- Laboratory of Integrative Vector Neuroendocrinology, Department of Biology, York University, Toronto, ON, Canada
GPA2/GPB5 and its receptor constitute a glycoprotein hormone-signaling system native to the genomes of most vertebrate and invertebrate organisms. Unlike the well-studied gonadotropins and thyrotropin, the exact function of GPA2/GPB5 remains elusive, and whether it elicits its functions as heterodimers, homodimers or as independent monomers remains unclear. Here, the glycoprotein hormone signaling system was investigated in adult mosquitoes, where GPA2 and GPB5 subunit expression was mapped and modes of its signaling were characterized. In adult Aedes aegypti mosquitoes, GPA2 and GPB5 transcripts co-localized to bilateral pairs of neuroendocrine cells, positioned within the first five abdominal ganglia of the central nervous system. Unlike GPA2/GPB5 homologs in human and fly, GPA2/GPB5 subunits in A. aegypti lacked evidence of heterodimerization. Rather, cross-linking analysis to determine subunit interactions revealed A. aegypti GPA2 and GPB5 subunits may form homodimers, although treatments with independent subunits did not demonstrate receptor activity. Since mosquito GPA2/GPB5 heterodimers were not evident by heterologous expression, a tethered fusion construct was generated for expression of the subunits as a single polypeptide chain to mimic heterodimer formation. Our findings revealed A. aegypti LGR1 elicited constitutive activity with elevated levels of cAMP. However, upon treatment with recombinant tethered GPA2/GPB5, an inhibitory G protein (Gi/o) signaling cascade is initiated and forskolin-induced cAMP production is inhibited. These results further support the notion that heterodimerization is a requirement for glycoprotein hormone receptor activation and provide novel insight to how signaling is achieved for GPA2/GPB5, an evolutionary ancient neurohormone.
Introduction
Members of the cystine knot growth factor (CKGF) superfamily, which are characterized with a CKGF domain as their primary structural feature, include (i) the glycoprotein hormones, (ii) the invertebrate bursicon hormone, (iii) the transforming growth factor beta (TGFβ) family, (iv) the bone morphogenetic protein (BMP) antagonist family, (v) the platelet-derived growth factor (PDGF) family and (vi) the nerve growth factor (NGF) family. Of the members of the CKGF superfamily, the glycoprotein hormones are of fundamental importance in the regulation of both vertebrate and invertebrate physiology.
In vertebrates, members of the glycoprotein hormone family include follicle-stimulating hormone (FSH), luteinizing hormone (LH), thyroid-stimulating hormone (TSH) as well as chorionic gonadotropin (CG), which are implicated in governing several aspects of physiology including reproduction, energy metabolism along with growth and development. Structurally, these hormones are formed by the heterodimerization of two cystine-knot glycoprotein subunits, an α subunit that is structurally identical for each hormone (GPA1), and a hormone-specific β subunit (GPB1-4) (1, 2).
Two additional glycoprotein hormone subunits were more recently identified in the human genome, glycoprotein α2 (GPA2) and glycoprotein β5 (GPB5), and were found to heterodimerize (GPA2/GPB5) and act on the same receptor as TSH. As a result, GPA2/GPB5 was coined the name thyrostimulin to differentiate it from TSH in vertebrates (3, 4). Unlike other glycoprotein hormones which are restricted to the vertebrate lineage, homologous genes encoding GPA2/GPB5 subunits exist in all bilaterian organisms, where its function appears to be pleiotropic (3–5). In vertebrates, the function of GPA2/GPB5 has been implicated, or at least suggested, to be involved in reproduction (6), thyroxine production (4, 7), skeletal development (8), immunoregulation (9, 10) and the proliferation of ovarian cancer cell lines (11). For invertebrate species, GPA2/GPB5 has been implicated or demonstrated to function in development (12–15), hydromineral balance (13–18) as well as in reproduction (15, 17, 19).
Heterodimerization by non-covalent interactions between the subunits forming FSH, LH, TSH, and CG is required for their respective biological functions (2, 20). However, whether heterodimerization is required for GPA2/GPB5 to activate its receptor and exert its physiological role in vertebrates and invertebrates is debated. For FSH, LH, TSH, and CG, the subunits are co-expressed in the same cells and each hormone is released into circulation as heterodimers (21, 22). On the other hand, GPA2 and GPB5 subunit expression profiles, both for vertebrate and invertebrate organisms, do not always occur in the same cells, and GPA2 is often expressed more widely and abundantly than GPB5 in some tissues (7, 12, 23–25). Additionally, unlike the beta subunits of the classic glycoprotein hormones, the structure of the GPB5 subunit lacks an extra pair of cysteine residues that form an additional disulfide linkage, referred to as the “seatbelt,” which strengthens and stabilizes its heterodimeric association with GPA2 (26).
Relative to other G protein-coupled receptors (GPCRs), members of the leucine-rich repeat-containing G protein-coupled receptor (LGR) family are often characterized with a large extracellular amino terminal domain responsible for the selective binding of their large hormone ligands (27). Following its initial genomic and molecular characterization (28), an invertebrate receptor for GPA2/GPB5, called LGR1, was functionally deorphanized in the fruit fly Drosophila melanogaster, where GPA2/GPB5 heterodimers were found to activate LGR1 increasing cyclic AMP (cAMP) levels (29). Interestingly, stimulatory G protein (Gs) coupling and signaling to elevate cAMP was also shown with GPA2/GPB5-TSH receptor activation in humans (4, 6).
In the mosquito Aedes aegypti, genes encoding GPA2, GPB5 and LGR1 were identified and shown to be expressed in all developmental life stages, with expression enriched in adults compared to juvenile stages (13). In adults, LGR1 was found localized to epithelia throughout the gut where GPA2/GPB5 could regulate feeding-related processes and hydromineral balance (17). Notably, LGR1 transcript expression was also observed in the reproductive organs of males and females (19). In adult male mosquitoes, knockdown of LGR1 expression led to abnormal spermatogenesis with spermatozoa displaying malformations such as shortened flagella and consequently, LGR1-knockdown males had 60% less spermatozoa as well as significantly reduced fecundity relative to control mosquitoes (19).
With an interest in better understanding GPA2/GPB5 signaling in A. aegypti mosquitoes, our work herein set out to characterize the tissue-specific and cellular distribution expression profile of GPA2/GPB5 in mosquitoes. As well, we sought to determine if GPA2/GPB5 could heterodimerize, whether heterodimers were required to activate LGR1, and determine downstream signaling events upon receptor activation using a heterologous system. Combining various molecular techniques, we demonstrate GPA2/GPB5 cellular co-expression in the central nervous system of adult mosquitoes, and that both subunits are indeed required to activate LGR1, which exhibits ligand-dependent G protein-coupling activity. Moreover, our results also provided evidence for GPA2 and GPB5 homodimers, with individually-expressed subunits being incapable of activating LGR1. Overall, these findings appreciably advance our understanding of GPA2/GPB5 signaling in mosquitoes and provide novel directions to uncover the functions of homologous systems in other organisms.
Materials and Methods
Animals
Adult A. aegypti (Liverpool) were derived from an established laboratory-reared colony raised under conditions described previously (17).
GPA2/GPB5 Transcript Analysis by RT-qPCR
Total RNA was isolated and purified from select A. aegypti tissues and organs, reverse transcribed into cDNA. GPA2 and GPB5 transcript abundance was quantified using a StepOnePlus™ Real-Time PCR System (Applied Biosystems) (see SI Materials and Methods for details) and transcript abundance was normalized to transcript levels of two reference genes; ribosomal protein 49 (GenBank accession: AY539746) and 60S ribosomal protein S18 (GenBank accession: XM_001660270), following the ΔΔCt method as previously described (13). Experiments were repeated using a total of three technical replicates per sample and three biological replicates for each tissue/organ.
Fluorescence in situ Hybridization
Using gene-specific primers (Table S1), A. aegypti GPA2 and GPB5 sequences were amplified from previously prepared constructs (13) that contained the GPA2 and GPB5 complete open reading frames (ORFs). Sense and antisense probes were then generated following a similar protocol as recently reported (19). Briefly, cDNA fragments were ligated to pGEM T Easy vector (Promega, Madison, WI, USA) and used to transform NEB 5-α competent Escherichia coli cells (New England Biolabs, Whitby, ON, Canada). After screening plasmid constructs for directionality using T7 promoter oligonucleotide and gene-specific primers (Table S1), template sense or anti-sense cDNA strands for GPA2 and GPB5 probe synthesis were created by PCR amplification and verified by Sanger sequencing for base accuracy (The Centre for Applied Genomics, Sick Kids Hospital, Toronto, ON, Canada). Digoxigenin (DIG)-labeled anti-sense and sense RNA probes corresponding to GPA2 and GPB5 subunits were synthesized using the HiScribe T7 High Yield RNA Synthesis kit (New England Biolabs, Whitby, ON, Canada). Fluorescence in situ hybridization (FISH) was then used to detect GPA2 and/or GPB5 transcript in the mosquito central nervous system using 4 ng μl−1 (GPB5) and/or 6 ng μl−1 (GPA2) RNA sense/antisense probes, following a previously established protocol (30). Preparations were analyzed with a Lumen Dynamics X-Cite™ 120Q Nikon fluorescence microscope (Nikon, Mississauga, ON, Canada), or a Yokogowa CSU-XI Zeiss Cell Observer Spinning Disk confocal microscope, and images were processed using Zeiss Zen and ImageJ software. All microscope settings were kept identical when acquiring images of control and experimental preparations.
Wholemount Immunohistochemistry
GPB5 immunoreactivity in the abdominal ganglia of adult mosquitoes was examined in newly emerged and four-day old A. aegypti that were lightly CO2 anesthetized, and dissected in PBS at RT. Tissues were fixed, permeabilized and incubated in a custom affinity-purified rabbit polyclonal GPB5 antibody (1 μg ml−1; Genscript, Piscataway, NJ) designed against an antigen sequence (CDSNEISDWRFP) positioned at residues 69–80 of the deduced A. aegypti GPB5 protein sequence (13) for 48 h at 4°C rocking. Control treatments involved pre-incubated A. aegypti GPB5 primary antibody solution containing 100:1 peptide antigen:antibody (mol:mol). After several washes, tissues were incubated overnight at 4°C in Alexa Fluor 488-conjugated goat anti-rabbit Ab (1:200) secondary antibody (Life Technologies, Carlsbad, CA) in PBS containing 10% normal sheep serum. The next day, samples were washed and then mounted onto coverslips using mounting media containing 5 μg μl−1 Diamidino-2-phenylindole dihydrochloride (DAPI), and analyzed using a Lumen Dynamics X-Cite™ 120Q Nikon fluorescence microscope (Nikon, Mississauga, ON, Canada), or optically sectioned using a Yokogowa CSU-XI Zeiss Cell Observer Spinning Disk confocal microscope. All images were processed using Zeiss Zen and ImageJ software. Further details concerning the wholemount immunohistochemical protocol were reported in an earlier study (17).
Plasmid Expression Constructs
Plasmid expression constructs were designed to study A. aegypti and H. sapiens GPA2/GPB5 subunit dimerization patterns and receptor signaling. Using previously available hexa-histidine-tagged A. aegypti GPA2-His (13), as well as FLAG (DYKDDDDK)-tagged (-FLAG) H. sapiens GPB5-FLAG (Genscript, Clone OHu31847D) plasmid vectors as template, the full ORF of each (A. aegypti and H. sapiens) GPA2 and GPB5 subunit coding sequence, including a consensus Kozak translation initiation sequence, was amplified and a hexa-histidine or FLAG tag sequence was incorporated on the carboxyl-terminus of subunits to produce the following fusion proteins; A. aegypti GPA2-FLAG, H. sapiens GPB5-His (Table S2). A pcDNA3.1+ mammalian expression construct containing mCherry, which was a gift from Scott Gradia (Addgene plasmid # 30125), was utilized to verify cell transfection efficiency. Experiments also utilized previously prepared pcDNA3.1+ constructs with A. aegypti GPB5-His and A. aegypti LGR1 coding sequences and dual promoter vector pBudCE4.1 containing both A. aegypti GPA2-His and GPB5-His (13). Additionally, pcDNA 3.1+ mammalian expression vector construct containing FLAG tagged H. sapiens thyrotropin receptor (TSHR-FLAG) (Genscript USA Inc., Clone OHu18318D), H. sapiens GPA2-FLAG (Genscript USA Inc., Clone OHu31847D), H. sapiens GPB5-FLAG (Genscript USA Inc., Clone OHu55827D) and pGlosensor™−22F cyclic adenosine monophosphate (cAMP) biosensor plasmid (Promega Corp., Madison, WI), which were used for receptor activation and intracellular signaling assays.
Generation of Tethered A. aegypti GPA2/GPB5 Fusion Construct
The ORFs of A. aegypti GPA2 and GPB5 sequences were tethered together in order to promote heterodimer interactions for testing in receptor activity assays with mammalian cell lines. A hexa-histidine tagged artificial linker sequence involving three glycine-serine repeats was used to fuse the amino-terminus of A. aegypti GPA2 propeptide sequence to the carboxyl-terminus of A. aegypti GPB5 prepropeptide sequence, using multiple PCR amplifications with several primer sets (Table S2) as performed previously using lamprey and Amphioxus GPA2 and GPB5 sequences (31, 32) (see SI Materials and Methods for details).
Transient Transfection of HEK 293T Cells
Human embryonic kidney (HEK 293T) cells were grown in complete growth media (Dulbecco's modified eagles medium: nutrient F12 (DMEM) media, 10% heat inactivated fetal bovine serum (Wisent, St. Bruno, QC) and 1X antimycotic-antibiotic) and maintained in a water-jacketed incubator at 37°C, 5% CO2. When cells reached ~80–90% confluency, they were transfected with mammalian expression plasmid constructs in 6-well tissue culture plates (Thermo Fisher Scientific, Burlington, ON) using Lipofectamine 3000 transfection reagent (Life Technologies, Carlsbad, CA) with 3:1 (μL:μg) transfection reagent to DNA ratio. Before transfection, culture media was replaced with either serum-free medium (DMEM and 1X antimycotic-antibiotic) for experiments that collected secreted proteins, or fresh complete growth medium for experiments that dually-transfected cells with pGlosensor™−22F cAMP biosensor plasmid and either H. sapiens TSHR, A. aegypti LGR1, or mCherry.
Preparation of Protein Samples
At 48 h post-transfection, serum-free culture media containing secreted proteins were collected and concentrated in 0.5 mL 3-kDa molecular weight cut-off centrifugal filters (VWR North America). In some experiments, cells were dislodged with PBS containing 5 mM ethylenediaminetetraacetic acid (EDTA; Life Technologies) (PBS-EDTA) pH 8.0, pelleted at 400 × g for 5 min, resuspended in PBS and transferred to 1.5 mL centrifuge tubes for a subsequent centrifugation. Cell lysates were then prepared by resuspending and sonicating cells for 5 s in cell lysis buffer containing 37.5 mM Tris, pH = 7.5, 1.5 mM EDTA, pH 8.0, 3% sodium dodecyl sulfate, 1.5% protease inhibitor cocktail (v/v), and 1.5 mM dithiothreitol (DTT). For receptor activity assays, in order to prevent carry-over of lysis buffer, cell lysates were concentrated in 3-kDa molecular weight cut-off centrifugal filters and re-constituted back to initial volumes with serum-free media for a total of three repetitions. Proteins were then used for cross-linking analysis, deglycosylation, and immunoblotting or used as ligands for functional receptor activation using the cAMP signaling biosensor assays.
Dissuccinimidyl suberate (DSS), a chemical cross-linker that is primarily reactive toward amino groups providing stabilization of weak or transient protein intermolecular interactions, was employed to study GPA2 and GPB5 protein-protein interactions. A. aegypti and H. sapiens GPA2-FLAG and GPB5-His proteins were kept separate or combined together (GPA2-FLAG/GPB5-His) and then treated with DSS (Sigma Aldrich, Oakville, ON). In some experiments, A. aegypti GPA2-His/GPB5-His were co-expressed using a dual promoter plasmid and as such, media containing both His-tagged subunits were directly treated with DSS. To treat secreted concentrates of culture media containing H. sapiens GPA2 and GPB5 proteins, 0.68 mM DSS was used whereas both 0.68 mM (data not shown) and 2.04 mM DSS was used to test the dimerization characteristics of A. aegypti GPA2-His/GPB5-FLAG proteins. Cross-linking was performed for 30 min at RT and reactions were quenched with 50 mM Tris, pH 7.4 for 10 min under constant mixing. To remove N-linked sugars, protein samples were treated with peptide-N-Glycosidase F (PNGase) (New England Biolabs, Whitby, ON) following manufacturers guidelines, with the only modification being that protein samples were not heated to 100°C before enzymatic deglycosylation. Experiments aimed to determine the effects of protein glycosylation on cross-linking ability first treated A. aegypti GPA2-His/GPB5-His subunits with PNGase and subsequently cross-linked samples with DSS after deglycosylation.
Western Blot Analysis
Samples were prepared in 2x Laemmli buffer (Sigma Aldrich, Oakville, ON) containing 4% SDS, 20% glycerol, 10% 2-mercaptoethanol, 0.004% bromophenol blue and 0.125 M Tris HCl, pH ~6.8, and resolved on 10 or 15% SDS-polyacrylamide gels under reducing conditions at 120 V for 90–110 min. Using a wet transfer system, proteins were then transferred to polyvinylidene difluoride (PVDF) membranes at 100 V for 75 min. For GPA2-FLAG/GPB5-His heterodimerization experiments, samples were run in duplicate on the same gel and following transfer, membranes were cut in half for separate primary antibody incubations. Membrane blots containing protein samples were blocked for 1 h in PBS containing 0.1% Tween-20 (Bioshop, Burlington, ON, Canada) and 5% skim milk powder (PBSTB) rocking at RT. After blocking, membranes were incubated overnight at 4°C on a rocking platform in PBSTB containing mouse monoclonal anti-His (1:500 dilution) or mouse monoclonal anti-FLAG (1:500) primary antibody solutions. The next day, membranes were washed three times with PBS containing 0.1% Tween-20 (PBST) for 15 min each wash and then were incubated in PBSTB containing anti-mouse HRP conjugated secondary antibody (1:2000–1:3000 dilution) for 1 h rocking at RT before washing again with PBST (3 × 15 min washes). Finally, blots were incubated with Clarity Western ECL substrate and images were developed using a ChemiDoc MP Imaging System (Bio-Rad Laboratories, Mississauga, ON) and molecular weight measurements and analysis were performed using Image Lab 5.0 software (Bio-Rad Laboratories, Mississauga, ON). Western blots used to study recombinant GPA2 and GPB5 dimerization were repeated at least three times.
Receptor Functional Activation Bioluminescence Assays
HEK 293T cells were co-transfected to express (i) H. sapiens TSHR, A. aegypti LGR1, or mCherry along with (ii) pGlosensor™−22F cAMP biosensor plasmid (Promega Corp., Madison, WI), which encodes a modified form of firefly luciferase with a fused cAMP binding moiety providing a biosensor for the direct detection of cAMP signaling in live cells. At 48 h post transfection, recombinant cells were dislodged with PBS-EDTA, pelleted at 400 × g for 5 min, resuspended in assay media [DMEM:F12 media with 10% fetal bovine serum (v/v)] containing cAMP GloSensor reagent (2% v/v), and incubated for 3 h rocking at RT shielded from light. White 96-well luminescence plates (Greiner Bio-One, Germany) were loaded under low light with previously prepared secreted or cell lysate protein concentrates (described above), forskolin or assay media alone, and incubated at 37°C for 30 min prior to performing receptor activity assays. For stimulatory G-protein (Gs) pathway detection, recombinant cells expressing either H. sapiens TSHR, A. aegypti LGR1, or mCherry along with the cAMP biosensor were pre-treated with 0.25 mM 3-isobutyl-1-methylxanthine (IBMX) for 30 min at RT with rocking and shielded from light. Using an automatic injector unit (BioTek Instruments Inc., Winooski VT), co-transfected HEK 293T cells were then loaded into wells containing various treatments, including 250 nM forskolin as a positive control or concentrates of protein fractions from mCherry-transfected cells as a negative control. For inhibitory G-protein (Gi/o) pathway testing, A. aegypti GPA2 and/or GPB5 was tested for the ability to inhibit a forskolin-induced cAMP-mediated bioluminescent response. Using an automatic injector unit (BioTek Instruments Inc., Winooski VT), cells expressing LGR1 or mCherry (LGR1 activation and signaling negative control) were loaded into wells that contained various ligand treatments. Subsequently, 250 nM or 1 μM forskolin was added to wells immediately after the addition of cells, using a second automatic injector unit. For bioluminescent assays with tethered GPA2/GPB5 fusion proteins, recombinant cells expressing or not expressing LGR1 were equally divided to test Gs and Gi signaling pathways simultaneously using the same batch of cells per biological replicate. In all assays, luminescence was measured every 2 min for 20 min at 37°C using a Synergy 2 Multimode Microplate Reader (BioTek, Winooski, VT) and averaged over 4–8 technical replicates for each treatment. To calculate the relative luminescent response, data were normalized to luminescent values recorded from treatments with 250 nM or 1 μM forskolin alone. Assays were performed repeatedly and involved 3–6 independent biological replicates. Relative luminescent values were compiled on Excel and transferred into GraphPad Prism 8.0 for figure preparation and statistical analysis. Data were analyzed using one-way or two-way ANOVA with Tukey's multiple comparison post-test (p < 0.05).
Results
A. aegypti GPA2 and GPB5 Subunit Expression Localization
To determine the distribution of GPA2 and GPB5 subunit expression in the central nervous system and peripheral tissues, adult mosquito organs were analyzed using RT-qPCR. GPA2 and GPB5 subunit transcript was detected in the central nervous system of adult male and female mosquitoes, with significantly enriched expression in the abdominal ganglia relative to other regions of the nervous system and peripheral tissues (Figures 1A,B). Fluorescence in situ hybridization was employed to localize cell-specific expression of the GPA2 and GPB5 transcripts in the abdominal ganglia. GPA2 and GPB5 anti-sense RNA probes identified two bilateral pairs of neuroendocrine cells (Figures 1C,D) within each of the first five abdominal ganglia in male and female mosquitoes whereas the control sense probes did not detect cells in the nervous system (Figures 1E,F). Within each of these first five abdominal ganglia, GPA2 transcript localized to similar laterally-positioned cells as the GPB5 transcript (Figures 1G,H). To determine if cells expressing GPA2 transcript were the same cells expressing GPB5 transcript, abdominal ganglia were simultaneously treated with both GPA2 and GPB5 anti-sense RNA probes. Using this dual probe approach, results confirmed the detection of only two bilateral pairs of cells (Figures 1I,J) that displayed a greater staining intensity compared to the intensity of cells detected when using either the GPA2 or GPB5 anti-sense probe alone (Figures 1C,D).
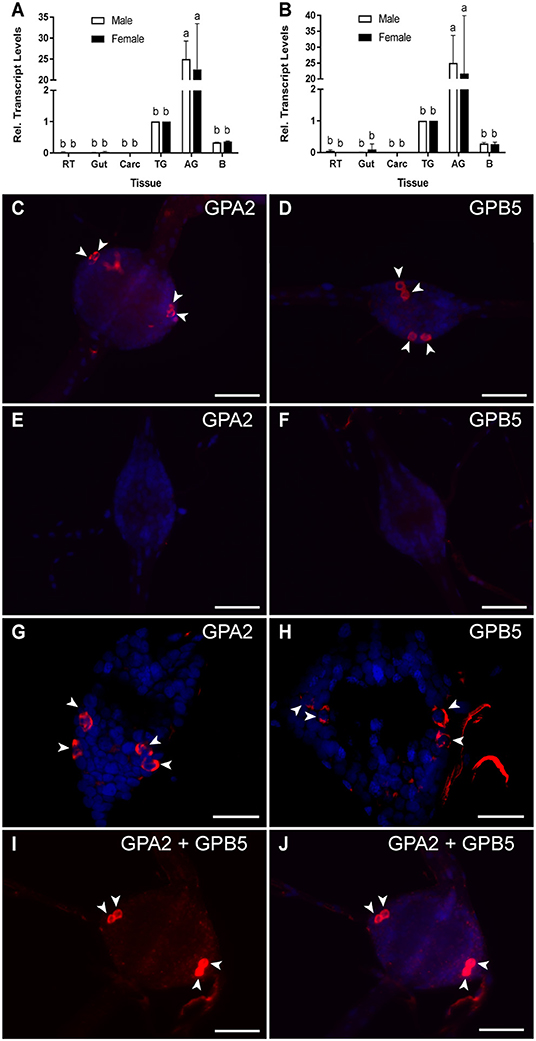
Figure 1. GPA2/GPB5 subunit transcript expression and localization in adult A. aegypti. (A,B) RT-qPCR examining GPA2 (A) and GPB5 (B) transcript expression in the central nervous system of adult mosquitoes, with significant enrichment in the abdominal ganglia (AG). Subunit transcript abundance is shown relative to their expression in the thoracic ganglia (TG). Mean ± SEM of three biological replicates. Columns denoted with different letters are significantly different from one another. Multiple comparisons two-way ANOVA test with Tukey's multiple comparisons (P < 0.05) to determine sex- and tissue-specific differences. Reproductive tissues (RT), alimentary canal (Gut), carcass (Carc), brain (B). Fluorescence in situ hybridization anti-sense (C,D,G–J) and sense (E,F) probes to determine GPA2 and GPB5 transcript localization (GPA2 and/or GPB5 transcript, red; nuclei, blue) in the abdominal ganglia of adult mosquitoes. Unlike sense probe controls (E,F), two bilateral pairs of cells (arrowheads) were detected with GPA2 (C,G) and GPB5 (D,H) anti-sense probes in the first five abdominal ganglia of adult male and female A. aegypti. Co-localization of the GPA2 (G) and GPB5 (H) transcript was verified by treating abdominal ganglia dually with a combination of GPA2 and GPB5 anti-sense probes (I,J) that revealed two, intensely-stained bilateral pairs of cells. In (C–F,I,J), microscope settings were kept identical when acquiring images of control and experimental ganglia. The second abdominal ganglion is depicted in each image as a representative, given that no differences in the number nor staining intensity of cells were observed between ganglia of a given mosquito. Scale bars are 50 μm in (C–F,I,J) and 40 μm in (G,H). Experimental procedures were repeated three-four times with five mosquitoes (of each sex) per trial.
Using a custom antibody targeting A. aegypti GPB5, we next sought to immunolocalize GPB5 protein in the abdominal ganglia. GPB5 immunoreactivity localized to two bilateral pairs of cells (Figure 2A) within the first five ganglia, which were in similar positions to cells expressing GPA2 and GPB5 transcript (Figures 1C,D,G–J). Regardless of sex, in 30% of mosquitoes examined, three instead of two bilateral pairs of cells immunolocalized to each of the first five abdominal ganglia of the ventral nerve cord (Figure 2B), whereas no cells were ever detected in the sixth terminal ganglion (Figure 2C). For a given mosquito, there were no differences in the number of GPB5 immunoreactive cells detected between different ganglia. Along the lateral sides of each of the first five abdominal ganglia, GPB5 immunoreactive processes were observed to closely associate into a tract of axons that emanated through the lateral nerve (Figure 2B). Control treatments with GPB5 antibody preabsorbed with the GPB5 immunogenic antigen did not detect any cells in ganglia (Figure 2D).
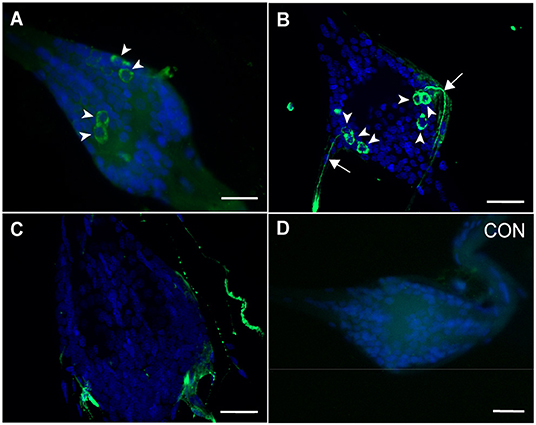
Figure 2. Immunolocalization of GPB5 subunit expression in the abdominal ganglia of adult A. aegypti. Experimental treatments demonstrated GPB5 immunoreactivity (green) in two (A), and in some mosquitoes, three (B) bilateral pairs of neuroendocrine cells (arrowheads) within the first five abdominal ganglia of the ventral nerve cord (DAPI, blue). Optical sections of ganglia revealed axonal projections emanate from GPB5-immunoreactive cells through the lateral nerves (arrows). No cells were detected in the sixth, terminal ganglion (C) and in control treatments (CON) where GPB5 antibody was preabsorbed with GPB5 synthetic antigen (D). In (A,D) or (B,C), microscope settings were kept identical when acquiring images of control and experimental ganglia. The second abdominal ganglion is depicted in (A,B,D) as a representative, given that no differences in the number nor staining intensity of cells were observed between ganglia of a given mosquito. Scale bars are 25 μm in (A–D) and 20 μm in (B,C). Experimental procedures were repeated three-four times with five mosquitoes (of each sex) per trial.
Cross-Linking Analyses to Determine A. aegypti GPA2 and GPB5 Subunit Interactions
A. aegypti GPA2 and GPB5 subunit protein interactions were studied using western blot analysis of recombinant proteins from HEK 293T cells expressing each subunit independently or co-expressing both subunits in the same cells using a dual promoter plasmid. Under control conditions, GPA2 protein is represented as two bands at 16 and 13 kDa, which correspond to the glycosylated and non-glycosylated forms of A. aegypti GPA2, respectively (Figure 3A). Following deglycosylation with PNGase, the higher molecular weight band of GPA2 is eliminated, and the non-glycosylated lower molecular weight band intensifies (Figure 3A). Interestingly, when the GPA2 subunit was tested to examine potential homodimerization, an additional strong band at ~32 kDa was detected, which migrates to ~30 kDa when cross-linked protein samples were deglycosylated using PNGase (Figure 3A). Under control conditions, GPB5 protein is represented as a band size at 24 kDa and the migration pattern is not affected by PNGase treatment (Figure 3B). Following treatment with DSS, a faint second band appears at 48 kDa, which does not change in molecular weight after treatment with PNGase (Figure 3B). Three independent band sizes at 24 kDa (GPB5), 16 kDa (glycosylated GPA2) and 13 kDa (non-glycosylated GPA2) were detected in lanes loaded with protein isolated from HEK 293T cells co-expressing GPA2 and GPB5 subunits (Figure 3C). After removal of N-linked sugars, the 24 kDa band is not affected but the 16 kDa band disappears and 13 kDa band intensifies (Figure 3C), as observed when assessing the GPA2 subunit independently (Figure 3A). Cross-linked samples show the addition of two higher molecular weight bands at ~48 and ~32 kDa, the latter of which migrates lower to ~30 kDa when subjected to PNGase treatment (Figure 3C). Given the resolution and intensity of the detected bands, the molecular weight band at ~45 kDa could indicate GPA2/ GPB5 heterodimeric interactions (13 or 16 kDa + 24 kDa = 37–40 kDa). Alternatively, these bands could reflect GPB5 (24 + 24kDa = 48 kDa) homodimers. As a result of this uncertainty, additional experiments were performed to clarify whether A. aegypti GPA2 and GPB5 subunits are heterodimeric partners.
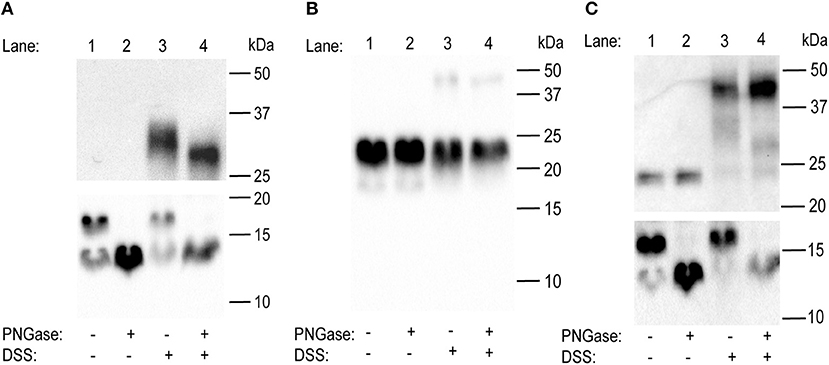
Figure 3. Western blot analyses to determine the effects of glycosylation on homo- and heterodimer formation on the glycoprotein hormone (GPA2/GPB5) subunits in the mosquito, A. aegypti. (A) In untreated conditions, western blot analysis of GPA2 subunit alone reveals two bands at 16 and 13 kDa. Whereas, following treatment with PNGase, the higher molecular weight band disappears and the 13 kDa band is intensified. A thick, additional band at ~32 kDa appears when GPA2 protein is cross-linked with DSS, and this band migrates slightly lower to ~30 kDa when GPA2 protein is treated with both DSS and PNGase. (B) A 24 kDa band is observed in lanes loaded with untreated GPB5 subunit alone. Upon PNGase treatment, the 24 kDa band is not affected; however, upon treatment with DSS, a second faint band appears at 48 kDa that is not affected by deglycosylation. (C) Western blot analyses of co-expressed GPA2 and GPB5 subunits shows three distinct band sizes at 24, 16, and 13 kDa, corresponding to the GPB5 subunit and two forms of GPA2 subunit protein. Similar to (A), after treatment with PNGase, the higher molecular weight form of GPA2 is eliminated and the 13 kDa band intensifies. When GPA2/GPB5 protein is cross-linked, two additional bands are detected at ~48 and ~32 kDa; however, following cross-linking and PNGase treatment, the ~32 kDa band is eliminated leaving only the 30 kDa band along with the unaffected ~48 kDa band. GPA2 (A) and GPA2/GPB5 (C) subunit protein was resolved on either a 10% (top) or 15% (bottom) polyacrylamide gel under denaturing conditions.
Heterodimerization of Mosquito and Human GPA2/GPB5
Using yeast two-hybrid analyses and cross-linking experiments, it was previously shown that human GPA2 (hGPA2) and GPB5 (hGPB5) subunits are capable of heterodimerization (4). As a result, to verify whether A. aegypti GPA2 and GPB5 subunit proteins are heterodimeric candidates, experiments were performed alongside hGPA2/hGPB5 subunit proteins, using the latter as a positive experimental control for heterodimer detection.
Initially, single-promoter expression constructs were designed to incorporate a FLAG-tag and His-tag on the C-terminus of (human and mosquito) GPA2 and GPB5 subunits, respectively (i.e., GPA2-FLAG and GPB5-His). When probed with an anti-His antibody, no bands were detected in lanes containing only GPA2-FLAG (human and mosquito) protein (Figures 4A,B). Similarly, when an anti-FLAG antibody, no bands were detected in lanes containing only GPB5-His protein (human and mosquito) (Figures 4A,B).
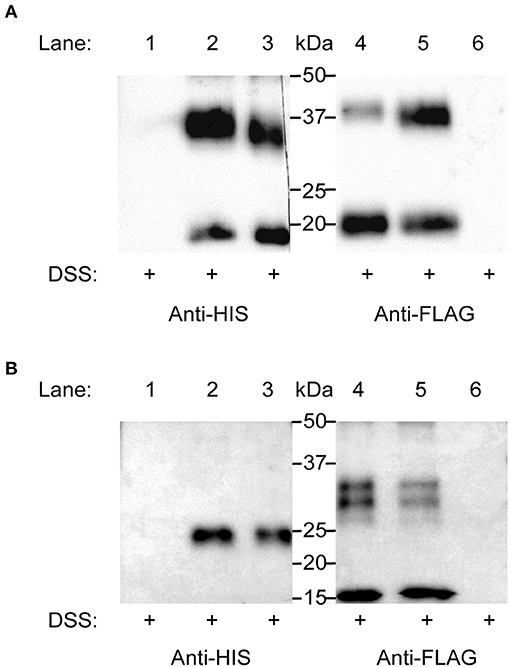
Figure 4. Elucidating heterodimerization of H. sapiens (human) (A) and A. aegypti (mosquito) (B) GPA2 and GPB5 subunits. Single promoter expression constructs for human and mosquito GPA2-FLAG and GPB5-His were used for transient expression in HEK 293T cells. Protein was harvested and subsequently concentrated, treated with DSS cross-linker, and probed with an anti-His or an anti-FLAG antibody after SDS-PAGE. (A,B) No bands were detected in lanes loaded with cross-linked GPA2-FLAG protein (Lane 1) or cross-linked GPB5-His protein (Lane 6), probed with an anti-His antibody or anti-FLAG antibody, respectively. (A) Bands corresponding to the monomeric form (18 kDa) and homodimer (36 kDa) of cross-linked human GPB5-His (Lane 3) and to the monomeric form (20 kDa) and homodimer (40 kDa) of cross-linked human GPA2-FLAG protein (Lane 4). A combination of the subunits with subsequent cross-linking of separately-produced human GPA2-FLAG and GPB5-His protein (Lane 2, 5) revealed a band size correlating to the human GPA2/GPB5 heterodimer (38 kDa), detected using an anti-His (Lane 2) or anti-FLAG (Lane 5) antibody. (B) Bands corresponding to the mosquito GPB5 monomer (24 kDa) (Lane 3), mosquito GPA2 glycosylated monomer (16 kDa) and homodimer pairs (30 and 32 kDa) (Lane 4). No detection of bands correlating to mosquito GPA2/GPB5 heterodimer (37–40 kDa) were observed, when probed with either anti-His (Lane 2) or anti-FLAG (Lane 5) antibodies.
Lanes loaded with cross-linked hGPB5-His protein revealed two bands at 18 kDa (monomer) and 36 kDa (homodimer) (Figure 4A). Similarly, in lanes loaded with cross-linked hGPA2-FLAG protein, two bands at 20 kDa (monomer) and 40 kDa (homodimer) were detected (Figure 4A). To determine subunit heterodimerization, hGPA2-FLAG protein was combined with hGPB5-His protein (i.e., produced separately in different cell batches) and subsequently crosslinked using DSS. Results showed an intense 38 kDa band size that correlated to the molecular weight of the hGPA2-FLAG/hGPB5-His heterodimers; detected with both anti-His and anti-FLAG primary antibody solutions (Figure 4A).
Identical experiments were conducted using A. aegypti GPA2-FLAG/GPB5-His protein but using three-fold higher concentration of DSS cross-linker to help improve detection of inter-subunit interactions. Results demonstrated that lanes loaded with DSS-treated GPB5-His protein resulted in a 24 kDa monomer band (Figure 4B), whereas lanes containing cross-linked GPA2-FLAG detected a 16 kDa (glycosylated monomer), 30 and 32 kDa (homodimers) band size (Figure 4B). As a result, unlike immunoblots containing hGPA2-FLAG and hGPB5-His protein (Figure 4A), lanes loaded with cross-linked A. aegypti GPA2-FLAG and GPB5-His failed to provide evidence of bands correlating to the predicted molecular weight of an A. aegypti GPA2/GPB5 heterodimer, which would be expected at 37–40 kDa (Figure 4B).
A. aegypti GPA2/GPB5 Unable to Activate LGR1-Mediated Gs and Gi/o Signaling Pathways
Bioluminescent assays were employed to confirm LGR1 interaction with mosquito GPA2 and/or GPB5 subunits and elucidate downstream signaling pathways upon receptor activation. Since human GPA2/GPB5 (hGPA2/hGPB5) has previously been shown to bind and activate human thyrotropin receptor (hTSHR) mediating a stimulatory G protein (Gs) signaling pathway (4), we first validated our experimental design using hGPA2/hGPB5 and hTSHR as a positive control. Recombinant hGPA2 and hGPB5 subunit proteins were produced in HEK 293T cells with single promoter expression constructs containing the hGPA2 or hGPB5 sequences. Conditioned culture media containing secreted proteins were subsequently concentrated, and crude extracts containing hGPA2, hGPB5 or a combination of hGPA2 and hGPB5 subunits were tested as ligands on HEK 293T cells expressing the hTSHR and a cAMP-sensitive firefly luciferase biosensor, which produces bioluminescence upon interacting with cAMP. Control treatments involved the incubation of hTSHR/ luciferase-expressing cells with concentrated media collected from mCherry-transfected cells (negative control), and data was normalized to treatments with 250 nM forskolin (positive control) (Figures 5A,B). As expected, the results indicate that incubation with extracts containing a combination of both hGPA2 and hGPB5 were required to stimulate a cAMP-mediated luminescent response from hTSHR-expressing cells, but not when incubated with extracts containing individual subunits (Figure 5A). We next performed identical experiments using A. aegypti GPA2/GPB5 and LGR1. Given the availability of a dual promoter plasmid (pBudCE4.1), an additional treatment was performed whereby GPA2/GPB5 subunits were co-expressed within the same cells and conditioned media was concentrated as described above. Unlike the results using hGPA2/hGPB5 subunit homologs on HEK 293T cells expressing the hTSHR (Figure 5A), no combination of mosquito GPA2 and/or GPB5 subunit proteins led to an increase in the cAMP-based luminescent response in HEK 293T cells expressing mosquito LGR1 (Figure 5B).
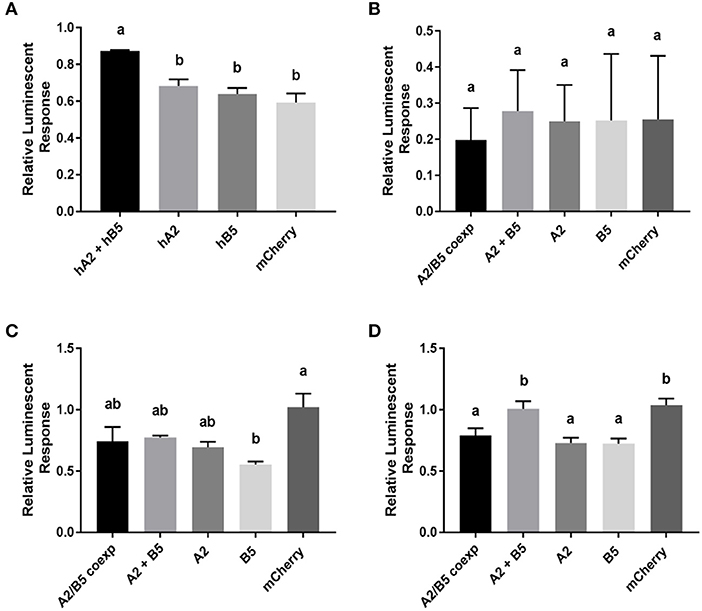
Figure 5. cAMP-mediated bioluminescence assays to determine the effect of GPA2, GPB5, and GPA2/GPB5 on G-protein signaling of H. sapiens (human) TSHR (A), A. aegypti (mosquito) LGR1 (B,C), or cells expressing a red fluorescent protein, mCherry (D). Secreted protein fractions for each subunit were prepared separately from HEK 293T cells expressing human GPA2 (hA2), human GPB5 (hB5), mosquito GPA2 (A2), mosquito GPB5 (B5), mCherry, or co-expressing mosquito GPA2 and GPB5 in a dual promoter plasmid (A2/B5 coexp). Secreted protein fractions were then tested separately or combined (A2 + B5) and then incubated with cells co-expressing the cAMP biosensor along with either (A) human TSHR, (B,C) mosquito LGR1 or (D) mCherry, the latter of which was used as a negative control in the functional assay and also served to verify transfection efficiency of HEK 293T cells. (A–D) Luminescent values were recorded and normalized to luminescence response from treatments with 250 nM forskolin. (A) Unlike treatments with human GPA2 (hA2) or human GPB5 (hB5) applied singly, a significant increase in cAMP-mediated luminescence was observed when TSHR-expressing cells were incubated with culture media containing both human GPA2 and human GPB5 (hA2 + hB5), relative to incubations with mCherry controls. (B) No differences in luminescence were observed when LGR1-expressing cells were incubated with media containing mosquito GPA2/GPB5 subunits, compared to mCherry controls. (C,D) To test Gi/o signaling pathways, experiments were performed in the presence of 250 nM forskolin to determine the ability for each ligand to inhibit a forskolin-induced cAMP response. (C) The addition of GPB5 on LGR1-expressing cells significantly inhibited forskolin-induced luminescent response, compared to treatments with mCherry controls; (D) however, this inhibition was also observed when GPA2 and GPB5 proteins were incubated with HEK 293T cells in the absence of LGR1. Mean ± SEM of three (A,B,D) or six (C) biological replicates. Columns denoted with different letters are significantly different from one another. Multiple comparisons one-way ANOVA test with Tukey's multiple comparisons (P < 0.05).
Using an in silico analysis to predict coupling specificity of A. aegypti LGR1 and human TSHR to different families of G-proteins (33), we determined that A. aegypti LGR1 is strongly predicted to couple to inhibitory (Gi/o) G proteins Table S3). As a result, to determine whether A. aegypti GPA2 and/or GPB5 activate a Gi/o signaling pathway, various combinations of GPA2 and GPB5 were tested for their ability to inhibit a 250 nM forskolin-induced rise in cAMP measured by changes in bioluminescence (Figures 5C,D). Results revealed that sole treatments of GPB5 proteins alone significantly inhibited a forskolin-induced luminescent response, relative to control treatments with mCherry proteins, when incubated with cells expressing LGR1 (Figure 5C). However, similar inhibitory effects of GPA2 and GPB5 proteins were also observed with cells lacking LGR1 expression (Figure 5D).
Characterization of Tethered A. aegypti GPA2/GPB5
Activity on the hTSHR was only observed when both hGPA2 and hGPB5 subunits were coapplied for receptor activation (Figure 5A) and, unlike the heterodimerization of hGPA2/hGPB5 observed in our experiments, mosquito GPA2/GPB5 lacked evidence of heterodimerization (Figure 4). In light of these observations, we hypothesized that the activation of A. aegypti LGR1 also required subunit heterodimerization. To mimic GPA2/GPB5 heterodimers using the heterologous expression system, both GPA2 and GPB5 mosquito subunits were expressed as a tethered, single-chain polypeptide by fusing the C-terminus of the GPB5 prepropeptide sequence with the N-terminus of the GPA2 propeptide sequence, using a tagged linker sequence composed of twelve amino acids, involving three glycine-serine repeats and six histidine residues.
HEK 293T cells were transfected to transiently express a single promoter plasmid construct containing the tethered GPA2/GPB5 sequence, or the red fluorescent protein (mCherry) as a negative control. To verify expression of the construct, at 48 h post-transfection, cell lysates along with the conditioned culture media, the latter of which contains secreted proteins, were collected for immunoblot analysis. No bands were detected in lanes containing cell lysate or secreted protein fractions of mCherry transfected cells (Figure 6A). However, in the lysates of cells transfected to express tethered GPA2/GPB5, an intense band at 32 kDa, and less intense band at 37 kDa were detected; the latter of which corresponds to the predicted molecular weight of non-glycosylated GPA2 (13 kDa) plus GPB5 (24 kDa) (Figure 6A). In lanes containing secreted fractions of tethered GPA2/GPB5- transfected cells, a band at 37 kDa was again detected, as well as a stronger 40 kDa band size that correlates to the predicted molecular weight of glycosylated GPA2 (16 kDa) plus GPB5 (24 kDa) (Figures 6A,B). After secreted protein extracts containing tethered GPA2/GPB5 proteins were treated with PNGase, the higher 40 kDa molecular weight band disappears and the 37 kDa band size intensifies, which confirms the observed molecular weight shift results from removal of N-linked oligosaccharides (Figure 6B). Thus, the tethered GPA2/GPB5 undergoes similar post-translational processing as observed for the subunits expressed individually.
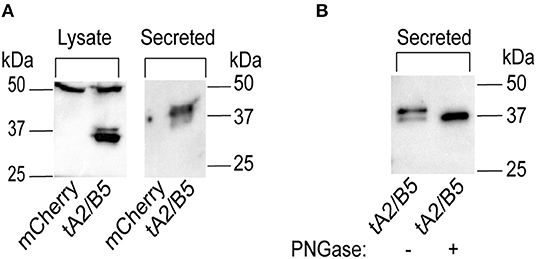
Figure 6. Verification of A. aegypti GPA2/GPB5 tethered protein expressed in HEK 293T cells. (A) Western blot analysis of secreted or cell lysate protein fractions of HEK 293T expressing tethered GPA2/GPB5 (tA2/B5) or red fluorescent protein (mCherry) as a control. Tethered GPA2/GPB5 is represented as two bands at 32 and 37 kDa band in cell lysate fractions, and as two bands at 37 and 40 kDa in secreted fractions; however, no bands were detected in lanes loaded with proteins from mCherry transfected cells. (B) Upon treatment of tethered GPA2/GPB5 secreted protein fractions with PNGase, the 40 kDa band is eliminated and the 37 kDa band intensifies, indicating removal of N-linked oligosaccharides.
Tethered A. aegypti GPA2/GPB5 Activates LGR1
The activity of tethered GPA2/GPB5 proteins on LGR1 activation was examined. Cell lysate or secreted protein fractions collected from mCherry- or tethered GPA2/GPB5-transfected cells were incubated with HEK 293T cells co-expressing the cAMP luciferase biosensor and either A. aegypti LGR1 or mCherry (i.e., not expressing LGR1). Whether tethered GPA2/GPB5 proteins (cell lysate or secreted fractions) could elevate cAMP or inhibit a forskolin-induced rise in cAMP was assessed and compared to negative control treatments with proteins harvested from mCherry-transfected cells.
Without the addition of tethered GPA2/GPB5 proteins, the basal luminescent levels were higher in LGR1-transfected cells compared to mCherry-transfected cells (Figure 7), suggesting constitutive activity of A. aegypti LGR1 elevating cAMP levels. However, the application of neither secreted protein fractions nor cell lysates of tethered GPA2/GPB5-transfected cells elicited an increase in the cAMP-mediated luminescent response relative to control treatments with mCherry proteins (Figures 7A,B). Secreted protein fractions containing tethered GPA2/GPB5 protein had no effect on the forskolin-induced cAMP-dependent luminescence, compared to control treatments with mCherry secreted proteins in LGR1-expressing cells (Figure 7C). Notably, however, treatments of LGR1-expressing cells with cell lysates containing tethered GPA2/GPB5 significantly inhibited the luminescent response owing to the forskolin-induced rise in cAMP relative to treatments with mCherry-transfected cell lysates, which was not observed in cells lacking LGR1 expression (Figure 7D).
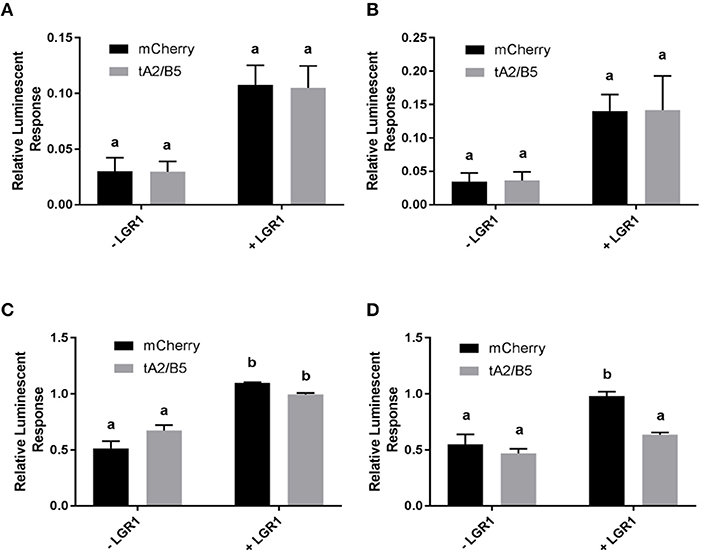
Figure 7. cAMP-mediated bioluminescence assay determining the effects of tethered GPA2/GPB5 on receptor activation and G protein signaling of LGR1. Secreted protein fractions (A,C) and cell lysates (B,D) derived from cells transiently expressing tethered GPA2/GPB5 (tA2/B5) or red fluorescent protein (mCherry) were tested for their ability to stimulate (Gs signaling) (A,B) or inhibit 1 μM forskolin-induced (Gi/o signaling) (C,D) cAMP-mediated luminescence. (A–D) Luminescence response was recorded and shown as normalized to the luminescence from sole treatments with 1 μM forskolin on LGR1-expressing cells. (C,D) In treatments involving mCherry proteins, the relative luminescence response was greater in LGR1-expressing cells (+ LGR1) compared to cells not expressing LGR1 (–LGR1). (A,B) Incubation of LGR1-expressing cells with tA2/B5 secreted (A) or cell lysate (B) proteins, failed to increase cAMP-mediated luminescence above background levels from incubations with mCherry proteins. (C,D) 1 μM forskolin along with either mCherry proteins or tA2/B5 proteins was added to cells in the presence or absence of LGR1 expression. The ability for each ligand treatment to reduce a forskolin-induced increase in cAMP luminescence was examined. (C) The tA2/B5 secreted protein samples incubated with LGR1-expressing cells did not significantly affect the forskolin-induced cAMP luminescence, compared to control treatments with secreted proteins from mCherry expressing cells. (D) Relative to incubations with mCherry cell lysate proteins, cell lysates containing tA2/B5 protein significantly inhibited forskolin-induced elevations of cAMP-mediated luminescence, and this inhibition was specific to LGR1-expressing cells. Mean ± SEM of three biological replicates. Columns denoted with different letters are significantly different from one another. Multiple comparisons two-way ANOVA test with Tukey's multiple comparisons (P < 0.05).
Discussion
GPA2/GPB5: A Neuropeptide Produced in the Abdominal Ganglia of Mosquitoes
The central nervous system (CNS) of adult mosquitoes is comprised of a brain and a ventral nerve cord, consisting of the suboesophageal ganglion, three fused thoracic ganglia and six abdominal ganglia. GPA2 and GPB5 transcripts are significantly enriched in the abdominal ganglia of adult mosquitoes relative to peripheral tissues and other regions of the CNS. Although low levels of GPA2 and GPB5 transcripts were detected in the thoracic ganglia and brain using RT-qPCR, fluorescence in situ hybridization (FISH) techniques used to localize GPA2 and GPB5 transcripts, along with immunohistochemical detection of GPB5, did not identify specific cells in these regions of the nervous system. Instead, GPA2 and GPB5 transcripts, as well as GPB5 immunoreactivity was identified in 2–3 laterally-localized bilateral pairs of neuroendocrine cells within the first five abdominal ganglia. These findings are consistent with previous findings in the fruit fly D. melanogaster, where GPA2 and GPB5 subunit transcripts were localized to four bilateral pairs of neuroendocrine cells within the abdominal neuromeres in the fused ventral nerve cord, that were distinct from cells expressing other neuropeptides including leucokinin, bursicon, crustacean cardioactive peptide or calcitonin-like diuretic hormone (18).
Both FISH and immunohistochemical techniques revealed GPA2 and GPB5 expression within two bilateral pairs of neuroendocrine cells, that were positioned slightly posterior to where the lateral nerves emanate from these ganglia. It is possible that GPB5 immunoreactive axons follow similar projection patterns as ovary ecdysteroidogenic hormone (OEH I), a gonadotropin produced in lateral neurosecretory cells in the abdominal ganglia of mosquitoes (34). OEH I positive cells emanate through the lateral nerves, similar to our findings with GPB5 immunoreactive cells, and terminate on perivisceral organs that function as neurohaemal release sites (34).
In some abdominal ganglia preparations, a third bilateral pair of cells immunoreactive for GPB5 protein was detected; however these additional cells were not detected using FISH, which suggests GPB5 transcript may be differentially regulated between different bilateral pairs of cells. Given that the same number of cells were observed to express GPA2 transcript, and these cells localized to similar positions as GPB5 expressing cells, the glycoprotein hormone subunits are likely co-expressed in the same cells. To investigate cellular co-expression of GPA2/GPB5, abdominal ganglia were simultaneously treated with both GPA2- and GPB5-targeted anti-sense RNA probes. From this analysis, again only two bilateral pairs of cells were detected and these were more intensly stained compared to preparations treated with either probe alone, which confirms that GPA2 and GPB5 are indeed co-expressed within the same neurosecretory cells of the first five abdominal ganglia in adult mosquitoes. The cellular co-expression of GPA2 and GPB5 proteins implies that, upon a given stimulus, both subunits are regulated in a similar manner and are likely simultaneously released following the appropriate stimulus. Importantly, since co-expression and heterodimerization of the classic vertebrate glycoprotein hormone subunits takes place within the same cells (21, 22), these findings indicate that the mosquito GPA2/GPB5 subunits may be produced and released as heterodimers in vivo.
Heterodimerization and Homodimerization of GPA2/GPB5
To study the interactions of A. aegypti GPA2 and GPB5 subunits in vitro, hexa-histidine tagged proteins secreted into the culture media of transfected HEK 293T cells were collected and analyzed under denaturing conditions after cross-linking treatments, which had been utilized previously to show GPA2/GPB5 heterodimerization in other organisms (4, 6, 29). Cross-linked protein samples were then deglycosylated to identify whether the removal of N-linked sugars affected dimerization. Treatment of mosquito GPA2 and GPB5 subunits individually with cross-linker resulted in the detection of bands with sizes corresponding to homodimers of GPA2 (~32 kDa) and GPB5 (48 kDa). GPA2 homodimer bands migrated lower to ~30 kDa following deglycosylation treatment with PNGase. However, experiments performed with cross-linked GPA2/GPB5 protein were not able to confirm heterodimerization since the detected band sizes could also reflect GPB5 homodimeric interactions. Similarly, previous studies that demonstrated GPA2/GPB5 heterodimerization in human (4, 6) and fruit fly (29) did not provide evidence on the interactions of each subunit alone to determine if homodimerization is possible. In these earlier studies, molecular weight band sizes that were identified as heterodimers could have been the result of homodimeric interactions (4, 6, 29). As a result, the findings herein with mosquito GPA2/GPB5 indicate additional experiments are required to confirm GPA2/GPB5 heterodimerization in these organisms.
To clarify whether A. aegypti (mosquito) GPA2/GPB5 heterodimerize, each subunit was differentially tagged (GPA2-FLAG and GPB5-His), and immunoblots containing various combinations of cross-linked subunits were probed with either anti-FLAG or anti-His antibody. As a positive control, experiments were first performed using H. sapiens (human) hGPA2/hGPB5 subunit proteins. Similar to mosquito GPA2 and GPB5 subunits, results showed hGPA2 and hGPB5 subunits are capable of homodimerization. To study heterodimeric interactions, GPA2 and GPB5 subunit proteins were expressed separately in HEK 293T cells. Upon combining and treating protein samples with DSS, a molecular weight band size at 38 kDa, corresponding to the molecular weight of hGPA2/hGPB5 heterodimers (hGPA2-FLAG and hGPB5-His tagged), was detected and migrated differently than bands corresponding to hGPA2 (40 kDa) and hGPB5 (36 kDa) homodimers. Taken together, our results confirm hGPA2 and hGPB5 subunits are indeed capable of heterodimerization in vitro. Further, an induction of cAMP was observed when both subunits were present for TSHR functional activation, whereas treatments with individual subunits failed to significantly increase cAMP-mediated luminescence. As a result, hGPA2/hGPB5 is capable of heterodimerization, and since a combination of both subunits were required to signal a TSHR-mediated elevation in cAMP, these heterodimers are required to functionally activate its cognate glycoprotein hormone receptor.
Analogous experiments using the same concentration of DSS cross-linker (data not shown) as well as three-fold higher concentrations were performed with mosquito GPA2-FLAG and GPB5-His subunit proteins. Irrespective of the DSS concentration used, no bands corresponding to the expected molecular weights of mosquito GPA2/GPB5 heterodimers (37–40 kDa) were observed, but rather, only GPA2 and GPB5 homodimers were detected. Moreover, mosquito GPA2/GPB5, either each subunit alone, mixed from different cell batches or co-expressed in the same cells using a dual promoter vector, was unable to stimulate a cAMP-mediated luminescent response in HEK 293T cells expressing LGR1. Since we identified A. aegypti LGR1 is predicted to couple a Gi/o signaling pathway (Table S3), we examined if mosquito GPA2/GPB5 could inhibit a forskolin-induced cAMP response. Sole treatments of GPA2 and GPB5 subunit proteins inhibited a forskolin-induced rise in cAMP, however these inhibitory actions were not owed to G protein signaling events related to A. aegypti LGR1, since inhibition was observed in control cell lines in the absence of LGR1. These results suggest mosquito GPA2 and GPB5 subunit proteins may non-specifically interact with other endogenously expressed proteins, like the orphan receptors LGR4 and LGR5 that are highly expressed in HEK 293T cells (35).
A. aegypti GPA2/GPB5 Heterodimers Activate LGR1 and Initiate a Switch From Gs to Gi Coupling
Activation of the human thyrotropin receptor was only observed when both hGPA2 and hGPB5 subunits were present which, unlike data obtained involving mosquito subunits, demonstrated hGPA2/hGPB5 subunit heterodimerization in vitro using the mammalian heterologous system. The inability of mosquito GPA2 and GPB5 subunits to successfully heterodimerize could result from improper protein folding of insect-derived secretory proteins in HEK 293T cells used for heterologous expression. As a result, future experiments should test the expression and heterodimerization of A. aegypti GPA2/GPB5 in other heterologous systems such as insect cell lines, which could provide a more appropriate environment for tertiary and quaternary protein structure formation. Nonetheless, given the observed co-localization of the subunits within bilateral pairs of cells in the first five abdominal ganglia in A. aegypti, which is comparable to cellular colocalization shown earlier in D. melanogaster (18), we proposed that A. aegypti GPA2/GPB5 heterodimers would be required to functionally activate LGR1 in vitro. To confirm this possibility, a tethered construct was designed linking the C-terminus of GPB5 to the N-terminus of GPA2 using a histidine tagged glycine/serine-rich linker sequence. Natural and synthetic linkers function as spacers that connect multidomain proteins, and are commonly used to study unstable or weak protein-protein interactions (36). The incorporation of a linker sequence between glycoprotein hormone subunits has been performed previously, and does not affect the assembly, secretion or bioactivity of human FSH (37), TSH (38), and CG (39). The conversion of two independent glycoprotein hormone subunits into a single polypeptide chain using a glycine-serine repeat linker sequence has also been performed recently with lamprey GPA2/GPB5 (31), which was shown to induce a cAMP response. Interestingly, similar proteins involving TSH alpha fused to TSH beta with carboxyl-terminal peptide (CTP) as a linker promoted a three-fold higher induction of cAMP compared to wild-type TSH, likely because the addition of a CTP linker increases protein stability and flexibility (40).
Thus, tethered A. aegypti GPA2/GPB5 was expressed in HEK 293T cells and secreted protein fractions along with cell lystaes were collected for expression studies. In cell lysates, immunoblot studies revealed the detection of two bands at 32 and 37 kDa which correlate to immature (incompletely processed) forms of tethered GPA2/GPB5 proteins and non-glycosylated GPA2 (13 kDa) plus GPB5 (24 kDa), respectively. In secreted fractions, a faint band corresponding to non-glycosylated GPA2/GPB5 as well as an intense band at 40 kDa, glycosylated GPA2 (16 kDa) plus GPB5 (24 kDa), was detected. Moreover, treatments with PNGase verified the higher molecular weight band size in secreted fractions at 40 kDa is glycosylated, as observed for GPA2 expressed independantly in earlier experiments herein and in previous studies (13). Bands in cell lysates indicate the retention of immature and non-glycosylated GPA2/GPB5 heterodimers. For this reason, cell lysates and secreted protein fractions of tethered GPA2/GPB5-expressing cells were separately tested for their ability to activate A. aegypti LGR1. Unlike secreted fractions that were more rich in glycosylated GPA2/GPB5 proteins, cell lysates that lacked glycosylated GPA2/GPB5 were able to activate LGR1, leading to a reduction in cAMP-mediated luminescence. Thus, our results suggest that post-translational modifications like glycosylation may impact ligand-receptor activity. Alternatively, it is possible that a lack of inhibitory activity of secreted fractions could be owed to smaller concentrations of tethered GPA2/GPB5 compared to lysate fractions, given that the amounts of secreted and cell lysate fractions were not equalized. Future experiments should aim to create novel constructs that improve expression and secretion of tethered GPA2/GPB5.
Evolutionary Conservation and Divergence of GPA2/GPB5 Receptor Signaling
In humans, GPA2/GPB5-TSHR signaling stimulates adenylyl cyclase activity to increase intracellular cAMP via interaction with a Gs protein (4, 6, 11), and these results were confirmed in our studies. Comparatively, GPA2/GPB5 signaling was also shown to increase levels of cAMP upon binding LGR1 in D. melanogaster (29). Interestingly, our experiments demonstrate low level constitutive activity of adenylyl cyclase in LGR1 expressing cells since cAMP luminescent response was moderately greater in LGR1-transfected cells compared to cells not expressing LGR1. High basal constitutive activity of LGR1 has been observed previously in D. melanogaster (29). Moreover, constitutive activity of glycoprotein hormone receptors is well-known in vertebrates and has been demonstrated to be stronger for the thyrotropin receptor than for the LH/CG receptor (41). Given that GPA2/GPB5 receptor homologs in vertebrate and invertebrate model organisms have been shown previsously, and herein, to promote constitutive synthesis of cAMP, it may be suggestive that a common downstream effector and/or function exists for LGR1 and homologs in different organisms.
Surprisingly, however, our experiments indicate that incubations of LGR1-expressing cells with mosquito GPA2/GPB5 tethered protein triggers a switch from low level constitutive Gs coupling to Gi/o coupling for A. aegypti LGR1, given that tethered GPA2/GPB5 signficantly inhibited the forskolin-induced increase in cAMP in LGR1-expressing HEK 293T cells but not in control cells lacking LGR1 expression. This finding, while highly interesting, is not entirely unusual since promiscuous G protein coupling has been reported for glycoprotein hormone receptors like the TSH receptor (Gs and Gq) and LH/CG (Gi and Gs) (42–44).
Regulation by GPA2/GPB5 Heterodimers
To help stabilize heterodimerization, the beta subunit sequences of the classic glycoprotein hormones (FSH, LH, TSH, and CG) contain two additional cysteine residues that form an additional disulfide bridge which wraps around and “buckles” the alpha subunit (26). Though heterodimerization can occur with mutated forms of this “seatbelt” structure, there is a dramatic decrease in heterodimer stability (21, 22). GPB5 in vertebrates and invertebrates lack the seatbelt structure required to stabilize heterodimerization (26). Thus, the hypothesis that GPA2/GPB5 functions as a heterodimer in a physiological situation (i.e., without chemical cross-linking) is challenged. The dissociation constant (Kd) associated with heterodimerization of the classic glycoproteins hormone subunits and GPA2/GPB5 is 10−7 to 10−6 M, which indicates heterodimeric interactions are favored at these concentrations (45, 46). However, since the classic beta subunits contain an additional disulfide bridge that strengthens its association with the common alpha subunit, heterodimeric interactions are stabilized in circulation at physiological concentrations as low as 10−11 to 10−9 M (21). Without this seatbelt structure, GPA2/GPB5 heterodimeric interactions are posssible only at micromolar concentrations, which are not typically observed in circulation (21, 26). Together, the limited evidence so far using heterolougous expression challenges the possibility of endocrine regulation by GPA2/GPB5 heterodimers. Nonetheless, it was argued in D. melanogaster that the large neurosecretory cells co-expressing the glycoprotein hormone subunits, along with their corresponding axonal projections that localized distinctly from organs that express the GPA2/GPB5 receptor (LGR1), did support that this system is indeed endocrine in nature (18). Alternatively, the subunits could function independently or regulate physiology as a heterodimer in a paracrine/autocrine fashion. In rats, GPA2/GPB5 is expressed in oocytes and may act as a paracrine regulator of TSHR-expressed granulosa cells in the ovary to regulate reproductive processes (6). Another possibility to consider is that additional endogenous co-factors may be involved, but remain unidentified, which help to strengthen interaction between the GPA2 and GPB5 subunits, since the tethered mosquito GPA2/GPB5 was indeed capable of activating LGR1 in vitro inducing a Gi/o signaling cascade.
GPA2 and GPB5 Homodimerization
Our results establish that human and mosquito GPA2 and GPB5 subunits can weakly and strongly, respectively, homodimerize. However, whether these homodimers have a physiological function in vivo is unknown. Treatments of either mosquito or human GPA2 and GPB5 subunits alone did not stimulate specific downstream signaling in LGR1- or TSHR-expressing cells, upholding that only GPA2/GPB5 heterodimers can activate their cognate glycoprotein hormone receptors. However, it is possible that GPA2 and GPB5 homodimers may target other unidentified receptors. In insects, the molting hormone bursicon is a heterodimer of two subunits called burs and pburs. Burs/pburs heterodimers act via a glycoprotein hormone receptor (i.e., LGR2) to regulate processes such as tanning and sclerotization of the insect cuticle as well as wing inflation after adult emergence (47). Recently, it was demonstrated that bursicon subunits can homodimerize (i.e., burs/burs and pburs/pburs) and these homodimers mediate actions independently of LGR2 to regulate immune responses in A. aegypti and D. melanogaster (48, 49).
In addition to the human and mosquito GPA2/GPB5 homodimers observed in our studies, human GPA2 was also shown to interact with the beta subunits of CG and FSH (4). Lastly, the expression patterns of GPA2 and GPB5 in a number of organisms do not always strictly co-localize, since GPA2 expression exhibits a much wider distribution and is expressed more abundantly than GPB5 in a number of vertebrate and invertebrate organisms (7, 12, 23, 24, 50, 51). Taken together, this raises the possibility that GPA2 and GPB5 subunits may interact with other unknown proteins that could activate different receptors or signaling pathways and elicit distinct functions.
Concluding Remarks
Although much is known about the classic vertebrate glycoprotein hormones including LH, FSH, TSH, and CG along with their associated receptors, little progress has been made thus far toward better understanding the function of GPA2/GPB5 signaling and subunit interactions, particularly for the invertebrate organisms. To our knowledge, this is the first study to demonstrate A. aegypti and H. sapiens GPA2 and GPB5 subunit homodimerization in vitro. Our results also confirm that heterodimerization of A. aegypti and H. sapiens GPA2/GPB5 are required for the activation of their cognate receptors LGR1 and TSHR, respectively. In constrast to previous reports showing GPA2/GPB5-induced LGR1 activation elevates intracellular cAMP by coupling a Gs pathway, the current findings provide novel information supporting that A. aegypti LGR1 couples to a Gi/o protein to inhibit cAMP levels following application of the GPA2/GPB5 fusion polypeptide. Further, our results revealed that mosquito LGR1 is constitutively active when overexpressed in the absence of its ligand, GPA2/GPB5, inducing a Gs signaling pathway that raises levels of cAMP levels, which is consistent with previous observations with overexpression of fruit fly LGR1 (29, 52) as well as mammals including dog and human TSH receptor (53, 54).
In the mosquito nervous system, our results confirm GPA2 and GPB5 subunits are co-expressed within the same neurosecretory cells of the first five abdominal ganlgia where their coordinated release and regulation are likely. As a result, whether GPA2/GPB5 are secreted as heterodimers, like the classic glycoprotein hormones, and/or as homodimers remains to be determined in vivo. While homodimers were inactive in the heterologous assay used herein, whether these homodimers are functional in vivo and what physiological role they play (if any) is a research direction that should be addressed in future studies. All in all, this investigation has provided novel information for an invertebrate GPA2/GPB5 and LGR1 signaling system and contributes toward advancing our understanding and the functional elucidation of this ancient glycoprotein hormone signaling system common to nearly all bilaterian organisms.
Data Availability Statement
All datasets generated for this study are included in the article/Supplementary Material.
Author Contributions
DR and J-PP contributed to experimental design, wrote the paper, and aided with data analysis. DR performed all the experiments.
Funding
This study was supported by a Natural Sciences and Engineering Research Council of Canada (NSERC) Discovery Grant and an Ontario Ministry of Research and Innovation Early Researcher Award to J-PP. This manuscript has been released as a Pre-Print on BioRxiv available at https://www.biorxiv.org/content/10.1101/694653v2.
Conflict of Interest
The authors declare that the research was conducted in the absence of any commercial or financial relationships that could be construed as a potential conflict of interest.
Supplementary Material
The Supplementary Material for this article can be found online at: https://www.frontiersin.org/articles/10.3389/fendo.2020.00158/full#supplementary-material
References
1. Hartree AS, Renwick AGC. Molecular structures of glycoprotein hormones and functions of their carbohydrate components. Biochem J. (1992) 287(Pt 3):665–79. doi: 10.1042/bj2870665
2. Pierce JG, Parsons TF. Glycoprotein hormones: structure and function. Annu Rev Biochem. (1981) 50:465–95. doi: 10.1146/annurev.bi.50.070181.002341
3. Hsu SY, Nakabayashi K, Bhalla A. Evolution of glycoprotein hormone subunit genes in bilateral metazoa: identification of two novel human glycoprotein hormone subunit family genes, GPA2 and GPB5. Mol Endocrinol. (2002) 16:1538–51. doi: 10.1210/mend.16.7.0871
4. Nakabayashi K, Matsumi H, Bhalla A, Bae J, Mosselman S, Hsu SY, et al. Thyrostimulin, a heterodimer of two new human glycoprotein hormone subunits, activates the thyroid-stimulating hormone receptor. J Clin Investig. (2002) 109:1445–52. doi: 10.1172/JCI0214340
5. Dos Santos S, Mazan S, Venkatesh B, Cohen-Tannoudji J, Quérat B. Emergence and evolution of the glycoprotein hormone and neurotrophin gene families in vertebrates. BMC Evol Biol. (2011) 11:332. doi: 10.1186/1471-2148-11-332
6. Sun SC, Hsu PJ, Wu FJ, Li SH, Lu CH, Luo CW. Thyrostimulin, but not thyroid-stimulating hormone (TSH), acts as a paracrine regulator to activate the TSH receptor in mammalian ovary. J Biol Chem. (2010) 285:3758–65. doi: 10.1074/jbc.M109.066266
7. Okada SL, Ellsworth JL, Durnam DM, Haugen HS, Holloway JL, Kelley ML, et al. A glycoprotein hormone expressed in corticotrophs exhibits unique binding properties on thyroid-stimulating hormone receptor. Mol Endocrinol. (2006) 20:414–25. doi: 10.1210/me.2005-0270
8. Bassett JHD, van der Spek A, Logan JG, Gogakos A, Chakraborty JB, Murphy E, et al. Thyrostimulin regulates osteoblastic bone formation during early skeletal development. Endocrinology. (2015) 159:3098–113. doi: 10.1210/en.2014-1943
9. Suzuki C, Nagasaki H, Okajima Y, Suga H, Ozaki N, Arima H, et al. Inflammatory cytokines regulate glycoprotein subunit beta5 of thyrostimulin through nuclear factor-kappaB. Endocrinology. (2009) 150:2237–43. doi: 10.1210/en.2008-0823
10. van Zeijl CJJ, Surovtseva OV, Kwakkel J, van Beeren HC, Duncan Bassett JH, Williams GR, et al. Thyrostimulin deficiency does not alter peripheral responses to acute inflammation-induced nonthyroidal illness. Am J Physiol Endocrinol Metab. (2014) 307:E527–37. doi: 10.1152/ajpendo.00266.2014
11. Huang W, Li Z, Lin T, Wang S, Wu F, Luo C. Thyrostimulin-TSHR signaling promotes the proliferation of NIH : OVCAR-3 ovarian cancer cells via trans-regulation of the EGFR pathway. Nat Publishing Group. (2016) 6:1–13. doi: 10.1038/srep27471
12. Heyland A, Plachetzki D, Donelly E, Gunaratne D, Bobkova Y, Jacobson J, et al. Distinct expression patterns of glycoprotein hormone subunits in the lophotrochozoan Aplysia: implications for the evolution of neuroendocrine systems in animals. Endocrinology. (2012) 153:5440–51. doi: 10.1210/en.2012-1677
13. Paluzzi J-P, Vanderveken M, O'Donnell MJ. The heterodimeric glycoprotein hormone, GPA2/GPB5, regulates ion transport across the hindgut of the adult mosquito, Aedes aegypti. PLoS ONE. (2014) 9:e86386. doi: 10.1371/journal.pone.0086386
14. Rocco DA, Paluzzi J-PV. Functional role of the heterodimeric glycoprotein hormone, GPA2/GPB5, and its receptor, LGR1: An invertebrate perspective. Gen Comp Endocrinol. (2016) 234:20–7. doi: 10.1016/j.ygcen.2015.12.011
15. Vandersmissen HP, Van Hiel MB, Van Loy T, Vleugels R, Vanden Broeck J. Silencing D. melanogaster lgr1 impairs transition from larval to pupal stage. Gen Comp Endocrinol. (2014) 209:135–47. doi: 10.1016/j.ygcen.2014.08.006
16. Jourjine N, Mullaney BC, Mann K, Scott K. Coupled sensing of hunger and thirst signals balances sugar and water consumption. Cell. (2016) 166:855–66. doi: 10.1016/j.cell.2016.06.046
17. Rocco DA, Kim DH, Paluzzi JPV. Immunohistochemical mapping and transcript expression of the GPA2/GPB5 receptor in tissues of the adult mosquito, Aedes aegypti. Cell Tissue Res. (2017) 369:313–30. doi: 10.1007/s00441-017-2610-3
18. Sellami A, Agricola HJ, Veenstra JA. Neuroendocrine cells in Drosophila melanogaster producing GPA2/GPB5, a hormone with homology to LH, FSH and TSH. Gen Comp Endocrinol. (2011) 170:582–8. doi: 10.1016/j.ygcen.2010.11.015
19. Rocco DA, Garcia ASG, Scudeler EL, Santos DC, Nóbrega RH, Paluzzi JPV. Glycoprotein hormone receptor knockdown leads to reduced reproductive success in male Aedes aegypti. Front Physiol. (2019) 10:266. doi: 10.3389/fphys.2019.00266
20. Combarnous Y. Molecular basis of the specificity of binding of glycoprotein hormones to their receptors. Endocr Rev. (1992) 13:670–91. doi: 10.1210/edrv-13-4-670
21. Galet C, Lecompte F, Combarnous Y. Association/dissociation of gonadotropin subunits involves disulfide bridge disruption which is influenced by carbohydrate moiety. Biochem Biophys Res Commun. (2004) 324:868–73. doi: 10.1016/j.bbrc.2004.09.143
22. Xing Y, Myers R, Cao D, Lin W, Jiang M, Bernard M, et al. Glycoprotein hormone assembly in the endoplasmic reticulum: II. Multiple roles of a redox sensitive (beta)-subunit disulfide switch. J Biol Chem. (2004) 279:35437–48. doi: 10.1074/jbc.M403053200
23. Dos Santos S, Bardet C, Bertrand S, Escriva H, Habert D, Querat B. Distinct expression patterns of glycoprotein hormone-alpha2 and -beta5 in a basal chordate suggest independent developmental functions. Endocrinology. (2009) 150:3815–22. doi: 10.1210/en.2008-1743
24. Nagasaki H, Wang Z, Jackson VR, Lin S, Nothacker HP, Civelli O. Differential expression of the thyrostimulin subunits, glycoprotein α2 and β5 in the rat pituitary. J Mol Endocrinol. (2006) 37:39–50. doi: 10.1677/jme.1.01932
25. Tando Y, Kubokawa K. A homolog of the vertebrate thyrostimulin glycoprotein hormone alpha subunit (GPA2) is expressed in Amphioxus neurons. Zoological Sci. (2009) 26:409–14. doi: 10.2108/zsj.26.409
26. Alvarez E, Cahoreau C, Combarnous Y. Comparative structure analyses of cystine knot-containing molecules with eight aminoacyl ring including glycoprotein hormones (GPH) alpha and beta subunits and GPH-related A2 (GPA2) and B5 (GPB5) molecules. Reprod Biol Endocrinol. (2009) 7:90. doi: 10.1186/1477-7827-7-90
27. Krause G, Kreuchwig A, Kleinau G. Extended and structurally supported insights into extracellular hormone binding, signal transduction and organization of the thyrotropin receptor. PLoS ONE. (2012) 7:e52920. doi: 10.1371/journal.pone.0052920
28. Hauser F, Nothacker HP, Grimmelikhuijzen CJ. Molecular cloning, genomic organization, and developmental regulation of a novel receptor from Drosophila melanogaster structurally related to members of the thyroid-stimulating hormone, follicle-stimulating hormone, luteinizing hormone/choriogonadotropin. J Biol Chem. (1997) 272:1002–10. doi: 10.1074/jbc.272.2.1002
29. Sudo S, Kuwabara Y, Park J, Il Sheau YH, Hsueh AJW. Heterodimeric fly glycoprotein hormone-α2 (GPA2) and glycoprotein hormone-β5 (GPB5) activate fly leucine-rich repeat-containing G protein-coupled receptor-1 (DLGR1) and stimulation of human thyrotropin receptors by chimeric fly GPA2 and human GPB5. Endocrinology. (2005) 146:3596–604. doi: 10.1210/en.2005-0317
30. Paluzzi JP, Russell WK, Nachman RJ, Orchard I. Isolation, cloning, and expression mapping of a gene encoding an antidiuretic hormone and other CAPA-related peptides in the disease vector, Rhodnius prolixus. Endocrinology. (2008) 149:4638–46. doi: 10.1210/en.2008-0353
31. Sower SA, Decatur WA, Hausken KN, Marquis TJ, Barton SL, Gargan J, et al. Emergence of an ancestral glycoprotein hormone in the pituitary of the Sea Lamprey, a Basal Vertebrate. Endocrinology. (2015) 156:3026–37. doi: 10.1210/en.2014-1797
32. Wang P, Liu S, Yang Q, Liu Z, Zhang S. Functional characterization of thyrostimulin in Amphioxus suggests an ancestral origin of the TH signaling pathway. Endocrinology. (2018) 159:3536–48. doi: 10.1210/en.2018-00550
33. Sgourakis NG, Bagos PG, Papasaikas PK, Hamodrakas SJ. A method for the prediction of GPCRs coupling specificity to G-proteins using refined profile Hidden Markov Models. BMC Bioinformatics. (2005) 12:1–12. doi: 10.1186/1471-2105-6-104
34. Brown MR, Cao C. Distribution of ovary ecdysteroidogenic hormone I in the nervous system and gut of mosquitoes. J Insect Sci. (2001) 1:1–11. doi: 10.1673/031.001.0301
35. Atwood B, Lopez J, Wager-Miller J, Mackie K, Straiker A. Expression of G protein-coupled receptors and related proteins in HEK293, AtT20, BV2, and N18 cell lines as revealed by microarray analysis. BMC Genomics. (2011) 12:14. doi: 10.1186/1471-2164-12-14
36. Priyanka V, Chichili R, Kumar V, Sivaraman J. Linkers in the structural biology of protein – protein interactions. Protein Sci. (2013) 22:153–167. doi: 10.1002/pro.2206
37. Fares F, Suganuma N, Nishimori K, LaPolt P, Hsueh A, Biome I. Design of a long-acting follitropin agonist by fusing the C-terminal sequence of the chorionic gonadotropin β subunit to the follitropin β subunit. Proc Natl Acad Sci USA. (1992) 89:4304–8. doi: 10.1073/pnas.89.10.4304
38. Joshi L, Murata Y, Wondisford F, Szkudlinski M, Desai R, Weintraub B. Recombinant thyrotropin containing a β-subunit chimera with the human chorionic gonadotropin-β carboxy terminal is biologically active with a prolonged plasma half-life: role of carbohydrate in bioactivity and metabolic clearance. Endocrinology. (1995) 136:3839–948. doi: 10.1210/endo.136.9.7544273
39. Furuhashi M, Shikone T, Fares F, Sugahara T, Hsueh A, Boime I. Fusing the carboxy-terminal peptide of the chorionic gonadotropin (CG) β-subunit to the common α-subunit: retention of O-linked glycosylation and enhanced in vivo bioactivity of chimeric human CG. Mol Endocrinol. (1995) 9:54–63. doi: 10.1210/mend.9.1.7539107
40. Azzam N, Bar-Shalom R, Fares F. Conversion of TSH heterodimer to a single polypeptide chain increases bioactivity and longevity. Endocrinology. (2012) 153:954–60. doi: 10.1210/en.2011-1856
41. Cetani F, Tonacchera M, Vassart G. Differential effects of NaCl concentration on the constitutive activity of the thyrotropin and the luteinizing hormone/chorionic gonadotropin receptors. FEBS Lett. (1996) 378:27–31. doi: 10.1016/0014-5793(95)01384-9
42. Grasberger H, Van Sande J, Hag-Dahood M, Tenenbaum-Rakover Y, Refetoff S. A familial thyrotropin (TSH) receptor mutation provides in vivo evidence that the inositol phosphates/Ca2+ cascade mediates TSH action on thyroid hormone synthesis. J Clin Endocrinol Metab. (2007) 92:2816–20. doi: 10.1210/jc.2007-0366
43. Herrlich A, Kuhn B, Grosse R, Schmid A, Schultz G, Gudermann T. Involvement of Gs and Gi proteins in dual coupling of the luteinizing hormone receptor to adenylyl cyclase and phospholipase C. J Biol Chem. (1996) 271:16764–72. doi: 10.1074/jbc.271.28.16764
44. Kleinau G, Jaeschke H, Worth C, Mueller S, Gonzalez J, Paschke R, et al. Principles and determinants of G-protein coupling by the rhodopsin-like thyrotropin receptor. PLoS ONE. (2010) 5:e9745. doi: 10.1371/journal.pone.0009745
45. Ingham K, Weintraub B, Edelhock H. Kinetics of recombination of the subunits of human chorionic gonadotropin. Effect of subunit concentration. Biochemistry. (1976) 15:1720–6. doi: 10.1021/bi00653a020
46. Strickland TW, Puett D. The kinetic and equilibrium parameters of subunit association and gonadotropin dissociation. J Biol Chem. (1982) 257:2954–60.
47. Luo C-W, Dewey EM, Sudo S, Ewer J, Hsu SY, Honegger H-W, et al. Bursicon, the insect cuticle-hardening hormone, is a heterodimeric cystine knot protein that activates G protein-coupled receptor LGR2. Proc Natl Acad Sci USA. (2005) 102:2820–5. doi: 10.1073/pnas.0409916102
48. An S, Dong S, Wang Q, Li S, Gilbert LI, Stanley D, et al. Insect neuropeptide bursicon homodimers induce innate immune and stress genes during molting by activating the NF-κB transcription factor relish. PLoS ONE. (2012) 7:e34510. doi: 10.1371/journal.pone.0034510
49. Zhang H, Dong S, Xi C, Stanley D, Beerntsen B. Relish2 mediates bursicon homodimer-induced prophylactic immunity in the mosquito Aedes aegypti. Sci Rep. (2017) 7:43163. doi: 10.1038/srep43163
50. Tando Y, Kubokawa K. Expression of the gene for ancestral glycoprotein hormone β subunit in the nerve cord of Amphioxus. Gen Comp Endocrinol. (2009) 162:329–39. doi: 10.1016/j.ygcen.2009.04.015
51. Trudeau VL. Really old hormones up to new tricks: glycoprotein hormone subunits may have roles in development. Endocrinology. (2009) 150:3446–7. doi: 10.1210/en.2009-0465
52. Nishi S, Hsu SY, Zell K, Hsueh AJ. Characterization of two fly LGR (leucine-rich repeat-containing, G protein-coupled receptor) proteins homologous to vertebrate glycoprotein hormone receptors. Endocrinology. (2000) 141:4081–90. doi: 10.1210/endo.141.11.7744
53. Sugawa ZML, Kosugi S, Mori T. Constitutive activation of the thyrotropin receptor by deletion of a portion of the extracellular domain. Biochem Biophys Res Commun. (1995) 211:205–10. doi: 10.1006/bbrc.1995.1797
54. Van Sande J, Swillens S, Gerard C, Allgeier A, Massart C, Vassart G, et al. In Chinese hamster ovary K1 cells dog and human thyrotropin receptors activate both the cyclic AMP and the phosphatidylinositol 4,5-bisphosphate cascades in the presence of thyrotropin and the cyclic AMP cascade in its absence. Eur J Biochem. (1995) 229:338–43. doi: 10.1111/j.1432-1033.1995.0338k.x
Keywords: GPA2/GPB5, mosquito, glycoprotein hormone, leucine-rich repeat-containing G protein coupled-receptor 1, homodimer, heterodimer, thyrostimulin
Citation: Rocco DA and Paluzzi J-PV (2020) Expression Profiling, Downstream Signaling, and Inter-subunit Interactions of GPA2/GPB5 in the Adult Mosquito Aedes aegypti. Front. Endocrinol. 11:158. doi: 10.3389/fendo.2020.00158
Received: 18 December 2019; Accepted: 06 March 2020;
Published: 31 March 2020.
Edited by:
Elizabeth Amy Williams, University of Exeter, United KingdomReviewed by:
Frank Hauser, University of Copenhagen, DenmarkMark R. Brown, University of Georgia, United States
Copyright © 2020 Rocco and Paluzzi. This is an open-access article distributed under the terms of the Creative Commons Attribution License (CC BY). The use, distribution or reproduction in other forums is permitted, provided the original author(s) and the copyright owner(s) are credited and that the original publication in this journal is cited, in accordance with accepted academic practice. No use, distribution or reproduction is permitted which does not comply with these terms.
*Correspondence: David A. Rocco, ZGF2cm9jY29AeW9ya3UuY2E=; Jean-Paul V. Paluzzi, cGFsdXp6aUB5b3JrdS5jYQ==
†ORCID: David A. Rocco orcid.org/0000-0001-9545-7713
Jean-Paul V. Paluzzi orcid.org/0000-0002-7761-0590