- 1Programa de Magíster en Fisiología Clínica del Ejercicio, Facultad de Ciencias, Universidad Mayor, Santiago, Chile
- 2Servicio de Kinesiología, Unidad de Medicina Física y Rehabilitación, Hospital Clínico UC-CHRISTUS, Santiago, Chile
- 3Department of Physical Therapy, Faculty of Medicine, University of Chile, Santiago, Chile
- 4International Physiotherapy Research Network (PhysioEvidence), Barcelona, Spain
- 5Institut d'Investigacions Biomèdiques August Pi i Sunyer (IDIBAPS), Barcelona, Spain
- 6Instituto Cardiovascular de Rosario, Rosario, Argentina
- 7Universidad del Gran Rosario, Rosario, Argentina
- 8Blanquerna School of Health Sciences, Global Research on Wellbeing (GRoW), Universitat Ramon Llull, Barcelona, Spain
- 9Department of Pulmonary Medicine, Hospital Clínic, University of Barcelona, Barcelona, Spain
- 10Biomedical Research Networking Center on Respiratory Diseases (CIBERES), Madrid, Spain
Background: Congenital heart disease (CHD) entails structural defects in the morphogenesis of the heart or its main vessels. Analyzing exercise capacity of children and adolescents with CHD is important to improve their functional condition and quality of life, since it can allow timely intervention on poor prognostic factors associated with higher risk of morbidity and mortality.
Objective: To describe exercise capacity in children and adolescents with CHD compared with healthy controls.
Methods: A systematic review was carried out. Randomized clinical trials and observational studies were included assessing exercise capacity through direct and indirect methods in children and adolescents between 5 and 17 years-old. A sensitive analysis was performed including studies with CHD repaired participants. Additionally, it was sub-analyzed by age range (< and ≥ 12 years old). Two independent reviewers analyzed the studies, extracted the data, and assessed the quality of the evidence.
Results: 5619 articles were found and 21 were considered for the review. Eighteen articles used the direct exercise capacity measurement method by cardiopulmonary exercise test (CPET). The CHD group showed significant differences in peak oxygen consumption (VO2peak) with a value of −7.9 ml/Kg/min (95% CI: −9.9, −5.9, p = 0.00001), maximum workload (Wmax) −41.5 (95% CI: −57.9, −25.1 watts, p = 0.00001), ventilatory equivalent (VE/VCO2) slope 2.6 (95% CI: 0.3, 4.8), oxygen pulse (O2 pulse)−2.4 ml/beat (95% CI: −3.7, −1.1, p = 0.0003), and maximum heart rate (HRmax) −15 bpm (95% CI: −18, −12 bpm, p = 0.00001), compared with healthy controls. Adolescents (≥ 12 yrs) with CHD had a greater reduction in VO2peak (−10.0 ml/Kg/min (95% CI: −12.0, −5.3), p <0.00001), Wmax (−45.5 watts (95% CI: −54.4, −36.7), p < 0.00001) and HRmax (−21 bpm (95% CI: −28, −14), p<0.00001).
Conclusion: Suffering CHD in childhood and adolescence is associated with lower exercise capacity as shown by worse VO2peak, Wmax, VE/VCO2 slope, O2 pulse, and HRmax compared with matched healthy controls. The reduction in exercise capacity was greater in adolescents.
Systematic Review Registration: www.crd.york.ac.uk/prospero/display_record.php?RecordID=208963, identifier: CRD42020208963.
What is Known
Exercise capacity is one of the main factors that affect health-related quality of life, prognosis, risk of morbidity, and early mortality from cardiovascular, metabolic, or respiratory disease.
Analyzing exercise capacity in children and adolescents with CHD is important to improve their functional condition and quality of life, since it can allow timely intervention on poor prognostic factors associated with higher risk of morbidity and mortality.
What the Study Adds
VO2peak, HRmax, Wmax, and O2 pulse were significantly lower in children and adolescents with partially or fully repaired CHD compared with healthy controls.
Introduction
Congenital heart disease (CHD) entails structural defects in the morphogenesis of the heart or its main vessels (1). They are the most common congenital defect in children worldwide, with an average prevalence of 8.22 per 1000 live newly born, ranging from 2.4 to 13.7 (2, 3). According to intracardiac morphology and physiology, they are classified as acyanotic and cyanotic, and according to its severity as either simple, or complex (1).
For its treatment, there are both corrective and palliative surgeries (1). Due to medical advances, greater and better diagnostic, surgical and postoperative care technology, it is expected that more than 90% of children with CHD will currently survive to adulthood (4). However, despite the increase in the life expectancy of children with CHD, the residual defects that may remain after surgery can have a negative effect on both morbidity and mortality (5).
It has been reported that physical capacity in children with CHD is lower compared to healthy controls with limited exercise capacity and a shorter lifespan related to health (6). Limited exercise capacity favors a more sedentary lifestyle, a situation that can be maintained into adulthood (7). Accordingly, less physical activity increases the risk of overweight and obesity in children with CHD, which means an additional health burden (8).
Exercise capacity is one of the main factors when assessing health-related quality of life, prognosis, risk of morbidity, and early mortality from cardiovascular, metabolic, or respiratory disease (9). It can be evaluated by a standardized laboratory test such as cardiopulmonary exercise test (CPET) or standardized field tests such as the six-minute walking test (6MWT), shuttle walking test (SWT), time up and go (TUG), or similar tests (10). The CPET, which assesses the maximum oxygen consumption (VO2max) or peak (VO2peak) and measures ventilatory efficiency, has obtained a prognostic value in adults with acquired heart failure and CHD, by identifying subjects with limited cardiovascular reserve (11). Studies that consider the measurement of VO2peak in subjects with cyanotic CHD and palliative surgery for complex CHD highlighted it as an independent predictor of death or hospitalization due to a cardiovascular event (12, 13). In children with chronic diseases, VO2peak can predict adverse or unfavorable outcomes (6). The ventilatory equivalent for carbon dioxide production (VE/VCO2) has been shown to have high sensitivity as a predictor of mortality in subjects with various CHD (14).
Analyzing exercise capacity of children and adolescents with CHD is important to improve their functional condition and quality of life, since it can allow timely intervention on poor prognostic factors associated with higher risk of morbidity and mortality.
This review aims to systematically analyse studies to summarize exercise capacity, assessed with laboratory tests or standardized field tests, in children and adolescents with CHD compared with their healthy counterparts, using a meta-analysis of observational studies. Our purposes are to find out if the main exercise capacity variables such as VO2peak or Wmax are reduced in children and adolescents with CHD, and to find out if the cardiac response to exercise is also influenced.
Methods
Protocol and Registration
We performed a systematic review using Preferred Reporting Items for Systematic Reviews and Meta-analyses (PRISMA) guidelines (15). The review was registered in the International Prospective Register of Systematic Reviews (PROSPERO) CRD42020208963.
Criteria for Considering the Studies in This Review
We included randomized clinical trials (RCTs) or observational studies (cross-sectional, longitudinal, case-control, and cohort) in children and adolescents with a diagnosis of CHD. The included studies aimed to determine the physical capacity in patients with CHD. Additionally, the studies should report VO2peak, maximal workload (Wmax), distance walked in the 6MWT (6MWD), or similar measurements obtained from objective tests. All editorials, letters, conference publications, review articles, systematic reviews, meta-analyses, in vivo and in vitro studies were excluded.
Search Strategies and Data Resources
We reviewed seven databases: Embase, Cochrane Central Register of Controlled Trials (CENTRAL), CINAHL, Web of Science, PubMed/MEDLINE, Scopus, and SciELO, from their inception to 01 June, 2021 and conducted manual searches using the followings terms: a) [(Congenital heart disease) OR (Congenital heart defects) OR (Congenital heart surgery) OR (Fontan operation) OR (Fontan circulation) OR (Fontan patient) OR (Fontan physiology) OR (Fontan procedure) OR (Tetralogy of Fallot) OR (Interventricular communication) OR (Atrial communication)] AND [(Exercise capacity) OR (Physical capacity) OR (Exercise tolerance) OR (Cardiopulmonary exercise test) OR (CPET) OR (Walking test) OR (Walk test) OR (6MWT) OR (Shuttle walking test) OR (SWT) OR (distance walked) OR (Oxygen uptake) OR (Oxygen consumption) OR (Wingate Anaerobic Test) OR (Timed Up and Go) OR (TUG) OR (Exercise Test) OR (sit to stand) OR (step test) OR (STS)] AND [(Children) OR (Adolescents) OR (Pediatrics) OR (Childhood) OR (Pediatric)].
The selected terms were combined using Boolean logical operators (OR, AND, NOT). Moreover, we performed a manual search of the references that were included in the selected articles. All the references were analyzed in Rayyan software, a web-based tool (16).
Reviewing Procedure and Study Selection
The review was performed independently by two investigators (YVR-JVM), who independently reviewed all articles titles and abstracts identified in the search strategy. The full text of potentially eligible studies was then read to verify their suitability for final inclusion. All studies that did not fulfill the predefined criteria were excluded, and their bibliographic details were listed with the specific reason for exclusion.
Data Extraction and Methodological Quality Assessment
Two investigators (YVR-JVM) independently extracted data from the selected articles and recorded them in an ad hoc spreadsheet of relevant data. This included author, country, year of publication, sample size, study design, age of subjects, diagnoses, evaluation instruments, evaluated variables, and results. Data from the first assessment were considered for randomized or non-randomized clinical trials. Differences obtained from data extraction were resolved by consensus. In the case of not reaching an agreement, a third investigator (RTC) resolved the differences. If some relevant data were not in the article, the author was contacted to request the information.
Assessment of the methodological quality of the primary articles was carried out using the quality assessment tools from the National Heart, Lung, and Blood Institute (NHLBI) (17). Each tool contains criteria based on which internal validity and risk of bias are evaluated. The criteria are evaluated as “yes” “no” or “other” (not reported, not applicable, or not determinable), and an overall rating is provided for each study based on the items rated with an affirmative answer (> 75% = good, 50–75% = fair, < 50% = poor).
Data Synthesis and Analysis
We reported summaries of the association between the outcomes for each study in terms of mean differences using Review Manager 5 (RevMan, Copenhagen: The Nordic Cochrane Center, The Cochrane Collaboration, 2014). We obtained combined measurements of effect for each primary outcome through meta-analysis under a random-effect model, due to the expected heterogeneity between the studies. Statistical heterogeneity was measured through the I2 statistic and classified as low (I2 <25%), moderate (I2 25–50%) or high (I2 >50%). A sensitivity analysis was conducted including studies with only children and adolescents surgically repaired. Additionally, when possible, a subanalysis by age group (< and ≥ 12 years) was performed in the group of patients with surgical correction.
Results
Study Selection
The flow chart of the study selection process is shown in Figure 1. In the initial search of the selected databases, 5619 potential studies were identified. Of the 107 studies assessed as full text, we excluded 57 for wrong study designs, 16 for wrong population, 13 for wrong publication type, and nine due to a wrong outcome. In total, 21 studies fulfilled the criteria for eligibility and were included in the review.
Characteristics of the Included Studies
Fifteen studies were conducted in Europe (18–32), three in the United States (33–35), one in Brazil (36), one in Japan (37), and one was conducted in Egypt (38). Regarding the design, 20 were cross-sectional (18–23, 25–38), and only one was an RCT (24). The characteristics of the included studies are summarized in Table 1.
Participants
In total, 1540 participants with CHD and 1248 healthy controls were enrolled in the included studies. Among the studies, the CHD sample size ranged from 12 (30) to 496 (21). Age varied from 7.7 ± 1.7 (28) to 14 ± 2.72 (20). The majority of the studies included only complex CHD (18–20, 22, 25, 27, 31, 33–36, 38), and nine studies included both simple and complex CHD (21–24, 26, 28–30, 32, 37). Sixteen studies included only repaired CHD (18–20, 22, 24–27, 30, 31, 33–38); however, only ten studies reported the age of surgery, which was before 3 years old in the majority of the cases (19, 22, 26–28, 30, 33). A summary of patients' characteristics is presented in Table 1.
Type of Assessment
To assess exercise capacity, 18 studies used the direct CPET method: seven of them performed the CPET on a treadmill (18–20, 27, 36–38) and 13 in a cycle ergometer (21–26, 29–35). One study used the 6MWT (24). The results of the studies that used the CPET to evaluate exercise capacity are shown in Table 2. The quantitative variables (VO2peak, Wmax, VE/CO2 slope, O2 Pulse, and HRmax) expressed as mean and SD were analyzed using a meta-analysis method. Results from Bowyer et al. (18) and Binkhorst et al. (29) were not included in the meta-analysis because they reported their results in other units.
Risk of Bias Assessment
Of the selected cross-sectional articles (n = 20), all were rated as “fair” (50–75% affirmative answers). The RCT study (n = 1) obtained a “fair” rating. The quality assessment results for the individual studies obtained using the NHBLI quality assessment tool are presented in the Supplementary File 1.
Main Findings
Peak Oxygen Consumption
Seventeen studies examined exercise capacity considering VO2peak (19–27, 30–37). These studies compared 1388 participants with CHD vs. 1102 healthy controls. The heterogeneity of the comparison was high (I2 = 91%). Participants with CHD averaged −7.9 ml/Kg/min (95% CI: −9.9, −5.9) of VO2peak compared with controls (p < 0.00001) (Figure 2). When considering the analysis by subgroups according to the type of test, those who performed the test on treadmill included 166 subjects with CHD and 120 controls, while on cycle ergometer it was 1222 CHD and 982 controls. Those who performed CPET on a treadmill had on average −9.6 ml/Kg/min (95% CI: −14.0, −5.2) of VO2peak compared with the control group (p < 0.0001) and those who performed test on cycle ergometer had an average of −7.1 ml/Kg/min (95% CI: −9.3, −5.0) of VO2peak as compared with the control group (p<0.00001). If we analyze only studies with patients surgically repaired (19, 20, 22, 24–27, 30, 31, 33–37), the participants with CHD averaged −8.7 ml/Kg/min (95% CI: −12.0, −5.4) of VO2peak compared with controls (p < 0.00001, I2 = 92%) (Supplementary File 2, Figure 1). In addition, we sub-analyzed by age range and observed that the VO2peak of the group under 12 years old had a decrease of −7.1 ml/Kg/min (95% CI: −11.7, −2.4) compared with the control group (p < 0.00001, I2 = 89%), and the group ≥ 12 years old had a reduction of −10.0 ml/Kg/min (95% CI: −12.0, −5.3) compared with the control group (p < 0.00001, I2 = 82%) (Figure 3).
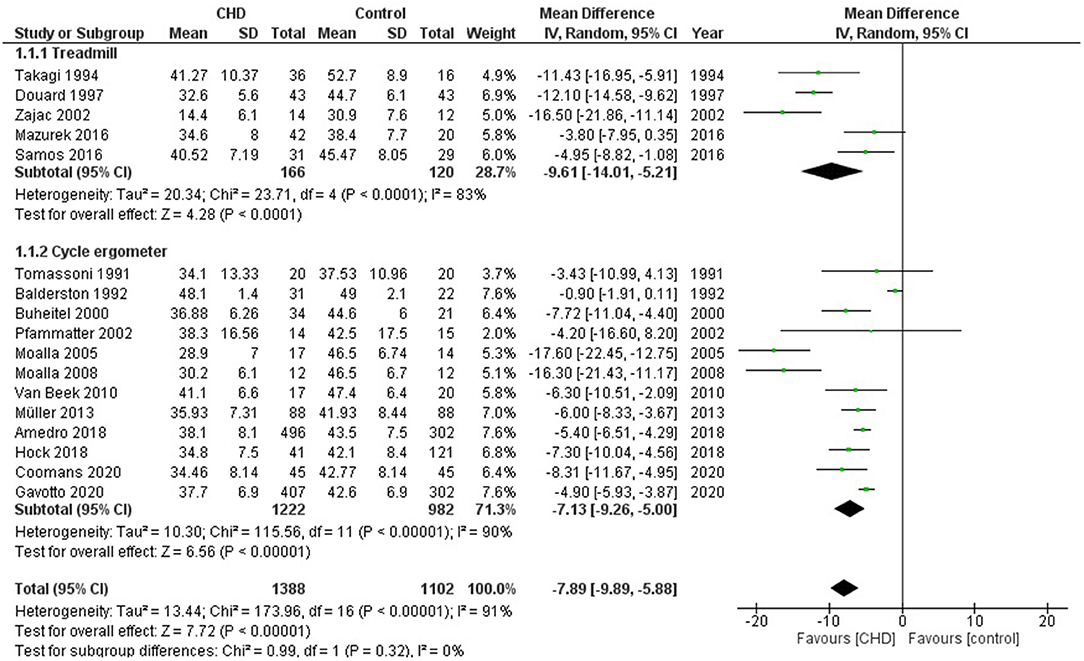
Figure 2. Forest plot for mean peak oxygen consumption for CHD in children and adolescents and healthy controls.
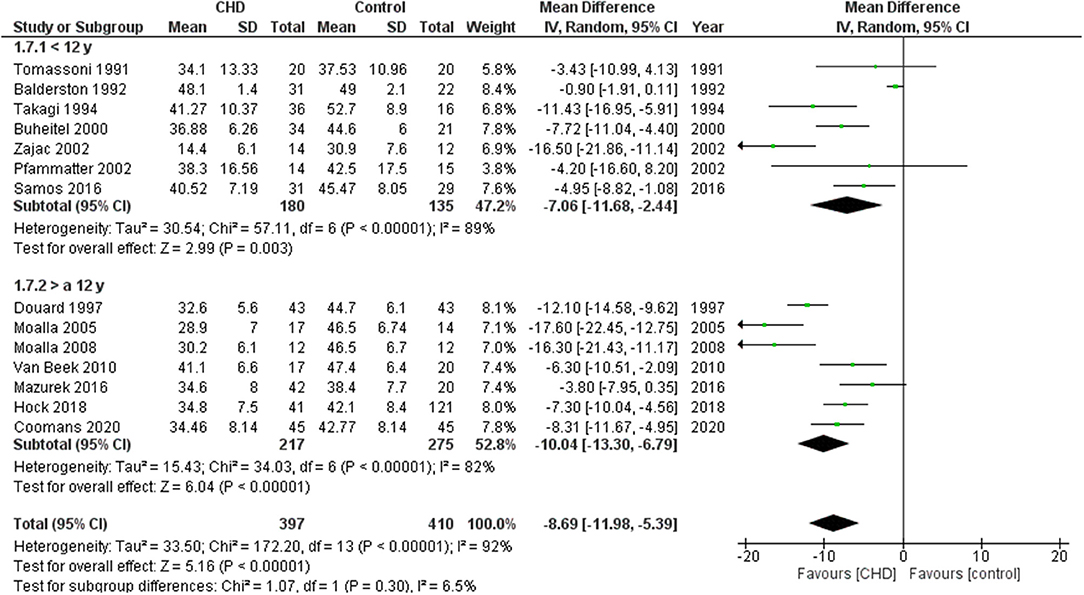
Figure 3. Forest plot for mean peak oxygen consumption for CHD in children and adolescents and healthy controls. Subanalysis by age.
Maximum Workload
Ten studies reported the Wmax in watts (21–24, 27, 30–32, 34, 35). These studies compared 1168 participants with CHD vs. 938 healthy controls. The heterogeneity of the comparison was high (I2 = 96%). Participants with CHD averaged −41.5 Wmax (95% CI: −57.9, −25.1 watts) compared with controls (p < 0.00001) (Figure 4). If we analyze only studies with patients surgically repaired (22, 24, 27, 30, 31, 34, 35) the participants with CHD averaged −49.9 watts (95% CI: −77.2, −22.6) of Wmax compared with controls (p < 0.0003, I2 = 95%) (Supplementary File 2, Figure 2). In addition, we sub-analyzed by age range and observed that the Wmax of the group under 12 years old was similar with the control group [−79.7 watts (95% CI: −229.2, 69.8), p = 0.3, I2 = 98%], and the group ≥ 12 years old had a reduction of −45.5 watts (95% CI: −54.4, −36.7) compared to the control group (p < 0.00001, I2 = 0%) (Figure 5).
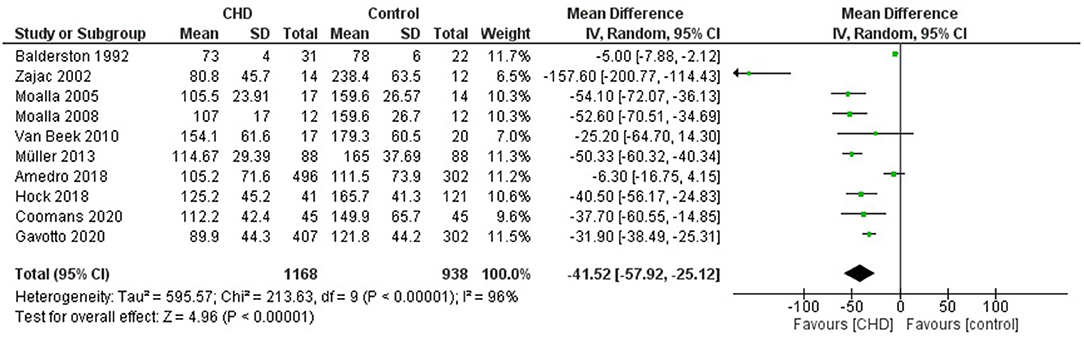
Figure 4. Forest plot for mean maximum workload for CHD in children and adolescents and healthy controls.
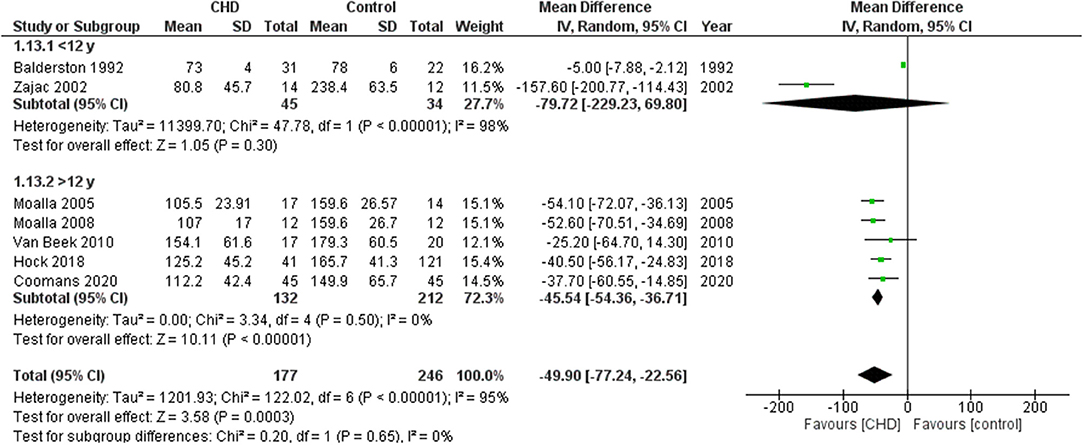
Figure 5. Forest plot for mean maximum workload for CHD in children and adolescents and healthy controls. Subanalysis by age.
VE/VCO2 Slope
Five studies examined the VE/VCO2 slope (19, 22, 32, 35, 36). These studies compared 248 participants with CHD vs. 326 healthy controls. The heterogeneity of the comparison was high (I2 = 92%). Participants with CHD had on average 2.6 more VE/VCO2 slope (95% CI: 0.3, 4.8) compared with controls (p < 0.02) (Figure 6). If we analyse only studies with patients surgically repaired (19, 22, 35, 36) the participants with CHD averaged 3.4 (95% CI: 1.7, 5.1) of VE/VCO2 compared with controls (p < 0.0001, I2 = 77%) (Supplementary File 2, Figure 3).
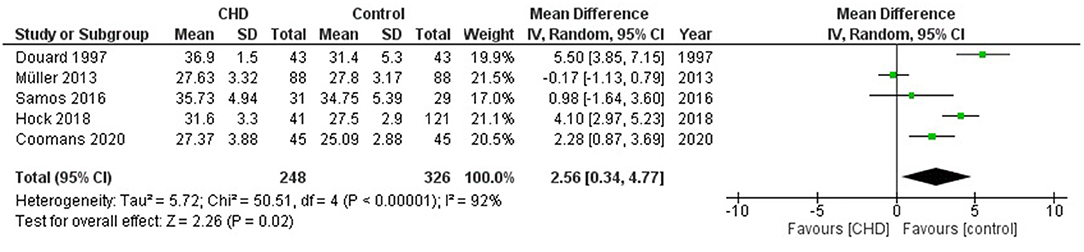
Figure 6. Forest plot for mean ventilatory equivalent for carbon dioxide at anaerobic threshold for CHD in children and adolescents and healthy controls.
Oxygen Pulse
Four studies examined O2 pulse in ml/beat (19, 27, 32, 36). These studies compared 176 participants with CHD vs 171 healthy controls. The heterogeneity of the comparison was high (I2 = 73%). Participants with CHD had on average −2.4 ml/beat of O2 pulse (95% CI: −3.7, −1.1 ml/beat) compared with controls (p < 0.0003) (Figure 7). If we analyse only studies with patients surgically repaired (19, 27, 36) the participants with CHD averaged−3.1 ml/beat (95% CI: −4.0, −2.1) of O2 pulse compared with controls (p < 0.00001, I2 = 6%) (Supplementary File 2, Figure 4).
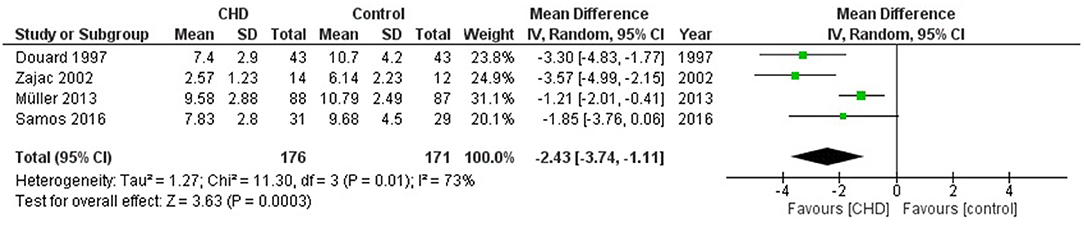
Figure 7. Forest plot for mean oxygen pulse for CHD in children and adolescents and healthy controls.
Maximum Heart Rate
Fifteen studies reported working HRmax in beats per minute (bpm) during CPET (19, 21, 23–27, 30–37). These studies compared 1305 participants with CHD vs. 961 healthy controls. The heterogeneity of the comparison was high (I2 = 67%). Participants with CHD averaged −15 bpm (95% CI: −18, −12 bpm) of HRmax compared with controls (p < 0.00001) (Figure 8). When considering the analysis by subgroups according to the test type, those who performed the test on a treadmill included 124 subjects with CHD and 100 controls, while on the cycle ergometer it was 1181 CHD and 861 controls. Those who performed the test on a treadmill had an average of −24 bpm (95% CI: −37, −11) of HRmax compared with the control group; in contrast, those who performed the test on a cycle ergometer had an average of −14 bpm (95% CI: −17, −11) of HRmax compared with the control group (p < 0.00001). If we analyze only studies with patients surgically repaired (19, 24–27, 30, 31, 33–37) the participants with CHD averaged −17 bpm (95% CI: −23, −12) of HRmax compared with controls (p <0.0001, I2 = 71%) (Supplementary File 2, Figure 5). In addition, we sub-analyzed by age range and observed that the HRmax of the group under 12 years old had a decrease of −14 bpm (95% CI: −22, −6) compared with the control group (p = 0.0004, I2 = 61%), and the group ≥ 12 years old had a reduction of −21 bpm (95% CI: −28, −14) compared with the control group (p < 0.00001, I2 = 82%) (Figure 9).
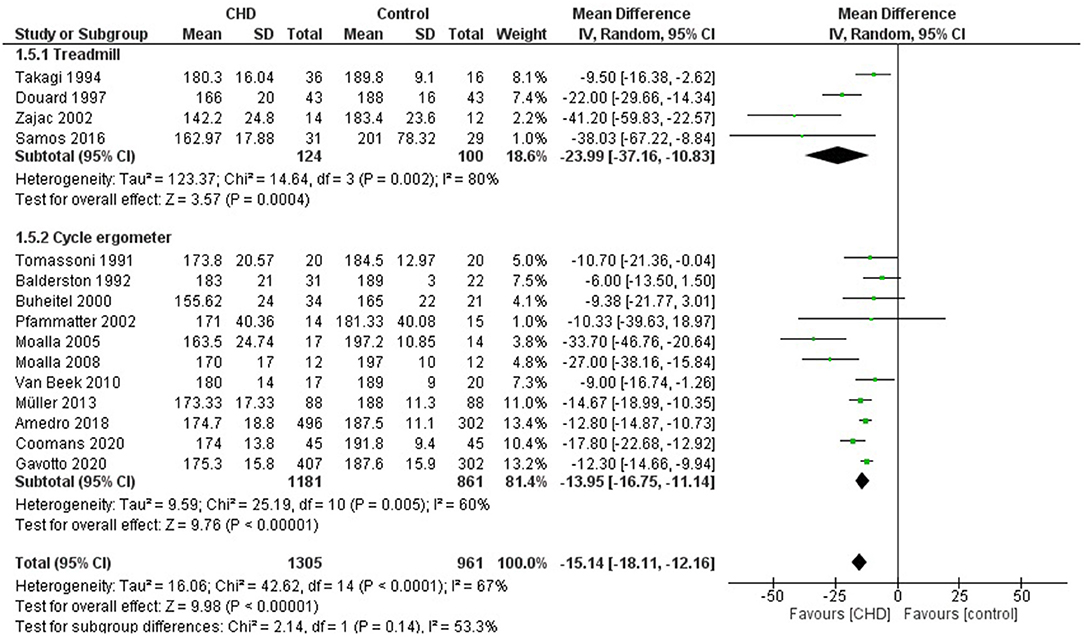
Figure 8. Forest plot for maximum heart rate for CHD in children and adolescents and healthy controls.
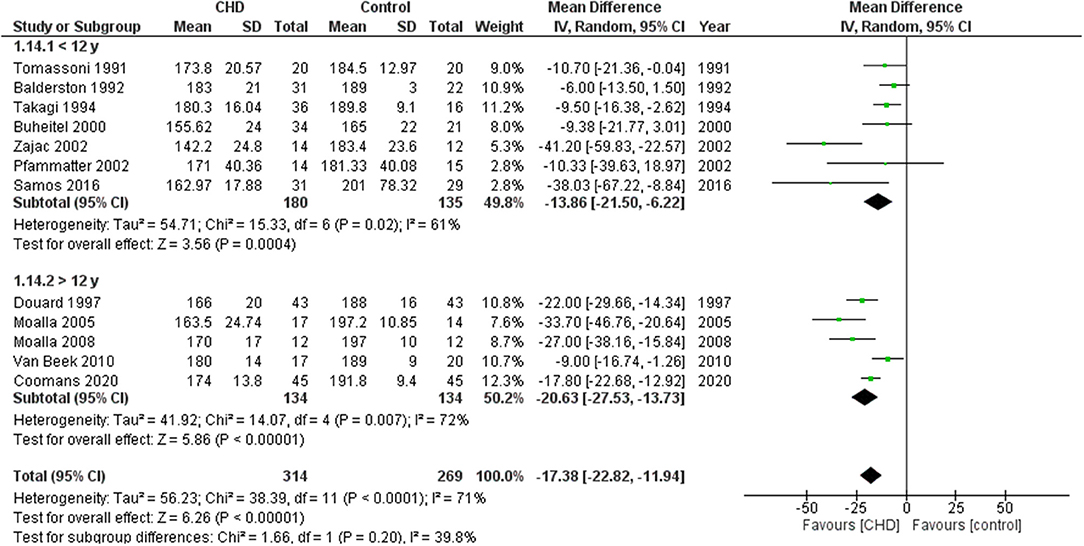
Figure 9. Forest plot for maximum heart rate for CHD in children and adolescents and healthy controls. Subanalysis by age.
Discussion
This systematic review with meta-analysis of observational studies showed that children and adolescents with CHD have a significant decrease in the exercise capacity compared with healthy controls of similar age. VO2peak, HRmax, Wmax, and O2 Pulse were significantly lower in children and adolescents with partially or fully repaired CHD compared with healthy controls.
Our results showed that children and adolescents with CHD have a reduction close to 8 ml/Kg/min, although 76% of the selected articles exclusively evaluated patients with repaired CHD, which shows a considerable residual effect of CHD. The significant impairment of VO2peak directly impacts on exercise capacity, morbidity and mortality and is a prognostic factor in this population, reaching clinical and functional relevance. The presence of pulmonary hypertension (PH) is also a condition that could affect exercise capacity evaluated through VO2peak; however, it was not reported in most articles. On the other hand, one of the few manuscripts which reported it did find that the presence of PH was significantly associated with lower VO2peak (21).
Coomans et al. (35) highlighted that, in patients with repaired Tetralogy of Fallot (TOF), VO2peak and HRmax is lower as compared with controls, attributing, in part, the lower performance to chronotropic insufficiency due to a positive and significant correlation between HRmax and VO2peak (r = 0.418; p < 0.01). Additionally, our results showed that patients with CHD, in the included studies, have a diminution close to 15 bpm compared with healthy peers. The decrease in HRmax is almost double in those who perform the test on a treadmill, which is in line with a greater decrease in VO2peak in this evaluation device. These results highlight the importance of chronotropic insufficiency in maximum exercise performance, limiting the physical performance of individuals with even repaired TOF and being in line with other publications with similar results (35).
The lower HRmax in subjects with CHD was also related to factors affecting the correct function of the sympathetic and parasympathetic nervous system, among which is ischemia and / or denervation resulting from various cardiac surgical procedures or, in cases of cyanotic CHD, due to chronic hypoxemia (39).
Since most of the articles investigated exercise capacity in subjects with repaired heart disease, we decided to perform a subanalysis with only this group. Surprisingly, the surgically repaired subjects had lower VO2peak, Wmax, O2 pulse, and HRmax and higher VE/VCO2. Several factors could influence this result.. On one hand, there could be a selection bias of the investigated subjects since the follow-up is stricter in those subjects who are more seriously sick. On the other hand, participants with surgical correction were more severe than those without surgery (23).
We also analyzed the influence of age on the reduced exercise capacity. Considering adolescents those subjects older than 12 years old, we showed a greater decrease in VO2peak and HRmax compared with children under 12. A special case was Wmax, which was shown to be reduced only in the group older than 12 years. These results lead us to think that the differences increase over the years and are more pronunced during adolescence. Although our research does not include adult patients, there are already reports with decreased exercise capacity similar or even greater than what we found in those over 12 years old (40, 41).
Subjects with incomplete CHD repair have significant reductions in age-adjusted peak work rates and peak ventilation compared with their counterparts who had complete repair surgery (42). Amedro et al. observed that, in 496 children with CHD who underwent CPET compared with controls, VO2peak alteration was more prevalent in most subjects with partial repair or complex CHD (single ventricles and complex anomalies of atrioventricular connections) (21). The lower VO2peak in their study group was also associated with right ventricular systolic hypertension and tricuspid regurgitation, which are frequently common in many right heart complex CHD cases. The literature has also highlighted this situation, especially in patients with TOF, transposition of the great arteries, and univentricular heart with Fontan physiology (43).
Sequential CPET studies in young adult subjects with Fontan physiology have emphasized the prognostic value of this test regarding survival, mortality and the need for transplantation. There is often a decrease in VO2peak that precedes these events (44). Cooney et al. postulate that a change in VO2peak is an independent prognostic factor, which may allow early identification of subjects who could benefit from more intensive and preventive management (44). The change in VO2peak between sequential CPETs predicts transplant-free survival during and above any risk predicted by a single VO2peak measurement. Studies that have followed individuals with Fontan physiology from childhood to adulthood have documented a gradual decline in VO2peak over the years (14, 45). The decrease in VO2peak with age may be a factor to be identified and considered in subjects with CHD as a selection parameter for timely cardiac rehabilitation programmes starting even in the early stages of life (schooling).
The VE/VCO2 slope is elevated in most subjects with heart failure as it is inversely related to CO at peak exercise and to pulmonary perfusion, a situation that could also be experienced in subjects with CHD (46). In addition, the elevation of this slope is commonly observed in pulmonary vascular anomalies such as PH (47), which can also be experienced in patients with partially repaired CHD due to greater physical exertion.
A decrease in the O2 pulse during progressive exercise could indicate circulatory insufficiency or cardiovascular limitation (48). It is generally associated with the appearance of PH and impaired cardiac perfusion. In combination with a sudden decrease in the VO2 / Wmax ratio, it could indicate myocardial ischemia (49). In addition, a low O2 pulse is indicative of a reduced cardiac index (CI) or stroke volume, which implies a greater dependence on HR to increase CO (48, 50), a situation that may be common to certain CHD considered in the different subgroups that constitute the total of subjects with CHD of this revision.
Although our objective was to measure exercise capacity and not physical activity, it is important to note that some articles reported it (31, 32). This is important because a previous investigation of our group has shown that the moderate-to-vigorous physical activity of 46% of children with CHD is less than what is recommended by WHO (51). However, Van Beek et al. and Müller et al. found that exercise capacity is decreased in children with CHD, but physical activity showed no differences between groups (31, 32). Even in Müller's study, both groups performed more physical activity than recommended by clinical guidelines (32).
Finally, although the search period was long, our results consider only studies from the last three decades that explored exercise capacity in a large cohort of children and adolescents with CHD worldwide, which gives high value to our main message.
Limitations
Our study has some limitations. Most of the studies included subjects with different types of CHD, which may limit the extrapolation of the results and recommendations to the entire spectrum of children and adolescents with CHD; even though our results were statistically significant. The heterogeneous nature of CHD implies that many lesions have different pathophysiological behaviors and conditions, a wide spectrum of severity, as well as the implication or impact that suffering from associated comorbidities may influence the congenital health condition they may have. Another important limitation is that most studies do not report whether patients presented PH. This point is key since it is well known that PH determines lower exercise capacity. In this context, it is important to systematically review associations in order to establish in more detail the factors that can condition a certain result.
Additionally, there was heterogeneity in the control participants since some of them performed the CPET to get the authorization to play sports (22) and others who presented any symptoms although the CPET showed no disease (21, 23, 35). On the other hand, physical activity analysis was not included, which could have provided us information on how sedentary the population was, and were not evaluated respiratory exchange ratio (RER) that indirectly shows the muscle's oxidative capacity to get energy. Sedentarism, exercise and physically active lifestyles modify it.
Concerning the possible bias occurred in VO2peak and other CPET variables due to variability in different countries (with different daily levels of physical activity, for example, due to different cultural habits) it is important to remark that the comparisons of these parameters, both for the studies and the meta-analysis, were performed comparing patients to controls (adjusted for sex and age in each article) and not regarding their percentages of the predicted values. Therefore, using a control population was essential to avoid these biases.
Conclusion
In conclusion, suffering from CHD in childhood and adolescence is associated with a lower exercise capacity as shown by worse VO2peak, Wmax, VE/VCO2 slope, O2 pulse, HRmax compared with healthy controls, not only in CPET but also in other variables as shown in indirect exercise capacity tests. These findings highlight the importance of carrying out a continuous evaluation and early determination of the factors associated with a potential decrease in exercise capacity. In this way, it would be possible to intervene in time by planning rehabilitation programmes and promoting an active lifestyle, since a lower exercise capacity can lead to a greater risk of morbidity, mortality, and deterioration of functionality.
Data Availability Statement
The original contributions presented in the study are included in the article/Supplementary Material, further inquiries can be directed to the corresponding author.
Author Contributions
YV-R: conceptualization, formal analysis, methodology, reviewing procedure and data extraction, writing—original draft, and writing—review & editing. JV-M: conceptualization, formal analysis, methodology, writing—original draft, and writing—review & editing. RT-C: conceptualization, formal analysis, methodology, supervision, writing—original draft, and writing—review & editing. LV-C: reviewing procedure and data extraction, writing—original draft, and writing—review & editing. GM: supervision and writing—review & editing. JV and IB: writing —original draft and writing—review & editing. All authors contributed to the article and approved the submitted version.
Funding
This study has been funded by Instituto de Salud Carlos III (ISCIII) through the projects (PI17/01515 and PI21/00555) and co-funded by the European Union. RT-C was funded by a grant from the National Agency for Research and Development (ANID)/Scholarship Program/DOCTORADO BECAS CHILE/2018-72190117.
Conflict of Interest
The authors declare that the research was conducted in the absence of any commercial or financial relationships that could be construed as a potential conflict of interest.
Publisher's Note
All claims expressed in this article are solely those of the authors and do not necessarily represent those of their affiliated organizations, or those of the publisher, the editors and the reviewers. Any product that may be evaluated in this article, or claim that may be made by its manufacturer, is not guaranteed or endorsed by the publisher.
Acknowledgments
RT-C is a Ph.D. candidate in Methodology of Biomedical Research and Public Health Program, at Universitat Autònoma de Barcelona, Barcelona, Spain.
Supplementary Material
The Supplementary Material for this article can be found online at: https://www.frontiersin.org/articles/10.3389/fcvm.2022.874700/full#supplementary-material
Abbreviations
6MWT, Six-minute walking test; CHD, Congenital heart disease; CI, Cardiac index; CO, Cardiac output; CPET, Cardiopulmonary exercise test; HRmax, Maximum Heart rate; NHLBI, National Heart, Lung and Blood Institute; PH, Pulmonary hypertension; PRISMA, Preferred reporting items for systematic reviews and meta-analyses; PROSPERO, International prospective register of systematic reviews; RCTs, Randomized clinical trials; SV, Stroke volume; SWT, Shuttle walking test; TUG, Timed up and go test; VE, Minute ventilation; VE/VCO2, Ventilatory equivalent for carbon dioxide; VE/VCO2 slope, Minute ventilation/carbon dioxide production slope; VO2, Oxygen consumption; VO2peak, Peak oxygen consumption; VO2max, Maximum oxygen consumption; Wmax, Maximum workload.
References
1. Puri K, Allen HD, Qureshi AM. Congenital heart disease. Pediatr Rev. (2017) 38:471–86. doi: 10.1542/pir.2017-0032
2. Liu Y, Chen S, Zühlke L, Black GC, Choy M, Li N, et al. Global birth prevalence of congenital heart defects 1970–2017: updated systematic review and meta-analysis of 260 studies. Int J Epidemiol. (2019) 48:455–63. doi: 10.1093/ije/dyz009
3. Best KE, Rankin J. Long-Term survival of individuals born with congenital heart disease: a systematic review and meta-analysis. J Am Hear Assoc Cardiovasc Cerebrovasc Dis. (2016) 5: e002846. doi: 10.1161/JAHA.115.002846
4. Huisenga D, Gemert SLB-V, Bergen A Van, Sweeney J, Hadders-Algra M. Developmental outcomes after early surgery for complex congenital heart disease: a systematic review and meta-analysis. Dev Med Child Neurol. (2021) 63:29–46. doi: 10.1111/dmcn.14512
5. Marelli AJ, Ionescu-Ittu R, Mackie AS, Guo L, Dendukuri N, Kaouache M. Lifetime prevalence of congenital heart disease in the general population from 2000 to 2010. Circulation. (2014) 130:749–56. doi: 10.1161/CIRCULATIONAHA.113.008396
6. Schaan CW, Macedo ACP de, Sbruzzi G, Umpierre D, Schaan BD, Pellanda LC. Functional capacity in congenital heart disease: a systematic review and meta-analysis. Arq Bras Cardiol. (2017) 109:357. doi: 10.5935/abc.20170125
7. Dulfer K, Helbing WA, Duppen N, Utens EM. Associations between exercise capacity, physical activity, and psychosocial functioning in children with congenital heart disease: a systematic review. Eur J Prev Cardiol. (2014) 21:1200–15. doi: 10.1177/2047487313494030
8. Stefan MA, Hopman WM, Smythe JF. Effect of activity restriction qwing to heart disease on obesity. Arch Pediatr Adolesc Med. (2005) 159:477–81. doi: 10.1001/archpedi.159.5.477
9. Barker K, Eickmeyer S. Therapeutic exercise. Med Clin North Am. (2020) 104:189–98. doi: 10.1016/j.mcna.2019.10.003
10. Takken T, Bongers BC, Brussel M. van, Haapala EA, Hulzebos EHJ. Cardiopulmonary exercise testing in pediatrics. Ann ATS. (2017) 14:S123–8. doi: 10.1513/AnnalsATS.201611-912FR
11. Swank AM, Horton J, Fleg JL, Fonarow GC, Keteyian S, Goldberg L, et al. Modest increase in peak VO2 is related to better clinical outcomes in chronic heart failure patients: results from heart failure and a controlled trial to investigate outcomes of exercise training (HF-ACTION). Circ Heart Fail. (2012) 5:579. doi: 10.1161/CIRCHEARTFAILURE.111.965186
12. Giardini A, Specchia S, Tacy TA, Coutsoumbas G, Gargiulo G, Donti A, et al. Usefulness of cardiopulmonary exercise to predict long-term prognosis in adults with repaired tetralogy of fallot. Am J Cardiol. (2007) 99:1462–7. doi: 10.1016/j.amjcard.2006.12.076
13. Giardini A, Hager A, Lammers AE, Derrick G, Müller J, Diller GP, et al. Ventilatory efficiency and aerobic capacity predict event-free survival in adults with atrial repair for complete transposition of the great arteries. J Am Coll Cardiol. (2009) 53:1548–55. doi: 10.1016/j.jacc.2009.02.005
14. Fernandes SM, Alexander ME, Graham DA, Khairy P, Clair M, Rodriguez E, et al. Exercise testing identifies patients at increased risk for morbidity and mortality following fontan surgery. Congenit Heart Dis. (2011) 6:294–303. doi: 10.1111/j.1747-0803.2011.00500.x
15. Moher D, Liberati A, Tetzlaff J, Altman DG, Altman D, Antes G, et al. Preferred reporting items for systematic reviews and meta-analyses: the PRISMA statement. Ann Intern Med. (2009) 151:264–9. doi: 10.7326/0003-4819-151-4-200908180-00135
16. Ouzzani M, Hammady H, Fedorowicz Z, Elmagarmid A. Rayyan-a web and mobile app for systematic reviews. Syst Rev. (2016) 5. doi: 10.1186/s13643-016-0384-4
17. National Heart Lung Blood Institute. Quality Assessment Tool for Observational Cohort and Cross-Sectional Studies. Available online at: https://www.nhlbi.nih.gov/health-topics/study-quality-assessment-tools [accessed May 25, 2020].
18. Bowyer JJ, Busst CM, Till JA, Lincoln C, Shinebourne EA. Exercise ability after mustard's operation. Arch Dis Child. (1990) 65:865. doi: 10.1136/adc.65.8.865
19. Douard H, Labbé L, Barat JL, Broustet JP, Baudet E, Choussat A. Cardiorespiratory response to exercise after venous switch operation for transposition of the great arteries. Chest. (1997) 111:23–9. doi: 10.1378/chest.111.1.23
20. Mazurek B, Szydlowski L, Mazurek M, Markiewicz-Loskot G, Pajak J, Morka A. Comparison of the degree of exercise tolerance in children after surgical treatment of complex cardiac defects, assessed using ergospirometry and the level of brain natriuretic peptide. Med (United States). (2016) 95:1–6. doi: 10.1097/MD.0000000000002619
21. Amedro P, Gavotto A, Guillaumont S, Bertet H, Vincenti M, De La Villeon G, et al. Cardiopulmonary fitness in children with congenital heart diseases versus healthy children. Heart. (2018) 104:1026–36. doi: 10.1136/heartjnl-2017-312339
22. Hock J, Reiner B, Neidenbach RC, Oberhoffer R, Hager A, Ewert P, et al. Functional outcome in contemporary children with total cavopulmonary connection – health-related physical fitness, exercise capacity and health-related quality of life. Int J Cardiol. (2018) 255:50–4. doi: 10.1016/j.ijcard.2017.11.092
23. Gavotto A, Vandenberghe D, Abassi H, Huguet H, Macioce V, Picot M-C, et al. Oxygen uptake efficiency slope: a reliable surrogate parameter for exercise capacity in healthy and cardiac children? Arch Dis Child. (2020) 105:1167–74. doi: 10.1136/archdischild-2019-317724
24. Moalla W, Gauthier R, Maingourd Y, Ahmaidi S. Six-Minute walking test to assess exercise tolerance and cardiorespiratory responses during training program in children with congenital heart disease. Int J Sports Med. (2005) 26:756–62. doi: 10.1055/s-2004-830558
25. Buheitel G, Hofbeck M, Gerling S, Koch A, Singer H. Similarities and differences in the exercise performance of patients after a modified fontan procedure compared to patients with complete transposition following a Senning operation. Cardiol Young. (2000) 10:201–7. doi: 10.1017/S1047951100009112
26. Pfammatter J-P, Zanolari M, Schibler A. Cardiopulmonary exercise parameters in children with atrial septal defect and increased pulmonary blood flow: short-term effects of defect closure. Acta Pædiatrica. (2002) 91:65–70. doi: 10.1111/j.1651-2227.2002.tb01643.x
27. Zajac A, Tomkiewicz L, Podolec P, Tracz W, Malec E. Cardiorespiratory response to exercise in children after modified Fontan operation. Scand Cardiovasc J. (2002) 36:80–5. doi: 10.1080/140174302753675348
28. Norozi K, Gravenhorst V, Hobbiebrunken E, Wessel A. Normality of cardiopulmonary capacity in children operated on to correct congenital heart defects. Arch Pediatr Adolesc Med. (2005) 159:1063–8. doi: 10.1001/archpedi.159.11.1063
29. Binkhorst M, Belt T. van de, Hoog M de, Dijk A van, Schokking M, Hopman M. Exercise capacity and participation of children with a ventricular septal defect. Am J Cardiol. (2008) 102:1079–84. doi: 10.1016/j.amjcard.2008.05.063
30. Moalla WM, Dupont GD, Temfemo AT, Maingourd YM, Weston MW, Ahmaidi SA. Assessment of exercise capacity and respiratory muscle oxygenation in healthy children and children with congenital heart diseases. Appl Physiol Nutr Metab. (2008) 33:434–40. doi: 10.1139/H07-196
31. Beek E van, Binkhorst M, Hoog M de, Groot P de, Dijk A van, Schokking M, et al. Exercise performance and activity level in children with transposition of the great arteries treated by the arterial switch operation. Am J Cardiol. (2010) 105:398–403. doi: 10.1016/j.amjcard.2009.09.048
32. Müller J, Böhm B, Semsch S, Oberhoffer R, Hess J, Hager A. Currently, children with congenital heart disease are not limited in their submaximal exercise performance. Eur J Cardio-Thoracic Surg. (2013) 43:1096–100. doi: 10.1093/ejcts/ezs712
33. Tomassoni TL, Galioto FM, Vaccaro P. Cardiopulmonary exercise testing in children following surgery for tetralogy of Fallot. Am J Dis Child. (1991) 145:1290–3. doi: 10.1001/archpedi.1991.02160110082025
34. Balderston SM, Daberkow E, Clarke DR, Wolfe RR. Maximal voluntary exercise variables in children with postoperative coarctation of the aorta. J Am Coll Cardiol. (1992) 19:154–8. doi: 10.1016/0735-1097(92)90067-W
35. Coomans I, Kinder S De, Belleghem H Van, Groote K De, Panzer J, Wilde H De, et al. Analysis of the recovery phase after maximal exercise in children with repaired tetralogy of Fallot and the relationship with ventricular function. PLoS ONE. (2020) 15: 244312. doi: 10.1371/journal.pone.0244312
36. Samos F, Fuenmayor G, Hossri C, Elias P, Ponce L, Souza R, et al. Exercise capacity long-term after arterial switch operation for transposition of the great arteries. Congenit Heart Dis. (2016) 11:155–9. doi: 10.1111/chd.12303
37. Takagi J. A new “Complex Exercise Test” for children with cardiac diseases. Kurume Med J. (1994) 41:97–107. doi: 10.2739/kurumemedj.41.97
38. Kotby AA, Elnabawy HM, El-Guindy WM, Elaziz RFA. Assessment of exercise testing after repair of tetralogy of fallot. ISRN Pediatr. (2012) 2012:324306. doi: 10.5402/2012/324306
39. Massin MM, Dessy H, Malekzadeh-Milani S-G, Khaldi K, Topac B, Edelman R. Chronotropic impairment after surgical or percutaneous closure of atrial septal defect. Catheter Cardiovasc Interv. (2009) 73:564–7. doi: 10.1002/ccd.21857
40. Trojnarska O, Gwizdała A, Katarzyński S, Katarzyńska A, Szyszka A, Lanocha M, et al. Evaluation of exercise capacity with cardiopulmonary exercise test and B-type natriuretic peptide in adults with congenital heart disease. Cardiol J. (2009) 16:133–41.
41. Eckerström F, Rex CE, Maagaard M, Heiberg J, Rubak S, Redington A, et al. Cardiopulmonary dysfunction in adults with a small, unrepaired ventricular septal defect: a long-term follow-up. Int J Cardiol. (2020) 306:168–74. doi: 10.1016/j.ijcard.2020.02.069
42. Rosenblum O, Katz U, Reuveny R, Williams CA, Dubnov-Raz G. Exercise performance in children and young adults after complete and incomplete repair of congenital heart disease. Pediatr Cardiol. 36:1573–81. doi: 10.1007/s00246-015-1198-1
43. Trojnarska O, Gwizdała A, Katarzyński S, Katarzyńska A, Oko-Sarnowska Z, Breborowicz P, et al. Evaluation of exercise capacity with cardiopulmonary exercise testing and BNP levels in adult patients with single or systemic right ventricles. Arch Med Sci. (2010) 6:192. doi: 10.5114/aoms.2010.13893
44. Cooney SJ, Campbell K, Wolfe K, DiMaria M V., Rausch CM. Is neurodevelopment related to exercise capacity in single ventricle patients who have undergone fontan palliation? Pediatr Cardiol. (2020) 42:408–16. doi: 10.1007/s00246-020-02497-7
45. Müller J, Christov F, Schreiber C, Hess J, Hager A. Exercise capacity, quality of life, and daily activity in the long-term follow-up of patients with univentricular heart and total cavopulmonary connection. Eur Heart J. (2009) 30:2915–20. doi: 10.1093/eurheartj/ehp305
46. Kaafarani M, Schroer C, Takken T. Reference values for blood pressure response to cycle ergometry in the first two decades of life: comparison with patients with a repaired coarctation of the aorta. Expert Rev Cardiovasc Ther. (2017) 15:945–51. doi: 10.1080/14779072.2017.1385392
47. Klaassen SHC, Liu LCY, Hummel YM, Damman K, van der Meer P, Voors AA, et al. Clinical and hemodynamic correlates and prognostic value of VE/VCO2 slope in patients with heart failure with preserved ejection fraction and pulmonary hypertension. J Card Fail. (2017) 23:777–82. doi: 10.1016/j.cardfail.2017.07.397
48. Torres-Castro R, Gimeno-Santos E, Vilaró J, Roqué-Figuls M, Moisés J, Vasconcello-Castillo L, et al. Effect of pulmonary hypertension on exercise tolerance in patients with COPD: a prognostic systematic review and meta-analysis. Eur Respir Rev. (2021) 30:200321. doi: 10.1183/16000617.0321-2020
49. Belardinelli R, Lacalaprice F, Tiano L, Muçai A, Perna GP. Cardiopulmonary exercise testing is more accurate than ECG-stress testing in diagnosing myocardial ischemia in subjects with chest pain. Int J Cardiol. (2014) 174:337–42. doi: 10.1016/j.ijcard.2014.04.102
50. Unnithan V, Rowland TW. Use of oxygen pulse in predicting doppler-derived maximal stroke volume in adolescents. Pediatr Exerc Sci. (2015) 27:412–8. doi: 10.1123/pes.2014-0215
Keywords: pediatrics, heart defects, congenital malformations, cardiopulmonary exercise test, oxygen consumption, six-minute walking test
Citation: Villaseca-Rojas Y, Varela-Melo J, Torres-Castro R, Vasconcello-Castillo L, Mazzucco G, Vilaró J and Blanco I (2022) Exercise Capacity in Children and Adolescents With Congenital Heart Disease: A Systematic Review and Meta-Analysis. Front. Cardiovasc. Med. 9:874700. doi: 10.3389/fcvm.2022.874700
Received: 12 February 2022; Accepted: 29 March 2022;
Published: 04 May 2022.
Edited by:
Anselm Uebing, University of Kiel, GermanyReviewed by:
Jannos Siaplaouras, Independent researcher, Fulda, GermanyVibeke Hjortdal, University of Copenhagen, Denmark
Copyright © 2022 Villaseca-Rojas, Varela-Melo, Torres-Castro, Vasconcello-Castillo, Mazzucco, Vilaró and Blanco. This is an open-access article distributed under the terms of the Creative Commons Attribution License (CC BY). The use, distribution or reproduction in other forums is permitted, provided the original author(s) and the copyright owner(s) are credited and that the original publication in this journal is cited, in accordance with accepted academic practice. No use, distribution or reproduction is permitted which does not comply with these terms.
*Correspondence: Javiera Varela-Melo, amF2aWVyYS52YXJlbGEubUBnbWFpbC5jb20=
†ORCID: Yenny Villaseca-Rojas orcid.org/0000-0003-0421-3487
Javiera Varela-Melo orcid.org/0000-0002-6912-8817
Rodrigo Torres-Castro orcid.org/0000-0001-7974-4333
Luis Vasconcello-Castillo orcid.org/0000-0003-0405-3831
Guillermo Mazzucco orcid.org/0000-0003-3937-2939
Jordi Vilaró orcid.org/0000-0002-2150-8992
Isabel Blanco orcid.org/0000-0001-9452-3432