- 1Clinical Research Domain, Baker Heart and Diabetes Institute, Melbourne, VIC, Australia
- 2Institute of Physical Activity and Nutrition, School of Exercise and Nutrition Sciences, Deakin University, Geelong, VIC, Australia
- 3Malignant Haematology and Stem Cell Transplantation Service, Alfred Hospital, Melbourne, VIC, Australia
- 4CSL Ltd, Melbourne, VIC, Australia
Introduction: Allogeneic hematopoietic cell transplantation (allo-HCT) offers a potential cure for high-risk hematological malignancy; however, long-term survivors experience increased cardiovascular morbidity and mortality. It is unclear how allo-HCT impacts cardiovascular function in the short-term. Thus, this 3-month prospective study sought to evaluate the short-term cardiovascular impact of allo-HCT in hematological cancer patients, compared to an age-matched non-cancer control group.
Methods: Before and ~3-months following allo-HCT, 17 hematological cancer patients (45 ± 18 years) underwent cardiopulmonary exercise testing to quantify peak oxygen uptake (VO2peak)—a measure of integrative cardiovascular function. Then, to determine the degree to which changes in VO2peak are mediated by cardiac vs. non-cardiac factors, participants underwent exercise cardiac MRI (cardiac reserve), resting echocardiography (left-ventricular ejection fraction [LVEF], global longitudinal strain [GLS]), dual-energy x-ray absorptiometry (lean [LM] and fat mass [FM]), blood pressure (BP) assessment, hemoglobin sampling, and arteriovenous oxygen difference (a-vO2diff) estimation via the Fick equation. Twelve controls (43 ± 13 years) underwent identical testing at equivalent baseline and 3-month time intervals.
Results: Significant group-by-time interactions were observed for absolute VO2peak (p = 0.006), bodyweight-indexed VO2peak (p = 0.015), LM (p = 0.001) and cardiac reserve (p = 0.019), which were driven by 26, 24, 6, and 26% reductions in the allo-HCT group (all p ≤ 0.001), respectively, as no significant changes were observed in the age-matched control group. No significant group-by-time interactions were observed for LVEF, GLS, FM, hemoglobin, BP or a-vO2diff, though a-vO2diff declined 12% in allo-HCT (p = 0.028).
Conclusion: In summary, allo-HCT severely impairs VO2peak, reflecting central and peripheral dysfunction. These results indicate allo-HCT rapidly accelerates cardiovascular aging and reinforces the need for early preventive cardiovascular intervention in this high-risk group.
Introduction
Hematological malignancies accounted for 1.28 million (6.6%) and 711, 840 (7.1%) cancer diagnoses and deaths globally in 2020 (1). Accordingly, allogeneic hematopoietic cell transplantation (allo-HCT) rates to manage these malignancies have more than doubled between 2006–2016 (2). This increase in allo-HCT, combined with advances in human leukocyte antigen-matched donor selection, graft-vs.-host disease (GvHD) prevention and management, and supportive care have contributed to a progressive growth in long-term cancer survivors (3, 4). However, the curative potential of allo-HCT continues to be offset by significant cardiovascular morbidity and mortality. Indeed, compared to age-matched non-cancer controls, long-term allo-HCT survivors (≥2 y) experience elevated rates of cardiovascular disease (CVD) (5–9) and serious cardiovascular events (9–11), culminating in a 2-to-4-fold increased risk of premature cardiovascular mortality (11, 12). These data provide compelling evidence of an accelerated cardiovascular aging phenotype among allo-HCT survivors and have sparked a call for studies aimed at understanding allo-HCT-induced CVD in order to inform efficacious preventive intervention (13).
The paradigm explaining the deterioration in cardiovascular health among allo-HCT survivors suggests there are multiple contributing factors including: the cancer itself (14); anti-cancer therapies (15); prolonged bedrest resulting in muscle loss and physical deconditioning (16); and the inflammatory perturbations of allografting which is exacerbated by GvHD and its prophylaxis/treatment (17, 18). Importantly, evidence extrapolated from studies with overlapping exposures suggests these insults are particularly deleterious to the heart (15–17), but also impact the entire cardiovascular-hematological-skeletal muscle axis (15–21). However, despite evidence of accelerated cardiovascular aging in long-term allo-HCT survivors (e.g., premature onset of overt CVD and related mortality), few studies have prospectively characterized the short-term cardiovascular impact of allo-HCT. Further, the cardiovascular impact of allo-HCT has been minimally characterized using sensitive biomarkers or state-of-the-art, high-resolution physiological testing at any point of the allo-HCT survivorship continuum. Therefore, the clinical trajectory and pathogenesis of allo-HCT related CVD remains unclear—two factors integral for informing the design (e.g., type and timing) of efficacious cardiovascular intervention.
Early detection of cancer treatment-related cardiac dysfunction is critical to facilitate prompt intervention and more effectively prevent irreversible damage and long-term morbidity. Accordingly, the application of exercise stress for the quantification of cardiovascular reserve (defined as the increase in cardiovascular function from rest to peak exercise) has emerged as an efficacious approach in unmasking subclinical cardiovascular pathology (22–24), and predicting all-cause, cardiovascular, and cancer-specific mortality (25–27). Cardiovascular reserve can be evaluated via a specific approach using exercise cardiac magnetic resonance imaging (exercise CMR) to directly quantify cardiac reserve (ability to augment cardiac output during exercise), or an integrative approach using cardiopulmonary exercise testing (CPET) for the assessment of peak oxygen uptake (VO2peak). Importantly, beyond capturing cardiac reserve, VO2peak also encapsulates the integrative function of non-cardiac, “peripheral” organ systems (hematological, vascular, skeletal muscle), which play an important role in the pathogenesis and pathophysiology of CVD (28–31), and are postulated to be impaired by allo-HCT. Hence, early cardiovascular follow-up with exercise-based measures that can provide a more accurate and comprehensive characterization of central and peripheral organ functioning may aid in guiding improved diagnostic and therapeutic approaches necessary to prevent long-term cardiovascular morbidity in this high-risk patient group.
Therefore, this 3-month prospective study sought to evaluate the short-term cardiovascular impact of allo-HCT, assessed primarily as VO2peak and cardiac reserve, with direct comparison to an untreated age-matched non-cancer control group.
Methods
Study population and design
We performed a prospective cohort study comparing adults with hematological cancer scheduled for allo-HCT and age-matched non-cancer controls. Allo-HCT patients were recruited via direct referral from the Alfred Health HCT coordinators in Melbourne, Australia. Controls were recruited from the community who responded to advertisements seeking ostensibly healthy adults. Exclusion criteria for both groups included: (1) age <18 years, (2) inability to speak/understand English, and (3) known contraindications to CPET or CMR (i.e., injury, pacemaker, implanted metallic foreign body or device). Additional exclusion criteria for controls included: (1) BMI ≥35 kg.m−2, (2) presence of a significant underlying medical condition(s), and (3) participation in ≥150-min of moderate intensity or ≥75-min of vigorous intensity aerobic physical activity per week.
Study protocol and experimental measurements
Participants underwent a comprehensive battery of physiological testing on two occasions. The allo-HCT group underwent testing prior to [median [IQR], 16 (11–27) days], and ~3-months following allo-HCT, while controls underwent identical testing, on two time-points, ~3-months apart. Participants were asked to refrain from moderate to vigorous intensity physical activity in the 24-h preceding testing and abstain from alcohol and caffeine on the day of testing.
Participant medical history
A complete medical history of the allo-HCT patients was obtained from the Alfred Health Clinical Database and information relating to diagnosis, cardiovascular risk profile, prior treatment history, allo-HCT (donor, graft source, conditioning intensity, GvHD prophylaxis, GvHD status) and current medication use were recorded. A general lifestyle questionnaire was administered to controls to obtain information relating to current health status and relevant medical history including use of any medications.
Cardiopulmonary fitness
An incremental ramp protocol CPET was conducted on an electronically braked cycle ergometer (Lode Excalibur Sport, Groningen, the Netherlands) for the measurement of VO2peak. Briefly, participants cycled at 10–25 Watts for 1-min, after which, the workload increased at a progressive rate of 10–30 Watts.min−1 until volitional fatigue. The ramp protocol was individualized according to participant age, weight, self-reported exercise capacity, and physical activity history, with the intention of achieving volitional fatigue within 8-to-12-min. Breath-by-breath expired air gas analysis was performed continuously throughout testing using a calibrated metabolic cart (Vyntus CPX, Carefusion, San Diego, USA). Blood pressure (BP) was measured at 2-min intervals using an ECG-gated electrosphygmomanometer BP cuff (Tango M2 Stress Test Monitor and Orbit-K Blood Pressure Cuff, SunTech Medical Inc. Morrisville, USA). Heart rate (HR) and electrical activity were monitored continuously with a 12-lead electrocardiogram (VyntusTM ECG 12-lead PC-ECG, Vyaire Medical, Mettawa, USA). VO2peak was defined as the average of the six consecutive highest 5-sec VO2 values, and percent of age-, height-, weight and sex-predicted VO2peak was calculated according to the FRIEND reference equation (32). VE/VCO2 was assessed from linear regression of VE and VCO2 values as it has been validated as an important prognostic marker in patients with heart failure, independent of VO2peak (33). Contraindications to CPET adhered to the American Thoracic Society recommendations (34). In addition, a lower limit of 80 g.L−1 of hemoglobin was employed in line with clinical hemoglobin transfusion thresholds.
Resting cardiac function
Resting cardiac function was evaluated via echocardiogram (Vivid E95, General Electric Medical Systems, Milwaukee, Wisconsin). Images were collected, saved in a digital format, and analyzed offline (Echopac v13.0.00, GE, Norway) by a trained sonographer. A three-dimensional full-volume dataset was acquired to measure left-ventricular ejection fraction (LVEF). Two-dimensional speckle tracking echocardiography-derived global longitudinal strain (GLS) was quantified from three apical views at a temporal resolution of 60–90 frames.sec−1 with GLS defined as the average negative value of the strain rate curves.
Peak cardiac function and cardiac reserve
The biventricular response to exercise was evaluated using a validated real-time CMR method (35). Exercise was performed within the CMR bore using an electronically braked supine cycle ergometer (MR Ergometer Pedal, Lode, Groningen, the Netherlands). Cardiac images were acquired using a Siemens MAGNETOM Prisma 3.0T CMR with a five-element phased array coil at rest and during exercise at 60% of the maximal power output achieved during CPET as this approximates maximal exercise capacity in supine (35). Real-time steady-state free-precession cine MR imaging was performed without cardiac or respiratory gating at a temporal resolution of 36–38 ms and a three-dimensional stack of 10–18 adjoining 8-mm image slices, encapsulating both ventricle and atria, were acquired in the short axis (SAX) and horizontal long-axis (HLA) planes.
Real-time cine images were analyzed offline in RightVol (KUL, Leuven, Belgium). End-diastole and end-systole were retrospectively marked at end-expiration, and the left- and right-ventricular endocardia (papillary muscles and trabeculations included in the blood pool) were manually contoured on the SAX images, with reference to the atrioventricular valve plane in the HLA. Ventricular volumes were quantified at rest and peak exercise via the summation of disc method. Stroke volume index (SVI) was calculated as the difference between end-diastolic volume and end-systolic volume, indexed to body surface area (BSA), while cardiac index (CI) was calculated as stroke volume multiplied by HR, indexed to BSA. Left- and right-ventricular ejection fractions (LVEF, RVEF) were calculated as SV/end-diastolic volume, multiplied by 100. Cardiac (CI, SVI, HR) and contractile (LVEF, RVEF) reserve were defined as the ability to augment cardiac function from rest to peak exercise (peak values—rest values). Arteriovenous oxygen difference (a-vO2diff) was estimated via the Fick equation using CPET-derived VO2peak and exercise CMR-derived peak cardiac output.
Biochemistry
Blood samples were collected in the morning after an overnight fast to measure hemoglobin concentration, cardiac Troponin-I (cTn-I) and B-natriuretic peptide (BNP).
Anthropometry and body composition
Height (m) and body mass (kg) were assessed and used to calculate BMI and BSA. Total lean mass (LM, kg), fat mass (FM, kg) and percentage body fat (%BF) were quantified using dual-energy X-ray absorptiometry (GE Lunar iDXA, GE Healthcare, Little Chalfont, UK), with scans manually analyzed using enCore software (version 14.10.022).
Blood pressure
After resting in the supine position for 10-min in a quiet room, resting BP and HR were measured in triplicate at the brachial artery using an automated oscillometric BP monitor (OMRON HEM-907, OMRON Corporation, Tokyo, Japan). The average of three measurements was used for analysis.
Definitions of cardiotoxicity and functional disability
Cardiotoxicity was defined using standard echocardiography criteria (15): (1) an absolute reduction in LVEF of >15%, to a value >50%, (2) an absolute reduction in LVEF of >10%, to a value <50% and (3) a >12% relative reduction in GLS. Functional disability was defined as VO2peak <18 ml.kg−1.min−1 as per the American Heart Association Scientific Statement (36).
Sample size
The sample size calculation for the allo-HCT group was based on the reported reduction in VO2peak following 4-weeks of bedrest in healthy individuals (37). Indeed, the pooling of 19 bedrest investigations suggests %Δ VO2peak can be explained by the linear regression = 1.4–0.85 (days), r = −0.73 (37). Considering the average hospital stay for allo-HCT patient is 28 days, a ~22.4% reduction in VO2peak was expected. To account for normal variation in test-retest reproducibility (4.4%), the study was powered to detect an 18% difference between allo-HCT and non-cancer control groups. With estimated standard deviation of 12% (obtained from our study in women treated for breast cancer), 15 allo-HCT and 8 non-cancer control completions were deemed necessary (SD = 12%; 90% power; alpha = 0.05). Sample size was increased ~20% to account for possible drop-out.
Statistical analysis
Analysis was performed using SPSS software (version 24.0, Statistical Package for the Social Sciences, IBM, Chicago, USA). Continuous data were inspected for normality, linearity and homoscedasticity and presented as mean ± SD or mean (95% CI). Categorical data are presented as n (%). Independent t-tests or Fishers exact tests were performed to assess baseline group differences for continuous and categorical variables, respectively. Treatment effects were assessed via generalized linear mixed modeling with covariance structure informed by the Akaike information criteria. The model included time as the repeated measure, group and group-by-time as fixed effects, and participants as random effects. Findings remained unchanged when adjusting for sex, thus, unadjusted results are presented. Within-group changes after 3-months are expressed as mean (95% CI) change from baseline and between-group differences for the mean changes after 3-months [net difference (95% CI)] were calculated by subtracting the within-group changes from baseline for controls from the within-group changes for allo-HCT. CTn-I was transformed to yield a normal distribution before analysis. Two-sided p < 0.05 indicated significance.
Results
Participant characteristics and transplant-related information
Twenty-six individuals scheduled for allo-HCT (17 men, 9 women) and 12 age-matched non-cancer controls (5 men, 7 women) were recruited and completed all baseline assessments. After 3-months, all controls (100%) and 17 (65%; 12 men, 5 women) allo-HCT participants completed follow up (n = 7 deceased, n = 1 declined due to perceived incapacity, n = 1 lost-to-follow-up) and were included in analyses. There were no significant differences in baseline participant characteristics or transplant-related factors between allo-HCT recipients who did and did not complete follow-up (see Supplementary Table S1).
Characteristics of the allo-HCT and control participants who completed follow-up are summarized in Tables 1, 2. There were no significant differences in demographic, anthropometric or traditional cardiovascular risk factors between allo-HCT recipients and controls (Table 1). However, allo-HCT recipients had a significantly lower VO2peak (p < 0.001), cardiac reserve (p = 0.004), LVEF (p = 0.043), and GLS (p = 0.018), relative to controls (Table 1). Acute myeloid leukemia was the most common transplant indication (65%) among allo-HCT recipients, and treatment history was diverse (A more detailed summary of prior treatments is provided in Supplementary Table S1). Sixteen participants (94%) had previous chemotherapy exposure, with anthracycline and anti-metabolite agents most frequently administered (both 82%). With regard to transplant related factors, the most common donor type, graft source, conditioning intensity and GvHD prophylaxis were unrelated, peripheral blood, reduced intensity, and methotrexate/ciclosporine, respectively (Table 2).
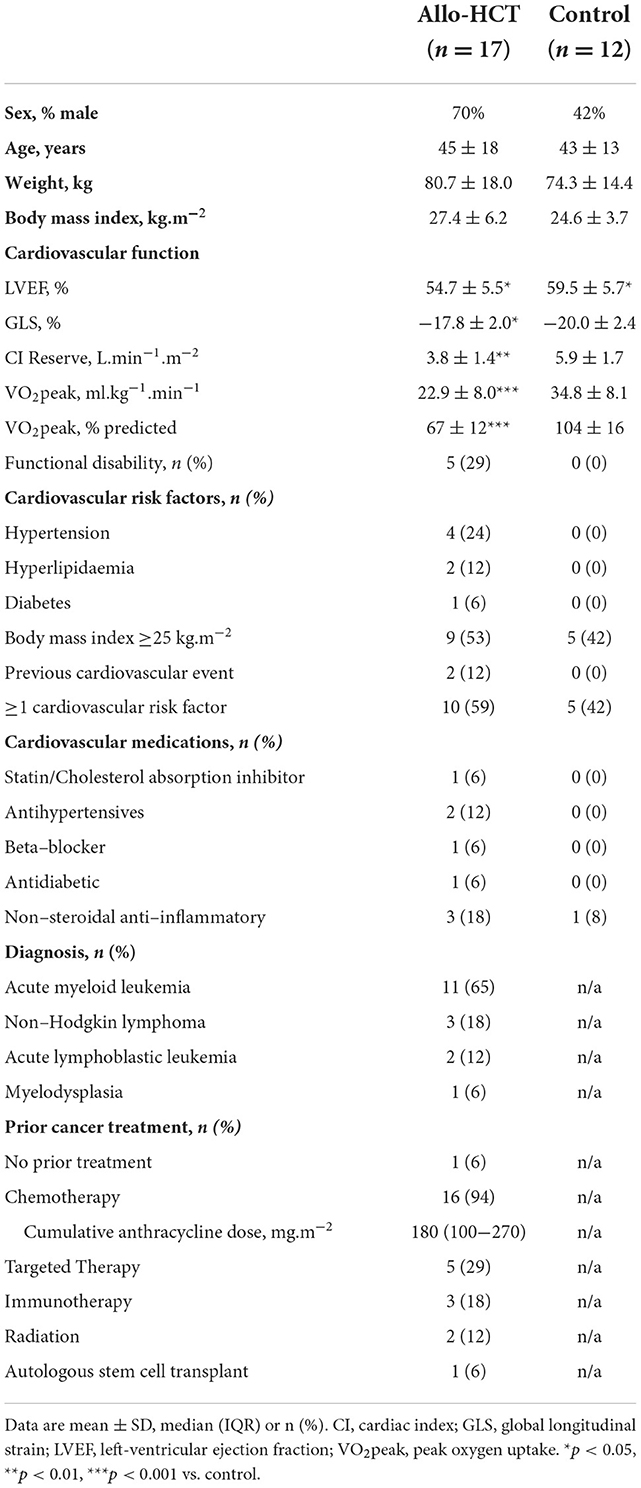
Table 1. Baseline characteristics for Allo-HCT and Control participants who completed baseline and follow-up assessments.
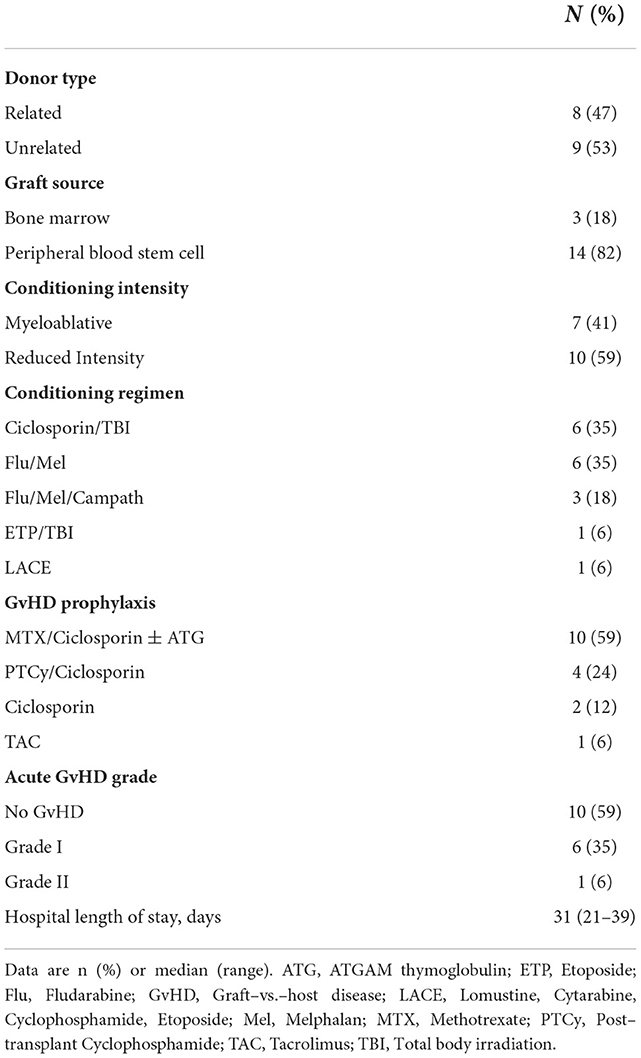
Table 2. Transplant related information for Allo-HCT participants that completed baseline and follow–up assessments.
Exercise capacity
As shown in Figures 1A,B, a significant between-group difference existed for the net change over 3-months for absolute (−0.4 L.min−1 [95% CI −0.7, −0.1]; group-by-time-interaction, p = 0.006) and bodyweight-indexed VO2peak (−4.5 ml.kg−1.min−1 [95% CI −8.1, −0.9]; group-by-time interaction, p = 0.015), due to a 26% (−0.5 L.min−1 [95% CI −0.7, −0.3]; p < 0.001) and 24% (−5.4 ml.kg−1.min−1 [95% CI −7.7, −3.1]; p < 0.001) decline in allo-HCT recipients, respectively, and no significant change in controls. Consequently, allo-HCT recipients achieved a follow-up VO2peak that was, on average, 49% below predicted (-16% from baseline; p < 0.001), with 53% considered functionally disabled. As shown in Table 3, significant between-group differences (group-by-time interactions) were also observed for peak power output (p < 0.001) and percentage of age-predicted HRpeak (p = 0.005), which was driven by a 30% and 11% reduction from baseline in the allo-HCT recipients (both p < 0.001) as no significant change was observed in controls. A similar significant group-by-time interaction was noted for VE/VCO2 slope (p = 0.008), which was due to a 17% increase in allo-HCT recipients (p < 0.001) as there was no change in controls. Peak a-vO2diff declined 12% in allo-HCT recipients (p = 0.028), but a significant between-group difference for the net change from baseline was not observed (interaction, p = 0.23), due to a slight, non-significant downward shift in controls.
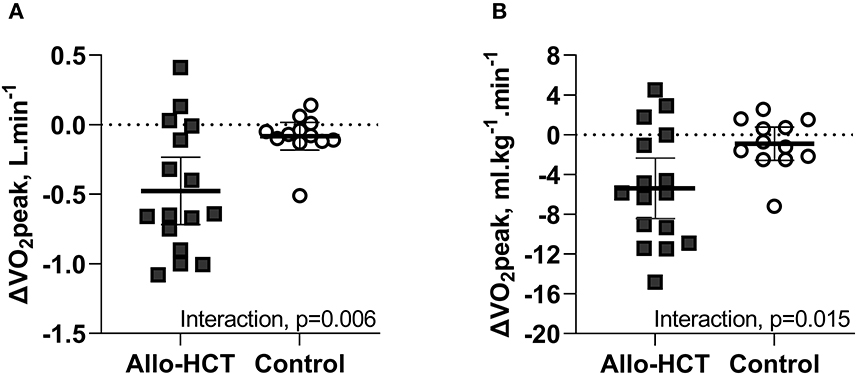
Figure 1. (A,B) Mean (95% CI) 3-month change from baseline in absolute and bodyweight-indexed VO2peak in Allo-HCT and Control assessed by cardiopulmonary exercise testing. There was a significant between-group difference for the net change from baseline for absolute and bodyweight-indexed VO2peak (p = 0.006 and p = 0.015, respectively), which was due to a significant decrease in allo-HCT and no change in controls.
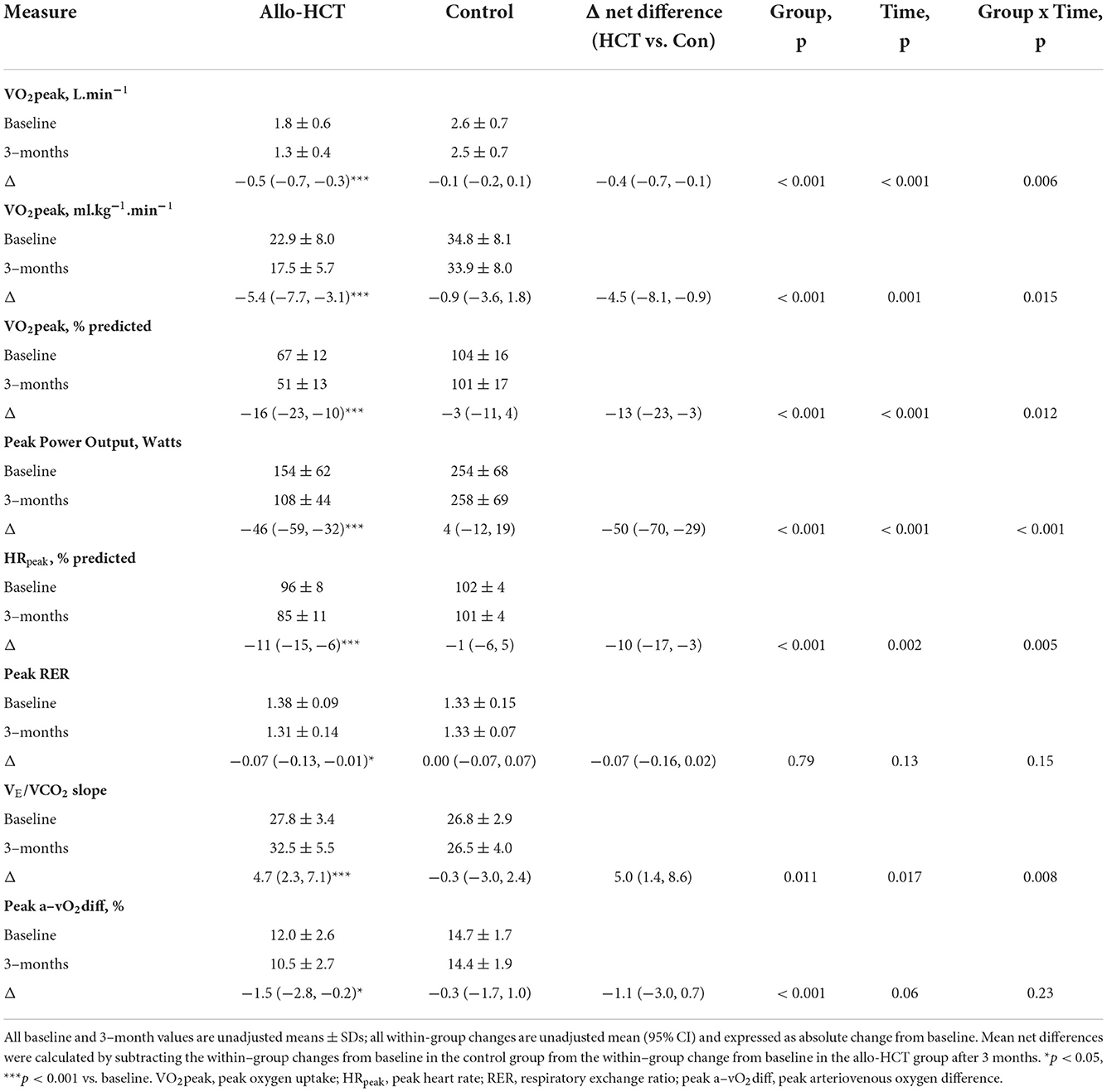
Table 3. Mean baseline values, within-group changes after 3–months and the net between-group differences for the change for peak CPET parameters in Allo-HCT and Control groups.
Resting cardiac function and cardiac biomarkers
Echocardiographic measures of resting cardiac function and cardiac biomarkers were unchanged in both groups at 3-months (Table 4). No participants developed overt CVD or met LVEF cardiotoxicity criteria, but one allo-HCT recipient commenced treatment for arrhythmia, and three allo-HCT recipients and two controls had clinically significant GLS declines. One allo-HCT recipient had a post-treatment troponin >15 ng.L−1 and two had a post-treatment BNP >100 ng.L−1, totalling a 35% incidence of subclinical cardiac pathology in allo-HCT and 17% in controls (between-group difference, p = 0.41).
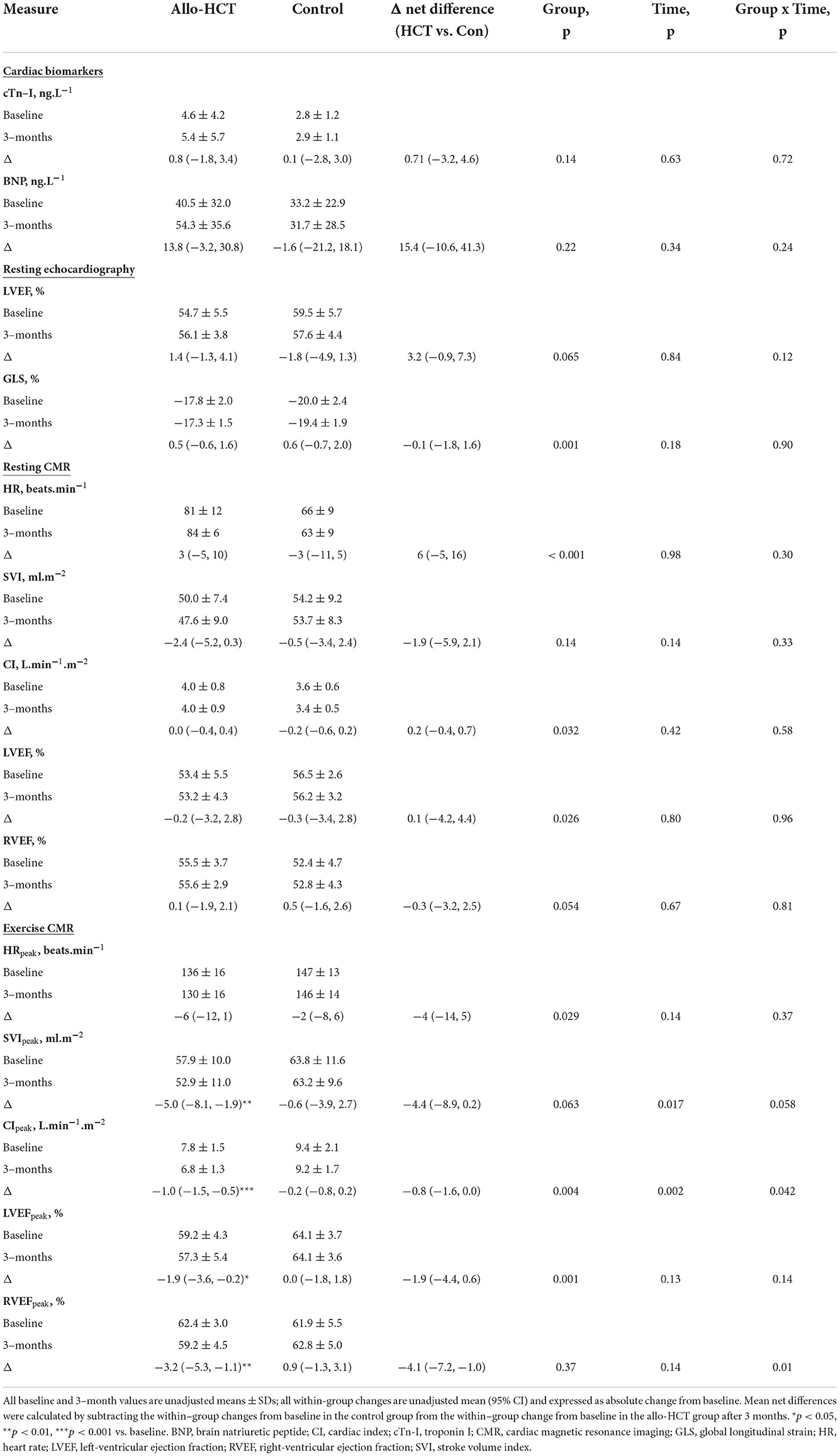
Table 4. Mean baseline values, within-group changes after 3–months and the net between-group differences for the change for cardiac parameters in Allo-HCT and Control groups.
Peak cardiac function and cardiac reserve
As shown in Table 4, allo-HCT was associated with a 13% reduction in CIpeak (p < 0.001) and a 9% reduction in SVIpeak (p = 0.003), but no change in HRpeak. CIpeak, SVIpeak and HRpeak remained unchanged in controls, resulting in a net group difference for the change at 3-months for CIpeak (interaction, p = 0.042), a trend toward a significant net group difference for SVIpeak (interaction, p = 0.058), but not HRpeak (interaction, p = 0.37). With respect to peak biventricular contractility, LVEFpeak and RVEFpeak remained unchanged in controls but decreased (absolute) 1.9% (p = 0.033) and 3.2% (p = 0.004), respectively, following allo-HCT, leading to a significant group-by-time interaction for RVEFpeak (p = 0.010), but not LVEFpeak (p = 0.14).
Results pertaining to changes in cardiac and contractile reserve (ability to augment function above resting) are shown in Figures 2A–E. CI, SVI and HR reserve were unchanged in controls at 3-months, but were further blunted in allo-HCT recipients (p = 0.001, p = 0.010, p = 0.020, respectively), resulting in a net group difference for the change at 3-months for CI reserve (interaction, p = 0.019) and trends toward significant net group differences for SVI reserve and HR reserve (interaction, p = 0.081 and p = 0.056, respectively). A significant interaction was observed for RVEF reserve (p = 0.010), due to a blunted augmentation from rest to peak exercise in allo-HCT (p = 0.001) and no change in controls. There were no within- or between-group differences for LVEF reserve.
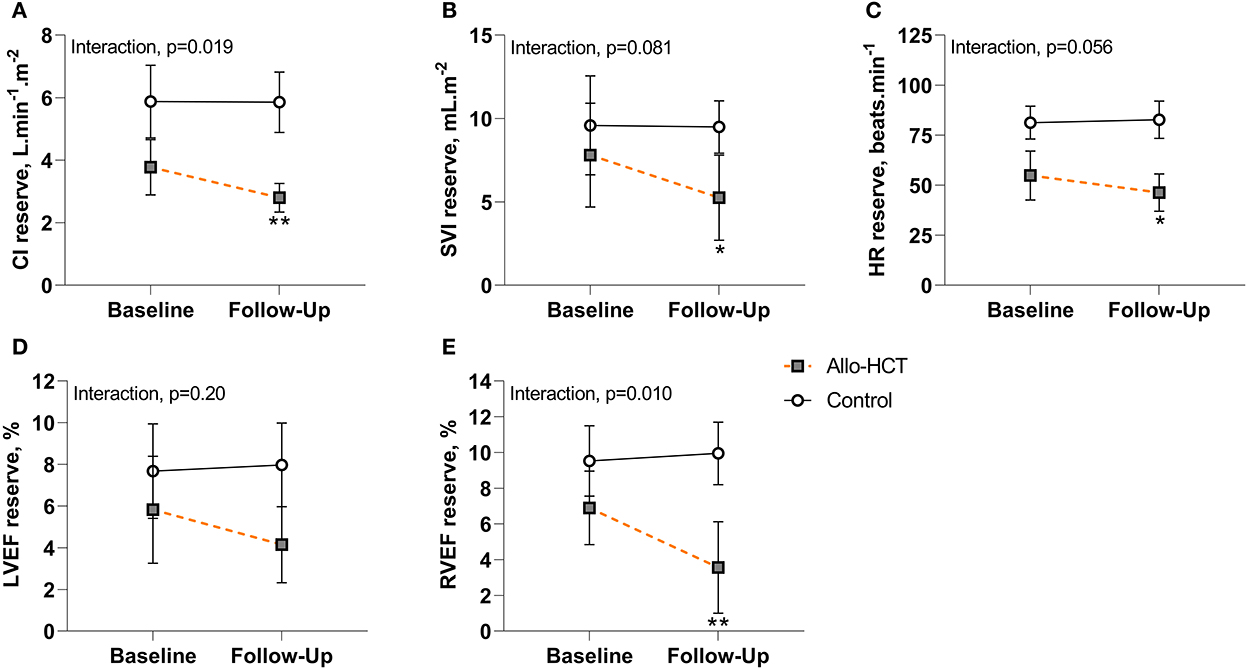
Figure 2. (A–E) Cardiac and contractile reserve at baseline and 3-month follow-up for Allo-HCT (n = 12) and Control (n = 11). After 3-months, cardiac and contractile reserve were maintained in controls, but allo-HCT experienced a blunted CI, SVI, HR, and RVEF reserve, resulting in a significant between-group difference for the net change from baseline for CI reserve and RVEF reserve and a trend toward a significant between-group difference for the net change from baseline for SVI reserve and HR reserve. *p < 0.05 and **p < 0.01 for within-group change in reserve. Data are unadjusted mean (95% CI). CI, cardiac index; HR, heart rate; LVEF, left-ventricular ejection fraction; RVEF, right-ventricular ejection fraction; SVI, stroke volume index.
Body composition and indices of vascular and hematological function
Weight declined 3.8 kg in allo-HCT recipients (p = 0.002), but this was not significantly different from the change in controls (−0.6 kg, p = 0.65; interaction, p = 0.082) (Table 5). Conversely, allo-HCT recipients experienced a significant net loss of 3.2 kg in LM relative to controls after 3-months (interaction, p = 0.001). No significant changes existed in either group for FM, %BF, hemoglobin, or BP. Three allo-HCT recipients and one control developed new-onset hypertension at 3-months, totaling a 35 and 8% prevalence, respectively (between-group difference, p = 0.19).
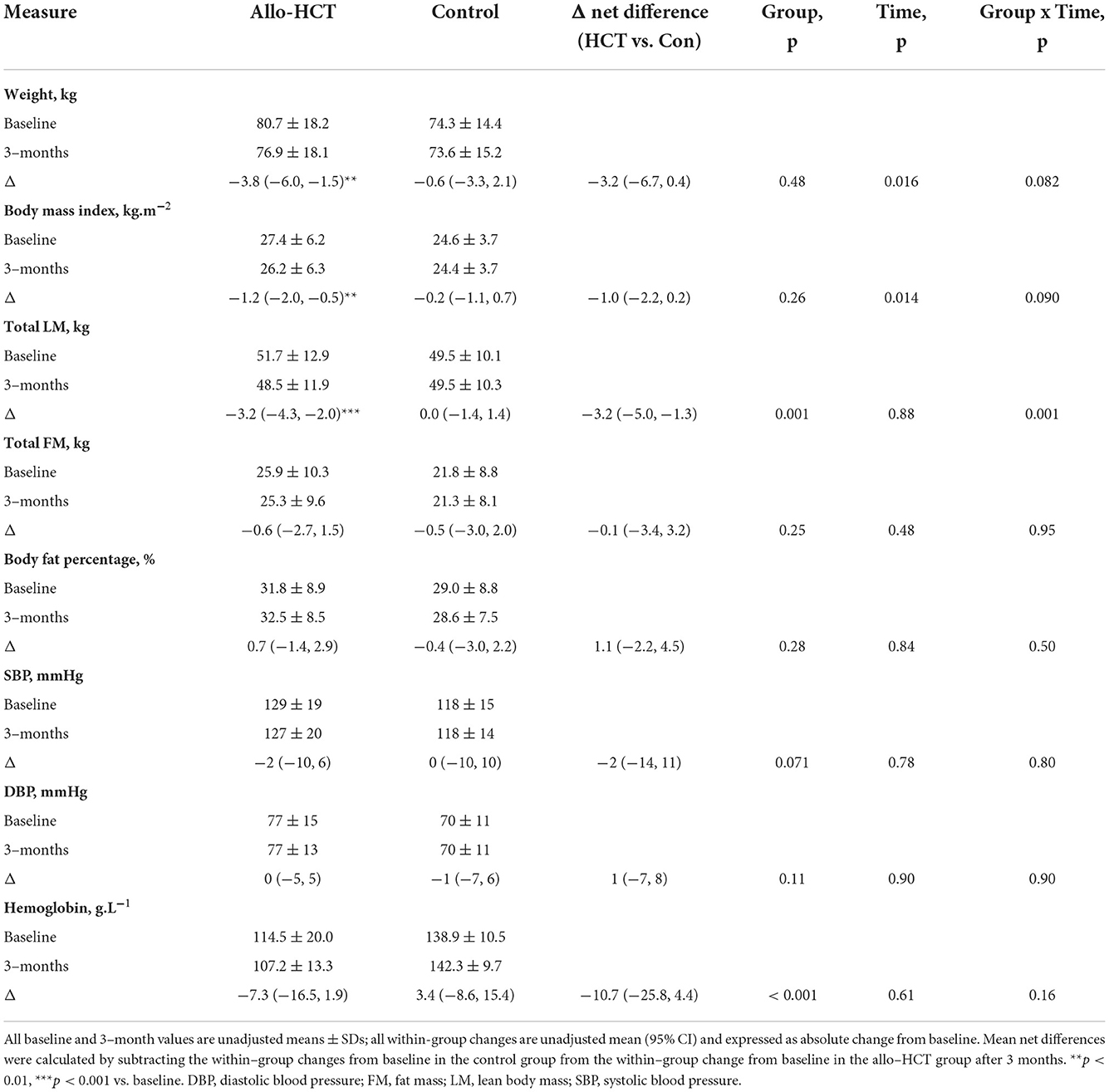
Table 5. Mean baseline values, within-group changes after 3–months and the net between-group differences for the change for body composition and indices of vascular and hematological function in Allo-HCT and Control groups.
Discussion
To our knowledge, this is the first study to prospectively evaluate the short-term cardiovascular impact of allo-HCT among early transplant survivors, with comparison to an age-matched control group. Utilizing novel, state-of-the-art, non-invasive measures of cardiac function, this study demonstrated that, relative to matched controls, patients scheduled for allo-HCT presented with marked impairment in VO2peak and cardiac reserve. Importantly, we extend these findings and demonstrate that VO2peak and cardiac reserve deteriorate further in the 3-months following allo-HCT. Moreover, such impairments coincided with a reduction in a-vO2diff which makes an important contribution to the symptomatology of cardiovascular disorders such as heart failure (30). Collectively, these findings provide evidence of an accelerated cardiovascular aging phenotype that is present prior to transplant but is further exacerbated by the transplant and hospitalization process.
The inverse relationship between VO2peak and risk of cardiovascular morbidity, cardiovascular mortality, all-cause mortality, and cancer-specific mortality has been well established (25–27). In the present study, we observed a 26 and 24% reduction in absolute and bodyweight-indexed VO2peak in allo-HCT recipients over 3-months, which was ~9-fold greater than that observed in age-matched controls and approximates the degree of cardiovascular aging expected over 24 years of normal aging (38). While the 5.4 ml.kg−1.min−1 decline in VO2peak in allo-HCT recipients is profound, the true vulnerability of this population becomes especially evident when viewed in the context of the already diminished cardiovascular function prior to undergoing allo-HCT. Indeed, evidence from large prospective studies in ostensibly healthy non-cancer populations demonstrates that for each 1 MET (3.5 ml.kg−1.min−1) decrement in VO2peak, the risk of incident heart failure and all-cause mortality increases 16–21 and 25%, respectively (25, 39). In the present study, allo-HCT recipients achieved a VO2peak at follow-up that was 50% (16.4 ml.kg−1.min−1 or 4.7 METs) lower than controls, but of clinical relevance is that 53% of allo-HCT recipients were classified as being functionally disabled (VO2peak <18 ml.kg−1.min−1) at 3-months. This threshold has been associated with a reduced capacity to independently perform activities of daily living (40), and serves as a strong prognostic threshold, below which, the risk of incident heart failure and all-cause mortality are heightened 7-to-9-fold (26, 36). Notably, cross-sectional evaluation of VO2peak among long-term allo-HCT survivors (median time since allo-HCT, 9.8 years [range, 3−20]) indicates that these deleterious impairments in VO2peak do not fully recover over time, remaining substantially lower than predicted (22% below predicted) (7). Taken together, and consistent with the increased cardiovascular burden reported among long-term survivors (5–7, 9–12), these findings infer that allo-HCT recipients face a substantially greater risk of developing CVD relative to controls and provide novel insight into the potential trajectory of cardiovascular dysfunction in this cohort.
Dissecting the cardiac contribution to these reductions in VO2peak is critical given the potential role for pharmacological and non-pharmacological (i.e., lifestyle) interventions to attenuate cardiotoxicity. Using state-of-the-art exercise CMR, we provide the first evidence that treatment with allo-HCT significantly blunts cardiac reserve. Indeed, compared to pre-transplant, allo-HCT recipients experienced a blunted increase in SVI and HR from rest to peak exercise, resulting in a reduced augmentation in CI during exercise. These changes are indicative of myocardial injury/maladaptation—likely ascribed to both direct (i.e., cardiotoxic conditioning regimens) and indirect (i.e., physical inactivity, sedentary behavior) pathological perturbations (15, 16). The exact pathological mechanisms of allo-HCT induced cardiotoxicity are incompletely understood, but growing evidence suggests chemotherapy, radiotherapy, physical inactivity, and the allograft itself (by way of alloreactive donor T cell mediated immune and pro-inflammatory cytokine activation) can perturb the redox and inflammatory balance, which would theoretically lead to DNA damage, mitochondrial dysfunction, impaired sarcoplasmic reticulum calcium uptake activity, and extracellular matrix remodeling (i.e., fibrosis), and ultimately contractile dysfunction and cardiomyocyte apoptosis (41–44). The “mechanical unloading” associated with physical inactivity and bedrest may further compromise cardiac output via deconditioning of the cardiac muscle or via reductions in venous return and therefore stroke volume (45, 46). Moreover, it is important to highlight these declines in cardiac reserve induced by allo-HCT occurred on top of a reserve that was already diminished at baseline, such that following allo-HCT, CIpeak, LVEFpeak and RVEFpeak were 26%, 7% (absolute), and 3% (absolute) lower than age-matched controls. Given that an inability to generate sufficient cardiac output during periods of high metabolic demand is an early hallmark of heart failure (24, 47), extrapolating these results over the years following allo-HCT—wherein normal age-related decline in cardiac function continues—may offer a possible explanation for the heightened prevalence of premature CVD and associated cardiovascular events and mortality in long-term survivors. Another intriguing finding from our study was that these allo-HCT induced reductions in cardiac reserve ensued whereas standard resting measures of cardiac function (LVEF, GLS, cardiac biomarkers) were unchanged. Indeed, echocardiographic parameters remained, on average, within normal ranges, and interpreted as an isolated assessment, would not flag an increased risk of CVD. These results are consistent with that observed among long-term allo-HCT survivors (normal LVEF despite impaired VO2peak at a median of 9.8 years after allo-HCT) (7) but are in contrast to Moriyama et al. (48) whom detected significant left-ventricular systolic dysfunction (characterized as a decrease in LVEF of ≥10% or LVEF ≤ 53%) in 17% of patients within 100 days after allo-HCT. These discrepant echocardiographic observations may be explained by differences in study design and potential selection bias. Indeed, we conducted a prospective echocardiographic assessment of all allo-SCT recipients whereas Moriyama et al. (48) conducted a retrospective review of allo-SCT recipients who underwent echocardiographic assessment at physician discretion (136/416 patients), biasing the likelihood of a cardiac finding. Nonetheless, the results of our study are consistent with the pattern of cardiac impairment seen among heart failure and anthracycline-treated cancer patients, wherein reductions in cardiac reserve often precede impairment in resting function (22–24, 47). Therefore, whilst not the primary aim of this study, our results also highlight the added utility of exercise cardiac reserve assessment in unmasking early treatment induced cardiac dysfunction in vulnerable populations.
Importantly, given non-cardiac factors also make important contributions to VO2peak (28) and the CVD phenotype (29–31), we explored whether the reduction in VO2peak observed among allo-HCT recipients could also reflect impairment in non-cardiac factors that determine peripheral muscle O2 delivery and utilization. In the present study, deficits in O2 carrying capacity as a result of anemia due to disease and prior therapies likely contributed to the baseline deficit in VO2peak among allo-HCT recipients (relative to controls) (49), but any further reductions in hemoglobin induced by allo-HCT had recovered at 3-months, and is therefore unlikely to explain the allo-HCT induced decline in VO2peak. We did, however, observe a significant reduction in peak a-vO2diff among allo-HCT recipients at 3-months. This is an important and novel finding as the impact of allo-HCT on skeletal muscle oxygenation has not been fully appreciated but may also contribute to the premature development of CVD and functional impairment in this population. Delineating the contribution of vascular and skeletal muscle factors to this decline in a-vO2diff will provide important insight into its clinical significance.
Premature vascular aging in allo-HCT patients may have been expected based on evidence from small cross-sectional and prospective studies which have evaluated vascular structure and function in the allo-HCT setting. Indeed, allo-HCT recipients have been shown to exhibit increased endothelial damage and dysfunction (evidenced by elevated circulating endothelial cells and soluble markers of endothelial damage, and lower endothelial dependent flow-mediated dilation) (50–53), central arterial stiffening (evidenced by increased aortic pulse wave velocity and reduced carotid distensibility, compliance and incremental elastic modulus) (54–56), and carotid intima-media thickening (54) compared to age-matched healthy controls or pre-transplant values. Consequently, hypertension is a common early (1-month incidence: 38–61%) (57, 58) and persistent complication of allo-HCT (odds ratio: 3.65 [95% CI, 1.82–7.32] at 8.6 years after allo-SCT) (59). It was therefore somewhat unexpected that the baseline prevalence and 3-month incidence of hypertension was similar between allo-HCT recipients and controls. This discrepancy could reflect the comparatively lower occurrence of grade II-IV GvHD and subsequent immunosuppressant exposure in our study (17, 18). Beyond this, it is important to note that subclinical vascular damage (i.e., endothelial dysfunction, arterial stiffness, intimal thickening) often precedes the development of hypertension and can remain “silent” for years before manifesting clinically, and therefore cannot be excluded as a possible mediator of allo-HCT-induced impairments in a-vO2diff and subsequently VO2peak. Moreover, there is emerging evidence that impairments in a-vO2diff, and subsequent exercise capacity following allo-HCT are explicable by concomitant skeletal muscle atrophy and mitochondrial dysfunction (60, 61). Indeed, as per-stated, generation of reactive oxygen species is a common effect of allo-HCT conditioning (41), allografting (43), and associated physical inactivity which can perturb homeostatic control of energy balance, upregulate muscle proteolytic and apoptotic signaling pathways, downregulate mitochondrial biogenesis and quality control pathways, and induce mitochondrial dysfunction (62). These deleterious processes may be further exacerbated by indirect treatment effects such as reductions in physical activity and dietary intake (63). Mitochondrial function was not directly assessed in the present study, but we did observe a significant reduction in LM which is a key determinant of VO2peak and risk factor for CVD (29). Taken together, with the cardiac insults, our results draw attention to the global nature of allo-HCT induced cardiovascular toxicity and highlights the need for cardiovascular preventive therapies capable of preserving and/or augmenting both central and peripheral determinants of VO2peak.
The strengths of this study include the prospective design, inclusion of a control group and the comprehensive cardiovascular evaluations employed which facilitated a more detailed characterization of the global cardiovascular consequences of allo-HCT than previously documented. A key limitation of the present study is the small cohort size which increases the possibility of type II error and precluded analyses of treatment-related and demographic modifiers of VO2peak and organ-specific function in our allo-SCT group. Such factors, particularly the impact of conditioning intensity (myeloablative vs. reduced intensity) which presumably impact the degree of cardiovascular damage incurred, warrant investigation in larger studies. Additionally, whilst we may speculate on the evolution of these changes in the years following allo-HCT, the short-term nature of this study limits our ability to explicitly discern the degree to which the observed changes depict persistent cardiovascular dysfunction that may culminate in overt CVD. Longitudinal assessment of these effects over subsequent years will be integral to understand their clinical trajectory and potential clinical significance. The selection of a cancer-free control group may be considered a limitation, however, the challenges associated with recruiting a suitable comparator should be acknowledged. Indeed, whilst ideal, it is implausible to compare to patients with similar hematological malignancies without allo-SCT due to the severity of the underlying illness and need for active treatment. From an alternate perspective, the inclusion of a cancer-free control group effectively highlights the pathological nature of the observed changes seen among allo-SCT recipients and provides important context of the true vulnerability of this high-risk patient group. Finally, we cannot exclude the possibility of subclinical allo-SCT induced vascular toxicity. A more detailed characterisation of effects of allo-HCT on subclinical vascular damage (e.g., endothelial dysfunction, arterial compliance) is required to provide a more complete understanding of the mechanisms underscoring the reduced VO2peak.
In summary, treatment with allo-HCT was associated with a marked reduction in VO2peak, reflecting a deterioration in both exercise cardiac reserve and a-vO2diff. Considering the inverse association between VO2peak and CVD risk, our results suggest that allo-HCT is a potent accelerator of cardiovascular aging, and provides valuable insight into the potential trajectory and pathogenesis of CVD in allo-HCT survivors. Combining these results with the existing cardiovascular dysfunction identified pre-allo-HCT, our study highlights the urgent need for preventive interventions—initiated early in, or even prior to, the allo-HCT process and capable of targeting the heart and periphery—to mitigate cardiovascular dysfunction in this high-risk patient group.
Data availability statement
The reported data will be shared upon reasonable request to the corresponding author.
Ethics statement
This study was reviewed and approved by the Alfred Hospital Ethics Committee. All experimental procedures conformed to the ethical standards set by the Helsinki Declaration. The patients/participants provided their written informed consent to participate in this study.
Author contributions
EH, BK, DD, ALG, and SA contributed to the conceptualization and design of the study. HD, SF, YH-O, EH, and DK were responsible for participant recruitment. HD, SF, YH-O, and EH conducted data collection. HD, ALG, SF, and EH were responsible for image analyses (DXA, Echo, and CMR). HD and RD conducted the statistical analysis. HD drafted the original manuscript. All authors critically revised the manuscript and approved the final version.
Funding
SF was supported by the Australian Government Research Training Program Scholarship (4635089552). EH and ALG are supported by the Australian National Heart Foundation Future Leader Fellowships (102536 and 102021, respectively). DD was supported by an NHMRC Senior Research Fellowship (GNT1078360).
Acknowledgments
The authors acknowledge the Alfred Hospital Malignant Hematology & Stem Cell Transplantation staff who was supported and referred patients to our study. The results of the study are presented clearly, honestly, and without fabrication, falsification, or inappropriate data manipulation.
Conflict of interest
BK was employed by CSL Ltd.
The remaining authors declare that the research was conducted in the absence of any commercial or financial relationships that could be construed as a potential conflict of interest.
Publisher's note
All claims expressed in this article are solely those of the authors and do not necessarily represent those of their affiliated organizations, or those of the publisher, the editors and the reviewers. Any product that may be evaluated in this article, or claim that may be made by its manufacturer, is not guaranteed or endorsed by the publisher.
Supplementary material
The Supplementary Material for this article can be found online at: https://www.frontiersin.org/articles/10.3389/fcvm.2022.926064/full#supplementary-material
References
1. Sung H, Ferlay J, Siegel RL, Laversanne M, Soerjomataram I, Jemal A et al. Global Cancer Statistics 2020: GLOBOCAN estimates of incidence and mortality worldwide for 36 cancers in 185 countries. CA Cancer J Clin. (2021) 71:209–49. doi: 10.3322/caac.21660
2. Niederwieser D, Baldomero H, Atsuta Y, Aljurf M, Seber A, Greinix HT, et al. One and half million Hematopoietic Stem Cell Transplants (HSCT). dissemination, trends and potential to improve activity by telemedicine from the Worldwide Network for Blood and Marrow Transplantation (WBMT). Blood. (2019) 134(Suppl. 1):2035. doi: 10.1182/blood-2019-125232
3. Penack O, Peczynski C, Mohty M, Yakoub-Agha I, Styczynski J, Montoto S et al. How much has allogeneic stem cell transplant–related mortality improved since the 1980s? A retrospective analysis from the EBMT. Blood Advan. (2020) 4:6283–90. doi: 10.1182/bloodadvances.2020003418
4. Copelan EA, Chojecki A, Lazarus HM, Avalos BR. Allogeneic hematopoietic cell transplantation the current renaissance. Blood Rev. (2019) 34:34–44. doi: 10.1016/j.blre.2018.11.001
5. Tichelli A, Bucher C, Rovó A, Stussi G, Stern M, Paulussen M, et al. Premature cardiovascular disease after allogeneic hematopoietic stem-cell transplantation. Blood. (2007) 110:3463–71. doi: 10.1182/blood-2006-10-054080
6. Massey RJ, Diep PP, Ruud E, Burman MM, Kvaslerud AB, Brinch L et al. Left ventricular systolic function in long-term survivors of allogeneic hematopoietic stem cell transplantation. JACC: CardioOncol. (2020) 2:460–71. doi: 10.1016/j.jaccao.2020.06.011
7. Armenian SH, Horak D, Scott JM, Mills G, Siyahian A, Teh JB et al. Cardiovascular function in long-term hematopoietic cell transplantation survivors. Biol Blood Marrow Transplant. (2017) 23:700–5. doi: 10.1016/j.bbmt.2017.01.006
8. Paiman EH, Louwerens M, Bresters D, Westenberg JJ, Tao Q, van der Geest RJ, et al. Late effects of pediatric hematopoietic stem cell transplantation on left ventricular function, aortic stiffness and myocardial tissue characteristics. J Cardiovasc Magn Reson. (2019) 21:6. doi: 10.1186/s12968-018-0513-4
9. Chow EJ, Baker KS, Lee SJ, Flowers ME, Cushing-Haugen KL, Inamoto Y, et al. Influence of conventional cardiovascular risk factors and lifestyle characteristics on cardiovascular disease after hematopoietic cell transplantation. J Clin Oncol. (2014) 32:191–8. doi: 10.1200/JCO.2013.52.6582
10. Tichelli A, Passweg J, Wójcik D, Rovó A, Harousseau JL, Masszi T, et al. Late cardiovascular events after allogeneic hematopoietic stem cell transplantation: a retrospective multicenter study of the late effects working party of the European Group for blood and marrow transplantation. Haematologica. (2008) 93:1203–10. doi: 10.3324/haematol.12949
11. Chow EJ, Mueller BA, Baker KS, Cushing-Haugen KL, Flowers ME, Martin PJ et al. Cardiovascular hospitalizations and mortality among recipients of hematopoietic stem cell transplantation. Ann Intern Med. (2011) 155:21–32. doi: 10.7326/0003-4819-155-1-201107050-00004
12. Bhatia S, Francisco L, Carter A, Sun CL, Baker KS, Gurney JG et al. Late mortality after allogeneic hematopoietic cell transplantation and functional status of long-term survivors: report from the bone marrow transplant survivor study. Blood. (2007) 110:3784–92. doi: 10.1182/blood-2007-03-082933
13. Armenian SH, Chemaitilly W, Chen M, Chow EJ, Duncan CN, Jones LW et al. National institutes of health hematopoietic cell transplantation late effects initiative: the cardiovascular disease and associated risk factors working group report. Biol Blood Marrow Transplant. (2017) 23:201–10. doi: 10.1016/j.bbmt.2016.08.019
14. Hamanaka RB, Chandel NS. Mitochondrial reactive oxygen species regulate cellular signaling and dictate biological outcomes. Trends Biochem Sci. (2010) 35:505–13. doi: 10.1016/j.tibs.2010.04.002
15. Zamorano JL, Lancellotti P, Rodriguez Munoz D, Aboyans V, Asteggiano R, Galderisi Met al. 2016 ESC position paper on cancer treatments and cardiovascular toxicity developed under the auspices of the ESC committee for practice guidelines: the task force for cancer treatments and cardiovascular toxicity of the European Society of Cardiology (ESC). Eur Heart J. (2016) 37:2768–801. doi: 10.1093/eurheartj/ehw211
16. McGuire DK, Levine BD, Williamson JW, Snell PG, Blomqvist CG, Saltin B, et al. A 30-year follow-up of the dallas bedrest and training study: I. Effect of age on the cardiovascular response to exercise. Circulation. (2001) 104:1350–7. doi: 10.1161/circ.104.12.1350
17. Chow EJ, Wong K, Lee SJ, Cushing-Haugen KL, Flowers ME, Friedman DL„, et al. Late cardiovascular complications after hematopoietic cell transplantation. Biol Blood Marrow Transplant. (2014) 20:794–800. doi: 10.1016/j.bbmt.2014.02.012
18. Armenian SH, Sun CL, Vase T, Ness KK, Blum E, Francisco L, et al. Cardiovascular risk factors in hematopoietic cell transplantation survivors: role in development of subsequent cardiovascular disease. Blood. (2012) 120:4505–12. doi: 10.1182/blood-2012-06-437178
19. Fearon KC, Glass DJ, Guttridge DC. Cancer cachexia: mediators, signaling, and metabolic pathways. Cell Metab. (2012) 16:153–66. doi: 10.1016/j.cmet.2012.06.011
20. Campelj DG, Goodman CA, Rybalka E. Chemotherapy-induced myopathy: the dark side of the cachexia sphere. Cancers. (2021) 13:3615. doi: 10.3390/cancers13143615
21. Grotto HZW. Anaemia of cancer: an overview of mechanisms involved in its pathogenesis. Medical Oncol. (2008) 25:12–21. doi: 10.1007/s12032-007-9000-8
22. Foulkes S, Costello BT, Howden EJ, Janssens K, Dillon H, Toro C et al. Exercise cardiovascular magnetic resonance reveals reduced cardiac reserve in pediatric cancer survivors with impaired cardiopulmonary fitness. J Cardiovasc Magn Reson. (2020) 22:64. doi: 10.1186/s12968-020-00658-4
23. Howden EJ, Bigaran A, Beaudry R, Fraser S, Selig S, Foulkes S et al. Exercise as a diagnostic and therapeutic tool for the prevention of cardiovascular dysfunction in breast cancer patients. Eur J Prev Cardiol. (2019) 26:305–15. doi: 10.1177/2047487318811181
24. Weber KT, Janicki JS. Cardiopulmonary exercise testing for evaluation of chronic cardiac failure. Am J Cardiol. (1985) 55:A22–31. doi: 10.1016/0002-9149(85)90792-1
25. Kupsky DF, Ahmed AM, Sakr S, Qureshi WT, Brawner CA, Blaha MJ, et al. Cardiorespiratory fitness and incident heart failure: the Henry Ford ExercIse Testing (FIT) Project. Am Heart J. (2017) 185:35–42. doi: 10.1016/j.ahj.2016.12.006
26. Wood WA, Deal AM, Reeve BB, Abernethy AP, Basch E, Mitchell SA, et al. Cardiopulmonary fitness in patients undergoing hematopoietic SCT: a pilot study. Bone Marrow Transplant. (2013) 48:1342–9. doi: 10.1038/bmt.2013.58
27. Kelsey CR, Scott JM, Lane A, Schwitzer E, West MJ, Thomas S et al. Cardiopulmonary exercise testing prior to myeloablative allo-SCT: a feasibility study. Bone Marrow Transplant. (2014) 49:1330–6. doi: 10.1038/bmt.2014.159
28. Balady GJ, Arena R, Sietsema K, Myers J, Coke L, Fletcher GF et al. Clinician's guide to cardiopulmonary exercise testing in adults: a scientific statement from the American Heart Association. Circulation. (2010) 122:191–225. doi: 10.1161/CIR.0b013e3181e52e69
29. Tyrovolas S, Panagiotakos D, Georgousopoulou E, Chrysohoou C, Tousoulis D, Haro JM et al. Skeletal muscle mass in relation to 10 year cardiovascular disease incidence among middle aged and older adults: the ATTICA study. J Epidemiol Community Health. (2020) 74:26. doi: 10.1136/jech-2019-212268
30. Houstis NE, Eisman AS, Pappagianopoulos PP, Wooster L, Bailey CS, Wagner PD et al. Exercise intolerance in heart failure with preserved ejection fraction: diagnosing and ranking its causes using personalized O(2) pathway analysis. Circulation. (2018) 137:148–61. doi: 10.1161/CIRCULATIONAHA.117.029058
31. Molina AJ, Bharadwaj MS, Van Horn C, Nicklas BJ, Lyles MF, Eggebeen J, et al. Skeletal muscle mitochondrial content, oxidative capacity, and Mfn2 expression are reduced in older patients with heart failure and preserved ejection fraction and are related to exercise intolerance. JACC: Heart Failure. (2016) 4:636–45. doi: 10.1016/j.jchf.2016.03.011
32. Silva CG, Kaminsky LA, Arena R, Christle JW, Araújo CG, Lima RM, et al. A reference equation for maximal aerobic power for treadmill and cycle ergometer exercise testing: Analysis from the FRIEND registry. Eur J Prevent Cardiol. (2018) 25:204748731876395. doi: 10.1177/2047487318763958
33. Keteyian SJ, Patel M, Kraus WE, Brawner CA, McConnell TR, Piña IL et al. Variables measured during cardiopulmonary exercise testing as predictors of mortality in chronic systolic heart failure. J Am Coll Cardiol. (2016) 67:780–9. doi: 10.1016/j.jacc.2015.11.050
34. Ross RM. ATS/ACCP statement on cardiopulmonary exercise testing. Am J Respir Crit Care Med. (2003) 167:1451. doi: 10.1164/ajrccm.167.10.950
35. La Gerche A, Claessen G, Van de Bruaene A, Pattyn N, Van Cleemput J, Gewillig M et al. Cardiac MRI: a new gold standard for ventricular volume quantification during high-intensity exercise. Circ Cardiovasc Imaging. (2012) 6:329–38. doi: 10.1161/CIRCIMAGING.112.980037
36. Forman DE, Arena R, Boxer R, Dolansky MA, Eng JJ, Fleg JL et al. Prioritizing functional capacity as a principal end point for therapies oriented to older adults with cardiovascular disease: a scientific statement for healthcare professionals from the American Heart Association. Circulation. (2017) 135:e894–918. doi: 10.1161/CIR.0000000000000483
37. Convertino VA. Cardiovascular consequences of bed rest: effect on maximal oxygen uptake. Med Sci Sports Exerc. (1997) 29:191–6. doi: 10.1097/00005768-199702000-00005
38. Hollenberg M, Yang J, Haight TJ, Tager IB. Longitudinal changes in aerobic capacity: implications for concepts of aging. J Gerontol A Biol Sci Med Sci. (2006) 61:851–8. doi: 10.1093/gerona/61.8.851
39. Imboden MT, Harber MP, Whaley MH, Finch WH, Bishop DA, Fleenor BS et al., editors. The influence of change in cardiorespiratory fitness with short-term exercise training on mortality risk from the Ball State Adult Fitness Longitudinal Lifestyle Study. In: Mayo Clinic Proceedings. Rochester, MN: Elsevier (2019). doi: 10.1016/j.mayocp.2019.01.049
40. Morey MC, Pieper CF, Cornoni-Huntley J. Is there a threshold between peak oxygen uptake and self-reported physical functioning in older adults? Med Sci Sports Exerc. (1998) 30:1223–9. doi: 10.1097/00005768-199808000-00007
41. Howden EJ, La Gerche A, Arthur JF, McMullen JR, Jennings GL, Dunstan DW et al. Standing up to the cardiometabolic consequences of hematological cancers. Blood Rev. (2018) 32:349–60. doi: 10.1016/j.blre.2018.02.005
42. Debevec T, Pialoux V, Ehrström S, Ribon A, Eiken O, Mekjavic IB„, et al. FemHab: The effects of bed rest and hypoxia on oxidative stress in healthy women. J Appl Physiol. (2016) 120:930–8. doi: 10.1152/japplphysiol.00919.2015
43. Sari I, Cetin A, Kaynar L, Saraymen R, Hacioglu SK, Ozturk A et al. Disturbance of pro-oxidative/antioxidative balance in allogeneic peripheral blood stem cell transplantation. Ann Clin Lab Sci. (2008) 38:120–5.
44. Reddy P, Ferrara JL. Immunobiology of acute graft-versus-host disease. Blood Rev. (2003) 17:187–94. doi: 10.1016/S0268-960X(03)00009-2
45. Knight J, Nigam Y, Jones A. Effects of bedrest 1: introduction and the cardiovascular system. Nurs Times. (2018) 114:54–7. doi: 10.2307/j.ctt1pwt9nj.4
46. Dorfman TA, Levine BD, Tillery T, Peshock RM, Hastings JL, Schneider SM, et al. Cardiac atrophy in women following bed rest. J Appl Physiol. (1985). (2007) 103:8–16. doi: 10.1152/japplphysiol.01162.2006
47. Borlaug BA, Olson TP, Lam CS, Flood KS, Lerman A, Johnson BD et al. Global cardiovascular reserve dysfunction in heart failure with preserved ejection fraction. J Am Coll Cardiol. (2010) 56:845–54. doi: 10.1016/j.jacc.2010.03.077
48. Moriyama S, Fukata M, Hieda M, Yokoyama T, Yoshimoto G, Kusaba H, et al. Early-onset cardiac dysfunction following allogeneic haematopoietic stem cell transplantation. Open Heart. (2022) 9:2007. doi: 10.1136/openhrt-2022-002007
49. Ekblom B, Goldbarg AN, Gullbring B. Response to exercise after blood loss and reinfusion. J Appl Physiol. (1972) 33:175–80. doi: 10.1152/jappl.1972.33.2.175
50. Woywodt A, Scheer J, Hambach L, Buchholz S, Ganser A, Haller H et al. Circulating endothelial cells as a marker of endothelial damage in allogeneic hematopoietic stem cell transplantation. Blood. (2004) 103:3603–5. doi: 10.1182/blood-2003-10-3479
51. Palomo M, Diaz-Ricart M, Carbo C, Rovira M, Fernandez-Aviles F, Martine C, et al. Endothelial dysfunction after hematopoietic stem cell transplantation: role of the conditioning regimen and the type of transplantation. Biol Blood Marrow Transplan. (2010) 16:985–93. doi: 10.1016/j.bbmt.2010.02.008
52. Poreba M, Gać P, Usnarska-Zubkiewicz L, Pilecki W, Kuliczkowski K, Mazur G et al. Endothelial function in patients with hematologic malignancies undergoing high-dose chemotherapy followed by hematopoietic stem cell transplantation. Cardiovasc Toxicol. (2016) 16:156–62. doi: 10.1007/s12012-015-9324-0
53. Gavriilaki E, Sakellari I, Anyfanti P, Batsis I, Vardi A, Bousiou Z, et al. Assessment of endothelial injury and pro-coagulant activity using circulating microvesicles in survivors of allogeneic hematopoietic cell transplantation. Int J Mol Sci. (2020) 21:9768. doi: 10.3390/ijms21249768
54. Borchert-Mörlins B, Memaran N, Sauer M, Maecker-Kolhoff B, Sykora KW, Blöte R et al. Cardiovascular risk factors and subclinical organ damage after hematopoietic stem cell transplantation in pediatric age. Bone Marrow Transplan. (2018) 53:983–92. doi: 10.1038/s41409-018-0104-x
55. Turanlahti MI, Taskinen M, Saarinen-Pihkala U, Jokinen EV. Time-related arterial changes after allogeneic hematopoietic stem cell transplantation in children. Pediatr Res. (2013) 73:777–82. doi: 10.1038/pr.2013.49
56. Dengel DR, Kelly AS, Zhang L, Wang Q, Hodges JS, Steinberger J, et al. Vascular structure and function in cancer survivors after hematopoietic stem cell transplantation. Biol Blood Marrow Transplantat. (2019) 25:151–6. doi: 10.1016/j.bbmt.2018.08.005
57. Majhail NS, Challa TR, Mulrooney DA, Baker KS, Burns LJ. Hypertension and diabetes mellitus in adult and pediatric survivors of allogeneic hematopoietic cell transplantation. Biol Blood Marrow Transplan. (2009) 15:1100–7. doi: 10.1016/j.bbmt.2009.05.010
58. Kwon DH, Jung S, Lee EJ, Lee JY, Moon S, Lee JW, et al. Incidence and risk factors for early-onset hypertension after allogeneic hematopoietic stem cell transplantation in children. Korean Circ J. (2013) 43:804–10. doi: 10.4070/kcj.2013.43.12.804
59. Scott Baker K, Ness KK, Steinberger J, Carter A, Francisco L, Burns LJ, et al. Diabetes, hypertension, and cardiovascular events in survivors of hematopoietic cell transplantation: a report from the bone marrow transplantation survivor study. Blood. (2007) 109:1765–72. doi: 10.1182/blood-2006-05-022335
60. Wakasugi T, Morishita S, Kaida K, Ikegame K, Uchiyama Y, Domen K. Muscle oxygen extraction and lung function are related to exercise tolerance after allogeneic hematopoietic stem cell transplantation. Support Care Cancer. (2021) 29:6039–48. doi: 10.1007/s00520-021-06178-w
61. DeFilipp Z, Troschel FM, Qualls DA Li S, Kuklinski MW, Kempner ME et al. Evolution of body composition following autologous and allogeneic hematopoietic cell transplantation: incidence of sarcopenia and association with clinical outcomes. Biol Blood Marrow Transplant. (2018) 24:1741–7. doi: 10.1016/j.bbmt.2018.02.016
62. Ábrigo J, Elorza AA, Riedel CA, Vilos C, Simon F, Cabrera D et al. Role of oxidative stress as key regulator of muscle wasting during cachexia. Oxid Med Cell Longev. (2018) 2018:2063179. doi: 10.1155/2018/2063179
Keywords: cardiac function, cardiopulmonary fitness, hematological cancer, exercise testing, cardiotoxicity, cardiovascular disease
Citation: Dillon HT, Foulkes S, Horne-Okano YA, Kliman D, Dunstan DW, Daly RM, Fraser SF, Avery S, Kingwell BA, La Gerche A and Howden EJ (2022) Rapid cardiovascular aging following allogeneic hematopoietic cell transplantation for hematological malignancy. Front. Cardiovasc. Med. 9:926064. doi: 10.3389/fcvm.2022.926064
Received: 22 April 2022; Accepted: 18 November 2022;
Published: 15 December 2022.
Edited by:
Jun-ichi Abe, University of Texas MD Anderson Cancer Center, United StatesReviewed by:
Venkataraghavan Ramamoorthy, Baptist Health South Florida, United StatesJuan Bautista Menendez Gonzalez, Harvard University, United States
Copyright © 2022 Dillon, Foulkes, Horne-Okano, Kliman, Dunstan, Daly, Fraser, Avery, Kingwell, La Gerche and Howden. This is an open-access article distributed under the terms of the Creative Commons Attribution License (CC BY). The use, distribution or reproduction in other forums is permitted, provided the original author(s) and the copyright owner(s) are credited and that the original publication in this journal is cited, in accordance with accepted academic practice. No use, distribution or reproduction is permitted which does not comply with these terms.
*Correspondence: Erin J. Howden, ZXJpbi5ob3dkZW5AYmFrZXIuZWR1LmF1