- 1Department of Fundamental and Community Nursing, School of Nursing, Nanjing Medical University, Nanjing, China
- 2Department of Nursing, The Affiliated Brain Hospital of Nanjing Medical University, Nanjing, China
- 3Department of Epidemiology, School of Public Health, Nanjing Medical University, Nanjing, China
- 4Department of Information, The First Affiliated Hospital of Nanjing Medical University, Nanjing, China
- 5Department of Epidemiology, Shanghai Cancer Institute, Shanghai, China
Background: It has been demonstrated that vitamin D receptor (VDR), a key gene in the metabolism of vitamin D (VD), may affect the development of Non-alcoholic fatty liver disease (NAFLD) by regulating VD level and its biological effects.
Objectives: To investigate the effects of serum VD level, VDR variation, and a combination of VDR SNP and environmental behavior factor on the risk of NAFLD.
Methods: A total of 3023 subjects from a community in Nanjing were enrolled, including 1120 NAFLD cases and 1903 controls. Serum 25(OH)D3 levels were measured and eight single nucleotide polymorphisms (SNPs) in VDR gene were genotyped.
Results: Logistic regression analyses indicated that VD sufficiency and VD insufficiency were significantly associated with a low risk of NAFLD (all P<0.05; all Ptrend <0.05, in a locus-dosage manner). After adjusting for gender and age, VDR rs2228570-A and rs11168287-A alleles were all reduced the risk of NAFLD (all PFDR=0.136, in dominant model; Ptrend =0.039, combined effects in a locus-dosage manner). The protective effects of two favorable alleles were more evident among subjects ≤40 years, non-hypertension, non-hyperglycemia and non-low high density lipoprotein-cholesterol (all P<0.05). The area under the receiver operating curve of the combination of VDR SNP and exercise time for assessing NAFLD risk was slightly higher than that of only including exercise time or neither (all P<0.05).
Conclusion: High serum VD levels and VDR variants (rs2228570-A and rs11168287-A) might contribute to a low risk of NAFLD in Chinese Han population. The inclusion of VDR SNP and exercise time could improve the efficiency in assessment of NAFLD risk, which might provide a novel perspective for early screening and preventing NAFLD.
Introduction
Non-alcoholic fatty liver disease (NAFLD), as the most prevalent liver disease worldwide, comprises a wide disease spectrum ranging from steatosis to nonalcoholic steatohepatitis (NASH) and NASH-related cirrhosis (1, 2). The reported prevalence reaches 10-30% in the United States, Europe and Asia (3, 4). Most patients with NAFLD are asymptomatic, and only few may complain of nonspecific symptoms, like discomfort, fatigue, and vague right upper abdominal pain (5), but NAFLD can increase the risk of type 2 diabetes mellitus (T2DM) as well as cardiovascular disease (6), and NASH may develop into fibrosis, even causing cirrhosis and hepatocellular carcinoma (7). There are currently no approved therapies for NAFLD (8, 9), and it is still necessary to explore the potential influencing factors affecting the development and progression of NAFLD.
Many factors are associated with NAFLD including insulin resistance (IR), metabolic syndrome and genetic variation (10, 11). Studies have increasingly found that vitamin D (VD) can affect the development of NAFLD by regulating IR (12), immune inflammation (13), lipid metabolism and target gene expression (14). Vitamin D deficiency is implicated in NAFLD etiology (15, 16), insulin resistance (17), visceral obesity, and metabolic syndrome (18). A meta-analysis found that compared to controls, patients with NAFLD had a lower level of VD (0.36 ng/mL) and were 1.26 times more likely to be VD-deficient (19). A population-based case-control study in China found that low serum VD was associated with advanced liver fibrosis in NAFLD patients (20). However, another systematic review and meta-analysis suggested that serum VD level might not change with NAFLD histologic severity (21). A randomized, double-blind, placebo-controlled trial in Italy suggested that although high-dose oral VD supplementation could increase the serum VD level, no effect was shown on hepatic steatosis or metabolic/cardiovascular parameters in T2DM patients with NAFLD (22). Thus, the relationship between VD and NAFLD was controversial.
VD must be transported, hydroxylated and finally combined with vitamin D receptor (VDR) to accomplish its biological roles (23). Serum VD may produce different biological effects due to genetic heterogeneity, which may help to explain why some people are prone to NAFLD, while others are not (even if they have metabolic syndrome components). Thus, exploring the influence of genetic variation on NAFLD related to VD metabolism is necessary. VDR, a key gene in VD metabolic pathway and a member of steroid/thyroid hormone receptor family (24), transcriptionally activates target genes after binding to vitamin D responsive elements localized in the promoter regions (25). These target genes mediate various NAFLD-associated processes, including lipid and glucose metabolism, cholesterol efflux and bile acid homeostasis, hepatic fibrogenesis, cellular differentiation, apoptosis and immune response (26, 27).
Notably, NAFLD is considered a complex disease trait such that interactions between the environment and a susceptible genetic host background influence disease development and progression (2). Studying data suggests that genetic background may influence the response to lifestyle modification. For example, reduction in liver fat and liver enzyme levels in response to weight loss was larger in subjects homozygous for the PNPLA3 I148M variant compared to non-carriers (28, 29). However, there is little information available on the association between environmental behaviors and VDR polymorphisms in the development and progression of NAFLD. Therefore, the aim of this study was to explore the effects of serum VD level, VDR variation, and a combination of VDR SNP and environmental behavior factor on the risk of NAFLD.
Methods
Participants and Study Design
A total of 3023 subjects from a community in Nanjing (Jiangsu, China) were enrolled in this case-control study during July to September 2018. The NAFLD cases were recruited from those diagnosed based on “Guideline of prevention and treatment for nonalcoholic fatty liver disease: a 2018 update”. NAFLD can be diagnosed if the following items 1-4 coexist with the fifth or sixth item: (1) no drinking or history of overdose drinking (less than 210g/week ethanol for men and 140g/week for women in the past 12 months); (2) excluding drug hepatitis, hepatitis C virus genotype 3 infection, hepatolenticular degeneration and other specific diseases that could result in fatty liver; (3) serum levels of transaminase and γ-glutamyl transpeptidase (γ-GT) increase mildly to moderately (<5 times above the upper normal limit), usually presenting as an increase of alanine aminotransferase (ALT); (4) metabolic syndrome constituents such as visceral obesity, hyperglycemia, blood lipid disorder and hypertension; (5) the result of liver imaging study meets the imaging diagnostic criteria of diffuse fatty liver; (6) the histological findings of liver biopsy meet the pathological diagnostic criteria of fatty liver disease. The non-NAFLD controls were collected from the same community during the study period and randomly assigned to the control group. The constituent ratios of gender and age between cases and controls were considered similar, according the results of frequency-matching.
Excluded were: (1) less than 18 years old; (2) subjects with infection, acute or chronic gastrointestinal diseases, autoimmune diseases or malignant tumors; (3) subjects with history of other viral hepatitis, alcoholic liver disease or primary liver cancer; (4) subjects of excessive drinking (alcohol consumption ≥30g/d in males and ≥20g/d in females); (5) subjects who received a liver transplant within the previous year, or had complications of advanced liver disease (varicose veins, ascites, etc.); (6) subjects with drug-induced fatty hepatitis; (7) subjects with history of psychiatric disorders.
By reviewing the literature, we assumed the frequency of gene mutation in the general population was 20%, odds ratio (OR) was 1.5, two-sided test α was 0.05, and power of test (1-β) was 90%. Therefore, the minimum sample size was estimated by NCSS-PASS 11 software (Dawson edition; Kaysville, UT) to be 784. This study had a sample size large enough to guarantee the production of reliable results.
The current study protocol was in accordance with the Declaration of Helsinki, and was approved by the Institutional Ethics Review Committee of Nanjing Medical University (Nanjing, China). Written informed consent was obtained before blood test and genetic analysis.
Collection of Basic Data and Blood Samples
Self-designed questionnaires and an electronic medical record system were used to collect the demographic and clinical characteristics of all participants. 5-mL ethylene diamine tetraacetic acid (EDTA) anticoagulant venous blood was collected from each subject in the morning after an overnight fast. Within 2 hours, the serum and blood cells in each blood sample were separated and frozen at -80°C until further serological tests and genotyping assays.
Serum 25(OH)D3 Level Detection
In two groups (gender and age matched at a ratio of 1:1, 336 subjects in the NAFLD group and 336 in the control group), the serum 25(OH)D3 level was measured by enzyme-linked immunosorbent assay (ELISA) according to the manufacturer’s instructions (Human 25-Dihydroxy vitamin D3 (25(OH)D3) ELISA Kit; Jin Yibai Biological Technology Co., Ltd.; Nanjing, China).
SNP Selection and Genotyping
Target SNPs with potential biological function were selected to better identify the susceptibility sites that affect the development of NAFLD. The selection processes were as follows: (1) Downloading the genotype database of VDR gene in Han Chinese in Beijing (CHB) from the 1000 Genomes Project database (http://asia.ensembl.org/Homo_sapiens/Info/Index); (2) Importing the genotype database into the Haploview software (version 4.2; Broad Institute, Cambridge, MA, USA). Setting parameters: Hardy-Weinberg P-value cutoff = 0.05; minor allele frequency (MAF) = 0.05; r2 threshold = 0.8. At this point, 56 tagging SNPs (tagSNPs) were captured; (3) Searching for literature in which these potential tagSNPs were associated with NAFLD, hypertension, type 2 diabetes, hyperlipidemia, metabolic syndrome, inflammatory diseases or immune-related disorders. Finally, eight disease-related target SNPs of VDR gene were selected, including rs3782905 (C>G), rs3847987 (C>A), rs11574129 (T>C), rs2228570 (C>A), rs11568820 (G>A), rs739837 (G>T), rs7975232 (C>A) and rs11168287 (G>A).
Genomic DNA was isolated from EDTA anticoagulated blood samples using magnetic bead method (blood genomic extraction kit; Pangu Genome Nanotechnology Co., Ltd.; Nanjing, China). The eight SNPs were genotyped using TaqMan allelic discrimination assays on the ABI 9700 system (Applied Biosystems, Foster City, California, USA; catalog numbers: C:_3290647_10, C:_2404006_10, C_175992105_10, C:12060045_20, C:_2880808_10, C:_2404007_10, C:28977635_10, C:_2404006_10). The quality control of experimental data was as follows: (1) blinding was adopted in genotyping, so that all laboratory personnel were unclear about the clinical data of the subjects; (2) 10% of the samples were randomly selected for repeated experiments with a repeatability of 100%. The success rates of genotyping all SNPs were higher than 99% in this study.
Statistical Analysis
All statistical analyses were processed using SPSS (version 22.0, SPSS Inc., Chicago, IL, USA) and MedCalc (Version 19.1, Ostend, Belgium). Distributions of demographic and clinical characteristics of two groups were compared using χ2-test, student’s t test or Mann-Whitney U test, wherever appropriate. Logistic regression analysis was used to calculate odds ratio (OR) and 95% confidence interval (95% CI) for quantifying the association of serum 25(OH)D3 level with the risk of NAFLD. The correlation of serum 25(OH)D3 level with VDR SNPs was assessed using general linear regression model adjusted for gender and age. The Hardy-Weinberg equilibrium (HWE) was tested using a goodness of-fit χ2 -test among the control subjects. The relationships between VDR SNPs and the risk of NAFLD were analyzed by dominant model (heterozygote + mutant homozygote VS. wild homozygote), recessive model (mutant homozygote VS. wild homozygote + heterozygote), and additive model (mutant homozygote VS. heterozygote VS. wild homozygote), respectively. For multiple SNPs comparisons, false discovery rate (FDR) correction was used and the PFDR value ≤ 0.25 was regarded as modest confidence that the correlation represented a positive result (30). Subgroup analysis was performed for positive SNPs, and Q test was performed to calculate the heterogeneity between subgroups. The area under the receiver operating curves (AUROCs) was performed to assess the predictive power of the combination of positive SNPs, environmental behavior factors and mainly related clinical factors for NAFLD risk. A two-tailed test with a P value < 0.05 was regarded as statistically significant in all analyses.
Results
Basic Characteristics of Participants
The demographic and clinical characteristics of 1120 NAFLD cases and 1903 controls were summarized in Table 1. No significant differences were observed in the distribution of gender and age between the two groups (all P>0.05). However, there were significant differences in exercise time, body mass index (BMI), waist circumference (WC), systolic blood pressure (SBP), diastolic blood pressure (DBP), triglyceride (TG), total cholesterol (TC), high density lipoprotein-cholesterol (HDL-C), low density lipoprotein-cholesterol (LDL-C), glucose (GLU), γ-glutamyl transpeptidase (γ-GT), alanine aminotransferase (ALT), aspartate aminotransferase (AST), direct bilirubin (DBIL), total bilirubin (TBIL) and serum 25(OH)D3 levels (all P<0.05).
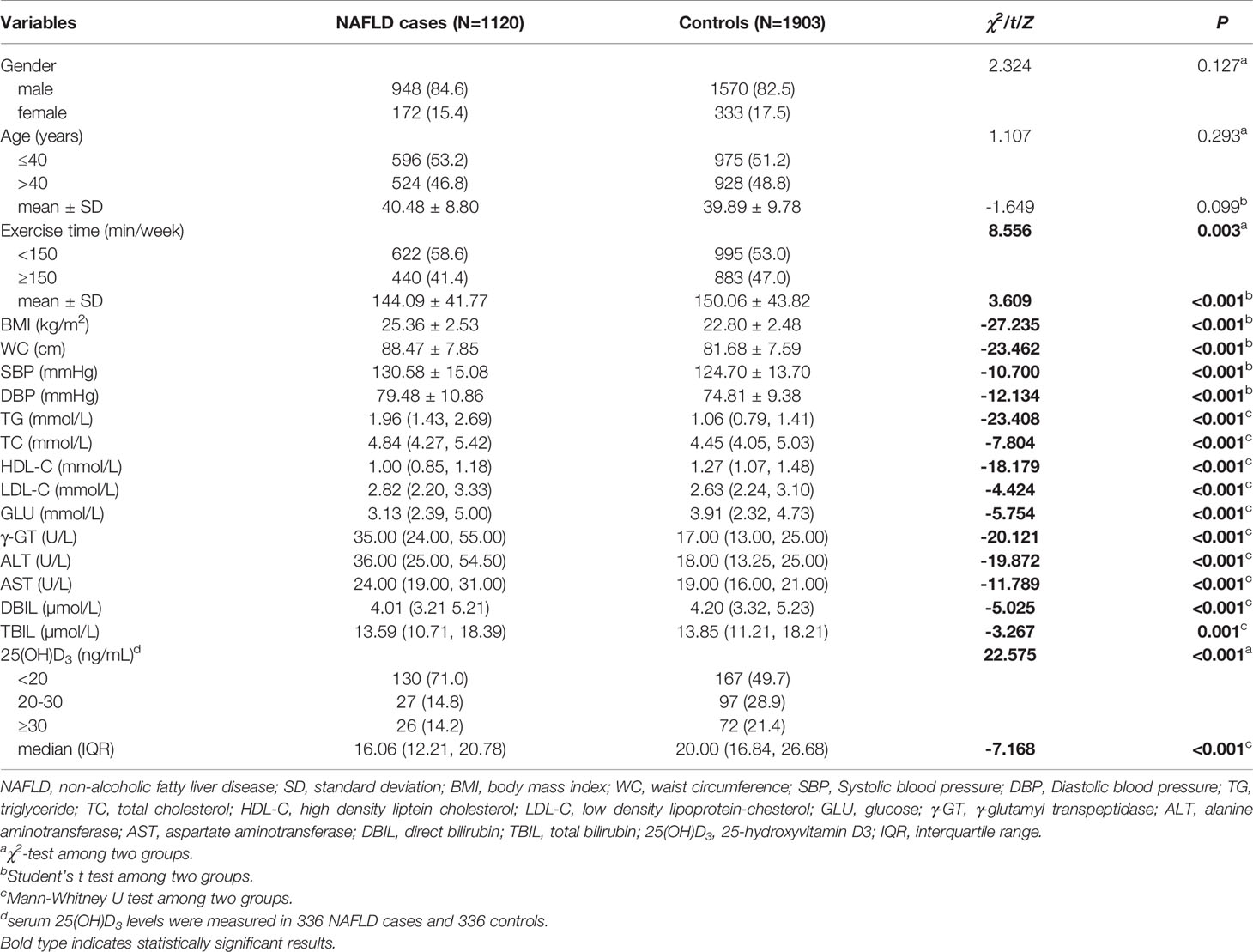
Table 1 Distributions and comparisons of demographic and clinical characteristics between NAFLD case and control groups.
Association Between Serum 25(OH)D3 Level and NAFLD Risk
According to the level of serum 25(OH)D3, 672 participants were divided into three categories: VD deficiency (<20ng/mL), VD insufficiency (20ng/mL-30ng/mL) and VD sufficiency (≥30ng/mL) (31). The constituent ratios of three categories were 60.9% (409/672), 21.4% (144/672), and 17.7% (119/672), respectively. The prevalences of NAFLD in the three categories were 59.2% (242/409), 32.6% (47/144) and 39.5% (47/199), respectively, with significant differences (χ2 = 36.366, P<0.05). In addition, multiple comparisons showed that the prevalence of NAFLD in patients with VD deficiency was significantly higher than that in patients with VD insufficient or VD sufficient (all PBonferroni<0.017), but there was no significant difference in the prevalence of NAFLD between categories with VD insufficiency and VD sufficiency (PBonferroni>0.017) (Supplementary Figure 1).
Further logistic regression analysis suggested that compared to VD deficiency, VD sufficiency (crude OR=0.450, 95%CI=0.297-0.684, P<0.001) and VD insufficiency (crude OR=0.334, 95%CI=0.224-0.499, P<0.001) were associated with a lower risk of NAFLD, and this association was dose-dependent (Ptrend<0.001) (model 1). After adjusting for gender, age, BMI and SBP (model 2), the subjects with VD sufficiency (adjusted OR=0.477, 95%CI=0.277-0.821, P=0.008) and VD insufficiency (adjusted OR=0.344, 95%CI=0.206-0.573, P<0.001) all had significantly decreased risk of NAFLD compared with those with VD deficiency, and a significant locus-dosage effect of VD level on NAFLD risk was also observed (Ptrend<0.001). When adjusting for the above factors and TG, HDL-C, GLU (model 3), the risk of NAFLD still decreased significantly with the increase of VD level (VD sufficiency vs. VD deficiency: adjusted OR=0.552, 95%CI=0.305-0.998, P=0.049; VD insufficiency vs. VD deficiency: adjusted OR=0.298, 95%CI=0.165-0.539, P<0.001; Ptrend=0.005) (Table 2).
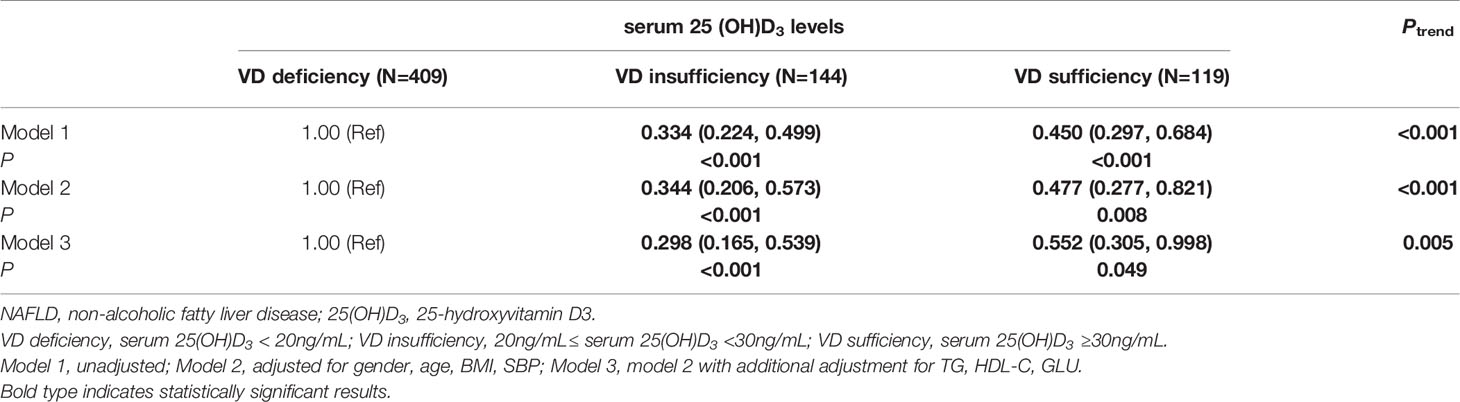
Table 2 Logistic regression analysis of the associations between serum 25(OH)D3 levels and risk of NAFLD.
Associations Between VDR SNPs and Serum 25(OH)D3 Level
Serum 25(OH)D3 levels were Lg transformed into an approximately normal distribution, and SNPs were coded in an additive genetic model. The general linear regression analysis indicated that there were no significant associations between eight VDR SNPs and serum 25(OH)D3 levels after adjusting for gender and age (all P>0.05) (Supplementary Table 1).
Association Between VDR SNPs and NAFLD Risk
The genotype distributions of the eight SNPs in both groups were shown in Table 3. After adjusting for gender and age, logistic regression analyses showed that VDR rs2228570-A variant (AA: adjusted OR=0.782, 95%CI=0.633-0.966, P=0.023; dominant model: adjusted OR=0.837, 95%CI=0.710-0.986, P=0.034; additive model: adjusted OR=0.883, 95%CI=0.794-0.981, P=0.020) and rs11168287-A variant (GA: adjusted OR=0.830, 95%CI=0.707-0.974, P=0.022; dominant model: adjusted OR=0.839, 95%CI=0.721-0.976, P=0.023) all significantly reduced the risk of NAFLD in different models. Under the premise of FDR threshold ≤ 0.25, FDR revealed with modest confidence that the associations between the two SNPs and a low risk of NAFLD were positive (all PFDR=0.136, in dominant model; Supplementary Table 2).
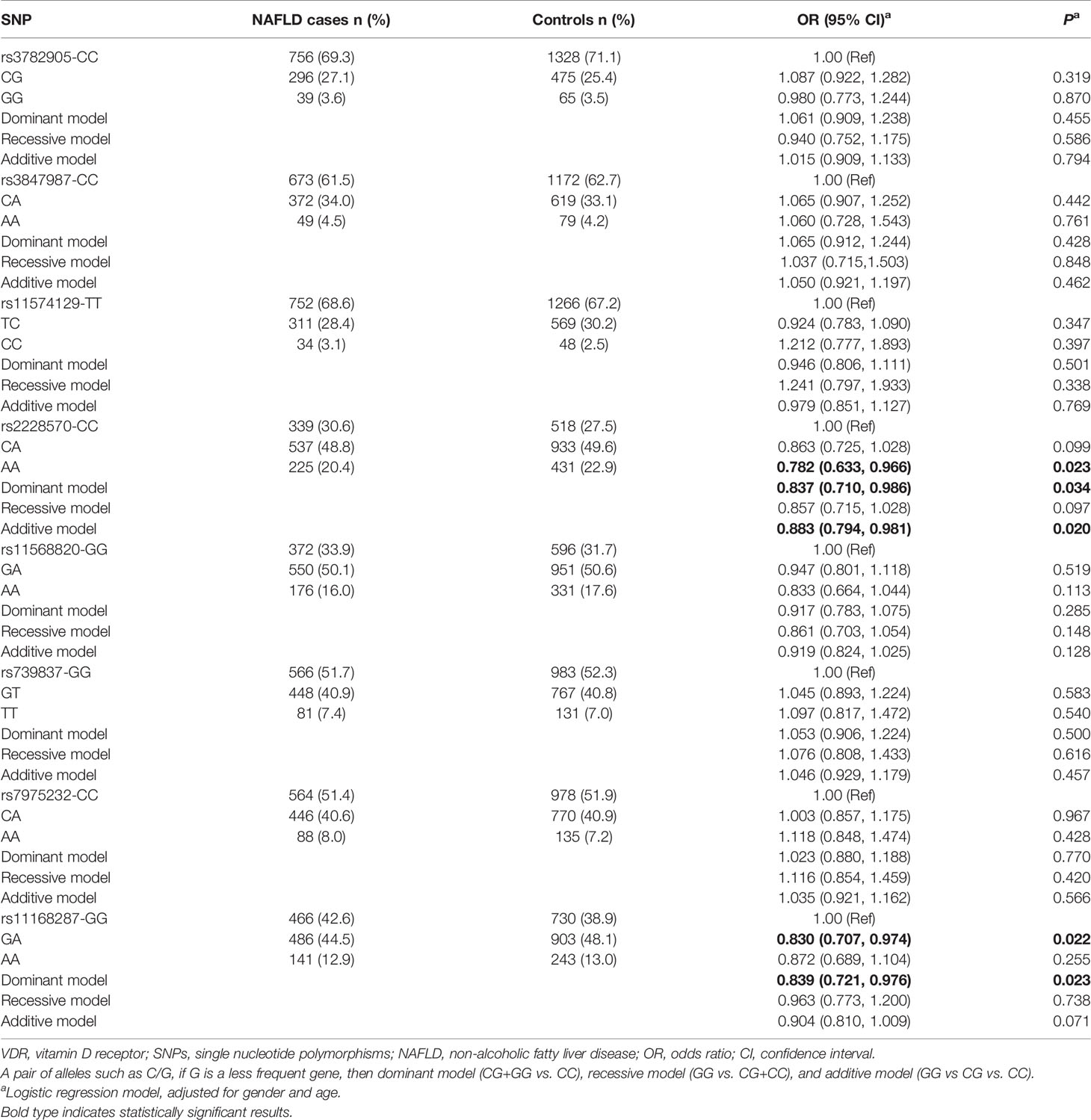
Table 3 Genotype distributions of VDR polymorphisms among the two study groups and association analyses of these eight SNPs and NAFLD.
In addition, the combined effects of VDR variants rs2228570 and rs11168287 on the risk of NAFLD were estimated by the number of favorable alleles from the two SNPs, as shown in Supplementary Table 3. The subjects were first divided into three groups with “0”, “1-2” and “3-4” favorable alleles. Compared with those who had 0 favorable alleles, subjects with “3-4” favorable alleles had significantly decreased risk of NAFLD (adjusted OR=0.750, 95%CI=0.577-0.974, P=0.031) and the more favorable alleles, the lower NAFLD risk, suggesting a significant locus-dosage effect of the combined alleles on NAFLD risk (Ptrend=0.039). The subjects were then distributed into two groups with “0” and “1-4” favorable alleles, and we found that the presence of “1-4” alleles was related to a 0.798-fold lower risk of NAFLD (adjusted 95% CI=0.644-0.990, P=0.040).
We further performed the stratification analyses to evaluate the combined effects of VDR rs2228570-A and rs11168287-A alleles on the risk of NAFLD adjusted with gender and age. As shown in Supplementary Figure 2, the combined protective effects of two alleles were more prominent in subjects ≤ 40 years (adjusted OR=0.696, 95%CI=0.515-0.940, P=0.018), non-hypertension (adjusted OR=0.770, 95%CI=0.602-0.986, P=0.038), non-hyperglycemia (adjusted OR=0.791, 95%CI=0.631-0.991, P=0.042) and non-low HDL-C (adjusted OR=0.710, 95%CI=0.536-0.942, P=0.018). The heterogeneity test discovered no significant differences among all the subgroups (all P>0.05).
Influence Factors of NAFLD
A stepwise regression model were performed with gender, age, visceral obesity, hypertension, hyperglycemia, hypertriglyceridemia, Low HDL-C, ALT, exercise time, rs2228570 and rs11168287. The coding of each variable was described in Supplementary Table 4. The results showed that age ≤40 years (OR=0.716, 95%CI=0.583-0.879, P=0.001), exercise time ≥150 min/week (OR=0.798, 95%CI=0.661-0.963, P=0.019) and rs2228570-A (OR=0.768, 95%CI=0.626-0.942, P=0.011) were independent protective factors of NAFLD. Conversely, visceral obesity (OR=3.653, 95%CI=2.984-4.471, P<0.001), hypertension (OR=1.654, 95%CI=1.290-2.121, P<0.001), hypertriglyceridemia (OR=3.455, 95%CI=2.784-4.288, P<0.001), Low HDL-C (OR=1.879, 95%CI=1.536-2.299, P<0.001) and ALT > 40U/L (OR=2.729, 95%CI=2.060-3.615, P<0.001) were independent risk factors of NAFLD (Table 4).
We further constructed combined factors based on the above factors for assessing NAFLD risk, and the combined factor 1 (a combination of clinical factors) = (-1.588) + (-0.229) × age + 1.333 × visceral obesity + 0.484 × hypertension + 1.233 × hypertriglyceridemia + 0.621 × Low HDL-C + 0.939 × ALT. Similarly, combined factor 2 (a combination of clinical factors and exercise time) = (-1.448) + (-0.254) × age + 1.294 × visceral obesity + 0.512 × hypertension + 1.222 × hypertriglyceridemia + 0.610 × low HDL-C + 0.958 × ALT + (-0.220) × exercise time; combined factor 3 (a combination of clinical factors, exercise time and SNP genetic variant) = (-1.519) + (-0.334) × age + 1.296 × visceral obesity + 0.503 × hypertension + 1.240 × hypertriglyceridemia + 0.631 × low HDL-C + 1.004 × ALT + (-0.225) × exercise time + (-0.264) × rs2228570. In Figure 1, the AUROCs of these combined factors were 0.770, 0.774, and 0.780, respectively, and the AUROC of combined factor 3 for assessing NAFLD risk was slightly higher than that of combined factor 2 (0.780 vs. 0.774, 95%CI=0.002-0.011, P=0.007) and combined factor 1 (0.780 vs. 0.770, 95%CI=0.005-0.016, P<0.001).
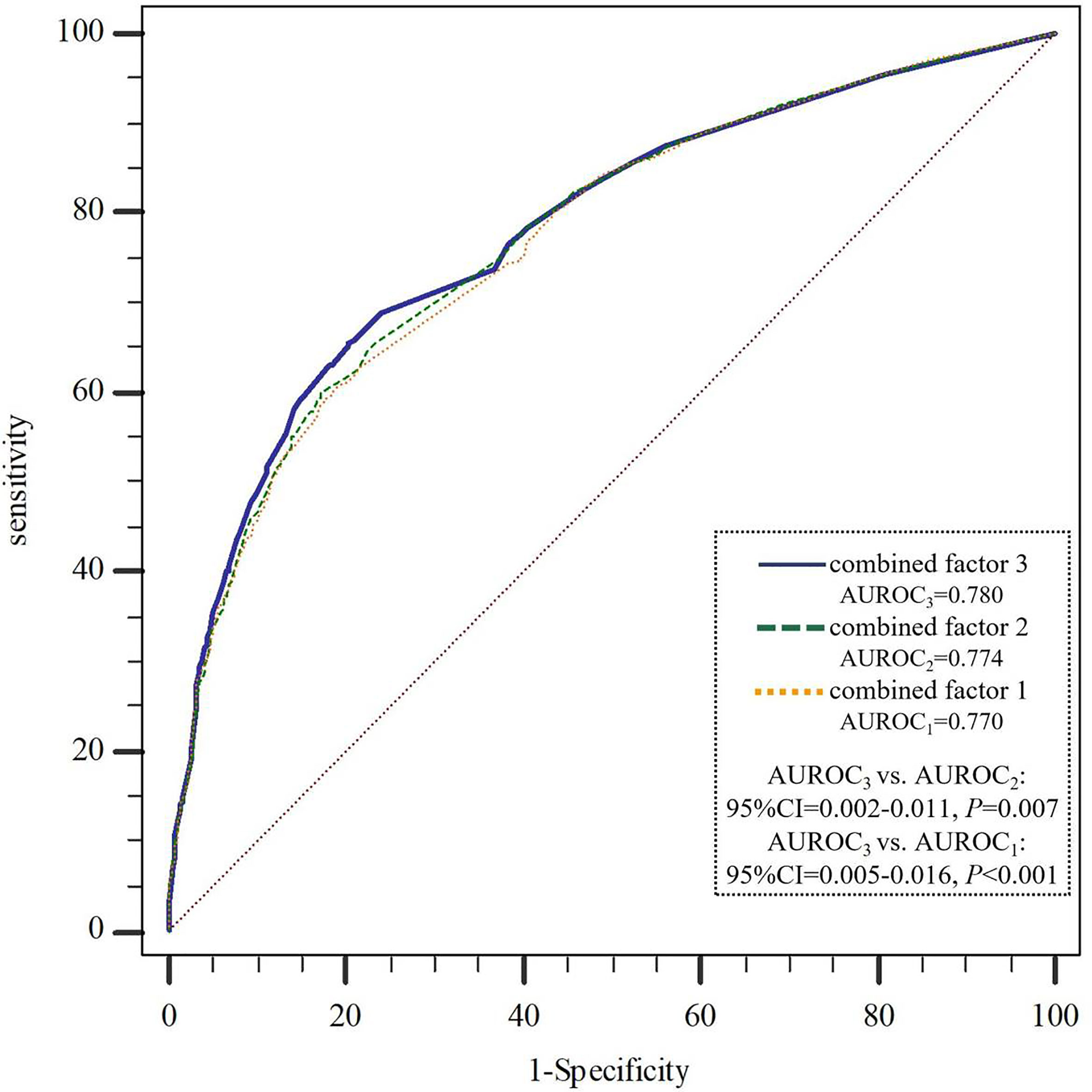
Figure 1 AUROCs comparison of the three combined factors to assess NAFLD risk. NAFLD, non-alcoholic fatty liver disease; AUROC, area under the receiver operating curve. combined factor 1: a combination of age, visceral obesity, blood pressure, TG, HDL-C and ALT; combined factor 2: a combination of combined factor 1 and exercise time; combined factor 3: a combination of combined factor 2 and VDR-rs2228570.
Discussion
In the current study, our findings suggested that high serum vitamin D level, VDR rs2228570-A and rs11168287-A were associated with a decreased risk of NAFLD, and the combination of VDR rs2228570 and exercise time was more effective in the risk assessment of NAFLD.
Accumulative evidence suggests that VD deficiency is highly prevalent among the general population in China (32), and low VD level is a risk factor of NAFLD (33). VD poses protective effects on many other metabolic-related diseases, such as obesity, hypertension, insulin resistance, type 2 diabetes, metabolic syndrome and cardiovascular disease (34–36). Consistently, our study manifested a high prevalence of NAFLD (59.2%) in the subjects with low serum 25(OH)D3 level (<20ng/mL), and VD deficiency might increase the risk of NAFLD. The regulatory mechanism of VD level and VDR activity in the development of NAFDL has not been fully elucidated yet (37, 38). Therefore, the present study provides a new insight into the association between VDR genetic mutation and NAFLD risk.
The VDR SNPs rs7975232, rs2228570 were reported to be associated with VD deficiency (39, 40). However, other research showed no association of rs7975232, rs11568820, rs11574129 with serum 25(OH)D3 in a Han Chinese population (41). A case-control study in Caucasian population found no correlation between VD level and rs2228570 genotype (42). In our study, no significant associations were found between eight VDR polymorphisms and 25(OH)D3 level either. Possible explanations include geographical location, racial backgrounds and sunlight exposure (43).
Our study suggested that the SNPs of rs2228570-A and rs11168287-A in VDR gene exhibited an association with the decrease in NAFLD risk, with an evident gene-gene combined effect. Metabolic impairment and alteration of the glucose-insulinhomeostasis are the primarily pathogenesis of NAFLD (44). Evidence indicated that the rs2228570 polymorphism of VDR gene was associated with the risk of fasting glucose in a Chinese Han population (45). It was found that the expression of VDR mRNA in the liver of obese individuals with biopsy-proven NAFLD was higher than that of non-NAFLD (46). Study reported that the combination of rs2228570 (FokI), rs1544410 (BsmI) and rs731236 (TaqI) was involved in the risk of T2DM in north Indians (47). However, another study showed that VDR-rs2225780 genetic variation was not associated with T2DM in a Caucasian population (42). The possible explanation might have various ethnic groups. The Jackson Heart Study indicated that VDR variants are associated with abdominal visceral adipose tissue volume and adiponectin concentrations, but not with BMI or WC in African Americans (48), a finding that is consistent with the stratified analysis of the combined effect of the two positive SNPs in this study. Although there is no research on rs11168287 polymorphisms and NAFLD, we found that rs11168287 is situated in the peak of H3k4me1 and near the peak of transcription levels by searching the UCSC genome browser (https://genome.ucsc.edu), which means that rs11168287 mutation might affect the serum VD level and its biological effects including insulin sensitivity, lipid metabolism as well as immune inflammation by dysregulating the transcription and expression of VDR gene, and ultimately affect the risk of NAFLD. Further study is warranted to identify the role and function of these VDR polymorphisms in VD metabolism and NAFLD risk.
This study also demonstrated that age ≤40 years, exercise time ≥150 min/week, and rs2228570-A were independent protective factors of NAFLD; visceral obesity, hypertension, hypertriglyceridemia, Low HDL-C and ALT >40U/L were independent risk factors of NAFLD, which is consistent with the results of previous studies (49–52). Based on these independent factors, we constructed combined factors to assess NAFLD risk. The results showed that the assessment efficiency of combined factor 3 (including age, visceral obesity, blood pressure, TG, HDL-C, ALT, exercise time and rs2228570) in NAFLD risk was higher than that of combined factor 2 (including age, visceral obesity, blood pressure, TG, HDL-C, ALT and exercise time) and combined factor 1 (including age, visceral obesity, blood pressure, TG, HDL-C and ALT). There is no research on the combination of genetic and environmental behavioral factors to evaluate the risk of NAFLD in Chinese population so far. A study of NAFLD risk in Italian obese children and adolescents found that combining genetic variants with clinical risk factors improved the predictability of NAFLD (53). For a disease related to heredity and environment, the predictive power of genetic-environmental factors is reasonably stronger than a single one. However, in our study, compared with the AUROC of combined factor 1 or combined factor 2, the AUROC of combined factor 3 only increased slightly, probably due to the weak effect of a single SNP on disease risk (54). In spite of this, we cannot ignore the effect of individual differences in genetic mutation on disease susceptibility. Once the risk of a particular genotype and environmental exposure combination is known, medical interventions, including medical surveillance, lifestyle advice, diet or drug treatment, could then be taken for high-risk groups or individuals to prevent disease (55, 56).
There are a few limitations in our study. Firstly, based on the case-control study, we collected the data of the subjects and measured their VD level, which may not represent the average level of different seasons. A convenient and inexpensive ELISA method was used to detect 25(OH)D3 levels in this study. Although it is not as accurate as liquid chromatography tandem mass spectrometry (LC-MS), it can reflect the level of 25(OH)D3 to some extent (41, 57). Secondly, only subjects from one community were enrolled, which may not be representative enough. In response to this problem, the frequency-matching of gender and age was used in the design stage, and multivariate analysis and stratified analysis were carried out to control the influence of the confounding factors to a certain extent. Finally, we only selected one key gene in the VD metabolic pathway, which may not be able to fully analyze the relationship between genetic factors and NAFLD risk. It is necessary to further explore the impact of polygenic polyloci and their combination with environmental factors on disease risk in a multicenter population of different races.
In conclusion, our results supported that high serum VD levels and VDR variants (rs2228570-A and rs11168287-A) might be involved in a low risk of NAFLD in the Chinese Han population, and a combination of VDR SNP and exercise time could improve the efficiency in assessment of NAFLD risk. These findings might provide new insight for risk evaluation of NAFLD and early screening of high-risk population.
Data Availability Statement
The original contributions presented in the study are included in the article/Supplementary Material. Further inquiries can be directed to the corresponding author.
Ethics Statement
The studies involving human participants were reviewed and approved by The Institutional Ethics Review Committee of Nanjing Medical University (Nanjing, China). The patients/participants provided their written informed consent to participate in this study.
Author Contributions
JW designed and organized the study. RZ, MXW, MW, LZ, YD, ZT, ZF, HF, and WZ contributed to the planning, designing and analyses of the experiments, data collection and quality control. RZ, MXW, and JW wrote and critical revised the manuscript. All authors contributed to the article and approved the submitted version.
Funding
This work was supported by the Natural Science Foundation of Jiangsu Province, China (Grant No. BK20181369) and Priority Academic Program Development of Juangsu Higher Education Institutions (Grant No. PAPD [2018] 87).
Conflict of Interest
The authors declare that the research was conducted in the absence of any commercial or financial relationships that could be construed as a potential conflict of interest.
Publisher’s Note
All claims expressed in this article are solely those of the authors and do not necessarily represent those of their affiliated organizations, or those of the publisher, the editors and the reviewers. Any product that may be evaluated in this article, or claim that may be made by its manufacturer, is not guaranteed or endorsed by the publisher.
Acknowledgments
Thank you Mr. Yongke Cao for providing article polishing support.
Supplementary Material
The Supplementary Material for this article can be found online at: https://www.frontiersin.org/articles/10.3389/fendo.2021.648844/full#supplementary-material
References
1. Seto WK, Yuen MF. Nonalcoholic Fatty Liver Disease in Asia: Emerging Perspectives. J Gastroenterol (2017) 52(2):164–74. doi: 10.1007/s00535-016-1264-3
2. Eslam M, Valenti L, Romeo S. Genetics and Epigenetics of NAFLD and NASH: Clinical Impact. J Hepatol (2018) 68(2):268–79. doi: 10.1016/j.jhep.2017.09.003
3. Vernon G, Baranova A, Younossi ZM. Systematic Review: The Epidemiology and Natural History of Non-Alcoholic Fatty Liver Disease and Non-Alcoholic Steatohepatitis in Adults. Aliment Pharmacol Ther (2011) 34(3):274–85. doi: 10.1111/j.1365-2036.2011.04724.x
4. Farrell GC, Wong VW, Chitturi S. NAFLD in Asia–as Common and Important as in the West. Nat Rev Gastroenterol Hepatol (2013) 10(5):307–18. doi: 10.1038/nrgastro.2013.34
5. Sweet PH, Khoo T, Nguyen S. Nonalcoholic Fatty Liver Disease. Prim Care (2017) 44(4):599–607. doi: 10.1016/j.pop.2017.07.003
6. Byrne CD, Targher G. NAFLD: A Multisystem Disease. J Hepatol (2015) 62(1 Suppl):S47–64. doi: 10.1016/j.jhep.2014.12.012
7. Zhou JH, Cai JJ, She ZG, Li HL. Noninvasive Evaluation of Nonalcoholic Fatty Liver Disease: Current Evidence and Practice. World J Gastroenterol (2019) 25(11):1307–26. doi: 10.3748/wjg.v25.i11.1307
8. Sumida Y, Yoneda M. Current and Future Pharmacological Therapies for NAFLD/NASH. J Gastroenterol (2018) 53(3):362–76. doi: 10.1007/s00535-017-1415-1
9. Mazhar K. The Future of Nonalcoholic Fatty Liver Disease Treatment. Med Clin North Am (2019) 103(1):57–69. doi: 10.1016/j.mcna.2018.08.005
10. Rinella ME. Nonalcoholic Fatty Liver Disease: A Systematic Review. JAMA (2015) 313(22):2263–73. doi: 10.1001/jama.2015.5370
11. Diehl AM, Day C. Cause, Pathogenesis, and Treatment of Nonalcoholic Steatohepatitis. N Engl J Med (2017) 377(21):2063–72. doi: 10.1056/NEJMra1503519
12. Giblin RJ, Bennett EJ, Zosky GR, Dwyer RM. The Impact of Sex and 25(OH)D Deficiency on Metabolic Function in Mice. Nutrients (2017) 9(9):985. doi: 10.3390/nu9090985
13. Drori A, Rotnemer-Golinkin D, Avni S, Drori A, Danay O, Levanon D, et al. Attenuating the Rate of Total Body Fat Accumulation and Alleviating Liver Damage by Oral Administration of Vitamin D-Enriched Edible Mushrooms in a Diet-Induced Obesity Murine Model Is Mediated by an Anti-Inflammatory Paradigm Shift. BMC Gastroenterol (2017) 17(1):130. doi: 10.1186/s12876-017-0688-4
14. Zhu CG, Liu YX, Wang H, Wang BP, Qu HQ, Wang BL, et al. Active Form of Vitamin D Ameliorates Non-Alcoholic Fatty Liver Disease by Alleviating Oxidative Stress in a High-Fat Diet Rat Model. Endocr J (2017) 64(7):663–73. doi: 10.1507/endocrj.EJ16-0542
15. Jablonski KL, Jovanovich A, Holmen J, Targher G, McFann K, Kendrick J, et al. Low 25-Hydroxyvitamin D Level Is Independently Associated With Non-Alcoholic Fatty Liver Disease. Nutr Metab Cardiovasc Dis (2013) 23(8):792–8. doi: 10.1016/j.numecd.2012.12.006
16. Cimini FA, Barchetta I, Carotti S, Morini S, Cavallo MG. Overview of Studies of the Vitamin D/Vitamin D Receptor System in the Development of Non-Alcoholic Fatty Liver Disease. World J Gastrointest Pathophysiol (2019) 10(2):11–6. doi: 10.4291/wjgp.v10.i2.11
17. Pirgon O, Cekmez F, Bilgin H, Eren E, Dundar B. Low 25-Hydroxyvitamin D Level Is Associated With Insulin Sensitivity in Obese Adolescents With Non-Alcoholic Fatty Liver Disease. Obes Res Clin Pract (2013) 7(4):e275–83. doi: 10.1016/j.orcp.2012.01.004
18. Lee SM, Jun DW, Cho YK, Jang KS. Vitamin D Deficiency in Non-Alcoholic Fatty Liver Disease: The Chicken or the Egg. Clin Nutr (2017) 36(1):191–7. doi: 10.1016/j.clnu.2015.10.017
19. Eliades M, Spyrou E, Agrawal N, Lazo M, Brancati FL, Potter JJ, et al. Meta-Analysis: Vitamin D and Non-Alcoholic Fatty Liver Disease. Aliment Pharmacol Ther (2013) 38(3):246–54. doi: 10.1111/apt.12377
20. Yang BB, Chen YH, Zhang C, Shi CE, Hu KF, Zhou J, et al. Low Vitamin D Status Is Associated With Advanced Liver Fibrosis in Patients With Nonalcoholic Fatty Liver Disease. Endocrine (2017) 55(2):582–90. doi: 10.1007/s12020-016-1152-x
21. Jaruvongvanich V, Ahuja W, Sanguankeo A, Wijarnpreecha K, Upala S. Vitamin D and Histologic Severity of Nonalcoholic Fatty Liver Disease: A Systematic Review and Meta-Analysis. Dig Liver Dis (2017) 49(6):618–22. doi: 10.1016/j.dld.2017.02.003
22. Barchetta I, Del Ben M, Angelico F, Di Martino M, Fraioli A, La Torre G, et al. No Effects of Oral Vitamin D Supplementation on Non-Alcoholic Fatty Liver Disease in Patients With Type 2 Diabetes: A Randomized, Double-Blind, Placebo-Controlled Trial. BMC Med (2016) 14:92. doi: 10.1186/s12916-016-0638-y
23. Kumar R, Himani, Gupta N, Singh V, Kumar V, Haq A, et al. Unveiling Molecular Associations of Polymorphic Variants of VDR Gene (FokI, BsmI and ApaI) in Multiple Myeloma Patients of Indian Population. J Steroid Biochem Mol Biol (2020) 199:105588. doi: 10.1016/j.jsbmb.2020.105588
24. Pike JW. Vitamin D3 Receptors: Structure and Function in Transcription. Annu Rev Nutr (1991) 11:189–216. doi: 10.1146/annurev.nu.11.070191.001201
25. Chen KS, DeLuca HF. Cloning of the Human 1 Alpha,25-Dihydroxyvitamin D-3 24-Hydroxylase Gene Promoter and Identification of Two Vitamin D-Responsive Elements. Biochim Biophys Acta (1995) 1263(1):1–9. doi: 10.1016/0167-4781(95)00060-T
26. Haussler MR, Whitfield GK, Kaneko I, Haussler CA, Hsieh D, Hsieh JC, et al. Molecular Mechanisms of Vitamin D Action. Calcif Tissue Int (2013) 92(2):77–98. doi: 10.1007/s00223-012-9619-0
27. Ding N, Yu RT, Subramaniam N, Sherman MH, Wilson C, Rao R, et al. A Vitamin D Receptor/SMAD Genomic Circuit Gates Hepatic Fibrotic Response. Cell (2013) 153(3):601–13. doi: 10.1016/j.cell.2013.03.028
28. Shen J, Wong GL, Chan HL, Chan RS, Chan HY, Chu WC, et al. PNPLA3 Gene Polymorphism and Response to Lifestyle Modification in Patients With Nonalcoholic Fatty Liver Disease. J Gastroenterol Hepatol (2015) 30(1):139–46. doi: 10.1111/jgh.12656
29. Sevastianova K, Kotronen A, Gastaldelli A, Perttilä J, Hakkarainen A, Lundbom J, et al. Genetic Variation in PNPLA3 (Adiponutrin) Confers Sensitivity to Weight Loss-Induced Decrease in Liver Fat in Humans. Am J Clin Nutr (2011) 94(1):104–11. doi: 10.3945/ajcn.111.012369
30. Woods NT, Monteiro AN, Thompson ZJ, Amankwah EK, Naas N, Haura EB, et al. Interleukin Polymorphisms Associated With Overall Survival, Disease-Free Survival, and Recurrence in Non-Small Cell Lung Cancer Patients. Mol Carcinog (2015) 54 Suppl 1(0 1):E172–84. doi: 10.1002/mc.22275
31. Henry HL, Bouillon R, Norman AW, Gallagher JC, Lips P, Heaney RP, et al. 14th Vitamin D Workshop Consensus on Vitamin D Nutritional Guidelines. J Steroid Biochem Mol Biol (2010) 121(1-2):4–6. doi: 10.1016/j.jsbmb.2010.05.008
32. Cheng Q, Du Y, Hong W, Tang W, Li H, Chen M, et al. Factors Associated to Serum 25-Hydroxyvitamin D Levels Among Older Adult Populations in Urban and Suburban Communities in Shanghai, China. BMC Geriatr (2017) 17(1):246. doi: 10.1186/s12877-017-0632-z
33. Cho YH, Kim JW, Shim JO, Yang HR, Chang JY, Moon JS, et al. Association Between Vitamin D Deficiency and Suspected Nonalcoholic Fatty Liver Disease in an Adolescent Population. Pediatr Gastroenterol Hepatol Nutr (2019) 22(3):233–41. doi: 10.5223/pghn.2019.22.3.233
34. Alvarez JA, Ashraf A. Role of Vitamin D in Insulin Secretion and Insulin Sensitivity for Glucose Homeostasis. Int J Endocrinol (2010) 2010:351385. doi: 10.1155/2010/351385
35. Pacifico L, Anania C, Osborn JF, Ferraro F, Bonci E, Olivero E, et al. Low 25(OH)D3 Levels Are Associated With Total Adiposity, Metabolic Syndrome, and Hypertension in Caucasian Children and Adolescents. Eur J Endocrinol (2011) 165(4):603–11. doi: 10.1530/EJE-11-0545
36. Motiwala SR, Wang TJ. Vitamin D and Cardiovascular Risk. Curr Hypertens Rep (2012) 14(3):209–18. doi: 10.1007/s11906-012-0262-y
37. Kulda V, Elangovan H, Stokes RA. Vitamin D Metabolism. Vnitr Lek (2012) 58(5):400–4. doi: 10.3390/nu10040496
38. Bikle DD. Vitamin D Metabolism, Mechanism of Action, and Clinical Applications. Chem Biol (2014) 21(3):319–29. doi: 10.1016/j.chembiol.2013.12.016
39. Santos BR, Mascarenhas LP, Satler F, Boguszewski MC, Spritzer PM. Vitamin D Deficiency in Girls From South Brazil: A Cross-Sectional Study on Prevalence and Association With Vitamin D Receptor Gene Variants. BMC Pediatr (2012) 12:62. doi: 10.1186/1471-2431-12-62
40. Alnahas Z, Fawzy M, Menyawi M, Shaker O, Ragab G. 25-Hydroxyvitamin D3 Deficiency and Vitamin D Receptor Polymorphisms in Egyptian Patients With Behçet’s Disease: A Pilot Study. Int J Clin Rheumatol (2017) 12:020. doi: 10.1093/rheumatology/kez060
41. Wu M, Yue M, Huang P, Zhang Y, Xie C, Yu R, et al. Vitamin D Level and Vitamin D Receptor Genetic Variations Contribute to HCV Infection Susceptibility and Chronicity in a Chinese Population. Infect Genet Evol (2016) 41:146–52. doi: 10.1016/j.meegid.2016.03.032
42. Bertoccini L, Sentinelli F, Leonetti F, Bailetti D, Capoccia D, Cimini FA, et al. The Vitamin D Receptor Functional Variant Rs2228570 (C>T) Does Not Associate With Type 2 Diabetes Mellitus. Endocr Res (2017) 42(4):331–5. doi: 10.1080/07435800.2017.1305965
43. Woo J, Lam CW, Leung J, Lau WY, Lau E, Ling X, et al. Very High Rates of Vitamin D Insufficiency in Women of Child-Bearing Age Living in Beijing and Hong Kong. Br J Nutr (2008) 99(6):1330–4. doi: 10.1017/S0007114507844382
44. Barchetta I, Cimini FA, Cavallo MG. Vitamin D and Metabolic Dysfunction-Associated Fatty Liver Disease (MAFLD): An Update. Nutrients (2020) 12(11):3302. doi: 10.3390/nu12113302
45. Jia J, Ding H, Yang K, Mao L, Zhao H, Zhan Y, et al. Vitamin D Receptor Genetic Polymorphism Is Significantly Associated With Risk of Type 2 Diabetes Mellitus in Chinese Han Population. Arch Med Res (2015) 46(7):572–9. doi: 10.1016/j.arcmed.2015.09.006
46. Barchetta I, Cimini FA, Chiappetta C, Bertoccini L, Ceccarelli V, Capoccia D, et al. Relationship Between Hepatic and Systemic Angiopoietin-Like 3, Hepatic Vitamin D Receptor Expression and NAFLD in Obesity. Liver Int (2020) 40(9):2139–47. doi: 10.1111/liv.14554
47. Bid HK, Konwar R, Aggarwal CG, Gautam S, Saxena M, Nayak VL, et al. Vitamin D Receptor (FokI, BsmI and TaqI) Gene Polymorphisms and Type 2 Diabetes Mellitus: A North Indian Study. Indian J Med Sci (2009) 63(5):187–94. doi: 10.4103/0019-5359.53164
48. Khan RJ, Riestra P, Gebreab SY, Wilson JG, Gaye A, Xu R, et al. Vitamin D Receptor Gene Polymorphisms Are Associated With Abdominal Visceral Adipose Tissue Volume and Serum Adipokine Concentrations But Not With Body Mass Index or Waist Circumference in African Americans: The Jackson Heart Study. J Nutr (2016) 146(8):1476–82. doi: 10.3945/jn.116.229963
49. Feng RN, Du SS, Wang C, Li YC, Liu LY, Guo FC, et al. Lean-Non-Alcoholic Fatty Liver Disease Increases Risk for Metabolic Disorders in a Normal Weight Chinese Population. World J Gastroenterol (2014) 20(47):17932–40. doi: 10.3748/wjg.v20.i47.17932
50. Wei JL, Leung JC, Loong TC, Wong GL, Yeung DK, Chan RS, et al. Prevalence and Severity of Nonalcoholic Fatty Liver Disease in Non-Obese Patients: A Population Study Using Proton-Magnetic Resonance Spectroscopy. Am J Gastroenterol (2015) 110(9):1306–14; quiz 1315. doi: 10.1038/ajg.2015.235
51. Rudwill F, Bergouignan A, Gastebois C, Gauquelin-Koch G, Lefai E, Blanc S, et al. Effect of Enforced Physical Inactivity Induced by 60-Day of Bed Rest on Hepatic Markers of NAFLD in Healthy Normal-Weight Women. Liver Int (2015) 35(6):1700–6. doi: 10.1111/liv.12743
52. Fukuda Y, Hashimoto Y, Hamaguchi M, Fukuda T, Nakamura N, Ohbora A, et al. Triglycerides to High-Density Lipoprotein Cholesterol Ratio Is an Independent Predictor of Incident Fatty Liver; A Population-Based Cohort Study. Liver Int (2016) 36(5):713–20. doi: 10.1111/liv.12977
53. Zusi C, Mantovani A, Olivieri F, Morandi A, Corradi M, Miraglia Del Giudice E, et al. Contribution of a Genetic Risk Score to Clinical Prediction of Hepatic Steatosis in Obese Children and Adolescents. Dig Liver Dis (2019) 51(11):1586–92. doi: 10.1016/j.dld.2019.05.029
54. Manolio TA, Collins FS, Cox NJ, Goldstein DB, Hindorff LA, Hunter DJ, et al. Finding the Missing Heritability of Complex Diseases. Nature (2009) 461(7265):747–53. doi: 10.1038/nature08494
55. Wallace HM. A Model of Gene-Gene and Gene-Environment Interactions and Its Implications for Targeting Environmental Interventions by Genotype. Theor Biol Med Model (2006) 3:35. doi: 10.1186/1742-4682-3-35
56. Collins FS, McKusick VA. Implications of the Human Genome Project for Medical Science. JAMA (2001) 285(5):540–4. doi: 10.1001/jama.285.5.540
Keywords: non-alcoholic fatty liver disease (NAFLD), vitamin D (VD) deficiency, 25-hydroxyvitamin D3, vitamin D receptor (VDR), genetic variation, risk assessment
Citation: Zhang R, Wang M, Wang M, Zhang L, Ding Y, Tang Z, Fu Z, Fan H, Zhang W and Wang J (2021) Vitamin D Level and Vitamin D Receptor Genetic Variation Were Involved in the Risk of Non-Alcoholic Fatty Liver Disease: A Case-Control Study. Front. Endocrinol. 12:648844. doi: 10.3389/fendo.2021.648844
Received: 02 February 2021; Accepted: 20 July 2021;
Published: 06 August 2021.
Edited by:
Stephen Atkin, Royal College of Surgeons in Ireland, BahrainReviewed by:
Maximilian Zeyda, Medical University of Vienna, AustriaIlaria Barchetta, Sapienza University of Rome, Italy
Copyright © 2021 Zhang, Wang, Wang, Zhang, Ding, Tang, Fu, Fan, Zhang and Wang. This is an open-access article distributed under the terms of the Creative Commons Attribution License (CC BY). The use, distribution or reproduction in other forums is permitted, provided the original author(s) and the copyright owner(s) are credited and that the original publication in this journal is cited, in accordance with accepted academic practice. No use, distribution or reproduction is permitted which does not comply with these terms.
*Correspondence: Jie Wang, d2FuZ2ppZW5qbXVAMTI2LmNvbQ==
†These authors have contributed equally to this work and share first authorship