenging management issue. Combination therapy with drug repositioning has been seen as a potential option due to the scarcity of novel antifungal medicines.
Everolimus (EVL), an analog of the naturally occurring macrolide rapamycin, is an orally bioactive inhibitor of the mammalian target of rapamycin (mTOR) serine/threonine kinase signal transduction pathway, which controls proliferation, cell growth, and survival. Its ability to directly inhibit tumor proliferation and cell growth and indirectly impede angiogenesis has garnered much interest as an anticancer drug (Hasskarl, 2018). TOR was first discovered in the yeast Saccharomyces cerevisiae, and subsequent research has shown that it is present in many other eukaryotic organisms, including plants, worms, flies, fungi, humans, and mammals (Crespo and Hall, 2002). Immunosuppressive medicines increase the likelihood of developing invasive fungal infections, although they also have antifungal activity. Rapamycin has shown intrinsic antifungal activity against Candida albicans, Microsporum gypseum, Trichophyton granulosum (Vézina et al., 1975), Aspergillus spp.(High and Washburn, 1997), Fusarium fujikuroi(Teichert et al., 2006)and cryptococcus neoformans(Cruz et al., 1999). Moreover, Manish Thakur et al. demonstrated a synergistic effect of caspofungin with rapamycin against Glomeromycetes(Thakur and Revankar, 2011). In addtion, Patrick Schwarz et al. observed that rapamycin may improve the efficacy of isavuconazole against Aspergillus species in vitro (Schwarz and Dannaoui, 2020). Despite this, EVL’s effectiveness against Cryptococcus has only been partially studied by itself or in conjunction with other treatments.
The rapalog effect of EVL against clinical Cryptococcus isolates was validated in vitro using AmB and azoles. To further investigate the potential treatment-related changes in larval survival, we extended these experiments to evaluate the effects of combination EVL+AmB or azoles therapy on Galleria mellonella larvae infected with Cryptococcus spp.
Materials and methods
Fungal strains and preparation of conidia
18 clinical Cryptococcus neoformans isolates were studied in total (Table 1). Microscopic examination, and the internal transcribed spacer (ITS) ribosomal DNA (rDNA) sequencing and D1/D2 were used to confirm Cryptococcus spp. identification. Cryptococcus conidia were collected by flooding the culture surface with phosphate-buffered saline (PBS) after being cultured at 37°C for 2 days on Potato dextrose agar (PDA) and then counted using a hemocytometer.
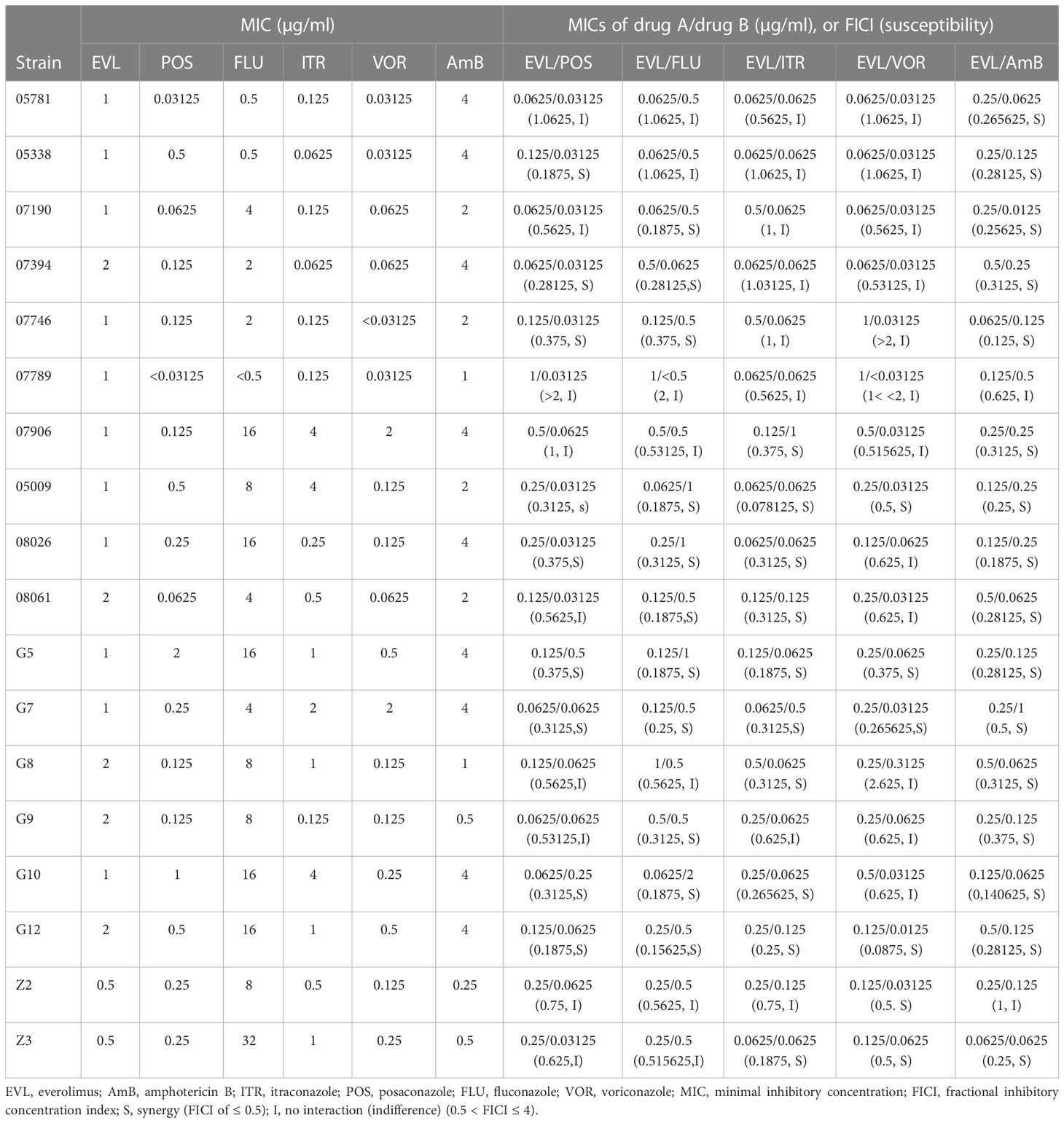
Table 1 MICs and FICIs results with combinations of Everolimus with antifungal agents against Cryptococcus neoformans.
Antifungals and chemical agents
The drugs, including posaconazole (POS; purity ≥ 99%), itraconazole (ITR; purity ≥ 99%), fluconazole (FLU; purity ≥ 99%), voriconazole (VOR; purity ≥ 99%), and amphotericin B (AmB; purity ≥ 80%) were bought form from SelleckChemicals, TX, USA, in powder form. Moreover, Everoliums (EVL; purity ≥ 99%) were purchased from Shanghai Yeasen Biotechnology Co., Ltd., China. All tested agents were diluted using dimethyl sulfoxide (DMSO) as stock solutions (EVL, 6600 μg/ml; azoles and AmB, 6400 μg/ml).
Broth microdilution assay
All 18 of the aforementioned Cryptococcus isolates and Candida parapsilosis (ATCC 22019) were used to assess the effects of EVL alone and in combination with azoles and AmB. All susceptibility testing for Cryptococcus spp. was performed per Clinical and Laboratory Standards Institute document M27-A4 (Clinical and Laboratory Standards Institute, 2017).The MICs were calculated as the concentrations required to limit growth by 50% (azoles) and 100% (AmB) (Clinical and Laboratory Standards Institute, 2017). The MIC values for EVL, POS, AmB, FLU, ITR and VOR were determined separately before any combination experiments were conducted. A total of 100μl of the inoculum suspension and 100μl of serial diluent of test drugs were used to inoculate a 96-well plate. Results were interpreted after incubation at 35°C for 48h for Cryptococcus spp. All tests were performed in duplicate.
Furthermore, the microdilution chequerboard method was used to investigate EVL’s interactions with antifungal drugs against all strains. On a 96-well plate containing 100 μl of prepared inoculum suspension, 50 μl of EVL with serial dilutions were inoculated horizontally, and 50μl of azoles or AmB with serial dilutions were inoculated vertically, as specified. Results were interpreted after incubation at 35°C for 48h for Cryptococcus spp.
FICI was determined to characterize the interaction of EVL with azoles, or AmB as follows: FICI= (Ac/Aa) +(Bc/Ba), where Ac and Bc are the MIC values for the combination of these medications and Aa and Ba are the MIC values for the drugs when taken alone. FICI of ≤ 0.5, synergy; FICI of 0.5 to ≤ 4, indifference; and FICI of > 4, antagonism (Odds, 2003). All analyses were performed in duplicate.
Galleria mellonella assay
According to the methods described previously (Mylonakis et al., 2005; Amorim-Vaz et al., 2015; Liu et al., 2017), G. mellonella larvae were used and split into 14 different experimental
groups: untreated (noninfected larvae hat received no treatments), saline (10 µL saline injected noninfected larvae), conidial (C. neoforman-infected larvae), POS (200 μg/mL)-treated (treatment of C. neoforman-infected larvae with POS), ITR (200 μg/mL)-treated (treatment of C. neoforman- infected larvae with ITR), VOR (200 μg/mL)-treated (treatment of C. neoforman-infected larvae with VOR), FLU (200 μg/mL)-treated (C. neoforman-infected larvae treated with FLU), EVL (200 μg/mL)-treated (C. neoforman-infected larvae treated with EVL), AmB (200 μg/mL)-treated (C. neoforman-infected larvae treated with AmB), and POS (200 μg/mL) + EVL (200 μg/mL)-treated (treatment of C. neoforman-infected larvae with POS and EVL) groups, ITR (200 μg/mL) + EVL (200 μg/mL)-treated (treatment of C. neoforman -infected larvae with ITR and EVL) groups, VOR (200 μg/mL) + EVL (200 μg/mL)-treated (treatment of C. neoforman-infected larvae with VOR and EVL) groups, FLU (200 μg/mL) + EVL (200 μg/mL)-treated (treatment of C. neoforman-infected larvae with FLU and EVL) groups, AmB(200 μg/mL) + EVL (200 μg/mL)-treated (treatment of C. neoforman-infected larvae with AmB and EVL) groups. Each experimental group had 20 larvae (weighing between 0.3 and 0.4 g) included and the tests were conducted three times. All in vivo studies used a single C. neoformans isolate (G7). C. neoformans G7 conidia were counted using a hemocytometer at 106 CFU/ml after a 2-day culture on PDA at 28°C, after which the agar was rinsed with PBS. After incubating G. mellonella larvae at 37°C for 2 hours, all groups except the untreated and saline control groups received an injection of 10 µL of a conidial solution and were treated with appropriate antifungal medicines (5 µl). The larvae were placed in a 37°C incubator and inspected once a day for six days to determine their survival rate.
Statistical analysis
The statistical analysis and figure preparation were done using GraphPad Prism 5.0. Kaplan-Meier curves and log-rank (Mantel-Cox) tests were used to analyze the survival data for G. mellonella at a significance level of P < 0.05.
Results
In vitro antifungal activity of the individual tested agent
The MIC ranges of the individual tested agents against Cryptococcus isolates were 0.5-2 µg/ml for EVL, 0.03125-2µg/ml for POS, 0.25-4µg/ml for AmB, 0.5-32µg/ml for FLU, 0.0625-4 µg/ml for ITR and 0.03125-2µg/ml for VOR (Table 1). EVL individually showed a substantial antifungal effect against all tested strains of Cryptococcus spp.
In vitro interactions between EVL and AmB or azoles against C.neoformans
Synergistic effects against 9 (50%) strains of C. neoformans were shown when EVL was combined with POS, and the MICs of EVL and POS against Cryptococcus spp. were reduced to 0.0625-1 μg/ml and 0.03125-0.5 μg/ml, respectively (Table 1).
The MICs of EVL and FLU against Cryptococcus spp. were reduced to 0.0625-1 μg/ml and 0.0625-2 μg/ml, respectively, when used in combination (Table 1). The synergistic effects of EVL and FLU were favorable against 11 (61.1%) strains of Cryptococcus spp.
The combination of EVL and ITR reduced the MICs of both drugs against Cryptococcus
spp. to 0.0625-0.5 μg/ml and 0.0625-1 μg/ml, respectively. When used together, EVL and VOR lowered the MICs of both against Cryptococcus spp. to 0.0625-1 μg/ml and 0.0125-0.3125 μg/ml, respectively. There were 10 (55.6%) and 6 (33.3%) Cryptococcus spp. strains that showed synergistic effects when treated with EVL/ITR and EVL/VOR, respectively (Table 1).
The EVL/AmB combination revealed good synergistic effects against 16 (88.9%) strains of Cryptococcus isolates (Table 1), where the MIC ranges of EVL and AmB decreased to 0.0625–0.5μg/ml and 0.0625–1μg/ml, respectively. Antagonism was never observed with AmB or azoles in combination with EVL.
Efficacy of EVL alone and in combination with AmB or Azoles in C.neoformans -infected G. mellonella
Next, using G. mellonella larvae infected with the C. neoformans G7 isolate as a model system, we investigated the in vivo antifungal effectiveness of EVL with AmB or azoles in combination with one another or isolation. Compared to monotherapy (POS, 56.7%; FLU, 50%; ITR, 48.3%; EVL, 55%) or infected but untreated larvae (5%), survival rates were greater in the ELV+POS treatment group (66.7%), ELV+FLU treatment group (60%), and ELV+ITR treatment group (61.7%) on day 2 post-infection.
Treatment combinations of EVL and POS (58.3%), ELV and FLU (50%), and ELV and ITR (51.7%) resulted in greater larval survival on day 4 post-infection compared to monotherapy (POS: 41.7%; FLU: 45%; ITR: 36.7%; EVL,: 43.3%). The conidia-infected group with no treatment had a survival rate of 0.
The larvae survival rates on day 6 in the POS, FLU, ITR, EVL, EVL+POS, EVL+FLU, and EVL+ITR groups were 36.7%, 38.3%, 35%, 40%, 53.3%, 50%, and 51.7%, respectively, confirming that EVL+POS, EVL+FLU, EVL+ITR treatment substantially increased larval survival in comparison to the POS group, FLU group, ITR group, and conidial group (P < 0.05) (Figure 1).
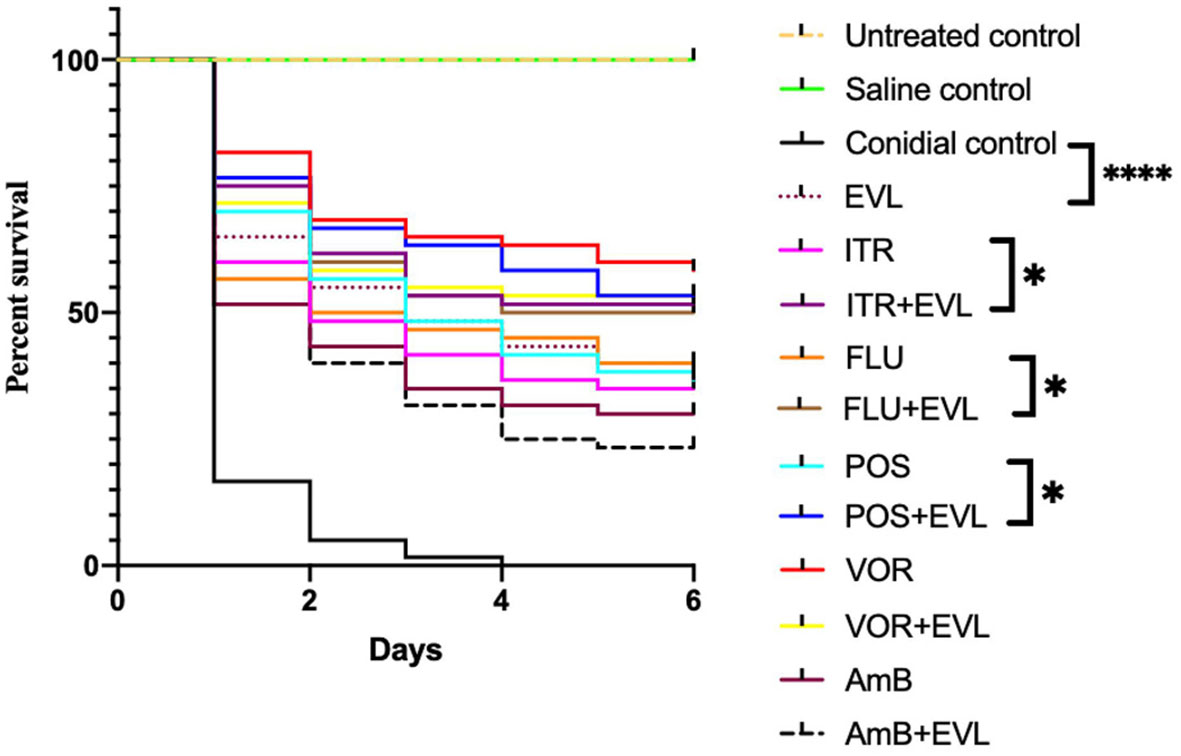
Figure 1 Galleria mellonella survival curves following infection with C.neoformans. Untreated group, noninfected larvae; Saline group, noninfected larvae injected with saline; Conidial group, C. neoforman-infected larvae without any treatment; POS, treatment of C. neoformans -infected larvae treated with posaconazole (POS) alone; POS+EVL, treatment of C. neoformans -infected larvae with POS and everolimus (EVL); ITR, treatment of C. neoformans -infected larvae with itraconazole alone; ITR+EVL, treatment of C.neoformans-infected larvae with ITR and EVL; VOR, treatment of C. neoformans - infected larvae with voriconazole alone; VOR+EVL, treatment of C. neoformans -infected larvae with VOR and EVL; FLU, treatment of C. neoformans -infected larvae with fluconazole alone; FLU+EVL, treatment of C. neoformans -infected larvae with FLU and EVL; AmB, treatment of C. neoformans -infected larvae with amphotericin B alone; AmB+EVL, treatment of C. neoformans-infected larvae with AmB and EVL; EVL, treatment of C. neoformans -infected larvae with EVL alone (*p<0.05. **** p<0.0001).
However, EVL-AmB and EVL-VOR combination did not show a synergistic effect when G. mellonella infected with C.neoformans G7 isolate were treated, which was not consistent with in vitro experiments. It was speculated that the specific mechanism of drug interaction is different in vivo and in vitro.
Discussion
Since its discovery, the TOR signaling pathway—of which TOR kinase is the primary component—has been the subject of many studies and has come to be regarded as a key regulator of cell proliferation in eukaryotes (Crespo and Hall, 2002). Rapamycin, a classical allosteric TOR inhibitor, was first found in screening for new antifungal drugs and has since shown great medicinal promise. Researchers have shown that rapamycin has strong antifungal effects against various fungi, including Penicillium spp., Fusarium spp., Aspergillus spp., Cryptococcus spp., Candida spp., Dermatophytes spp.(Rohde and Cardenas, 2004).However, rapamycin’s much more potent immunosuppressive properties prevented it from being employed as an antifungal agent (Gao et al., 2016).
Initially created to treat cancer, EVL was an orally active, potent TOR kinase inhibitor that directly inhibited tumor cell proliferation and growth and indirectly blocked angiogenesis (Hasskarl, 2018). We tested EVL’s antifungal potential against Cryptococcus spp. in vitro, alone and in combination with other antifungal drugs. The results discovered that EVL alone was active against all tested strains, which could be explained by the fact that FKBP protein (FK-506 Binding Proteins) and TOR protein are ubiquitous in eukaryotes, and rapalogs could bind to the FKBP12, and then, this complex inhibits mTOR to regulate the response of fungi to the external environment, and kills microorganism (Stan et al., 1994; Hasskarl, 2018). Furthermore, synergistic activities between EVL and AmB(88.9%), FLU(61.1%), ITR(55.6%), POS(50%), VOR(33.3%) were observed in Cryptococcus spp. The effective working ranges of EVL were 0.0625-0.5 μg/ml against Cryptococcus spp.; no antagonism was observed. The synergy between EVL and FLU was seen in as many as 61.1% of Cryptococcus strains, suggesting that EVL might increase the in vitro susceptibility of FLU-inactive Cryptococcus strains even when their MICs against FLU were high (0.5-32 μg/ml).
Rapamycin has been shown to have synergistic interactions with POS (40%), ITR (50%), and AmB (70%) against Mucorales (formerly called zygomycetes) by the broth microdilution checkerboard procedure (Dannaoui et al., 2009). Additionally, it has been reported that Mucorales exhibits antagonism of rapamycin/ITR, and no substantial agonism of rapamycin/POS (Dannaoui et al., 2009; Narreddy et al., 2010). In contrast, no antagonism was identified between EVL and POS or ITR in the current investigation, and EVL increased the antifungal activity of POS (50%) and ITR (55.6%). The different effect of EVL and rapamycin on TOR and antifungals, as well as the differential responsiveness of the tested species to these drugs, may account for these discrepancies.
Since G. mellonella larvae exhibit immunological responses comparable to those of mammals without the accompanying ethical concerns with advantages such as being easy to manipulate and inexpensive, these larvae have recently emerged as an appropriate in vivo model for the preclinical investigation of the antifungal effects of novel medicines (Vilcinskas, 2011; Favre-Godal et al., 2014; Liu et al., 2017). To further verify the interaction between EVL and azoles, EVL and AmB detected in our study, we tested the in vivo effect of this combination against one of the isolates of C.neoformans (G7) with the G. mellonella model. We found that EVL-POS, EVL-FLU, and ELV-ITR combination treatment were associated with significant improvements in larval survival compared to POS, FLU, or ITR treatment in isolation, further confirming the synergistic benefits of EVL-POS, ELV-FLU, and ELV-ITR combination treatment when used to treat infections caused by C.neoformans spp.
Ergosterol biosynthesis in fungal cell membranes was inhibited by azole antifungal compounds by inhibiting lanosterol 14α-demethylase activity (Keady and Thacker, 2005; Singal and Khanna, 2011; Jo Siu et al., 2013). Micropores in the cell membrane are formed, and the membrane’s permeability to monovalent and divalent cations is increased when ergosterol on the fungal cell membrane binds with AmB to form the sterol-polyene complex, killing fungus.
As an oral mTOR protein kinase inhibitor, EVL might control cell growth to kill fungi and potentiate the activities of azoles by inhibiting TOR signaling which could affect the amino acid permeases regulation, ribosome biogenesis, protein synthesis initiation, actin cytoskeleton organization, autophagy inhibition, control of phosphatases by TOR, and transcriptional control of nutrient metabolism(Crespo and Hall, 2002).
In conclusion, the current investigation expands prior results in the combination interactions between conventional antifungals and TOR inhibitors. Against Cryptococcus spp., EVL may augment the antifungal activity of AmB, POS, FLU, ITR, and VOR in vitro. In addition, for some individuals with clinical cancer, combining EVL with AmB or azoles may be a safe alternative for treating Cryptococcus infections. However, further research is required to clarify the underlying process and identify viable, safe therapeutic applications.
Data availability statement
The original contributions presented in the study are included in the article/supplementary material. Further inquiries can be directed to the corresponding authors.
Author contributions
PL conceived and designed the study. PL performed all the experiments, analyzed the data and wrote the manuscript. JS and QL provided general guidance and revised the manuscript. All authors contributed to the article and approved the submitted version.
Funding
This work was supported by the National Natural Science Foundation of China [81602771 to PL].
Acknowledgments
We thank the staff of Dermatology of Zhongnan Hospital of Wuhan University, for assistance in field sampling and processing samples in the laboratory, and the National Natural Science Foundation of China (Grant 81602771).
Conflict of interest
The authors declare that the research was conducted in the absence of any commercial or financial relationships that could be construed as a potential conflict of interest.
Publisher’s note
All claims expressed in this article are solely those of the authors and do not necessarily represent those of their affiliated organizations, or those of the publisher, the editors and the reviewers. Any product that may be evaluated in this article, or claim that may be made by its manufacturer, is not guaranteed or endorsed by the publisher.
References
Amorim-Vaz, S., Delarze, E., Ischer, F., Sanglard, D., Coste, A. T. (2015). Examining the virulence of candida albicans transcription factor mutants using galleria mellonella and mouse infection models. Front. Microbiol. 6. doi: 10.3389/fmicb.2015.00367
Cho, Y. J., Han, S. I., Lim, S. C. (2021). Cryptococcal infection presenting as soft tissue abscess and arthritis: Case report. Med. (Baltimore). 100 (28), e26656. doi: 10.1097/MD.0000000000026656
Clinical and Laboratory Standards Institute (2017). Reference Method for Broth Dilution Antifungal Susceptibility Testing of Yeasts; Approved Standard-4th ed CLSI Document M27-A4.
Crespo, J. L., Hall, M. N. (2002). Elucidating TOR signaling and rapamycin action: lessons from saccharomyces cerevisiae. Microbiol. Mol. Biol. Rev. 66 (4), 579–591. doi: 10.1128/MMBR.66.4.579-591.2002
Cruz, M. C., Cavallo, L. M., Görlach, J. M., Cox, G., Perfect, J. R., Cardenas, M. E., et al. (1999). Rapamycin antifungal action is mediated via conserved complexes with FKBP12 and TOR kinase homologs in cryptococcus neoformans. Mol. Cell Biol. 19 (6), 4101–4112. doi: 10.1128/MCB.19.6.4101
Dannaoui, E., Schwarz, P., Lortholary, O. (2009). In vitro interactions between antifungals and immunosuppressive drugs against zygomycetes. Antimicrob. Agents Chemother. 53 (8), 3549–3551. doi: 10.1128/AAC.00184-09
DiNardo, A. R., Schmidt, D., Mitchell, A., Kaufman, Y., Tweardy, D. J. (2015). First description of oral cryptococcus neoformans causing osteomyelitis of the mandible, manubrium and third rib with associated soft tissue abscesses in an immunocompetent host. Clin. Microbiol. Case Rep. 1 (3), 017.
Favre-Godal, Q., Dorsaz, S., Queiroz, E. F., Conan, C., Marcuort, L., Eko Wardojo, B. P., et al. (2014). Comprehensive approach for the detection of antifungal compounds using a susceptible strain of candida albicans and confirmation of in vivo activity with the galleria mellonella model. Phytochemistry 105, 68–78. doi: 10.1016/j.phytochem.2014.06.004
Fonseca, F. L., Reis, F. C. G., Sena, B. A. G., Jozefowicz, L. J., Kmetzsch, L., Rodrigues, M. L. (2019). The overlooked glycan components of the cryptococcus capsule. Curr. Top. Microbiol. Immunol. 422, 31–43. doi: 10.1007/82_2018_140
Gao, L., Sun, Y., He, C., Li, M., Zeng, T., Lu, Q. (2016). INK128 exhibits synergy with azoles against exophiala spp. and fusarium spp. Front. Microbiol. 7. doi: 10.3389/fmicb.2016.01658
Hasskarl, J. (2018). Everolimus. Recent Results Cancer Res. 211, 101–123. doi: 10.1007/978-3-319-91442-8_8
Henao-Martínez, A. F., Beckham, J. D. (2015). Cryptococcosis in solid organ transplant recipients. Curr. Opin. Infect. Dis. 28 (4), 300–307. doi: 10.1097/QCO.0000000000000171
High, K. P., Washburn, R. G. (1997). Invasive aspergillosis in mice immunosuppressed with cyclosporin a, tacrolimus (FK506), or sirolimus (rapamycin). J. Infect. Dis. 175 (1), 222–225. doi: 10.1093/infdis/175.1.222
Jo Siu, W. J., Tatsumi, Y., Senda, H., Pillai, R., Nakamura, T., Sone, D., et al. (2013). Comparison of in vitro antifungal activities of efinaconazole and currently available antifungal agents against a variety of pathogenic fungi associated with onychomycosis. Antimicrob. Agents Chemother. 57 (4), 1610–1616. doi: 10.1128/AAC.02056-12
Keady, S., Thacker, M. (2005). Voriconazole in the treatment of invasive fungal infections. Intensive Crit. Care Nurs. 21 (6), 370–373. doi: 10.1016/j.iccn.2005.02.006
Liu, X., Li, T., Wang, D., Yang, Y., Sun, W., Liu, J., et al. (2017). Synergistic antifungal effect of fluconazole combined with licofelone against resistant candida albicans. Front. Microbiol. 8. doi: 10.3389/fmicb.2017.02101
Maziarz, E. K., Perfect, J. R. (2016). Cryptococcosis. Infect. Dis. Clin. North Am. 30 (1), 179–206. doi: 10.1016/j.idc.2015.10.006
Mylonakis, E., Moreno, R., El Khoury, J. B., Idnurm, A., Heitman, J., Calderwood, S. B., et al. (2005). Galleria mellonella as a model system to study cryptococcus neoformans pathogenesis. Infect. Immun. 73 (7), 3842–3850. doi: 10.1128/IAI.73.7.3842-3850.2005
Narreddy, S., Manavathu, E., Chandrasekar, P. H., Alangaden, G. J., Revankar, S. G. (2010). In vitro interaction of posaconazole with calcineurin inhibitors and sirolimus against zygomycetes. J. Antimicrob. Chemother. 65 (4), 701–703. doi: 10.1093/jac/dkq020
Odds, F. C. (2003). Synergy, antagonism, and what the chequerboard puts between them. J. Antimicrob. Chemother. 52 (1), 1. doi: 10.1093/jac/dkg301
Pappas, P. G., Perfect, J. R., Cloud, G. A., Larsen, R. A., Pankey, G. A., Lancaster, D. J., et al. (2001). Cryptococcosis in human immunodeficiency virus-negative patients in the era of effective azole therapy. Clin. Infect. Dis. 33 (5), 690–699. doi: 10.1086/322597
Perfect, J. R., Dismukes, W. E., Dromer, F., Goldman, D. L., Graybill, J. R., Hamill, R. J., et al. (2010). Clinical practice guidelines for the management of cryptococcal disease: 2010 update by the infectious diseases society of america. Clin. Infect. Dis. 50 (3), 291–322. doi: 10.1086/649858
Rohde, J. R., Cardenas, M. E. (2004). Nutrient signaling through TOR kinases controls gene expression and cellular differentiation in fungi. Curr. Top. Microbiol. Immunol. 279, 53–72. doi: 10.1007/978-3-642-18930-2_4
Schwarz, P., Dannaoui, E. (2020). In vitro interaction between isavuconazole and tacrolimus, cyclosporin a, or sirolimus against aspergillus species. J. Fungi (Basel). 6 (3), 103. doi: 10.3390/jof6030103
Singal, A., Khanna, D. (2011). Onychomycosis: Diagnosis and management. Indian J. Dermatol. Venereol. Leprol. 77 (6), 659–672. doi: 10.4103/0378-6323.86475
Singh, N., Forrest, G. (2009). AST infectious diseases community of practice. cryptococcosis in solid organ transplant recipients. Am. J. Transplant. 9 Suppl 4, S192–S198. doi: 10.1111/j.1600-6143.2009.02911.x
Stan, R., McLaughlin, M. M., Cafferkey, R., Johnson, R. K., Rosenberg, M., Livi, G. P. (1994). Interaction between FKBP12-rapamycin and TOR involves a conserved serine residue. J. Biol. Chem. 269 (51), 32027–32030.
Teichert, S., Wottawa, M., Schönig, B., Tudzynski, B. (2006). Role of the fusarium fujikuroi TOR kinase in nitrogen regulation and secondary metabolism. Eukaryot. Cell. 5 (10), 1807–1819. doi: 10.1128/EC.00039-06
Thakur, M., Revankar, S. G. (2011). In vitro interaction of caspofungin and immunosuppressives against agents of mucormycosis. J. Antimicrob. Chemother. 66 (10), 2312–2314. doi: 10.1093/jac/dkr297
Vézina, C., Kudelski, A., Sehgal, S. N. (1975). Rapamycin (AY-22,989), a new antifungal antibiotic. i. taxonomy of the producing streptomycete and isolation of the active principle. J. Antibiot. (Tokyo). 28 (10), 721–726. doi: 10.7164/antibiotics.28.721
Keywords: Cryptococcus neoformans, amphotericin B, azoles, everolimus, synergistic
Citation: Liang P, Song J and Liu Q (2023) Interactions between antifungals and everolimus against Cryptococcus neoformans. Front. Cell. Infect. Microbiol. 13:1131641. doi: 10.3389/fcimb.2023.1131641
Received: 25 December 2022; Accepted: 03 March 2023;
Published: 21 March 2023.
Edited by:
Yi Sun, Jingzhou Hospital Affiliated to Yangtze University, ChinaReviewed by:
Yinggai Song, First Hospital, Peking University, ChinaQiaoyun Lu, Hubei University of Arts and Science, China
Copyright © 2023 Liang, Song and Liu. This is an open-access article distributed under the terms of the Creative Commons Attribution License (CC BY). The use, distribution or reproduction in other forums is permitted, provided the original author(s) and the copyright owner(s) are credited and that the original publication in this journal is cited, in accordance with accepted academic practice. No use, distribution or reproduction is permitted which does not comply with these terms.
*Correspondence: Jiquan Song, c29uZ2ppcUAxMjYuY29t; Qin Liu, MTA5NDE1MDEzQHFxLmNvbQ==