- 1National Research Center for Protozoan Diseases, Obihiro University of Agriculture and Veterinary Medicine, Obihiro, Hokkaido, Japan
- 2Jiangsu Key Laboratory for High-tech Research and Development of Veterinary Biopharmaceuticals, Jiangsu Agri-animal Husbandry Vocational College, Taizhou, China
- 3Engineering Technology Research Center for Modern Animal Science and Novel Veterinary Pharmaceutic Development, Jiangsu Agri-animal Husbandry Vocational College, Taizhou, China
- 4Department of Biology, Premedical Sciences, Dokkyo Medical University, Tochigi, Japan
Introduction: Malaria and Babesiosis are acute zoonotic disease that caused by infection with the parasite in the phylum Apicomplexa. Severe anemia and thrombocytopenia are the most common hematological complication of malaria and babesiosis. However, the mechanisms involved have not been elucidated, and only a few researches focus on the possible role of anti-erythrocyte and anti-platelet antibodies.
Methods: In this study, the Plasmodium yoelii, P. chabaudi, Babesia microti and B. rodhaini infected SCID and ICR mice. The parasitemia, survival rate, platelet count, anti-platelet antibodies, and the level of IFN-γ and interleukin (IL) -10 was tested after infection. Furthermore, the P. yoelii, P. chabaudi, B. rodhaini and B. microti infected ICR mice were treated with artesunate and diminaze, the development of the anti-erythrocyte and anti-platelet antibodies in chronic stage were examined. At last, the murine red blood cell and platelet membrane proteins probed with auto-antibodies induced by P. yoelii, P. chabaudi, B. rodhaini, and B. microti infection were characterized by proteomic analysis.
Results and discussion: The high anti-platelet and anti-erythrocyte antibodies were detected in ICR mice after P. yoelii, P. chabaudi, B. rodhaini, and B. microti infection. Actin of murine erythrocyte and platelet is a common auto-antigen in Plasmodium and Babesia spp. infected mice. Our findings indicate that anti-erythrocyte and anti-platelet autoantibodies contribute to thrombocytopenia and anemia associated with Plasmodium spp. and Babesia spp. infection. This study will help to understand the mechanisms of malaria and babesiosis-related thrombocytopenia and hemolytic anemia.
Introduction
The intraerythrocytic apicomplexan parasites Plasmodium and Babesia spp. cause malaria and babesiosis, respectively. Malaria and babesiosis are accountable for significant mortality and morbidity to humans and animals globally (Zoleko Manego et al., 2019; Zottig and Shanks, 2021; Zowonoo et al., 2023). Thrombocytopenia and anemia, are common symptoms of malaria and Babesia spp. infection (Yuan-Yuan et al., 2016; Yu et al., 2021; Waked and Krause, 2022). It has been reported that acute malaria infection is associated with autoimmune hemolytic anemia (AIHA), but it has not been well characterized. The symptom of AIHA include shortened red blood cells (RBCs) survival as well as the autoantibodies found in direct antiglobulin tests (DATs). DATs test complement C3d and/or immunoglobulins against autologous RBCs (Santos et al., 2020; Rajapakse and Bakirhan, 2021; Sporn et al., 2021; Ueda, 2022).
Anemia and thrombocytopenia are the most common hematological complications of malaria and babesiosis. Several studies have documented a high rate of thrombocytopenia in malaria patients. Over the past four decades, research has been conducted on the malaria thrombocytopenia, however, the exact mechanism behind this phenomenon remains unclear (Zumla and Hui, 2019; Tao et al., 2020; Tołkacz et al., 2021; Vanheer and Kafsack, 2021; Voisin et al., 2021; Zottig and Shanks, 2021). Thrombocytopenia in malaria is a multifactorial phenomenon likely caused by increased platelet destruction and consumption. The explanation for malaria-induced thrombocytopenia has been proposed by several authors (Coelho et al., 2013). There was some research suggested that the low level of platelet count in malaria might be because of apoptosis and/or activation of platelets. However, immune complexes elicited by malarial antigen may also be able to sequester injured platelets in the spleen and then be phagocytosed by splenic macrophages (Thapa et al., 2009; Lacerda et al., 2011; Zhan et al., 2019). The immune system attacks platelets resulting in immune thrombocytopenia (ITP). There are a few tests available to test antibodies against platelets (Michel et al., 2002). A majority of IgG subclasses are found bound to platelets in ITP. It may be useful to test for platelets-bound IgG in patients with thrombocytopenia.
Plasmodium yoelii and P. chabaudai are widely used as murine models to identify parasite induced immune responses. Babesia rodhaini and B. microti have been served as useful experimental model in mice for the analysis of human babesiosis (Rizk et al., 2020; Ji et al., 2021; Li et al., 2022). Anti-erythrocyte and anti-platelet autoantibodies producing is the crucial step between hematological complication and the host defense mechanisms after Plasmodium and Babesia spp. infection. To investigate the development of anti-erythrocyte and anti-platelet auto-antibodies and identify the related auto-antigens, in this study, the anti-erythrocyte and anti-platelet auto-antibodies were detected in Plasmodium and Babesia spp. infected mice, and the mechanism of antibody-mediated hemolytic anemia and thrombocytopenia were investigated.
Materials and methods
Mice ethics statement
From Central Institute for Experimental Animals (CIEA) in Japan, we purchased 6-week-old female ICR mice as well as C.B-17/Icr-scid/scid (SCID) mice. In accordance with the research protocol, the experimental animals were handled under the permit issued by Obihiro’s Animal Care and Use in Research Committee Promulgated by Obihiro University of Agriculture and Veterinary Medicine, Japan (Permit Number: 201109–5).
Maintenance of the parasites and mice infections
Plasmodium yoelii, P. chabaudi, B. rodhaini and B. microti were maintained in mice by intraperitoneal (i.p.) passage as previously described (Li et al., 2012). SCID mice are severely combined immunodeficient mice. The weight of the thymus, spleen, and lymph nodes was less than 30% of normal, and histologically there were significant lymphocyte defects. To determine the role of B and T lymphocytes in the protection against infection with P. yoelii, P. chabaudi, B. rodhaini and B. microti, four groups of SCID mice were also infected with P. yoelii, P. chabaudai, B. rodhaini and B. microti by i.p. inoculation with 107 of parasitized erythrocytes (pRBCs). At the same time, four groups of ICR mice were infected with P. yoelii, P. chabaudai, B. rodhaini and B. microti as mentioned above.
Determination of survival rates and parasitemia
The survival rates and parasitemia of these groups of mice were monitored after P. yoelii, P. chabaudi, B. rodhaini and B. microti infection. The parasitemia of each mouse was tested by Giemsa-stained thin blood films. The blood samples from the ICR and SCID mice were serially collected every two days post-infection (dpi). The plasma was separated from RBCs by centrifuge (3000 r/min, 3 min), the RBCs and plasma were stored at -70°C until use.
Detection of serum cytokines
The blood samples from the ICR and SCID mice infected with P. yoelii, P. chabaudi, B. rodhaini and B. microti were serially collected. The plasma was separated and used to detect the serum cytokines. A standard curve was used to determine the concentration of individual cytokines in the samples by using enzyme-linked immunosorbent assay (ELISA) kits. According to the manufacturer’s instructions, standard curves were prepared with mouse recombinant IFN-γ and interleukin (IL) -10 (Cusabio Biotech Co., Germany) according to the manufacturer’s instructions. Double-antibody one-step sandwich ELISA was used. The samples, standard products, and HRP labeled detection antibodies were added into the coated micropores pre-coated with antibodies, incubated and thoroughly washed. Substrate TMB was added to the micropores for color development. The depth of the color is positively correlated with the concentration of the substance in the sample. The absorbance (OD value) was measured at 450 nm wavelength and the sample concentration was calculated.
Establishment of chronic infection
The mice should survive for more than 60 days to monitor the anti-erythrocyte and anti-platelet autoantibody level during infection. Therefore, we established the chronic infection and tried to extend the survival period of the Plasmodium and Babesia spp. infected mice. P. yoelii, P. chabaudi, B. rodhaini and B. microti were infected to 5 ICR mice for each group. The Plasdodium spp. infected-mice were treated by Artesunate, and the Babesia spp. infected-mice were treated by Diminazen for collecting the chronic stage serum. The parasitemia of each mouse was assessed every 2 days for 60 days. In order to determine the parasitemia percentage, thin blood smears were prepared, fixed in methanol, and stained for 30 minutes with 10% Giemsa solution. The level of parasitemia was estimated according to the matching method. A serial blood sample was collected from the ICR mice every two days. The plasma and RBCs were harvested and stored at -70°C until use.
Platelets and red blood cells (RBCs) membrane isolation
Isolated platelets and RBC membranes were used for ELISA and two-dimensional LC and MS assays. To generate platelet-rich plasma (PRP), whole blood was centrifuged for 10 minutes at 200 x g. Platelets were acidified with citric acid 0.15 M until pH 6.4, then prostaglandin E-1 (PGE-1) was added at 1 mg/mL to prevent aggregation and activation.
After centrifugation, the pellet was resuspended in phosphate buffered saline (PBS). The whole blood was spun to separate packed red cells. To isolate host erythrocyte membrane, packed erythrocytes were extensively washed and lysed. The packed erythrocytes were washed in a ten-fold volume of 1X PBS. For the remaining steps, the washed cells were placed on ice. The washed RBC pellets were lysed with cold lysis buffer; after that, the lysis was spun on a Beckman Coulter ultracentrifuge, and five washes were performed after removing the supernatant. The resulting host membranes were collected and frozen at −70 °C.
Detection of anti-erythrocyte and anti-platelet antibodies
The platelets and RBC membrane were washed two times, 100 ng of platelets and RBC membrane were coated in each well to detect of anti-erythrocyte and anti-platelet antibodies. After blocking, the mice serum collecting at all time points was added (50 µL/well, 100-time diluted) after incubating 1 hour and washing. We add 50 µL/well of a 1:2000 dilution in blocking buffer of HRP-labelled 2nd antibody. ELISA reader was used to read absorbance at 450 nm after incubation with stop solution. The quantity of erythrocyte and platelet-associated immunoglobulin IgM, IgG, IgG1, IgG2a, and IgG2b in plasma were determined by using the ELISA kit.
Two-dimensional electrophoresis (2-DE) and Western blot analysis
The purified platelets and RBC membrane proteins were treated separately with DTT and iodoacetamide, and digested with trypsin. The sample was loaded on IPG strip, rehydration and focusing steps were run with isoelectric focusing carried out simultaneously over 17 hours at a total voltage of 35 kV/h. Second-dimension electrophoresis was performed at 200 volts for 45-50 minutes with Criterion precast gels. In the next step, colloidal Coomassie blue was used to stain the 2DE gels, followed by acetic acid to destain them. At the same time, 2DE gels were detected by Western blot using a mouse serum (diluted 1:100), which was generated in the laboratory by infecting with Plasmodium spp. and Babesia spp.
Trypsin digestion and two-dimensional LC with MS/MS
In each gel, selected spots were manually removed and dehydrated with acetonitrile for 10 minutes before being dried with a Speed-Vac system. After overnight incubation, each dried protein spot was pipetted with trypsin solution and incubated. A Speed-Vac system was used to dry the supernatants after each step. The peptides were solubilized in 0.5% formic acid using an ultrahigh-performance liquid chromatography system. A reverse phase column Pepmap C18 was used for peptide separation. The most abundant peptides were analyzed by mass spectrometry (MS) using a continuous MS scan followed by 10 times analyses. The Mascot search engine was used to identify proteins in the NCBI Genbank database. The contaminants were excluded during the process.
Statistical analysis
An analysis of one-way Analysis of variance test was used to determine if there were any significant differences between the means of all variables (GraphPad Prism 5; GraphPad Software, Inc.). The significance of P-values was denoted as follows: ns, non-significant; *, p ≤ 0.05; **, p ≤ 0.01; ***, p ≤ 0.001; and ****, p ≤ 0.0001.
Results
Thrombocytopenia and parasitemia after Plasmodium spp. and Babesia spp. infection
To determine the role of B and T lymphocytes during lethal infection with Plasmodium spp. and Babesia spp, the immune sufficient ICR mice and SCID mice were infected with P. yoelii, P. chabaudi, B. microti and B. rodhaini. The percentage parasitemia, survival rate, platelet count and anti-platelet autoantibodies were tested (Figures 1A–P). In the group that infected with P. yoelii and B. Rodhaini, all the infected mice died within ten days (Figures 1B, J); parasitemia was similar in ICR and SCID mice (Figures 1A, I). The low survival rate was correlated with the high percentage of parasitemia. In the group that was infected with P. chabaudi andB. microti, all the mice were alive for more than 20 days (Figures 1F, N); parasitemia was similar in P. chabaudi-infected ICR and SCID mice (Figures 1G, O), the parasitemia in B. microti-infected ICR and SCID mice was significant different after 20 days infection (P<0.05). Both ICR and SCID mice developed rapid increases in parasitemia within one week of infection (Figures 1E, M). Compared with the SCID mice, high anti-platelet autoantibodies were found in P. yoelii, P. chabaudi, B. microti and B. rodhaini infected ICR mice (P<0.05). Furthermore, negative correlation existed between platelet count and anti-platelet in P. yoelii (Figures 1C, D), P. chabaudi (Figures 1G, H), B. rodhaini (Figures 1K, L) and B. microti (Figures 1O, P) infected ICR mice. The concentration of individual cytokines in Plasmodium and Babesia spp. infected mice has been tested (Figures 2A–G). Likewise, detectable IFN-γ and IL-10 levels were lower in SCID mice (P<0.01) than those detected in ICR mice at days 5 and 7 post challenge with P. yoelii and B. rodhaini (Figure 2H). According to these findings, the anti-platelet autoantibodies induced by Plasmodium spp. and Babesia spp infection might be impaired by B and T lymphocytes.
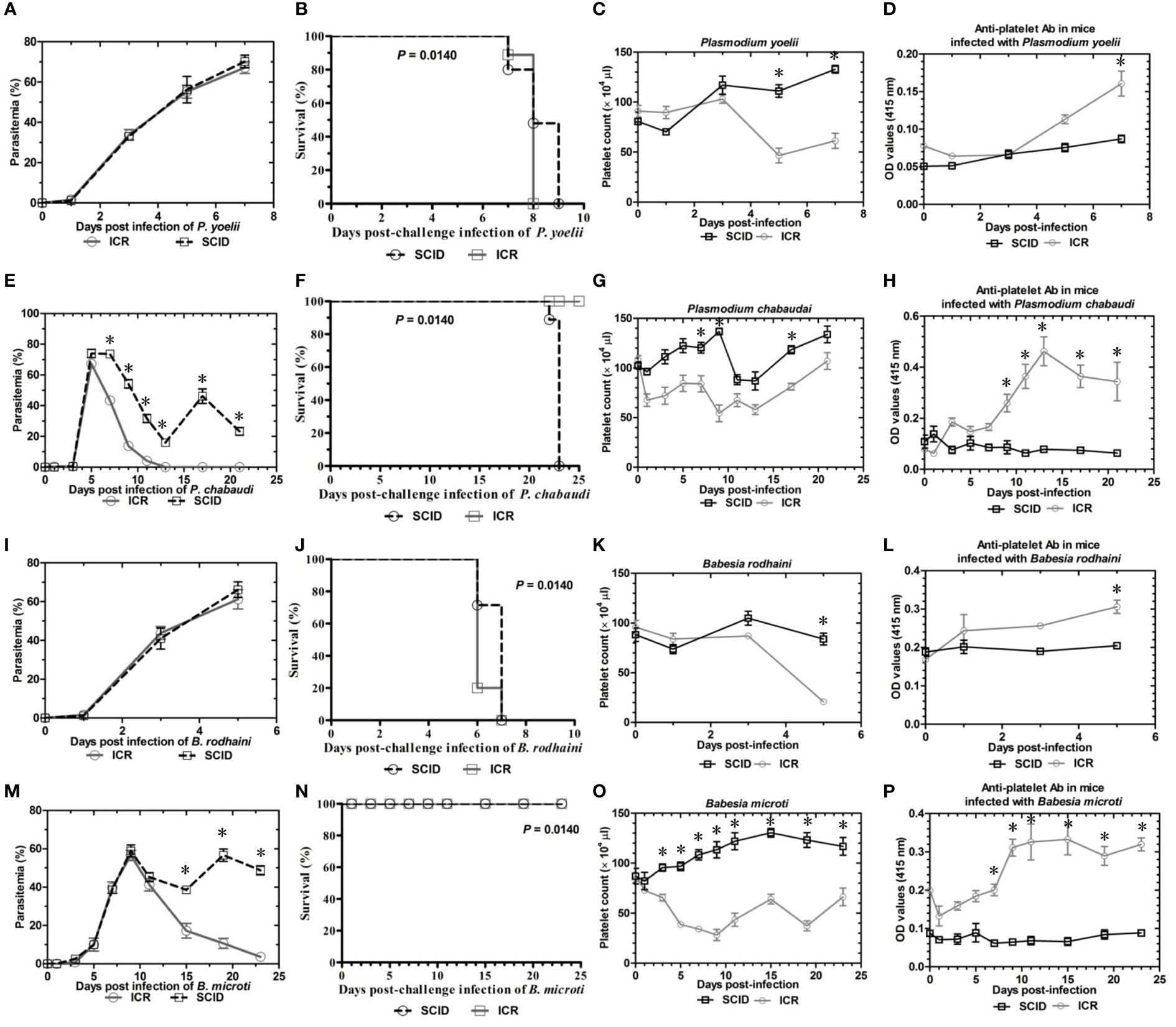
Figure 1 Parasitemia and survival rates, platelet counts and anti-platelet antibody of ICR mice and SCID mice after Plasmodium yoelii, P. chabaudi, Babesia rodhaini and B. microti challenge infection. (A–D) Parasitemia profiles of P. yoelii, P. chabaudi, B. rodhaini and B. microti infected ICR mice and SCID mice. (B–H) Survival rates of P. yoelii, P. chabaudi, B. rodhaini and B. microti infected ICR mice and SCID mice. (I–L) Platelet counts of P. yoelii, P. chabaudi, B. rodhaini and B. microti infected ICR mice and SCID mice. (M–P) anti-platelet antibody of P. yoelii, P. chabaudi, B. rodhaini and B. microti infected ICR mice and SCID mice. I.p. inoculations infected test mice with 107 of parasitized erythrocytes (pRBCs). The results are expressed as mean value ± the SD for five mice. The significance of P-values was denoted as follows: ns, non-significant; *, p ≤ 0.05; **, p ≤ 0.01; ***, p ≤ 0.001; and ****, p ≤ 0.0001.
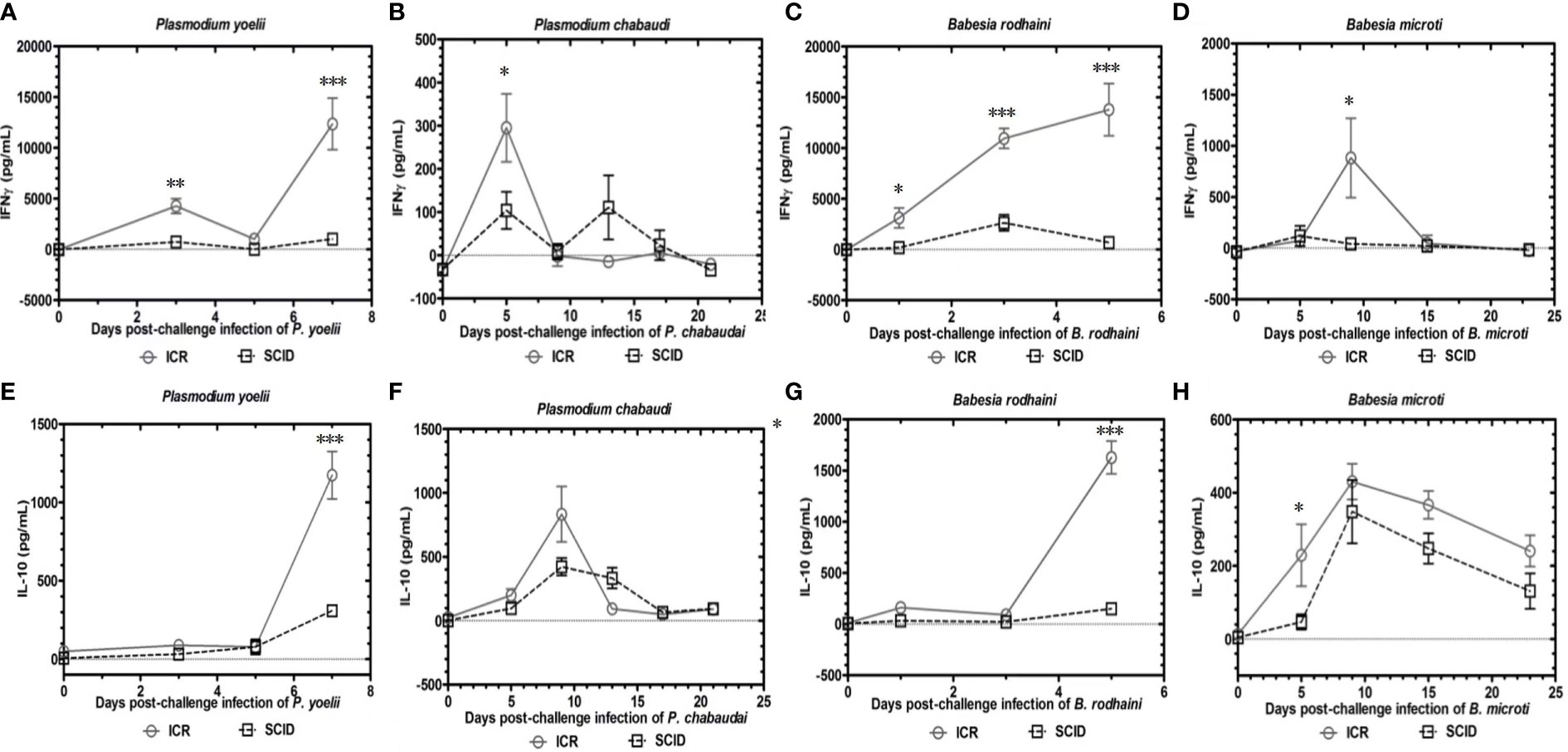
Figure 2 Kinetics of serum cytokines of ICR mice and SCID mice after P. yoelii, P. chabaudi, B. rodhaini and B. microti challenge infection. (A–D) Production of IFN-γ. (E–H) Production of IL-10 post challenge infection with P. yoelii, P. chabaudi, B. rodhaini andB. microti. The results are expressed as mean value ± the SD for five mice. The significance of P-values was denoted as follows: ns, non-significant; *, p ≤ 0.05; **, p ≤ 0.01; ***, p ≤ 0.001; and ****, p ≤ 0.0001.
The Plasmodium spp. and Babesia spp. infection induces the production of anti-erythrocyte and anti-platelet autoantibodies
To examine the possible contribution of anti-erythrocyte and anti-platelet autoantibodies in thrombocytopenia and hemolytic anemia caused by lethal infection with Babesia spp. and Plasmodium spp. The chronic Babesia spp. and Plasmodium spp. infections were established by administering artesunate and diminaze to infected-ICR mice. The trend of parasitemia was similar in Babesia spp. and Plasmodium spp. infected mice, and hematocrit and parasitemia were negatively correlated (Figures 3A–H). The IgM and IgG reached to the highest level after reaching high parasitemia (Figures 3A–D). These results indicated that Plasmodium spp. and Babesia spp. infection lead to the destruction of RBCs and the production of anti-erythrocyte autoantibodies may not be the important reason for anemia. The platelet counts significantly decreased in the mice infected with Plasmodium spp. and Babesia spp. Moreover, IgM and IgG levels negatively correlated with platelet count (Figures 3E, F). Thus, anti-platelet auto-antibodies may be the cause of thrombocytopenia.
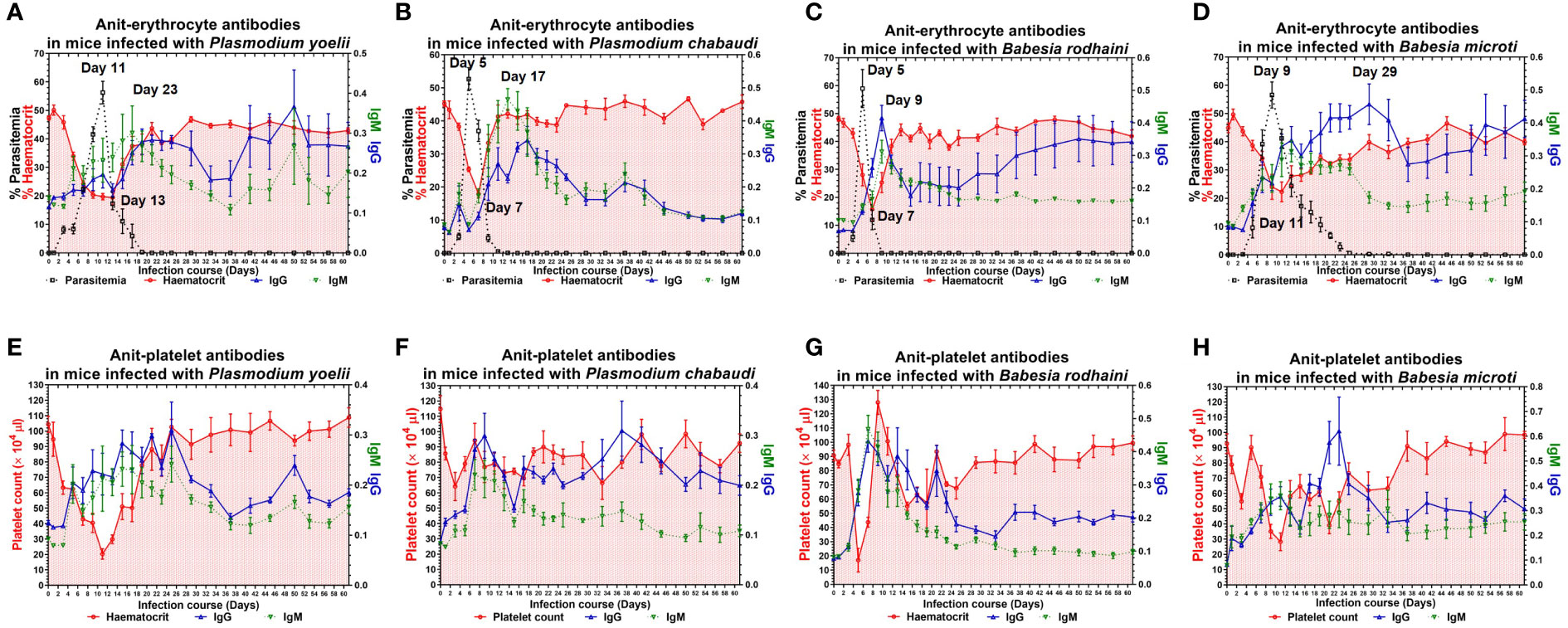
Figure 3 The anti-erythocyte and anti-platelet antibodies of chronically P. yoelii, P. chabaudi, B. rodhaini and B. microti. infected ICR mice. (A–D) The parasitemia, hematocrit, and the production of anti-erythocyte IgG and IgM in ICR mice challenge infection of P. yoelii, P. chabaudi, B. rodhaini and B. microti. (E–H) The platelet counts and the production of anti-platelet IgG and IgM in ICR mice challenge infection of P. yoelii, P. chabaudi, B. rodhaini and B. microti. Test mice with chronic P. yoelii, P. chabaudi, B. rodhaini andB. microti infection, the Plasmodium spp. infected mice were treated with artesunate, and the Babesia spp. infected mice were treated with diminaze for collecting the chronic stage sera. The parasitemia of each mouse was examined every 2 days for 60 days. The results are expressed as mean value ± the SD for five mice.
The IgG isotypes of anti-erythrocyte and anti-platelet autoantibodies
The infected mice produced a high amount of anti-platelet and anti-erythrocyte IgG2a and a low amount of IgG1 and IgG2b after Plasmodium spp. and Babesia spp. infection. The anti-erythrocyte IgG2a was detectable ten days after infection and peaked between 22 and 30 days and 40 and 50 days (Figures 4A–D) in plasmodium spp. and Babesia spp. infection. The anti-platelet IgG2a was detectable within six days after Plasmodium spp. and Babesia spp. infection. The anti-platelet IgG2a reached to high level at 24 days and 48 days after illness in P. yoelii-infected mice. The P. chabaudi-infected mice had elevated levels of anti-platelet antibody at 40 days post-infection. Furthermore, anti-platelet antibody also reached the peak at 22 days post-infection in B. microti infected mice. However, the B. rodhaini-infected mice developed low moderate levels anti-platelet IgG2a (Figures 4E–H).
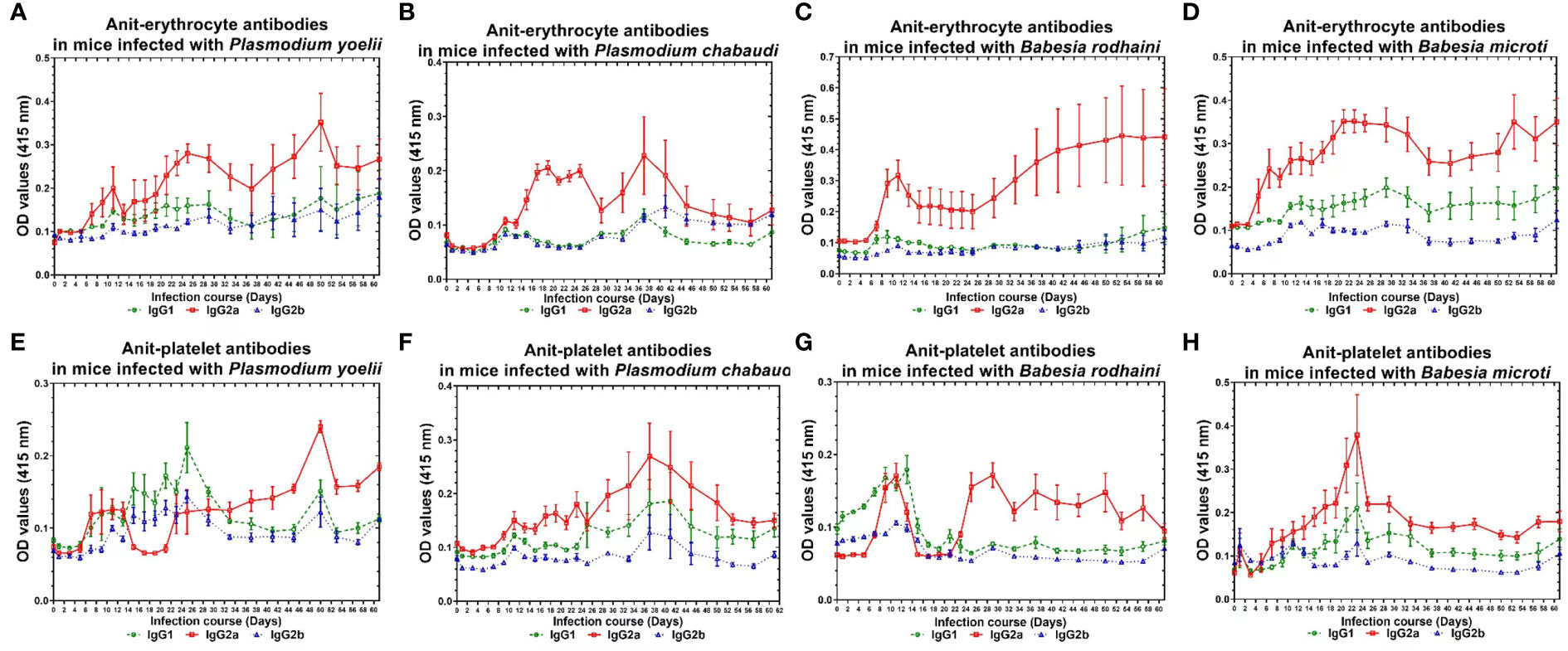
Figure 4 The production of anti-erythocyte and anti-platelet IgG1, IgG2a, IgG2b of of chronically P. yoelii, P. chabaudi, B. rodhaini and B. microti infected ICR mice. (A–D) The production of anti-erythocyte IgG1, IgG2a, IgG2b in ICR mice challenge infection of P. yoelii, P. chabauai, B. rodhaini and B. microti (E–H). The anti-platelet IgG1, IgG2a, IgG2b in ICR mice challenge infection of P. yoelii, P. chabaudi, B. rodhaini and B. microti. Test mice with chronic P. yoelii, P. chabaudi, B. rodhaini and B. microti. infection, the Plasmodium spp. infected mice were treated with artesunate, and the Babesia rodhaini infected mice were treated with diminaze for collecting the chronic stage sera. The parasitemia of each mouse was examined every 2 days for 60 days. The results are expressed as mean value ± the SD for five mice.
Identification of murine erythrocyte and platelet auto-antigens
In order to further investigate and understand the mechanism of autoimmune antibody-mediated thrombocytopenia and hemolytic anemia, we isolated the platelets and RBC membrane proteins followed by identification of the autoimmune antibody-binding proteins by 2DE and Western blot analysis. 2DE analysis of platelets samples showed differentially expressed spots (Figure 5A). In Figures 5B–E, representative gel images for each group are shown. A total of sixteen spots were selected for mass spectrometry analysis. 2DE image analysis of RBC membrane showed differentially expressed spots (Figure 6A). Each experimental group is represented by a gel image in Figures 6B, C. For mass spectrometry, six spots were chosen. Overall, the results showed that many host polypeptides are bound to autoimmune antibodies specifically (P<0.05).
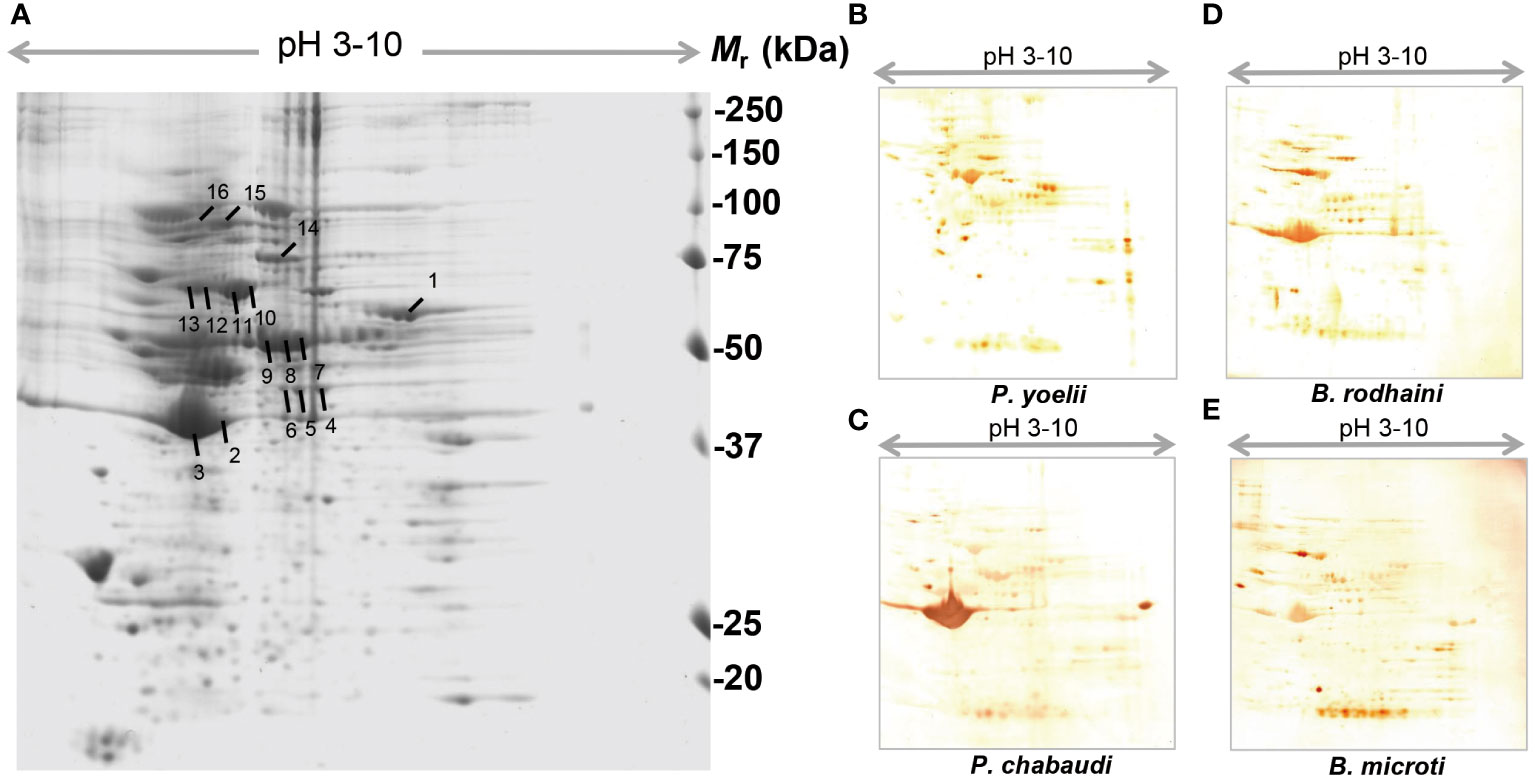
Figure 5 Reference image 2DE map of differentially expressed platelet proteins with marked selected spots and Western blot analysis of murine platelet proteins probed with auto-antibodies induced by P. yoelii, P. chabaudi, B. rodhaini and B. microti. infection. (A) Representative 2DE map of murine platelet proteins. (B) Western blot analysis of murine platelet proteins probed with auto-antibodies induced by P. yoelii infection. (C) Western blot analysis of murine platelet proteins probed with auto-antibodies induced by P. chabaudi infection. (D) Western blot analysis of murine platelet proteins probed with auto-antibodies induced by B. rodhaini infection. (E) Western blot analysis of murine platelet proteins probed with auto-antibodies induced by B. microti infection. A representative 2DE map of murine platelet proteins was obtained by performing the first dimension (IEF) on IPG strips pH 3–10 and the second dimension on 4–12% gradient SDS-PAGE gels. The protein spots were visualized by Coomassie blue staining. The indicated spots were excised from the gel and identified by MS/MS.
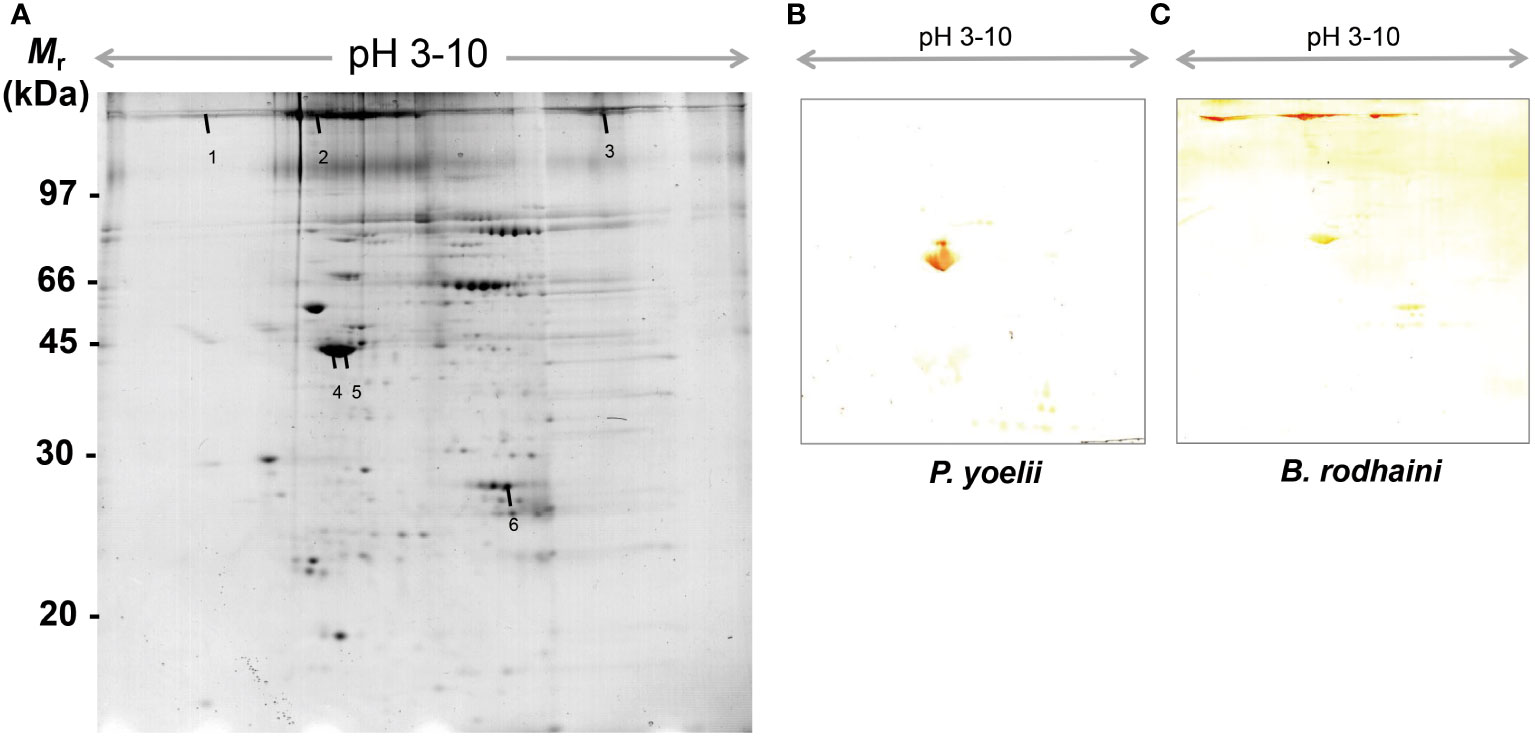
Figure 6 Reference image 2DE map of differentially expressed erythrocyte proteins with marked selected spots and Western blot analysis of murine erythrocyte proteins probed with auto-antibodies induced by P. yoelii and B. rodhaini infection. (A) Representative 2DE map of murine erythrocyte proteins. (B) Western blot analysis of murine erythrocyte proteins probed with auto-antibodies induced by P. yoelii infection. (C) Western blot analysis of murine erythrocyte proteins probed with auto-antibodies induced by B. rodhaini infection. Representative 2DE map of murine platelet proteins obtained by performing the first dimension (IEF) on IPG strips pH 3–10 and the second dimension on 4–12% gradient SDS-PAGE gels. The protein spots were visualized by Coomassie blue staining. The indicated spots were excised from gel and identified by MS/MS.
Comparative analysis of the antibody-binding proteins
The selected spots were analyzed by 2D LC-MS/MS after trypsinization to identify the autoimmune antibody-binding proteins. Our study combined Western blot, 2-DE, and proteomic analysis. All of the above proteins have been identified via analysis of the antibody-binding proteins. As shown in Tables 1 and 2, the MS/MS spectra have been analyzed. Masses of the peptides identified by LC-MS/MS were compared with sequences from the National Center for Biotechnology Information database (NCBI: http://www.ncbi.nlm.nih.gov/), separately. By using the ion score, we compare the fragment ions to all tryptic peptides calculated from parasites and mice. Actin of murine erythrocyte and platelet is a common auto-antigen in Plasmodium spp. and Babesia spp. infected mice.
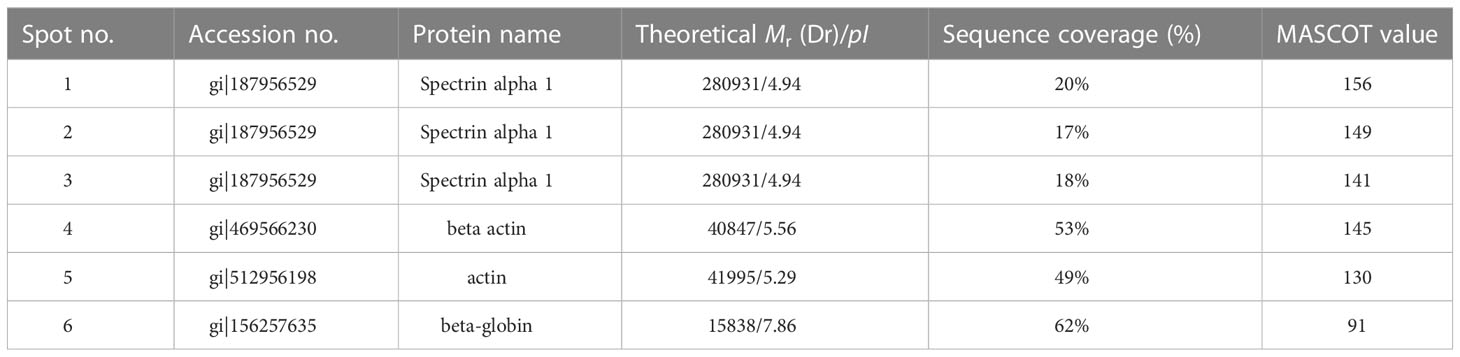
Table 1 Protein identification of murine erythrocyte proteins probed with anti-erythrocyte auto-antibodies.
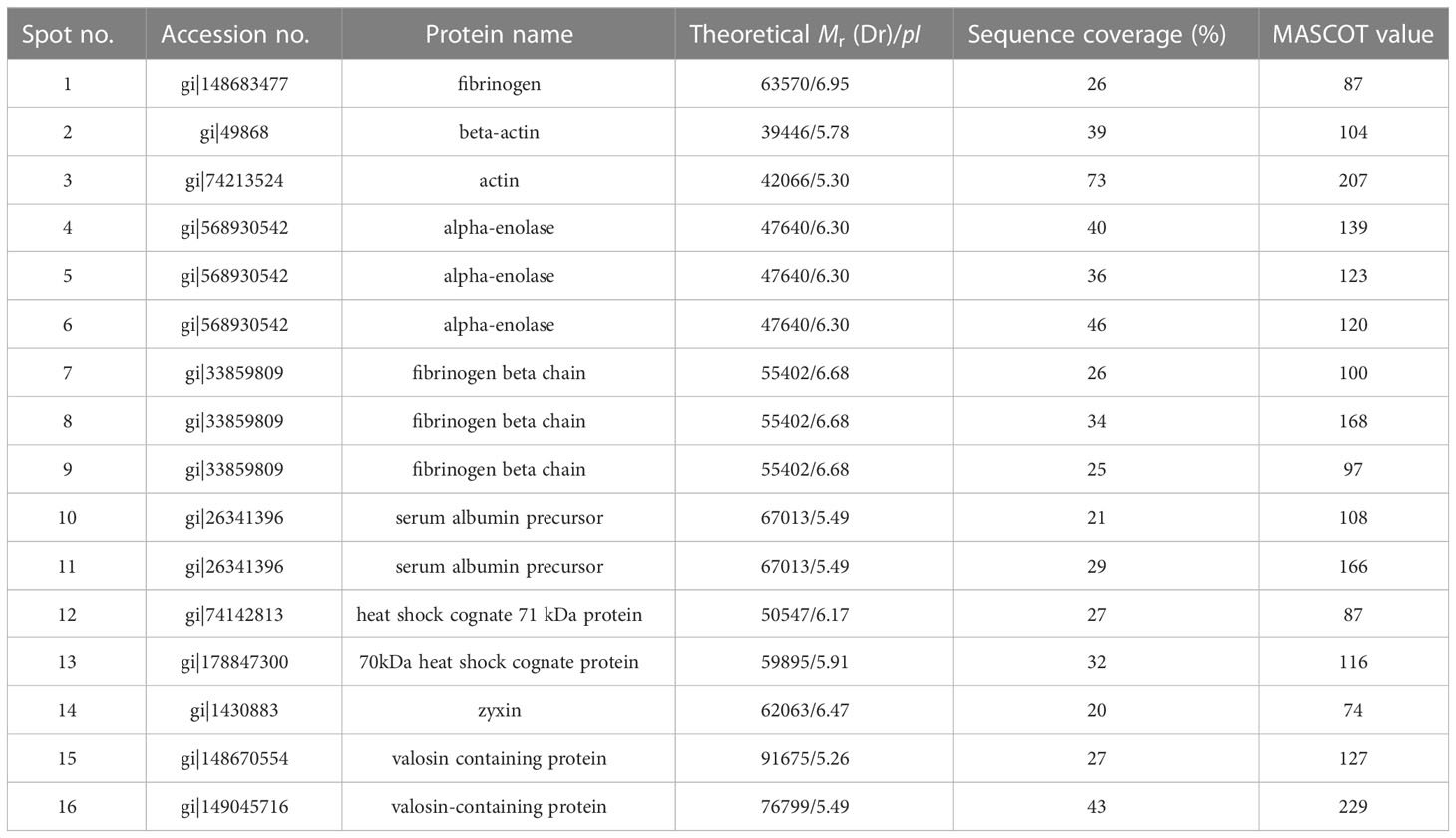
Table 2 Protein identification of murine platelet proteins probed with anti-platelet auto-antibodies.
Discussion
Malaria and babesiosis continue to be important diseases in the world. The varied presentations of these diseases and their diversity in terms of hematological manifestations have been well endowed in literature (Zhan et al., 2019; Wang et al., 2020; Varshavsky et al., 2021; Voisin et al., 2021). The most common hematological complications of malaria and babesiosis are thrombocytopenia and anemia. Anemia is caused by a various of pathophysiologic mechanisms, including accelerated RBCs removal by the spleen, obligatory RBCs destruction at parasite schizogony, and ineffective erythropoiesis (Nie et al., 2021; Rizk et al., 2021; Rizk et al., 2022). Recent advancements have shown that a variety of cytokine dysregulations are indeed vital participants in inducing and accelerating the pathogenesis of hemolysis in malaria and babesiosis. They include a significant increase in IFN-γ, IL-6 and IL-1 and a decrease in IL-10 and IL-12 levels. In patients with malaria and babesiosis, autoimmune hemolytic anemia (AIHA) has been described previously (Narurkar et al., 2017; Santos et al., 2020; Rajapakse and Bakirhan, 2021). Several parasite and virus infections have been reported to be associated with AIHA, such as influenza virus, Leishmania species, hepatitis virus, and cytomegalovirus. Multiple studies have documented the high frequency of thrombocytopenia in malaria patients (Rodríguez-Morales et al., 2005; Coelho et al., 2013). For more than four decades, researchers have investigated the pathogenesis of malaria thrombocytopenia, but it remains unclear how it occurs (McMorran et al., 2009). According to some studies, malaria may cause low platelet counts due to activation or apoptosis of platelets, which prevents the immune system from removing them. Nevertheless, malarial antigens have also been implicated in sequestering injured platelets in the spleen due to immune complexes formed. In addition, there are some evidences of platelet-associated IgG involvement in malaria thrombocytopenia. Immune-mediated hemolytic anemia and thrombocytopenia in malaria and babesiosis has gathered more attentions in recent years (Lacerda et al., 2011).
We investigated the autoimmune-mediated hemolytic anemia and thrombocytopenia during Plasmodium spp. and Babesia spp. infection in this study. High levels of anti-platelet auto-antibodies were found in P. yoelii, P. chabaudi, B. rodhaini and B. microti infected ICR mice. In contrast, SCID mice displayed lower level of anti-platelet auto-antibodies. There was obvious relation between platelet count and anti-platelet. According to the findings of a previous study, acute malaria and babesiosis infection are associated with AIHA. B and T lymphocytes are the important inducers of the immune effector mechanisms, which are needed for initial control of Plasmodium spp. and Babesia spp. infection. Therefore, the SCID mice are unable to produce anti-platelet auto-antibodies (Watier et al., 1992; Wheeler et al., 2011; Aschermann et al., 2013). Our data indicate that the absence of B and T lymphocytes impaired the production of anti-platelet auto-antibodies. There are a few possible reasons of the presence of anti-platelet auto-antibodies. Such as the erythrocyte share some similar peptides with platelet, which induced auto-antibodies against both, or the broken erythrocyte induced disrupt of some platelet, which consequently induced anti-platelet auto-antibodies thus lead to more platelet disruption and more antibody. We found that ICR mice infected with Plasmodium and Babesia species emitted significantly higher levels of IL-10 and IFN-γ. It is a strong support for the idea that the timing and magnitude of specific cytokines influence the severity of malaria and babesiosis. It is necessary to study the cytokine production in Plasmodium spp. and Babesia spp. infected mice.
In addition, chronic infection with Plasmodium spp. and Babesia spp. was established after the Plasdodium spp. infectedmice were treated by artesunate, and the Babesia spp. infectedmice were treated by diminaze. The trend of parasitemia was similar in Plasmodium spp. and Babesia spp. infected mice, a negative correlation was observed between parasitemia and hematocrit. Moreover, The IgM and IgG reached to the highest level after the day of high parasitemia (Boes et al., 2000). These observations suggest that the production of anti-erythrocyte autoantibody is not the main reason of anemia. Generally, platelet antibodies in ITP are IgG or IgM, but IgA and IgE have also been reported (Klaassen et al., 1989). IgG are responsible for interacting with macrophages in the reticuloendothelial system when antibodies bind to platelets. Complement-mediated lysis can also remove antibodies-sensitized platelets from circulation. Therefore, the platelet-associated IgG and IgM were comparably elevated in the majority of Plasmodium spp. and Babesia spp. infected mice, and the IgG might be the majority of platelet antibodies in ITP. In this study, the anti-platelet IgG2a and low amounts of IgG1 and IgG2b also was detected after Plasmodium spp. and Babesia spp. infection.
The autoimmune antibody-binding proteins were identified in the study. The autoimmune antibody-binding proteins identified for platelet and RBC membrane were similar in both subcellular location and function categories. In contrast, membrane-associated cytoskeleton proteins from platelet and RBCs membrane was found (Tables 1, 2). Though we could not rule out that the actual number of platelet and RBC membrane proteins, which were more than that of the membrane-associated cytoskeleton proteins may affect the production of autoimmune antibodies. The membrane associated cytoskeleton proteins triggering the autoimmune response in Plasmodium spp. and Babesia spp.infected mice require further characterization.
In conclusion, we have demonstrated that the autoimmune response is elicited during Plasmodium spp. and Babesia spp. infection. The autoimmune antibody may participate in thrombocytopenia and hemolytic anemia and regulate the autoimmune response. As a result of this research, we can develop an effective babesiosis and malaria therapeutic that modulates autoimmune responses for overcoming infection. Understanding of the effector molecules that inhibit autoimmune responses may provide important clues for future infection control strategies. In addition to antibiotics for the treatment of malaria and babesiosis, ITP treatment should be initiated in severe cases.
Data availability statement
The datasets presented in this study can be found in online repositories. The names of the repository/repositories and accession number(s) can be found in the article/supplementary material.
Ethics statement
The animal study was reviewed and approved by Animal Care and Use in Research Committee Promulgated by Obihiro University of Agriculture and Veterinary Medicine, Japan (Permit Number: 201109–5).
Author contributions
XX, SC, and MZ designed the study. MZ, SC carried out the experiments. OK and YN provide technical support. MZ, SC, JX, SJ, SZ, and XX wrote and read the manuscript, and all authors reviewed the manuscript. All authors contributed to the article and approved the submitted version.
Funding
This work was supported by a grant from the key project of Jiangsu Province’s Key Research and Development Plan (modern Agriculture) (BE2020407), the project of Jiangsu Agri-animal Husbandry Vocational College (NSF2022CB04, NSF2022CB25), the Division of Swine Infectious disease prevention and control (NSF2023TC01) and the Qing Lan Project of Jiangsu Province.
Conflict of interest
The authors declare that the research was conducted in the absence of any commercial or financial relationships that could be construed as a potential conflict of interest.
Publisher’s note
All claims expressed in this article are solely those of the authors and do not necessarily represent those of their affiliated organizations, or those of the publisher, the editors and the reviewers. Any product that may be evaluated in this article, or claim that may be made by its manufacturer, is not guaranteed or endorsed by the publisher.
References
Aschermann, S., Lehmann, C. H., Mihai, S., Schett, G., Dudziak, D., Nimmerjahn, F. (2013). B cells are critical for autoimmune pathology in scurfy mice. Proc. Natl. Acad. Sci. U.S.A. 110, 19042–19047. doi: 10.1073/pnas.1313547110
Boes, M., Schmidt, T., Linkemann, K., Beaudette, B. C., Marshak-Rothstein, A., Chen, J. (2000). Accelerated development of IgG autoantibodies and autoimmune disease in the absence of secreted IgM. Proc. Natl. Acad. Sci. U.S.A. 97, 1184–1189. doi: 10.1073/pnas.97.3.1184
Coelho, H. C., Lopes, S. C., Pimentel, J. P., Nogueira, P. A., Costa, F. T., Siqueira, A. M., et al. (2013). Thrombocytopenia in plasmodium vivax malaria is related to platelets phagocytosis. PloS One 8, e63410. doi: 10.1371/journal.pone.0063410
Ji, S., Liu, M., Galon, E. M., Rizk, M. A., Li, J., Li, Y., et al. (2021). In vitro screening of novel anti-babesia gibsoni drugs from natural products. Parasitol. Int. 85, 102437. doi: 10.1016/j.parint.2021.102437
Klaassen, R. J., van der Lelie, J., Vlekke, A. B., Weigel, H. M., Eeftinck Schattenkerk, J. K., Reiss, P., et al. (1989). The serology and immunochemistry of HIV-induced platelet-bound immunoglobulin. Blut 59, 75–81. doi: 10.1007/BF00320253
Lacerda, M. V., Mourão, M. P., Coelho, H. C., Santos, J. B. (2011). Thrombocytopenia in malaria: Who cares. Mem. Inst. Oswaldo Cruz 106 Suppl 1, 52–63. doi: 10.1590/s0074-02762011000900007
Li, Y., Terkawi, M. A., Nishikawa, Y., Aboge, G. O., Luo, Y., Ooka, H., et al. (2012). Macrophages are critical for cross-protective immunity conferred by babesia microti against babesia rodhaini infection in mice. Infect. Immun. 80, 311–320. doi: 10.1128/IAI.05900-11
Li, M., Yang, X., Masoudi, A., Xiao, Q., Li, N., Wang, N., et al. (2022). The regulatory strategy of proteins in the mouse kidney during babesia microti infection. Exp. Parasitol. 235, 108232. doi: 10.1016/j.exppara.2022.108232
McMorran, B. J., Marshall, V. M., de Graaf, C., Drysdale, K. E., Shabbar, M., Smyth, G. K., et al. (2009). Platelets kill intraerythrocytic malarial parasites and mediate survival to infection. Science 323, 797–800. doi: 10.1126/science.1166296
Michel, M., Lee, K., Piette, J. C., Fromont, P., Schaeffer, A., Bierling, P., et al. (2002). Platelet autoantibodies and lupus-associated thrombocytopenia. Br. J. Haematol. 119, 354–358. doi: 10.1046/j.1365-2141.2002.03817.x
Narurkar, R., Mamorska-Dyga, A., Nelson, J. C., Liu, D. (2017). Autoimmune hemolytic anemia associated with babesiosis. biomark. Res. 5, 14. doi: 10.1186/s40364-017-0095-6
Nie, Z., Ao, Y., Wang, S., Shu, X., Li, M., Zhan, X., et al. (2021). Erythrocyte adhesion of merozoite surface antigen 2c1 expressed during extracellular stages of babesia orientalis. Front. Immunol. 12. doi: 10.3389/fimmu.2021.623492
Rajapakse, P., Bakirhan, K. (2021). Autoimmune hemolytic anemia associated with human babesiosis. J. Hematol. 10, 41–45. doi: 10.14740/jh820
Rizk, M. A., Baghdadi, H. B., El-Sayed, S., Eltaysh, R., Igarashi, I. (2022). Repurposing of the malaria box for babesia microti in mice identifies novel active scaffolds against piroplasmosis. Parasit Vectors 15, 329. doi: 10.1186/s13071-022-05430-4
Rizk, M. A., El-Sayed, S., Alkhoudary, M. S., Alsharif, K. F., Abdel-Daim, M. M., Igarashi, I. (2021). Compounds from the medicines for malaria venture box inhibit In vitro growth of babesia divergens, a blood-borne parasite of veterinary and zoonotic importance. Molecules 26, 7118. doi: 10.3390/molecules26237118
Rizk, M. A., El-Sayed, S., Nassif, M., Mosqueda, J., Xuan, X., Igarashi, I. (2020). Assay methods for in vitro and in vivo anti-babesia drug efficacy testing: Current progress, outlook, and challenges. Vet. Parasitol. 279, 109013. doi: 10.1016/j.vetpar.2019.109013
Rodríguez-Morales, A. J., Sánchez, E., Vargas, M., Piccolo, C., Colina, R., Arria, M., et al. (2005). Occurrence of thrombocytopenia in plasmodium vivax malaria. Clin. Infect. Dis. 41, 130–131. doi: 10.1086/430837
Santos, M. A., Tierney, L. M., Jr, Manesh, R. (2020). Babesiosis-associated warm autoimmune hemolytic anemia. J. Gen. Intern. Med. 35, 928–929. doi: 10.1007/s11606-019-05506-5
Sporn, Z. A., Fenves, A. Z., Sykes, D. B., Al-Samkari, H. (2021). Severe babesiosis with associated splenic infarcts and asplenia. Proc. IEEE Glob Humanit Technol. Conf 34, 597–599. doi: 10.1080/08998280.2021.1930632
Tao, Z. Y., Liu, W. P., Dong, J., Feng, X. X., Yao, D. W., Lv, Q. L., et al. (2020). Purification of plasmodium and babesia- infected erythrocytes using a non-woven fabric filter. Trop. BioMed. 37, 911–918. doi: 10.47665/tb.37.4.911
Thapa, R., Biswas, B., Mallick, D., Sardar, S., Modak, S. (2009). Childhood plasmodium vivax malaria with severe thrombocytopenia and bleeding manifestations. J. Pediatr. Hematol. Oncol. 31, 758–759. doi: 10.1097/MPH.0b013e3181b7eb12
Tołkacz, K., Rodo, A., Wdowiarska, A., Bajer, A., Bednarska, M. (2021). Impact of babesia microti infection on the initiation and course of pregnancy in BALB/c mice. Parasit Vectors 14, 132. doi: 10.1186/s13071-021-04638-0
Ueda, Y. (2022). [Advances in understanding the pathogenesis and treatment of autoimmune hemolytic anemia]. Rinsho Ketsueki 63, 1014–1025. doi: 10.11406/rinketsu.63.1014
Vanheer, L. N., Kafsack, B. (2021). Activity comparison of epigenetic modulators against the hemoprotozoan parasites babesia divergens and plasmodium falciparum. ACS Infect. Dis. 7, 2277–2284. doi: 10.1021/acsinfecdis.0c00853
Varshavsky, T., Cuthbert, D., Riggs, R. (2021). Babesiosis in the emergency department: A case report. J. Emerg. Med. 61, e7–e10. doi: 10.1016/j.jemermed.2021.01.040
Voisin, O., Monpierre, L., Le Lorc'h, E., Pilmis, B., Le Monnier, A., Mourad, J. J., et al. (2021). A typical babesiosis in an immunocompetent patient. Ann. Biol. Clin. (Paris) 79, 456–459. doi: 10.1684/abc.2021.1675
Waked, R., Krause, P. J. (2022). Human babesiosis. Infect. Dis. Clin. North Am. 36, 655–670. doi: 10.1016/j.idc.2022.02.009
Wang, F., Jiang, J. F., Tian, J., Du, C. H. (2020). [Clinical characteristics, diagnosis and treatment of human babesiosis: A review]. Zhongguo Xue Xi Chong Bing Fang Zhi Za Zhi 33, 218–224. doi: 10.16250/j.32.1374.2020168
Watier, H., Verwaerde, C., Landau, I., Werner, E., Fontaine, J., Capron, A., et al. (1992). T-Cell-dependent immunity and thrombocytopenia in rats infected with plasmodium chabaudi. Infect. Immun. 60, 136–142. doi: 10.1128/iai.60.1.136-142.1992
Wheeler, K., Tardif, S., Rival, C., Luu, B., Bui, E., Del Rio, R., et al. (2011). Regulatory T cells control tolerogenic versus autoimmune response to sperm in vasectomy. Proc. Natl. Acad. Sci. U.S.A. 108, 7511–7516. doi: 10.1073/pnas.1017615108
Yu, L., Liu, Q., Luo, W., Zhao, J., Alzan, H. F., He, L. (2021). The structural basis of babesia orientalis lactate dehydrogenase. Front. Cell Infect. Microbiol. 11. doi: 10.3389/fcimb.2021.790101
Yuan-Yuan, L., Heng, P., Huai-Min, Z., Jian, L., Shao-Li, X. (2016). [Investigation of two blood parasitic protozoa infection in farmed macaca fascicularis in guangxi zhuang autonomous region]. Zhongguo Xue Xi Chong Bing Fang Zhi Za Zhi 28, 141–145. doi: 10.16250/j.32.1374.2015241
Zhan, X., He, J., Yu, L., Liu, Q., Sun, Y., Nie, Z., et al. (2019). Identification of a novel thrombospondin-related anonymous protein (BoTRAP2) from babesia orientalis. Parasit Vectors 12, 200. doi: 10.1186/s13071-019-3457-0
Zoleko Manego, R., Koehne, E., Kreidenweiss, A., Nzigou Mombo, B., Adegbite, B. R., Dimessa Mbadinga, L. B., et al. (2019). Description of plasmodium falciparum infections in central Gabon demonstrating high parasite densities among symptomatic adolescents and adults. Malar. J. 18, 371. doi: 10.1186/s12936-019-3002-9
Zottig, V. E., Shanks, G. D. (2021). Historical perspective: The evolution of post-exposure prophylaxis for vivax malaria since the Korean war. MSMR 28, 8–10.
Zowonoo, F., Iverson, G., Doyle, M., Richards, S. L. (2023). Retrospective spatiotemporal analysis of malaria cases reported between 2000 and 2020 in north Carolina, USA. Travel Med. Infect. Dis. 51, 102505. doi: 10.1016/j.tmaid.2022.102505
Keywords: malaria, Babesiosis, thrombocytopenia, anemia, anti-erythrocyte auto-antibodies, anti-platelet auto-antibodies
Citation: Zhou M, Xie J, Kawase O, Nishikawa Y, Ji S, Zhu S, Cao S and Xuan X (2023) Characterization of anti-erythrocyte and anti-platelet antibodies in hemolytic anemia and thrombocytopenia induced by Plasmodium spp. and Babesiaspp. infection in mice. Front. Cell. Infect. Microbiol. 13:1143138. doi: 10.3389/fcimb.2023.1143138
Received: 12 January 2023; Accepted: 20 March 2023;
Published: 14 April 2023.
Edited by:
Kun Li, Nanjing Agricultural University, ChinaReviewed by:
De-Hua Lai, Sun Yat-sen University, ChinaRuttayaporn Ngasaman, Prince of Songkla University, Thailand
Simon Peter Musinguzi, Kyambogo University, Uganda
Copyright © 2023 Zhou, Xie, Kawase, Nishikawa, Ji, Zhu, Cao and Xuan. This is an open-access article distributed under the terms of the Creative Commons Attribution License (CC BY). The use, distribution or reproduction in other forums is permitted, provided the original author(s) and the copyright owner(s) are credited and that the original publication in this journal is cited, in accordance with accepted academic practice. No use, distribution or reproduction is permitted which does not comply with these terms.
*Correspondence: Shinuo Cao, c2hpbnVvX2Nhb0AxNjMuY29t; Xuenan Xuan, Z2VuQG9iaWhpcm8uYWMuanA=
†These authors have contributed equally to this work