- 1Department of Cardiology, Xuzhou Central Hospital, Xuzhou, China
- 2Department of Cardiology, The Affiliated Hospital of Xuzhou Medical University, Xuzhou, China
Background: More than half of ST-segment elevation myocardial infarction (STEMI) patients have coronary microcirculatory dysfunction (CMD) after percutaneous coronary intervention (PCI). This study aimed to explore the role of CMD in the occurrence of contrast-induced acute kidney injury (CI-AKI) in patients with STEMI.
Methods: This was a single-centre retrospective clinical observational study. Coronary angiography–derived index of microcirculatory resistance (caIMR) was measured and used to assess CMD. Regression analysis was used to identify risk factors for CI-AKI. Restricted cubic splines (RCS) was employed to examine the dose-response relationship between caIMR and CI-AKI. The predictive accuracy of the models was assessed with net reclassification index (NRI), and integrated discrimination improvement (IDI).
Results: This study included 745 patients, the incidence of CI-AKI was 10.6% (79/745). Multivariate logistic regression identified caIMR (OR = 1.072, 95% CI: 1.051–1.094) as an independent predictor of CI-AKI. RCS analysis indicated a linear dose-response relationship between caIMR and CI-AKI. Receiver operating characteristic (ROC) analysis demonstrated that the areas under the curve for caIMR was 0.725, the optimal cutoff value was 25.95 U. Integration of caIMR could significantly improve the risk model for CI-AKI in STEMI patients (NRI = 0.721, IDI = 0.102, P < 0.001).
Conclusions: Elevated caIMR is an independent risk factor for the development of CI-AKI after PCI in STEMI patients. Integrating caIMR significantly improves the risk model for CI-AKI.
Introduction
With the acceleration of population aging, the burden of cardiovascular diseases is also increasing. Among them, ST-segment elevation myocardial infarction (STEMI) poses a significant challenge to human health (1). Percutaneous coronary intervention (PCI) is currently the main treatment for STEMI patients, but 15%–35% of patients develop contrast-induced acute kidney injury (CI-AKI) after PCI (2). Evidence suggests that STEMI is one of the key factors contributing to the increased incidence of CI-AKI and the need for dialysis, which may be related to the hemodynamic instability, neuroendocrine activation, and intense inflammatory response in STEMI patients (3, 4). Although CI-AKI is associated with poor prognosis, there is currently a lack of specific clinical drugs and interventions for preventing CI-AKI (5). Therefore, early identification of high-risk populations and active prevention of CI-AKI is crucial.
Although PCI successfully restores coronary epicardial blood flow, more than half of STEMI patients still experience coronary microcirculatory dysfunction (CMD) (6, 7). The index of microcirculatory resistance (IMR) is the “gold standard” for quantitatively measuring coronary microcirculatory dysfunction (8, 9). However, the additional procedure time, increased procedural costs, and the requirement for maximal hyperemia may hinder its use in clinical practice. This is particularly true in STEMI patients undergoing primary PCI, as it further increases patient risk. In recent years, coronary angiography-derived index of microcirculatory resistance (caIMR) has been extensively validated for accuracy and is widely used to assess CMD (10–12). Compared to traditional IMR, caIMR does not require pressure wires or adenosine, making it safer and more convenient. Previous studies have shown that CMD in STEMI patients significantly impacts prognosis (7, 13, 14). Notably, in one study, Thrombolysis in Myocardial Infarction (TIMI) was identified as an independent predictor of CI-AKI after PCI in STEMI patients (15). Additionally, nicorandil has been shown to effectively prevent CI-AKI, likely through its beneficial effects on microcirculation (16–18). To date, the relationship between IMR and CI-AKI remains unclear. This study aims to explore the relationship between caIMR and CI-AKI following primary PCI in STEMI patients.
Methods
Study population
This retrospective study included patients diagnosed with STEMI (19) between January 2021 and November 2024. The inclusion criteria were: (1) successful primary PCI performed within 12 h of symptom onset (TIMI = 3); (2) availability of complete clinical data. Patients were excluded if they met any of the following criteria: (1) pre-admission dialysis or chronic renal failure (eGFR <30 ml·min−1·1.73 m−2); (2) active inflammatory conditions (such as pulmonary infection, intestinal inflammation, or autoimmune diseases); (3) history of malignancy or hematologic disorders; (4) exposure to other radiographic contrast agents or nephrotoxic medications within 48 h before or 72 h after the procedure; (5) Poor CAG images or insufficient caIMR measurements. The study was approved by the Institutional Review Board (IRB) of the Affiliated Hospital of Xuzhou Medical University (No. XYFY2023-KL203-01). As a retrospective study posing no risk to patients, the requirement for written informed consent was waived in accordance with IRB guidelines. A total of 745 patients were enrolled in the study.
Clinical data collection
Clinical data were obtained from patient records, including age, sex, body mass index (BMI), medical history, medications, left ventricular ejection fraction (LVEF), and PCI-related information. Pre-PCI serum creatinine (Scr) levels, as well as Scr measurements taken 48–72 h after contrast agent exposure, were documented. Contrast-induced acute kidney injury (CI-AKI) was defined as a Scr increase of at least 50% or 0.3 mg/dl within 48–72 h following contrast exposure (20). According to the relevant previous standards (21, 22), chronic kidney disease (CKD) and estimated glomerular filtration rate (eGFR) were calculated and defined. Additionally, peak levels of C-reactive protein (CRP), high-sensitivity troponin T (hs-TnT), and N-terminal pro B-type natriuretic peptide (NT-proBNP) during hospitalization were recorded. Medications prescribed included aspirin, P2Y12 inhibitors, β-blockers, statins, nitrates, angiotensin-converting enzyme inhibitors (ACEI) or angiotensin receptor blockers (ARB), and diuretics.
Measurement of caIMR
The caIMR was assessed using commercial software (FlashAngio, Rainmed) (10). Two angiographic images of the target vessel, separated by at least 30°, were selected to create a three-dimensional reconstruction of the coronary artery. Invasive arterial pressure data from the guide catheter were obtained and entered into the FlashAngio console. The caIMR was calculated through a specialized hemodynamic method, as described by the formula: caIMR = Pdhyp × (L/K·Vdiastole). Where Pdhyp is the mean pressure at the distal site under maximal congestion. Pdhyp was obtained based on the pressure recorded by CAG, and it is available for each patient. L is a constant representing the distance from the inlet to the distal site, Vdiastole is the mean flow rate at the distal site during diastole, and K is another constant. Additionally, Vhyp = K·V represents the mean flow rate at the distal site under maximal congestion. PCI was performed by a team unaware of the study protocol, with hydration initiated within 12 h post-angiography according to the guideline (19). All angiograms were independently analyzed by core laboratory staff, who were blinded to the study data (Figure 1).
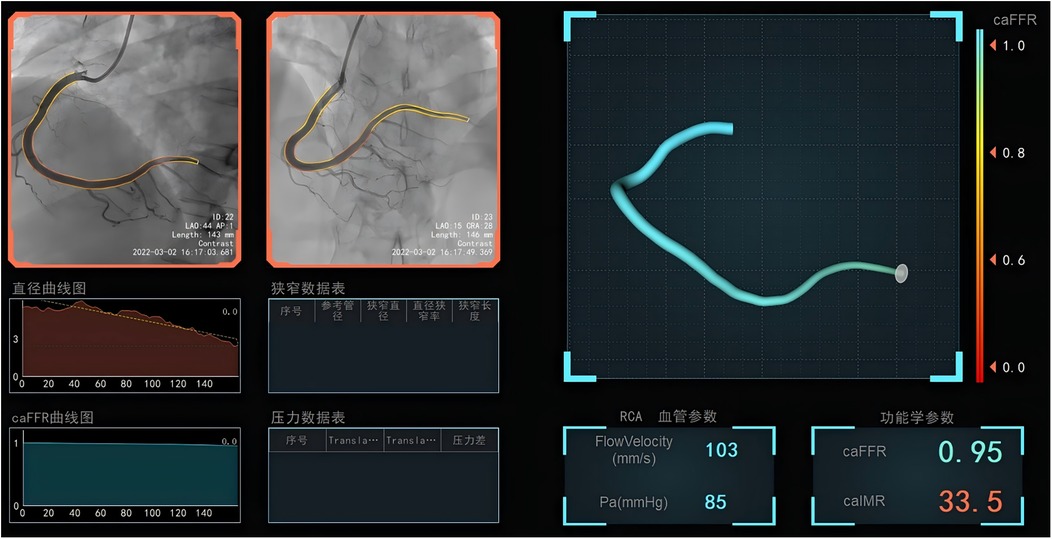
Figure 1. The measurement of coronary angiography-derived index of microcirculatory resistance in the infarct-related arteries after successful primary percutaneous coronary intervention.
Statistical analysis
Data were analyzed using SPSS (version 27.0, Chicago, USA) and R (version 4.3.1). The Kolmogorov–Smirnov test was applied to assess data normality. Continuous variables with a normal distribution were presented as mean ± standard deviation and compared using independent t-tests. Non-normally distributed variables were reported as median (interquartile range) and analyzed with the Mann–Whitney U-test. Categorical variables were summarized as counts and percentages and compared using the χ2 test. All the variables with a p-value less than 0.10 in the univariate analysis were included in the multivariate regression analysis using a stepwise forward method to identify independent risk factors for CI-AKI. To examine the dose-response relationship between caIMR and CI-AKI, restricted cubic splines (RCS) were employed. The predictive accuracy of the new and baseline models was assessed with receiver operating characteristic (ROC) curves, net reclassification index (NRI), and integrated discrimination improvement (IDI). A P-value of <0.05 was considered statistically significant.
Results
Patient characteristics
This study included 745 patients, of which 27.4% were female, with a mean age of 63.38 ± 13.10 years. The overall incidence of CI-AKI during hospitalization was 10.6% (79/745). Patients in the CI-AKI group were older, had higher levels of fasting blood glucose (FBG), caIMR, CRP, hs-TnT, and NT-proBNP, and a higher prevalence of diabetes and left anterior descending artery (LAD) involvement. Additionally, they had a lower left ventricular ejection fraction (LVEF) compared to those in the control group. All differences were statistically significant (P < 0.05) (Table 1).
Logistic regression analysis of Ci-AKI
Univariate logistic regression revealed that caIMR (OR = 1.070, 95% CI: 1.050–1.090), FBG, NT-proBNP, diabetes, LAD, and LVEF were significantly associated with the development of CI-AKI during hospitalization (P < 0.05). When the caIMR increases by 10 U each time, the OR was 1.965 (95% CI: 1.628–2.371) (Supplementary Table S1). Multivariate logistic regression, which included all variables with P < 0.10 in univariate logistic regression, identified LVEF (OR = 0.931, 95% CI: 0.900–0.964), FBG (OR = 1.105, 95% CI: 1.019–1.198), NT-proBNP (OR = 1.401, 95% CI: 1.132–1.735), and caIMR (OR = 1.072, 95% CI: 1.051–1.094) as independent predictors of CI-AKI. When the caIMR increases by 10 U each time, the OR was 2.010 (95% CI: 1.646–2.454) (Table 2). RCS analysis indicated a linear dose-response relationship between caIMR and CI-AKI both before and after adjustments, suggesting that higher caIMR levels are associated with an increased risk of CI-AKI (Figure 2).
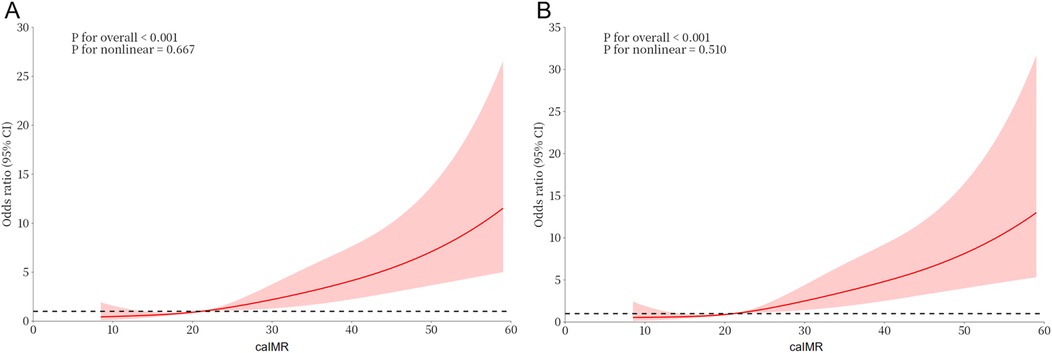
Figure 2. Dose-response relationship between caIMR and CI-AKI. (A) A unadjusted dose-response relationship between caIMR and CI-AKI; (B) an adjusted dose-response relationship between caIMR and CI-AKI. CI-AKI, contrast-induced acute kidney injury; caIMR, coronary angiography-derived index of microcirculatory resistance.
ROC analysis of CI-AKI
Receiver operating characteristic (ROC) analysis demonstrated that the areas under the curve (AUC) for LVEF, FBG, NT-proBNP, and caIMR in predicting CI-AKI were 0.677, 0.613, 0.671, and 0.725, respectively (P < 0.05). The optimal cutoff value for caIMR was 25.95 U, yielding a sensitivity of 68.4% and specificity of 71.5% (Table 3 and Figure 3).
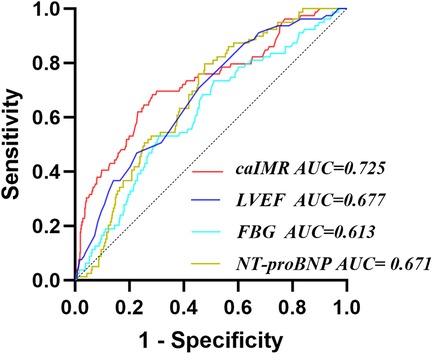
Figure 3. Receiver operating characteristic analysis (ROC) of caIMR for identifying CI-AKI. CI-AKI, contrast-induced acute kidney injury; caIMR, coronary angiography-derived index of microcirculatory resistance; LVEF, left ventricular ejection fraction; NT-proBNP, N-terminal pro-B-type natriuretic peptide; FBG, fasting blood glucose.
Comparison between baseline and new models
Constructing a new model (LVEF, FBG, NT-proBNP, and caIMR) after integrating caIMR in a baseline model (LVEF, FBG, and NT-proBNP). ROC analysis showed that the baseline model had an AUC of 0.732 (95% CI: 0.682–0.783), with a sensitivity of 84.8% and specificity of 57.1%. The new model had an AUC of 0.806 (95% CI: 0.759–0.853), with a sensitivity of 82.3% and specificity of 68.5%. NRI and IDI for the new model were 0.721 (95% CI: 0.4968–0.9452), P < 0.001, and 0.102 (95% CI: 0.0626–0.1412), P < 0.001, respectively. These findings indicate that the new model significantly improves the risk model for CI-AKI in STEMI patients (Table 4, Figure 4 and Supplementary Table S2).
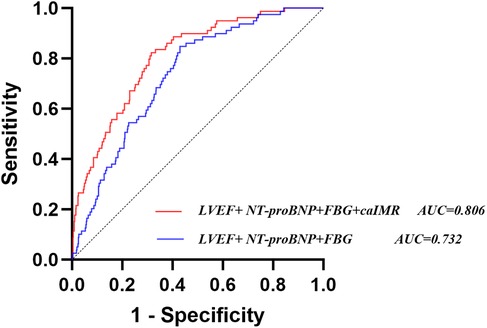
Figure 4. Receiver operating characteristic analysis (ROC) of models for identifying CI-AKI. CI-AKI, contrast-induced acute kidney injury; caIMR, coronary angiography-derived index of microcirculatory resistance; LVEF, left ventricular ejection fraction; NT-proBNP, N-terminal pro-B-type natriuretic peptide; FBG, fasting blood glucose.
Discussion
To our knowledge, this is the first study on the relationship between CMD and CI-AKI. The main findings of this study are as follows: first, elevated caIMR is an independent risk factor for the development of CI-AKI after PCI in STEMI patients; second, there is a linear dose-response relationship between caIMR and CI-AKI; and third, integrating caIMR significantly improves the risk model for CI-AKI.
CI-AKI is characterized by kidney dysfunction occurring within 48–72 h after the administration of contrast agents (23). Although recent evidence suggests that the risk of CI-AKI may have been overestimated, an important prerequisite is the potential exclusion of high-risk patients (24). Numerous studies have shown that CKD patients are less likely to undergo coronary angiography or PCI due to concerns about worsening kidney function (25, 26). In our study, 10.6% of patients still developed CI-AKI. Given the relationship between CI-AKI and poor outcomes (27, 28), and the limited pharmacological treatment options (29), identifying additional risk factors and optimizing risk stratification are essential.
Despite the successful restoration of epicardial coronary flow in STEMI patients via PCI, more than half of STEMI patients still experience myocardial reperfusion injury due to the presence of coronary microcirculatory dysfunction (CMD) (6, 7). In our study, there were 277 (37.2%) patients whose caIMR was greater than 25 U. The reason why CMD is at a relatively low level may be that all the patients included in our study have a TIMI flow grade of 3. Current clinical tools for assessing CMD include cardiac magnetic resonance imaging (CMR) and the index of microcirculatory resistance (IMR). While CMR is non-invasive, its use is limited by indications and high costs, meaning that not all STEMI patients undergo this examination (30). IMR, although the “gold standard” for evaluating microcirculatory dysfunction, requires a pressure-temperature sensing wire and induced maximal hyperemia, which limits its routine clinical application, especially in STEMI patients undergoing primary PCI (8, 9). Recently, caIMR, as an alternative to traditional IMR, has been widely used for prognosis stratification in STEMI patients (10–12). There is a significant correlation (R = 0.782, P < 0.001) and consistency (Bias = −0.398, SD of Bias = 11.96) between caIMR and traditional IMR (10). Given its simplicity and safety, caIMR is particularly beneficial in STEMI patients. In a previous study, Çınar et al. demonstrated that TIMI was independently associated with CI-AKI in STEMI patients undergoing primary PCI and provided better predictive value for CI-AKI than traditional risk factors (15). Nicorandil, a commonly used drug for improving microcirculatory dysfunction, has been shown in prior studies to effectively prevent CI-AKI, whether administered intravenously or orally (16–18). Consistent with these findings, our study identified elevated caIMR as an independent risk factor for the development of CI-AKI after PCI in STEMI patients, and we found a linear dose-response relationship between caIMR and CI-AKI. Given the established relationship between CMD and poor prognosis in STEMI patients, our findings appear to be well-founded. Previous studies have shown that CMD in STEMI patients is associated with larger infarct sizes, worse left ventricular remodeling, and intense inflammatory response, which play an important role in the mechanism of CI-AKI (3, 4, 7, 13, 14). Additionally, AKI is closely associated with endothelial dysfunction (31). It is known that endothelial dysfunction is also an important mechanism of CMD. Therefore, systemic endothelial dysfunction may be a potential mechanism underlying the relationship between caIMR and CI-AKI. In our study, ROC analysis showed that caIMR is a strong predictor of CI-AKI, outperforming FBG, LVEF, and NT-proBNP, aligning with the previous study (15). In previous studies, a threshold value of 25 U for either caIMR or traditional IMR has been proposed for diagnosing microcirculatory dysfunction (8, 9, 32, 33). Interestingly, in our study, we found that a caIMR value of 25.95 U was the cutoff for predicting CI-AKI in STEMI patients after PCI. To our knowledge, this is the first study to establish a cutoff for caIMR in relation to CI-AKI, potentially providing additional insights for IMR-based prognosis assessment in STEMI patients. Differently, in another previous study on STEMI patients, the cutoff value of the angiography-derived IMR was higher than that in our study. This may be attributed to the differences in the measurement software for IMR and the end-point events (34). Our study also demonstrates that caIMR can effectively enhance risk models for CI-AKI. Given its simplicity and safety, caIMR represents a valuable tool for optimizing risk stratification for CI-AKI after PCI in STEMI patients. These findings suggest that patients with elevated caIMR may require more clinical attention and intervention to reduce the risk of CI-AKI.
There are several limitations to this study. First, given its retrospective and observational design, the current study remains inherently descriptive, hypothesis-generating, and speculative in nature. While our study provides a compelling association between CMD and CI-AKI, the capacity for causal inference remains limited. The observed elevation in caIMR in these patients may not solely reflect isolated microvascular dysfunction but rather a broader picture of myocardial damage, impaired reperfusion, and a generally more adverse in-hospital clinical course. Consequently, caIMR might function as a surrogate marker of overall disease severity rather than a direct mechanistic contributor to CI-AKI. Second, the sample size is relatively small, and some conclusions may need to be validated in larger cohorts. Third, this study specifically involves STEMI patients who underwent successful primary PCI, meaning the findings may not be applicable to other patient populations. Fourth, in our study, caIMR was evaluated after the completion of PCI, which made strategies to reduce the use of contrast agents no longer feasible. This limits the clinical utility of caIMR as a tool for early risk stratification or surgical planning. For STEMI patients undergoing primary PCI, the practical measurement of caIMR at an earlier stage may still be a significant challenge. In addition, the implementation of postoperative renal protection strategies mainly based on saline hydration may precisely be restricted in the subgroup of patients identified as high-risk, who have poorer cardiac function. These factors highlight the importance of considering caIMR in a way that can effectively provide a basis for personalized treatment decisions. Fifth, given that the application of functional coronary angiography remains limited in clinical practice, it may still be too early to incorporate caIMR into the prediction model for CI-AKI. Finally, CI-AKI is a surrogate marker for adverse outcomes, but whether the elevated caIMR is associated with persistent renal dysfunction or mortality may require more follow-up in the future to determine.
Conclusion
Elevated caIMR is an independent risk factor for the development of CI-AKI after PCI in STEMI patients. Integrating caIMR significantly improves the risk model for CI-AKI.
Data availability statement
The raw data supporting the conclusions of this article will be made available by the authors, without undue reservation.
Ethics statement
The studies involving humans were approved by Institutional Review Board of the Affiliated Hospital of Xuzhou Medical University (No. XYFY2023-KL203-01). The studies were conducted in accordance with the local legislation and institutional requirements. The ethics committee/institutional review board waived the requirement of written informed consent for participation from the participants or the participants’ legal guardians/next of kin because as a retrospective study posing no risk to patients, the requirement for written informed consent was waived in accordance with IRB guidelines.
Author contributions
SZ: Writing – original draft, Writing – review & editing. JL: Writing – original draft, Writing – review & editing. KG: Writing – original draft, Writing – review & editing. YW: Writing – original draft, Writing – review & editing. ZD: Writing – original draft, Writing – review & editing. YL: Writing – original draft, Writing – review & editing.
Funding
The author(s) declare that financial support was received for the research and/or publication of this article. This work was partly supported by Xuzhou Municipal Health Commission (Grant XWKYHT20230073).
Conflict of interest
The authors declare that the research was conducted in the absence of any commercial or financial relationships that could be construed as a potential conflict of interest.
Generative AI statement
The author(s) declare that no Generative AI was used in the creation of this manuscript.
Publisher's note
All claims expressed in this article are solely those of the authors and do not necessarily represent those of their affiliated organizations, or those of the publisher, the editors and the reviewers. Any product that may be evaluated in this article, or claim that may be made by its manufacturer, is not guaranteed or endorsed by the publisher.
Supplementary material
The Supplementary Material for this article can be found online at: https://www.frontiersin.org/articles/10.3389/fcvm.2025.1541208/full#supplementary-material
References
1. Virani SS, Alonso A, Benjamin EJ, Bittencourt MS, Callaway CW, Carson AP, et al. Heart disease and stroke statistics-2020 update: a report from the American Heart Association. Circulation. (2020) 141(9):e139–596. doi: 10.1161/CIR.0000000000000757
2. Silvain J, Nguyen LS, Spagnoli V, Kerneis M, Guedeney P, Vignolles N, et al. Contrast-induced acute kidney injury and mortality in ST elevation myocardial infarction treated with primary percutaneous coronary intervention. Heart. (2018) 104(9):767–72. doi: 10.1136/heartjnl-2017-311975
3. Libby P, Pasterkamp G, Crea F, Jang IK. Reassessing the mechanisms of acute coronary syndromes. Circ Res. (2019) 124(1):150–60. doi: 10.1161/CIRCRESAHA.118.311098
4. McCullough PA, Choi JP, Feghali GA, Schussler JM, Stoler RM, Vallabahn RC, et al. Contrast-induced acute kidney injury. J Am Coll Cardiol. (2016) 68(13):1465–73. doi: 10.1016/j.jacc.2016.05.099
5. Rihal CS, Textor SC, Grill DE, Berger PB, Ting HH, Best PJ, et al. Incidence and prognostic importance of acute renal failure after percutaneous coronary intervention. Circulation. (2002) 105(19):2259–64. doi: 10.1161/01.cir.0000016043.87291.33
6. Lerman A, Holmes DR, Herrmann J, Gersh BJ. Microcirculatory dysfunction in ST-elevation myocardial infarction: cause, consequence, or both? Eur Heart J. (2007) 28(7):788–97. doi: 10.1093/eurheartj/ehl501
7. Camici PG, Crea F. Coronary microvascular dysfunction. N Engl J Med. (2007) 356(8):830–40. doi: 10.1056/NEJMra061889
8. Fearon WF, Balsam LB, Farouque HM, Caffarelli AD, Robbins RC, Fitzgerald PJ, et al. Novel index for invasively assessing the coronary microcirculation [published correction appears in Circulation. (2003) 108(25):3165]. Circulation. (2003) 107(25):3129–32. doi: 10.1161/01.CIR.0000080700.98607.D1
9. De Maria GL, Alkhalil M, Wolfrum M, Fahrni G, Borlotti A, Gaughran L, et al. Index of microcirculatory resistance as a tool to characterize microvascular obstruction and to predict infarct size regression in patients with STEMI undergoing primary PCI. JACC Cardiovasc Imaging. (2019) 12(5):837–48. doi: 10.1016/j.jcmg.2018.02.018
10. Choi KH, Dai N, Li Y, Kim J, Shin D, Lee SH, et al. Functional coronary angiography-derived index of microcirculatory resistance in patients with ST-segment elevation myocardial infarction [published correction appears in JACC Cardiovasc Interv. (2022) 15(19):2001. doi: 10.1016/j.jcin.2022.08.032]. JACC Cardiovasc Interv. (2021) 14(15):1670–84. doi: 10.1016/j.jcin.2021.05.027
11. Abdu FA, Liu L, Mohammed AQ, Yin G, Xu B, Zhang W, et al. Prognostic impact of coronary microvascular dysfunction in patients with myocardial infarction with non-obstructive coronary arteries. Eur J Intern Med. (2021) 92:79–85. doi: 10.1016/j.ejim.2021.05.027
12. Chen L, Sang C, Wu Y, Chen W, Ren Y, Che W, et al. Coronary angiography-derived index of microcirculatory resistance associated with new-onset atrial fibrillation in patients with STEMI. Can J Cardiol. (2024) 40(3):434–43. doi: 10.1016/j.cjca.2023.10.025
13. Hombach V, Grebe O, Merkle N, Waldenmaier S, Höher M, Kochs M, et al. Sequelae of acute myocardial infarction regarding cardiac structure and function and their prognostic significance as assessed by magnetic resonance imaging. Eur Heart J. (2005) 26(6):549–57. doi: 10.1093/eurheartj/ehi147
14. Eitel I, de Waha S, Wöhrle J, Fuernau G, Lurz P, Pauschinger M, et al. Comprehensive prognosis assessment by CMR imaging after ST-segment elevation myocardial infarction. J Am Coll Cardiol. (2014) 64(12):1217–26. doi: 10.1016/j.jacc.2014.06.1194
15. Çınar T, Karabağ Y, Ozan Tanık V, Çağdaş M, Rencüzoğulları İ, Öz A. The investigation of TIMI risk index for prediction of contrast-induced acute kidney injury in patients with ST elevation myocardial infarction. Acta Cardiol. (2020) 75(1):77–84. doi: 10.1080/00015385.2018.1551263
16. Nawa T, Nishigaki K, Kinomura Y, Tanaka T, Yamada Y, Kawasaki M, et al. Continuous intravenous infusion of nicorandil for 4 h before and 24 h after percutaneous coronary intervention protects against contrast-induced nephropathy in patients with poor renal function. Int J Cardiol. (2015) 195:228–34. doi: 10.1016/j.ijcard.2015.05.078
17. Fan Z, Li Y, Ji H, Jian X. Efficacy of oral nicorandil to prevent contrast-induced nephropathy in patients with chronic renal dysfunction undergoing an elective coronary procedure. Kidney Blood Press Res. (2019) 44(6):1372–82. doi: 10.1159/000503160
18. Yu ZZ, Xu ZX, Ruan YH, Hu LL, Wen MH, Huang TQ, et al. Protective effect of nicorandil on contrast-induced acute kidney injury after emergency percutaneous coronary intervention. J Cardiovasc Pharmacol Ther. (2023) 28:10742484231174296. doi: 10.1177/10742484231174296
19. Ibanez B, James S, Agewall S, Antunes MJ, Bucciarelli-Ducci C, Bueno H, et al. 2017 ESC guidelines for the management of acute myocardial infarction in patients presenting with ST-segment elevation: the task force for the management of acute myocardial infarction in patients presenting with ST-segment elevation of the European Society of Cardiology (ESC). Eur Heart J. (2018) 39(2):119–77. doi: 10.1093/eurheartj/ehx393
20. van der Molen AJ, Reimer P, Dekkers IA, Bongartz G, Bellin MF, Bertolotto M, et al. Post-contrast acute kidney injury—part 1: definition, clinical features, incidence, role of contrast medium and risk factors: recommendations for updated ESUR contrast medium safety committee guidelines. Eur Radiol. (2018) 28(7):2845–55. doi: 10.1007/s00330-017-5246-5
21. Inker LA, Schmid CH, Tighiouart H, Eckfeldt JH, Feldman HI, Greene T, et al. Estimating glomerular filtration rate from serum creatinine and cystatin C [published correction appears in N Engl J Med. (2012) 367(7):681] [published correction appears in N Engl J Med. (2012) 367(21):2060]. N Engl J Med. (2012) 367(1):20–9. doi: 10.1056/NEJMoa1114248
22. Kidney Disease: Improving Global Outcomes (KDIGO) CKD Work Group. KDIGO 2024 cinical practice guideline for the evaluation and management of chronic kidney disease. Kidney Int. (2024) 105(4S):S117–314. doi: 10.1016/j.kint.2023.10.018
23. Mehran R, Nikolsky E. Contrast-induced nephropathy: definition, epidemiology, and patients at risk. Kidney Int Suppl. (2006) 69(100):S11–5. doi: 10.1038/sj.ki.5000368
24. Caspi O, Habib M, Cohen Y, Kerner A, Roguin A, Abergel E, et al. Acute kidney injury after primary angioplasty: is contrast-induced nephropathy the culprit? J Am Heart Assoc. (2017) 6(6):e005715. doi: 10.1161/JAHA.117.005715
25. Wong JA, Goodman SG, Yan RT, Wald R, Bagnall AJ, Welsh RC, et al. Temporal management patterns and outcomes of non-ST elevation acute coronary syndromes in patients with kidney dysfunction. Eur Heart J. (2009) 30(5):549–57. doi: 10.1093/eurheartj/ehp014
26. Medi C, Montalescot G, Budaj A, Fox KA, López-Sendón J, FitzGerald G, et al. Reperfusion in patients with renal dysfunction after presentation with ST-segment elevation or left bundle branch block: GRACE (global registry of acute coronary events). JACC Cardiovasc Interv. (2009) 2(1):26–33. doi: 10.1016/j.jcin.2008.09.010
27. Griffiths RI, Cavalcante R, McGovern AM, Bhave A, Hargens LM, Solid CA, et al. Cost to medicare of acute kidney injury in percutaneous coronary intervention. Am Heart J. (2023) 262:20–8. doi: 10.1016/j.ahj.2023.03.013
28. Ng AK, Ng PY, Ip A, Lam LT, Ling IW, Wong AS, et al. Impact of contrast-induced acute kidney injury on long-term major adverse cardiovascular events and kidney function after percutaneous coronary intervention: insights from a territory-wide cohort study in Hong Kong. Clin Kidney J. (2021) 15(2):338–46. doi: 10.1093/ckj/sfab212
29. Efe SC, Keskin M, Toprak E, Arslan K, Öz A, Güven S, et al. A novel risk assessment model using urinary system contrast blush grading to predict contrast-induced acute kidney injury in low-risk profile patients. Angiology. (2021) 72(6):524–32. doi: 10.1177/00033197211005206
30. Ahn SG, Hung OY, Lee JW, Lee JH, Youn YJ, Ahn MS, et al. Combination of the thermodilution-derived Index of microcirculatory resistance and coronary flow reserve is highly predictive of microvascular obstruction on cardiac magnetic resonance imaging after ST-segment elevation myocardial infarction. JACC Cardiovasc Interv. (2016) 9(8):793–801. doi: 10.1016/j.jcin.2015.12.025
31. Zuk A, Bonventre JV. Acute kidney injury. Annu Rev Med. (2016) 67:293–307. doi: 10.1146/annurev-med-050214-013407
32. Liu L, Dai N, Yin G, Zhang W, Mohammed AQ, Xu S, et al. Prognostic value of combined coronary angiography-derived IMR and myocardial perfusion imaging by CZT SPECT in INOCA. J Nucl Cardiol. (2023) 30(2):684–701. doi: 10.1007/s12350-022-03038-w
33. Dai N, Che W, Liu L, Zhang W, Yin G, Xu B, et al. Diagnostic value of angiography-derived IMR for coronary microcirculation and its prognostic implication after PCI. Front Cardiovasc Med. (2021) 8:735743. doi: 10.3389/fcvm.2021.735743
34. De Maria GL, Scarsini R, Shanmuganathan M, Kotronias RA, Terentes-Printzios D, Borlotti A, et al. Angiography-derived index of microcirculatory resistance as a novel, pressure-wire-free tool to assess coronary microcirculation in ST elevation myocardial infarction. Int J Cardiovasc Imaging. (2020) 36(8):1395–406. doi: 10.1007/s10554-020-01831-7
Keywords: cardiovascular disease, contrast-induced acute kidney injury, STEMI, coronary microvascular dysfunction, index of microcirculatory resistance
Citation: Zhong S, Lu J, Gong K, Wu Y, Dong Z and Lu Y (2025) Coronary angiography–derived index of microcirculatory resistance associated with contrast-induced acute kidney injury in patients with STEMI. Front. Cardiovasc. Med. 12:1541208. doi: 10.3389/fcvm.2025.1541208
Received: 7 December 2024; Accepted: 22 April 2025;
Published: 1 May 2025.
Edited by:
Gabriele Pesarini, Integrated University Hospital Verona, ItalyReviewed by:
Simone Fezzi, University of Verona, ItalyRoxana Oana Darabont, Carol Davila University of Medicine and Pharmacy, Romania
Copyright: © 2025 Zhong, Lu, Gong, Wu, Dong and Lu. This is an open-access article distributed under the terms of the Creative Commons Attribution License (CC BY). The use, distribution or reproduction in other forums is permitted, provided the original author(s) and the copyright owner(s) are credited and that the original publication in this journal is cited, in accordance with accepted academic practice. No use, distribution or reproduction is permitted which does not comply with these terms.
*Correspondence: Yuan Lu, eHlmeWx1eXVhbkAxNjMuY29t; Zishuang Dong, eGlhb21vZ3VkenNAMTYzLmNvbQ==
†These authors have contributed equally to this work