- 1School of Education, Ben-Gurion University of the Negev, Beer-Sheva, Israel
- 2Homeland Security Institute, Ben-Gurion University of the Negev, Beer-Sheva, Israel
Introduction: Complex systems are prevalent in many scientific and engineering disciplines, which makes system thinking important for students of these fields. Duchifat 3 is a unique engineering educational extracurricular program, where high school students designed, assembled, and tested a nano-satellite.
Methods: This study applied qualitative methods to explore how the participants’ systems-thinking developed during the program. Participants were interviewed using the repertory grid interview, and a semi structured interview at the beginning and at the end of the project, while various observations were conducted throughout.
Results: While the participants were initially assigned narrow roles, each dealing with a single sub-system of the satellite, some chose to be involved with other sub-systems and aspects of the project. Our findings show that the broader the participants’ involvement was, the greater the progress they experienced in their systems-thinking. Participants who stayed focused on a single subsystem did not show progress, while participants who involved themselves with several sub-systems exhibited a more meaningful progress.
Discussion: Although the program design aimed to assign students to a narrow role to enable them to achieve the educational goals, from the perspective of systems-thinking this was counterproductive. These findings shed light on the design of engineering programs such as the one examined here in terms of systems-thinking development. We discuss the implications of the findings for similar programs and make suggestions for improvement.
Introduction
Trends in science education have emphasized the integration of core ideas and crosscutting concepts in STEM subjects—including the complex systems ideas that comprise systems thinking (NGSS Lead States, 2013). Complex systems are prevalent in many scientific and engineering fields, and at all scales—from the micro-scale of a single cell to complex macro systems such as cities or ecosystems (Yoon et al., 2017). The ability to understand the whole system and see the big picture, understand interconnections, understand synergies, and understand the system from multiple perspectives is one of the cognitive characteristics required of successful systems professionals (Frank, 2010). Although research in engineering system thinking is somewhat limited, it is becoming ever more important as engineering systems become more complex (Greene and Papalambros, 2016).
This study examines a specially designed engineering extracurricular program, in which high school students participated in the task of assembling a nanosatellite, Duchifat 3, which was launched into orbit in 2019 and was functional in space for about a year. The program involved the use of STEM content, and is part of a series of projects where high school students engage in the task of engineering, integrating and testing CubeSat satellites (Brosch et al., 2017; Millan et al., 2019). Although there are a number of programs that introduce satellite design into high schools (Millan et al., 2019), to the best of our knowledge, this is the first in-depth study of such an educational program. In this paper, we analyze the development of the participants’ systems thinking, describing the progress the participants made and offering insights into what may encourage such progress in terms of program design.
Background
Systems thinking
Systems thinking is a way to understand, explain, and interpret complex and dynamic systems. A complex system is composed of various elements that interact via simple local rules (Fuentes, 2014) and give rise to certain functions (Gilissen et al., 2020). Interactions are characterized by feedback loops and are usually nonlinear (Fuentes, 2014; Yoon et al., 2018). Complex systems have a hierarchical structure, with multiple components that interact dynamically, nonlinearly, and simultaneously, within or across levels. Such interactions, moreover, are often implicit, occurring over time, at varying microscopic and macroscopic levels, and with indirect causality that is difficult for students to trace and grasp (Hmelo-Silver and Azevedo, 2006; Schneeweiß and Gropengießer, 2019). Systems thinking is a set of skills that can be taught separately, and a learning strategy that explicitly considers system characteristics in trying to understand and predict natural phenomena and complex man-made systems (Verhoeff et al., 2018).
There are several conceptualizations of systems thinking skills in the literature. Table 1 maps these conceptualizations, highlighting their common themes. Though not a comprehensive or exhaustive review of the literature, the table features those works in the field that are most prominent and relevant to this study. As this table shows, a proficient systems thinker must identify the systems’ components and their interrelations, consider the system and its subsystems at various scales and levels of organization, identify and describe feedback loops that may control processes in the system, identify the dynamic relationships and consider the temporal dynamics of the system, make generalizations and predictions in regard to the system, and identify emergent properties and understand decentralized control.
Fostering systems thinking
Several strategies for fostering systems thinking have emerged from the literature. Our study focused on the three most prominent, as identified by Gilissen et al. (2021): (1) Modeling; (2) Cross-level reasoning; and (3) Use of systems language.
Modeling
Models are representations of natural phenomenon, data, theory, or of engineered manmade objects and processes. They may consist of 3D or 2D representations, and they may be verbal, mathematical, or computational. Models represent a subset of the parts of the modeled entity depending on its purposes. (Krell et al., 2019). Modeling can help students better understand the dynamics of a system and integrate knowledge about it (Wilson et al., 2020). Its use in education consists of two central parts: models that communicate scientific or engineering content to students and modeling done by students to gain insight (Upmeier zu Belzen et al., 2019). In the latter case, modeling is instrumental in making students’ understanding visible, helping students organize their ideas, and facilitating constructive and collaborative discussion (Hmelo-silver et al., 2017; Bielik et al., 2021). It allows students to engage in inquiry practices by gathering data, generating hypotheses, and testing them (Hmelo-Silver et al., 2015).
Cross level reasoning
Weintrop et al. (2016) identify cross-level reasoning (thinking across levels in their terms) as core to systems thinking. Systems can be understood by analyzing different levels of organization from the micro scale to the macro scale. Different insights can be gained from examining different levels which can lead to a better understanding of the emergent characteristics of the system as a whole (Weintrop et al., 2016). Challenging students to reason between various levels of organization has been shown to improve system thinking (Verhoeff et al., 2008; Gilissen et al., 2021).
Systems language
Systems language is the explicit use of terms that refer to system characteristics. Proponents of this strategy contend that when teaching about complex systems and encouraging system thinking, teachers should make explicit use of systems language and encourage their students to use that language explicitly (Eberbach et al., 2021). Deconstructing a phenomenon to its characteristics and discussing them explicitly has been shown to help clarify it for both students and teachers (Zion and Klein, 2015). Jordan et al. (2013) showed that exposure to the systems language helps students in their explanations by linking multiple ideas and improving their explanations’ sophistication by enriching references to invisible elements. Nguyen and Santagata (2021) have shown that the teacher’s prompts greatly affect how middle school students respond when asked about connections in systems. The language teacher’s use is adopted by students, not only in their discussions with the teacher but also in their group discussions without the immediate presence of the teacher, thus assisting their understanding of systems (Hmelo-silver et al., 2015). Systems language may help students to better communicate information about s system, which is also important for systems thinking according to Weintrop et al. (2016).
Evaluating students’ conceptualization of complex phenomena
Several models have been put forth as useful means of representing the various forms and levels of system thinking. One promising approach is Structure-Behavior-Function (SBF) thinking (Hmelo-Silver et al., 2007). The “structure” in SBF models is represented in terms of the system’s elements or components, the substances contained in the components, and connections among the components (Goel et al., 2009). “Behavior” refers to the mechanisms by which the structures perform their function, represented as a sequence of states and transitions between these states. Explanations for state transitions may include scientific laws, functions of the subsystems, structural constraints, or other behaviors (Hmelo-Silver et al., 2007; Goel et al., 2009). “Function” refers to the role or the purpose of elements in the system. A function is represented as a schema containing a reference to the behavior that accomplishes the function (Hmelo-Silver et al., 2007; Goel et al., 2009). Hmelo-Silver et al. (2017) modified the SBF model, creating an alternative conceptual framework called Components-Mechanisms-Phenomena (CMP). This framework provides a representation of all the system’s attributes, including the structures (components) within the system, the specific processes and interactions (mechanisms) that occur between them, and the macro scale of processes and patterns within a system—the phenomena.
In addition to providing a vocabulary for discussing complex systems, these frameworks also serve as a means of externalizing and assessing the development of students’ systems thinking. Thus, for instance, when explaining complex systems within the CMP framework, referring to phenomena and mechanisms indicates more advanced systems thinking than referring to components (Hmelo-Silver et al., 2017; Snapir et al., 2017).
Assessing traditional learning is often challenging enough, but modern notions of science teaching, such as system thinking, require creative assessment designs. One tool that has been used on multiple occasions as a means of assessing systems thinking is Kelly’s repertory grid (RG) technique, which explores learners’ perceptions through the personal constructs they create. The technique is based on Kelly’s theory of personal constructs, which states that the world is perceived in terms of the meaning people apply to it (Kelly, 1955). According to this theory, people make sense of the world by viewing reality through personal constructs. These allow them to make predictions about the future, which are later tested against reality and reformulated in an iterative process (Jankowicz, 2001). As a person experiences repeated events, he or she starts to make sense of them, identifying similarities and differences between events, and separating them based on constructs (Rozenszajn et al., 2021). As a result, one’s personality, attitudes, and concepts are developed on a system of personal constructs which are tacit in nature.
Kelly developed a methodology for exploring these systems of personal constructs with repertory grids (RG). This technique is a form of highly structured interview, designed to assign relationships to personal constructs and given objects of discourse (Kelly, 1955). The repertory grid technique has been acknowledged for several decades as a reliable way to represent how learners think and help them represent their mental models explicitly (Bezzi, 1999; Ben-Zvi Assaraf and Orion, 2010; Rozenszajn and Yarden, 2015; Snapir et al., 2017; Wu et al., 2018). Previous studies have demonstrated the strength and validity of the RG as an effective tool for assessing learners’ conceptual models, providing valuable insights into the learning process, and identifying problems in understanding biological concepts (McCloughlin, 2017). Several studies have demonstrated the added value of the technique for assessing students’ conceptual models and system thinking abilities in the context of ecology (Keynan et al., 2014), biogeochemical cycles (Ben-Zvi Assaraf and Orion, 2010), and human body complexity (Ben Zvi Assaraf et al., 2013; Snapir et al., 2017).
Research question
This paper presents part of a larger study that examined the Duchifat 3 extracurricular project, in which high school students were involved in designing, building, and testing a nano-satellite. It addresses the following question:
What aspects of systems thinking were exhibited by the participants and how did their systems-thinking progress during the program?
Methods
Methodological foundation
This study is based on the qualitative research paradigm and utilizes a case study approach (Mills et al., 2010). The context-dependent knowledge that we glean from it takes into consideration the idiosyncrasies of the examined case, including its different elements, such as students, teachers, resources, and overall culture (Case and Light, 2011). As the data did not allow for a fully worked-up grounded theory, the main analytic method used in this work is thematic analysis (Braun and Clarke, 2006).
Research setting
This study examines a unique engineering education program, which involved high school students in the design and assembly of a fully functional CubeSat that was subsequently launched to space. CubeSats are a type of a very small satellite, based on a standardized unit of mass and volume (10 cm × 10 cm × 10 cm). CubeSats have been incorporated into education in several previous initiatives, but to the best of our knowledge, ours is the first study of a satellite building project conducted at a high school. One study, for instance, followed engineering graduate students while developing components such as antennae for inter-satellite communications (Martínez Rodríguez-Osorio and Fueyo Ramírez, 2012). Another studied students from Cal Poly and Stanford, who were involved in developing the CubeSat standard (Lan et al., 2006). These studies, however, are quite superficial, only briefly describing the programs and the authors’ impressions of them.
The program examined here is the Duchifat program—a CubeSat-based program, which involves 12–18-year-old students in the task of engineering, integrating, and testing a satellite. The program was a joint endeavor by an extracurricular science center for high school students situated in a major city within Israel’s central urban area and a Southern Israeli high school. This study follows the construction of Duchifat 3, the third of a series of satellite-building extracurricular projects undertaken by high school students, focusing specifically on the participating students from this high school.
The Duchifat program was designed as a project-based learning experience. According to a recent review, PBL is the dominant educational paradigm used in interdisciplinary engineering education (Van den Beemt et al., 2020). PBL in engineering education can expose students to core engineering competencies (Nguyen et al., 2020).
Research participants and program description
Participation in the program was voluntary and required students to be in the top 25% in their class in science, mathematics, and English. More than 20 students started this program, but only 15 students from the high school completed it. Thirteen of the who eventually finished the program also agreed to take part in this study. However, not all the participants in the study were able to complete all the research tools. The program had a high attrition rate, and the study demands proved to be too much for some of the participants who elected to drop from the research. The study was thus completed by only seven participants in total (Table 2). All names given in this paper are pseudonyms in order to protect the participants’ anonymity. Participants who did not finish all research tools but appear in some of the observed interactions are referred to as “student.” Because this study focuses specifically on the development of the participants’ systems thinking, we will focus on the case studies of four of the participants that exemplify the trends we observed. A comprehensive and detailed description of all the participants would be well beyond the scope of this paper.
The satellite designed in the Duchifat 3 program was a CubeSat—a satellite composed of 10 cm × 10 cm × 10 cm cubes that can be fitted with different components, which can be bought off-the-shelf from various companies. The satellite was designed as three cubes joined together (3 U) to create a 30 cm × 10 cm × 10 cm structure. Subsystems included an Electrical Power System (EPS), communications system, Attitude Determining and Control System (ADCS), On-Board Computer (OBC), and an RGB camera as the main payload.
Figures 1A,C show the satellite at the initial stage of testing upon its arrival at the school. The initial stage of testing, which consisted of turning the different subsystems on and off was conducted by some of the participants. The students worked in teams, participating in various aspects of the satellite’s design, as well as a number of design reviews. These reviews consisted of a preparation period of 2–3 weeks, where students prepared presentations of their work and were reviewed by staff and various experts. The preparations for these reviews and other learning activities entailed the analysis of system models and their construction. The modeling activities necessitated cross-level reasoning in order to consider subsystems and their components (which are subsystems themselves) and considering system characteristics, such as boundaries, input and output, components and their interactions. The systems engineer’s practices led to the implicit introduction of these strategies.
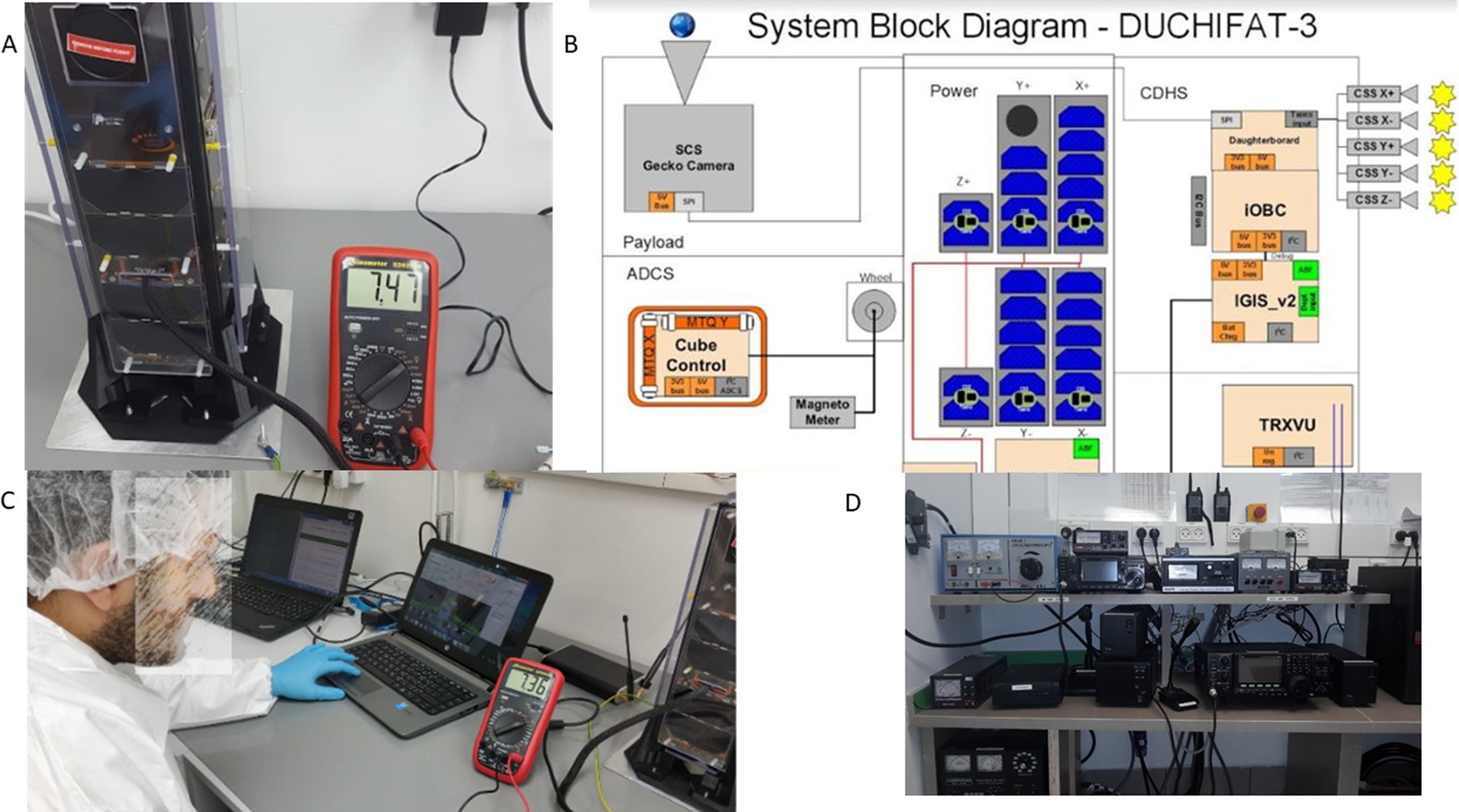
Figure 1. The Duchifat 3 CubeSat during initial tests, the ground station and a diagram representing the satellite’s subsystems.
The students were also involved in the operation of the ground station that was created in the school (Figure 1D).
It is essential to emphasize that this program is an after-school program and that an expert systems engineer led the majority of the learning activities. The nature of the tasks necessitated verbal instructions, and the work was performed in line with the systems engineer’s implementation of industry practices and standards. Therefore, we are unable to provide the materials that would have been accessible if this program had been included in the standard curriculum.
Figure 1B shows a diagram of the satellite’s subsystems which the participants analyzed and used as a resource in their models of how the different subsystems interact in terms of both hardware and software. The analysis of this diagram entails considering system characteristics—identifying the components, how they connect with each other and their interactions, their input and output when considering them in terms of hardware and in terms of software and data transfer. Going into further details regarding the subsystems of these subsystems entails cross-level reasoning (for instance, how the reactions wheels, magnetometers and magnetotorquers interact and are controlled in order to implement the function of the ADCS). Some of the students took part in designing testing protocols and scenarios but testing the satellite itself was eventually conducted elsewhere and not by the participants. The satellite was eventually launched from India, onboard the PSLV C-48, on December 11, 2019.
The program began with a semester of preparation, in which lecturers from various universities conducted weekly classes to provide the students with an introduction to the topic of satellites (Millan et al., 2019). The students were then divided into teams (according to their preference and field of interest) and assigned to work on various components of the larger project, under the guidance of expert scientists or engineers. At the program’s early stages, the project was guided by a physics PhD student who was in the final stage of his degree. After that, the students were supported by a computer science student in the last year of his first degree and mentored by a professional systems engineer with experience in the space industry. Teachers from the school were also involved with the project at different stages. Their roles were to head the establishment of the ground station in the school, teach and mentor the students and coordinate with the outside experts. Each team of students also independently studied relevant topics, such as the conditions in space that might be relevant to their mission, the satellite’s optional components, and other subjects as they became relevant. For a detailed description of the program and its contents, see Table 3.
Although the original program planned for the participants to take part in programming the satellite, the participants described in this paper did not take part in that aspect of the project. They did study some programming and the algorithms of the satellite’s software in relation to the various subsystems, but did not participate in writing any code.
The participants mainly focused on learning the scientific background, researching the components and subsystems, and presenting the design to various stakeholders in several design reviews. The design reviews entailed producing models of the satellite at different levels of organization, from the single component (e.g., reaction wheels) through the subsystem (e.g., the ADCS) to the satellite as a whole (e.g., input and output of the entire satellite system). They were explicitly asked to consider the boundaries at each scale, the input and output, unintended consequences, system requirements and constraints research tools.
Repertory grid
We used the repertory grid twice—once during months 1–2 of the program and again after month 16 (Table 3). Using the repertory grid allowed us to explore how the participants understand certain terms relevant to their project, subsystems in the satellite, and the various relationships among them. This allowed us to determine the level of sophistication in the participants’ understanding of these concepts and their level of systems thinking. Comparing their sophistication level at the beginning and end of the program can illuminate the process that the students went through while participating in the project, (i.e., whether their understanding of the subject matter changed and whether they made any progress in their systems thinking).
The building blocks of the RG are elements (the topics of study within the domain of the investigation), constructs (the participants’ ideas about these elements), and ratings (relations among elements and constructs as viewed by the participants). Elements can either be supplied by the researcher, or elicited from the participants themselves (Latta and Swigger, 1992). In this case, they consisted of a list of terms obtained after consulting several academics and industry experts.
Each provided a list of 15–20 terms that they deemed most important for a student participating in such a project to understand. These lists were pooled together, and each term received a numeric score based on the number of times it occurred. The 15 top-scoring terms were gathered into a new list of terms: ADCS, communications, launch, remote sensing, EPS, flight software, rockets, structure design, thermal control, solar panels, payload, orbit control, tests and integration, space environment, telemetry, and command. These elements represented all the subsystems within the satellite (ADCS, EPS, etc.) as well as parts of those subsystems (solar panels). The list also contained processes (structure design, thermal control, etc.), elements that are peripheral to actual satellite-building (launch, rockets), and non-physical elements (flight software).
Constructs represent the participants’ interpretations of the elements and the relationships between them. This study employed the most common method of eliciting constructs, the triadic elicitation process, in which the participants are asked to compare three elements and describe in what ways two are similar to one another and different from the third (Hunter and Beck, 2000; Edwards et al., 2009). Each participant drew eight triads for every RG interview.
From these descriptions, we extracted a short sentence to represent the construct that was reflected by the participant’s explanation, using the participant’s own words as closely as possible. These were then used to create a bipolar description relating to the components of the investigation. For example, a student may say that the ADCS and EPS are both subsystems in the satellite while the space environment is not. From this, the researchers would deduce the construct of a subsystem/not a subsystem in the satellite.
In the second stage, the participant received a large table containing all the elements (terms), one in each column, and the eight constructs, one in each row. They were then asked to rate, on a scale of 1–5, the strength of the relationship between each term and each of their constructs, where 1 represents the strongest relationship and 5 the weakest. For example, regarding the construct of a subsystem/not a subsystem, a student may indicate a high relation (1) for the term “communications,” and a low relation (5) for the term “launch.”
The three building blocks, (elements, constructs, and ratings) were mapped onto a grid using Rep Plus v1.1 software. This software calculates correlations between the elements as well as between the constructs based on the participant’s ratings, and presents grids of relations that demonstrate how similar the participant perceives them to be. The more similar the ratings are for two constructs or two elements, the higher they are correlated by the program. An example of such a grid with its explanation is given in Figure 2.
In the top part of the grid the two polars of each construct are written on opposite sides of the table, which shows the ratings given by the participants. The scale above the constructs on the right side indicates the degree of similarity between the constructs based on the point at which they branch out. The list of elements is written on the bottom and organized by the degree of similarity which is indicated by the point at which elements branch out. Thus, the more similar a participant perceives two elements to be, the closer they would be to each other. For further explanations of the method see Rozenszajn et al. (2021).
Observations
Participatory observations were carried out throughout the program by the first author, and audio recordings of several of those observations were transcribed verbatim by a research assistant. One major activity, where students were preparing for a preliminary design review by producing a presentation that described the proposed software architecture, was videotaped and analyzed.
Analytic process
The data presented here were discussed by the authors throughout the analytic process until we were able to reach a consensus. The first author was deeply involved with the project and took part in most activities—both documented and undocumented. In essence, he performed participant observation—a central method in cultural anthropology, in which the researcher takes part in a group and participates in its daily activities, interactions and events (DeWalt and DeWalt, 2011). Doing this allowed the first author to gain an extensive familiarity with the participants. The other researchers had only a few very brief encounters with the participants. These different positionalities allowed us to gain an in-depth understanding of the participants and their experience, while ensuring the validity of our interpretation of the data.
Repertory grid analysis
Each of the seven participating students produced two repertory grids—one pre and one post—representing the constructs arising from their descriptions of the elements they had picked and the relationships between them. We then compared the grids from the end of the project with the ones from the early stages, which allowed us to infer the development of each participant’s perceptions regarding these terms. In the second stage of analysis, the elicited constructs were grouped into primary categories according to SBF theory (Hmelo-Silver and Pfeffer, 2004; Goel et al., 2009). We used the SBF model to determine the level of sophistication of the understanding exhibited by the participants (Table 4).
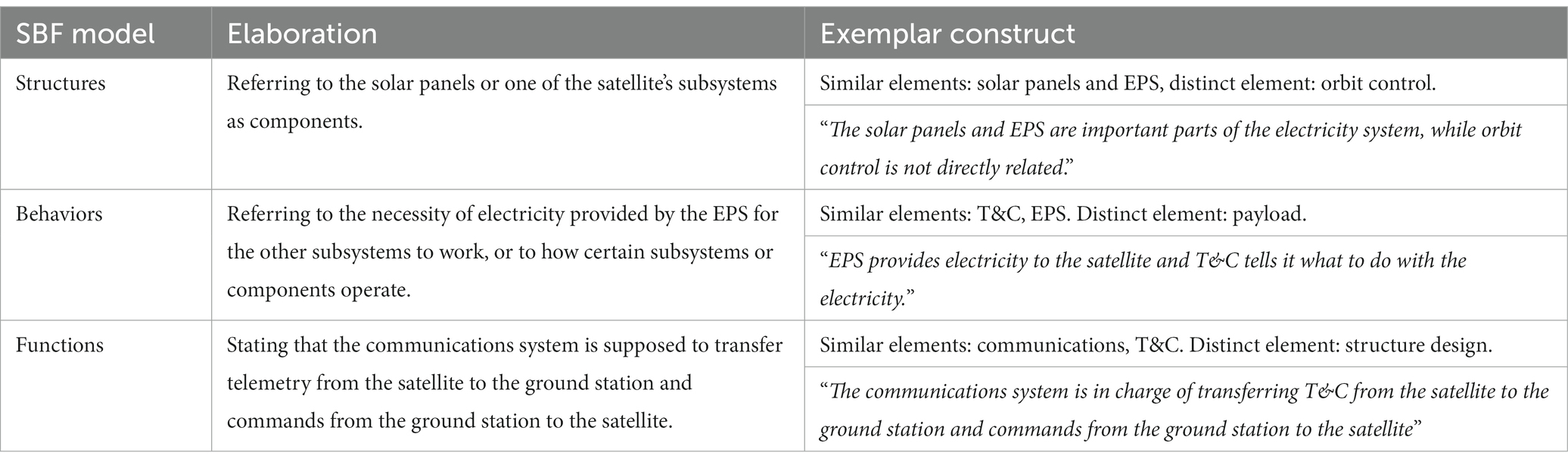
Table 4. Examples of how structures, behaviors and functions are displayed in the repertory grid interview.
This categorization process was conducted separately and discussed by the researchers until a consensus was reached.
Results
Systems thinking
We analyzed the participants’ answers in the first stage of the repertory grid, assigning each construct to either the structure, behavior, or function category. The results are presented in Figure 3.
Overall, participants demonstrated varying degrees of change in their system thinking. As Figure 3 shows, most participants had constructs categorized as functions even at the start of the program. An exception to that is John who had no functions in either occasion, while Sarah moved from having none at the first to having two at the end. Interestingly, we found that the participants’ system thinking development was dependent on the roles they assumed in the program and on how broad their involvement was with the project. We illustrate this point below using four representative case studies. However, it is important to stress that the other three participants exhibited the same pattern and were not included for the sake of brevity.
John’s case
John was a gifted student who was also a ham radio enthusiast. He skipped a grade in primary school and was involved in an advanced mathematics program in one of the Israeli universities. He served as the head of the communications team and managed the ground station which was built in the school. He was very involved with the communications aspect of the project, but refrained from involving himself in any other system and refused to delve into programming, which the students were encouraged to do. He was one of the few students who were able to finish their research projects, which also focused on satellite-ground station communications. Thus, John was deeply involved with a narrow aspect of the project.
In his first repertory grid (Figure 4), John referred primarily to structures, but also to some behaviors. For instance, he said that “T&C is bidirectional [from the earth to the satellite and vice versa], while the launch and ADCS is only leaving the atmosphere.” He implicitly considers the boundaries of the earth and satellite systems and their input and output. However, even though he referred to the behavior of the communications system, and implicitly considers system characteristics he did so in a perfunctory way. In the second repertory grid (Figure 5), all of John’s constructs were categorized as structures. He said, for instance, that the “EPS is a system in the satellite while structure design and launch are stages in getting the satellite into space.” He did not refer, however, to the importance of structure design in withstanding launch (which might have been considered a function). He could also have considered the EPS as a component which plays a major role in the process of structure design but did not do so. In another answer, he said that “the ADCS and the payload are both systems in the satellite, while tests and integration are a stage in the satellite’s development.” In doing so, he disregarded possible non-structural connections between the elements, like the function of the ADCS in allowing the payload (RGB camera) to perform its function. Moreover, although he referred to the ADCS and payload as systems, he did not exhibit meaningful consideration of system characteristics. All in all, John did not show any progress in his perceptions of the complexity of the system, and maybe even regressed in that regard. He went from mentioning a few behaviors at the beginning of the project to only referring to structures at the end.
When comparing the grid maps, we can see that in the first, the most related elements in his eyes were thermal control to communications, structure design and space environment, and remote sensing and orbit control (Figure 4). However, in his second repertory grid, he considered flight software, payload, and solar panels to be identical in terms of their ratings (Figure 5). His ratings show that he rated all three in the highest degree of relation to all his constructs. However, we would expect that at least in relation to outside/inside the satellite, some discrimination between the three would be evident. Thus, here he exhibits a lack of consideration for the system boundaries.
This lack of nuance seems to suggest a lower degree of complexity in John’s understanding of the satellite as a system. The appearance of elements perceived as identical in the second grid also suggests a lack of progress, if not regression, in his systems thinking. Not only did he not explicitly consider system characteristics, it seems that his previous implicit consideration for system boundaries and input and output was lacking in his second repertory grid. This is corroborated by the results from analyzing his constructs through the SBF model.
The high relatedness of communications to structure design is a bit puzzling as well, since there is no reason to relate those two elements closer than any other subsystem. This may indicate the centrality of communications in his eyes, since he dealt with that subsystem almost exclusively. Since structure design involves all the subsystems and their components this can also be attributed to his lack of cross-level reasoning, where he did not understand the relationships between different levels of organization.
As mentioned before, John was quite involved with the project and was a gifted student who achieved advanced learning goals. We therefore feel safe in determining that the reasons for his lack of progress with regard to systems thinking are a result of neither cognitive difficulties nor lack of engagement. His narrow focus on the communications aspect was evident not only in his choices (i.e., focusing only on communications and avoiding any other aspect like programming, and his choice of research project), but also in our observations of him during the learning process. In an activity where the students were producing several models of the software architecture, software demands, and the relationships between subsystems at various levels, he was expressive only when the communications system was very explicitly brought up. He was quiet throughout discussion of the ADCS system, in which students from various teams participated, but as soon as the communications came up, he became very vocal:
Teacher: What goes into the TxRx?
John: Commands are going in.
Teacher: Commands?
John: Like, input… all sorts of input go in, noise.
Teacher: What goes out?
John: A receiving signal, roger, acknowledged.
This exchange went on exclusively between John and the teacher, where John displayed a comprehensive familiarity of the communications system. However, when the communications system’s relations to other systems came up, John became less sure of himself, and other students became part of the discussion:
Teacher: What else goes into this system? Not necessarily from the outside, but from the inside as well?
John: Electricity.
Teacher: No, that’s not a system capacity.
Andy: Its capacity is to relay commands.
Here we see an example where Andy, who is the head of the ADCS team, gets involved in the discussion regarding the communications system. This behavior was displayed by Andy throughout our observations of him, but was not displayed by John. John’s reluctance to involve himself with any other aspect of the project other than communications was very noticeable throughout our observations. Since he did not take an active part in considering how the communications subsystem relates to other subsystems, thereby also not thinking about the satellite at higher levels of organization, it may not be surprising that his systems thinking did not show progress. Andy, who we describe next, showed quite the opposite tendencies.
Andy’s case
Andy was a very active student in the project. He started as the head of the ADCS team. During the program, the students assumed the roles of a quasi-independent organization and Andy was chosen to serve as CEO. He involved himself with all aspects of the project, communicated with all teams and was active in representing the project and school to visitors. He chose not to complete his research project, which was supposed to be related to the testing and integration of the satellite.
Andy had representations of all the categories in both repertory grids. For instance, when he explained the connection he saw between the EPS and the solar panels in the first repertory grid (Figure 6), he said: “both terms are related to the EPS. One is the EPS itself and the solar panels are part of the EPS.” He noted the hierarchy between the two elements, and identified the different levels of organization but not their relationship in terms of their functions or behaviors. In another triad, he said that: “T&C is an ability the communications system can perform.”
He thus referred to the behavior of the communications system, if only in a perfunctory way. He was also able to consider functions: “The solar panels generate electricity with which you can send T&C to the different systems.” He considered the function of the solar panels and connected the generation of electricity to handling T&C.
In his second repertory grid (Figure 7) Andy described more functions, but perhaps more importantly, his reasoning seemed more complex. For instance, he said: “The EPS operates different systems in the satellite. It activates wheels and other things. As a byproduct, it causes the satellite to heat up and the thermal control moderates that phenomenon.” Andy’s description here goes beyond merely noting two elements that affect one another. He considered unwanted consequences of certain elements through a series of connections. He was also aware that solutions are not complete, since he used the word “moderates,” and not “solves,” for example. He also identified causal relationships between different levels of organization of subsystems, subsystems’ components and their relation to physical processes such as the buildup of heat. Andy’s recognition of cause and effect as a series of couplings (EPS–reaction wheels–thermal control), and his concern with the regulation of excess heat, which suggest he is taking the dynamics of the system into consideration are indicative of progress in his systems thinking. We can also see various related elements in his grid, but no identical elements, as opposed to John. This demonstrated a high resolution in his thinking. For instance, remote sensing and payload could have been considered identical by participants, since their payload was a camera, yet Andy makes a distinction between the two.
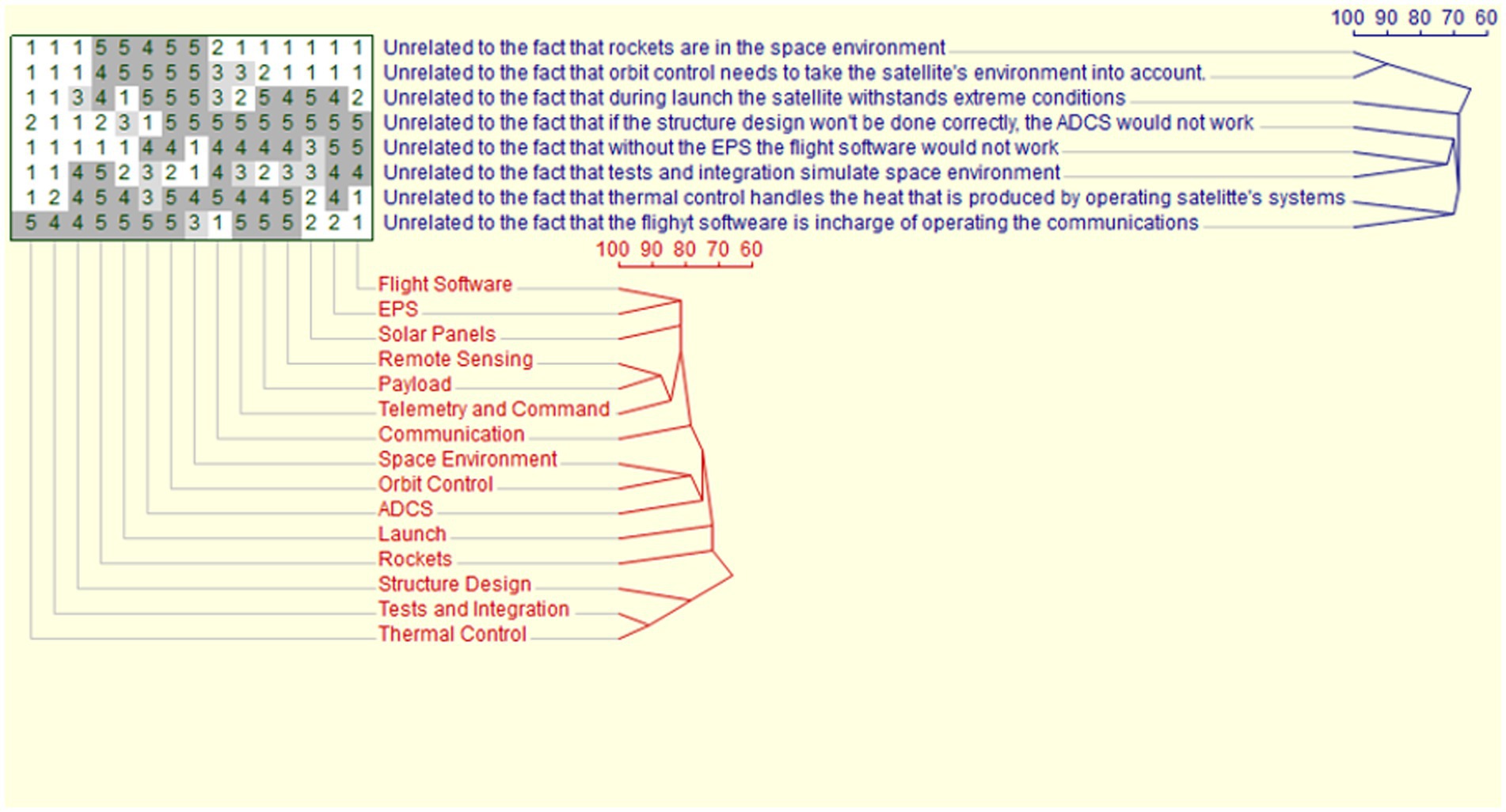
Figure 7. Andy’s post repertory grid. The left side constructs are truncated for the sake of readability.
In an activity where the students had to represent their systems in different levels of organization, Andy tried to figure out how the remote sensing system was represented (even though he was in the ADCS team). He spoke to another student about the remote sensing system:
Student: What’s this whole thing on the board?
Andy: That’s the system. Actually, that’s level 2, which begins at the…first level. Actually, that’s the whole satellite. So, what’s level 0?…
Andy: So, what are we doing? Imaging?
Student: Yes, that’s in the camera.
Andy: And where does it go? Here?
Student: Yes, and then it goes out, image data.
Andy: What goes out?
Student: The image data, the index, I do not know.
Andy: What do you mean you do not know? You took an image, what data do you have?
… I’m asking because I do not know. I want to know… So, I’m guessing what the image data is, maybe it’s its size, date, time…
As in the previous instance, where Andy involved himself in the communications system discussion, here he involves himself in the remote sensing system. He is asking questions, thinking across levels when considering different levels (“that’s the whole satellite, what’s level 0?”), trying to identify connections and making educated guesses. His tendency to initiate discussions with other teams, thinking about other systems and not only his own, may have contributed to the development of his systems thinking.
Sarah’s case
Sarah was a gifted student who joined the program (on the communications team) 2 months after it began. She wrote a research proposal that was accepted in computer science which would have been equivalent to a full matriculation exam. Her research proposal dealt with trying to develop an algorithm that could use the input from the camera to determine the satellite’s attitude and position. She made progress on her project but chose not to finish it. However, she did a lot of research into the ADCS and remote sensing parts of the project, as well as programming in MATLAB. Sarah was involved with various aspects, but put less time into the program than other participants. Still, she was able to display a marked progression in her systems thinking. Our recording of a communications assignment in which she participated showed her to be very active, demonstrating understanding and engagement. The communications team were modeling their system by creating a 2D representation:
Sarah: Let us move that up and connect this. Now let us move to the manager. Let us see, it connects to these two, does not it?
Student: Yes.
John: The manager goes to…
Sarah: Both through here and here.
Despite joining the project late, Sarah was quite involved with her team, as we can see from her making suggestions and observations regarding their model. On other occasions, we observed her helping other teams. She also wrote a reasearch proposal for a five-point CS credit, where she was planning on designing a star sensor as part of an ADCS system. She started working on her personal project for quite some time, but was unable to finish it. Thus, she did not limit herself to the sub-system team she initially joined, but was broadly involved with other aspects of the project.
When analyzing her constructs through the SBF framework, we can see that she showed marked advancement in her systems thinking, from focusing on structures to focusing on behaviors and functions. For instance, in her first repertory grid, when explaining why space environment is less related to ADCS and communications, she states: “space environment refers to the position [of the satellite]” (Figure 8). In her post repertory grid (Figure 9), on the other hand, she addressed the control flows, feedback loops and dynamics of the satellite. For instance: “There are times when there is no communication with the satellite and the flight software compensates for that.” Thus, she is considering system characteristics in her reasoning.
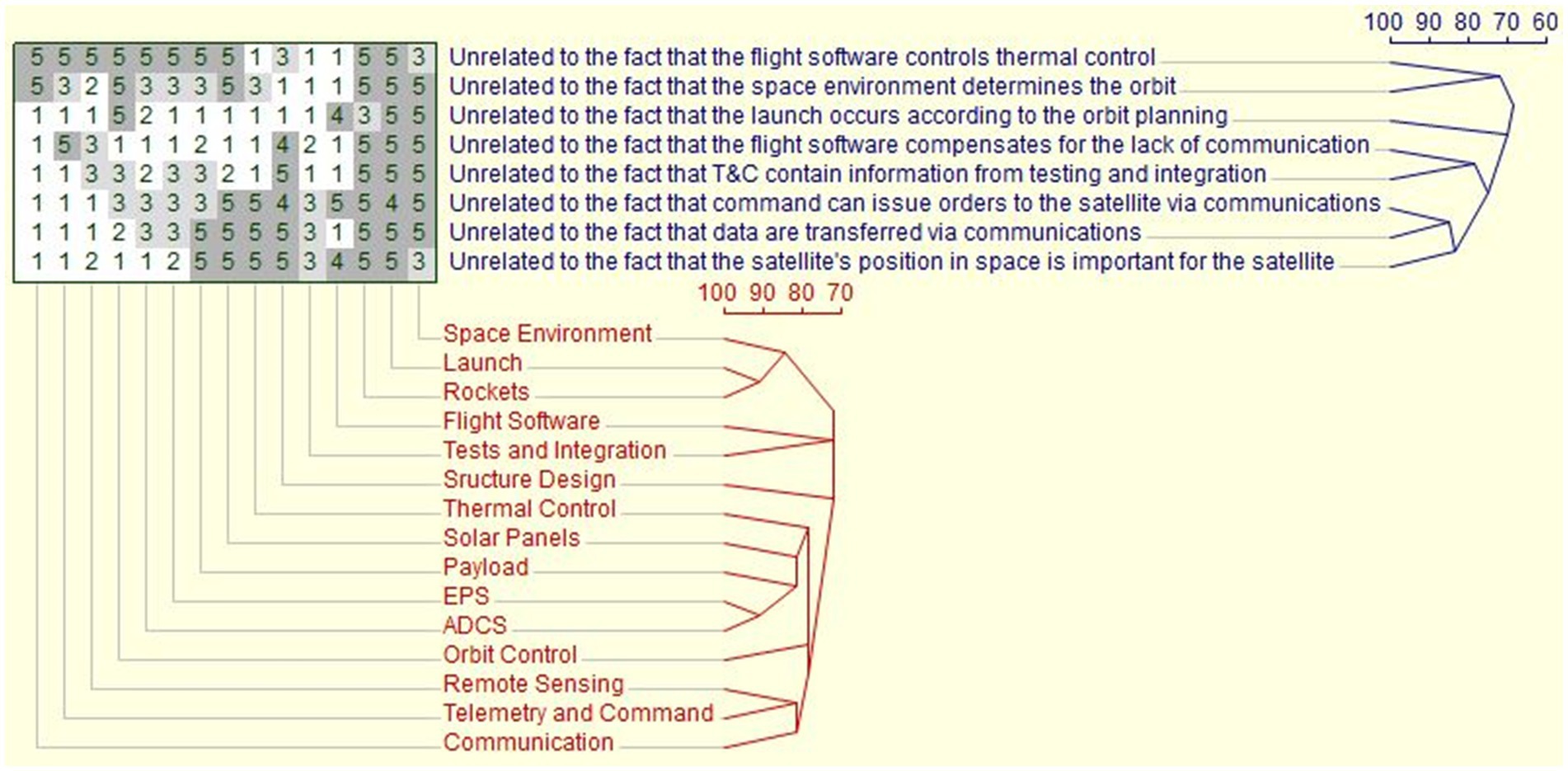
Figure 9. Sarah’s post repertory grid. The left side constructs are truncated for the sake of readability.
Her pre vs. post repertory grids show a shift from considering simple relations, such as “related to the satellite’s orbit,” to more complex interactions in the form of her construct: “related to the fact that space environment determines the orbit.” She also referred to various control processes in her post grid, which were lacking in the pre grid. This shows development of her systems thinking, even though she spent less time on the project. As a byproduct of her lower consistency in attending the group sessions, she was involved with various teams and aspects of the project, and that may have contributed to her systems thinking.
When describing her research proposal in her reflective interview, she said:
At the beginning I wanted something else, to examine something else related to images of stars. That would have required much bigger images, not like the ones from our satellite. I was trying to come up with something else and then I read a few interesting papers about the use of star sensors. I got very excited and if we can determine not only the attitude of the satellite but also its position. I saw this method that also uses Earth sensor. I thought I might be able to combine them and use a star sensor instead of the Earth sensor. We’ll see if it works.
This too reflects how she further broadened her interests beyond what her team, communications, focused on. Even though she was not able to complete her project, her research in the context of writing a proposal exposed her to various aspects of ADCS features and remote sensing.
Adam’s case
Adam was part of the EPS team. At the project’s outset, Adam displayed a lack of confidence in his ability to contribute. He had some misgivings regarding his ability to participate, and especially to carry out a research project.
Q: If we are talking about questions, do you expect any difficulties [in the project]?
Adam: Sure, it is not easy, this satellite thing.
Q: Why is not it easy?
Adam: Cause it is a lot of stuff.
Q: What do you mean a lot of stuff? Like what?
Adam: All the studying, we are just joining this, it is not as if we have knowledge to begin with.
Q: How is that any different from any other subject?
Adam: You need to do research here. It is not as if you have material you need to study. You need to research by yourself.
Q: What do you mean by research?
Adam: This whole satellite programming, the EPS, we need to study it, we do not know what it is. It is not like a teacher already knows it and just explains the code to us. We’re studying it together from the beginning.
Q: Do you think that will be hard for you?
Adam: Yes.
Adam took part in the various activities and group tasks we observed. He was never the most prominent member in the team, and other members demonstrated more involvement and leadership in the teamwork. However, the subject he chose to study in his personal research project was quite different. He studied dynamics of algae in a freshwater lake (the sea of galilee) using remote sensing. His research project was equivalent to a five-point matriculation exam in biology and received a very high mark (97%). Thus, he studied remote sensing in addition to his part in the EPS team. Moreover, he had the opportunity to deeply explore another complex system in the context of ecology. In the reflective interview at the study’s later stages, his attitude toward his ability to carry out a research project was completely changed, and he was very confident in that regard.
Adam’s pre repertory grid (Figure 10) reveals several misconceptions. For instance, he regards solar panels as very closely related to thermal control, perhaps because of his perceptions of their relations to the sun. When considering the triad of “space environment,” “solar panels” and “communications,” he considered the first couple to be more related, saying “there is no connection between the sun and communications.” This argument corroborates the importance he assigns to the sun’s influence. In his post repertory grid (Figure 11) this association does not exist.
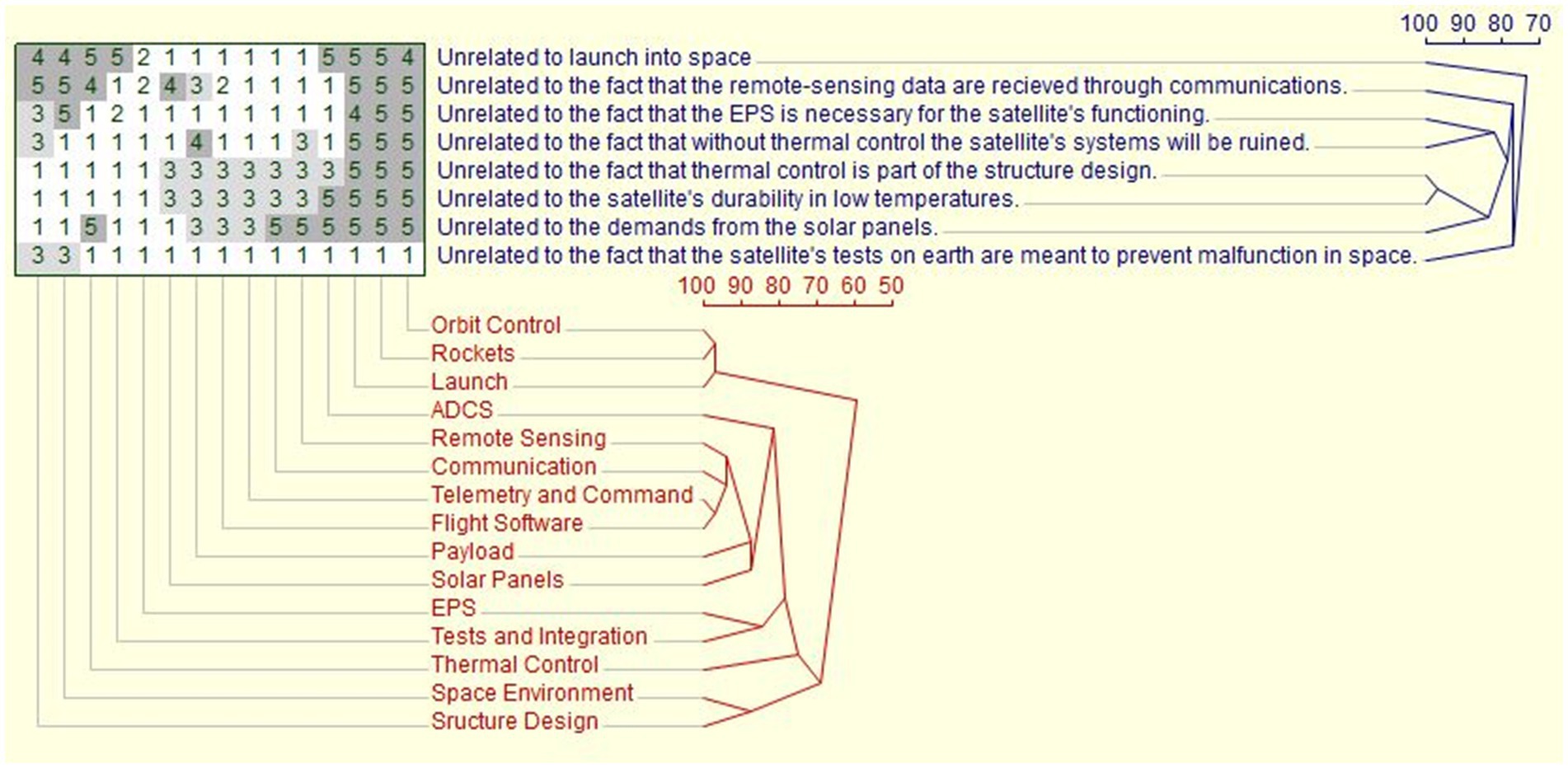
Figure 11. Adam’s post repertory grid. The left side constructs are truncated for the sake of readability.
Another interesting point is that the payload is quite far removed from other satellite systems in his first repertory grid. In the post repertory grid, on the other hand, he recognizes the payload as being more closely related to the other systems. The second repertory grid shows that Adam perceives the various subsystems as closely related, while still making high resolution distinctions between them. It also shows how the complexity of his constructs has increased. He considers the function of the thermal control and unwanted consequences of its hypothetical lack of function (“Related to the fact that without thermal control the satellite will be ruined”). He generally pays more attention to the functions of the different subsystems, instead of mainly referring to them as components (structures) only.
Discussion
Understanding complex systems is known to be difficult for students at various stages of their education (Hmelo-Silver and Azevedo, 2006; Schneeweiß and Gropengießer, 2019) and our findings support this. At the early stages of the program, the participants’ personal constructs demonstrated a low level of sophistication in their systems thinking. Although they seemed to understand the terms they were given in the repertory grid interview, their constructs revealed a relatively simple understanding of the satellite as a system. In contrast, by the program’s end, most participants showed varying degrees of progress in their systems thinking, and for some participants, a much deeper understanding of the satellite as a system. All the participants were very capable in terms of cognitive abilities. Thus, despite the fact that systems thinking is a higher-order thinking skill, cognitive capacity cannot explain the observed disparities in systems-thinking development. The varying levels of systems-thinking growth could not be explained by the amount of time spent working on the project either. These two themes are best shown by John, who was both cognitively adept and devoted a great deal of time to his work on the communications team, but displayed very little systems-thinking growth.
What seemed to explain the differences was the breadth of the different participants’ involvement, rather than other possibilities, like level of engagement. By breadth of involvement, we mean the degree to which a certain participant was involved with the various aspects of the project. In other words, the contributing factor was not whether a participant was deeply involved with the program, but rather how many different aspects he or she was involved with.
Involvement with several subsystems allowed students to better identify and understand interrelations between the subsystems, feedback loops (for instance, how the ADCS relies on the EPS to maintain the satellite’s attitude and in turn affects the power generation of the solar panels), and dynamic interdependencies. Furthermore, they were able to better identify the intended purpose of their subsystem in relation to other subsystems, which may have contributed to their identification of behaviors and functions in the system rather than only structures. All of these abilities characterize proficient system thinkers (Table 1).
The program designers’ reason for focusing the students on narrow roles was to enable them to successfully accomplish the goals of the program without formal engineering education (Millan et al., 2019). However, our study suggests that, with respect to the development of systems thinking, this may be counterproductive. Some might argue that in order to foster the development of systems thinking, education programs need to expose students to a wide variety of ideas and practices in different disciplines (Brookes, 2017). Indeed, systems thinking has been suggested to be closely linked to multidisciplinarity, problem-based learning, design and the management of risk and uncertainty (Milke, 2017).
People with a great depth of knowledge in a single area are referred to as “I-shaped,” which can describe most engineering graduates. People with some knowledge in many areas are “dash shaped.” The combination of the two is called “T shaped,” meaning someone with deep knowledge of one field with broad familiarity with other fields. There are quite a few studies that discuss the benefits of T-shaped education in fostering systems thinking approaches, but most of them advocate for initial specialization followed by acquiring broad skills, also known as soft skills (van den Beemt et al., 2020). This is the common route of first gaining deep expertise in a single area during one’s studies while gaining a broad knowledge in many areas during one’s career as an engineer (Boehm and Mobasser, 2015; Brookes, 2017).
However, there is a recent approach that suggests that not only should engineering graduates be T-shaped, but also that engineering programs can start by giving the students a broad education before going deep into one area (Boehm and Mobasser, 2015). Our results suggest that even at the high school stage, students may benefit from a broad engineering education, and that the approach of forming teams of “experts” in programs like the one studied here may need to be reconsidered. Still, there remains a question of what may be the underlying mechanisms that can explain the pattern we observed. In other words, why did students who were more broadly involved with various aspects of the project developed a more sophisticated systems-thinking in the context of the satellite? We suggest that broader involvement may have provided more opportunity to engage in practices that foster systems-thinking development.
Under the guidance of the systems engineer, the participants in this study were very involved with models of the satellite, which consisted of 2-D representations of the various levels of organization—from the whole satellite, through its subsystems, down to the individual components of that subsystem, as well as the physical characteristics of the space environment (radiation, temperature) and micro scale processes such as power generation through the solar panels. The practice of modeling was a major part of the participants’ work in preparation for the design reviews, as can be seen in our results.
Central artifacts of model-based engineering education are conceptual models of complex systems, which build a common language between the scientific education and engineering education communities by modeling natural or artificial systems (Lavi and Dori, 2019). Modeling is part of scientific reasoning and enables comprehension of complicated systems by employing simpler hypothetical systems that, in certain respects, resemble the complex system they represent. Knowledge about the model is converted to knowledge about the phenomenon (Krell et al., 2019).
Modeling can help students better understand the dynamics of a system and integrate knowledge about it (Wilson et al., 2020). Modeling while studying complex systems allows students to evaluate the system’s properties and to express its complexity by depicting the relationships between its components while iteratively revising them. The model revision process allows students to think about the system in new ways (Bielik et al., 2022). The use of models in education consists of two central parts: models that communicate scientific or engineering content to students, and modeling done by students to gain insight (Upmeier zu Belzen et al., 2019). The latter mode of modeling, which was evident in our observations, is instrumental in making students’ understanding visible, helping students organize their ideas, and facilitating constructive and collaborative discussion (Hmelo-silver et al., 2017; Bielik et al., 2021). It allows students to engage in inquiry practices by gathering data, generating hypotheses, and testing them (Hmelo-Silver et al., 2015).
However, the modeling the participants were engaging in consisted of constructing 2D-representations of the satellites systems and components. The fact that they were ultimately unable to test their models may have well been a factor in hindering the development of more sophisticated systems thinking. Studies show that modeling has multiple cognitive benefits in terms of scientific reasoning and understanding (Louca and Zacharia, 2012). However, an important part of modeling is testing the model, revising it and validating it (Bielik et al., 2021; Nielsen and Nielsen, 2021), activities which were lacking in this case. This illustrates the importance of professional development for educators of metamodeling knowledge as Bielik et al. (2021) point out.
Gilissen et al. (2019) have described several system characteristics that are relevant to complex systems in nature. These characteristics include components, interactions, boundaries, input and output, hierarchy, emergence, feedback loops, and dynamics. Although many of the complex systems characteristics may be addressed by generating 2D representations, some may not be. For instance, the dynamics of a complex system in terms of changes in time and space may not be addressed by static representations (Hmelo-Silver et al., 2015). Feedback loops, which also contain a time dimension, can be only partially represented in static models. Modeling in this program was also done mostly with a reductionistic approach where each system was modeled by dividing it to subsystems, while less attention was given to how subsystems interactions may give rise to emergent properties. Thus, it may be worthwhile to employ software that will enable students to create dynamic models that will allow them to explore the system’s changes over time, emergent properties, and other system characteristics in a more meaningful way.
Depending on their goals, models represent a subset of the parts of the modeled item. They must reflect certain facets of the examined phenomena and be utilized to produce predictions. Models are evaluated by comparing their predictions with data from the real world and changing them as necessary (Krell et al., 2019). In this program, the students did study the parts that were needed to be modeled and were used to predict the functioning of the satellite. The testing period could have served as a means of comparing the model to the real world and revision of the model, but unfortunately, most of the testing was not carried out by the participants, hence we cannot remark on the meaningfulness of that phase in terms of systems thinking development.
While the participants were involved in modeling, they also engaged in thinking across levels of organization, which fosters systems thinking (Ben-Zvi Assaraf and Knippels, 2022). Weintrop et al. (2016) identify cross-level reasoning as core to systems thinking (though they call this “thinking in levels”). Systems can be understood by analyzing different levels of organization from the micro scale to the macro scale. Different insights can be gained from examining different levels, which can lead to a better understanding of the emergent characteristics of the system as a whole (Weintrop et al., 2016). Challenging students to reason between various levels of organization has been shown to improve systems thinking (Verhoeff et al., 2018; Gilissen et al., 2021). Thus, it may be the case that participants who modeled more subsystems, thereby also utilizing thinking across levels more than participants with a narrow focus, were able to make more progress in their systems thinking. This is in accordance with Bielik et al. (2021), who suggest that repeated use of models in different contexts fosters systems thinking.
Another relevant suggestion is to constantly revisit the level of the whole system. While working on specifics, students may get bogged down in detail and lose the bigger picture, as some of the students in this case might have done by focusing only on the subsystem they were initially assigned to. Deliberately encouraging students to reconsider the satellite as a whole may be an important guideline for complex engineering projects such as the one presented here. Bielik et al. (2022) stress the need to revisit the overarching phenomenon, since students can easily lose the big picture of what they are modeling, and our findings seem to support that recommendation.
A final aspect of the program was the use of explicit systems language by the systems engineer, as we saw in our observations. Under his guidance, the students were asked to consider the system components and the interactions between them, input and output, system boundaries at various levels of organization, unintended consequences, and the dynamics of the systems. Systems language is the explicit use of terms that refer to system characteristics. Proponents of this strategy contend that when teaching about complex systems and encouraging system thinking, teachers should make explicit use of systems language and encourage their students to use that language explicitly (Eberbach et al., 2021). Deconstructing a phenomenon to its characteristics and discussing them explicitly has been shown to help clarify it for both students and teachers (Klein and Zion, 2015). Jordan et al. (2013) showed that exposure to systems language helps students in their explanations by linking multiple ideas and improving their explanations’ sophistication by enriching references to invisible elements. The use of systems language seemed to have been a natural tendency as a result of the instructor’s experience as a systems engineer. An engineering teacher who lacks such experience may need to consciously incorporate the use of systems language, since such use supports the development of systems thinking (Fick et al., 2022).
Nguyen and Santagata (2021) have shown that the teacher’s prompts greatly affect how middle school students respond when asked about connections in systems. The language teacher’s use is adopted by students, not only in their discussions with the teacher but also in their group discussions without the immediate presence of the teacher, thus assisting their understanding of systems (Hmelo-silver et al., 2015). However, since instructors with no experience in systems engineering may not utilize systems language as naturally as the engineer in our program did, program designers need to consider how to deliberately incorporate this language into instruction.
Reflecting on the body of work we have included in Table 1, there are several themes that are involved in systems thinking. We have observed the implicit use of some of the themes by the systems engineer such as the analysis of systems’ elements and relationships, consideration of feedback loops and cross level reasoning to a certain degree. Some attention was also paid to the dynamics of the system, but since there was no dynamic modeling, or testing done by the participants, it was limited. Moreover, other themes such as emergence was not evident in our observations. A dynamic model of the satellite, which could be built from physical components that are not intended for use in space, could be very affordable considering the overall budget of such a program. Such a model which could be tested by students, experimented upon and manipulated, could serve as a very meaningful scaffolding for fostering systems thinking. Emergence (Table 1) could be made evident in terms of understanding how the behavior of a system arises from the interaction of its agents over time (Sweeney and Sterman, 2000). Generalizations (Table 1) could be achieved by comparing the model satellite to the satellite itself which could facilitate observing the system from a generic viewpoint—looking for similarity between the system and other systems (Gero and Danino, 2016). Dynamic relationships (Table 1) could be made more explicit by using the model to identify different types of procedural sequences—linear, divergent, convergent, and looping (Lavi and Dori, 2019).
This study has some limitations. The number of participants who were able to finish all research tools is quite small. The program studied here is a very demanding program for students. There is a natural attrition as a result from the participation in the program itself. The research tools used add to that attrition and result in a low completion rate. Since this is a unique program, this study may present a challenge to replicate. We certainly acknowledge that the pattern observed here requires more evidence, but we think it is interesting and important enough to be relevant to most engineering educational programs and any other program that involves the approach of assigning students to narrow roles.
In sum, we suggest that the instinctive use of various strategies by the systems engineer had the potential to facilitate the development of the students’ systems thinking. However, for that to take place, students need to be involved with various aspects of the system, in order to engage with the system from different aspects and through different lenses. This broad view may be achieved through students assuming broader roles in engineering projects, or by engaging in different learning activities that research projects can provide. Moreover, since not all learning programs can involve expert system engineers, it may be advisable to design similar programs while explicitly considering the role of modeling, cross level reasoning and systems language in fostering systems thinking.
Data availability statement
The raw data supporting the conclusions of this article will be made available by the authors, without undue reservation.
Ethics statement
Ethical review and approval was not required for the study on human participants in accordance with the local legislation and institutional requirements. Written informed consent to participate in this study was provided by the participants’ legal guardian/next of kin.
Author contributions
RT collected data, performed most of the interviews, carried out observations, analyzed the data, and was a major contributor in writing the manuscript. OB-Z conducted interviews, reviewed the data, and its analysis and substantially revised the manuscript. SM interviewed experts, reviewed data and analysis, and revised the manuscript. All authors contributed to the article and approved the submitted version.
Acknowledgments
We would like to thank Yael Lebel and Yael Beja with their help with transcriptions. We would also like to thank Thom Rofe for her help with editing the manuscript.
Conflict of interest
The authors declare that the research was conducted in the absence of any commercial or financial relationships that could be construed as a potential conflict of interest.
Publisher’s note
All claims expressed in this article are solely those of the authors and do not necessarily represent those of their affiliated organizations, or those of the publisher, the editors and the reviewers. Any product that may be evaluated in this article, or claim that may be made by its manufacturer, is not guaranteed or endorsed by the publisher.
References
Arnold, R. D., and Wade, J. P. (2015). A definition of systems thinking: a systems approach. Proc. Comput. Sci. 44, 669–678. doi: 10.1016/j.procs.2015.03.050
Ben Zvi Assaraf, O., Dodick, J., and Tripto, J. (2013). High school students’ understanding of the human body system. Res. Sci. Educ. 43, 33–56. doi: 10.1007/s11165-011-9245-2
Ben-Zvi Assaraf, O., and Knippels, M.C.P.J. (eds). (2022). Fostering Understanding of Complex Systems in Biology Education. Series: Contributions From Biology Education Research. Netherlands: Springer
Ben-Zvi Assaraf, O., and Orion, N. (2005). Development of system thinking skills in the context of earth system education. J. Res. Sci. Teach. 42, 518–560. doi: 10.1002/tea.20061
Ben-Zvi Assaraf, O., and Orion, N. (2010). Four case studies, six years later: developing system thinking skills in junior high school and sustaining them over time. J. Res. Sci. Teach. 47, 1253–1280. doi: 10.1002/tea.20383
Bezzi, A. (1999). What is this thing called geoscience? Epistemological dimensions elicited with the repertory grid and their implications for scientific literacy. Sci. Educ. 83, 675–700. doi: 10.1002/(SICI)1098-237X(199911)83:6<675::AID-SCE3>3.0.CO;2-Q
Bielik, T., Fonio, E., Feinerman, O., Duncan, R. G., and Levy, S. T. (2021). Working together: integrating computational modeling approaches to investigate complex phenomena. J. Sci. Educ. Technol. 30, 40–57. doi: 10.1007/s10956-020-09869-x
Bielik, T., Stephens, L., McIntyre, C., Damelin, D., and Krajcik, J. S. (2022). Supporting student system modelling practice through curriculum and technology design. J. Sci. Educ. Technol. 31, 217–231. doi: 10.1007/s10956-021-09943-y
Boehm, B., and Mobasser, S. K. (2015). System thinking: educating T-shaped software engineers. Int. Conf. Softw. Eng. 2, 333–342. doi: 10.1109/ICSE.2015.166
Braun, V., and Clarke, V. (2006). Using thematic analysis in psychology. Qual. Res. Psychol. 3, 77–101. doi: 10.1191/1478088706qp063oa
Brookes, W. (2017). “Transdisciplinary learning in technology degrees” in 2017 16th International Conference on Information Technology Based Higher Education and Training, ITHET 2017.
Brosch, N., De Castro, A. I. G., Murthy, J., Ribak, E. N., Behar, E., and Balabanov, V. (2017). Ultraviolet astronomy with small space telescopes. Contrib. Astronom. Observ. Skalnat. Pleso 47, 200–207.
Case, J. M., and Light, G. (2011). Emerging research methodologies in engineering education research. J. Eng. Educ. 100, 186–210. doi: 10.1002/j.2168-9830.2011.tb00008.x
DeWalt, K. M., and DeWalt, B. R. (2011). Participant Observation a Guide for Fieldworkers. 2nd Edn Rowman & Littlefield:AltaMira Press.
Eberbach, C., Hmelo-Silver, C. E., Jordan, R., Taylor, J., and Hunter, R. (2021). Multidimensional trajectories for understanding ecosystems. Sci. Educ. 105, 521–540. doi: 10.1002/sce.21613
Edwards, H. M., McDonald, S., and Michelle Young, S. (2009). The repertory grid technique: its place in empirical software engineering research. Inf. Softw. Technol. 51, 785–798. doi: 10.1016/j.infsof.2008.08.008
Evagorou, M., Korfiatis, K., Nicolaou, C., and Constantinou, C. (2009). An investigation of the potential of interactive simulations for developing system thinking skills in elementary school: a case study with fifth-graders and sixth-graders. Int. J. Sci. Educ. 31, 655–674. doi: 10.1080/09500690701749313
Fick, S. J., Chiu, J. L., and McElhaney, K. W. (2022). An examination of elementary classroom dialogue: implicit and explicit use of the NGSS crosscutting concepts in an integrated STEM unit. J. Sci. Teach. Educ. 1–23. doi: 10.1080/1046560x.2021.1961974
Frank, M. (2010). Assessing the interest for systems engineering positions and other engineering positions' required capacity for engineering systems thinking (CEST). Syst. Eng. 13, 161–174. doi: 10.1002/sys.20140
Fuentes, M. A. (2014). Complexity and the emergence of physical properties. Entropy 16, 4489–4496. doi: 10.3390/e16084489
Gero, A., and Danino, O. (2016). High-school course on engineering design: enhancement of students’ motivation and development of systems thinking skills. Int. J. Eng. Educ. 32, 100–110. doi: 10.24908/pceea.v0i0.5846
Gilissen, M. G., Knippels, M. C. P., and van Joolingen, W. R. (2020). Bringing systems thinking into the classroom. Int. J. Sci. Educ. 42, 1253–1280. doi: 10.1080/09500693.2020.1755741
Gilissen, M. G., Knippels, M. C. P., and van Joolingen, W. R. (2021). Fostering students’ understanding of complex biological systems. CBE—Life Sci. Educ. 20:ar37. doi: 10.1187/cbe.20-05-0088
Gilissen, M. G., Knippels, M. C. P., Verhoeff, R. P., and van Joolingen, W. R. (2019). Teachers’ and educators’ perspectives on systems thinking and its implementation in Dutch biology education. J. Biol. Educ. 54, 485–496. doi: 10.1080/00219266.2019.1609564
Goel, A. K., Rugaber, S., and Vattam, S. (2009). Structure, behavior, and function of complex systems: the structure, behavior, and function modeling language. Arti. Intell. Eng. Des. Anal. Manuf. 23, 23–35. doi: 10.1017/S0890060409000080
Greene, M. T., and Papalambros, P. Y. (2016). (Gud-cognitive)a cognitive framework for engineering systems thinking. Conference on Systems Engineering Research (CSER), 1–7. Available at: https://pdfs.semanticscholar.org/5f41/629356a56d2e78f77afe3daab4dc78c139cb.pdf
Hmelo-Silver, C. E., and Azevedo, R. (2006). Understanding complex systems: some core challenges. J. Learn. Sci. 15, 53–61. doi: 10.1207/s15327809jls1501_7
Hmelo-Silver, C. E., Jordan, R., Eberbach, C., and Sinha, S. (2017). Systems learning with a conceptual representation: a quasi-experimental study. Instr. Sci. 45, 53–72. doi: 10.1007/s11251-016-9392-y
Hmelo-Silver, C. E., Liu, L., Gray, S., and Jordan, R. (2015). Using representational tools to learn about complex systems: a tale of two classrooms. J. Res. Sci. Teach. 52, 6–35. doi: 10.1002/tea.21187
Hmelo-Silver, C. E., Marathe, S., and Liu, L. (2007). Fish swim, rocks sit, and lungs breathe: expert-novice understanding of complex systems. J. Learn. Sci. 16, 307–331. doi: 10.1080/10508400701413401
Hmelo-Silver, C. E., and Pfeffer, M. G. (2004). Comparing expert and novice understanding of a complex system from the perspective of structures, behaviors, and functions. Cogn. Sci. 28, 127–138. doi: 10.1016/S0364-0213(03)00065-X
Hunter, M. G., and Beck, J. E. (2000). Using repertory grids to conduct cross-cultural information systems research. Inf. Syst. Res. 11, 93–101. doi: 10.1287/isre.11.1.93.11786
Jankowicz, D. (2001). Why does subjectivity make us nervous?: making the tacit explicit. J. Intellect. Cap. 2, 61–73. doi: 10.1108/14691930110380509
Jordan, R. C., Hmelo-Silver, C., Liu, L., and Gray, S. A. (2013). Fostering reasoning about complex systems: using the aquarium to teach systems thinking. Appl. Environ. Educ. Commun. 12, 55–64. doi: 10.1080/1533015X.2013.797860
Keynan, A., Ben-Zvi Assaraf, O., and Goldman, D. (2014). The repertory grid as a tool for evaluating the development of students’ ecological system thinking abilities. Stud. Educ. Eval. 41, 90–105. doi: 10.1016/j.stueduc.2013.09.012
Klein, S., and Zion, M. (2015). The characteristics of homeostasis: a new perspective on teaching a fundamental principle in biology. Sch. Sci. Rev. 97, 85–93.
Krell, M., Walzer, C., Hergert, S., and Krüger, D. (2019). Development and application of a category system to describe pre-service science teachers’ activities in the process of scientific modelling. Res. Sci. Educ. 49, 1319–1345. doi: 10.1007/s11165-017-9657-8
Lan, W., Brown, J., Toorian, A., Coelbo, R., Brooks, L., Puig-Suari, J., et al. (2006). CubeSat development in education and into industry. AIAA SPACE Forum. Space 2006:7296. doi: 10.2514/6.2006-7296
Latta, G. F., and Swigger, K. (1992). Validation of the repertory grid for use in modeling knowledge. J. Am. Soc. Inf. Sci. 43, 115–129. doi: 10.1002/(SICI)1097-4571(199203)43:2<115::AID-ASI2>3.0.CO;2-I
Lavi, R., and Dori, Y. J. (2019). Systems thinking of pre- and in-service science and engineering teachers. Int. J. Sci. Educ. 41, 248–279. doi: 10.1080/09500693.2018.1548788
Louca, L. T., and Zacharia, Z. C. (2012). Modeling-based learning in science education: cognitive, metacognitive, social, material and epistemological contributions. Educ. Rev. 64, 471–492. doi: 10.1080/00131911.2011.628748
Mambrey, S., Timm, J., Landskron, J. J., and Schmiemann, P. (2020). The impact of system specifics on systems thinking. J. Res. Sci. Teach. 57, 1632–1651. doi: 10.1002/tea.21649
Martínez Rodríguez-Osorio, R., and Fueyo Ramírez, E. (2012). A hand s-on education project: antenna design for Inter-CubeSat communications. IEEE Antenn. Propag. Magaz. 54, 211–224. doi: 10.1109/MAP.2012.6348155
McCloughlin, T. J. (2017). Personal constructions of biological concepts–the repertory grid approach. Center Educ. Policy Stud. J. 7, 93–110. doi: 10.26529/cepsj.16
Mehren, R., Rempfler, A., Buchholz, J., Hartig, J., and Ulrich-Riedhammer, E. M. (2018). System competence modelling: theoretical foundation and empirical validation of a model involving natural, social and human-environment systems. J. Res. Sci. Teach. 55, 685–711. doi: 10.1002/tea.21436
Milke, M. W. (2017). The distinctiveness of civil engineering in engineering systems thinking and in new models in engineering education. Civ. Eng. Environ. Syst. 34, 78–87. doi: 10.1080/10286608.2017.1313246
Millan, R. M., von Steiger, R., Ariel, M., Bartalev, S., Borgeaud, M., Campagnola, S., et al. (2019). Small satellites for space science: a COSPAR scientific roadmap. Adv. Space Res. 64, 1466–1517. doi: 10.1016/j.asr.2019.07.035
Mills, A. J., Durepos, G., and Wiebe, E. (2010). Encyclopedia of Case Study Research. Thousand Oaks, CA: SAGE Publications
NGSS Lead States (2013). Next Generation Science Standards: For States, By States. Washington, DC: The National Academies Press.
Nguyen, H., and Santagata, R. (2021). Impact of computer modeling on learning and teaching systems thinking. J. Res. Sci. Teach. 58, 661–688. doi: 10.1002/tea.21674
Nguyen, H., Wu, L., Fischer, C., Washington, G., and Warschauer, M. (2020). Increasing success in college: examining the impact of a project-based introductory engineering course. J. Eng. Educ. 109, 384–401. doi: 10.1002/jee.20319
Nielsen, S. S., and Nielsen, J. A. (2021). A competence-oriented approach to models and modelling in lower secondary science education: practices and rationales among Danish teachers. Res. Sci. Educ. 51, 565–593. doi: 10.1007/s11165-019-09900-1
Rozenszajn, R., and Yarden, A. (2015). Exposing biology teachers’ tacit views about the knowledge that is required for teaching using the repertory grid technique. Stud. Educ. Eval. 47, 19–27. doi: 10.1016/j.stueduc.2015.06.001
Rozenszajn, R., Zer Kavod, G., and Machluf, Y. (2021). What do they really think? The repertory grid technique as an educational research tool for revealing tacit cognitive structures. Int. J. Sci. Educ. 43, 906–927. doi: 10.1080/09500693.2021.1891323
Samon, S., and Levy, S. T. (2020). Interactions between reasoning about complex systems and conceptual understanding in learning chemistry. J. Res. Sci. Teach. 57, 58–86. doi: 10.1002/tea.21585
Schneeweiß, N., and Gropengießer, H. (2019). Organising levels of organisation for biology education: a systematic review of literature. Educ. Sci. 9:207. doi: 10.3390/educsci9030207
Snapir, Z., Eberbach, C., Ben-Zvi-Assaraf, O., Hmelo-Silver, C., and Tripto, J. (2017). Characterising the development of the understanding of human body systems in high-school biology students–a longitudinal study. Int. J. Sci. Educ. 39, 2092–2127. doi: 10.1080/09500693.2017.1364445
Sweeney, L. B., and Sterman, J. D. (2000). Bathtub dynamics: initial results of a systems thinking inventory. System dynamics review. J. Syst. Dynam. Soc. 16, 249–286. doi: 10.1002/sdr.198
Upmeier zu Belzen, A., van Driel, J., and Krüger, D. (2019). “Introducing a framework for modeling competence” in Towards a Competence-Based View on Models and Modeling in Science Education. eds. A. U. zu Belzen, D. Krüger, and J. van Driel (Cham: Springer), 3–19.
Van den Beemt, A., MacLeod, M., Van der Veen, J., Van de Ven, A., van Baalen, S., Klaassen, R., et al. (2020). Interdisciplinary engineering education: a review of vision, teaching, and support. J. Eng. Educ. 109, 508–555. doi: 10.1002/jee.20347
Verhoeff, R. P., Knippels, M. C. P., Gilissen, M. G., and Boersma, K. T. (2018). The theoretical nature of systems thinking. Perspectives on systems thinking in biology education. Front. Educ. 3:40. doi: 10.3389/feduc.2018.00040
Verhoeff, R. P., Waarlo, A. J., and Boersma, K. T. (2008). Systems modelling and the development of coherent understanding of cell biology. Int. J. Sci. Educ. 30, 543–568. doi: 10.1080/09500690701237780
Weintrop, D., Beheshti, E., Horn, M., Orton, K., Jona, K., Trouille, L., et al. (2016). Defining computational thinking for mathematics and science classrooms. J. Sci. Educ. Technol. 25, 127–147. doi: 10.1007/s10956-015-9581-5
Wilson, K. J., Long, T. M., Momsen, J. L., and Bray Speth, E. (2020). Modeling in the classroom: making relationships and systems visible. CBE—Life Sci. Educ. 19:fe1. doi: 10.1187/cbe.19-11-0255
Wu, P. H., Hwang, G. J., Yang, M. L., and Chen, C. H. (2018). Impacts of integrating the repertory grid into an augmented reality-based learning design on students’ learning achievements, cognitive load and degree of satisfaction. Interact. Learn. Environ. 26, 221–234. doi: 10.1080/10494820.2017.1294608
Yoon, S. A., Anderson, E., Koehler-Yom, J., Evans, C., Park, M., Sheldon, J., et al. (2017). Teaching about complex systems is no simple matter: building effective professional development for computer-supported complex systems instruction. Instr. Sci. 45, 99–121. doi: 10.1007/s11251-016-9388-7
Yoon, S. A., Goh, S. E., and Park, M. (2018). Teaching and learning about complex systems in K–12 science education: a review of empirical studies 1995–2015. Rev. Educ. Res. 88, 285–325. doi: 10.3102/0034654317746090
Keywords: systems-thinking, project-based learning, case study, repertory grid, CubeSats
Citation: Tamir R, Ben-Zvi Assaraf O and Maman S (2023) System-thinking progress in engineering programs: A case for broadening the roles of students. Front. Educ. 8:1138503. doi: 10.3389/feduc.2023.1138503
Edited by:
André Bresges, University of Cologne, GermanyReviewed by:
Alfonso Garcia De La Vega, Autonomous University of Madrid, SpainCristal Schult, University of Cologne, Germany
Copyright © 2023 Tamir, Ben-Zvi Assaraf and Maman. This is an open-access article distributed under the terms of the Creative Commons Attribution License (CC BY). The use, distribution or reproduction in other forums is permitted, provided the original author(s) and the copyright owner(s) are credited and that the original publication in this journal is cited, in accordance with accepted academic practice. No use, distribution or reproduction is permitted which does not comply with these terms.
*Correspondence: Ram Tamir, cmFtdGFtQHBvc3QuYmd1LmFjLmls