- 1Department of Dermatology, Osaka University Graduate School of Medicine, Osaka, Japan
- 2Cutaneous Allergy and Host Defense, Immunology Frontier Research Center, Osaka University, Osaka, Japan
- 3Department of Pathology and Rogel Cancer Center, University of Michigan Medical School, Ann Arbor, MI, United States
The skin is home to various bacteria, archaea, fungi, and viruses, collectively referred to as the skin microbiota. Patients with certain skin diseases reportedly have unique skin “dysbiosis,” a condition involving imbalanced microbiota, suggesting that dysbiosis in the skin may be either causal or a consequence of specific skin diseases. Atopic dermatitis (AD) is the most common allergic skin disease that affects 15-20% of children and 2-10% of adults worldwide. Both intrinsic genetic factors, such as susceptibility to type 2 inflammation or skin barrier dysfunction, and extrinsic environmental factors, such as air pollen and skin microbiota, contribute to AD. Staphylococcus aureus, which does not often colonize the skin of healthy individuals, is commonly identified in the lesional skin of patients with AD and is correlated with the disease flare. However, the role of S. aureus in the pathogenesis of AD has not been elucidated. Here, we discuss the pathological behavior of S. aureus, focusing on accessory gene regulator (Agr) quorum sensing, which is a fundamental bacterial cell-to-cell interaction mechanism that affects the behavior of S. aureus and other members of the microbial community. Importantly, beyond bacteria-bacteria interactions, the Agr quorum sensing system also regulates various virulence factors, which induce type 2 and IL-17-dependent skin inflammation in the host. Furthermore, the colonization of Agr-positive S. aureus in early life accelerates the development of pediatric AD. Finally, we aim to highlight the current efforts to establish novel therapeutic methods to ameliorate or prevent AD through Agr-targeted intervention.
Introduction
The skin is the largest organ of the human body and plays several crucial roles in maintaining human health. It is a physical barrier that protects the host from bacterial invasion and harmful external environmental factors (Langan et al., 2020). The skin contains a diverse microbial population comprising bacteria, fungi, and viruses, referred to as the microbiota. Recent studies have revealed a correlation between the microbiota and various diseases, including atopic dermatitis (AD) (Cani and Jordan, 2018; Paller et al., 2019). AD is a chronic inflammatory skin disorder that affects a significant proportion of the population in industrialized nations, with a prevalence of 20% in children and approximately 5% in adults in high-income countries (Langan et al., 2020). AD is clinically characterized by red, dry and itchy skin lesion. Sleep disturbance due to severe itching is one of the major symptoms of AD, and it can significantly impair patients’ and their caregivers’ quality of life and social productivity (Chang and Chiang, 2018).
AD is a complex disease characterized by a Th2 cell-mediated immune response, which is the central mechanism underlying its pathogenesis (Gittler et al., 2012). In acute phase of AD, the skin barrier is disrupted and allergens are presented to antigen-presenting cells bearing specific IgE to enhance Th2 response (Werfel et al., 2016). Thymic stromal lymphopoietin (TSLP) and interleukins released from keratinocytes stimulate Th2 cell and Langerhans cell development. Th2 cell subsequently produces cytokines, Interleukin (IL)-4, IL-13, and IL-31, which directly activate sensory nerves and promote pruritus (Sonkoly et al., 2006). At the same time, IL-4, IL-13 induces inflammatory cytokines and decreases keratinocytes production of filaggrin, loricrin, and involucrin, further decreasing the barrier function (Kim et al., 2008; Werfel et al., 2016). In contrast, in the chronic phase, the expression of the type-1 cytokine such as interferon-gamma are heightened (Werfel et al., 1996; Tsoi et al., 2020). It is also linked to the activities of various T cell subsets, such as the Th22, Th17/IL-23, and Th1 cytokine pathways (Gittler et al., 2012). Although various immune dysfunctions related to AD have been widely investigated, the underlying causes of aberrant immune responses remain unclear. The development of AD is believed to result from the interplay between environmental factors, as represented by the hygiene hypothesis, and the host’s genetic background (Flohr et al., 2005). One such environmental factor is skin dysbiosis. Our group has made important discoveries on the relationship between AD and Staphylococcus. aureus. (Nakamura et al., 2013; Nakagawa et al., 2017; Nakamura et al., 2020) Here, we review the relationship between skin dysbiosis and AD, focusing on the accessory gene regulator (Agr) quorum sensing mechanism, which plays an essential role in the virulence of S. aureus.
The role of the skin microbiota in host immunity
The surface area of an adult individual’s skin including not only exposed interfollicular epithelium but the epithelial lining of hair follicles, eccrine ducts, apocrine ducts, and sebaceous glands encompasses approximately at least 30 square meters and harbors an estimated 40 distinct bacterial strains, with a concentration of 106 bacteria per square centimeter (Belkaid and Segre, 2014; Gallo, 2017). The skin microbiota of neonates is acquired during parturition and varies depending on the delivery mode. Neonates born via vaginal delivery possess skin microbiota similar to the maternal vaginal microbiota, whereas those born via cesarean section exhibit skin microbiota similar to the maternal skin microbiota (Mueller et al., 2015). As individuals age, skin microbial composition gradually shifts. During puberty, the skin microbiota undergoes significant changes, particularly in areas where the sebaceous glands develop, leading to a stable condition of skin microbial communities in adults (Oh et al., 2012; Si et al., 2015). The normal skin microbiota obtained through this process plays a vital role in the host’s immune system (Chen et al., 2018). Human skin acquires memory regulatory T cells progressively from the neonatal to adult stages, which contribute to developing immune tolerance against commensal bacteria. One study showed that the colonization of Staphylococcus epidermidis, a representative normal skin microbiota species, during the neonatal period causes CD4+ T regulatory cells to migrate to the skin and generate tolerance towards commensals in mice (Naik et al., 2015; Scharschmidt et al., 2015). In contrast, IL-17A+ CD8+ T cells act as a defensive mechanism against pathogenic microorganisms (Scharschmidt et al., 2015). Additionally, in adult mice, S. epidermidis on the skin stimulates the migration of IL-17A+ CD8+ T cells, enhancing innate immunity against pathogens such as Candida albicans and Leishmania major (Belkaid et al., 2002; Naik et al., 2015). Among staphylococci, S. epidermidis, and Staphylococcus hominis are representative commensal species of coagulase-negative staphylococci in human skin (Byrd et al., 2018), and produce antimicrobial peptides that target pathological bacteria, such as S. aureus. Commensal staphylococci also stimulate the host to produce antimicrobial peptides, including β-defensin-2, which aid in eliminating pathogenic microorganisms (Nagy et al., 2006; Gallo and Hooper, 2012; Zipperer et al., 2016; Nakatsuji et al., 2017). Thus, the resident bacterial flora plays an essential role in maintaining the balance of the host skin immune system in a healthy state (Sanchez Rodriguez et al., 2014; Naik et al., 2015).
Skin microbiota and Staphylococcus virulence factors in AD
An initial study, which was conducted in the 1960s, revealed that individuals with AD had the presence of S. aureus in their affected skin (Selwyn, 1963). In 1974, a significant amount of S. aureus was found on the skin of patients with AD, even in cases with no visible signs of infection (Leyden et al., 1974). Recent studies have revealed that disturbances in normal microbiota or dysbiosis can be an underlying cause or consequence of various diseases (Sanford and Gallo, 2013). Patients with AD typically exhibit a distinctive composition of skin microbiota, characterized by reduced richness, depletion of some commensal bacteria, and enrichment of staphylococci (Kong et al., 2012; Tay et al., 2021). Specifically, skin lesions of patients with AD often show colonization by pathogenic S. aureus, which does not normally colonize the skin of healthy individuals, and abnormal growth of S. epidermidis (Kong et al., 2012; Byrd et al., 2017). The percentage of individuals carrying S. aureus in their lesional skin area varied between reports. According to a meta-analysis of 95 observational studies among patients with AD, the prevalence of S. aureus colonization on lesional skin was 70%. In contrast, it was only 39% in non-lesional or healthy control skin (Totté et al., 2016). Additionally, the prevalence of S. aureus colonization increases with disease severity (Totté et al., 2016). Furthermore, real-time PCR has revealed that the density of S. aureus correlates with disease severity (Tauber et al., 2016).
Presumably, molecules of S. aureus, such as α-hemolysin (Hla) (Travers et al., 2003), lipoteichoic acid (LTA) (Travers et al., 2010), peptidoglycan (PGN) (Matsui and Nishikawa, 2012), and staphylococcal protein A (SPA), are involved in inflammatory reactions of the skin. LTA and PGN from S. aureus have been reported to induce inflammatory responses in the skin as Toll-like receptor ligands (Travers et al., 2010; Matsui and Nishikawa, 2012). However, these molecules are also present in other commensal skin bacteria, and their specificity has not yet been demonstrated. Hla triggers potassium efflux upon insertion into the membrane of human keratinocytes, and topical application of Hla induces skin barrier disruption and inflammation in SKH-HR1 hairless mice (Travers et al., 2003; Hong et al., 2014). Although SPA can disrupt opsonization and phagocytosis by the host immune system and trigger IL-18 production by keratinocytes (Spika et al., 1981), topical application of SPA did not induce skin inflammation in a mouse model (Nakano et al., 2003). Superantigens (SAgs) such as toxic shock syndrome toxin-1 (TSST-1) and staphylococcal enterotoxins bind to major histocompatibility class II (MHC II) molecules on the surface of antigen-presenting cells and T cell receptors on T cells, bypassing the constraint of antigenic peptide presentation. Although these SAgs can cause toxic shock syndrome in humans, their effect on AD severity is not significant (Kozman et al., 2010; Rojo et al., 2014). However, these previous studies did not analyze the role of S. aureus pathogenic factors in the skin of mice epicutaneously colonized by live S. aureus. Therefore, whether these factors contribute to AD pathogenesis on the skin surface remains unclear.
In addition to S. aureus, S. epidermidis has also been reported as a pathogenic bacterium in AD. A previous report has mentioned that an increasing number of S. epidermidis in the lesional skin of patients with AD produce EcpA, which contributes to dermatitis (Cau et al., 2021). In contrast, individual strains of S. epidermidis can inhibit biofilm formation by S. aureus through the production of Esp, a serine protease present in normal microbiota (Iwase et al., 2010). Thus, the pathogenicity of S. epidermidis in AD is not as well established as that of S. aureus and remains debatable.
In summary, differences in the microbiota exist between the normal skin and the skin of patients with AD. Dysbiosis of the lesional skin, characterized by a shift in the proportion of staphylococci and an increase in pathogenic strains, contributes to the exacerbation of AD.
Staphycoccus aureus Agr quorum sensing in AD pathogenesis
Quorum Sensing (QS) is the ability of bacteria to detect the cell population density. When the bacterial population is high, they are at risk of nutrient scarcity. Thus, bacteria change their behavior by regulating the production of various genes and substances to obtain more nutrients from the host or eliminate bacteria with similar nutritional requirements. S. aureus possesses an auto-regulatory operon, Agr system, as a QS function. S. aureus, similar to a pheromone, secretes an exocrine auto-inducing peptide (AIP) to communicate with and sense its species. When the population density is high, and AIP reaches the threshold for activating the transmembrane receptor (AgrC) on the cell surface, autophosphorylation of the histidine kinase AgrC is triggered. AgrC binds to AgrA, a DNA-binding response regulator, and activates AgrA via phosphorylation. AgrA directly binds to agr region to activate two bidirectional promoters, P2 and P3. Under the P2 promoter regulation, agrB encodes the AIP transporter in the extracellular space and agrD, encoding the precursor of AIP, and agrC and agrA. There are four subcategories of Agr, exhibiting distinctions in the amino acid sequence of the mature autoinducing peptide (AIP) (Ji et al., 1997). Recently, the AIPs of groups 1, 2, and likely 4 are reported to be exported by AgrB and trimmed at the N-terminus by the non-Agr-encoded membrane-located protease MroQ (Stock et al., 2022; Zhao et al., 2022). Thus, the agr operon is activated in a positive-feedback manner once QS is triggered. In contrast, the P3 promoter regulates various toxins via RNAIII, a regulatory RNA (Le and Otto, 2015) (Figure 1).
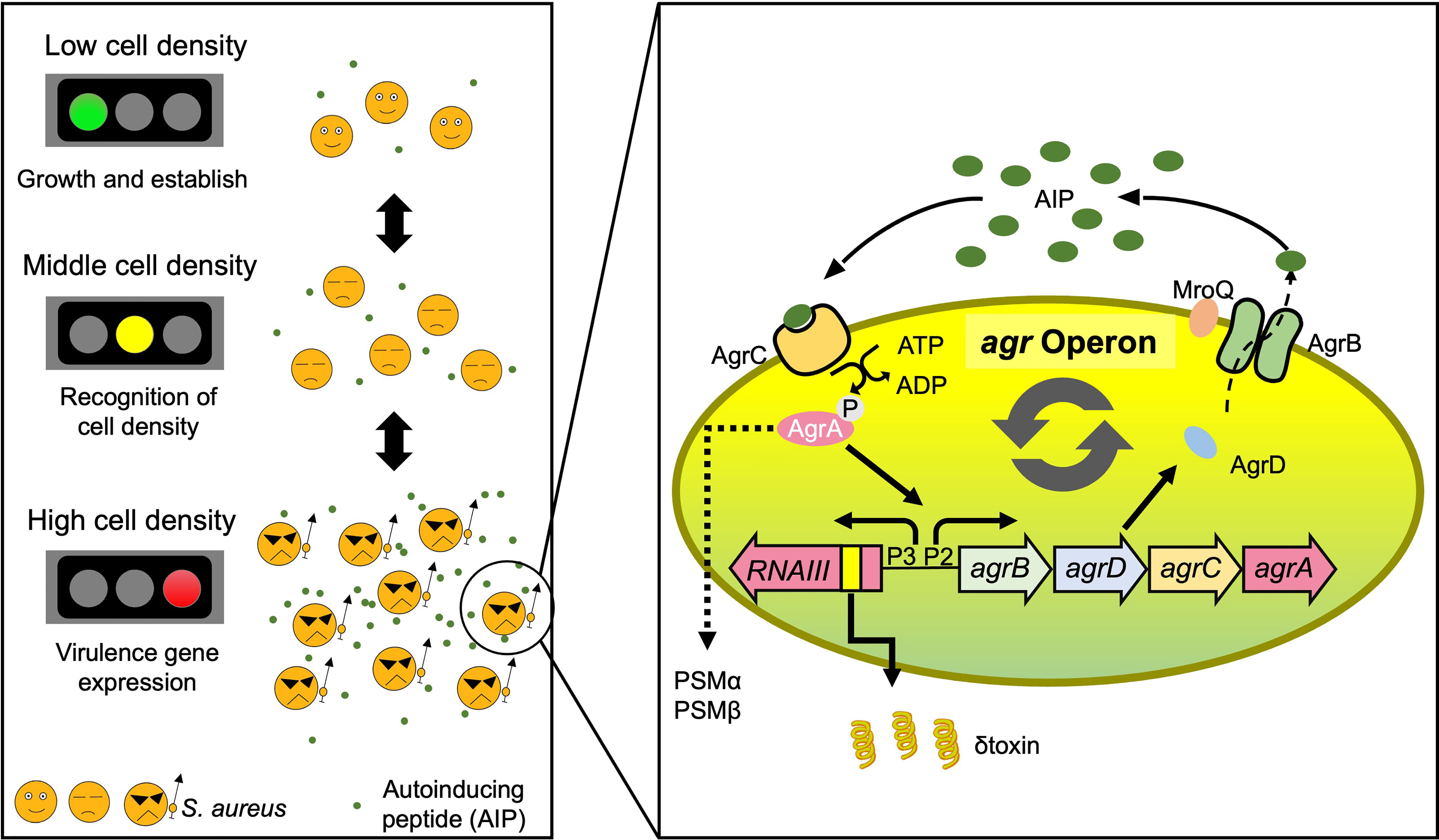
Figure 1 A proposed model for the role of S. aureus QS-regulated Agr virulence. S. aureus secretes AIP, thereby informing each other of their own cell population density, and when the concentration exceeds a certain threshold, they turn on virulence gene expression. The agr operon is activated in a positive feedback manner once QS is triggered by AIP. The AIPs of groups 1, 2, and likely 4 are reported to be exported by AgrB and trimmed at the N-terminus by the non-Agr-encoded membrane-located protease MroQ. The Agr-QS system controls the expression of several virulence factors in S. aureus. AD, atopic dermatitis; QS, quorum sensing; AIP, auto inducing peptide.
The phenol-soluble modulin (PSM) family is a major virulence factor of Staphylococcus and is regulated by the Agr-QS system (Wang et al., 2007; Otto, 2014). S. aureus possesses four PSMα peptides (PSMα1–4), two PSMβ peptides (PSMβ1 and PSMβ2), and δ-toxin (PSMγ). The psmα and psmβ operons are directly regulated by AgrA, while δ-toxin is encoded within the RNAIII operon and regulated under the P3 promoter (Le and Otto, 2015) (Figure 1).
S. aureus uses Agr-QS to behave differently in various situations. During chronic infections, S. aureus forms biofilms and exhibits strong antibiotic resistance when agr is suppressed (Schilcher and Horswill, 2020). However, as the biofilm matures, Agr-QS is turned on, and PSM production enables bacterial cells to detach from the biofilm and spread (Boles and Horswill, 2008). Additionally, α-type PSMs, particularly PSMα3, strongly induce neutrophil chemotaxis and Ca2+ flux, which are highly toxic to neutrophils (Wang et al., 2007; Otto, 2014). Using community-acquired methicillin-resistant S. aureus (CA-MRSA), the PSMα deletion strain, but not the other PSM deletion strains, had a significantly decreased ability to cause skin abscess formations in mice (Wang et al., 2007). These results suggest that PSMα, via Agr-QS activation, may contribute to developing CA-MRSA skin and soft tissue infections (Wang et al., 2007; Otto, 2014). S. aureus utilizes Agr-QS to control the expression of virulence factors during growth (Miller and Bassler, 2001). We have previously reported that Agr-QS in S. aureus plays a crucial role in skin adaptation and AD pathogenesis (Nakamura et al., 2013; Nakagawa et al., 2017). In the following sections, we elaborate on the pathogenic role of S. aureus Agr-QS in AD.
δ-toxin
Although the severity of AD is associated with the presence of S. aureus, the precise role of this bacterium in disease pathophysiology remained unclear (White and Noble, 1986). This may be because, despite the presence of bacteria in patients with AD on the outermost layer of the skin, the stratum corneum, the previous mouse models examining the pathogenicity of S. aureus in the skin utilized bacterial injection into the subcutaneous tissue (Yoshioka et al., 2003; Bin et al., 2012; Malachowa et al., 2013). To address this issue, we adapted an ovalbumin (OVA) epicutaneous sensitization model and established an epicutaneous S. aureus inoculation mouse model (Spergel et al., 1998; Nakamura et al., 2013). Using this model, we revealed that the expression of RNAIII, which is indicative of the positive expression of Agr-QS, was observed four days after epicutaneous inoculation of live S. aureus. Mice colonized with S. aureus developed severe dermatitis on day 7. Because we identified δ-toxin from S. aureus via activation of Agr-QS strongly inducing mast cell degranulation in vitro, we compared S. aureus wild-type and δ-toxin deficient strains in this model (Nakamura et al., 2013). Mice colonized with S. aureus lacking δ-toxin showed significantly reduced skin inflammation compared to wild-type colonized mice. In addition, precolonization with wild-type S. aureus, but not with δ-toxin deficient S. aureus, enhanced the production of OVA-specific IgE in OVA-exposed mice. These responses are abolished in mast cell-deficient mice. Thus, we found that Th2-type dermatitis was induced through mast cell degranulation triggered by δ-toxin under Agr-QS expression. Furthermore, we analyzed RNAIII expression in wash fluid obtained from the lesional and non-lesional skin of patients with AD and found that RNAIII expression was only upregulated in AD lesional skin, indicating the involvement of Agr-QS in AD exacerbation in humans (Nakamura et al., 2013; Nakagawa et al., 2017) (Figure 2).
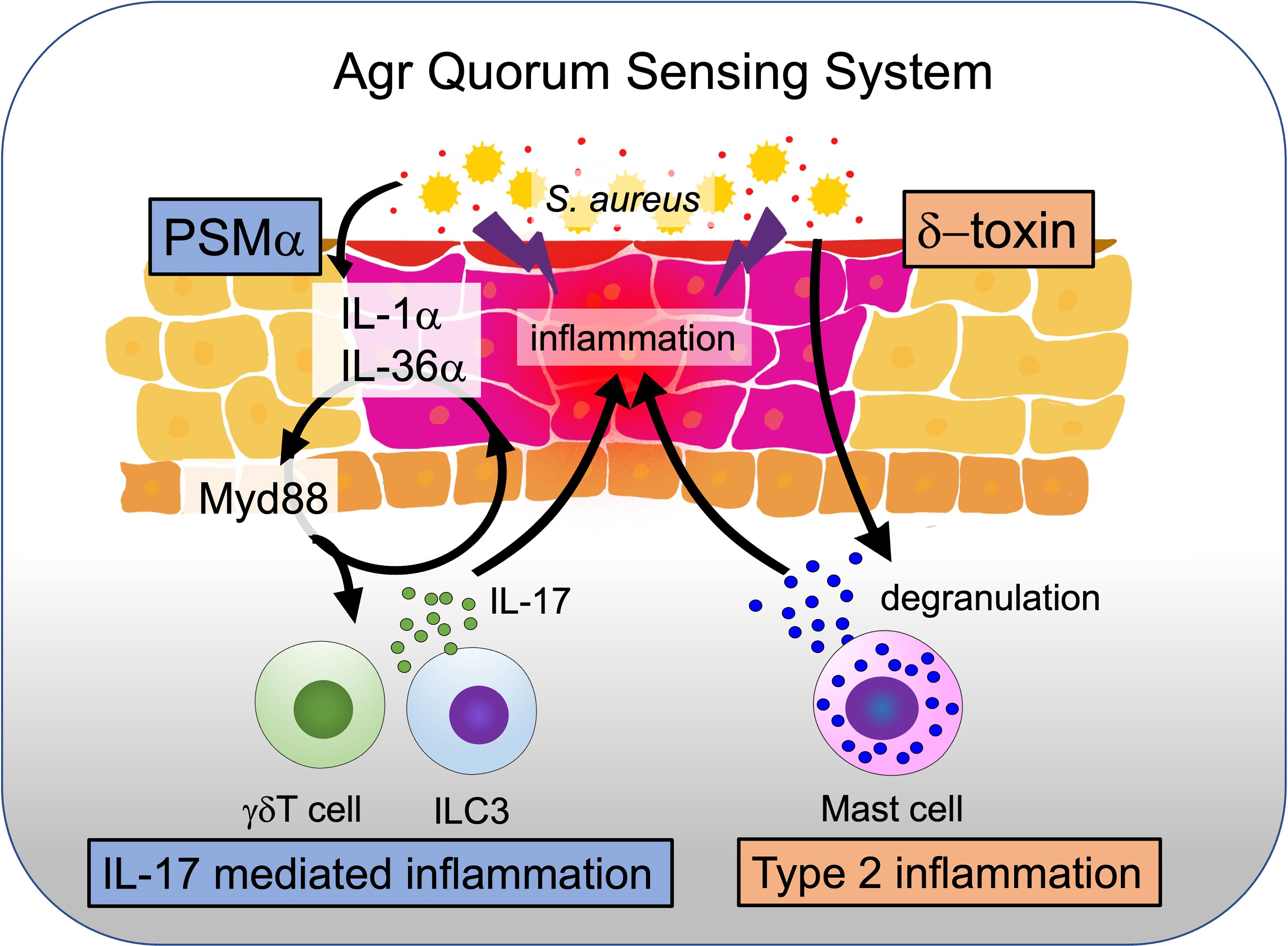
Figure 2 How δ-toxin and PSMα contribute to exacerbating AD. When S. aureus colonizes the skin surface and triggers the quorum sensing system, virulence factors such as δ-toxin and PSMα are produced. δ-toxin causes mast cell degranulation and triggers type II inflammation, while PSMα causes the release of IL-1α and IL-36α alarmin from keratinocytes and triggers IL17-mediated dermatitis.
PSMα
Our research group further elucidated how PSMα acts on keratinocytes to induce dermatitis (Nakagawa et al., 2017). Using primary keratinocytes, we found that PSMα induces epidermal keratinocyte cell death and stimulates the release and secretion of the alarmins IL-1α and IL-36α via Myd88, a signaling adaptor of IL-1 receptor (IL-1R) and IL-36 receptor (IL-36R). Furthermore, we performed epicutaneous S. aureus inoculation using IL-1R deficient (Ilr1-/-) mice and an IL-36R neutralizing antibody. Only Ilr1-/- mice treated with anti-IL-36R antibody, but not Ilr1-/- or IL-36R antibody-treated wild-type mice, showed dramatically suppressed dermatitis compared to wild-type mice, indicating that both IL-1 and IL-36 are important for the induction of skin inflammation in epidermal S. aureus inoculation. Additionally, IL-1R signaling plays an important role in Th17 cell differentiation (Chung et al., 2009). Flow cytometry analysis of cells from skin lesions in a mouse model of S. aureus epidermal inoculation showed a dramatic increase in IL-17A-producing cells and a small increase in cells producing IL-17F and IL-22, suggesting that IL-1R signaling is involved in Th17 cell differentiation and the subsequent induction of skin inflammation. When S. aureus was inoculated on IL-17A and IL-17F double-knockout mice lacking IL-17 signaling, skin inflammation was attenuated compared to that in wild-type mice, whereas the number of S. aureus grown on the skin was comparable. To identify the molecule responsible for S. aureus that releases alarmin from keratinocytes, we stimulated keratinocytes with culture supernatants of S. aureus wild-type and various toxin-deficient strains. The culture supernatant of the S. aureus wild-type strain, but not that of the PSMα deficient strain, strongly enhanced the release of alarmins from keratinocytes. PSMα was also essential for inducing IL-17-dependent dermatitis via the release of alarmins in our epicutaneous S. aureus inoculation model (Nakagawa et al., 2017). A previous report also demonstrated that IL-17 had been reported to be involved in the pathogenesis of AD in infancy (Esaki et al., 2016). The induction of IL-17-dependent dermatitis by PSMα suggests that the activated Agr-QS of S. aureus on the skin may be responsible for AD development (Figure 2).
Other molecules
Previously, it was reported that MroQ is an important mediator of S. aureus pathogenesis in strains harboring a type I and type II Agr variant (Zhao et al., 2022). MroQ functions as a membrane protease regulator of Agr and is also an essential component of AIP biosynthesis (Zhao et al., 2022). In a mouse intradermal infection model, a mroQ mutant of Agr type I showed a dramatic reduction in abscess formation (Stock et al., 2022). However, the importance of MroQ in an epicutaneous model has not been investigated, and a mroQ mutant was not found in infant skin isolates from an AD infant cohort study. Therefore, the role of MroQ in the pathogenesis of AD remains unclear. Another report mentioned that eight of 10 AD skin-adapted S. aureus strains harbored mutations in fumC and other metabolic genes and stimulate inflammatory responses in keratinocytes in vitro (Acker et al., 2019). Although an AD-associated mutant strain was also only tested in intradermal injection mouse model, a skin-adapted isolate failed to generate protection from a secondary infectious challenge (Acker et al., 2019).
Agr quorum sensing drives Staphycoccus aureus skin colonization in AD infants
Examination of the virulence factors modulated downstream of Agr-QS indicated that S. aureus exacerbates skin inflammation in AD. However, it remains unclear why S. aureus is disproportionately present in AD skin and rarely found in healthy skin. Therefore, to elucidate the mechanism of S. aureus colonization before the onset of AD, we conducted a cohort study to chronologically obtain skin microbiota from the cheeks of 268 Japanese infants 1-6 months after birth (Nakamura et al., 2020). Approximately 45% of the infants were colonized with S. aureus at one month; however, the presence or absence of this colonization did not affect the risk of developing AD at one year of age. In contrast, the risk of developing AD was significantly higher in infants colonized with S. aureus at six months. We examined the genomic differences between S. aureus strains associated with AD and healthy skin. Using whole-genome sequencing of S. aureus strains, we discovered that loss-of-function mutations in the agr locus of S. aureus occurred in strains from infants who did not develop AD at 1 year of age. In contrast, no mutations in this region have been identified in infants with AD (Nakamura et al., 2020). Furthermore, our study, using an epicutaneous S. aureus colonization mouse model, revealed that the Agr-QS system plays a critical role in the epidermal colonization of S. aureus and the development of AD-like inflammation (Nakamura et al., 2020). These results suggest that the presence of a functioning Agr-QS system in S. aureus isolates from AD-associated infant skin contributes to skin colonization and AD development. In contrast, the absence of this system, due to a loss-of-function mutation in healthy infant skin, leads to the elimination of S. aureus. Thus, the maintenance of pathogenicity through the Agr-QS system correlates with S. aureus skin colonization and the emergence of AD (Figure 3). In contrast, another report mentioned that human skin grafts maintained on severe combined immunodeficiency disease (SCID) mice to follow the consequences of S. aureus infection showed that many more agr mutant USA300 MRSA strain are seen in the infected graft compared to its wild-type strain (Soong et al., 2015). Even though this may seem contrary to what we observed in AD infant cohort (Nakamura et al., 2020), when considering the background of an immunodeficiency in SCID mice, it may reflect the importance of host immunity in the colonization and pathogenesis of S. aureus on the skin (Soong et al., 2015).
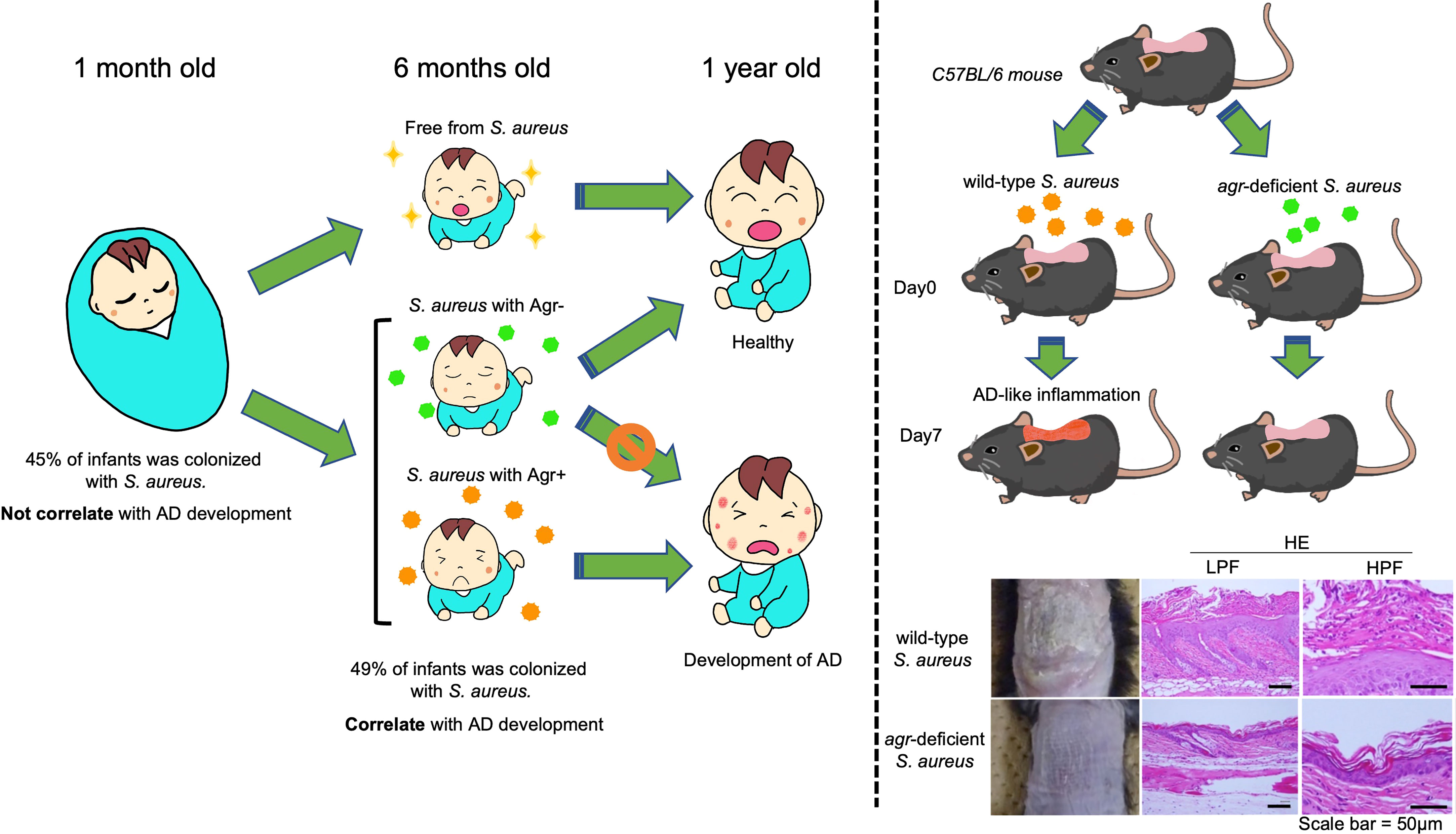
Figure 3 Agr quorum sensing drives S. aureus skin colonization in AD infants. The risk of developing AD was significantly higher in infants who were colonized with S. aureus at six months. The agr mutations of S. aureus occurred in strains from those infants who did not develop AD, while no agr mutations of S. aureus were identified in the infants who did develop AD. Epicutaneous S. aureus colonization mouse model revealed that the Agr-QS system plays a critical role in the development of AD-like inflammation. The photos and pathological images of mice were adapted from (Nakamura et al., 2020). HE, Hematoxylin-Eosin Stain; HPF, high power field; LPF low power field.
Interactions between Staphycoccus aureus and other bacterial species
The communication between bacteria in the human body has not been well understood until recently. Many researches are currently investigating the interactions of S. aureus with other bacterial species via QS and other molecules. Notably, recent work revealed that S. epidermidis can inhibit the formation of S. aureus biofilms by the secretion of Esp, a serine protease, which consequently inhibit the colonization of S. aureus upon epithelial surfaces (Iwase et al., 2010). In fact, in a Japanese epidemiological study analyzing S. epidermidis nasal colonization from 88 healthy volunteers, 44% of S. epidermidis strains were expressing Esp protease and those carrying “inhibitory type” S. epidermidis had significantly lower carriage rate of S. aureus in their nasal cavity (Iwase et al., 2010). After this discovery, other studies had also advanced the understanding of interactions between bacteria, particularly S. aureus and other bacteria species. For example, an in vivo study revealed that PSM γ and δ produced by S. epidermidis directly induce lipid vesicle leakage and exerted selective antimicrobial action against S. aureus. (Cogen et al., 2010) In another study, in 2016, a novel antimicrobial peptide named lugdunin, produced by Staphylococcus lugdunensis, was discovered and subsequently demonstrated to effectively inhibit the growth and colonization of S. aureus. (Zipperer et al., 2016) Whereas the presence of antibiotics pressure bacteria to adapt and eventually accelerate antimicrobial resistance in general, S. aureus incubated with lugdunin over a long period of time did not gain the resistance to lugdunin, letting this molecule a potential therapeutic drug to counter the drug-resistant S. aureus. (Zipperer et al., 2016) Moreover, a human microbiome-based study elucidated that coagulase-negative Staphylococcus (CoNS), which is predominant in the commensal flora, secrete lantibiotics, an antimicrobial peptides, to compete with S. aureus (Nakatsuji et al., 2017). Other researches are still investigating antimicrobial substances that do not act on commensal bacteria, but selectively on pathogenic bacteria such as S. aureus and Escherichia coli, and contribute to the stability of the resident microflora.
Other than the anti-microbial peptides, there is another attempt to inhibit S.aureus pathogenicity through inhibition of QS. These molecules with the capability of inhibiting the QS signals are named as Quorum Quenching (QQ) or quorum sensing inhibitor (QSI). Other staphylococci besides S. aureus also utilize AIPs for Agr-QS. Although these species share a common AIP structure, several AIP have different amino acid sequences. Thus, staphylococci with different types of AIP compete with each other, upregulating the expression of the Agr in bacteria of the same type of AIP while downregulating the expression of the agr in other staphylococci of different types of AIP (Ji et al., 1997; Otto et al., 2001). Most of the AIPs produced by non-S. aureus staphylococci have been found to act as quorum quenching against S. aureus. For example, S. hominis AIP inhibits the Agr of S. aureus and S. epidermidis (Severn et al., 2022). In addition to staphylococci, there are other bacterial genera that have the ability of QQ. In 2018, fengycins, a lipopeptide produced by Bacillus sp. were shown to inhibit S. aureus colonization of the nasal cavity and intestinal tract in humans and mice, through quorum quenching (Piewngam et al., 2018). Corynebacterium species, which are also important members of the human skin, seem to inhibit the S. aureus by quorum quenching as well. (Hardy et al., 2019) For example, Corynebacterium striatum has been reported to inhibit the production of toxins downstream of the quorum sensing mechanism, promote biofilm formation, and alter gene expression in S. aureus to make it behave more like a commensal bacteria than a pathogen (Ramsey et al., 2016). In summary, some coagulase-negative staphylococcus (CoNS) and Corynebacterium species help eliminate pathogenic bacteria by producing antimicrobial substances or through quorum quenching. In healthy skin, CoNS strains with such ability have been reported to be predominant, and the pathogenicity of S. aureus is more likely to demonstrate when these CoNS strains are absent or reduced (Nakatsuji et al., 2017; Williams et al., 2019). Thus, commensal bacteria cross-talk and form a microflora by interacting with each other through QS system and other signals.
Agr quorum sensing is a potential therapeutic target for AD and Staphycoccus aureus skin infection
Based on previous research, it has been established that the Agr-QS in S. aureus is crucial for the bacterial colonization of the skin and the exacerbation of AD. Various approaches have been attempted to target S. aureus for the treatment of AD, including the use of antibiotics and disinfectants to eliminate S. aureus. In industrialized countries, including the United States, sodium hypochlorite bleach baths have been used for the treatment of AD to effectively eradicate S. aureus (Chopra et al., 2017). However, some reports have indicated that bleach baths do not significantly affect the commensal microbiota of the skin or S. aureus colonization (Gonzalez et al., 2016; Sawada et al., 2019). Moreover, although attempts have been made to eliminate S. aureus using antibiotics, such methods are ineffective and increase the risk of further dysbiosis and antimicrobial-resistant bacteria (Bath-Hextall et al., 2010).
Therefore, Agr-QS in S. aureus, rather than in the bacteria, has attracted the attention of researchers as a potential therapeutic target. Our group has also reported that Agr-QS inhibitors suppress the virulence of S. aureus and reduce skin inflammation in a mouse model of inflammatory skin disease variants (Baldry et al., 2018; Nakagawa et al., 2020). There have been numerous attempts to target AgrA and AgrC and other targets for quorum quenching, but rare in vivo experiments have shown efficacy in animal infection models, and the fact that it was found to be counterproductive biofilm-increasing effects makes it uncertain whether it can actually be used for treatment (Otto, 2023). There are also emerging approaches to using bacteria competing against S. aureus for bacteriotherapy. It has been reported that skin commensal CoNS can prevent skin infections of pathogenic S. aureus by exploiting its different AIP sequences (Paharik et al., 2017). Analysis of samples from patients has demonstrated that AIPs derived from CoNS, specifically S. epidermidis, inhibited the Agr-QS system of S. aureus and potentially ameliorated skin symptoms (Williams et al., 2019). A novel therapeutic approach utilizing competing bacteria to suppress the proliferation of S. aureus and Agr-QS is currently under development. In a phase I clinical trial, the efficacy of the ShA9 strain of Staphylococcus hominis was demonstrated by its application to the lesional skin of patients with AD for 1 week, which resulted in the suppression of S. aureus, correction of dysbiosis, and a certain degree of improvement in local eczema (Nakatsuji et al., 2021b). ShA9 also produces a lantibiotic that contributes to the suppression of S. aureus, although even the lantibiotic deletion mutant showed an effect by quorum quenching (Nakatsuji et al., 2021b). Additionally, in a small clinical trial, CoNS were collected from patients with AD and reintroduced into the lesion area of the same patient through autologous bacteriotherapy. This approach competitively inhibits and eradicates pathogenic S. aureus, leading to significant improvement in cutaneous symptoms (Nakatsuji et al., 2021a). Based on these findings, using commensal bacteria and compounds that effectively inhibit S. aureus Agr-QS shows great potential as a strategy for ameliorating AD symptoms.
Conclusions
As discussed above, the Agr-QS is widely recognized as necessary for S. aureus colonization of the skin of patients with AD. The toxins produced by the Agr-QS can exacerbate the disease. However, whether atopic predisposition or bacterial colonization is the primary cause of AD development remains debatable. Further analysis is required to understand the mechanism that primarily controls the colonization and elimination of S. aureus from the skin. Targeting the mechanism of S. aureus Agr-QS in patients with AD may prevent the development and exacerbation of AD. This approach is completely different from conventional treatments for AD and is significant because it has the potential to prevent the development of AD and can lead to breakthroughs in the treatment of other S. aureus infections and, by extension, infections caused by other bacterial species.
Author contributions
All authors listed have made a substantial, direct, and intellectual contribution to the work and approved it for publication.
Funding
This work was supported by grants from the Japan Society for the Promotion of Science, JSPS KAKENHI 20H03701 (YN), JSPS KAKENHI Grant Number 16H06279 (PAGS) (YN), JSPS KAKENHI 22K16278 (YY), Advanced Research and Development Programs for Medical Innovation, AMED-PRIME JP 18gm6010016h0002 (YN), AMED-CREST 21gm1610004s0201 (YN) and The Leo Foundation (YN).
Acknowledgments
We would like to appreciate kindly feedback offered by Bernard C. Lo, and also thank Editage [http://www.editage.com] for editing and reviewing this manuscript for English language.
Conflict of interest
The authors declare that the research was conducted in the absence of any commercial or financial relationships that could be construed as a potential conflict of interest.
Publisher’s note
All claims expressed in this article are solely those of the authors and do not necessarily represent those of their affiliated organizations, or those of the publisher, the editors and the reviewers. Any product that may be evaluated in this article, or claim that may be made by its manufacturer, is not guaranteed or endorsed by the publisher.
References
Acker, K. P., Wong Fok Lung, T., West, E., Craft, J., Narechania, A., Smith, H., et al. (2019). Strains of staphylococcus aureus that colonize and infect skin harbor mutations in metabolic genes. iScience 19, 281–290. doi: 10.1016/j.isci.2019.07.037
Baldry, M., Nakamura, Y., Nakagawa, S., Frees, D., Matsue, H., Núñez, G., et al. (2018). Application of an agr-specific antivirulence compound as therapy for staphylococcus aureus-induced inflammatory skin disease. J. Infect. Dis. 218 (6), 1009–1013. doi: 10.1093/infdis/jiy259
Bath-Hextall, F. J., Birnie, A. J., Ravenscroft, J. C., Williams, H. C. (2010). Interventions to reduce staphylococcus aureus in the management of atopic eczema: an updated cochrane review. Br. J. Dermatol. 163 (1), 12–26. doi: 10.1111/j.1365-2133.2010.09743.x
Belkaid, Y., Segre, J. A. (2014). Dialogue between skin microbiota and immunity. Science 346 (6212), 954–959. doi: 10.1126/science.1260144
Belkaid, Y., Von Stebut, E., Mendez, S., Lira, R., Caler, E., Bertholet, S., et al. (2002). CD8+ T cells are required for primary immunity in C57BL/6 mice following low-dose, intradermal challenge with leishmania major. J. Immunol. 168 (8), 3992–4000. doi: 10.4049/jimmunol.168.8.3992
Bin, L., Kim, B. E., Brauweiler, A., Goleva, E., Streib, J., Ji, Y., et al. (2012). Staphylococcus aureus α-toxin modulates skin host response to viral infection. J. Allergy Clin. Immunol. 130 (3), 683–691.e682. doi: 10.1016/j.jaci.2012.06.019
Boles, B. R., Horswill, A. R. (2008). Agr-mediated dispersal of staphylococcus aureus biofilms. PloS Pathog. 4 (4), e1000052. doi: 10.1371/journal.ppat.1000052
Byrd, A. L., Belkaid, Y., Segre, J. A. (2018). The human skin microbiome. Nat. Rev. Microbiol. 16 (3), 143–155. doi: 10.1038/nrmicro.2017.157
Byrd, A. L., Deming, C., Cassidy, S. K. B., Harrison, O. J., Ng, W. I., Conlan, S., et al. (2017). Staphylococcus aureus and staphylococcus epidermidis strain diversity underlying pediatric atopic dermatitis. Sci. Transl. Med. 9 (397). doi: 10.1126/scitranslmed.aal4651
Cani, P. D., Jordan, B. F. (2018). Gut microbiota-mediated inflammation in obesity: a link with gastrointestinal cancer. Nat. Rev. Gastroenterol. Hepatol. 15 (11), 671–682. doi: 10.1038/s41575-018-0025-6
Cau, L., Williams, M. R., Butcher, A. M., Nakatsuji, T., Kavanaugh, J. S., Cheng, J. Y., et al. (2021). Staphylococcus epidermidis protease EcpA can be a deleterious component of the skin microbiome in atopic dermatitis. J. Allergy Clin. Immunol. 147 (3), 955–966.e916. doi: 10.1016/j.jaci.2020.06.024
Chang, Y. S., Chiang, B. L. (2018). Sleep disorders and atopic dermatitis: a 2-way street? J. Allergy Clin. Immunol. 142 (4), 1033–1040. doi: 10.1016/j.jaci.2018.08.005
Chen, Y. E., Fischbach, M. A., Belkaid, Y. (2018). Skin microbiota-host interactions. Nature 553 (7689), 427–436. doi: 10.1038/nature25177
Chopra, R., Vakharia, P. P., Sacotte, R., Silverberg, J. I. (2017). Efficacy of bleach baths in reducing severity of atopic dermatitis: a systematic review and meta-analysis. Ann. Allergy Asthma Immunol. 119 (5), 435–440. doi: 10.1016/j.anai.2017.08.289
Chung, Y., Chang, S. H., Martinez, G. J., Yang, X. O., Nurieva, R., Kang, H. S., et al. (2009). Critical regulation of early Th17 cell differentiation by interleukin-1 signaling. Immunity 30 (4), 576–587. doi: 10.1016/j.immuni.2009.02.007
Cogen, A. L., Yamasaki, K., Sanchez, K. M., Dorschner, R. A., Lai, Y., MacLeod, D. T., et al. (2010). Selective antimicrobial action is provided by phenol-soluble modulins derived from staphylococcus epidermidis, a normal resident of the skin. J. Invest. Dermatol. 130 (1), 192–200. doi: 10.1038/jid.2009.243
Esaki, H., Brunner, P. M., Renert-Yuval, Y., Czarnowicki, T., Huynh, T., Tran, G., et al. (2016). Early-onset pediatric atopic dermatitis is T(H)2 but also T(H)17 polarized in skin. J. Allergy Clin. Immunol. 138 (6), 1639–1651. doi: 10.1016/j.jaci.2016.07.013
Flohr, C., Pascoe, D., Williams, H. C. (2005). Atopic dermatitis and the ‘hygiene hypothesis’: too clean to be true? Br. J. Dermatol. 152 (2), 202–216. doi: 10.1111/j.1365-2133.2004.06436.x
Gallo, R. L. (2017). Human skin is the largest epithelial surface for interaction with microbes. J. Invest. Dermatol. 137 (6), 1213–1214. doi: 10.1016/j.jid.2016.11.045
Gallo, R. L., Hooper, L. V. (2012). Epithelial antimicrobial defence of the skin and intestine. Nat. Rev. Immunol. 12 (7), 503–516. doi: 10.1038/nri3228
Gittler, J. K., Shemer, A., Suárez-Fariñas, M., Fuentes-Duculan, J., Gulewicz, K. J., Wang, C. Q., et al. (2012). Progressive activation of T(H)2/T(H)22 cytokines and selective epidermal proteins characterizes acute and chronic atopic dermatitis. J. Allergy Clin. Immunol. 130 (6), 1344–1354. doi: 10.1016/j.jaci.2012.07.012
Gonzalez, M. E., Schaffer, J. V., Orlow, S. J., Gao, Z., Li, H., Alekseyenko, A. V., et al. (2016). Cutaneous microbiome effects of fluticasone propionate cream and adjunctive bleach baths in childhood atopic dermatitis. J. Am. Acad. Dermatol. 75 (3), 481–493.e488. doi: 10.1016/j.jaad.2016.04.066
Hardy, B. L., Dickey, S. W., Plaut, R. D., Riggins, D. P., Stibitz, S., Otto, M., et al. (2019). Corynebacterium pseudodiphtheriticum exploits staphylococcus aureus virulence components in a novel polymicrobial defense strategy. mBio 10 (1). doi: 10.1128/mBio.02491-18
Hong, S. W., Choi, E. B., Min, T. K., Kim, J. H., Kim, M. H., Jeon, S. G., et al. (2014). An important role of α-hemolysin in extracellular vesicles on the development of atopic dermatitis induced by staphylococcus aureus. PloS One 9 (7), e100499. doi: 10.1371/journal.pone.0100499
Iwase, T., Uehara, Y., Shinji, H., Tajima, A., Seo, H., Takada, K., et al. (2010). Staphylococcus epidermidis esp inhibits staphylococcus aureus biofilm formation and nasal colonization. Nature 465 (7296), 346–349. doi: 10.1038/nature09074
Ji, G., Beavis, R., Novick, R. P. (1997). Bacterial interference caused by autoinducing peptide variants. Science 276 (5321), 2027–2030. doi: 10.1126/science.276.5321.2027
Kim, B. E., Leung, D. Y., Boguniewicz, M., Howell, M. D. (2008). Loricrin and involucrin expression is down-regulated by Th2 cytokines through STAT-6. Clin. Immunol. 126 (3), 332–337. doi: 10.1016/j.clim.2007.11.006
Kong, H. H., Oh, J., Deming, C., Conlan, S., Grice, E. A., Beatson, M. A., et al. (2012). Temporal shifts in the skin microbiome associated with disease flares and treatment in children with atopic dermatitis. Genome Res. 22 (5), 850–859. doi: 10.1101/gr.131029.111
Kozman, A., Yao, Y., Bina, P., Saha, C., Yao, W., Kaplan, M. H., et al. (2010). Encoding a superantigen by staphylococcus aureus does not affect clinical characteristics of infected atopic dermatitis lesions. Br. J. Dermatol. 163 (6), 1308–1311. doi: 10.1111/j.1365-2133.2010.09966.x
Langan, S. M., Irvine, A. D., Weidinger, S. (2020). Atopic dermatitis. Lancet 396 (10247), 345–360. doi: 10.1016/s0140-6736(20)31286-1
Le, K. Y., Otto, M. (2015). Quorum-sensing regulation in staphylococci-an overview. Front. Microbiol. 6. doi: 10.3389/fmicb.2015.01174
Leyden, J. J., Marples, R. R., Kligman, A. M. (1974). Staphylococcus aureus in the lesions of atopic dermatitis. Br. J. Dermatol. 90 (5), 525–530. doi: 10.1111/j.1365-2133.1974.tb06447.x
Malachowa, N., Kobayashi, S. D., Braughton, K. R., DeLeo, F. R. (2013). Mouse model of staphylococcus aureus skin infection. Methods Mol. Biol. 1031, 109–116. doi: 10.1007/978-1-62703-481-4_14
Matsui, K., Nishikawa, A. (2012). Peptidoglycan from staphylococcus aureus induces T(H)2 immune response in mice. J. Investig. Allergol Clin. Immunol. 22 (2), 80–86.
Miller, M. B., Bassler, B. L. (2001). Quorum sensing in bacteria. Annu. Rev. Microbiol. 55, 165–199. doi: 10.1146/annurev.micro.55.1.165
Mueller, N. T., Bakacs, E., Combellick, J., Grigoryan, Z., Dominguez-Bello, M. G. (2015). The infant microbiome development: mom matters. Trends Mol. Med. 21 (2), 109–117. doi: 10.1016/j.molmed.2014.12.002
Nagy, I., Pivarcsi, A., Kis, K., Koreck, A., Bodai, L., McDowell, A., et al. (2006). Propionibacterium acnes and lipopolysaccharide induce the expression of antimicrobial peptides and proinflammatory cytokines/chemokines in human sebocytes. Microbes Infect. 8 (8), 2195–2205. doi: 10.1016/j.micinf.2006.04.001
Naik, S., Bouladoux, N., Linehan, J. L., Han, S. J., Harrison, O. J., Wilhelm, C., et al. (2015). Commensal-dendritic-cell interaction specifies a unique protective skin immune signature. Nature 520 (7545), 104–108. doi: 10.1038/nature14052
Nakagawa, S., Hillebrand, G. G., Nunez, G. (2020). Rosmarinus officinalis l. (Rosemary) extracts containing carnosic acid and carnosol are potent quorum sensing inhibitors of staphylococcus aureus virulence. Antibiotics 9 (4). doi: 10.3390/antibiotics9040149
Nakagawa, S., Matsumoto, M., Katayama, Y., Oguma, R., Wakabayashi, S., Nygaard, T., et al. (2017). Staphylococcus aureus virulent PSMα peptides induce keratinocyte alarmin release to orchestrate IL-17-Dependent skin inflammation. Cell Host Microbe 22 (5), 667–677.e665. doi: 10.1016/j.chom.2017.10.008
Nakamura, Y., Oscherwitz, J., Cease, K. B., Chan, S. M., Muñoz-Planillo, R., Hasegawa, M., et al. (2013). Staphylococcus δ-toxin induces allergic skin disease by activating mast cells. Nature 503 (7476), 397–401. doi: 10.1038/nature12655
Nakamura, Y., Takahashi, H., Takaya, A., Inoue, Y., Katayama, Y., Kusuya, Y., et al. (2020). Staphylococcus agr virulence is critical for epidermal colonization and associates with atopic dermatitis development. Sci. Transl. Med. 12 (551). doi: 10.1126/scitranslmed.aay4068
Nakano, H., Tsutsui, H., Terada, M., Yasuda, K., Matsui, K., Yumikura-Futatsugi, S., et al. (2003). Persistent secretion of IL-18 in the skin contributes to IgE response in mice. Int. Immunol. 15 (5), 611–621. doi: 10.1093/intimm/dxg062
Nakatsuji, T., Chen, T. H., Narala, S., Chun, K. A., Two, A. M., Yun, T., et al. (2017). Antimicrobials from human skin commensal bacteria protect against staphylococcus aureus and are deficient in atopic dermatitis. Sci. Transl. Med. 9 (378). doi: 10.1126/scitranslmed.aah4680
Nakatsuji, T., Gallo, R. L., Shafiq, F., Tong, Y., Chun, K., Butcher, A. M., et al. (2021a). Use of autologous bacteriotherapy to treat staphylococcus aureus in patients with atopic dermatitis: a randomized double-blind clinical trial. JAMA Dermatol. 157 (8), 978–982. doi: 10.1001/jamadermatol.2021.1311
Nakatsuji, T., Hata, T. R., Tong, Y., Cheng, J. Y., Shafiq, F., Butcher, A. M., et al. (2021b). Development of a human skin commensal microbe for bacteriotherapy of atopic dermatitis and use in a phase 1 randomized clinical trial. Nat. Med. 27 (4), 700–709. doi: 10.1038/s41591-021-01256-2
Oh, J., Conlan, S., Polley, E. C., Segre, J. A., Kong, H. H. (2012). Shifts in human skin and nares microbiota of healthy children and adults. Genome Med. 4 (10), 77. doi: 10.1186/gm378
Otto, M. (2014). Staphylococcus aureus toxins. Curr. Opin. Microbiol. 17, 32–37. doi: 10.1016/j.mib.2013.11.004
Otto, M. (2023). Critical assessment of the prospects of quorum-quenching therapy for staphylococcus aureus infection. Int. J. Mol. Sci. 24 (4). doi: 10.3390/ijms24044025
Otto, M., Echner, H., Voelter, W., Götz, F. (2001). Pheromone cross-inhibition between staphylococcus aureus and staphylococcus epidermidis. Infect. Immun. 69 (3), 1957–1960. doi: 10.1128/iai.69.3.1957-1960.2001
Paharik, A. E., Parlet, C. P., Chung, N., Todd, D. A., Rodriguez, E. I., Van Dyke, M. J., et al. (2017). Coagulase-negative staphylococcal strain prevents staphylococcus aureus colonization and skin infection by blocking quorum sensing. Cell Host Microbe 22 (6), 746–756.e745. doi: 10.1016/j.chom.2017.11.001
Paller, A. S., Kong, H. H., Seed, P., Naik, S., Scharschmidt, T. C., Gallo, R. L., et al. (2019). The microbiome in patients with atopic dermatitis. J. Allergy Clin. Immunol. 143 (1), 26–35. doi: 10.1016/j.jaci.2018.11.015
Piewngam, P., Zheng, Y., Nguyen, T. H., Dickey, S. W., Joo, H. S., Villaruz, A. E., et al. (2018). Pathogen elimination by probiotic bacillus via signalling interference. Nature 562 (7728), 532–537. doi: 10.1038/s41586-018-0616-y
Ramsey, M. M., Freire, M. O., Gabrilska, R. A., Rumbaugh, K. P., Lemon, K. P. (2016). Staphylococcus aureus shifts toward commensalism in response to corynebacterium species. Front. Microbiol. 7. doi: 10.3389/fmicb.2016.01230
Rojo, A., Aguinaga, A., Monecke, S., Yuste, J. R., Gastaminza, G., España, A. (2014). Staphylococcus aureus genomic pattern and atopic dermatitis: may factors other than superantigens be involved? Eur. J. Clin. Microbiol. Infect. Dis. 33 (4), 651–658. doi: 10.1007/s10096-013-2000-z
Sanchez Rodriguez, R., Pauli, M. L., Neuhaus, I. M., Yu, S. S., Arron, S. T., Harris, H. W., et al. (2014). Memory regulatory T cells reside in human skin. J. Clin. Invest. 124 (3), 1027–1036. doi: 10.1172/jci72932
Sanford, J. A., Gallo, R. L. (2013). Functions of the skin microbiota in health and disease. Semin. Immunol. 25 (5), 370–377. doi: 10.1016/j.smim.2013.09.005
Sawada, Y., Tong, Y., Barangi, M., Hata, T., Williams, M. R., Nakatsuji, T., et al. (2019). Dilute bleach baths used for treatment of atopic dermatitis are not antimicrobial in vitro. J. Allergy Clin. Immunol. 143 (5), 1946–1948. doi: 10.1016/j.jaci.2019.01.009
Scharschmidt, T. C., Vasquez, K. S., Truong, H. A., Gearty, S. V., Pauli, M. L., Nosbaum, A., et al. (2015). A wave of regulatory T cells into neonatal skin mediates tolerance to commensal microbes. Immunity 43 (5), 1011–1021. doi: 10.1016/j.immuni.2015.10.016
Schilcher, K., Horswill, A. R. (2020). Staphylococcal biofilm development: structure, regulation, and treatment strategies. Microbiol. Mol. Biol. Rev. 84 (3). doi: 10.1128/mmbr.00026-19
Selwyn, S. (1963). Bacterial infections in a skin department. Br. J. Dermatol. 75, 26–28. doi: 10.1111/j.1365-2133.1963.tb13529.x
Severn, M. M., Williams, M. R., Shahbandi, A., Bunch, Z. L., Lyon, L. M., Nguyen, A., et al. (2022). The ubiquitous human skin commensal staphylococcus hominis protects against opportunistic pathogens. mBio 13 (3), e0093022. doi: 10.1128/mbio.00930-22
Si, J., Lee, S., Park, J. M., Sung, J., Ko, G. (2015). Genetic associations and shared environmental effects on the skin microbiome of Korean twins. BMC Genomics 16, 992. doi: 10.1186/s12864-015-2131-y
Sonkoly, E., Muller, A., Lauerma, A. I., Pivarcsi, A., Soto, H., Kemeny, L., et al. (2006). IL-31: a new link between T cells and pruritus in atopic skin inflammation. J. Allergy Clin. Immunol. 117 (2), 411–417. doi: 10.1016/j.jaci.2005.10.033
Soong, G., Paulino, F., Wachtel, S., Parker, D., Wickersham, M., Zhang, D., et al. (2015). Methicillin-resistant staphylococcus aureus adaptation to human keratinocytes. mBio 6 (2). doi: 10.1128/mBio.00289-15
Spergel, J. M., Mizoguchi, E., Brewer, J. P., Martin, T. R., Bhan, A. K., Geha, R. S. (1998). Epicutaneous sensitization with protein antigen induces localized allergic dermatitis and hyperresponsiveness to methacholine after single exposure to aerosolized antigen in mice. J. Clin. Invest. 101 (8), 1614–1622. doi: 10.1172/jci1647
Spika, J. S., Verbrugh, H. A., Verhoef, J. (1981). Protein a effect on alternative pathway complement activation and opsonization of staphylococcus aureus. Infect. Immun. 34 (2), 455–460. doi: 10.1128/iai.34.2.455-460.1981
Stock, M. R., Fang, L., Johnson, K. R., Cosgriff, C., Teoh, W. P., Alonzo, F., 3rd (2022). Characterization of MroQ-dependent maturation and export of the staphylococcus aureus accessory gene regulatory system autoinducing peptide. Infect. Immun. 90 (10), e0026322. doi: 10.1128/iai.00263-22
Tauber, M., Balica, S., Hsu, C. Y., Jean-Decoster, C., Lauze, C., Redoules, D., et al. (2016). Staphylococcus aureus density on lesional and nonlesional skin is strongly associated with disease severity in atopic dermatitis. J. Allergy Clin. Immunol. 137 (4), 1272–1274.e1273. doi: 10.1016/j.jaci.2015.07.052
Tay, A. S. L., Li, C., Nandi, T., Chng, K. R., Andiappan, A. K., Mettu, V. S., et al. (2021). Atopic dermatitis microbiomes stratify into ecologic dermotypes enabling microbial virulence and disease severity. J. Allergy Clin. Immunol. 147 (4), 1329–1340. doi: 10.1016/j.jaci.2020.09.031
Totté, J. E., van der Feltz, W. T., Hennekam, M., van Belkum, A., van Zuuren, E. J., Pasmans, S. G. (2016). Prevalence and odds of staphylococcus aureus carriage in atopic dermatitis: a systematic review and meta-analysis. Br. J. Dermatol. 175 (4), 687–695. doi: 10.1111/bjd.14566
Travers, J. B., Kozman, A., Mousdicas, N., Saha, C., Landis, M., Al-Hassani, M., et al. (2010). Infected atopic dermatitis lesions contain pharmacologic amounts of lipoteichoic acid. J. Allergy Clin. Immunol. 125 (1), 146–152.e141-142. doi: 10.1016/j.jaci.2009.09.052
Travers, J. B., Leung, D. Y., Johnson, C., Schlievert, P., Marques, M., Cosgrove, J., et al. (2003). Augmentation of staphylococcal alpha-toxin signaling by the epidermal platelet-activating factor receptor. J. Invest. Dermatol. 120 (5), 789–794. doi: 10.1046/j.1523-1747.2003.12149.x
Tsoi, L. C., Rodriguez, E., Stölzl, D., Wehkamp, U., Sun, J., Gerdes, S., et al. (2020). Progression of acute-to-chronic atopic dermatitis is associated with quantitative rather than qualitative changes in cytokine responses. J. Allergy Clin. Immunol. 145 (5), 1406–1415. doi: 10.1016/j.jaci.2019.11.047
Wang, R., Braughton, K. R., Kretschmer, D., Bach, T. H., Queck, S. Y., Li, M., et al. (2007). Identification of novel cytolytic peptides as key virulence determinants for community-associated MRSA. Nat. Med. 13 (12), 1510–1514. doi: 10.1038/nm1656
Werfel, T., Allam, J. P., Biedermann, T., Eyerich, K., Gilles, S., Guttman-Yassky, E., et al. (2016). Cellular and molecular immunologic mechanisms in patients with atopic dermatitis. J. Allergy Clin. Immunol. 138 (2), 336–349. doi: 10.1016/j.jaci.2016.06.010
Werfel, T., Morita, A., Grewe, M., Renz, H., Wahn, U., Krutmann, J., et al. (1996). Allergen specificity of skin-infiltrating T cells is not restricted to a type-2 cytokine pattern in chronic skin lesions of atopic dermatitis. J. Invest. Dermatol. 107 (6), 871–876. doi: 10.1111/1523-1747.ep12331164
White, M. I., Noble, W. C. (1986). Consequences of colonization and infection by staphylococcus aureus in atopic dermatitis. Clin. Exp. Dermatol. 11 (1), 34–40. doi: 10.1111/j.1365-2230.1986.tb00421.x
Williams, M. R., Costa, S. K., Zaramela, L. S., Khalil, S., Todd, D. A., Winter, H. L., et al. (2019). Quorum sensing between bacterial species on the skin protects against epidermal injury in atopic dermatitis. Sci. Transl. Med. 11 (490). doi: 10.1126/scitranslmed.aat8329
Yoshioka, T., Hikita, I., Matsutani, T., Yoshida, R., Asakawa, M., Toyosaki-Maeda, T., et al. (2003). DS-Nh as an experimental model of atopic dermatitis induced by staphylococcus aureus producing staphylococcal enterotoxin c. Immunology 108 (4), 562–569. doi: 10.1046/j.1365-2567.2003.01588.x
Zhao, A., Bodine, S. P., Xie, Q., Wang, B., Ram, G., Novick, R. P., et al. (2022). Reconstitution of the s. aureus agr quorum sensing pathway reveals a direct role for the integral membrane protease MroQ in pheromone biosynthesis. Proc. Natl. Acad. Sci. U.S.A. 119 (33), e2202661119. doi: 10.1073/pnas.2202661119
Keywords: atopic dermatits, Staphycoccus aureus, Agr, quorum sensing, Th2, IL-17, dysbiosis
Citation: Tamai M, Yamazaki Y, Ito T, Nakagawa S and Nakamura Y (2023) Pathogenic role of the staphylococcal accessory gene regulator quorum sensing system in atopic dermatitis. Front. Cell. Infect. Microbiol. 13:1178650. doi: 10.3389/fcimb.2023.1178650
Received: 03 March 2023; Accepted: 03 April 2023;
Published: 14 April 2023.
Edited by:
Seth Dickey, University of Maryland, College Park, United StatesReviewed by:
Marc Burian, University Hospital RWTH Aachen, GermanyBernhard Krismer, University of Tübingen, Germany
Copyright © 2023 Tamai, Yamazaki, Ito, Nakagawa and Nakamura. This is an open-access article distributed under the terms of the Creative Commons Attribution License (CC BY). The use, distribution or reproduction in other forums is permitted, provided the original author(s) and the copyright owner(s) are credited and that the original publication in this journal is cited, in accordance with accepted academic practice. No use, distribution or reproduction is permitted which does not comply with these terms.
*Correspondence: Yuumi Nakamura, eW1hdHN1b2thQGRlcm1hLm1lZC5vc2FrYS11LmFjLmpw; Yuriko Yamazaki, eS55YW1hemFraUBkZXJtYS5tZWQub3Nha2EtdS5hYy5qcA==