- 1National Clinical Research Center for Metabolic Diseases, Department of Metabolism and Endocrinology, The Second Xiangya Hospital, Central South University, Changsha, China
- 2Department of Endocrinology, Xuhui District Central Hospital, Shanghai, China
- 3Department of Anorectal Surgery, Shuguang Hospital, Shanghai University of Traditional Chinese Medicine, Shanghai, China
- 4Anorectal Disease Institute of Shuguang Hospital, Shanghai, China
- 5State Key Laboratory of Pharmaceutical Biotechnology, The University of Hong Kong, Hong Kong, China
- 6Department of Medicine, The University of Hong Kong, Hong Kong, China
- 7Department of Pharmacology and Pharmacy, The University of Hong Kong, Hong Kong, China
- 8Research Center of Heart, Brain, Hormone, and Healthy Aging, The University of Hong Kong, Hong Kong, China
Objective: Fibroblast growth factor 19 (FGF19) plays an indispensable role in regulating bile acid, glucose, and lipid metabolism, and alterations of its circulating concentration is associated with the development of type 2 diabetes (T2D). Atherosclerosis is directly related to the death-deriving diabetic macroangiopathy in T2D, yet relationships between FGF19 and atherosclerosis in T2D remain unclear. The aim of this study was to investigate the association of circulating FGF19 levels with the development of subclinical atherosclerosis (subAS) in patients with T2D in a 3-year prospective study.
Methods: In the present study, 153 newly diagnosed T2D patients without subAS were recruited at baseline, and 137 of them completed a 3-year follow-up. FGF19 levels were measured in fasting serum samples collected at baseline and the third-year visits. Carotid, femoral, and iliac intima-media thickness (IMT) were detected by high-resolution B-mode ultrasound to determine the presence of subAS. Logistic regression analysis was applied to assess the relationship between serum FGF19 and subAS in patients with T2D.
Results: At baseline, serum FGF19 levels were positively correlated with carotid IMT and iliac IMT in men (r = 0.239, P = 0.036; r = 0.309, P = 0.006). At the 3-year follow-up, 25 out of 153 patients developed subAS, and FGF19 levels in men were higher in the subAS group than in the non-subAS group [202.7 (177.9–373.6) vs. 133.4 (85.6–171.3) pg/ml, P = 0.028]. Furthermore, in men, higher baseline levels of FGF19 were independently associated with a greater risk of subAS at year 3 in patients with T2D with an odds ratio (OR) of 4.798 per 1 standard deviation (SD) of the FGF19 concentration [OR = 4.798 (95% CI, 1.680–13.706), P = 0.003]. Baseline FGF19 levels yielded an area under the receiver operating characteristic curve of 0.769 to predict the development of subAS at year 3 in men with T2D.
Conclusions: Serum FGF19 levels could help in predicting the development of atherosclerosis in men with T2D.
Introduction
Diabetes mellitus is currently burdening approximately 463 million people worldwide with booming healthcare cost and plummeting disability adjusted life year (1–3). Among them, nearly 90% were with type 2 diabetes (T2D) (1). Diabetic macroangiopathy, which is characterized by atherosclerosis, is the most severe diabetic complication, which makes major contributions to diabetic mortality and morbidity (4). Atherosclerosis is the reformation of blood vessels through the accumulation of lipids and redox signaling induction of factors in the arterial wall, which could result in rupture and stenosis in arteries and subsequent death-deriving processes (5). Subclinical atherosclerosis (subAS) is an early indicator of the development of atherosclerosis, which could be assessed by increased intima-media thickness (IMT) of arteries. This early identification could be conducive to early intervention and treatment, thus preventing further development of cardiovascular disease (6, 7).
Fibroblast growth factor 19 (FGF19) is a circulating hormone that actively participates in governing bile acid synthesis, glycogenesis, and lipid metabolism (8, 9). Given these functions, FGF19 is a potential molecular in investigating human chronic disease, including T2D and atherosclerosis, in which glucose and lipid metabolism play a vital role (9, 10). In this regard, a recent study conducted on C57BL/6 and FGF19-knockout mice found that the expression of FGF19 could inhibit the absorption of cholesterol in the liver (11).
In human, scientists have revealed a negative correlation between FGF19 and cardiovascular risk factors (12) and found FGF19 as an independent factor of the development of coronary artery disease (13). The concentration of FGF19 was negatively correlated with the concentration of triglycerides (TG) and the plasma atherosclerosis index but was positively correlated to high-density lipoprotein cholesterol (HDL-c), which proved the vital importance of FGF19 in lipid metabolism and atherosclerosis (12–14). Based on these results, FGF19 analogs were applied in clinical trials to evaluate its potential in modulating lipid metabolism, and results revealed increased serum cholesterol, high-density lipoprotein cholesterol (HDL-C), low-density lipoprotein cholesterol (LDL-C), and reduced TG levels in human participants (15). However, the relationship between FGF19 and diabetic macroangiopathy has not been demonstrated extensively. In this study, we investigated serum concentration of FGF19 and early atherosclerosis events in human T2D patients to assess whether serum FGF19 could be applied in predicting atherosclerosis in T2D.
Methods
Participants
Participants were recruited in the Chinese National Tenth and Eleventh Five Tackling Key Project from 2002 to 2007 at the Second Xiangya Hospital of Central South University. In this prospective study, 153 newly diagnosed T2D patients were defined by the World Health Organization (WHO) and American Diabetes Association (ADA) criteria (16, 17). Patients with (1) other types of diabetes (as defined by the ADA classification), (2) overt cardiovascular and cerebrovascular diseases (including angina, myocardial infarction, stroke, peripheral vascular disease), (3) severe liver and kidney dysfunction, (4) tumors, and (5) severe trauma or surgery were excluded. The diagnosis of dyslipidemia was based on the 2001 American National Cholesterol Education Program Adult Treatment Panel III (NECP-ATPIII) standard (18). Hypertension was diagnosed when systolic blood pressure (SBP) ≥140 or diastolic blood pressure (DBP) ≥90 mmHg, or if patients were taking antihypertensive medications. Smoking referred to both current and past smokers. Alcohol consumption referred to both current and past alcohol consumption. Metformin was prescribed for patients with a body mass index (BMI) of 25 kg/m2 or above, and glipizide was recommended for patients with a BMI <25 kg/m2 (19). This study was carried out in accordance with the Helsinki Declaration of the World Medical Association and approved by the Ethics Committee of the Second Xiangya Hospital of Central South University. Written informed consent was obtained from each subject upon recruitment.
Clinical and Biochemical Assessments
Age, sex, and medical history of each patient were recorded, and their height, weight, and waist and hip circumference were measured with a standard procedure. Subjects were asked to undertake 8 h fasting before the visit in early morning, and venous blood was collected. Separated serum was used to detect biochemical parameters, and the remaining specimens were stored at −80°C for FGF19 detection. Plasma glucose, serum cholesterol, TG, HDL-C, LDL-C, and high-sensitivity C-reactive protein (hsCRP) were measured on a Hitachi 7170 analyzer (Boehringer Mannheim, Mannheim, Germany). Serum insulin was measured by Bayer 180SE's automated chemiluminescence system (Bayer AG, Leverkusen, Germany) (20). Insulin resistance (IR) was assessed using the homeostasis model assessment of insulin resistance (HOMA-IR). The plasma atherosclerosis index (AIP) was calculated as log (triglyceride/high-density lipoprotein cholesterol) (13). Serum FGF19 were measured with an enzyme-linked immunosorbent assay (ELISA) kit (The University of Hong Kong Antibody and Immunoassay Service), and the intra- and interassay precision met the manufacturer's instructions (19).
Intima-Media Thickness Assessment
Doppler vascular ultrasound examination was performed annually on all patients with the Doppler ultrasound system (128XP/10 system; Acuson, Mountain View, CA, USA) (21). The IMT of the patient's carotid, femoral, and iliac arteries were measured, and intra- and interassay coefficients of variance were 3.9–4.2% and 5.0–6.0%, respectively. Patients with one or more arteries with an IMT >1.0 mm or plaque formation without clinical manifestations were considered with subAS (22, 23).
Statistical Analysis
Statistical analyses were performed with SPSS 25.0 software and GraphPad Prism 8.0 software. Normally distributed data determined by Kolmogorov–Smirnov test were presented as mean ± standard deviation (SD). Non-normally distributed data were presented as median and interquartile range (IQR). Comparisons between groups were performed using χ2 tests for categorical variables. Unpaired Student's t-tests and non-parametric tests were used to compare differences in normally distributed and non-normally distributed data between two groups. Spearman correlation analysis was performed to analyze the correlation between serum FGF19 levels and other variables. The values of serum FGF19 were standardized, and logistic regression analysis was applied to investigate the association between the change in serum FGF19 level per 1 standard deviation (SD) and subAS in men with T2D. The area under the curve (AUC) of baseline FGF19 levels or FGF19 levels at year 3 was calculated to evaluate the predictive value or the diagnostic performance by receiver operating characteristic curve (ROC) analysis. Two-sided P < 0.05 was considered statistically significant.
Results
Baseline characteristics of a total of 153 patients with T2D (77 men and 76 women) are summarized in Table 1. No significant differences were shown in age, BMI, fasting blood glucose (FBG), 2-h plasma glucose (2hPG), LDL-c, total cholesterol (TC), blood urea nitrogen (BUN), fasting insulin (FINS), SBP, DBP, HOMA-IR, femoral IMT, and iliac IMT between men and women (P > 0.05). Hemoglobin A1c (HbA1c) of all participants was 7.6 (6.0–9.4)%, suggesting overall hyperglycemia. Compared with women, men were with higher waist/hip ratio (WHR), HbA1c, alanine transaminase (ALT), total bilirubin (TBIL), TG, 24-h urine microalbumin (24hUALB), AIP, percentage of smoking, percentage of alcohol consumption, and carotid IMT, but lower HDL-c levels. No statistically significant differences in baseline serum FGF19 levels between men and women were observed [131.4 (76.4–193.5) vs. 150.4 (90.6–280.2) pg/ml, P = 0.61]. However, men had greater carotid IMT than women.
Correlations between baseline serum FGF19 levels and baseline characteristics are shown in Table 2. Among all participants, serum levels of FGF19 were negatively correlated with body weight, FINS, and HOMA-IR (r = −0.181, P = 0.026; r = −0.189, P = 0.020; r = −0.163, P = 0.045) but positively correlated with iliac IMT (r = 0.208, P = 0.010). We then assessed these associations based on a sex-specific manner. In women, we only found the FGF19 level to be positively correlated with WHR (r = 0.248, P = 0.032). In men, FGF19 levels were negatively correlated with BMI, FINS, and HOMA-IR (r = −0.251, P = 0.022; r = −0.388, P = 0.001, r = −0.353, P = 0.002). In addition, we found significant correlations between serum FGF19 levels and carotid IMT, iliac IMT in men (r = 0.239, P = 0.036; r = 0.309, P = 0.006; Figure 1). Partial correlation analysis adjusted for age and BMI showed that positive correlations between FGF19 and IMT were still statistically significant (r = 0.313, P = 0.006; r = 0.285, P = 0.013).
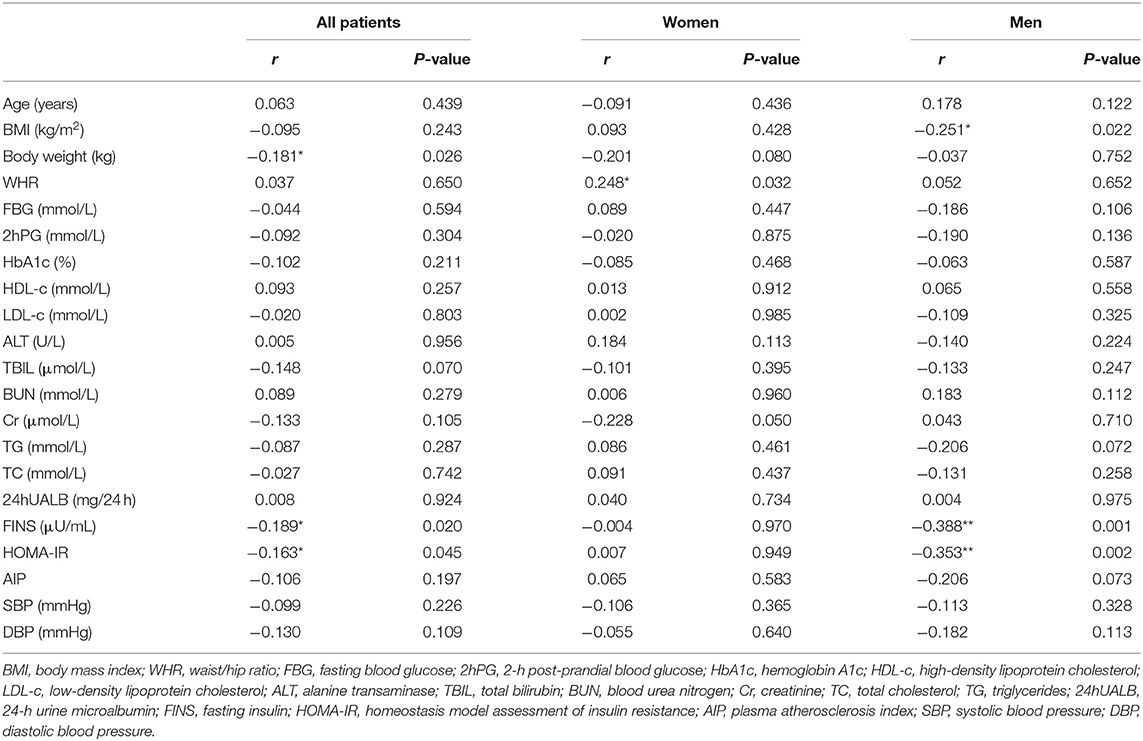
Table 2. Spearman correlations between serum FGF19 levels and various physiological and clinical indicators.
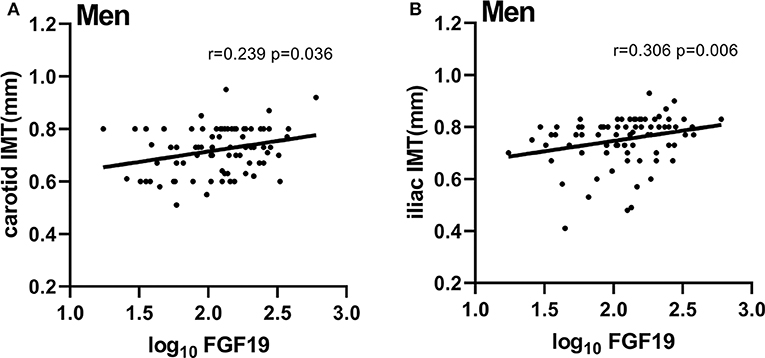
Figure 1. Serum FGF19 levels were positively correlated with carotid IMT and iliac IMT in men. (A) Correlation of FGF19 and carotid IMT in men, carotid IMT = 0.07958 × log10FGF19 + 0.5549. (B) Correlation of FGF19 and iliac IMT in men, iliac IMT = 0.07939 × log10FGF19 + 0.5873. FGF19, fibroblast growth factor 19; carotid IMT, carotid intima-media thickness; iliac IMT, iliac intima-media thickness; log10 FGF19, log10 transformed FGF19 levels.
Of the 153 patients, none were lost during the first year of follow-up, 4 were lost to follow-up in the second year, and 12 were lost to follow-up in the third year. The main reasons for their loss of follow-up were changes in patients' mobile numbers, changes in medication, lack of consent, removal, or death. There was no statistically significant difference in baseline data between patients who were lost during the follow-up and those who were not, except that the patients who were lost to follow-up were younger and had lower serum Cr (Supplementary Table 2). However, all participants had no serious kidney disease. We then assessed this difference based on a sex-specific manner, and the results were consistent with those of all participants. Twenty-five of the 153 (16.3%) participants developed subAS at the end of the 3-year follow-up (Supplementary Table 1).
In men, in order to investigate the association between serum FGF19 levels and the development of subAS, unadjusted and multivariable adjusted odds ratios (ORs) for per 1-SD increase in baseline serum FGF19 level are presented in Table 3. Multiple logistic regression analysis was conducted with the diagnosis of subAS at year 3 as the dependent variable and per 1-SD increase in baseline serum FGF19 level, age, BMI, HbA1c, smoking, alcohol consumption, presence of hypertension, and presence of dyslipidemia as independent variables. Per 1-SD increase in serum FGF19 level was significantly associated with a 4.798-fold increased risk of subAS in men with T2D (Table 3). Furthermore, per 1-SD increase in serum FGF19 level at year 3 was significantly associated with a 2.987-fold increased risk of subAS in men with T2D (Supplementary Table 3).
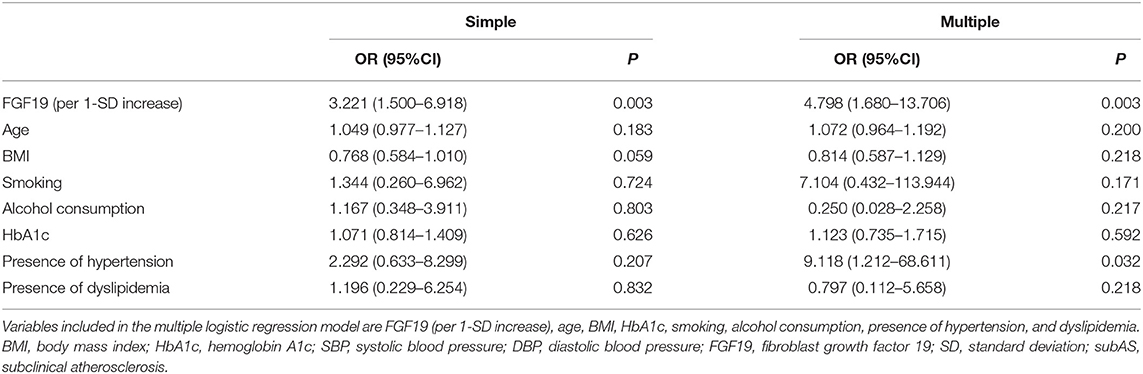
Table 3. Logistic regression analysis showing baseline FGF19 concentrations independently associated with the subAS at year 3 in men.
By the third year, serum FGF19 levels of all participants were 171.2 (101.6–250.4) pg/ml. Among all participants, there was no statistical difference in FGF19 levels between subjects with subAS and subjects without subAS [187.6 (120.8–309.5) vs. 166.3 (98.9–244.9) pg/ml, P > 0.05]. In men, FGF19 levels at year 3 were higher in the subAS group than in the non-subAS group [202.7 (177.9–373.6) vs. 133.4 (85.6–171.3) pg/ml, P = 0.028; Figure 2]. Baseline serum FGF19 levels in men who developed subAS by year 3 were higher than those who did not [215.6 (148.9–297.6) vs. 133.4 (68.9–165.6) pg/ml, P = 0.003]. We further divided patients who developed subAS into two groups with the cutoff value of BMI at 25 kg/m2. No significant difference in the development of subAS was observed between groups of patients with BMI higher or lower than 25 kg/m2 (P > 0.05).
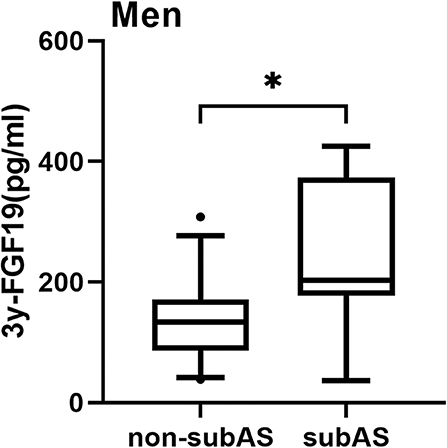
Figure 2. Box-and-whisker plot showed a comparison of serum levels of FGF19 at year 3 in the non-subAS group and subAS group in men. In this figure, the horizontal lines inside the boxes represent the median; the upper and lower horizontal boundaries of the boxes represent the 25th and 75th percentiles, respectively, and the upper and lower horizontal lines outside the boxes represent the 95th and 5th percentiles, respectively. *P < 0.05.
In men, baseline FGF19 levels attained a good predictive value for the development of subAS, with an area under the receiver operating characteristic curve (AUC) of 0.769 (Youden index = 0.501, cutoff value = 156.18 pg/ml, sensitivity = 77%, specificity = 73%; Figure 3). In addition, using the FGF19 levels at year 3 yielded an AUC of 0.770 (Youden index = 0.643, cutoff value = 174.66 pg/ml, sensitivity = 86%, specificity = 79%; Supplementary Figure 1).
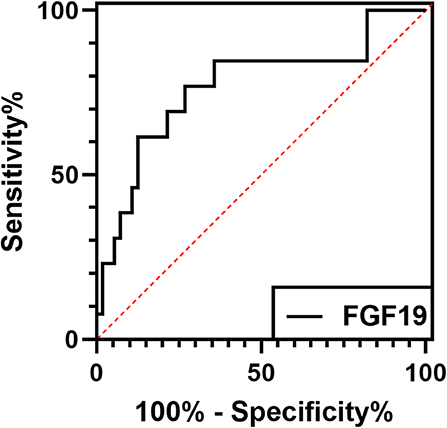
Figure 3. The receiver operating characteristic (ROC) curve showed that FGF19 had a high predictive value of subAS in men. The serum FGF19 levels in the baseline group had an area under the curve (AUC) of 0.769 in men.
Discussion
In our study, we found that serum levels of FGF19 were positively correlated with carotid IMT and iliac IMT in men. Serum FGF19 levels were higher in men who developed subAS than those who did not. High serum FGF19 levels were associated with an increased risk of subAS in men with T2D.
Patients with T2D are characterized by high blood glucose and insulin resistance as well as accompanied with obesity and dyslipidemia (24, 25). Long-term complications, especially those resulted from diabetic macroangiopathy, are intimately associated with dyslipidemia and atherosclerosis and are death-deriving events during the T2D natural history (4). These make unraveling mechanisms involved in metabolism helpful in understanding diabetic macroangiopathy and ultimately improving the prognosis of T2D. FGF19, a hormone mainly secreted by the ileum, plays a role in the bile acid cycle, regulating lipid, and glucose metabolism (26–28). Previous studies have demonstrated the critical link between these metabolic processes and human chronic diseases including T2D and atherosclerosis and suggested FGF19 as an independent factor for T2D and coronary artery disease (13, 29). Nevertheless, how FGF19 functions in atherosclerosis during the development of T2D is still unclear.
Previous studies found that patients with coronary artery disease had lower levels of FGF19 compared to healthy controls, and FGF19 may be a protective factor for severe coronary artery disease (13). Wong et al. found that FGF19 is related to the occurrence of major cardiovascular adverse events (30). Our results revealed that FGF19 was an early predictor for atherosclerosis in men. One possible explanation is the effect of FGF19 on reducing triglycerides. Dyslipidemia is a significant risk factor for atherosclerosis, and increased TG is an independent factor for atherosclerosis (14). FGF19 can reduce TG by increasing fatty acid oxidation (31). Another possible explanation is that patients with cardiovascular disease have reduced hepatic responsiveness to FGF19, leading to a compensatory increase in serum FGF19 levels, which is consistent with an increased risk of cardiovascular disease (30, 32). In addition, no correlation was detected between FGF19 and femoral IMT in this study. The reason may be that the hemodynamic environment of the arteries in different locations is different, which causes the wall shear stress, hydrostatic pressure, and periodic strains in different parts of the body to be different. Therefore, atherosclerosis formation in each artery is not consistent (33).
Although FGF19 plays an important role in bile acid synthesis in the liver, the molecular mechanism of FGF19 cross-talking with the cholesterol metabolism pathway is not fully understood (8, 26). Most of the cholesterol catabolism in the human body is converted into bile acid, which is a ligand for farnesoid X receptor (FXR) (34). FXR can induce the production of FGF19 (35). Previous studies have shown that FGF15 (FGF19 in human) can inhibit the expression of hepatic paraoxonase-1 (PON-1) in mice (36) and that human liver cancer cells treated with recombinant human FGF19 can inhibit the expression of PON-1 (37). PON-1 is an HDL-related hydrolase (38). Recent studies have found that PON-1 can prevent atherosclerosis through direct and indirect approaches (38–40). Therefore, FGF19 may promote atherosclerosis by inhibiting the protective effect of PON-1. In this study, it was found that FGF19 was an independent factor for the development of subAS, and more research is needed to clarify its specific mechanism. The range of atherosclerotic lesions in the aorta of ApoE−/− mice with dyslipidemia was significantly reduced in the treatment with NGM282 (the analog of FGF19) (15). FGF19 can increase liver expression of ATP binding cassette transporter G5 (ABCG5) and ATP binding cassette transporter G8 (ABCG8), which affect cholesterol transport, to reduce the accumulation of liver cholesterol (41). In addition, FGF19 can be induced by FXR to inhibit bile acid production (35). Previous studies have reported that bile acids can promote the adhesion of monocytes and endothelium (42) and can directly affect the immune regulation of macrophages and the proliferation and migration of smooth muscle cells on the vessel wall, which is the key to the development of atherosclerotic diseases (15, 43, 44). A hepatic FGF15/19-Src-FXR phosphorylation signal cascade pathway, which was recently identified by Byun et al., has been proven to regulate cholesterol homeostasis and reduce the risk of atherosclerosis (45). We speculate that the elevated FGF19 levels in subclinical atherosclerotic patients may be secondary compensatory responses compared to non-subclinical atherosclerotic patients.
In the study, we found that serum FGF19 levels were only associated with subAS in men but not in women. It could be directly related to the sex-specific, in this condition male-specific, changes made by FGF19 on atherosclerosis. Women have smaller arterial diameters and fewer plaques (46, 47). Men have more atherosclerotic risk factors than women, such as smoking and alcohol, which is in line with our findings (46). The incidence of atherosclerosis is higher in men than in women at the same age (48), and gender differences could be attributed to differences in sex hormones between men and women (49, 50). Compared to men, women catabolize less cholesterol through bile acid synthesis (51). Estrogen can affect the synthesis of bile acids by affecting the activity of cytochrome P450 family 7 subfamily A member 1 (CYP7A1), and the concentration of chenodeoxycholic acid (CDCA) in bile acids of women is reduced, which may affect the promotion of bile acids in the intestinal tract to the expression of FGF19 (51). Further research is needed to investigate the mechanisms by which estrogen and androgen affect bile acid synthesis.
Our study has several limitations. First, the sample size of the study is relatively small. Indeed, with an alpha error of 5%, the calculated power for our study was <80% to detect the correlation between FGF19 and IMT in women, which may have limited the power to detect the significance between FGF19 and subAS in women. Thus, the predictive role of FGF19 for subAS remains to be validated by larger-scale studies, especially in women. Second, due to a limited follow-up period, the incidence of subAS is low to a certain extent. Third, although our data might suggest a causal relationship between circulating FGF19 levels and the development of subAS, further basic studies are warranted to examine the role of FGF19 in the pathogenesis of atherosclerosis. Fourth, the absence of normal control subjects without T2D in this study may prevent the results from being directly applicable to the general population.
Conclusion
In conclusion, we have demonstrated that high circulating FGF19 levels predicted the development of subclinical atherosclerosis in men with type 2 diabetes in this Chinese diabetes cohort. The significance of these exciting data on a new biomarker for the subAS remains to be confirmed by larger long-term follow-up studies involving other ethnic populations and correlations with other cardiovascular end points.
Data Availability Statement
The datasets generated for this study are available on request to the corresponding author.
Ethics Statement
The studies involving human participants were reviewed and approved by Ethics Committee of the Second Xiangya Hospital of Central South University. The patients/participants provided their written informed consent to participate in this study.
Author Contributions
JH and ZL performed the experiments and analyzed the data. JH wrote the manuscript. YT edited the manuscript. ZM helped with the statistical analysis. AX and PZ provided the ELISA kits. XC and WT collected the samples. YX and ZZ designed the study and revised the manuscript. All authors were involved in the interpretation of data, and approved the final version of the manuscript.
Conflict of Interest
The authors declare that the research was conducted in the absence of any commercial or financial relationships that could be construed as a potential conflict of interest.
Acknowledgments
This work was supported by the National Natural Science Foundation of China (NSFC) grant (81870577, 81670772 to YX) and Science and Technology Major Project of Hunan Province (2017SK1020 to ZZ).
Supplementary Material
The Supplementary Material for this article can be found online at: https://www.frontiersin.org/articles/10.3389/fendo.2020.00282/full#supplementary-material
References
1. Saeedi P, Petersohn I, Salpea P, Malanda B, Karuranga S, Unwin N, et al. Global and regional diabetes prevalence estimates for 2019 and projections for 2030 and 2045: Results from the International Diabetes Federation Diabetes Atlas, 9th edition. Diabetes Res Clin Pract. (2019) 157:107843. doi: 10.1016/j.diabres.2019.107843
2. Li X, Xu Z, Ji L, Guo L, Liu J, Feng K, et al. Direct medical costs for patients with type 2 diabetes in 16 tertiary hospitals in urban China: a multicenter prospective cohort study. J Diabetes Investig. (2019) 10:539–51. doi: 10.1111/jdi.12905
3. Zhang Y, Liu C, Luo S, Xie Y, Liu F, Li X, et al. Factors influencing patients' intentions to use diabetes management apps based on an extended unified theory of acceptance and use of technology model: web-based survey. J Med Internet Res. (2019) 21:e15023. doi: 10.2196/15023
4. Zheng Y, Ley SH, Hu FB. Global aetiology and epidemiology of type 2 diabetes mellitus and its complications. Nat Rev Endocrinol. (2018) 14:88–98. doi: 10.1038/nrendo.2017.151
5. Nguyen MT, Fernando S, Schwarz N, Tan JT, Bursill CA, Psaltis PJ. Inflammation as a therapeutic target in atherosclerosis. J Clin Med. (2019) 8:1109. doi: 10.3390/jcm8081109
6. Burtenshaw D, Kitching M, Redmond EM, Megson IL, Cahill PA. Reactive Oxygen Species (ROS), intimal thickening, and subclinical atherosclerotic disease. Front Cardiovasc Med. (2019) 6:89. doi: 10.3389/fcvm.2019.00089
7. Singh SS, Pilkerton CS, Shrader CD Jr., Frisbee SJ. Subclinical atherosclerosis, cardiovascular health, and disease risk: is there a case for the Cardiovascular Health Index in the primary prevention population? BMC Public Health. (2018) 18:429. doi: 10.1186/s12889-018-5263-6
9. Degirolamo C, Sabba C, Moschetta A. Therapeutic potential of the endocrine fibroblast growth factors FGF19, FGF21 and FGF23. Nat Rev Drug Discovery. (2016) 15:51–69. doi: 10.1038/nrd.2015.9
10. Babaknejad N, Nayeri H, Hemmati R, Bahrami S, Esmaillzadeh A. An overview of FGF19 and FGF21: the therapeutic role in the treatment of the metabolic disorders and obesity. Horm Metab Res. (2018) 50:441–52. doi: 10.1055/a-0623-2909
11. Kim YC, Byun S, Seok S, Guo G, Xu HE, Kemper B, et al. Small heterodimer partner and fibroblast growth factor 19 inhibit expression of NPC1L1 in mouse intestine and cholesterol absorption. Gastroenterology. (2019) 156:1052–65. doi: 10.1053/j.gastro.2018.11.061
12. Stejskal D, Karpisek M, Hanulova Z, Stejskal P. Fibroblast growth factor-19: development, analytical characterization and clinical evaluation of a new ELISA test. Scand J Clin Lab Invest. (2008) 68:501–7. doi: 10.1080/00365510701854967
13. Hao Y, Zhou J, Zhou M, Ma X, Lu Z, Gao M, et al. Serum levels of fibroblast growth factor 19 are inversely associated with coronary artery disease in Chinese individuals. PLoS ONE. (2013) 8:e72345. doi: 10.1371/journal.pone.0072345
14. Barutcuoglu B, Basol G, Cakir Y, Cetinkalp S, Parildar Z, Kabaroglu C, et al. Fibroblast growth factor-19 levels in type 2 diabetic patients with metabolic syndrome. Ann Clin Lab Sci. (2011) 41:390–6.
15. Zhou M, Learned RM, Rossi SJ, Tian H, DePaoli AM, Ling L. Therapeutic FGF19 promotes HDL biogenesis and transhepatic cholesterol efflux to prevent atherosclerosis. J Lipid Res. (2019) 60:550–65. doi: 10.1194/jlr.M089961
16. Bennett PH. Impact of the new WHO classification and diagnostic criteria. Diabetes Obes Metab. (1999) 1(Suppl. 2):S1–6. doi: 10.1046/j.1463-1326.1999.0010s2001.x
17. Expert Committee on the Diagnosis and Classification of Diabetes Mellitus. Report of the expert committee on the diagnosis and classification of diabetes mellitus. Diabetes Care. (2003) S5–20. doi: 10.2337/diacare.26.2007.s5
18. Expert Panel on Detection, Evaluation and Treatment of High Blood Cholesterol in Adults. Executive summary of the third report of the National Cholesterol Education Program (NCEP) expert panel on detection, evaluation, and treatment of high blood cholesterol in adults (adult treatment panel III). JAMA. (2001). 285:2486–97. doi: 10.1001/jama.285.19.2486
19. Xiao Y, Xiao X, Xu A, Chen X, Tang W, Zhou Z. Circulating adipocyte fatty acid-binding protein levels predict the development of subclinical atherosclerosis in type 2 diabetes. J Diabetes Complic. (2018) 32:1100–4. doi: 10.1016/j.jdiacomp.2018.09.001
20. Xiao Y, Xu A, Hui X, Zhou P, Li X, Zhong H, et al. Circulating lipocalin-2 and retinol-binding protein 4 are associated with intima-media thickness and subclinical atherosclerosis in patients with type 2 diabetes. PLoS ONE. (2013) 8:e66607. doi: 10.1371/journal.pone.0066607
21. Xiao Y, Liu L, Xu A, Zhou P, Long Z, Tu Y, et al. Serum fibroblast growth factor 21 levels are related to subclinical atherosclerosis in patients with type 2 diabetes. Cardiovasc Diabetol. (2015) 14:72. doi: 10.1186/s12933-015-0229-9
22. Wang M, Long W, Li D, Wang D, Zhong Y, Mu D, et al. Plasma 7-ketocholesterol levels and the risk of incident cardiovascular events. Heart. (2017) 103:1788–94. doi: 10.1136/heartjnl-2016-310914
23. Li H, Xiao Y, Liu H, Chen XY, Li XY, Tang WL, et al. Hypoadiponectinemia predicts impaired endothelium-independent vasodilation in newly diagnosed type 2 diabetic patients: an 8-year prospective study. Chin Med J. (2011) 124:3607–12. doi: 10.3760/cma.j.issn.0366-6999.2011.22.002
24. Perveen S, Shahbaz M, Ansari MS, Keshavjee K, Guergachi A. A hybrid approach for modeling type 2 diabetes mellitus progression. Front Genet. (2019) 10:1076. doi: 10.3389/fgene.2019.01076
25. Eid S, Sas KM, Abcouwer SF, Feldman EL, Gardner TW, Pennathur S, et al. New insights into the mechanisms of diabetic complications: role of lipids and lipid metabolism. Diabetologia. (2019) 62:1539–49. doi: 10.1007/s00125-019-4959-1
26. Dolegowska K, Marchelek-Mysliwiec M, Nowosiad-Magda M, Slawinski M, Dolegowska B. FGF19 subfamily members: FGF19 and FGF21. J Physiol Biochem. (2019) 75:229–40. doi: 10.1007/s13105-019-00675-7
27. Dongiovanni P, Crudele A, Panera N, Romito I, Meroni M, De Stefanis C, et al. β-Klotho gene variation is associated with liver damage in children with NAFLD. J Hepatol. (2019) 72:411–9. doi: 10.1016/j.jhep.2019.10.011
28. Antonellis PJ, Droz BA, Cosgrove R, O'Farrell LS, Coskun T, Perfield JW, et al. The anti-obesity effect of FGF19 does not require UCP1-dependent thermogenesis. Mol Metab. (2019) 30:131–9. doi: 10.1016/j.molmet.2019.09.006
29. Fang Q, Li H, Song Q, Yang W, Hou X, Ma X, et al. Serum fibroblast growth factor 19 levels are decreased in Chinese subjects with impaired fasting glucose and inversely associated with fasting plasma glucose levels. Diabetes Care. (2013) 36:2810–4. doi: 10.2337/dc12-1766
30. Wong YK, Cheung CYY, Tang CS, Au KW, Hai JSH, Lee CH, et al. Age-biomarkers-clinical risk factors for prediction of cardiovascular events in patients with coronary artery disease. Arterioscler Thromb Vasc Biol. (2018) 38:2519–27. doi: 10.1161/ATVBAHA.118.311726
31. Fu L, John LM, Adams SH, Yu XX, Tomlinson E, Renz M, et al. Fibroblast growth factor 19 increases metabolic rate and reverses dietary and leptin-deficient diabetes. Endocrinology. (2004) 145:2594–603. doi: 10.1210/en.2003-1671
32. Schreuder TC, Marsman HA, Lenicek M, van Werven JR, Nederveen AJ, Jansen PL, et al. The hepatic response to FGF19 is impaired in patients with nonalcoholic fatty liver disease and insulin resistance. Am J Physiol Gastrointest Liver Physiol. (2010) 298:G440–5. doi: 10.1152/ajpgi.00322.2009
33. Gimbrone MA Jr., Garcia-Cardena G. Vascular endothelium, hemodynamics, and the pathobiology of atherosclerosis. Cardiovasc Pathol. (2013) 22:9–15. doi: 10.1016/j.carpath.2012.06.006
34. Duan Y, Zhang F, Yuan W, Wei Y, Wei M, Zhou Y, et al. Hepatic cholesterol accumulation ascribed to the activation of ileum Fxr-Fgf15 pathway inhibiting hepatic Cyp7a1 in high-fat diet-induced obesity rats. Life Sci. (2019) 232:116638. doi: 10.1016/j.lfs.2019.116638
35. Schumacher JD, Kong B, Wu J, Rizzolo D, Armstrong LE, Chow MD, et al. Direct and indirect effects of fibroblast growth factor (FGF) 15 and FGF19 on liver fibrosis development. Hepatology. (2019) 71:670–85. doi: 10.1002/hep.30810
36. Gutierrez A, Ratliff EP, Andres AM, Huang X, McKeehan WL, Davis RA. Bile acids decrease hepatic paraoxonase 1 expression and plasma high-density lipoprotein levels via FXR-mediated signaling of FGFR4. Arterioscler Thromb Vasc Biol. (2006) 26:301–6. doi: 10.1161/01.ATV.0000195793.73118.b4
37. Shih DM, Kast-Woelbern HR, Wong J, Xia YR, Edwards PA, Lusis AJ. A role for FXR and human FGF-19 in the repression of paraoxonase-1 gene expression by bile acids. J Lipid Res. (2006) 47:384–92. doi: 10.1194/jlr.M500378-JLR200
38. Lioudaki S, Verikokos C, Kouraklis G, Kontopodis N, Markakis G, Ioannou C, et al. Paraoxonase-1 and symptomatic status in carotid artery disease. Ann Vasc Surg. (2019) 64:355–60. doi: 10.1016/j.avsg.2019.07.020
39. Vaisar T, Kanter JE, Wimberger J, Irwin AD, Gauthier J, Wolfson E, et al. High concentration of medium-sized HDL particles and enrichment in HDL paraoxonase 1 associate with protection from vascular complications in people with long-standing type 1 diabetes. Diabetes Care. (2019) 43:178–86. doi: 10.2337/dc19-0772
40. Kotur-Stevuljevic J, Vekic J, Stefanovic A, Zeljkovic A, Ninic A, Ivanisevic J, et al. Paraoxonase 1 and atherosclerosis-related diseases. Biofactors. (2019) 46:193–205. doi: 10.1002/biof.1549
41. Patel SB, Graf GA, Temel RE. ABCG5 and ABCG8: more than a defense against xenosterols. J Lipid Res. (2018) 59:1103–13. doi: 10.1194/jlr.R084244
42. Qin P, Tang X, Elloso MM, Harnish DC. Bile acids induce adhesion molecule expression in endothelial cells through activation of reactive oxygen species, NF-kappaB, and p38. Am J Physiol Heart Circ Physiol. (2006) 291:H741–7. doi: 10.1152/ajpheart.01182.2005
43. Calmus Y, Poupon R. Shaping macrophages function and innate immunity by bile acids: mechanisms and implication in cholestatic liver diseases. Clin Res Hepatol Gastroenterol. (2014) 38:550–6. doi: 10.1016/j.clinre.2014.07.007
44. Shimizu H, Hagio M, Iwaya H, Tsuneki I, Lee JY, Fukiya S, et al. Deoxycholic acid is involved in the proliferation and migration of vascular smooth muscle cells. J Nutr Sci Vitaminol. (2014) 60:450–4. doi: 10.3177/jnsv.60.450
45. Byun S, Jung H, Chen J, Kim YC, Kim DH, Kong B, et al. Phosphorylation of hepatic farnesoid X receptor by FGF19 signaling-activated Src maintains cholesterol levels and protects from atherosclerosis. J Biol Chem. (2019) 294:8732–44. doi: 10.1074/jbc.RA119.008360
46. Spence JD, Pilote L. Importance of sex and gender in atherosclerosis and cardiovascular disease. Atherosclerosis. (2015) 241:208–10. doi: 10.1016/j.atherosclerosis.2015.04.806
47. Krejza J, Arkuszewski M, Kasner SE, Weigele J, Ustymowicz A, Hurst RW, et al. Carotid artery diameter in men and women and the relation to body and neck size. Stroke. (2006) 37:1103–5. doi: 10.1161/01.STR.0000206440.48756.f7
48. Herring MJ, Oskui PM, Hale SL, Kloner RA. Testosterone and the cardiovascular system: a comprehensive review of the basic science literature. J Am Heart Assoc. (2013) 2:e000271. doi: 10.1161/JAHA.113.000271
49. Vitale C, Mendelsohn ME, Rosano GM. Gender differences in the cardiovascular effect of sex hormones. Nat Rev Cardiol. (2009) 6:532–42. doi: 10.1038/nrcardio.2009.105
50. Franconi F, Rosano G, Basili S, Montella A, Campesi I. Human cells involved in atherosclerosis have a sex. Int J Cardiol. (2017) 228:983–1001. doi: 10.1016/j.ijcard.2016.11.118
Keywords: fibroblast growth factor 19, atherosclerosis, intima-media thickness, type 2 diabetes, prediction
Citation: Hu J, Liu Z, Tong Y, Mei Z, Xu A, Zhou P, Chen X, Tang W, Zhou Z and Xiao Y (2020) Fibroblast Growth Factor 19 Levels Predict Subclinical Atherosclerosis in Men With Type 2 Diabetes. Front. Endocrinol. 11:282. doi: 10.3389/fendo.2020.00282
Received: 25 December 2019; Accepted: 15 April 2020;
Published: 22 May 2020.
Edited by:
Yang Yang, Northwest University, ChinaReviewed by:
Subrata Chakrabarti, University of Western Ontario, CanadaMarta Letizia Hribal, University of Catanzaro, Italy
Julian R. F. Walters, Imperial College London, United Kingdom
Copyright © 2020 Hu, Liu, Tong, Mei, Xu, Zhou, Chen, Tang, Zhou and Xiao. This is an open-access article distributed under the terms of the Creative Commons Attribution License (CC BY). The use, distribution or reproduction in other forums is permitted, provided the original author(s) and the copyright owner(s) are credited and that the original publication in this journal is cited, in accordance with accepted academic practice. No use, distribution or reproduction is permitted which does not comply with these terms.
*Correspondence: Zhiguang Zhou, emhvdXpoaWd1YW5nQGNzdS5lZHUuY24=; Yang Xiao, eGlhb3lhbmcyOUBjc3UuZWR1LmNu