- 1Department of Neonatology, Women’s Hospital of Jiangnan University, Wuxi Maternity and Child Health Care Hospital, Wuxi, China
- 2Wuxi Children’s Hospital, Children’s Hospital of Jiangnan University, Wuxi, China
- 3Department of Pediatric, Jiangyin People’s Hospital of Nantong University, Wuxi, China
- 4School of Medicine, Nantong University, Nantong, China
- 5College of Resources and Environment, Innovative Institute for Plant Health, Zhongkai University of Agriculture and Engineering, Guangzhou, China
- 6Research Institute for Reproductive Health and Genetic Diseases, Women’s Hospital of Jiangnan University, Wuxi Maternity and Child Health Care Hospital, Wuxi, China
Objectives: Digestive system diseases have evolved into a growing global burden without sufficient therapeutic measures. Lactobacillus reuteri (L. reuteri) is considered as a new potential economical therapy for its probiotic effects in the gastrointestinal system. We have provided an overview of the researches supporting various L. reuteri strains’ application in treating common digestive system diseases, including infantile colic, diarrhea, constipation, functional abdominal pain, Helicobacter pylori infection, inflammatory bowel disease, diverticulitis, colorectal cancer and liver diseases.
Methods: The summarized literature in this review was derived from databases including PubMed, Web of Science, and Google Scholar.
Results: The therapeutic effects of L. reuteri in digestive system diseases may depend on various direct and indirect mechanisms, including metabolite production as well as modulation of the intestinal microbiome, preservation of the gut barrier function, and regulation of the host immune system. These actions are largely strain-specific and depend on the activation or inhibition of various certain signal pathways. It is well evidenced that L. reuteri can be effective both as a prophylactic measure and as a preferred therapy for infantile colic, and it can also be recommended as an adjuvant strategy to diarrhea, constipation, Helicobacter pylori infection in therapeutic settings. While preclinical studies have shown the probiotic potential of L. reuteri in the management of functional abdominal pain, inflammatory bowel disease, diverticulitis, colorectal cancer and liver diseases, its application in these disease settings still needs further study.
Conclusion: This review focuses on the probiotic effects of L. reuteri on gut homeostasis via certain signaling pathways, and emphasizes the importance of these probiotics as a prospective treatment against several digestive system diseases.
1 Introduction
Digestive system diseases arise rapidly through the interaction of genetic and environmental characteristics and are considered to cause considerable healthcare burdens and costs (Peery et al., 2022; Ricciardiello, 2022). Based on the latest data, digestive system diseases are responsible for millions of healthcare encounters and thousands and thousands of deaths that cost billions of dollars in the United States every year (Peery et al., 2022). Of these digestive system diseases, inflammatory bowel disease (IBD) is estimated to affect 790 per 100,000 people in 2025 (Kaplan, 2015). According to the most recent worldwide estimates, there were 930,000 deaths of colorectal cancer (CRC) in 2020 and over 1.9 million new cases (Morgan et al., 2023). The similar phenomenon of increasing incidence is also seen in infantile colic (IC), functional abdominal pain (FAP), and other gastrointestinal (GI) diseases (Thapar et al., 2020; Peery et al., 2022; Indrio et al., 2023). Given this situation, there is an urgent need to deploy personalized treatments for these diseases.
Nowadays public interest of probiotics applications in therapeutic settings is growing. One of the most widely used probiotics, Lactobacillus, can be detected in the GI tract of animals in varying concentrations depending on the species, age of the host, or placement inside the gut (Zhao et al., 2023). The Lactobacillus is a large heterogeneous set of facultative anaerobic bacteria that are nonsporulating, and Gram-positive comprising Lactobacillus (L.) acidophilus, L. reuteri, L. casei, L. bulgaricus, L. rhamnosus, and so on (Mu et al., 2018). Among these, L. reuteri is an extensively investigated probiotic that was first isolated in 1962, resides in tissues of numerous mammals, and provides multiple health benefits for the host including producing antimicrobial molecules and regulating the host immune system (Yu et al., 2023b). L. reuteri was discovered in various body sites, which included breast milk, skin, urinary tract, and GI tract (Yu et al., 2023b). Numerous L. reuteri strains were found to play unique roles in different diseases covering hypercholesterolemia, skin infection, allergic asthma, periodontitis, and autism spectrum disorders as essential probiotics, especially in GI diseases (Sgritta et al., 2019; Kubota et al., 2020; Dargenio et al., 2021). Mounting evidence indicates that the symbiotic gut microbiota and host immune system work together to preserve gut homeostasis (Saeed et al., 2022). Growing data has revealed that the etiology of disorders of the digestive system is strongly influenced by disrupted gut microbes (Quaglio et al., 2022). Various L. reuteri strains have been investigated in digestive system diseases like IC, diarrhea, constipation, FAP, IBD, CRC, as well as liver diseases, and the findings are generally promising (Dias et al., 2021; Saviano et al., 2021; Werlinger et al., 2022; Happy Tummy et al., 2023).
But there are still doubts about clinical application of L. reuteri, so it is important to understand the underlying mechanism of L. reuteri in gut health. The effects of L. reuteri on GI diseases may include preservation of gut barrier function, suppression of excessive immune responses as well as oxidative stress, modification of the composition of the intestinal flora, and production of metabolites. In this review, we discussed the application of L. reuteri in digestive system diseases and outlined the potential mechanisms.
2 Clinical application of L. reuteri in digestive system diseases
2.1 Infantile colic
IC is typically characterized by daily full-force crying for a minimum of 3 hrs, on a minimum of 3 days per week, for at least 3 weeks (Gordon et al., 2018). It is reported about one in four infants younger than three months develop colic (Indrio et al., 2023). Although IC often resolves by three months of age, it remains to be stressful for parents and may even cause maternal postpartum depressive symptoms (Mercuri et al., 2023). Considerable research indicates that the intestinal microbiome’s composition is related to infant colic symptoms, even though the pathophysiology of this digestive condition is still inadequately comprehended (Korpela et al., 2020). Firstly, it has been hypothesized that the developing GI microbiome and the occurrence of excessive crying in infants between the ages of two weeks and three months-the normal time frame during which the gut is typically colonized by bacteria are related (Zeevenhooven et al., 2018). Additionally, the gut microbiota interacts with the gut-brain axis through multiple signaling pathways, including neural, endocrine, immune, and humoral signaling pathways (Deng et al., 2021). Given this, it may influence central and gut neural functions, such as pain perception in infants via the gut-brain axis, which may be associated with excessive crying (Socala et al., 2021). In the past decades, numerous studies illustrated that the GI microbial profiles of infants with colic differ from those without colic in microbial diversity, stability, and community composition (de Weerth et al., 2013; Savino et al., 2017). Moreover, the results showed colicky infants were less frequently colonized by Lactobacillus, Bifidobacterium and more frequently enriched with the gas-forming Coliforms (mostly Escherichia, Klebsiella) or other species (Savino et al., 2009; de Weerth et al., 2013; Savino et al., 2017; Sommermeyer et al., 2022; Kozhakhmetov et al., 2023).
We concluded that L. reuteri may be useful in the improvement of breastfeeding babies with IC based on six randomized controlled trials (RCTs), shown in Table 1. Accordingly, administering 1×108 colony-forming units (CFU) of L. reuteri DSM 17938 per day for 21 to 30 days can significantly decrease daily crying and fussing times, and reduce the duration of crying episodes in colicky breast-fed infants, consistent with remission of maternal depression (Savino et al., 2010; Szajewska et al., 2013; Chau et al., 2015; Mi et al., 2015; Savino et al., 2018a; Savino et al., 2018b). Similar results were reported when analyzing the probiotic potential of L. reuteri ATCC 55730 in colicky infants, the superiority was still maintained compared with simethicone intervention (Savino et al., 2007). However, whether L. reuteri is useful in the treatment of formula-fed colicky infants is still controversial. Data shown in the other two RCT studies demonstrated that L. reuteri did not help manage the symptoms of colicky infants (Sung et al., 2014; Turco et al., 2021). One study showed that a partially hydrolyzed formula added with maltodextrins and L. reuteri DSM 17938 showed no preference for the standard formula on colicky symptoms, but the unsatisfactory results may attribute to the addition of maltodextrins in the probiotics group (Turco et al., 2021). In another study included breastfed and formula-fed colicky newborns, researchers found that L. reuteri DSM 17938 had no beneficial impact on crying or fusing time, instead, more fussing occurred in formula-fed infants following probiotic treatment (Sung et al., 2014). The conflict results may be explained by the differences in gut microbiota, whose composition in early infancy seemed to be influenced by various factors, including the delivery mode, infant feeding pattern, gestational age, and the history of antibiotic use (Kapourchali and Cresci, 2020). Formula-fed babies exhibited an increased gut bacterial community richness relative to breast-fed babies, with a greater abundance of Clostridium difficile (Azad et al., 2013). Following the administration of L. reuteri DSM 17938, breast-fed colicky infants showed a substantial rise in fecal Lactobacilli and a drop in fecal Escherichia (E.) coli (Savino et al., 2010; Savino et al., 2018b). From these findings, the therapeutic effectiveness of L. reuteri could be influenced by the microbiome composition of the infant’s GI tract, which would account for the differences between the effect on crying duration in breast-fed and formula-fed infants.
It is also reported that colicky infants showed increased serum concentrations of inflammatory interleukin (IL)-8, monocyte chemoattractant protein-1, and higher fecal calprotectin levels, indicating the existence of systemic and intestinal local inflammation (Partty et al., 2017). L. reuteri DSM 17938 treatment can increase circulating FoxP3 concentration and reduce fecal calprotectin, indicating the underlying mechanisms of L. reuteri to improve colic may be linked to the property of alleviating inflammation (Savino et al., 2018a; Savino et al., 2018b). According to a recent secondary analysis research based on a large observational study, L. reuteri-containing formula was superior to standard formula without probiotics in preventing infant colic, similar to breast feeding (Happy Tummy et al., 2023). Furthermore, the probiotic potential of L. reuteri DSM 17938 given at a dosage of 1×108 CFU per day for 90 days to prevent IC is demonstrated by two RCT studies in newborns (Indrio et al., 2014; Savino et al., 2015). This statement was also supported by one prospective cohort study, which showed maternal consumption with L. reuteri DSM 26866 in the latter four weeks of gestation was able to protect infants from colic (Pourmirzaiee et al., 2020). In conclusion, since L. reuteri may ameliorate colic symptoms mainly by altering the microbial colonization pattern in colicky infants, it is a receptive therapy for colicky infants without side effects and recommendable supplements for newborns to prevent IC.
2.2 Diarrhea
Recent data has shown that acute diarrheal disease leads to 179 million outpatient visits every year in the United States, associated with various pathogen infections such as Shigella, E. coli, rotavirus, norovirus and antibiotic use (Meisenheimer Es Md et al., 2022). Diarrhea is also identified as the leading infectious cause of childhood morbidity and mortality (Ahmed et al., 2021). Previously several specific probiotic strains have demonstrated antidiarrheal effects (Skrzydlo-Radomanska et al., 2020; Lukasik et al., 2022). An increasing number of studies have been conducted to clarify the function of L. reuteri in diarrhea since Shornikova and his colleagues first analyzed the effectiveness of L. reuteri DSM 17938 in pediatric acute watery diarrhea and demonstrated that it can dosage-dependently shorten the duration of acute watery diarrhea (Shornikova et al., 1997a; Shornikova et al., 1997b). Previous nine RCTs, which demonstrated that L. reuteri DSM 17938 was capable of reducing the frequency, length of time, and incidence of diarrhea in children and adults, especially in those with lower nutritional status, supported the drug’s potential benefits in treating and preventing diarrheal diseases (Agustina et al., 2012; Francavilla et al., 2012; Jones et al., 2013; Dinleyici et al., 2014; Gutierrez-Castrellon et al., 2014; Dinleyici et al., 2015; Pernica et al., 2017; Maragkoudaki et al., 2018; Kambale et al., 2023). Additionally, L. reuteri DSM 17938 could shorten a child’s stay in the hospital due to acute gastroenteritis or infectious diarrhea (Dinleyici et al., 2014; Szymanski and Szajewska, 2019). However, in the situation of nosocomial diarrhea, three RCTs produced contrary findings (Wanke and Szajewska, 2012; Urbanska et al., 2016; Kolodziej and Szajewska, 2019). The investigators found that L. reuteri DSM 17938 was ineffective in preventing diarrhea in inpatient children received antibiotics, regardless of the given dosage (1×108 CFU once daily, or 2×108 CFU twice daily, or 2×109 CFU once daily while in hospital) (Wanke and Szajewska, 2012; Urbanska et al., 2016; Kolodziej and Szajewska, 2019). While L. reuteri ATCC 55730 was reported to be able to prevent antibiotic-associated diarrhea in hospitalized adults in another study (Cimperman et al., 2011). Different from the prior studies, this clinical trial analyzed adult patients who were mainly treated with combined antibiotic therapy, and the subjects were given L. reuteri ATCC 55730 1×108 CFU twice daily for 28 days (Cimperman et al., 2011). Given these limited data, the function of L. reuteri in preventing antibiotic-associated diarrhea is ambiguous, which may be strain-specific and depend on the gut microbiota community disrupted by antibiotics. Of note, none of the mentioned clinical studies analyzed the fecal L. reuteri abundance before or after L. reuteri administration. All these RCT studies are depicted in Table 2.
Enterotoxigenic E. coli is a main pathogen of infectious diarrhea in childhood and postweaning piglets (Kotloff, 2022; Qin et al., 2023b). Gut microbiota disruption observed in diarrheal piglets was described as a reduction of Lactobacillus, enriched abundance of E. coli, and increased lipopolysaccharide (LPS) biosynthesis (Li et al., 2023b). Supplement of L. reuteri can reverse the enriched Pseudomonadota and the depleted Bacteroidota triggered by E. coli infection and reduce jejunum E. coli content, thus preserving intestinal disruption (Wang et al., 2018; Lu et al., 2023). L. reuteri stains including L. reuteri HCM2 and L. reuteri PSC102 have been reported to suppress the growth of E. coli in vitro and inhibit its adherence to intestinal epithelial cells (Wang et al., 2018; Ali et al., 2023). Moreover, L. reuteri was also reported to possess the ability to upregulate the expression of tight junction (TJ) proteins and E-cadherin, thereby maintaining intestinal permeability in infected mice (Karimi et al., 2018; Lu et al., 2023). In a rotavirus-infected murine model, L. reuteri can reduce diarrhea duration and alleviate inflammation, probably associated with accelerated intestinal epithelium turnover and restored gut microbiome diversity (Preidis et al., 2012). Particularly, the efficiency of L. reuteri to increase enterocyte proliferation and migration seemed to be influenced by host nutritional status (Preidis et al., 2012). Except these, the therapeutic application of L. reuteri in infectious diarrhea may also be related to its metabolite production such as reuterin, with the ability to inhibit an extensive range of germs (Collinson et al., 2020).
These results illustrated that the therapeutic efficiency of L. reuteri in diarrhea may depend on regulation of gut microbiota and barrier, which is strain-specific and reliant on age, pathogen, nutritional status, and the disease situation as well. To sum up, L. reuteri can be recommended as an adjuvant to rehydration treatment for diarrhea in therapeutic settings, but its function in prophylactic settings still requires more research, especially in the antibiotics-use setting.
2.3 Constipation
Constipation is an intestinal condition that could affect individuals of all ages and dramatically lower their quality of life (Saviano et al., 2021). With a prevalence spanning from 7% to 30%, constipation is also one of the most prevalent pediatric disorders (Wegner et al., 2018). The fundamental mechanism of constipation, which typically has a functional cause without an organic origin, is largely unknown (Mulhem et al., 2022). It is suggested that disruption of the intestinal flora play a role in functional constipation (FC) (Zhang et al., 2021). The results may be conflict reliant on measurements. Based on traditional microbiological culture tests, enriched Bifidobacteria and Lactobacillus were observed in children with FC, while decreased abundance of Bifidobacterium and Lactobacillus was seen in adult FC patients (Zoppi et al., 1998; Khalif et al., 2005). Nowadays though gene sequencing analysis, it seems consistent that Firmicutes relative abundance is increased while Bacteroidetes frequency is heterogeneous based on population, and the gut microbe pattern differs in different subtypes of constipation as well (Zhu et al., 2014; Mancabelli et al., 2017; Yu et al., 2023a).
A couple of RCTs have evaluated the effectiveness of L. reuteri DSM 17938 in treating FC, depicted in Table 2. The investigations demonstrated that L. reuteri DSM 17938 single-drug administration significantly alleviated constipation both in children and adults, involving improvement in defecation frequency, painful defecation, and reduced fecal mass in the rectum (Coccorullo et al., 2010; Ojetti et al., 2014; Riezzo et al., 2018; Garcia Contreras et al., 2020; Kubota et al., 2020). However, L. reuteri DSM 17938 did not show additional improvement in FC when regarded as adjuvant therapy for lactulose or macrogol (Jadresin et al., 2018; Wegner et al., 2018; Kubota et al., 2020). Only one study investigated the gut microbiome community alternations, in which the limited data revealed that L. reuteri treatment did not alter the gut microbiota composition of the pediatric subjects with FC (Kubota et al., 2020).
To note, current evidence showed that L. reuteri DSM 17938 improved bowel movements, but did not affect stool consistency (Coccorullo et al., 2010; Kubota et al., 2020). The defecation frequency was found to be negatively correlated with genus Oscillospira, Megasphaera, and Ruminococcus (Kubota et al., 2020). On one hand, L. reuteri DSM 17938 can improve intestinal motility and promote intestinal transit, partially via controlling some GI peptide pathways. According to research by Riezzo and colleagues, the therapeutic efficacy of L. reuteri DSM 17938 in FC may be associated with serum brain-derived neurotrophic factor and serotonin (5-HT) concentrations (Riezzo et al., 2019). Tryptophan metabolism may be impacted by L. reuteri, which would reduce the level of 5-HT in circulation (Montgomery et al., 2022). The connection between the intestinal flora and the enteric neural systems involves the 5-HT pathways, which have advantages for irritating the local enteric nerve responses and promoting motility (Ge et al., 2017; Agus et al., 2018). On the other hand, L. reuteri DSM 17938 is capable of producing short-chain fatty acids (SCFAs), the substances involved in boosting intestinal peristalsis and colonic myoelectric cell response, all of which are effective for treating chronic FC (Wu et al., 2013; Hurst et al., 2014; Calvigioni et al., 2023).
However, there is still no sufficient data in the field of L. reuteri application for constipation prevention. A prospective RCT study carried out by Indrio and colleagues revealed that oral supplementation with L. reuteri DSM 1793 in newborns effectively prevented constipation within the first 3 months after birth (Indrio et al., 2014). In conclusion, present findings indicate that L. reuteri can be recommended to be used in the management of FC as an alternative therapy for traditional drugs.
2.4 Functional abdominal pain
FAP, which is the most prevalent type of abdominal pain in children and teenagers, with a prevalence of about 14 percent, is described as chronic or recurring pain without an organic cause (Bao et al., 2023). Although most FAP is often self-managed, it can have adverse effects on life quality, suffered children’s after-school activities, and school attendance, and may interfere with regular family life (Thapar et al., 2020). The disease was currently considered a disorder of the “brain-gut axis”, and gut microbiome changes could be engaged in its pathogenesis, but the available data is insufficient to draw a conclusion (Zhou et al., 2022a). Given the limitations of medication in children, probiotics arise as a possibly pleasant therapeutic approach for the treatment of pediatric FAP (Trivic et al., 2021). Six RCT investigations on L. reuteri DSM 17938’s medicinal potential are summarized in Table 3. The studies demonstrated that administration of L. reuteri DSM 17938 for 4 to 12 weeks reduced the pain intensity, the frequency of episodes, and increased days without pain in irritable bowel syndrome or FAP-affected children without adverse events (Romano et al., 2014; Eftekhari et al., 2015; Weizman et al., 2016; Jadresin et al., 2017; Maragkoudaki et al., 2017; Jadresin et al., 2020). However, one study proposed that L. reuteri DSM 17938 was equally effective in relieving pain in both the placebo and L. reuteri groups of children with FAP (Eftekhari et al., 2015). The authors speculated that the result may be explained by psychological effects since L. reuteri DSM 17938 showed no superiority to placebo (Eftekhari et al., 2015). None of the studies explored gut flora profiles after L. reuteri treatment, so we cannot draw a conclusion about the precise mechanism of L. reuteri application in pediatric FAP.
By enhancing opioid receptor activity in the afflicted tissues, Hegde and his colleagues reported that the dorsal root ganglia neurons projecting to the dilated colon exhibited attenuated hyperexcitability after L. reuteri recolonization, thus preventing visceral hypersensitivity in lumen distension in rats (Hegde et al., 2020). Additionally, L. reuteri may exhibit the ability to reduce gut pain by reducing signaling from the pain receptor transient receptor potential vanilloid 1 channel in the intestine (Pang et al., 2022). Collectively, although L. reuteri could be an attractive choice in treating FAP-related diseases due to its safety feature, there is currently no powerful proof to recommend L. reuteri application in FAP and further long-run researches are still needed to explore the definite mechanism.
2.5 Helicobacter pylori infection
Helicobacter pylori (H. pylori) is the key pathogen of chronic active gastritis, peptic and duodenal ulcers, with the global prevalence of infection exceeding 50%, also considered a high-risk factor for gastric cancer (de Brito et al., 2019). Currently, the effectiveness of treatment has been greatly impaired by the fast global emergence of antibiotic resistance to H. pylori, and the quadruple approach for H. pylori elimination could further exacerbate GI disease (Chen et al., 2021). It is suggested that eradication of H. pylori may lead to disruption of gut microbiota, featured by reduced Actinobacteria as well as Bacteroidetes abundances and enriched Proteobacteria (Sitkin et al., 2022). Specific probiotics may be helpful in reducing side effects triggered by first-line antimicrobial therapy and enhancing the efficacy of H. pylori eradication therapy (Liang et al., 2022). In previous RCT studies, L. reuteri was shown to reduce H. pylori load but seemed to be unable to increase eradication rates of H. pylori as an adjuvant for first-line therapy (Imase et al., 2007; Francavilla et al., 2008; Mehling and Busjahn, 2013; Francavilla et al., 2014; Holz et al., 2015; Dore et al., 2019; Poonyam et al., 2019; Yang et al., 2021; Dore et al., 2022; Moreno Marquez et al., 2022; Naghibzadeh et al., 2022), shown in Table 4. Notably, several studies showed that L. reuteri can reduce abdominal pain, distension, and other eradication treatment-related adverse events, consistent with decreased GI symptom rating scale scores in H. pylori-positive subjects, thus governing patient compliance (Lionetti et al., 2006; Francavilla et al., 2008; Francavilla et al., 2014; Poonyam et al., 2019; Yang et al., 2021; Moreno Marquez et al., 2022). Two studies analyzed the alternations of the gut flora after combined therapy of this probiotics and eradication treatment, which revealed that L. reuteri can change the gut microbiota composition in H. pylori-positive patients but cannot fully counteract gut dysbiosis induced by antibiotics (Yang et al., 2021; Dore et al., 2022). It means that the benefits brought by L. reuteri supplement cannot be attributed to restored gut microbiota balance. Particularly, L. reuteri can survive the gastric acid environment and colonize the gastric mucosa (Dargenio et al., 2021). It is reported that L. reuteri can inhibit the early colonization stages of H. pylori in the human GI tract, associated with the suppression of the binding of H. pylori to putative glycolipid receptor molecules (Holz et al., 2015). Besides, L. reuteri produces reuterin, an antibiotic that targets H. pylori, thus reducing H. pylori load (Urrutia-Baca et al., 2018). Meanwhile, a most recent survey also revealed that L. reuteri 2892 attenuated H. pylori-induced gastritis by its anti-inflammatory and anti-oxidative stress characteristics as well as suppressing the gene expression of the virulence factor CagA (Forooghi Nia et al., 2023). Recent studies also focus on the relationship between L. reuteri and cancer diseases, it has been demonstrated that L. reuteri exerts an anti-tumor effect in gastric cancer MKN1 cells, but data is still limited about L. reuteri application in gastric cancers related to H. pylori infection (Kim et al., 2022a).
Overall, L. reuteri has shown superiority as a salvageable treatment for side effects brought by eradication therapy, yet we still need further studies to delve deeper into the research gap between L. reuteri application and H. pylori-associated cancer.
2.6 Inflammatory bowel disease and diverticulitis
Crohn’s disease and ulcerative colitis are categorized as chronic IBD, which have evolved into a worldwide burden with increasing incidence (Nishikawa et al., 2021). It is reported that about one in five children needing a colectomy during childhood while the intestinal damage and complications brought by Crohn’s disease are still a difficulty for disease management (Cheng et al., 2021; Gordon et al., 2022). Multiple factors, such as the interaction between genetic background and environment, especially including a potential association between impaired gut microbial homeostasis and inflammation, and gut barrier disruption as well, have been suggested to be engaged in IBD pathogenesis (Larabi et al., 2020). It was observed in IBD subjects that the relative abundance of Proteobacteria, especially E. coli, was increased while the content of Bacteroidetes and Firmicutes was depleted, along with the reduced gut microbiota diversity (Nishino et al., 2018; Vester-Andersen et al., 2019). It has been revealed in a previous clinical trial, whereby, rectal infusion of L. reuteri ATCC 55730 effectively improved inflamed mucous membranes and reduced mucosal expression levels of inflammatory markers in active distal ulcerative colitis in children (Oliva et al., 2012), shown in Table 5.
According to Liu and his colleagues, peroral treatment with L. reuteri ATCC PTA 4659 improved colitis severity clinically and morphologically in mouse colitis caused by dextran sulfate sodium (Liu et al., 2022a). Other studies investigated the efficiency of L. reuteri R2LC, ATCC PTA 4659, F-9-35, and 5454, these strains also can alleviate inflammation in mice colitis, characterized by downregulated pro-inflammatory tumor necrosis factor-α (TNF-α), IL-1β, and interferon-γ, the decisive cytokines in colitis development (Ahl et al., 2016; Sun et al., 2018; Hrdy et al., 2020). This may be explained by the boosted expression of the TJ proteins along with cytoprotective heat shock proteins after L. reuteri therapy, thereby conferring protection against defects in gut barrier function (Ahl et al., 2016; Liu et al., 2022a). Meanwhile, L. reuteri supplement can also reverse gut microbiota dysbiosis induced by colitis. It was reported that after L. reuteri FYNDL13 treatment, enriched beneficial bacteria (Bifidobacterium, Akkermansia, Blautia and Oscillospira) together with reduced harmful bacteria (Bacteroides and Sutterella) content was observed (Lin et al., 2023). Moreover, L. reuteri therapy can offset bacterial translocation to the mesenteric lymph nodes caused by colitis, leading to the amelioration of excessive immune reactions (Liu et al., 2022a). Secretory immunoglobulin (Ig) A is responsible for bacterial translocation and bacterial toxins neutralization in the intestinal mucosa (Tezuka and Ohteki, 2019). Recently, it was discovered that orally administered L. reuteri R2LC stimulated the proliferation of the B lymphocytes in Peyer’s patches and induced IgA production and secretion to the intestinal lumen via the PD-1 pathway (Liu et al., 2021a). Consequently, treatment based on L. reuteri R2LC regulated alterations in the gut microbiome and shielded against colitis caused by dextran-sulfate-sodium (Liu et al., 2021a). Dendritic cells (DCs) are responsible for handling pathogens when the gut barriers are disrupted in IBD (Wei et al., 2022). L. reuteri was proven to possess the potential to promote DCs differentiation and maturation and boost regulatory T cells (Tregs) induction (Haileselassie et al., 2016; Hrdy et al., 2020). In addition, it is demonstrated that L. reuteri may reduce intestine inflammation by the mechanisms dependent on aryl hydrocarbon receptor activation, an immune and bacteria sensor receptor, correlated to inflammatory responses in IBD pathogenesis (Pernomian et al., 2020). The tryptophan metabolites from L. reuteri can activate aryl hydrocarbon receptors, inducing IL-22 production to maintain mucosal protection (Zelante et al., 2013). And the microbial histamine produced by L. reuteri has been proven to ameliorate intestinal inflammation in mice via activating H2R signaling (Gao et al., 2015). It was also reported that LPxTG-motif surface protein derived from L. reuteri SH 23 can alleviate inflammation in a mice colitis model via the inhibition of the MAPK-dependent NF-κB transduction way (Zong et al., 2023).
Colon mucosa and submucosa may inflame and herniate through the muscular layer in cases of diverticulitis (Barbaro et al., 2022). Diet and lifestyle factors may induce changes in the gut microbiota community that contributes to mucosal inflammation and diverticulitis (Piccioni et al., 2021). Probiotics could be beneficial in diverticular disease because they can prevent harmful bacteria from adhering to the intestinal mucosa and from producing inflammatory markers (Piccioni et al., 2021). Two previous RCTs examined L. reuteri’s function in managing acute uncomplicated diverticulitis (AUD) in light of recent recommendations that the condition be treated without the use of antibiotics (Petruzziello et al., 2019; Ojetti et al., 2022). They revealed that the treatment with L. reuteri ATCC PTA 4659 can alleviate abdominal pain and reduce inflammatory markers as well as the duration of hospitalization in patients with AUD (Petruzziello et al., 2019; Ojetti et al., 2022), shown in Table 5.
Therefore, the above preclinical studies validate the therapeutic potential of specific strains of L. reuteri to enhance the natural defense of gut epitheliums, alleviate hyperimmune response and restore gut microecology balance in inflammatory intestinal diseases. Although clinical data are limited, L. reuteri can be an attractive adjuvant therapy for IBD and diverticulitis.
2.7 Colorectal cancer
As per global statistics, CRC is ranked third as the most prevalent type of malignancy and the fourth major contributor to deaths related to cancer worldwide (Baidoun et al., 2021). As is known to all, IBD is linked to the onset and progression of CRC (Mathey et al., 2021). Dysplasia, clonal proliferation, and malignant progression might all be caused by gut dysbacteriosis, which may also have dramatic effects on genetics and epigenetics (Eslami et al., 2019). Previously, researchers identified gut flora dysbiosis in patients with CRC, including the depletion of L. gallinarum, Streptococcus thermophilus and enriched Firmicutes and Bacteroidetes, along with decreased bacterial diversity (Dai et al., 2018). Reduced abundance of Lactobacillus, Roseburia and Bifidobacterium in gut microbiota composition was observed in early precursors of CRC including adenoma and serrated polyp (Rezasoltani et al., 2018).
Although the prognosis of CRC patients without local or distant metastatic disease is generally favorable, there are currently inadequate viable therapies for individuals with metastatic cancer (Bell et al., 2022). Accordingly, probiotic therapy has recently gained popularity as a promising CRC therapeutic approach. Gao and colleagues demonstrated that treatment with (histidine decarboxylase) HDC+ L. reuteri can induce luminal histamine production, consequently, decreased colon tumor frequency and size were observed in HDC-/- mice (Gao et al., 2017). Moreover, they discovered that an L. reuteri mutant with isogenic HDC deficiency that cannot generate histamine did not inhibit tumorigenesis, highlighting the essential function of histamine in preventing inflammatory responses and the development of colorectal tumors (Gao et al., 2017). Reuterin, another major compound produced by L. reuteri, was found to be downregulated in mice and human CRC (Bell et al., 2022). Consistently, reuterin has been shown to have an inhibitory impact on the proliferative rate of CRC cell lines such as RKO at a concentration of 25µM, but not at a higher dosage of 100µM on normal colonic epithelium (Yu et al., 2023b). Indole-3-lactic acid, a tryptophan catabolite that inhibits the IL-17 signaling pathway, was shown in another recent study to be a key player in the suppression of colorectal carcinogenesis following L. reuteri treatment (Han et al., 2023). RAR-related orphan receptor γt, a nuclear receptor, was the target of this microbial metabolite product, which prevented Th17 cell development (Han et al., 2023). The potential underlying mechanism of L. reuteri’s pro-apoptotic actions was investigated but still needs further investigation. L. reuteri MG5346 was reported to inhibit tumor growth by inducing cell apoptosis though upregulated caspase-9 activity (Kim et al., 2022b). Additionally, it has been demonstrated that sirtuin-3–L. reuteri interaction is essential for the development of gut tumors (Zhang et al., 2018). Sirtuin-3 is a tumor-suppressing gene and L. reuteri can inhibit the downregulation of Sirt3 expression during colorectal tumorigenesis (Zhang et al., 2018). A previous meta-analysis analyzed the relationship between intestine E-cadherin and the prognosis of CRC, they concluded that low expression of E-cadherin is a risk factor for poor prognosis in CRC sufferers (Chang et al., 2022). It has been evidenced that L. reuteri can boost E-cadherin expression, indicating a possible mechanism for treating CRC (Karimi et al., 2018).
In summary, considering these evidence, L. reuteri owns the ability to suppress intestinal tumor progression in direct and indirect ways, thereby it may develop into a potential adjuvant treatment, but it is premature to recommend widespread use of L. reuteri in CRC due to insufficient clinical data.
2.8 Liver diseases
The total cirrhosis incidence is growing and estimated to reach 112.1/100,000 person-years in 2040, largely attributed to the increase in non-alcoholic fatty liver disease and alcohol-related liver disease (Flemming et al., 2021). The liver is a central immunological organ, which is frequently exposed to metabolites derived from the intestinal microbiome, thus it is not surprising that the GI microbial community also plays a part in the pathologic process of liver diseases (Jones and Neish, 2021; Wang et al., 2021a). Particularly, gut microbiota dysbiosis may already exist at the early period of liver damage. Studies have shown alcohol-dependent subjects with liver disorder show compositional changes in the fecal microbiota, presented as a reduction in bacterial diversity and decreased relative abundance of Lactobacillus, Bifidobacterium, as well as Akkermansia muciniphila (Leclercq et al., 2014; Grander et al., 2018). The phenotype of ethanol-elicited hepatitis was reversed by L. reuteri, partially by regulating fatty acid metabolic pathways in mice alcoholic liver disease models (Zheng et al., 2020). It was also reported that L. reuteri MJM60668 supplementation to mice fed a high-fat diet ameliorated hepatic steatosis by inhibiting lipogenesis, which may be attributed to the improvement of the gut microbiome dysbiosis (Werlinger et al., 2022). Chen and colleagues reported that gut microbiota imbalance promoted liver tumorigenesis and administration with L. reuteri can improve tryptophan metabolism and reduce hepatic sterol regulatory element-binding protein 2 expression, thus suppressing tumorigenesis (Chen et al., 2022). L. reuteri was also reported to reduce IL-17A secretion of group 3 innate lymphoid cells (ILC3s) in liver by upregulating acetate levels, thus exerting anti-tumor effects in mice with hepatocellular carcinoma (Hu et al., 2023). These evidences revealed the interaction between gut microbiome and hepatic immune response as well as metabolite signal pathways. Hence the use of L. reuteri in managing liver disorders has drawn a lot of attention from researchers, although RCT studies on the bacteria’s protective effects for patients with hepatic ailments are still inadequate. In one RCT research, Ferolla et al. discovered that a 3-months supplementation of L. reuteri with guar gum as well as inulin reduced hepatic steatosis in subjects with nonalcoholic steatohepatitis (Ferolla et al., 2016). However, another RCT study carried out in 2017 showed that in obese patients with type 2 diabetes, oral dosing of L. reuteri DSM 17938 for 12 weeks seemed to have no impact on the microbiota composition, adiposity, or liver steatosis, although, in some subjects with higher gut microbiota diversity before intervention, it increased insulin sensitivity (Mobini et al., 2017). The contrary outcomes may be attributed to the of microbial diversity in complicated metabolic disease conditions and the crosstalk caused by obesity. Taken together, these preclinical literatures provide evidence that L. reuteri may relieve liver injury in several signaling pathways probably by reversing gut microbiota dysbiosis. Given this, it may act as a potential probiotic for the prevention or therapeutic strategy for liver diseases, but further long-term well-designed clinical trials are still needed.
3 Potential L. reuteri mechanisms in digestive system diseases
As illustrated in Figure 1, growing preclinical investigations revealed that L. reuteri restores gut microbiota balance, products antimicrobial metabolites, regulates intestinal immunity and mediates mucosal homeostasis, thereby exhibiting protective effects on digestive system diseases.
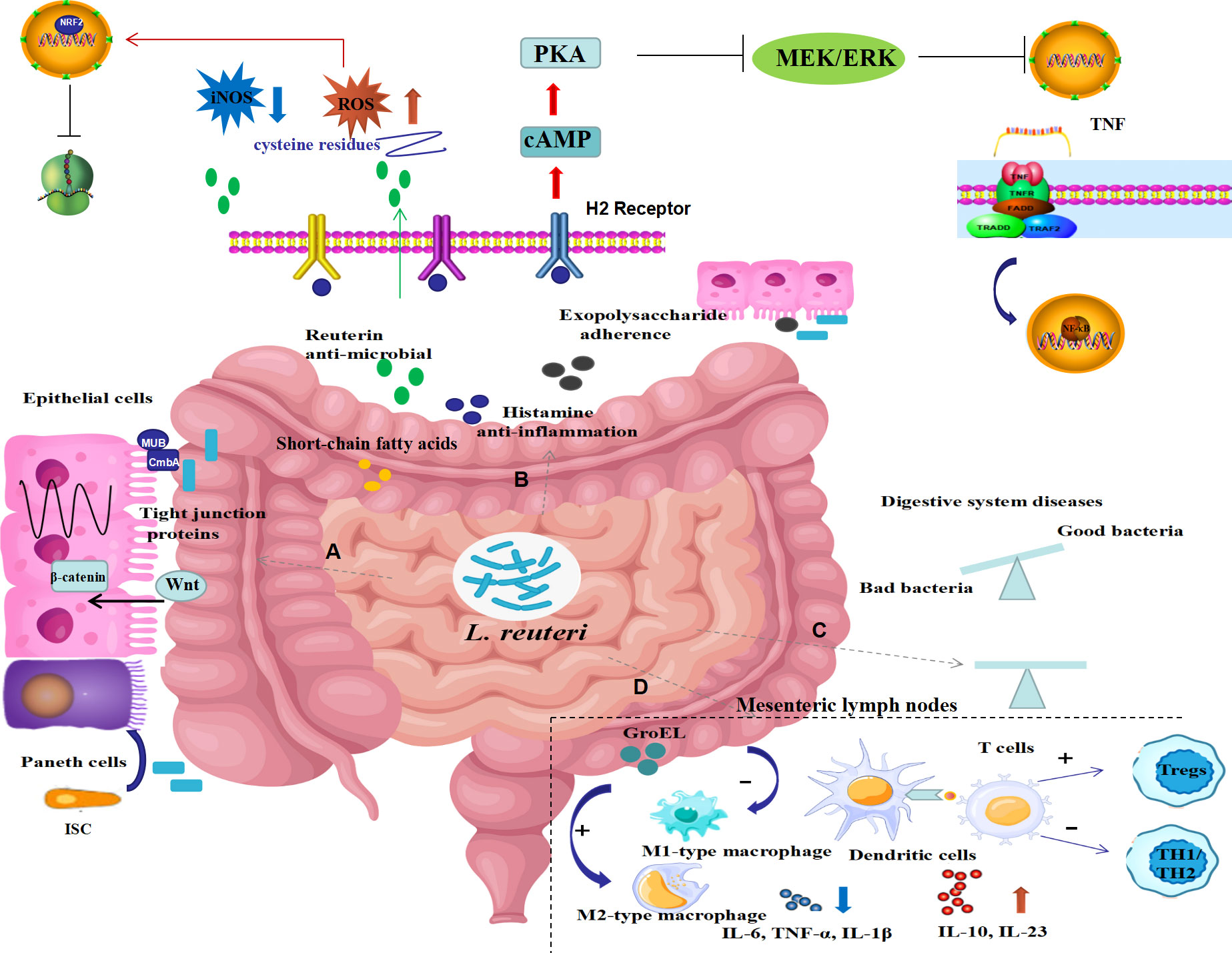
Figure 1 Possible underlying mechanisms of L. reuteri in digestive system diseases. The underlying mechanisms of L. reuteri application in intestinal diseases may include the following ways: (A) preservation of gut barrier function by increasing expression of tight junction proteins, promoting the intestine epithelial cell proliferation, and inducing intestinal stem cell differentiation to Paneth cells by activating Wnt/β-catenin pathway; (B) production of metabolites including reuterin, histamine, exopolysaccharide, short-chain fatty acids, thus mediating antibacterial, anti-inflammatory and anti-oxidative stress properties via inhibited NF-κB signaling pathways as well as activated NRF2 signaling pathways; (C) modification of the composition of the gut bacteria to restore balance; (D) regulation of intestinal immune response by selecting macrophage phenotype, promoting dendritic cell differentiation, suppressing Th1/Th2 responses, inducing proliferation of regulatory T cells, thereby suppressing inflammatory responses. ISC, intestine epithelial cell; ROS, reactive oxygen species; iNOS, inducible nitric oxide synthase; cAMP, cyclic adenosine monophosphate; PKA, Protein kinase A; TNF, tumor necrosis factor; NRF2, nuclear factor erythroid-derived 2; H2 receptor, histamine 2 receptor; IL, interleukin; Tregs, regulatory T cells.
3.1 Remodeling the gut microbiota
It is well-documented that L. reuteri can alter the bacterial diversity and abundance of various microorganisms, largely strain-specific. For instance, administration with L. reuteri LR6 and KT260178 both resulted in higher counts of total Lactobacillus, Bifidobacterium (Garg et al., 2020; Yang et al., 2020). While L. reuteri DSM 17938 supplement improved bacterial diversity along with enriched Firmicutes and reduced abundance of Bacteroidetes (Liu et al., 2019). Similar results have been observed in disease conditions. After supplement with L. reuteri DSM 17938, investigators observed enriched fecal Lactobacilli and a decrease in the colicky infants’ fecal E. coli counts (Savino et al., 2010; Savino et al., 2018b). Consistently, L. reuteri ATCC PTA 4659 peroral therapy alleviated mice colitis by maintaining the diversity of the colon’s microbiome (Liu et al., 2022a).
The beneficial properties of L. reuteri on gut microflora dysbiosis may be partially explained by the competition of L. reuteri and these harmful genera for the intestinal epithelium binding sites (Walsham et al., 2016). Walsham et al. reported that L. reuteri can bind to the epithelial cell surface by mucus-binding proteins CmbA and MUB, thus inhibiting enteropathogenic E. coli adherence to the gut epithelium (Walsham et al., 2016). Bioinformatics analysis further revealed that the inhibition mechanism may be associated with PI3K-Akt and MAPK signaling pathways (Qin et al., 2023b). Furthermore, L. reuteri may inhibit harmful bacteria by the production of antimicrobial metabolites like reuterin (Asare et al., 2020). The probiotic management of the gastrointestinal microbiota may also be mediated by regulating the intestinal pH through lactic acid production as well as some other antimicrobial substances like acetic acid and ethanol of some L. reuteri strains (Vitale et al., 2023). L. reuteri is demonstrated to be effective against a range of GI microbial infections, including Salmonella, Enterobacteriaceae, and others, partially depending on the synthesis of these compounds (Kao et al., 2020; Laosee et al., 2022; Qin et al., 2023a). In mice with colitis caused by dextran-sulfate-sodium, dosing of L. reuteri R2LC may remodel the makeup of the gut microbiome via enhanced intestinal IgA production (Liu et al., 2021a). It has been demonstrated that IgA is responsible for the selection of intestinal colonized bacteria and tends to resist pathogenic bacteria (Schnupf et al., 2018).
Overall, L. reuteri is shown to modulate the gut microbial community, characterized by enriched beneficial genera and diminished pathogenetic genera. Nonetheless, further research is required to determine the interaction between GI health and the modulated gut microbiota.
3.2 Production of antimicrobial metabolites
With the development of metabolomics and bacterial genetics strategy, small compounds produced by L. reuteri were identified. It has been proposed that the metabolite products of L. reuteri strains are responsible for their antibacterial and immunoregulatory properties. We covered a few well-known metabolites associated with L. reuteri’s probiotic potential in this section.
3.2.1 Reuterin
Reuterin is a broad-spectrum antimicrobial compound generated by specific strains of L. reuteri during the anaerobic metabolism of glycerol (Asare et al., 2020). This antimicrobial compound, which is a blend of different 3-hydroxypropionaldehyde (3-HPA) forms, may suppress the proliferation of both Gram-positive and -negative bacteria, protozoa, and fungi (Asare et al., 2020; Saviano et al., 2021). Apart from being used as a food preservative product, reuterin was also identified as the most inhibitory compound in CRC cell lines (Bell et al., 2022). Recently, it was shown that reuterin can suppress tumor growth in the mouse model implanted with CRC xenograft tumors by inducing oxidative stress (Bell et al., 2022). The authors showed that reuterin can inhibit the growth of CRC cell lines with a dose of 25μM but the concentration of reuterin at a dose of 100μM seemed not cytotoxic to non-cancerous colon cell lines (Bell et al., 2022). The molecular mechanism might be explained by that reuterin can increase reactive oxygen species and thus reduce ribosomal biogenesis by selectively binding to cysteine residues, accompanied by increased gene expression downstream of nuclear factor erythroid-derived 2 (Nrf-2) (Bell et al., 2022). However, it was also revealed in vitro experiments that reuterin treatment at a dose of 250μM significantly suppressed LPS-induced oxidative stress by enhancing Nrf-2 expression and reducing reactive oxygen species production in HD11 macrophage cells (Xu et al., 2022). These data revealed that the effects of reuterin depend on certain cell types, reuterin concentration, and stimulation time.
3.2.2 Histamine
Biogenic amines, especially histamine, were reported to be able to modulate the host immune system (Dvornikova et al., 2023). Several strains of L. reuteri such as L. reuteri DSM 20016 are capable of producing histamine from the dietary component L-histidine, an amino acid (Smythe and Efthimiou, 2022). According to Lin and his colleagues, L. reuteri ATCC PTA 6475 inhibited the MAPK signaling pathway, reducing the generation of TNF in monocyte-derived macrophages isolated from children with Crohn’s disease (Lin et al., 2008). By using mass spectrometry, investigators further proved that the key substance of L. reuteri ATCC PTA 6475-mediated TNF inhibition was histamine (Thomas et al., 2012). Histamine’s pleiotropic actions are achieved by activating the H1R, H2R, H3R, and H4R histamine receptors present in mammalian cells (Shulpekova et al., 2021). Via activation of H2R on human monocytoid cell line THP-1 cells, histamine stimulated increased levels of cyclic adenosine monophosphate (cAMP) and improved protein kinase A activity, thus inhibiting MEK/ERK MAPK signaling and alleviating inflammation (Thomas et al., 2012). Consistently, Gao and his colleagues discovered that oral administration of HDC+ L. reuteri capable of converting L-histidine to histamine might successfully alleviate inflammatory reactions in a murine model of colitis (Gao et al., 2015). Subsequently, they found that by causing immature myeloid cells to aggregate, HDC deficiency can accelerate the development of CRC that is linked to inflammation, hinting that histamine may have antitumorigenic properties (Gao et al., 2017). H1R, H2R, and, to a less extent, H4R are expressed by myeloid cells in the GI tract (Sander et al., 2006). Consequently, histamine may be involved in intestinal immunomodulation and inflammation by mediating myeloid cells (Sander et al., 2006). Besides, it is reported that improved overall survival outcomes in patients with CRC were correlated with elevated gene expression of H2R and decreased gene expression of H1R in the intestinal mucosa (Shi et al., 2019). The study further demonstrated that histamine-producing L. reuteri can suppress tumorigenesis in an established CRC mouse model but compared to H1R antagonists, H2R antagonists dramatically increased both the size and number of tumors (Shi et al., 2019). Given these data, different receptors of histamine may play distinct roles in the gut.
3.2.3 Exopolysaccharide
Exopolysaccharide (EPS), a substance generated by L. reuteri, is essential for the development of biofilms and L. reuteri’s adhesion to surfaces of the epithelium (Wang et al., 2021b). And in vitro E. coli adherence to porcine epithelial cells was also inhibited by EPS synthesized by L. reuteri (Ksonzekova et al., 2016). Consequently, the gene expression of proinflammatory cytokines like TNF-α and IL-6 brought on by E. coli or Salmonella Typhimurium infection was suppressed by EPS derived from L. reuteri (Ksonzekova et al., 2016; Kissova et al., 2022).
3.2.4 Short-chain fatty acids
SCFAs are a set of metabolite products derived from L. reuteri and engaged in regulating immune response and inflammatory reactions, thereby, promoting intestinal health (Smith et al., 2013; Oh et al., 2021). Intestinal SCFAs mainly consist of acetate, propionate, and butyrate, which can be generated by L. reuteri in different situations (Liu et al., 2022c; Calvigioni et al., 2023; Van den Abbeele et al., 2023). SCFAs are also suggested to be essential for ameliorating gut microbiota dysbiosis in mice colitis treated with L. reuteri (Lee et al., 2022). Particularly, butyrate is the typical metabolite which can enhance gastrointestinal motility by mediating the enteric neurons and maintaining the proliferation of Cajal cells though the AKT-NF-κB pathway (He et al., 2020). Besides, L. reuteri was reported to exert anti-tumor effects in mice with hepatocellular carcinoma by upregulating acetate levels (Hu et al., 2023). Recently propionate and butyrate were also reported to suppress the adhesion and invasion ability of Salmonella Typhimurium in vitro experiments (Liu et al., 2022b).
Together, these observations provide substantial evidence that metabolites generated by L. reuteri are involved in the regulation of gut immune response and remodeling of the commensal microbiota composition via the activation of various signal pathways.
3.3 Enhancement of intestinal epithelial barrier
Numerous studies showed that intestinal epithelial barrier damage disrupts immune homeostasis and contributes to many intestinal disorders, especially IBD and CRC (Leibovitzh et al., 2023). The capacity of L. reuteri to boost gut barrier function has been demonstrated in several investigations (Yi et al., 2018; Gao et al., 2022). On one hand, L. reuteri owns the ability to maintain the intestinal epithelial repair function. Wu and colleagues found that L. reuteri reconstructed the epithelial integrity by activating the Wnt/β-catenin pathway, thereby promoting the intestine epithelial cell proliferation and inducing intestinal stem cell differentiation to Paneth cells, even under the condition of TNF-induced intestinal inflammation (Wu et al., 2020). On the other hand, L. reuteri was also reported to maintain the integrity of the gut barrier by upregulating the expression of TJ proteins, evidenced in in preclinical studies (Gao et al., 2022; Liu et al., 2022a; Li et al., 2023a). TJ proteins are primary for epithelial cell proliferation and gut permeability (Kuo et al., 2021). Bacterial extracellular membrane vesicles derived from L. reuteri contain lipoteichoic acid, identified as a Toll-like receptor (TLR) 2 activator (Pang et al., 2022). According to research, TLR2 activation strengthened the intestinal barrier by increased expression of TJ proteins via the PI3K-Akt-signaling pathway (Gu et al., 2016). L. reuteri was also reported to induce TJ proteins expression via the activated PKC-Nrf-2/HO-1pathway and inhibited NF-κB pathway, along with reduced apoptosis of intestinal epithelial cells (Zhou et al., 2022b). In conclusion, these results indicated the therapeutic benefits of L. reuteri in gastrointestinal diseases by repairing the gut barrier function though different pathways.
3.4 Regulation of immune cell differentiation and function
L. reuteri may exhibit beneficial effects in digestive system diseases via modulation of the immune system. DCs are crucial in the interaction between microorganisms and gut immune responses because of their advantageous location and capacity to present luminal antigens as “gatekeepers” (Saez et al., 2023). Mature DCs promote the polarization of naive T cells toward T helper (Th) 1, Th2, Th17, or Treg responses (Tiberio et al., 2018). L. reuteri 5454 treatment alleviated colitis inflammation in mice, the potential molecular mechanism might be explained by the efficiency of DCs primed with L. reuteri 5454 to promote Tregs differentiation and trigger IL-22 secretion (Hrdy et al., 2020). Another study revealed that L. reuteri 17938 may activate DCs via TLR2 and lead to Tregs expansion in the intestinal mucosa, thereby alleviating experimental necrotizing enterocolitis (Hoang et al., 2018). Further evidenced by in vitro experiments, L. reuteri cell-free supernatants promoted DCs differentiation with up-regulated surface markers (CCR7, CD83, CD86, HLA-DR) and enhanced cytokine synthesis (IL-6, IL-10, and IL-23), nonetheless, these DCs exhibited diminished phagocytic activity (Haileselassie et al., 2016). The similar results were also reported by Lasaviciute and his colleagues who showed that a mixed secondary response profile was produced in DCs after priming human monocytes with L. reuteri DSM17938 secretions, secreting low levels of TNF-α, and IL-27 and high levels of IL-1β and IL-6 (Lasaviciute et al., 2022). The particular manner by which L. reuteri modulates the phenotypic and operation of mucosal-like DC is yet fully understood. Notably, the involvement of surface proteins was examined recently in the interaction between L. reuteri and DCs. The study demonstrated that L. reuteri can promote the release of the immune-regulating IL-10 in monocyte-derived DCs independent of its mucus adhesins while the production of pro-inflammatory cytokines (IL-6, TNF-α, IL-1β) was enhanced by mucus adhesins (Bene et al., 2017).
Meanwhile, numerous preclinical studies showed L. reuteri also had the property to promote the proliferation of Tregs while suppressing the production of Th cells, specifically Th1 and Th2, accompanied by decreased mRNA expression of NF-κB, TLR4, TNF-α, IL-6, and upregulated IL-10 mRNA expression in the intestine (Hoang et al., 2018; Liu et al., 2019; Liu et al., 2023b). The forkhead box P3 (FoxP3) transcription factor is crucial in generating Tregs (Harada et al., 2022). And the FoxP3 mRNA expression level was shown to be elevated in peripheral blood after L. reuteri DSM 17938 administration in colicky infants (Savino et al., 2018b). In addition, the mechanism of Tregs induction may be associated with reprogrammed gut microbiota composition and SCFAs production by L. reuteri (Liu et al., 2019; Wen et al., 2021). However, the ability of L. reuteri to induce Tregs is generally strain-dependent, and certain L. reuteri strains have anti-inflammatory properties that are not always reliant on Tregs (Liu et al., 2021b; Liu et al., 2023a). Orally feeding L. reuteri can suppress Th1/Th2 responses in mesenteric lymph nodes in Treg-deficient mice via activation of adenosine A2A receptors (Liu et al., 2023a). This phenomenon may be explained by the specific property of L. reuteri to increase the level of plasma adenosine metabolites such as inosine (Liu et al., 2021b). Proteomics studies using mass spectrometry confirmed that bacterial extracellular membrane vesicles derived from L. reuteri carried numerous bacterial cell surface proteins, including 5’-nucleotidase, which initiate the process by which AMP is transformed into the signal molecule adenosine (Pang et al., 2022).
Besides, L. reuteri seemed to be able to promote M2 macrophage polarization. Pro-inflammatory M1-like macrophage indicators may be suppressed by the stress protein GroEL isolated from L. reuteri, whereas M2-like markers are favored, consequently suppressing intestinal production of pro-inflammatory markers (TNF-α, IL-1β, interferon-γ) and increasing anti-inflammatory IL-10 and IL-13 by the activation of a TLR4 pathway (Dias et al., 2021). Except for these, Wang and colleagues reported that oral therapy of L. reuteri effectively inhibited the progression of mice colitis induced by immune checkpoint blockade treatment, and they highlighted that the therapeutic impact of L. reuteri ATCC PTA 6475 was related to a decrease of ILC3s (Wang et al., 2019). ILC3s are mainly found in the intestinal mucosa and can drive proinflammatory responses and cause immunopathological damage (Jarade et al., 2021). The mechanism is not fully clear. It is possible that L. reuteri’s capacity to metabolize tryptophan into indole derivatives, which subsequently activate the aryl hydrocarbon receptor in ILC3s, is responsible for the underlying mechanism (Zelante et al., 2013; Schiering et al., 2017). Furthermore, a previous study investigated two strains extracted from piglets, L. reuteri ZJ617 and L. reuteri ZJ615, characterized by high and low adhesive ability respectively (Gao et al., 2016). The study showed that L. reuteri ZJ615 reduced phosphorylation levels of ERK1/2 while L. reuteri ZJ617 had no significant effects on the ERK1/2 signaling pathway in the ilea of the LPS-stimulated mice model (Gao et al., 2016). Given this, L. reuteri’s capacity to modulate the immune system may be somewhat dependent on its adhesive property.
Overall, these studies demonstrated that metabolites and bacterial components of L. reuteri as well as the restored balance of the gut microbiota are both engaged in the suppression of excessive immune response and protection the intestine from injury.
4 Conclusions and future perspectives
In the past decades, research regarding the link between digestive diseases and the gut microbiome is growing quickly, accompanied by increased interest in probiotics in gut health. L. reuteri may serve as a viable candidate for the treatment of digestive system disorders owing to its potent antimicrobial, immunomodulatory, and anti-inflammatory activities with nearly no safety risks.
In this review, we systematically interpreted its application in different digestive disorders, including IC, diarrhea and constipation, FAP, H. pylori infection, IBD, and liver diseases. The results discussed here showed that L. reuteri may alleviate the symptoms of digestive problems through a variety of mechanisms, such as manipulation of the intestinal microbial population, barrier function of the epithelium, regulation of immunity, and modulation of numerous metabolites. Most of the in vitro and in vivo experiments revealed beneficial impacts of L. reuteri but the results of some clinical trials may be controversial. This phenomenon may be explained, in part, by specific and different functions of multiple stains and the high diversity of the human gut microbiota affected by sex, population, diet as well as other factors.
According to well-established studies, L. reuteri treatment is effective in improving IC, diarrhea, constipation, and H. pylori infection, whether to be served as a monotherapy or adjuvant strategy. While given the limited clinical data, there is no sufficient support data to recommend L. reuteri application in FAP, IBD, diverticulitis, CRC and liver diseases. In conclusion, it is important to conduct more research on L. reuteri’s potential benefits for treating digestive system diseases so that it may be used in clinical settings and as a therapeutic strategy.
Author contributions
RY: Conceptualization, Funding acquisition, Methodology, Project administration, Resources, Supervision, Visualization, Writing – review & editing. YP: Writing – original draft. YM: Funding acquisition, Writing – original draft. ZL: Conceptualization, Writing – review & editing. YJ: Software, Writing – review & editing. ZX: Methodology, Supervision, Validation, Writing – review & editing.
Funding
This work was supported by the Jiangsu Provincial Department of Science and Technology (No. BE2022698), the Wuxi Science and Technology Bureau (No.Y20222003), the Wuxi Municipal Medical Innovation Team (No.CXTD2021013), the Wuxi Commission of Health and Family Planning (No. FYKY202109).
Acknowledgments
We would like to thank Bullet Edits for English language editing.
Conflict of interest
The authors declare that the research was conducted in the absence of any commercial or financial relationships that could be construed as a potential conflict of interest.
Publisher's note
All claims expressed in this article are solely those of the authors and do not necessarily represent those of their affiliated organizations, or those of the publisher, the editors and the reviewers. Any product that may be evaluated in this article, or claim that may be made by its manufacturer, is not guaranteed or endorsed by the publisher.
References
Agus, A., Planchais, J., Sokol, H. (2018). Gut microbiota regulation of tryptophan metabolism in health and disease. Cell Host Microbe 23 (6), 716–724. doi: 10.1016/j.chom.2018.05.003
Agustina, R., Kok, F. J., van de Rest, O., Fahmida, U., Firmansyah, A., Lukito, W., et al. (2012). Randomized trial of probiotics and calcium on diarrhea and respiratory tract infections in Indonesian children. Pediatrics 129 (5), e1155–e1164. doi: 10.1542/peds.2011-1379
Ahl, D., Liu, H., Schreiber, O., Roos, S., Phillipson, M., Holm, L. (2016). Lactobacillus reuteri increases mucus thickness and ameliorates dextran sulphate sodium-induced colitis in mice. Acta Physiol. (Oxf) 217 (4), 300–310. doi: 10.1111/apha.12695
Ahmed, T., Chisti, M. J., Rahman, M. W., Alam, T., Ahmed, D., Parvin, I., et al. (2021). Effect of 3 days of oral azithromycin on young children with acute diarrhea in low-resource settings: a randomized clinical trial. JAMA Netw. Open 4 (12), e2136726. doi: 10.1001/jamanetworkopen.2021.36726
Ali, M. S., Lee, E. B., Lim, S. K., Suk, K., Park, S. C. (2023). Isolation and identification of Limosilactobacillus reuteri PSC102 and evaluation of its potential probiotic, antioxidant, and antibacterial properties. Antioxid. (Basel) 12 (2). doi: 10.3390/antiox12020238
Asare, P. T., Zurfluh, K., Greppi, A., Lynch, D., Schwab, C., Stephan, R., et al. (2020). Reuterin demonstrates potent antimicrobial activity against a broad panel of human and poultry meat campylobacter spp. isolates. Microorganisms 8 (1). doi: 10.3390/microorganisms8010078
Azad, M. B., Konya, T., Maughan, H., Guttman, D. S., Field, C. J., Chari, R. S., et al. (2013). Gut microbiota of healthy Canadian infants: profiles by mode of delivery and infant diet at 4 months. CMAJ 185 (5), 385–394. doi: 10.1503/cmaj.121189
Baidoun, F., Elshiwy, K., Elkeraie, Y., Merjaneh, Z., Khoudari, G., Sarmini, M. T., et al. (2021). Colorectal cancer epidemiology: recent trends and impact on outcomes. Curr. Drug Targets 22 (9), 998–1009. doi: 10.2174/1389450121999201117115717
Bao, X., Yu, W., Chu, Z., Gao, J., Zhou, M., Gu, Y. (2023). Functional abdominal pain disorders in children in southern Anhui province, China are related to academic stress rather than academic performance. BMC Pediatr. 23 (1), 333. doi: 10.1186/s12887-023-04154-3
Barbaro, M. R., Cremon, C., Fuschi, D., Marasco, G., Palombo, M., Stanghellini, V., et al. (2022). Pathophysiology of diverticular disease: from diverticula formation to symptom generation. Int. J. Mol. Sci. 23 (12). doi: 10.3390/ijms23126698
Bell, H. N., Rebernick, R. J., Goyert, J., Singhal, R., Kuljanin, M., Kerk, S. A., et al. (2022). Reuterin in the healthy gut microbiome suppresses colorectal cancer growth through altering redox balance. Cancer Cell 40 (2), 185–200.e186. doi: 10.1016/j.ccell.2021.12.001
Bene, K. P., Kavanaugh, D. W., Leclaire, C., Gunning, A. P., MacKenzie, D. A., Wittmann, A., et al. (2017). Lactobacillus reuteri surface mucus adhesins upregulate inflammatory responses through interactions with innate C-type lectin receptors. Front. Microbiol. 8. doi: 10.3389/fmicb.2017.00321
Calvigioni, M., Bertolini, A., Codini, S., Mazzantini, D., Panattoni, A., Massimino, M., et al. (2023). HPLC-MS-MS quantification of short-chain fatty acids actively secreted by probiotic strains. Front. Microbiol. 14. doi: 10.3389/fmicb.2023.1124144
Chang, K., Jiang, L., Sun, Y., Li, H. (2022). Effect of E-cadherin on prognosis of colorectal cancer: a meta-analysis update. Mol. Diagn. Ther. 26 (4), 397–409. doi: 10.1007/s40291-022-00593-3
Chau, K., Lau, E., Greenberg, S., Jacobson, S., Yazdani-Brojeni, P., Verma, N., et al. (2015). Probiotics for infantile colic: a randomized, double-blind, placebo-controlled trial investigating Lactobacillus reuteri DSM 17938. J. Pediatr. 166 (1), 74–78. doi: 10.1016/j.jpeds.2014.09.020
Chen, C. C., Liou, J. M., Lee, Y. C., Hong, T. C., El-Omar, E. M., Wu, M. S. (2021). The interplay between Helicobacter pylori and gastrointestinal microbiota. Gut Microbes 13 (1), 1–22. doi: 10.1080/19490976.2021.1909459
Chen, W., Wen, L., Bao, Y., Tang, Z., Zhao, J., Zhang, X., et al. (2022). Gut flora disequilibrium promotes the initiation of liver cancer by modulating tryptophan metabolism and up-regulating SREBP2. Proc. Natl. Acad. Sci. U.S.A. 119 (52), e2203894119. doi: 10.1073/pnas.2203894119
Cheng, W. X., Ren, Y., Lu, M. M., Xu, L. L., Gao, J. G., Chen, D., et al. (2021). Palmitoylation in Crohn's disease: current status and future directions. World J. Gastroenterol. 27 (48), 8201–8215. doi: 10.3748/wjg.v27.i48.8201
Cimperman, L., Bayless, G., Best, K., Diligente, A., Mordarski, B., Oster, M., et al. (2011). A randomized, double-blind, placebo-controlled pilot study of Lactobacillus reuteri ATCC 55730 for the prevention of antibiotic-associated diarrhea in hospitalized adults. J. Clin. Gastroenterol. 45 (9), 785–789. doi: 10.1097/MCG.0b013e3182166a42
Coccorullo, P., Strisciuglio, C., Martinelli, M., Miele, E., Greco, L., Staiano, A. (2010). Lactobacillus reuteri (DSM 17938) in infants with functional chronic constipation: a double-blind, randomized, placebo-controlled study. J. Pediatr. 157 (4), 598–602. doi: 10.1016/j.jpeds.2010.04.066
Collinson, S., Deans, A., Padua-Zamora, A., Gregorio, G. V., Li, C., Dans, L. F., et al. (2020). Probiotics for treating acute infectious diarrhoea. Cochrane Database Syst. Rev. 12 (12), CD003048. doi: 10.1002/14651858.CD003048.pub4
Dai, Z., Coker, O. O., Nakatsu, G., Wu, W. K. K., Zhao, L., Chen, Z., et al. (2018). Multi-cohort analysis of colorectal cancer metagenome identified altered bacteria across populations and universal bacterial markers. Microbiome 6 (1), 70. doi: 10.1186/s40168-018-0451-2
Dargenio, C., Dargenio, V. N., Bizzoco, F., Indrio, F., Francavilla, R., Cristofori, F. (2021). Limosilactobacillus reuteri strains as adjuvants in the management of Helicobacter pylori infection. Medicina (Kaunas) 57 (7). doi: 10.3390/medicina57070733
de Brito, B. B., da Silva, F. A. F., Soares, A. S., Pereira, V. A., Santos, M. L. C., Sampaio, M. M., et al. (2019). Pathogenesis and clinical management of Helicobacter pylori gastric infection. World J. Gastroenterol. 25 (37), 5578–5589. doi: 10.3748/wjg.v25.i37.5578
Deng, Y., Zhou, M., Wang, J., Yao, J., Yu, J., Liu, W., et al. (2021). Involvement of the microbiota-gut-brain axis in chronic restraint stress: disturbances of the kynurenine metabolic pathway in both the gut and brain. Gut Microbes 13 (1), 1–16. doi: 10.1080/19490976.2020.1869501
de Weerth, C., Fuentes, S., Puylaert, P., de Vos, W. M. (2013). Intestinal microbiota of infants with colic: development and specific signatures. Pediatrics 131 (2), e550–e558. doi: 10.1542/peds.2012-1449
Dias, A. M. M., Douhard, R., Hermetet, F., Regimbeau, M., Lopez, T. E., Gonzalez, D., et al. (2021). Lactobacillus stress protein GroEL prevents colonic inflammation. J. Gastroenterol. 56 (5), 442–455. doi: 10.1007/s00535-021-01774-3
Dinleyici, E. C., Dalgic, N., Guven, S., Metin, O., Yasa, O., Kurugol, Z., et al. (2015). Lactobacillus reuteri DSM 17938 shortens acute infectious diarrhea in a pediatric outpatient setting. J. Pediatr. (Rio J) 91 (4), 392–396. doi: 10.1016/j.jped.2014.10.009
Dinleyici, E. C., Group, P. S., Vandenplas, Y. (2014). Lactobacillus reuteri DSM 17938 effectively reduces the duration of acute diarrhoea in hospitalised children. Acta Paediatr. 103 (7), e300–e305. doi: 10.1111/apa.12617
Dore, M. P., Bibbo, S., Loria, M., Salis, R., Manca, A., Pes, G. M., et al. (2019). Twice-a-day PPI, tetracycline, metronidazole quadruple therapy with Pylera(R) or Lactobacillus reuteri for treatment naive or for retreatment of Helicobacter pylori. Two randomized pilot studies. Helicobacter. 24 (6), e12659. doi: 10.1111/hel.12659
Dore, M. P., Sau, R., Niolu, C., Abbondio, M., Tanca, A., Bibbo, S., et al. (2022). Metagenomic changes of gut microbiota following treatment of Helicobacter pylori Infection with a simplified low-dose quadruple therapy with bismuth or Lactobacillus reuteri. Nutrients 14 (14). doi: 10.3390/nu14142789
Dvornikova, K. A., Platonova, O. N., Bystrova, E. Y. (2023). Inflammatory bowel disease: crosstalk between histamine, immunity, and disease. Int. J. Mol. Sci. 24 (12). doi: 10.3390/ijms24129937
Eftekhari, K., Vahedi, Z., KaMali Aghdam, M., Noemi Diaz, D. (2015). A randomized double-blind placebo-controlled trial of Lactobacillus reuteri for chronic functional abdominal pain in children. Iran J. Pediatr. 25 (6), e2616. doi: 10.5812/ijp.2616
Eslami, M., Yousefi, B., Kokhaei, P., Hemati, M., Nejad, Z. R., Arabkari, V., et al. (2019). Importance of probiotics in the prevention and treatment of colorectal cancer. J. Cell Physiol. 234 (10), 17127–17143. doi: 10.1002/jcp.28473
Fatheree, N. Y., Liu, Y., Taylor, C. M., Hoang, T. K., Cai, C., Rahbar, M. H., et al. (2017). Lactobacillus reuteri for infants with colic: a double-blind, placebo-controlled, randomized clinical trial. J. Pediatr. 191, 170–178.e172. doi: 10.1016/j.jpeds.2017.07.036
Ferolla, S. M., Couto, C. A., Costa-Silva, L., Armiliato, G. N., Pereira, C. A., Martins, F. S., et al. (2016). Beneficial effect of synbiotic supplementation on hepatic steatosis and anthropometric parameters, but not on gut permeability in a population with nonalcoholic steatohepatitis. Nutrients 8 (7). doi: 10.3390/nu8070397
Flemming, J. A., Djerboua, M., Groome, P. A., Booth, C. M., Terrault, N. A. (2021). NAFLD and alcohol-associated liver disease will be responsible for almost all new diagnoses of cirrhosis in Canada by 2040. Hepatology 74 (6), 3330–3344. doi: 10.1002/hep.32032
Forooghi Nia, F., Rahmati, A., Ariamanesh, M., Saeidi, J., Ghasemi, A., Mohtashami, M. (2023). The anti-Helicobacter pylori effects of Limosilactobacillus reuteri strain 2892 isolated from camel milk in C57BL/6 mice. World J. Microbiol. Biotechnol. 39 (5), 119. doi: 10.1007/s11274-023-03555-x
Francavilla, R., Lionetti, E., Castellaneta, S., Ciruzzi, F., Indrio, F., Masciale, A., et al. (2012). Randomised clinical trial: Lactobacillus reuteri DSM 17938 vs. placebo in children with acute diarrhoea–a double-blind study. Aliment Pharmacol. Ther. 36 (4), 363–369. doi: 10.1111/j.1365-2036.2012.05180.x
Francavilla, R., Lionetti, E., Castellaneta, S. P., Magista, A. M., Maurogiovanni, G., Bucci, N., et al. (2008). Inhibition of Helicobacter pylori infection in humans by Lactobacillus reuteri ATCC 55730 and effect on eradication therapy: a pilot study. Helicobacter. 13 (2), 127–134. doi: 10.1111/j.1523-5378.2008.00593.x
Francavilla, R., Polimeno, L., DemiChina, A., Maurogiovanni, G., Principi, B., Scaccianoce, G., et al. (2014). Lactobacillus reuteri strain combination in Helicobacter pylori infection: a randomized, double-blind, placebo-controlled study. J. Clin. Gastroenterol. 48 (5), 407–413. doi: 10.1097/MCG.0000000000000007
Gao, J., Cao, S., Xiao, H., Hu, S., Yao, K., Huang, K., et al. (2022). Lactobacillus reuteri 1 enhances intestinal epithelial barrier function and alleviates the inflammatory response induced by enterotoxigenic escherichia coli K88 via suppressing the MLCK signaling pathway in IPEC-J2 cells. Front. Immunol. 13. doi: 10.3389/fimmu.2022.897395
Gao, C., Ganesh, B. P., Shi, Z., Shah, R. R., Fultz, R., Major, A., et al. (2017). Gut microbe-mediated suppression of inflammation-associated colon carcinogenesis by luminal histamine production. Am. J. Pathol. 187 (10), 2323–2336. doi: 10.1016/j.ajpath.2017.06.011
Gao, K., Liu, L., Dou, X., Wang, C., Liu, J., Zhang, W., et al. (2016). Doses Lactobacillus reuteri depend on adhesive ability to modulate the intestinal immune response and metabolism in mice challenged with lipopolysaccharide. Sci. Rep. 6, 28332. doi: 10.1038/srep28332
Gao, C., Major, A., Rendon, D., Lugo, M., Jackson, V., Shi, Z., et al. (2015). Histamine H2 receptor-mediated suppression of intestinal inflammation by probiotic Lactobacillus reuteri. mBio 6 (6), e01358–e01315. doi: 10.1128/mBio.01358-15
Garcia Contreras, A. A., Vasquez Garibay, E. M., Sanchez Ramirez, C. A., Fafutis Morris, M., Delgado Rizo, V. (2020). Lactobacillus reuteri DSM 17938 and agave inulin in children with cerebral palsy and chronic constipation: a double-blind randomized placebo controlled clinical trial. Nutrients 12 (10). doi: 10.3390/nu12102971
Garg, S., Singh, T. P., Malik, R. K. (2020). In vivo implications of potential probiotic Lactobacillus reuteri LR6 on the gut and immunological parameters as an adjuvant against protein energy malnutrition. Probiotics Antimicrob. Proteins 12 (2), 517–534. doi: 10.1007/s12602-019-09563-4
Ge, X., Zhao, W., Ding, C., Tian, H., Xu, L., Wang, H., et al. (2017). Potential role of fecal microbiota from patients with slow transit constipation in the regulation of gastrointestinal motility. Sci. Rep. 7 (1), 441. doi: 10.1038/s41598-017-00612-y
Gordon, I. O., Abushamma, S., Kurowski, J. A., Holubar, S. D., Kou, L., Lyu, R., et al. (2022). Paediatric ulcerative colitis is a fibrotic disease and is linked with chronicity of inflammation. J. Crohns Colitis 16 (5), 804–821. doi: 10.1093/ecco-jcc/jjab216
Gordon, M., Biagioli, E., Sorrenti, M., Lingua, C., Moja, L., Banks, S. S., et al. (2018). Dietary modifications for infantile colic. Cochrane Database Syst. Rev. 10 (10), CD011029. doi: 10.1002/14651858.CD011029.pub2
Grander, C., Adolph, T. E., Wieser, V., Lowe, P., Wrzosek, L., Gyongyosi, B., et al. (2018). Recovery of ethanol-induced Akkermansia muciniphila depletion ameliorates alcoholic liver disease. Gut 67 (5), 891–901. doi: 10.1136/gutjnl-2016-313432
Gu, M. J., Song, S. K., Lee, I. K., Ko, S., Han, S. E., Bae, S., et al. (2016). Barrier protection via Toll-like receptor 2 signaling in porcine intestinal epithelial cells damaged by deoxynivalnol. Vet. Res. 47, 25. doi: 10.1186/s13567-016-0309-1
Gutierrez-Castrellon, P., Lopez-Velazquez, G., Diaz-Garcia, L., Jimenez-Gutierrez, C., Mancilla-Ramirez, J., Estevez-Jimenez, J., et al. (2014). Diarrhea in preschool children and Lactobacillus reuteri: a randomized controlled trial. Pediatrics 133 (4), e904–e909. doi: 10.1542/peds.2013-0652
Haileselassie, Y., Navis, M., Vu, N., Qazi, K. R., Rethi, B., Sverremark-Ekstrom, E. (2016). Lactobacillus reuteri and Staphylococcus aureus differentially influence the generation of monocyte-derived dendritic cells and subsequent autologous T cell responses. Immun. Inflammation Dis. 4 (3), 315–326. doi: 10.1002/iid3.115
Han, J. X., Tao, Z. H., Wang, J. L., Zhang, L., Yu, C. Y., Kang, Z. R., et al. (2023). Microbiota-derived tryptophan catabolites mediate the chemopreventive effects of statins on colorectal cancer. Nat. Microbiol. 8 (5), 919–933. doi: 10.1038/s41564-023-01363-5
Happy Tummy, C., Lavalle, L., Sauvageot, N., Cercamondi, C. I., Jankovic, I., Egli, D., et al. (2023). Limosilactobacillus reuteri DSM 17938-containing infant formulas and the associations with gastrointestinal tolerance: a cross-sectional observational study. Nutrients 15 (3). doi: 10.3390/nu15030530
Harada, Y., Miyamoto, K., Chida, A., Okuzawa, A. T., Yoshimatsu, Y., Kudo, Y., et al. (2022). Localization and movement of Tregs in gastrointestinal tract: a systematic review. Inflammation Regener. 42 (1), 47. doi: 10.1186/s41232-022-00232-8
He, Q., Han, C., Huang, L., Yang, H., Hu, J., Chen, H., et al. (2020). Astragaloside IV alleviates mouse slow transit constipation by modulating gut microbiota profile and promoting butyric acid generation. J. Cell Mol. Med. 24 (16), 9349–9361. doi: 10.1111/jcmm.15586
Hegde, S., Lin, Y. M., Fu, Y., Savidge, T., Shi, X. Z. (2020). Precision Lactobacillus reuteri therapy attenuates luminal distension-associated visceral hypersensitivity by inducing peripheral opioid receptors in the colon. Pain 161 (12), 2737–2749. doi: 10.1097/j.pain.0000000000001967
Hoang, T. K., He, B., Wang, T., Tran, D. Q., Rhoads, J. M., Liu, Y. (2018). Protective effect of Lactobacillus reuteri DSM 17938 against experimental necrotizing enterocolitis is mediated by Toll-like receptor 2. Am. J. Physiol. Gastrointest. Liver Physiol. 315 (2), G231–G240. doi: 10.1152/ajpgi.00084.2017
Holz, C., Busjahn, A., Mehling, H., Arya, S., Boettner, M., Habibi, H., et al. (2015). Significant reduction in Helicobacter pylori load in humans with non-viable Lactobacillus reuteri DSM17648: a pilot study. Probiotics Antimicrob. Proteins 7 (2), 91–100. doi: 10.1007/s12602-014-9181-3
Hrdy, J., Alard, J., Couturier-Maillard, A., Boulard, O., Boutillier, D., Delacre, M., et al. (2020). Lactobacillus reuteri 5454 and Bifidobacterium aniMalis ssp. lactis 5764 improve colitis while differentially impacting dendritic cells maturation and antimicrobial responses. Sci. Rep. 10 (1), 5345. doi: 10.1038/s41598-020-62161-1
Hu, C., Xu, B., Wang, X., Wan, W. H., Lu, J., Kong, D., et al. (2023). Gut microbiota-derived short-chain fatty acids regulate group 3 innate lymphoid cells in HCC. Hepatology 77 (1), 48–64. doi: 10.1002/hep.32449
Hurst, N. R., Kendig, D. M., Murthy, K. S., Grider, J. R. (2014). The short chain fatty acids, butyrate and propionate, have differential effects on the motility of the Guinea pig colon. Neurogastroenterol. Motil. 26 (11), 1586–1596. doi: 10.1111/nmo.12425
Imase, K., Tanaka, A., Tokunaga, K., Sugano, H., Ishida, H., Takahashi, S. (2007). Lactobacillus reuteri tablets suppress Helicobacter pylori infection–a double-blind randomised placebo-controlled cross-over clinical study. Kansenshogaku Zasshi 81 (4), 387–393. doi: 10.11150/kansenshogakuzasshi1970.81.387
Indrio, F., Dargenio, V. N., Francavilla, R., Szajewska, H., Vandenplas, Y. (2023). Infantile colic and long-term outcomes in childhood: a narrative synthesis of the evidence. Nutrients 15 (3). doi: 10.3390/nu15030615
Indrio, F., Di Mauro, A., Riezzo, G., Civardi, E., Intini, C., Corvaglia, L., et al. (2014). Prophylactic use of a probiotic in the prevention of colic, regurgitation, and functional constipation: a randomized clinical trial. JAMA Pediatr. 168 (3), 228–233. doi: 10.1001/jamapediatrics.2013.4367
Jadresin, O., Hojsak, I., Misak, Z., Kekez, A. J., Trbojevic, T., Ivkovic, L., et al. (2017). Lactobacillus reuteri DSM 17938 in the treatment of functional abdominal pain in children: RCT Study. J. Pediatr. Gastroenterol. Nutr. 64 (6), 925–929. doi: 10.1097/MPG.0000000000001478
Jadresin, O., Sila, S., Trivic, I., Misak, Z., Hojsak, I., Kolacek, S. (2018). Lack of benefit of Lactobacillus reuteri DSM 17938 as an addition to the treatment of functional constipation. J. Pediatr. Gastroenterol. Nutr. 67 (6), 763–766. doi: 10.1097/MPG.0000000000002134
Jadresin, O., Sila, S., Trivic, I., Misak, Z., Kolacek, S., Hojsak, I. (2020). Lactobacillus reuteri DSM 17938 is effective in the treatment of functional abdominal pain in children: results of the double-blind randomized study. Clin. Nutr. 39 (12), 3645–3651. doi: 10.1016/j.clnu.2020.04.019
Jarade, A., Di Santo, J. P., Serafini, N. (2021). Group 3 innate lymphoid cells mediate host defense against attaching and effacing pathogens. Curr. Opin. Microbiol. 63, 83–91. doi: 10.1016/j.mib.2021.06.005
Jones, M. L., Martoni, C. J., Ganopolsky, J. G., Sulemankhil, I., Ghali, P., Prakash, S. (2013). Improvement of gastrointestinal health status in subjects consuming Lactobacillus reuteri NCIMB 30242 capsules: a post-hoc analysis of a randomized controlled trial. Expert Opin. Biol. Ther. 13 (12), 1643–1651. doi: 10.1517/14712598.2013.833601
Jones, R. M., Neish, A. S. (2021). Gut microbiota in intestinal and liver disease. Annu. Rev. Pathol. 16, 251–275. doi: 10.1146/annurev-pathol-030320-095722
Kambale, R. M., Ntagazibwa, J. N., Kasengi, J. B., Zigashane, A. B., Francisca, I. N., Mashukano, B. N., et al. (2023). Probiotics for children with uncomplicated severe acute malnutrition (PruSAM study): a randomized controlled trial in the Democratic Republic of Congo. Am. J. Clin. Nutr. 117 (5), 976–984. doi: 10.1016/j.ajcnut.2023.01.019
Kao, L., Liu, T. H., Tsai, T. Y., Pan, T. M. (2020). Beneficial effects of the commercial lactic acid bacteria product, Vigiis 101, on gastric mucosa and intestinal bacterial flora in rats. J. Microbiol. Immunol. Infect. 53 (2), 266–273. doi: 10.1016/j.jmii.2018.06.002
Kaplan, G. G. (2015). The global burden of IBD: from 2015 to 2025. Nat. Rev. Gastroenterol. Hepatol. 12 (12), 720–727. doi: 10.1038/nrgastro.2015.150
Kapourchali, F. R., Cresci, G. A. M. (2020). Early-Life gut microbiome-the importance of maternal and infant factors in its establishment. Nutr. Clin. Pract. 35 (3), 386–405. doi: 10.1002/ncp.10490
Karimi, S., Jonsson, H., Lundh, T., Roos, S. (2018). Lactobacillus reuteri strains protect epithelial barrier integrity of IPEC-J2 monolayers from the detrimental effect of enterotoxigenic Escherichia coli. Physiol. Rep. 6 (2). doi: 10.14814/phy2.13514
Khalif, I. L., Quigley, E. M., Konovitch, E. A., Maximova, I. D. (2005). Alterations in the colonic flora and intestinal permeability and evidence of immune activation in chronic constipation. Dig Liver Dis. 37 (11), 838–849. doi: 10.1016/j.dld.2005.06.008
Kim, S. J., Kang, C. H., Kim, G. H., Cho, H. (2022b). Anti-tumor effects of heat-killed L. reuteri MG5346 and L. casei MG4584 against human colorectal carcinoma through caspase-9-dependent apoptosis in xenograft model. Microorganisms 10 (3). doi: 10.3390/microorganisms10030533
Kim, S., Lee, H. H., Choi, W., Kang, C. H., Kim, G. H., Cho, H. (2022a). Anti-tumor effect of heat-killed Bifidobacterium bifidum on human gastric cancer through Akt-p53-dependent mitochondrial apoptosis in xenograft models. Int. J. Mol. Sci. 23 (17). doi: 10.3390/ijms23179788
Kissova, Z., Tkacikova, L., Mudronova, D., Bhide, M. R. (2022). Immunomodulatory effect of Lactobacillus reuteri (Limosilactobacillus reuteri) and its exopolysaccharides investigated on epithelial cell line IPEC-J2 challenged with Salmonella Typhimurium. Life (Basel) 12 (12). doi: 10.3390/life12121955
Kolodziej, M., Szajewska, H. (2019). Lactobacillus reuteri DSM 17938 in the prevention of antibiotic-associated diarrhoea in children: a randomized clinical trial. Clin. Microbiol. Infect. 25 (6), 699–704. doi: 10.1016/j.cmi.2018.08.017
Korpela, K., Renko, M., Paalanne, N., Vanni, P., Salo, J., Tejesvi, M., et al. (2020). Microbiome of the first stool after birth and infantile colic. Pediatr. Res. 88 (5), 776–783. doi: 10.1038/s41390-020-0804-y
Kotloff, K. L. (2022). Bacterial diarrhoea. Curr. Opin. Pediatr. 34 (2), 147–155. doi: 10.1097/MOP.0000000000001107
Kozhakhmetov, S., Meiirmanova, Z., Mukhanbetzhanov, N., Jarmukhanov, Z., Vinogradova, E., Mureyev, S., et al. (2023). Compositional and functional variability of the gut microbiome in children with infantile colic. Sci. Rep. 13 (1), 9530. doi: 10.1038/s41598-023-36641-z
Ksonzekova, P., Bystricky, P., Vlckova, S., Patoprsty, V., Pulzova, L., Mudronova, D., et al. (2016). Exopolysaccharides of Lactobacillus reuteri: their influence on adherence of E. coli to epithelial cells and inflammatory response. Carbohydr Polym. 141, 10–19. doi: 10.1016/j.carbpol.2015.12.037
Kubota, M., Ito, K., Tomimoto, K., Kanazaki, M., Tsukiyama, K., Kubota, A., et al. (2020). Lactobacillus reuteri DSM 17938 and magnesium oxide in children with functional chronic constipation: a double-blind and randomized clinical trial. Nutrients 12 (1). doi: 10.3390/nu12010225
Kuo, W. T., Zuo, L., Odenwald, M. A., Madha, S., Singh, G., Gurniak, C. B., et al. (2021). The tight junction protein ZO-1 is dispensable for barrier function but critical for effective mucosal repair. Gastroenterology 161 (6), 1924–1939. doi: 10.1053/j.gastro.2021.08.047
Laosee, W., Kantachote, D., Chansuwan, W., Sirinupong, N. (2022). Effects of probiotic fermented fruit juice-based biotransformation by lactic acid bacteria and Saccharomyces boulardii CNCM I-745 on anti-Salmonella and antioxidative properties. J. Microbiol. Biotechnol. 32 (10), 1315–1324. doi: 10.4014/jmb.2206.06012
Larabi, A., Barnich, N., Nguyen, H. T. T. (2020). New insights into the interplay between autophagy, gut microbiota and inflammatory responses in IBD. Autophagy 16 (1), 38–51. doi: 10.1080/15548627.2019.1635384
Lasaviciute, G., Barz, M., van der Heiden, M., Arasa, C., Tariq, K., Quin, J., et al. (2022). Gut commensal Limosilactobacillus reuteri induces atypical memory-like phenotype in human dendritic cells in vitro. Gut Microbes 14 (1), 2045046. doi: 10.1080/19490976.2022.2045046
Leclercq, S., Matamoros, S., Cani, P. D., Neyrinck, A. M., Jamar, F., Starkel, P., et al. (2014). Intestinal permeability, gut-bacterial dysbiosis, and behavioral markers of alcohol-dependence severity. Proc. Natl. Acad. Sci. U.S.A. 111 (42), E4485–E4493. doi: 10.1073/pnas.1415174111
Lee, H. L., Kim, J. M., Moon, J. H., Kim, M. J., Jeong, H. R., Go, M. J., et al. (2022). Anti-amnesic effect of synbiotic supplementation containing corni fructus and Limosilactobacillus reuteri in DSS-induced colitis mice. Int. J. Mol. Sci. 24 (1). doi: 10.3390/ijms24010090
Leibovitzh, H., Lee, S. H., Raygoza Garay, J. A., Espin-Garcia, O., Xue, M., Neustaeter, A., et al. (2023). Immune response and barrier dysfunction-related proteomic signatures in preclinical phase of Crohn's disease highlight earliest events of pathogenesis. Gut 72 (8), 1462–1471. doi: 10.1136/gutjnl-2022-328421
Li, J., Feng, S., Wang, Z., He, J., Zhang, Z., Zou, H., et al. (2023b). Limosilactobacillus mucosae-derived extracellular vesicles modulates macrophage phenotype and orchestrates gut homeostasis in a diarrheal piglet model. NPJ Biofilms Microbiomes 9 (1), 33. doi: 10.1038/s41522-023-00403-6
Li, C., Su, Z., Chen, Z., Cao, J., Liu, X., Xu, F. (2023a). Lactobacillus reuteri strain 8008 attenuated the aggravation of depressive-like behavior induced by CUMS in high-fat diet-fed mice through regulating the gut microbiota. Front. Pharmacol. 14. doi: 10.3389/fphar.2023.1149185
Liang, B., Yuan, Y., Peng, X. J., Liu, X. L., Hu, X. K., Xing, D. M. (2022). Current and future perspectives for Helicobacter pylori treatment and management: from antibiotics to probiotics. Front. Cell Infect. Microbiol. 12. doi: 10.3389/fcimb.2022.1042070
Lin, Y. P., Thibodeaux, C. H., Pena, J. A., Ferry, G. D., Versalovic, J. (2008). Probiotic Lactobacillus reuteri suppress proinflammatory cytokines via c-Jun. Inflammation Bowel Dis. 14 (8), 1068–1083. doi: 10.1002/ibd.20448
Lin, C., Zheng, Y., Lu, J., Zhang, H., Wang, G., Chen, W. (2023). Differential reinforcement of intestinal barrier function by various Lactobacillus reuteri strains in mice with DSS-induced acute colitis. Life Sci. 314, 121309. doi: 10.1016/j.lfs.2022.121309
Lionetti, E., Miniello, V. L., Castellaneta, S. P., Magista, A. M., de Canio, A., Maurogiovanni, G., et al. (2006). Lactobacillus reuteri therapy to reduce side-effects during anti-Helicobacter pylori treatment in children: a randomized placebo controlled trial. Aliment Pharmacol. Ther. 24 (10), 1461–1468. doi: 10.1111/j.1365-2036.2006.03145.x
Liu, Y., Armbrister, S. A., Okeugo, B., Mills, T. W., Daniel, R. C., Oh, J. H., et al. (2023a). Probiotic-derived ecto-5'-nucleotidase produces anti-Inflammatory adenosine metabolites in Treg-deficient scurfy mice. Probiotics Antimicrob. Proteins 15, 1001–1013. doi: 10.1007/s12602-023-10089-z
Liu, H. Y., Giraud, A., Seignez, C., Ahl, D., Guo, F., Sedin, J., et al. (2021a). Distinct B cell subsets in Peyer's patches convey probiotic effects by Limosilactobacillus reuteri. Microbiome 9 (1), 198. doi: 10.1186/s40168-021-01128-4
Liu, H. Y., Gu, F., Zhu, C., Yuan, L., Zhu, C., Zhu, M., et al. (2022a). Epithelial heat shock proteins mediate the protective effects of Limosilactobacillus reuteri in dextran sulfate sodium-induced colitis. Front. Immunol. 13. doi: 10.3389/fimmu.2022.865982
Liu, Y., Hoang, T. K., Park, E. S., Freeborn, J., Okeugo, B., Tran, D. Q., et al. (2023b). Probiotic-educated Tregs are more potent than naive Tregs for immune tolerance in stressed new-born mice. Benef. Microbes 14 (1), 73–84. doi: 10.3920/BM2022.0095
Liu, Y., Hoang, T. K., Taylor, C. M., Park, E. S., Freeborn, J., Luo, M., et al. (2021b). Limosilactobacillus reuteri and Lacticaseibacillus rhamnosus GG differentially affect gut microbes and metabolites in mice with Treg deficiency. Am. J. Physiol. Gastrointest. Liver Physiol. 320 (6), G969–G981. doi: 10.1152/ajpgi.00072.2021
Liu, Y., Tian, X., He, B., Hoang, T. K., Taylor, C. M., Blanchard, E., et al. (2019). Lactobacillus reuteri DSM 17938 feeding of healthy newborn mice regulates immune responses while modulating gut microbiota and boosting beneficial metabolites. Am. J. Physiol. Gastrointest. Liver Physiol. 317 (6), G824–G838. doi: 10.1152/ajpgi.00107.2019
Liu, Y., Zhong, X., Lin, S., Xu, H., Liang, X., Wang, Y., et al. (2022c). Limosilactobacillus reuteri and caffeoylquinic acid synergistically promote adipose browning and ameliorate obesity-associated disorders. Microbiome 10 (1), 226. doi: 10.1186/s40168-022-01430-9
Liu, J., Zhu, W., Qin, N., Ren, X., Xia, X. (2022b). Propionate and butyrate inhibit biofilm formation of Salmonella Typhimurium grown in laboratory media and food models. Foods 11 (21). doi: 10.3390/foods11213493
Lu, X., Zhang, M., Ma, Y., Li, G., Zhao, X., Qian, W. (2023). Protective effect of Limosilactobacillus reuteri-fermented yogurt on mouse intestinal barrier injury induced by enterotoxigenic Escherichia coli. J. Sci. Food Agric. doi: 10.1002/jsfa.12836
Lukasik, J., Dierikx, T., Besseling-van der Vaart, I., de Meij, T., Szajewska, H., Multispecies Probiotic in, A.A.D.S.G. (2022). Multispecies probiotic for the prevention of antibiotic-associated diarrhea in children: a randomized clinical trial. JAMA Pediatr. 176 (9), 860–866. doi: 10.1001/jamapediatrics.2022.1973
Mancabelli, L., Milani, C., Lugli, G. A., Turroni, F., Mangifesta, M., Viappiani, A., et al. (2017). Unveiling the gut microbiota composition and functionality associated with constipation through metagenomic analyses. Sci. Rep. 7 (1), 9879. doi: 10.1038/s41598-017-10663-w
Maragkoudaki, M., Chouliaras, G., Moutafi, A., Thomas, A., Orfanakou, A., Papadopoulou, A. (2018). Efficacy of an oral rehydration solution enriched with Lactobacillus reuteri DSM 17938 and Zinc in the management of acute diarrhoea in infants: a randomized, double-blind, placebo-controlled trial. Nutrients 10 (9). doi: 10.3390/nu10091189
Maragkoudaki, M., Chouliaras, G., Orel, R., Horvath, A., Szajewska, H., Papadopoulou, A. (2017). Lactobacillus reuteri DSM 17938 and a placebo both significantly reduced symptoms in children with functional abdominal pain. Acta Paediatr. 106 (11), 1857–1862. doi: 10.1111/apa.13992
Mathey, M. D., Pennella, C. L., Zubizarreta, P. (2021). Colorectal carcinoma in children and adolescents. Arch. Argent Pediatr. 119 (5), e487–e498. doi: 10.5546/aap.2021.eng.e487
Mehling, H., Busjahn, A. (2013). Non-viable Lactobacillus reuteri DSMZ 17648 (PylopassTM) as a new approach to Helicobacter pylori control in humans. Nutrients 5 (8), 3062–3073. doi: 10.3390/nu5083062
Meisenheimer Es Md, M. B. A., Epstein, C. D., Thiel D Md, M. P. H. (2022). Acute diarrhea in adults. Am. Fam. Physician 106 (1), 72–80.
Mercuri, M., Stack, D. M., Mantis, I., Moszkowski, R., Field, T. M. (2023). Maternal and infant touching behaviours during perturbed interactions: Associations with maternal depressive symptomatology and infant crying. Infant Behav. Dev. 71, 101821. doi: 10.1016/j.infbeh.2023.101821
Mi, G. L., Zhao, L., Qiao, D. D., Kang, W. Q., Tang, M. Q., Xu, J. K. (2015). Effectiveness of Lactobacillus reuteri in infantile colic and colicky induced maternal depression: a prospective single blind randomized trial. Antonie Van Leeuwenhoek 107 (6), 1547–1553. doi: 10.1007/s10482-015-0448-9
Mobini, R., Tremaroli, V., Stahlman, M., Karlsson, F., Levin, M., Ljungberg, M., et al. (2017). Metabolic effects of Lactobacillus reuteri DSM 17938 in people with type 2 diabetes: a randomized controlled trial. Diabetes Obes. Metab. 19 (4), 579–589. doi: 10.1111/dom.12861
Montgomery, T. L., Eckstrom, K., Lile, K. H., Caldwell, S., Heney, E. R., Lahue, K. G., et al. (2022). Lactobacillus reuteri tryptophan metabolism promotes host susceptibility to CNS autoimmunity. Microbiome 10 (1), 198. doi: 10.1186/s40168-022-01408-7
Moreno Marquez, C., Fernandez Alvarez, P., Valdes Delgado, T., Castro Laria, L., Arguelles Arias, F., Caunedo Alvarez, A., et al. (2022). Randomized, double-blind, placebo-controlled clinical trial on the usefulness of probiotic Lactobacillus reuteri in bismuth-containing quadruple eradication therapy for infection with Helicobacter pylori. Rev. Esp. Enferm. Dig 114 (2), 89–95. doi: 10.17235/reed.2021.7931/2021
Morgan, E., Arnold, M., Gini, A., Lorenzoni, V., Cabasag, C. J., Laversanne, M., et al. (2023). Global burden of colorectal cancer in 2020 and 2040: incidence and mortality estimates from GLOBOCAN. Gut 72 (2), 338–344. doi: 10.1136/gutjnl-2022-327736
Mu, Q., Tavella, V. J., Luo, X. M. (2018). Role of Lactobacillus reuteri in human health and diseases. Front. Microbiol. 9. doi: 10.3389/fmicb.2018.00757
Mulhem, E., Khondoker, F., Kandiah, S. (2022). Constipation in children and adolescents: evaluation and treatment. Am. Fam. Physician 105 (5), 469–478.
Naghibzadeh, N., Salmani, F., Nomiri, S., Tavakoli, T. (2022). Investigating the effect of quadruple therapy with Saccharomyces boulardii or Lactobacillus reuteri strain (DSMZ 17648) supplements on eradication of Helicobacter pylori and treatments adverse effects: a double-blind placebo-controlled randomized clinical trial. BMC Gastroenterol. 22 (1), 107. doi: 10.1186/s12876-022-02187-z
Nishikawa, H., Nakamura, S., Miyazaki, T., Kakimoto, K., Fukunishi, S., Asai, A., et al. (2021). Inflammatory bowel disease and sarcopenia: its mechanism and clinical importance. J. Clin. Med. 10 (18). doi: 10.3390/jcm10184214
Nishino, K., Nishida, A., Inoue, R., Kawada, Y., Ohno, M., Sakai, S., et al. (2018). Analysis of endoscopic brush samples identified mucosa-associated dysbiosis in inflammatory bowel disease. J. Gastroenterol. 53 (1), 95–106. doi: 10.1007/s00535-017-1384-4
Oh, J. K., Vasquez, R., Kim, S. H., Hwang, I. C., Song, J. H., Park, J. H., et al. (2021). Multispecies probiotics alter fecal short-chain fatty acids and lactate levels in weaned pigs by modulating gut microbiota. J. Anim. Sci. Technol. 63 (5), 1142–1158. doi: 10.5187/jast.2021.e94
Ojetti, V., Ianiro, G., Tortora, A., D'Angelo, G., Di Rienzo, T. A., Bibbo, S., et al. (2014). The effect of Lactobacillus reuteri supplementation in adults with chronic functional constipation: a randomized, double-blind, placebo-controlled trial. J. Gastrointest. Liver Dis. 23 (4), 387–391. doi: 10.15403/jgld.2014.1121.234.elr
Ojetti, V., Saviano, A., Brigida, M., Petruzziello, C., Caronna, M., Gayani, G., et al. (2022). Randomized control trial on the efficacy of Limosilactobacillus reuteri ATCC PTA 4659 in reducing inflammatory markers in acute uncomplicated diverticulitis. Eur. J. Gastroenterol. Hepatol. 34 (5), 496–502. doi: 10.1097/MEG.0000000000002342
Oliva, S., Di Nardo, G., Ferrari, F., Mallardo, S., Rossi, P., Patrizi, G., et al. (2012). Randomised clinical trial: the effectiveness of Lactobacillus reuteri ATCC 55730 rectal enema in children with active distal ulcerative colitis. Aliment Pharmacol. Ther. 35 (3), 327–334. doi: 10.1111/j.1365-2036.2011.04939.x
Pang, Y., Ermann Lundberg, L., Mata Forsberg, M., Ahl, D., Bysell, H., Pallin, A., et al. (2022). Extracellular membrane vesicles from Limosilactobacillus reuteri strengthen the intestinal epithelial integrity, modulate cytokine responses and antagonize activation of TRPV1. Front. Microbiol. 13. doi: 10.3389/fmicb.2022.1032202
Partty, A., Kalliomaki, M., Salminen, S., Isolauri, E. (2017). Infantile colic is associated with low-grade systemic inflammation. J. Pediatr. Gastroenterol. Nutr. 64 (5), 691–695. doi: 10.1097/MPG.0000000000001340
Peery, A. F., Crockett, S. D., Murphy, C. C., Jensen, E. T., Kim, H. P., Egberg, M. D., et al. (2022). Burden and cost of gastrointestinal, liver, and pancreatic diseases in the United States: Update 2021. Gastroenterology 162 (2), 621–644. doi: 10.1053/j.gastro.2021.10.017
Pernica, J. M., Steenhoff, A. P., MokOmane, M., Moorad, B., Lechiile, K., Smieja, M., et al. (2017). Rapid enteric testing to permit targeted antimicrobial therapy, with and without Lactobacillus reuteri probiotics, for paediatric acute diarrhoeal disease in Botswana: a pilot, randomized, factorial, controlled trial. PloS One 12 (10), e0185177. doi: 10.1371/journal.pone.0185177
Pernomian, L., Duarte-Silva, M., de Barros Cardoso, C. R. (2020). The aryl hydrocarbon receptor (AHR) as a potential target for the control of intestinal inflammation: insights from an immune and bacteria sensor receptor. Clin. Rev. Allergy Immunol. 59 (3), 382–390. doi: 10.1007/s12016-020-08789-3
Petruzziello, C., Migneco, A., Cardone, S., Covino, M., Saviano, A., Franceschi, F., et al. (2019). Supplementation with Lactobacillus reuteri ATCC PTA 4659 in patients affected by acute uncomplicated diverticulitis: a randomized double-blind placebo controlled trial. Int. J. Colorectal Dis. 34 (6), 1087–1094. doi: 10.1007/s00384-019-03295-1
Piccioni, A., Franza, L., Vaccaro, V., Saviano, A., Zanza, C., Candelli, M., et al. (2021). Microbiota and probiotics: the role of Limosilactobacillus Reuteri in diverticulitis. Medicina (Kaunas) 57 (8). doi: 10.3390/medicina57080802
Poonyam, P., Chotivitayatarakorn, P., Vilaichone, R. K. (2019). High effective of 14-day high-dose PPI- bismuth-containing quadruple therapy with probiotics supplement for Helicobacter pylori eradication: a double blinded-randomized placebo-controlled study. Asian Pac. J. Cancer Prev. 20 (9), 2859–2864. doi: 10.31557/APJCP.2019.20.9.2859
Pourmirzaiee, M. A., Famouri, F., Moazeni, W., Hassanzadeh, A., Hajihashemi, M. (2020). The efficacy of the prenatal administration of Lactobacillus reuteri LR92 DSM 26866 on the prevention of infantile colic: a randomized control trial. Eur. J. Pediatr. 179 (10), 1619–1626. doi: 10.1007/s00431-020-03641-4
Preidis, G. A., Saulnier, D. M., Blutt, S. E., Mistretta, T. A., Riehle, K. P., Major, A. M., et al. (2012). Host response to probiotics determined by nutritional status of rotavirus-infected neonatal mice. J. Pediatr. Gastroenterol. Nutr. 55 (3), 299–307. doi: 10.1097/MPG.0b013e31824d2548
Qin, W., Ren, Z., Xu, C., Cao, Y. N., Sun, M. A., Huang, R., et al. (2023a). Chromatin accessibility and transcriptional landscape during inhibition of Salmonella enterica by Lactobacillus reuteri in IPEC-J2 cells. Cells 12 (6). doi: 10.3390/cells12060968
Qin, W., Xie, Y., Ren, Z., Xu, C., Sun, M. A., Yin, Z., et al. (2023b). Integrative ATAC-seq and RNA-seq analyses of IPEC-J2 cells reveals porcine transcription and chromatin accessibility changes associated with Escherichia coli F18ac inhibited by Lactobacillus reuteri. Front. Microbiol. 14. doi: 10.3389/fmicb.2023.1101111
Quaglio, A. E. V., Grillo, T. G., De Oliveira, E. C. S., Di Stasi, L. C., Sassaki, L. Y. (2022). Gut microbiota, inflammatory bowel disease and colorectal cancer. World J. Gastroenterol. 28 (30), 4053–4060. doi: 10.3748/wjg.v28.i30.4053
Rezasoltani, S., Asadzadeh Aghdaei, H., Dabiri, H., Akhavan Sepahi, A., Modarressi, M. H., Nazemalhosseini Mojarad, E. (2018). The association between fecal microbiota and different types of colorectal polyp as precursors of colorectal cancer. Microb. Pathog. 124, 244–249. doi: 10.1016/j.micpath.2018.08.035
Ricciardiello, L. (2022). Digestive diseases: Big burden, low funding? Results of the new United European gastroenterology white book on digestive diseases. United Eur. Gastroenterol. J. 10 (7), 627–628. doi: 10.1002/ueg2.12297
Riezzo, G., Chimienti, G., Orlando, A., D'Attoma, B., Clemente, C., Russo, F. (2019). Effects of long-term administration of Lactobacillus reuteri DSM-17938 on circulating levels of 5-HT and BDNF in adults with functional constipation. Benef. Microbes 10 (2), 137–147. doi: 10.3920/BM2018.0050
Riezzo, G., Orlando, A., D'Attoma, B., Linsalata, M., Martulli, M., Russo, F. (2018). Randomised double blind placebo controlled trial on Lactobacillus reuteri DSM 17938: improvement in symptoms and bowel habit in functional constipation. Benef. Microbes 9 (1), 51–60. doi: 10.3920/BM2017.0049
Romano, C., Ferrau, V., Cavataio, F., Iacono, G., Spina, M., Lionetti, E., et al. (2014). Lactobacillus reuteri in children with functional abdominal pain (FAP). J. Paediatr. Child Health 50 (10), E68–E71. doi: 10.1111/j.1440-1754.2010.01797.x
Saeed, N. K., Al-Beltagi, M., Bediwy, A. S., El-Sawaf, Y., Toema, O. (2022). Gut microbiota in various childhood disorders: Implication and indications. World J. Gastroenterol. 28 (18), 1875–1901. doi: 10.3748/wjg.v28.i18.1875
Saez, A., Herrero-Fernandez, B., Gomez-Bris, R., Sanchez-Martinez, H., Gonzalez-Granado, J. M. (2023). Pathophysiology of inflammatory bowel disease: innate immune system. Int. J. Mol. Sci. 24 (2). doi: 10.3390/ijms24021526
Sander, L. E., Lorentz, A., Sellge, G., Coeffier, M., Neipp, M., Veres, T., et al. (2006). Selective expression of histamine receptors H1R, H2R, and H4R, but not H3R, in the human intestinal tract. Gut 55 (4), 498–504. doi: 10.1136/gut.2004.061762
Saviano, A., Brigida, M., Migneco, A., Gunawardena, G., Zanza, C., Candelli, M., et al. (2021). Lactobacillus Reuteri DSM 17938 (Limosilactobacillus reuteri) in diarrhea and constipation: two sides of the same coin? Medicina (Kaunas) 57 (7). doi: 10.3390/medicina57070643
Savino, F., Ceratto, S., Poggi, E., Cartosio, M. E., Cordero di Montezemolo, L., Giannattasio, A. (2015). Preventive effects of oral probiotic on infantile colic: a prospective, randomised, blinded, controlled trial using Lactobacillus reuteri DSM 17938. Benef. Microbes 6 (3), 245–251. doi: 10.3920/BM2014.0090
Savino, F., Cordisco, L., Tarasco, V., Calabrese, R., Palumeri, E., Matteuzzi, D. (2009). Molecular identification of coliform bacteria from colicky breastfed infants. Acta Paediatr. 98 (10), 1582–1588. doi: 10.1111/j.1651-2227.2009.01419.x
Savino, F., Cordisco, L., Tarasco, V., Palumeri, E., Calabrese, R., Oggero, R., et al. (2010). Lactobacillus reuteri DSM 17938 in infantile colic: a randomized, double-blind, placebo-controlled trial. Pediatrics 126 (3), e526–e533. doi: 10.1542/peds.2010-0433
Savino, F., Galliano, I., Garro, M., Savino, A., Dapra, V., Montanari, P., et al. (2018a). Regulatory T cells and Toll-like receptor 2 and 4 mRNA expression in infants with colic treated with Lactobacillus reuteri DSM17938. Benef. Microbes 9 (6), 917–925. doi: 10.3920/BM2017.0194
Savino, F., Garro, M., Montanari, P., Galliano, I., Bergallo, M. (2018b). Crying time and RORgamma/FOXP3 expression in Lactobacillus reuteri DSM17938-treated infants with colic: a randomized trial. J. Pediatr. 192, 171–177 e171. doi: 10.1016/j.jpeds.2017.08.062
Savino, F., Pelle, E., Palumeri, E., Oggero, R., Miniero, R. (2007). Lactobacillus reuteri (American type culture collection strain 55730) versus simethicone in the treatment of infantile colic: a prospective randomized study. Pediatrics 119 (1), e124–e130. doi: 10.1542/peds.2006-1222
Savino, F., Quartieri, A., De Marco, A., Garro, M., Amaretti, A., Raimondi, S., et al. (2017). Comparison of formula-fed infants with and without colic revealed significant differences in total bacteria, Enterobacteriaceae and faecal ammonia. Acta Paediatr. 106 (4), 573–578. doi: 10.1111/apa.13642
Schiering, C., Wincent, E., Metidji, A., Iseppon, A., Li, Y., Potocnik, A. J., et al. (2017). Feedback control of AHR signalling regulates intestinal immunity. Nature 542 (7640), 242–245. doi: 10.1038/nature21080
Schnupf, P., Gaboriau-Routhiau, V., Cerf-Bensussan, N. (2018). Modulation of the gut microbiota to improve innate resistance. Curr. Opin. Immunol. 54, 137–144. doi: 10.1016/j.coi.2018.08.003
Sgritta, M., Dooling, S. W., Buffington, S. A., Momin, E. N., Francis, M. B., Britton, R. A., et al. (2019). Mechanisms underlying microbial-mediated changes in social behavior in mouse models of autism spectrum disorder. Neuron 101 (2), 246–259.e246. doi: 10.1016/j.neuron.2018.11.018
Shi, Z., Fultz, R. S., Engevik, M. A., Gao, C., Hall, A., Major, A., et al. (2019). Distinct roles of histamine H1- and H2-receptor signaling pathways in inflammation-associated colonic tumorigenesis. Am. J. Physiol. Gastrointest. Liver Physiol. 316 (1), G205–G216. doi: 10.1152/ajpgi.00212.2018
Shornikova, A. V., Casas, I. A., Isolauri, E., Mykkanen, H., Vesikari, T. (1997a). Lactobacillus reuteri as a therapeutic agent in acute diarrhea in young children. J. Pediatr. Gastroenterol. Nutr. 24 (4), 399–404. doi: 10.1097/00005176-199704000-00008
Shornikova, A. V., Casas, I. A., Mykkanen, H., Salo, E., Vesikari, T. (1997b). Bacteriotherapy with Lactobacillus reuteri in rotavirus gastroenteritis. Pediatr. Infect. Dis. J. 16 (12), 1103–1107. doi: 10.1097/00006454-199712000-00002
Shulpekova, Y. O., Nechaev, V. M., Popova, I. R., Deeva, T. A., Kopylov, A. T., Malsagova, K. A., et al. (2021). Food intolerance: the role of histamine. Nutrients 13 (9). doi: 10.3390/nu13093207
Sitkin, S., Lazebnik, L., Avalueva, E., Kononova, S., Vakhitov, T. (2022). Gastrointestinal microbiome and Helicobacter pylori: eradicate, leave it as it is, or take a personalized benefit-risk approach? World J. Gastroenterol. 28 (7), 766–774. doi: 10.3748/wjg.v28.i7.766
Skrzydlo-Radomanska, B., Prozorow-Krol, B., Cichoz-Lach, H., Majsiak, E., Bierla, J. B., Kosikowski, W., et al. (2020). The effectiveness of synbiotic preparation containing Lactobacillus and Bifidobacterium probiotic strains and short chain fructooligosaccharides in patients with diarrhea predominant irritable bowel syndrome-a randomized double-blind, placebo-controlled study. Nutrients 12 (7). doi: 10.3390/nu12071999
Smith, P. M., Howitt, M. R., Panikov, N., Michaud, M., Gallini, C. A., Bohlooly, Y. M., et al. (2013). The microbial metabolites, short-chain fatty acids, regulate colonic Treg cell homeostasis. Science 341 (6145), 569–573. doi: 10.1126/science.1241165
Smythe, P., Efthimiou, G. (2022). In silico genomic and metabolic atlas of Limosilactobacillus reuteri DSM 20016: an Insight into human health. Microorganisms 10 (7). doi: 10.3390/microorganisms10071341
Socala, K., Doboszewska, U., Szopa, A., Serefko, A., Wlodarczyk, M., Zielinska, A., et al. (2021). The role of microbiota-gut-brain axis in neuropsychiatric and neurological disorders. Pharmacol. Res. 172, 105840. doi: 10.1016/j.phrs.2021.105840
Sommermeyer, H., Bernatek, M., Pszczola, M., Krauss, H., Piatek, J. (2022). Supporting the diagnosis of infantile colic by a point of care measurement of fecal calprotectin. Front. Pediatr. 10. doi: 10.3389/fped.2022.978545
Sun, M. C., Zhang, F. C., Yin, X., Cheng, B. J., Zhao, C. H., Wang, Y. L., et al. (2018). Lactobacillus reuteri F-9-35 prevents DSS-induced colitis by inhibiting proinflammatory gene expression and restoring the gut microbiota in mice. J. Food Sci. 83 (10), 2645–2652. doi: 10.1111/1750-3841.14326
Sung, V., Hiscock, H., Tang, M. L., Mensah, F. K., Nation, M. L., Satzke, C., et al. (2014). Treating infant colic with the probiotic Lactobacillus reuteri: double blind, placebo controlled randomised trial. BMJ 348, g2107. doi: 10.1136/bmj.g2107
Szajewska, H., Gyrczuk, E., Horvath, A. (2013). Lactobacillus reuteri DSM 17938 for the management of infantile colic in breastfed infants: a randomized, double-blind, placebo-controlled trial. J. Pediatr. 162 (2), 257–262. doi: 10.1016/j.jpeds.2012.08.004
Szymanski, H., Szajewska, H. (2019). Lack of efficacy of Lactobacillus reuteri DSM 17938 for the treatment of acute gastroenteritis: a randomized controlled trial. Pediatr. Infect. Dis. J. 38 (10), e237–e242. doi: 10.1097/INF.0000000000002355
Tezuka, H., Ohteki, T. (2019). Regulation of IgA production by intestinal dendritic cells and related cells. Front. Immunol. 10. doi: 10.3389/fimmu.2019.01891
Thapar, N., Benninga, M. A., Crowell, M. D., Di Lorenzo, C., Mack, I., Nurko, S., et al. (2020). Paediatric functional abdominal pain disorders. Nat. Rev. Dis. Primers 6 (1), 89. doi: 10.1038/s41572-020-00222-5
Thomas, C. M., Hong, T., van Pijkeren, J. P., Hemarajata, P., Trinh, D. V., Hu, W., et al. (2012). Histamine derived from probiotic Lactobacillus reuteri suppresses TNF via modulation of PKA and ERK signaling. PloS One 7 (2), e31951. doi: 10.1371/journal.pone.0031951
Tiberio, L., Del Prete, A., Schioppa, T., Sozio, F., Bosisio, D., Sozzani, S. (2018). Chemokine and chemotactic signals in dendritic cell migration. Cell Mol. Immunol. 15 (4), 346–352. doi: 10.1038/s41423-018-0005-3
Trivic, I., Niseteo, T., Jadresin, O., Hojsak, I. (2021). Use of probiotics in the treatment of functional abdominal pain in children-systematic review and meta-analysis. Eur. J. Pediatr. 180 (2), 339–351. doi: 10.1007/s00431-020-03809-y
Turco, R., Russo, M., Bruzzese, D., Staiano, A. (2021). Efficacy of a partially hydrolysed formula, with reduced lactose content and with Lactobacillus reuteri DSM 17938 in infant colic: a double blind, randomised clinical trial. Clin. Nutr. 40 (2), 412–419. doi: 10.1016/j.clnu.2020.05.048
Urbanska, M., Gieruszczak-Bialek, D., Szymanski, H., Szajewska, H. (2016). Effectiveness of Lactobacillus reuteri DSM 17938 for the prevention of nosocomial diarrhea in children: a randomized, double-blind, placebo-controlled trial. Pediatr. Infect. Dis. J. 35 (2), 142–145. doi: 10.1097/INF.0000000000000948
Urrutia-Baca, V. H., Escamilla-Garcia, E., de la Garza-Ramos, M. A., Tamez-Guerra, P., Gomez-Flores, R., Urbina-Rios, C. S. (2018). In vitro antimicrobial activity and downregulation of virulence gene expression on Helicobacter pylori by reuterin. Probiotics Antimicrob. Proteins 10 (2), 168–175. doi: 10.1007/s12602-017-9342-2
Van den Abbeele, P., Goggans, M., Deyaert, S., Baudot, A., Van de Vliet, M., Calatayud Arroyo, M., et al. (2023). Lacticaseibacillus rhamnosus ATCC 53103 and Limosilactobacillus reuteri ATCC 53608 synergistically boost butyrate levels upon tributyrin administration ex vivo. Int. J. Mol. Sci. 24 (6). doi: 10.3390/ijms24065859
Vester-Andersen, M. K., Mirsepasi-Lauridsen, H. C., Prosberg, M. V., Mortensen, C. O., Trager, C., Skovsen, K., et al. (2019). Increased abundance of proteobacteria in aggressive Crohn's disease seven years after diagnosis. Sci. Rep. 9 (1), 13473. doi: 10.1038/s41598-019-49833-3
Vitale, I., Spano, M., Puca, V., Carradori, S., Cesa, S., Marinacci, B., et al. (2023). Antibiofilm activity and NMR-based metabolomic characterization of cell-free supernatant of Limosilactobacillus reuteri DSM 17938. Front. Microbiol. 14. doi: 10.3389/fmicb.2023.1128275
Walsham, A. D., MacKenzie, D. A., Cook, V., Wemyss-Holden, S., Hews, C. L., Juge, N., et al. (2016). Lactobacillus reuteri inhibition of enteropathogenic Escherichia coli adherence to human intestinal epithelium. Front. Microbiol. 7. doi: 10.3389/fmicb.2016.00244
Wang, W., Ma, H., Zhu, Y., Ni, K., Qin, G., Tan, Z., et al. (2021b). Screening of lactic acid bacteria with inhibitory activity against ETEC K88 as feed additive and the effects on sows and piglets. Anim. (Basel) 11 (6). doi: 10.3390/ani11061719
Wang, R., Tang, R., Li, B., Ma, X., Schnabl, B., Tilg, H. (2021a). Gut microbiome, liver immunology, and liver diseases. Cell Mol. Immunol. 18 (1), 4–17. doi: 10.1038/s41423-020-00592-6
Wang, T., Teng, K., Liu, G., Liu, Y., Zhang, J., Zhang, X., et al. (2018). Lactobacillus reuteri HCM2 protects mice against enterotoxigenic Escherichia coli through modulation of gut microbiota. Sci. Rep. 8 (1), 17485. doi: 10.1038/s41598-018-35702-y
Wang, T., Zheng, N., Luo, Q., Jiang, L., He, B., Yuan, X., et al. (2019). Probiotics Lactobacillus reuteri abrogates immune checkpoint blockade-associated colitis by inhibiting group 3 innate lymphoid cells. Front. Immunol. 10. doi: 10.3389/fimmu.2019.01235
Wanke, M., Szajewska, H. (2012). Lack of an effect of Lactobacillus reuteri DSM 17938 in preventing nosocomial diarrhea in children: a randomized, double-blind, placebo-controlled trial. J. Pediatr. 161 (1), 40–43.e41. doi: 10.1016/j.jpeds.2011.12.049
Wegner, A., Banaszkiewicz, A., Kierkus, J., Landowski, P., Korlatowicz-Bilar, A., Wiecek, S., et al. (2018). The effectiveness of Lactobacillus reuteri DSM 17938 as an adjunct to macrogol in the treatment of functional constipation in children. A randomized, double-blind, placebo-controlled, multicentre trial. Clin. Res. Hepatol. Gastroenterol. 42 (5), 494–500. doi: 10.1016/j.clinre.2018.03.008
Wei, W., Mu, S., Han, Y., Chen, Y., Kuang, Z., Wu, X., et al. (2022). Gpr174 knockout alleviates DSS-induced colitis via regulating the immune function of dendritic cells. Front. Immunol. 13. doi: 10.3389/fimmu.2022.841254
Weizman, Z., Abu-Abed, J., Binsztok, M. (2016). Lactobacillus reuteri DSM 17938 for the management of functional abdominal pain in childhood: a randomized, double-blind, placebo-controlled trial. J. Pediatr. 174, 160–164.e161. doi: 10.1016/j.jpeds.2016.04.003
Wen, S., He, L., Zhong, Z., Zhao, R., Weng, S., Mi, H., et al. (2021). Stigmasterol restores the balance of Treg/Th17 cells by activating the butyrate-PPARgamma axis in colitis. Front. Immunol. 12. doi: 10.3389/fimmu.2021.741934
Werlinger, P., Nguyen, H. T., Gu, M., Cho, J. H., Cheng, J., Suh, J. W. (2022). Lactobacillus reuteri MJM60668 prevent progression of non-alcoholic fatty liver disease through anti-adipogenesis and anti-inflammatory pathway. Microorganisms 10 (11). doi: 10.3390/microorganisms10112203
Wu, R. Y., Pasyk, M., Wang, B., Forsythe, P., Bienenstock, J., Mao, Y. K., et al. (2013). Spatiotemporal maps reveal regional differences in the effects on gut motility for Lactobacillus reuteri and rhamnosus strains. Neurogastroenterol. Motil. 25 (3), e205–e214. doi: 10.1111/nmo.12072
Wu, H., Xie, S., Miao, J., Li, Y., Wang, Z., Wang, M., et al. (2020). Lactobacillus reuteri maintains intestinal epithelial regeneration and repairs damaged intestinal mucosa. Gut Microbes 11 (4), 997–1014. doi: 10.1080/19490976.2020.1734423
Xu, Y., Ding, X., Wang, Y., Li, D., Xie, L., Liang, S., et al. (2022). Bacterial metabolite reuterin attenuated LPS-induced oxidative stress and inflammation response in HD11 macrophages. Antioxid. (Basel) 11 (9). doi: 10.3390/antiox11091662
Yang, C., Liang, L., Lv, P., Liu, L., Wang, S., Wang, Z., et al. (2021). Effects of non-viable Lactobacillus reuteri combining with 14-day standard triple therapy on Helicobacter pylori eradication: A randomized double-blind placebo-controlled trial. Helicobacter 26 (6), e12856. doi: 10.1111/hel.12856
Yang, J., Wang, C., Liu, L., Zhang, M. (2020). Lactobacillus reuteri KT260178 supplementation reduced morbidity of piglets through its targeted colonization, improvement of cecal microbiota profile, and immune functions. Probiotics Antimicrob. Proteins 12 (1), 194–203. doi: 10.1007/s12602-019-9514-3
Yi, H., Wang, L., Xiong, Y., Wang, Z., Qiu, Y., Wen, X., et al. (2018). Lactobacillus reuteri LR1 improved expression of genes of tight junction proteins via the MLCK pathway in IPEC-1 Cells during infection with enterotoxigenic Escherichia coli K88. Mediators Inflammation 2018, 6434910. doi: 10.1155/2018/6434910
Yu, Z., Chen, J., Liu, Y., Meng, Q., Liu, H., Yao, Q., et al. (2023b). The role of potential probiotic strains Lactobacillus reuteri in various intestinal diseases: New roles for an old player. Front. Microbiol. 14. doi: 10.3389/fmicb.2023.1095555
Yu, T., Ding, Y., Qian, D., Lin, L., Tang, Y. (2023a). Characteristics of fecal microbiota in different constipation subtypes and association with colon physiology, lifestyle factors, and psychological status. Therap. Adv. Gastroenterol. 16, 17562848231154101. doi: 10.1177/17562848231154101
Zeevenhooven, J., Browne, P. D., L'Hoir, M. P., de Weerth, C., Benninga, M. A. (2018). Infant colic: mechanisms and management. Nat. Rev. Gastroenterol. Hepatol. 15 (8), 479–496. doi: 10.1038/s41575-018-0008-7
Zelante, T., Iannitti, R. G., Cunha, C., De Luca, A., Giovannini, G., Pieraccini, G., et al. (2013). Tryptophan catabolites from microbiota engage aryl hydrocarbon receptor and balance mucosal reactivity via interleukin-22. Immunity 39 (2), 372–385. doi: 10.1016/j.immuni.2013.08.003
Zhang, S., Wang, R., Li, D., Zhao, L., Zhu, L. (2021). Role of gut microbiota in functional constipation. Gastroenterol. Rep. (Oxf) 9 (5), 392–401. doi: 10.1093/gastro/goab035
Zhang, Y., Wang, X. L., Zhou, M., Kang, C., Lang, H. D., Chen, M. T., et al. (2018). Crosstalk between gut microbiota and sirtuin-3 in colonic inflammation and tumorigenesis. Exp. Mol. Med. 50 (4), 1–11. doi: 10.1038/s12276-017-0002-0
Zhao, T., Wang, H., Liu, Z., Liu, Y., De, J., Li, B., et al. (2023). Recent perspective of Lactobacillus in reducing oxidative stress to prevent disease. Antioxid. (Basel) 12 (3). doi: 10.3390/antiox12030769
Zheng, T. X., Pu, S. L., Tan, P., Du, Y. C., Qian, B. L., Chen, H., et al. (2020). Liver metabolomics reveals the effect of Lactobacillus reuteri on alcoholic liver disease. Front. Physiol. 11. doi: 10.3389/fphys.2020.595382
Zhou, Q., Wu, F., Chen, S., Cen, P., Yang, Q., Guan, J., et al. (2022b). Lactobacillus reuteri improves function of the intestinal barrier in rats with acute liver failure through Nrf-2/HO-1 pathway. Nutrition 99-100, 111673. doi: 10.1016/j.nut.2022.111673
Zhou, L., Zeng, Y., Zhang, H., Ma, Y. (2022a). The role of gastrointestinal microbiota in functional dyspepsia: A Review. Front. Physiol. 13. doi: 10.3389/fphys.2022.910568
Zhu, L., Liu, W., Alkhouri, R., Baker, R. D., Bard, J. E., Quigley, E. M., et al. (2014). Structural changes in the gut microbiome of constipated patients. Physiol. Genomics 46 (18), 679–686. doi: 10.1152/physiolgenomics.00082.2014
Zong, M., Chang, C., Anjum, R., Xu, H., Guo, Y., Pan, D., et al. (2023). Multifunctional LPxTG-motif surface protein derived from Limosilactobacillus reuteri SH 23 in DSS-induced ulcerative colitis of mice. FASEB J. 37 (5), e22895. doi: 10.1096/fj.2022-0252-RR
Keywords: Lactobacillus reuteri, gut microbiota, infantile colic, diarrhea, constipation, functional abdominal pain, inflammatory bowel disease, colorectal cancer
Citation: Peng Y, Ma Y, Luo Z, Jiang Y, Xu Z and Yu R (2023) Lactobacillus reuteri in digestive system diseases: focus on clinical trials and mechanisms. Front. Cell. Infect. Microbiol. 13:1254198. doi: 10.3389/fcimb.2023.1254198
Received: 06 July 2023; Accepted: 07 August 2023;
Published: 18 August 2023.
Edited by:
Tingtao Chen, Nanchang University, ChinaReviewed by:
Li Huang, Southern Medical University, ChinaChen Wang, Southwest University, China
Gang Hu, Nanchang University, China
Copyright © 2023 Peng, Ma, Luo, Jiang, Xu and Yu. This is an open-access article distributed under the terms of the Creative Commons Attribution License (CC BY). The use, distribution or reproduction in other forums is permitted, provided the original author(s) and the copyright owner(s) are credited and that the original publication in this journal is cited, in accordance with accepted academic practice. No use, distribution or reproduction is permitted which does not comply with these terms.
*Correspondence: Zhimin Xu, eHV6aGltaW5AemhrdS5lZHUuY24=; Renqiang Yu, eXVyZW5xaWFuZzU1M0AxNjMuY29t
†These authors have contributed equally to this work and share first authorship