- 1Applied Conservation and Ecological Research Lab, Biology Department, William & Mary, Williamsburg, VA, United States
- 2Applied Conservation Lab, Biology Department, Millersville University, Millersville, PA, United States
Our planet is home to an incredible array of species; however, relatively few studies have compared how anthropogenic threats impact taxonomic groups over time. Our objective was to identify temporal trends in threats facing the four most speciose phyla protected by the United States Endangered Species Act: angiosperms, arthropods, chordates, and mollusks. We determined presence or absence of threats for each species in these phyla by reviewing Final Rule listing decisions. For each phylum, we evaluated whether there was a linear, quadratic, or pseudo-threshold association between year of listing and the presence of 24 anthropogenic threats. We identified temporal trends for 80% of the 96 threat-phylum combinations. We classified threats as topmost (probability of being included in a species' listing decision peaking at ≥ 0.81) and escalating (probability of being included in a listing decision increasing by ≥ 0.81 between a species' first and most recent years of listing). Angiosperms, arthropods, and mollusks each had more topmost and escalating threats than chordates. Percentages of topmost threats were 42.9% (N = 21) for mollusks, 36.4% (N = 22) for angiosperms, and 33.3% (N = 21) for arthropods. Percentages of escalating threats were 22.7% (N = 22) for angiosperms and 14.3% (N = 21) for arthropods and mollusks. In contrast, percentages of topmost and escalating threats were only 4.2% (N = 24) for chordates, this one threat being climate change. Our research suggests potential conservation successes; some overutilization and pollution threats showed only gradually increasing or declining trends for certain phyla. We identified authorized take impacting angiosperms as the sole threat-phylum combination for which the threat had been consistently decreasing since the phylum's first year of listing. Conversely, species interactions, environmental stochasticity, and demographic stochasticity threats have seen drastic increases across all phyla; we suggest conservation efforts focus on these areas of increasing concern. We also recommend that resources be allocated to phyla with numerous topmost and escalating threats, not just to chordates.
Introduction
Our planet harbors a rich suite of species, each with unique life histories and habitat requirements. Given this wide variation, it is to be expected that anthropogenic changes to the environment do not impact each species in the same manner (Leclerc et al., 2018). However, current research focuses on evaluating threats at the biodiversity level rather than accounting for variability among taxa. Additionally, the “charismatic” endothermic vertebrates have been studied far more thoroughly than other species; this information gap makes it difficult to assess relative extinction risk among taxa (McKinney, 1999). Because of the alarming rate at which extinctions are occurring (Barnosky et al., 2011; Pimm et al., 2014), it is important to identify how factors of endangerment differ among taxa (Ducatez and Shine, 2017). Doing so will help to ensure that extant biodiversity can be preserved, maintained, and recovered.
In response to accelerating biodiversity losses, several nations have enacted laws with the purpose of protecting the imperiled species within their countries, such as the Endangered Species Protection Act (1992) in Australia, the Species at Risk Act (2002) in Canada, the Conservation Act (1987) in New Zealand, and the Wildlife and Countryside Act (1981) in the United Kingdom. In the United States, the legislation tasked with mitigating biodiversity loss is the Endangered Species Act (ESA) (1973). Signed into law in 1973, its aim has been to list imperiled species as “Threatened” or “Endangered” and subsequently provide regulations and resources to promote their recovery. As with conservation efforts in general, there are several taxa-based differences in how the ESA has been implemented. The first of these is reflected in the taxonomic composition of species protected by the ESA. Only 20% of the U.S. species NatureServe considered to be imperiled have been listed under the ESA; while birds, mammals, and reptiles were well-represented, there were far fewer protected amphibians, fishes, invertebrates, and plants (Evans et al., 2016). A second difference is apparent in the distribution of resources. Over 60% of the funding allotted for all listed species in 2012 went to fishes, primarily the economically important salmon and sturgeon species which are harvested despite their imperiled status (Evans et al., 2016). Conversely, plants, which comprised over half of all listed species, only received 3% of 2012's total funds (Evans et al., 2016). Taxa also plays a role in how quickly a species is listed under the ESA; plant and invertebrate species had a longer wait between their first consideration to be protected and their final listing compared to vertebrates (Puckett et al., 2016). Haines et al. (2021) found that both the amount of financial support a species received and the length of time it had been listed were important factors in its recovery. Accordingly, only 26% of the species that had been delisted due to recovery as of January 2020 were plants and invertebrates, even though these taxa comprised 72% of all listed species (Haines et al., 2021).
In addition to these taxonomic differences in ESA implementation, there is also variation among the stressors which impact taxa. Leidner and Neel (2011) found that a larger percentage of plants had extirpations without range reductions, while a larger percentage of vertebrates had range reductions without extirpations; important distinctions to identify for effective management. In addition, Wilcove et al. (1998) examined differences in anthropogenic threats to ESA listed species and found that: 1) broad-scale habitat modification threats impacted several taxonomic groups, 2) alien species affected birds, fishes, and plants more than other animal groups, and 3) pollution was one of the most prevalent threats for fishes, mussels, and crayfish, while this threat had a lesser impact on non-aquatic species. While this research has provided an important benchmark, there have been 22 additional years of species listings since its publication during which new trends may have emerged.
Given that taxa are treated differently in the implementation of the ESA and that they are susceptible to different threats, it is important to consider taxonomic differences when analyzing factors of species endangerment. Doing so could improve conservation efforts by allowing recovery strategies to be more effective for newly listed species (Foin et al., 1998). In addition to taxonomic differences, understanding temporal variation of threats is also valuable to conservation efforts. Threats that have been increasing in prevalence should be the focus of resources to mitigate their impacts, while threats that have been decreasing in prevalence may provide insights on management strategies which have proven successful for certain threats and/or taxa. This temporal aspect is infrequently analyzed, with many studies considering threats in just one instance in time. Lack of information on temporal dynamics of threats makes them less likely to be properly addressed by recovery efforts (Lawler et al., 2002). Our objective was to identify and compare most prevalent threats and to evaluate temporal trends in the threats which impact taxa listed as Threatened and Endangered under the ESA.
Materials and Methods
Threatened and Endangered Species Analyzed
We included every species listed as Threatened or Endangered by the U.S Fish and Wildlife Service (USFWS) or National Oceanic and Atmospheric Administration's National Marine Fisheries Service (NMFS) in our analysis. Species that were delisted due to recovery or extinction were included, while species that were delisted due to original errors in the listing decision, revisions in taxonomy, or discovery of new information were omitted. We did not include species which were listed due to “similarity of appearance” to an already listed Threatened or Endangered species. Each Distinct Population Segment (DPS) was treated as its own species, as delineated in the ESA's definition of “species” [ESA section 3(16)]. We did not include foreign species (found only in areas outside of U.S. jurisdiction) listed under the ESA, as the U.S. holds no regulatory power for those species beyond trade restrictions. Final Rule documents of species listed prior to 1975 did not contain information on threats facing the species, so we only included species listed 1975–2020. Our sample size was 1,569 species.
Taxonomic Groups
As of 2020, the taxonomic groups represented by species listed under the ESA included amphibians, arachnids, birds, cephalopods, clams, conifers & cycads, corals, crustaceans, ferns & allies, fish, flowering plants, insects, lichen, mammals, and reptiles. These groups had widely varying sample sizes, ranging from 910 flowering plants to a single cephalopod. Because many of these taxa lacked sufficient replication, we broadened our resolution to the phylum level. Members of eight phyla were protected by the ESA as of 2020: angiosperms (flowering plants; N = 910), arthropods (arachnids, crustaceans, and insects; N = 128), ascomycotans (lichen; N = 2), chordates (amphibians, birds, fish, mammals, and reptiles; N = 350), cnidarians (corals; N = 13), gymnosperms (conifers & cycads; N = 4), mollusks (cephalopods, clams, and snails; N = 124), and pteridophytes (ferns & allies; N = 38).
Threat Data Collection
We used Final Rule listing decisions as the source of our threat data collection. These documents are published in the U.S. Federal Register when a species is listed under the ESA, providing justification for why the species needs federal resources. In instances when a Final Rule was missing or incomplete, we reviewed the Proposed Rule for listing. These documents were accessed through the USFWS's Environmental Conservation Online System (ECOS; ecos.fws.gov) and the United States Federal Register (federalregister.gov). Within each document, we focused on parts A, B, C, and E of the “Summary of Factors Affecting the Species” section as well as the “Determination” section if present. We recorded any threats facing the species from these sections if the threats were (1) affecting the species at the time of its listing (i.e., not historical threats) and (2) written with “certain,” not “potential” language. Refer to Leu et al. (2019) for further details of threat language collection protocols.
We sorted the threat language collected from the species' listing documents into 147 preliminary threat categories (Appendix 1). Upon review of the types of threats frequently encountered in the listing decisions, we grouped related threats into 24 fine-resolution categories for analysis. Refer to Appendix 1 for the preliminary threats included in each fine-resolution threat category and the number of species in each phylum they impacted.
Statistical Analysis
We used general linear models (GLM) with a binomial error structure and logit link to relate year of listing to the probability that a threat impacted a given phylum at time of listing. We constructed three predictor models per threat-phylum combination, each with a different form of year: linear, quadratic, and pseudo-threshold (log year; Scherer et al., 2012). For each threat-phylum combination, we also included a null model (no year effect). The best model out of each set of four was selected based on Akaike information criterion adjusted for small sample sizes (AICc; Burnham and Anderson, 2002; Appendices 2, 3), which we calculated using the MuMIn package (version 1.43.17; Barton, 2020). If a simpler model (with fewer terms) was within 2 AICc of the best performing model, we selected the simpler model (Burnham and Anderson, 2002). If the best-fitting model was within 2 AICc of the null model (Burnham and Anderson, 2002), we determined that year did not explain temporal variation in that threat-phylum combination. We used R (version 3.6.2; R Core Team, 2019) to perform all analyses.
To highlight important threats, we classified them into two categories. First, we defined threats as topmost if their probability of being included in a phylum's listing decision peaked at ≥ 0.81. Second, we defined threats as escalating if their probability of being included in a phylum's listing decision increased by ≥ 0.81 between their first and most recent years of listing.
Results
Our analysis was based on 24 fine-resolution threats evaluated across four phyla. We combined the majority of our 147 preliminary threats into these fine-resolution categories, but eliminated nine preliminary threats because they were too vague (Appendix 1). Leu et al. (2019) had already examined temporal trends among broad-resolution threats, so no value would be added by including them in our analysis. In addition, we omitted five threats due to small sample sizes (representation in analyzed phyla) and/or because they did not fit into any of our categories (Appendix 1). The phyla impacted by these five threats were predominantly chordates (offshore development, light pollution, noise pollution, and unfavorable environmental conditions) and angiosperms (unfavorable environmental conditions and volcanoes; Appendix 1). We also omitted four phyla with sample sizes too small for our analysis: cnidarians, ascomycotans, gymnosperms, and pteridophytes. The latter three phyla were faced predominantly by the same threats that impacted the angiosperms. The cnidarians, however, faced a unique set of threats. All 13 listed coral species were impacted by offshore development and sea level rise, and 11 were impacted by ocean acidification - all threats which were relatively rare among the other phyla. These four omitted phyla constituted only 57 (3.6%) of the 1,569 listed species we considered in this study.
We found that the 24 fine-resolution threats did not impact the phyla equally (Figure 1). For angiosperms, arthropods, and mollusks, both species interactions threats, two out of the three environmental stochasticity threats, and all three of the demographic stochasticity threats were topmost threats. Mollusks had the greatest number of topmost threats (42.9%, N = 21), followed by angiosperms (36.4%, N = 22) and arthropods (30%, N = 21; Figure 1). For chordates, the only topmost and escalating threat was climate change (Figures 1, 2). The arthropods and mollusks each had three escalating threats, whereas angiosperms had five (Figure 2). However, angiosperms were also the only phylum for which threat probabilities had decreased in intensity: mining and oil/gas by 0.05 and authorized take by a more substantial 0.25. The latter was the only threat we identified to have been constantly decreasing through time (Figure 2). We also found differences among the phyla when considering broad-resolution threat categories of habitat modification, overutilization, pollution, species interactions, environmental stochasticity, and demographic stochasticity (Figures 1, 2).
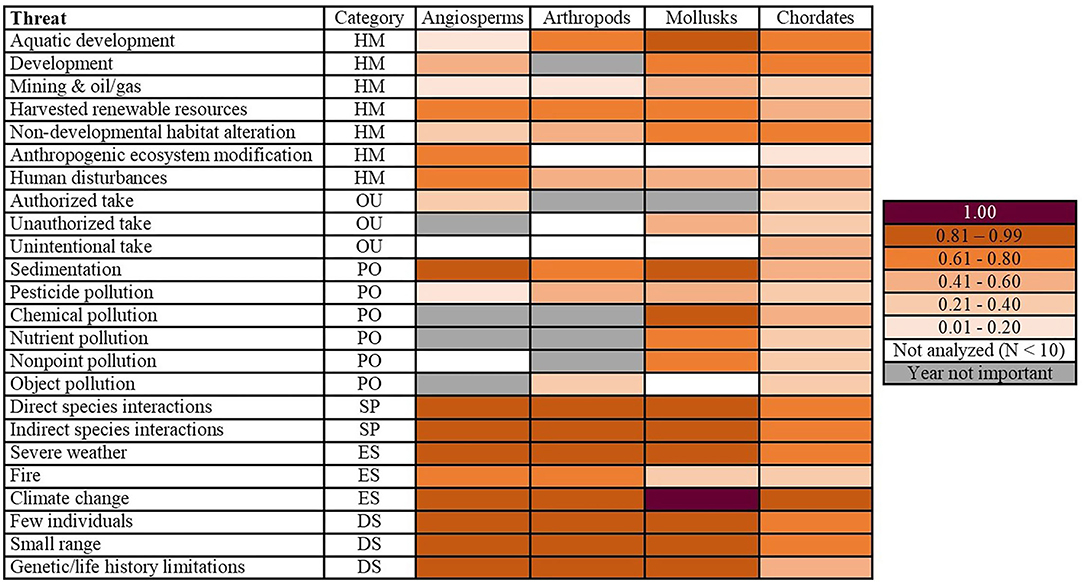
Figure 1. Peak probabilities of being impacted by a threat. Mollusks had the greatest number of topmost threats (peaking at ≥ 0.81). Category corresponds to the broad-resolution threats of habitat modification (HM), overutilization (OU), pollution (PO), species interactions (SP), environmental stochasticity (ES), and demographic stochasticity (DS). Year not important denotes that there was no year effect for a particular threat/phyla combination (the null model was the best fit). Not analyzed (N < 10) is noted for phyla for which a given threat was not analyzed due to insufficient sample size. The 1.00 probability indicates we were unable to model the data because of perfect fit.
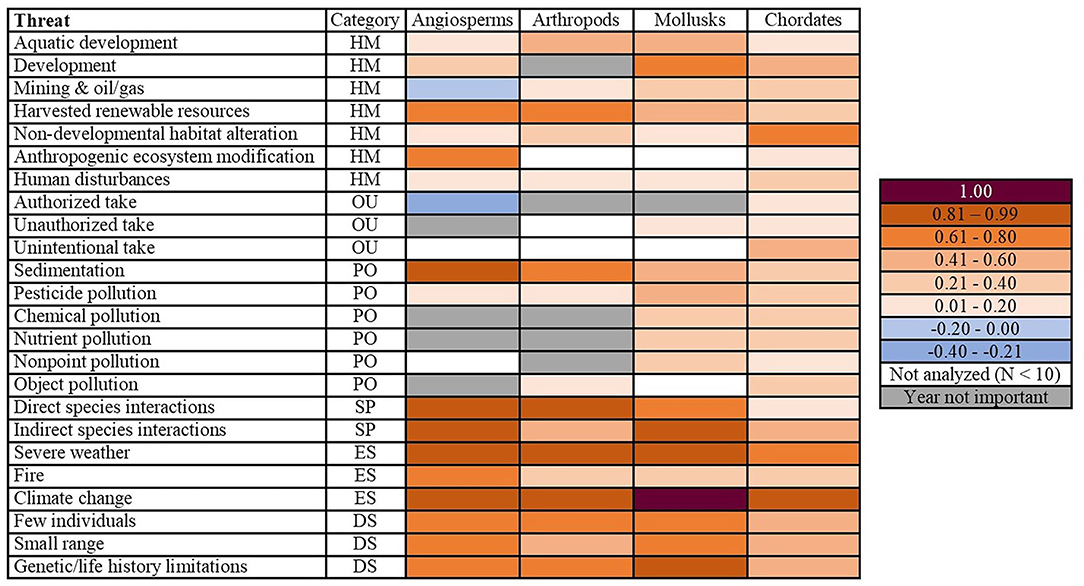
Figure 2. Differences in probabilities of a threat impacting a phylum between their first and most recent years of listing. Angiosperms had both the greatest number of escalating threats (increased by ≥ 0.81) and the only threats which had decreased in probability. Category corresponds to the broad-resolution threats of habitat modification (HM), overutilization (OU), pollution (PO), species interactions (SP), environmental stochasticity (ES), and demographic stochasticity (DS). Year not important denotes that there was no year effect for a particular threat/phyla combination (the null model was the best fit). Not analyzed (N < 10) is noted for phyla for which a given threat was not analyzed due to insufficient sample size. The 1.00 probability indicates we were unable to model the data because of perfect fit.
Habitat Modification
Seven threats fell within the category of habitat modification: human disturbances, harvested renewable resources, aquatic development, mining & oil/gas, non-developmental habitat alteration, development, and anthropogenic ecosystem modification (for threat classifications see Appendix 1). In all phyla, year related to each of these threats (Figure 3; Appendix 4), except for arthropods for development (null model) and arthropods and mollusks for anthropogenic ecosystem modification (N < 10).
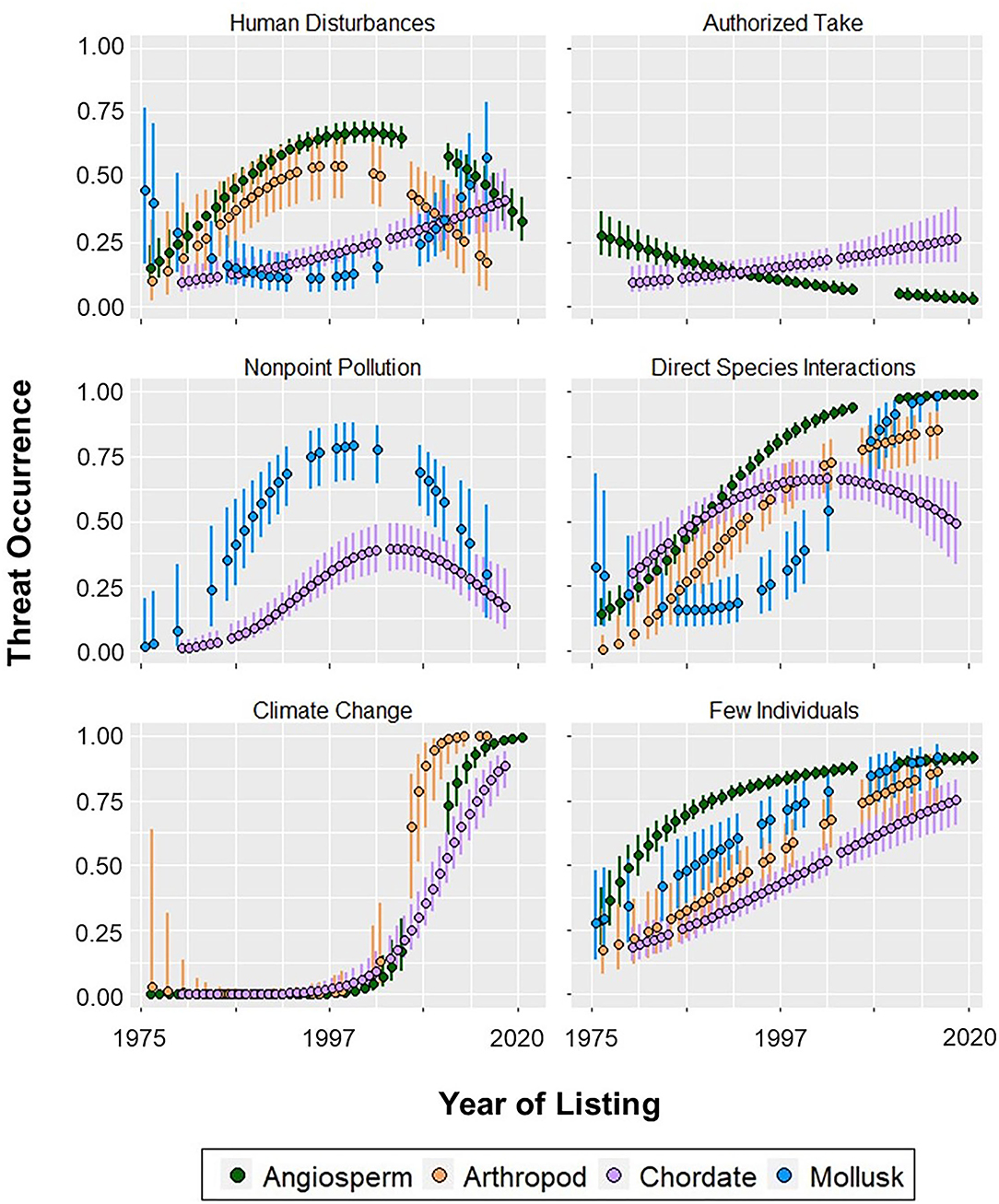
Figure 3. Probability (±95% CI) of threats impacting angiosperm, arthropod, chordate, and mollusk phyla at the time of their listing. Year of listing was scaled and centered for the models.
Of all the threat categories considered in this study, the only one for which a phylum showed a convex quadratic trend over all their years of listing was human disturbances (Figure 3). The probability that mollusks were impacted by this threat was 0.45 in 1977, decreased to 0.11 in 1997, and increased to 0.58 in 2018 (Figure 3; Appendix 5). Aquatic development impacting mollusks was the only topmost habitat modification threat (Figure 1). There were no escalating threats in the habitat modification category.
While it had always had a relatively low magnitude, the probability of mining & oil/gas affecting angiosperms decreased between their first and most recent years of listing (Figure 2; Appendix 6). This was one of only two threats in our study where we documented a net decrease in the probability of a threat impacting a phylum, and the only habitat modification threat to do so.
Overutilization
Overutilization encompasses three of our fine-resolution threats: authorized take, unauthorized take, and unintentional take (see Appendix 1 for threat classifications). Overall, these were the least frequently occurring threats in our analysis (Figure 3; Appendix 7). Overutilization was also the threat category with the greatest number of phyla omissions due to small sample size (33%) and was one of two categories with the greatest proportion of models for which year was not a predictor of threat occurrence (25%).
Chordates were the only phylum to be impacted by all three overutilization threats. The probability that chordates were affected by these threats increased between 0.10 and 0.45 since their first year of listing (Appendix 8). Conversely, the probability of authorized take affecting angiosperms decreased from 0.28 in 1977 to 0.03 in 2017 (Figure 3). Notably, this was the only threat-phylum combination for which the threat peaked in the earliest year of listing and constantly decreased thereafter. We also identified one overutilization threat for which year of listing was a predictor for mollusks: unauthorized take, which peaked in 2000 (Appendices 4, 5). Year of listing was not a predictor of any overutilization threats facing arthropods.
Pollution
Pollution included six threat categories: sedimentation, pesticide pollution, chemical pollution, nutrient pollution, object pollution, and non-point pollution (classifications in Appendix 1). Pollution tied with overutilization for the greatest proportion of phyla (25%) for which year was not an important predictor. There were also two threats, non-point pollution and object pollution, for which angiosperms and mollusks, respectively, could not be analyzed due to small sample size (N < 10).
Of the six pollution threats, year was an important predictor only for sedimentation and pesticides across all four phyla (Appendix 9). There were three topmost pollution threats: sedimentation for angiosperms and mollusks and chemical pollution for mollusks (Figure 1). The only escalating threat within pollution was angiosperms impacted by sedimentation (Appendix 6). This small number of escalating threats was likely because half of the pollution threat-phylum combinations had a concave quadratic association with year (Figure 3; Appendix 9). Because this model form occurred so frequently, 75% of pollution threat-phylum combinations increased by a probability of ≤ 0.40 between a phylum's first and most recent year of listing (Figure 2).
Species Interactions
We broke species interactions into two threats: direct species interactions and indirect species interactions (refer to Appendix 1 for classifications). For both threats, we identified year of listing as a predictor for all four phyla (Figure 3; Appendix 9). Direct and indirect species interactions were topmost threats for angiosperms, arthropods, and mollusks (Figure 1). There were also several escalating threats: direct species interactions for angiosperms and arthropods and indirect species interactions for angiosperms and mollusks (Figure 2). Exceptions to the overall trend of escalating threats were chordates and arthropods, for which direct and indirect species interactions, respectively, decreased after 2006 (Appendices 8, 10). For mollusks, the threat of direct species interactions decreased from a probability of 0.32 in 1977 to 0.16 in 1989, and subsequently increased more than 6-fold by 2018 (Appendix 5).
Environmental Stochasticity
Three threat categories fell under the scope of environmental stochasticity: fire, severe weather, and climate change (for classifications see Appendix 1). Year was an important predictor of environmental stochasticity threats for all phyla, except for climate change with mollusks (Figure 3; Appendix 7). For this threat-phylum combination, all forms of year yielded a perfect model fit because all but one mollusk species was listed with climate change after 2012, and none had this threat before 2011.
Climate change was a topmost and escalating threat for all phyla modeled, increasing drastically after 2000 (Figures 2, 3). Severe weather was also a topmost and escalating threat for angiosperms, arthropods, and mollusks (Figures 1, 2; Appendix 7). Fire had a relatively lesser impact compared to the other two environmental stochasticity threats (Appendix 7). It was most prevalent among angiosperms, peaking at a probability of 0.77 in 2017 (Appendix 6). Fire impacting arthropods was the only environmental stochasticity threat which did not peak in a phyla's most recent year of listing (Appendix 10).
Demographic Stochasticity
Our analysis included three threats which relate to demographic stochasticity: few individuals, small range, and genetic/life history limitations (see Appendix 1 for classifications). The threat of few individuals was the only one to have been increasing for all phyla over time (Figure 3). Chordates were the only phylum for which small range and genetic/life history limitations did not peak in their most recent year of listing, their maximum probabilities occurring in 2004 and 2013, respectively (Appendix 8). All three demographic stochasticity threats were topmost for angiosperms, arthropods, and mollusks (Figure 1); however, most of these phyla started off with a relatively high probability of these threats. The only escalating threat was genetic/life history limitations impacting mollusks (Figure 2).
Discussion
In total, we analyzed 96 threat-phylum combinations in our study, of which we were able to identify a temporal trend for 80%. We found that angiosperms, arthropods, and mollusks each had a far greater number of topmost and escalating threats than chordates. Topmost threats that impacted all three of these phyla included both species interactions threats (direct and indirect species interactions), two out of the three environmental stochasticity threats (severe weather and climate change), and all three of the demographic stochasticity threats (few individuals, small range, and genetic/life history limitations). Additionally, aquatic development and chemical pollution were topmost threats for mollusks, and sedimentation was a topmost threat for angiosperms and mollusks. Escalating threats consisted of three for the arthropods (direct species interactions, severe weather, and climate change) and mollusks (indirect species interactions, severe weather, & genetic/life history limitations), whereas angiosperms had five (direct and indirect species interactions, severe weather, and climate change). For chordates, the only topmost and escalating threat was climate change.
We identified both direct and indirect species interactions as topmost threats for angiosperms, arthropods, and mollusks. However, there has been some debate (Boltovskoy et al., 2018) as to whether invasive species are a threat of great concern to biodiversity (McGeoch et al., 2010; Early et al., 2016), or if their impacts are lesser compared to those of other threats (Ricciardi and Ryan, 2017; Dueñas et al., 2018). While our direct and indirect species interactions threats encompassed all non-human species, including those designated as native, non-native, domestic, or unspecified, they were largely driven by invasive species. Our findings were in agreement with studies which have identified invasive species as problematic for angiosperms (Wilcove et al., 1998; Hernández-Yáñez et al., 2016), arthropods (Wilcove et al., 1998; Sánchez-Bayo and Wyckhuys, 2019; Wagner et al., 2021), and mollusks (Lydeard et al., 2004; Johnson et al., 2013; Lopes-Lima et al., 2014; Böhm et al., 2020). While the species interaction threats were not escalating and topmost for chordates as they were for the other three phyla, there is evidence that they are still having noteworthy impacts on this phylum (Wilcove et al., 1998; Ducatez and Shine, 2017; Currie and Marconi, 2020). One reason our findings may have differed from the literature was that we considered chordates as a phylum as opposed to breaking them down into classes as is common for comparative studies (e.g., Ducatez and Shine, 2017). For example, while invasive species impact a greater proportion of birds than mammals (Wilcove et al., 1998; Ducatez and Shine, 2017), our analysis would not account for among-class differences. Additionally, while our analysis did not examine differences in threats based on species' geography, it is widely known that invasive species disproportionately impact insular over mainland species (Wilcove et al., 1998; Hernández-Yáñez et al., 2016; Ducatez and Shine, 2017). An avenue of further research would be to quantify the difference between invasive species' impacts on these two groups. We did identify two threat-phylum combinations that have been declining since 2006: direct species interactions for chordates and indirect species interactions for arthropods. While this may indicate some success in mitigating invasive species threats, certain invasive species, such as insular vertebrate populations, are far easier to eradicate compared to large invasive plant populations (Rejmánek and Pitcairn, 2002; Keitt et al., 2011). Preventing future invasions and identifying effective techniques to remove species which have already invaded will be key to reducing species interaction threats for every phylum.
Topmost threats we found to be prevalent among all four phyla, and which have been widely acknowledged as a major concern to biodiversity, are those relating to the broad-resolution threat of environmental stochasticity (Thomas et al., 2004; Javeline et al., 2013; Evans et al., 2016). Topmost threat intensity differed among phyla, with severe weather being prevalent only in angiosperms, arthropods and mollusks whereas climate change was prevalent across all four phyla. While we were not able to model temporal changes in climate change for mollusks, all but one mollusk species was listed with climate change after 2012, and none had this threat before 2011. Our findings confirm those of several studies which have identified climate change as an important threat for arthropods (Sánchez-Bayo and Wyckhuys, 2019; Wagner et al., 2021) and chordates (Ducatez and Shine, 2017). Not only is climate change a threat in itself, but it also intensifies other forms of severe weather, such as storms, fires, and drought (IPCC, 2014). We found severe weather to have a similar impact as climate change, being topmost and escalating for angiosperms, arthropods, and mollusks. Reviews of threats to mollusks (Lydeard et al., 2004; Johnson et al., 2013; Böhm et al., 2020) cite habitat alteration, pollution, and invasive species as the threats of greatest concern; however, our results indicate that severe weather and climate change should be included as top threats as well. The environmental stochasticity threat typically cited as having the greatest impact on angiosperms is fire (Wilcove et al., 1998; Hernández-Yáñez et al., 2016); however, we found both climate change and severe weather to be greater threats to this phylum. As climate change and environmental stochasticity continue to intensify, we expect them to threaten every species listed in the coming years in some capacity.
Another broad-resolution threat with topmost threats for angiosperms, arthropods, and mollusks was demographic stochasticity: few individuals, small range, and genetic/life history limitations. Interestingly, these demographic threats are not frequently included in taxa-wide assessments of threats, a few exceptions citing only a small or reduced range as a threat to arthropods (Bland, 2017) and mollusks (Johnson et al., 2013). One reason for these omissions may be that demographic stochasticity is not an “anthropogenic” threat, even though in most cases it results from anthropogenic pressures (Belovsky et al., 1994; Butchart et al., 2010). Another reason may be that research focusing on imperiled biodiversity may assume that their focal species are faced by some form of few individuals or small range, so they do not elaborate further on these threats. While there was only one escalating demographic stochasticity threat-phylum combination, genetic/life history limitations for mollusks, all three threats have all been increasing to some degree for all phyla. We would have expected these threats to decrease, as species with the fewest individuals and smallest populations were likely listed in the first years of the ESA. A potential explanation is the delay many species experience between their initial proposal for listing and their final listing. Puckett et al. (2016) found that the average time to listing across all species was 12.8 years; however, for chordate species, this average time was a substantially lower 7.3 years. This shorter time to listing may have resulted in less demographic peril for chordates. This is reflected in our results: while each demographic stochasticity threat was topmost for angiosperms, arthropods, and mollusks, none were topmost for chordates. Because the longer we wait to protect a species, the more likely they are to be faced by severe, synergistically acting threats from which recovery becomes more difficult (Gilpin and Soulé, 1986), it is important to promptly list all imperiled species, not just the charismatic ones.
In addition to the disproportionate impact these topmost and escalating threats have on each phylum, there is also a discrepancy in the funding allocated toward their recovery. Gerber (2016) contrasted the funding allocated for the recovery of species listed under the ESA with the amount they actually received (Table 1). Chordates, faced by only one topmost and escalating threat, were not only allocated more funding per species than the other three phyla combined, but they received an average of 7% more funding than they required (Gerber, 2016). Conversely, mollusks, the phylum with the greatest number of topmost threats, had the least amount of funding allocated and received per species (Gerber, 2016). Angiosperms, which we found to have the greatest number of escalating threats, were the most underfunded phylum, receiving an average of only 38% of their allocated funds per species (Gerber, 2016). Several studies (Male and Bean, 2005; Evans et al., 2016; Haines et al., 2021) have identified a positive association between a species' recovery success and the amount of funding they were given. With complex threats of invasive species, environmental stochasticity, and demographic stochasticity escalating for the non-chordate phyla, it is important that limited conservation resources be allotted to the species in most dire need.
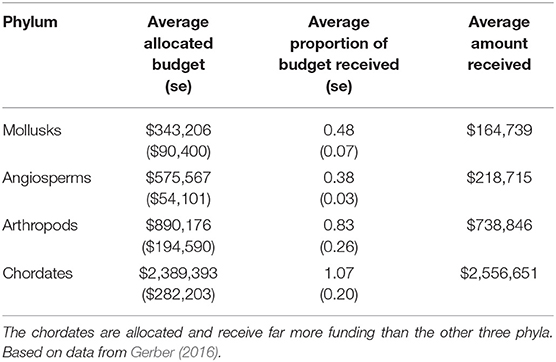
Table 1. Average allocated budget and proportion of budget received for each of the four phyla (se = standard error).
Our research points to several successes in biodiversity conservation. Of all the threat-phylum combinations analyzed, only authorized take decreased for angiosperms in every year since they were listed. This threat encompasses take for commercial, recreational, and scientific or educational purposes, but not illegal take or poaching. One reason for this decrease may be that these forms of legal take are relatively easy to manage. Plants occurring on protected state or federal lands are often provided regulations governing their take, thereby reducing the detrimental impact of legal take (Evans et al., 2016). The success of mitigating this threat with angiosperms mirrors the findings of Leu et al. (2019), who found that overutilization impacting ESA-listed species was the only broad-resolution threat to have always been decreasing over time. However, our results indicate that this success is limited to the angiosperms. For chordates, all three overutilization threats—authorized take, unauthorized take (illegal take, vandalism on the species), and unintentional take (accidental take, accidental collisions)—have been increasing. While none of these threats were near the magnitude of a topmost or escalating threat, their increasing presence in chordate listing decisions is cause for attention. To make matters more complex, the management strategies which have proven effective for angiosperms may not yield the same results for chordates. In addition to having drastically different characteristics, e.g., being motile vs. non-motile, there are also some chordate species which are widely disliked, such as snakes and wolves (Knight, 2008; Houston et al., 2010). Effectively conserving these species from overutilization will likely involve greater efforts to sway public opinion than were required for angiosperms. However, because exploitation is a prominent cause of plant endangerment and extinction worldwide (Leclerc et al., 2018), learning from the success with authorized take in the U.S. could benefit imperiled plants globally.
A second threat category in which we have identified conservation success is pollution. Mollusks are known to be highly susceptible to pollution, and multiple studies (Lydeard et al., 2004; Johnson et al., 2013; Lopes-Lima et al., 2014; Böhm et al., 2020) have cited pollutants such as sedimentation, chemicals, nutrients, and non-point pollution as top threats to this phylum. However, we found that the probabilities of each of those four threats being included in mollusk listing decisions have been decreasing since the mid-2000s. Similarly, two out of the three pollution threats for which year was a predictor for arthropods and two out of the six pollution threats for which year was a predictor for chordates have also been decreasing since the mid-2000s, even though pollution was regarded to be a prominent threat for these phyla (Wilcove et al., 1998; Sánchez-Bayo and Wyckhuys, 2019; Currie and Marconi, 2020; Wagner et al., 2021). Our findings supported those of Leu et al. (2019), who found the broad-resolution threat of pollution shifted from increasing to decreasing in the mid-2000s. This reduction of detrimental pollutants is in large part due to legislation such as the U.S. Clean Air Act (CAA; 1970) and the U.S. Clean Water Act (CWA; 1972). In those three decades since their enactment, sufficient progress had been made to both reduce the amount of pollutants put into the environment and to clean up areas which had been severely degraded by pollution (McKitrick, 2007; Gibson-Reinemer et al., 2017). Haines et al. (2021) found that regulations on pollution, such as the CWA, CAA, and other actions such as the banning of DDT in 1972, played an essential role in the recovery of nine species that formerly required the ESA's protection. The CWA alone was cited as protecting 24% of the recovered species even after they had been delisted by the ESA (Haines et al., 2021). Our findings support the conclusion of Haines et al. (2021) that strong policies outside of the ESA are effective tools in mitigating threats to imperiled species.
A third threat category in which we have identified potential conservation success is habitat modification. While there was no universal trend, 11 out of the 25 habitat modification threat-phylum combinations for which year was a predictor had decreased around the early 2000s: aquatic development for angiosperms, chordates, and mollusks; development for angiosperms; harvested renewable resources for chordates; human disturbances for angiosperms and arthropods; mining & oil/gas for angiosperms and chordates, and non-developmental habitat alteration for angiosperms and mollusks. Notably, mining & oil/gas impacting angiosperms was one of two threat-phylum combinations for which we saw a decrease in the threat's probability between the phylum's first and most recent years of listing. It was the only habitat modification threat to do so. While this threat has always had a low prevalence for angiosperms, peaking at just 0.14 in 1993, we are not sure why it has declined. We recommend further research into factors which have caused mining and oil/gas to be less of a threat to this phylum over time. Additionally, factors which have recently caused the other habitat modification threats to decrease warrant further investigation to better understand mechanisms that may help reduce habitat modification threats.
Understanding the dynamic nature of threats is imperative to developing effective conservation strategies. We were able to identify several threats relating to habitat modification, overutilization, and pollution which have been declining in recent years for some phyla, with authorized take constantly declining for angiosperms. However, we also identified threats which were topmost and escalating for most phyla, primarily those in species interactions, environmental stochasticity, and demographic stochasticity categories. Not only are these threats causing the most endangerment, but they are more difficult to manage. To mitigate these escalating threats, we recommend that conservation efforts focus on invasive species management and preserving habitat and corridors so that species can move into suitable habitat as the climate changes (Bernazzani et al., 2012). Additionally, adequately funding all phyla, or at least providing need-based funding for phyla with the greatest number of topmost and escalating threats, will be crucial to providing all species the opportunity to recover. By understanding the temporal nature of threats which have impacted protected phyla, we can increase the effectiveness with which we preserve our biodiversity.
Data Availability Statement
The data and R code is publicly available at: https://osf.io/w9k5b/, further inquiries can be made to the corresponding author.
Author Contributions
DC contributed writing, data analysis, and overall construction of this manuscript. ML and AH contributed writing, data analysis, and figure development. All authors contributed to the article and approved the submitted version.
Funding
Funding for this project came from the Virginia Space Grant Consortium Graduate Student Fellowship and the Broderick Family/Goldman Sachs Associate Professor of Biology.
Conflict of Interest
The authors declare that the research was conducted in the absence of any commercial or financial relationships that could be construed as a potential conflict of interest.
Publisher's Note
All claims expressed in this article are solely those of the authors and do not necessarily represent those of their affiliated organizations, or those of the publisher, the editors and the reviewers. Any product that may be evaluated in this article, or claim that may be made by its manufacturer, is not guaranteed or endorsed by the publisher.
Acknowledgments
We would like to thank Lauren Bleyer, Matthew Dungan, Carli Parenti, Emily Ritter, Olivia Rosensteel, Ann Marie Rydberg, Michella Salvitti, and Grace Smoot for their assistance in data collection.
Supplementary Material
The Supplementary Material for this article can be found online at: https://www.frontiersin.org/articles/10.3389/fcosc.2021.727517/full#supplementary-material
References
Barnosky, A. D., Matzke, N., Tomiya, S., Wogan, G. O. U., Swartz, B., Quental, T. B., et al. (2011). Has the Earth's sixth mass extinction already arrived? Nature 471, 51–57. doi: 10.1038/nature09678
Barton, K. (2020). MuMIn: Multi-Model Inference. Available online at: https://CRAN.R-project.org/package=MuMIn (accessed June 14, 2021).
Belovsky, G. E., Bissonette, J. A., Dueser, R. D., Edwards, T. C. Jr., Luecke, C. M., Ritchie, M. E., et al. (1994). Management of small populations: concepts affecting the recovery of endangered species. Wildl. Soc. Bull. 22, 307–316.
Bernazzani, P., Bradley, B. A., and Opperman, J. J. (2012). Integrating climate change into habitat conservation plans under the U.S. Endangered Species Act. Environ. Manage. 49, 1103–1114. doi: 10.1007/s00267-012-9853-2
Bland, L. M. (2017). Global correlates of extinction risk in freshwater crayfish. Anim. Conserv. 20, 532–542. doi: 10.1111/acv.12350
Böhm, M., Dewhurst-Richman, N. I., Seddon, M., Ledger, S. E. H., Albrecht, C., Allen, D., et al. (2020). The conservation status of the world's freshwater molluscs. Hydrobiologia 848, 3231–3254. doi: 10.1007/s10750-020-04385-w
Boltovskoy, D., Sylvester, F., and Paolucci, E. M. (2018). Invasive species denialism: sorting out facts, beliefs, and definitions. Ecol. Evol. 8, 11190–11198. doi: 10.1002/ece3.4588
Burnham, K. P., and Anderson, D. R. (2002). Model Selection and Multimodel Inference: A Practical Information-Theoretic Approach, 2nd Edn. New York, NY: Springer.
Butchart, S. H. M., Walpole, M., Collen, B., van Strien, A., Scharlemann, J. P. W., Almond, R. E. A., et al. (2010). Global biodiversity: indicators of recent declines. Science 328, 1164–1168. doi: 10.1126/science.1187512
Currie, J., and Marconi, V. (2020). An analysis of threats and factors that predict trends in Canadian vertebrates designated as at-risk. Facets 5, 49–66. doi: 10.1139/facets-2019-0017
Ducatez, S., and Shine, R. (2017). Drivers of extinction risk in terrestrial vertebrates. Conserv. Lett. 10, 186–194. doi: 10.1111/conl.12258
Dueñas, M. A., Ruffhead, H. J., Wakefield, N. H., Roberts, P. D., Hemming, D. J., and Diaz-Soltero, H. (2018). The role played by invasive species in interactions with endangered and threatened species in the United States: a systematic review. Biodivers. Conserv. 27, 3171–3183. doi: 10.1007/s10531-018-1595-x
Early, R., Bradley, B. A., Dukes, J. S., Lawler, J. J., Olden, J. D., Blumenthal, D. M., et al. (2016). Global threats from invasive alien species in the twenty-first century and national response capacities. Nat. Commun. 7:12485. doi: 10.1038/ncomms12485
Endangered Species Act (ESA) (1973). Public Law No. 93–205, 87 U. S. Statutes at Large 884, Dec. 23, 1973, codified as amended at 16 16 U.S.C. secs. 1531–43. ESA.
Evans, D. M., Che-Castaldo, J. P., Crouse, D., Davis, F. W., Epanchin-Niell, R., Flather, C. H., et al. (2016). Species recovery in the United States: increasing the effectiveness of the Endangered Species Act. Issues Ecol. 20, 1–28.
Foin, T. C., Riley, S. P. D., Pawley, A. L., Ayres, D. R., Carlsen, T. M., Hodum, P. J., et al. (1998). Improving recovery planning for threatened and endangered species. BioScience 48, 117–184. doi: 10.2307/1313263
Gerber, L. R. (2016). Conservation triage or injurious neglect in endangered species recovery. Proc. Natl. Acad. Sci. U. S. A. 113, 3563–3566. doi: 10.1073/pnas.1525085113
Gibson-Reinemer, D. K., Sparks, R. E., Parker, J. L., Deboer, J. A., Fritts, M. W., McClelland, M. A., et al. (2017). Ecological recovery of a river fish assemblage following the implementation of the Clean Water Act. BioScience 67, 957–970. doi: 10.1093/biosci/bix110
Gilpin, M. E., and Soulé, M. E. (1986). “Minimum viable populations: processes of extinction,” in Conservation Biology: The Science of Scarcity and Diversity, ed. M. E. Soulé (Sunderland, MA: Sinauer Associates), 19–34.
Haines, A. M., Leu, M., Costante, D. M., Treakle, T. C., Parenti, C., Miller, J. R. B., et al. (2021). Benchmark for the ESA: having a backbone is good for recovery. Front. Conserv. Sci. 2:630490. doi: 10.3389/fcosc.2021.630490
Hernández-Yáñez, H., Kos, J. T., Bast, M. D., Griggs, J. L., Hage, P. A., Killian, A., et al. (2016). A systematic assessment of threats affecting the rare plants of the United States. Biol. Conserv. 203, 260–267. doi: 10.1016/j.biocon.2016.10.009
Houston, M. J., Bruskotter, J. T., and Fan, D. (2010). Attitudes toward wolves in the United States and Canada: a content analysis of the print news media, 1999-2008. Hum. Dimens. Wildl. 15, 389–403. doi: 10.1080/10871209.2010.507563
IPCC (2014). “Climate change 2014: synthesis report,” in Contribution of Working Groups I, II and III to the Fifth Assessment Report of the Intergovernmental Panel on Climate Change, eds Core Writing Team, R. K. Pachauri, and L. A. Meyer (Geneva: IPCC), 151.
Javeline, D., Hellmann, J. J., Cornejo, R. C., and Shufeldt, G. (2013). Expert opinion on climate change and threats to biodiversity. BioScience 63, 666–673. doi: 10.1525/bio.2013.63.8.9
Johnson, P. D., Bogan, A. E., Brown, K. M., Burkhead, N. M., Cordeiro, J. R., Garner, J. T., et al. (2013). Conservation status of freshwater gastropods of Canada and the United States. Fisheries 38, 247–282. doi: 10.1080/03632415.2013.785396
Keitt, B., Campbell, K., Saunders, A., Clout, M., Wang, Y., Heinz, R., et al. (2011). “The Global Islands Invasive Vertebrate Eradication Database: a tool to improve and facilitate restoration of island ecosystems”, in Island Invasives: Eradication and Management, eds C. R. Veitch, M. N. Clout, and D. R. Towns (Gland: IUCN), 74–77.
Knight, A. J. (2008). “Bats, snakes and spiders, Oh my!” How aesthetic and negativistic attitudes, and other concepts predict support for species protection. J. Environ. Psychol. 28, 94–103. doi: 10.1016/j.jenvp.2007.10.001
Lawler, J. J., Campbell, S. P., Guerry, A. D., Kolozsvary, M. B., O'Connor, R. J., and Seward, L. C. N. (2002). The scope and treatment of threats in endangered species recovery plans. Ecol. Appl. 12, 663–667. doi: 10.1890/1051-0761(2002)012[0663:TSATOT]2.0.CO;2
Leclerc, C., Courchamp, F., and Bellard, C. (2018). Insular threat associations within taxa worldwide. Sci. Rep. 8, 6393–6398. doi: 10.1038/s41598-018-24733-0
Leidner, A. K., and Neel, M. C. (2011). Taxonomic and geographic patterns of decline for Threatened and Endangered species in the United States. Conserv. Biol. 25, 716–725. doi: 10.1111/j.1523-1739.2011.01689.x
Leu, M., Haines, A. M., Check, C. E., Costante, D. M., Evans, J. C., Hollingsworth, M. A., et al. (2019). Temporal analysis of threats causing species endangerment in the United States. Conserv. Sci. Pract. 1:e78. doi: 10.1111/csp2.78
Lopes-Lima, M., Teixeira, A., Froufe, E., Lopes, A., Varandas, S., and Sousa, R. (2014). Biology and conservation of freshwater bivalves: past, present, and future perspectives. Hydrobiologia 735, 1–13. doi: 10.1007/s10750-014-1902-9
Lydeard, C., Cowie, R. H., Ponder, W. F., Bogan, A. E., Bouchet, P., Clark, S. A., et al. (2004). The global decline of nonmarine mollusks. BioScience 54, 321–330. doi: 10.1641/0006-3568(2004)054[0321:TGDONM]2.0.CO;2
Male, T. D., and Bean, M. J. (2005). Measuring progress in US endangered species conservation. Ecol. Lett. 8, 986–992. doi: 10.1111/j.1461-0248.2005.00806.x
McGeoch, M. A., Butchart, S. H. M., Spear, D., Marais, E., Kleynhans, E. J., Symes, A., et al. (2010). Global indicators of biological invasion: species numbers, biodiversity impact and policy responses. Divers. Distrib. 16, 95–108. doi: 10.1111/j.1472-4642.2009.00633.x
McKinney, M. L. (1999). High rates of extinction and threat in poorly studied taxa. Conserv. Biol. 13, 1273–1281. doi: 10.1046/j.1523-1739.1999.97393.x
McKitrick, R. (2007). Why did US air pollution decline after 1970? Empir. Econ. 33, 491–513. doi: 10.1007/s00181-006-0111-4
Pimm, S. L., Jenkins, C. N., Abell, R., Brooks, T. M., Gittleman, J. L., Joppa, L. N., et al. (2014). The biodiversity of species and their rates of extinction, distribution, and protection. Science 344:987. doi: 10.1126/science.1246752
Puckett, E. E., Kesler, D. C., and Greenwald, D. N. (2016). Taxa, petitioning agency, and lawsuits affect time spent awaiting listing under the US Endangered Species Act. Biol. Conserv. 201, 220–229. doi: 10.1016/j.biocon.2016.07.005
R Core Team (2019). R: A Language and Environment for Statistical Computing. Vienna: R Foundation for Statistical Computing. www.R-project.org.
Rejmánek, M., and Pitcairn, M. J. (2002). “When is eradication of exotic pest plants a realistic goal?,” in Turning the Tide: The Eradication of Invasive Species, eds C. R. Veitch, and M. N. Clout (Gland; Cambridge: IUCN), 249–253.
Ricciardi, A., and Ryan, R. (2017). The exponential growth of invasive species denialism. Biol. Invas. 20, 549–553. doi: 10.1007/s10530-017-1561-7
Sánchez-Bayo, F., and Wyckhuys, K. A. G. (2019). Worldwide decline of the entomofauna: a review of its drivers. Biol. Conserv. 232, 8–27. doi: 10.1016/j.biocon.2019.01.020
Scherer, R. D., Muths, E., and Noon, B. R. (2012). The importance of local and landscape-scale processes to the occupancy of wetlands by pond-breeding amphibians. Popul. Ecol. 54, 487–498. doi: 10.1007/s10144-012-0324-7
Thomas, C. D., Cameron, A., Green, R. E., Bakkenes, M., Beaumont, L. J., Collingham, Y. C., et al. (2004). Extinction risk from climate change. Nature 427, 145–148. doi: 10.1038/nature02121
Wagner, D. L., Grames, E. M., Forister, M. L., Berenbaum, M. R., and Stopak, D. (2021). Insect decline in the Anthropocene: death by a thousand cuts. Proc. Natl. Acad. Sci. U. S. A. 118:e2023989118. doi: 10.1073/pnas.2023989118
Keywords: angiosperms, arthropods, chordates, endangered species act, mollusks, temporal, threats
Citation: Costante DM, Haines AM and Leu M (2022) Threats to Neglected Biodiversity: Conservation Success Requires More Than Charisma. Front. Conserv. Sci. 2:727517. doi: 10.3389/fcosc.2021.727517
Received: 18 June 2021; Accepted: 29 November 2021;
Published: 14 January 2022.
Edited by:
Krithi K. Karanth, Centre for Wildlife Studies, IndiaReviewed by:
Matthew Grainger, Norwegian Institute for Nature Research (NINA), NorwayCourtney Hughes, Government of Alberta, Canada
Copyright © 2022 Costante, Haines and Leu. This is an open-access article distributed under the terms of the Creative Commons Attribution License (CC BY). The use, distribution or reproduction in other forums is permitted, provided the original author(s) and the copyright owner(s) are credited and that the original publication in this journal is cited, in accordance with accepted academic practice. No use, distribution or reproduction is permitted which does not comply with these terms.
*Correspondence: Matthias Leu, mleu@wm.edu