- 1Foshan Fetal Medicine Research Institute, Foshan Women and Children Hospital Affiliated to Southern Medical University, Foshan, China
- 2Department of Obstetrics, Foshan Women and Children Hospital Affiliated to Southern Medical University, Foshan, China
Objective: Previously, we found that the presence of maternal serum metals before the 24th week of gestation prospectively increased fasting plasma glucose (FPG) at 24–28 weeks. We further explored the prospective association between levels of metals and neonatal outcomes and assessed the mediating effects of FPG on these relationships.
Methods: A total of 7,644 pregnant women were included in a retrospective cohort study, and the relationships between metals [manganese (Mn), copper (Cu), lead (Pb), zinc (Zn), and magnesium (Mg)] and birth outcomes were explored. Quantile and linear regressions were performed to detect the shifts and associations between metals and neonatal size distribution focused on the 10th, 50th, and 90th percentiles. Mediation analysis was performed to assess the mediating effect of FPG on metals and birth outcomes.
Results: After adjustment, a 50% increase in Mn and Zn levels was related to a 0.136-cm (95% CI: 0.067–0.205) and 0.120-cm (95% CI: 0.046–0.193) increase in head circumference, respectively. Based on head circumference distribution, the magnitude of the association with Mn was smaller at the upper tail, while the magnitude of correlation with Zn was greater at the upper tail. A 50% increase in Mn and Zn levels was related to a 0.135-cm (95% CI: 0.058–0.212) and 0.095-cm (95% CI: 0.013–0.178) increase in chest circumference, respectively. The magnitude of the association with Mn increased with increasing chest circumference, while the magnitude of correlation with Zn decreased with increasing chest circumference. FPG explained 10.00% and 17.65% of the associations of Mn with head and chest circumference. A positive indirect effect of Zn associated with head circumference (0.004, 95% CI: 0.002–0.006) and chest circumference (0.005, 95% CI: 0.003–0.008) through FPG was also observed, and the estimated proportion of the mediating effect was 13.79% and 26.32%, respectively.
Conclusion: Maternal serum Mn and Zn levels before the 24th week of gestation may prospectively increase the circumference of the neonatal head and chest. FPG at 24–28 weeks had positive mediating effects on these relationships. Further research is needed to identify a balance between maternal blood glucose and birth size.
Introduction
Environmental exposure including metals in pregnant women has been associated with health effects in both the mother and her offspring (1, 2). Such metals can enter the maternal body through air, water, food, and other forms of exposure and, thus, have an impact on health (3). Moreover, the metals can have greater health implications on the offspring through the mother as fetal organs and systems are in a critical period of plasticity and sensitivity to the environment (4). Metal exposure has been related to fetal growth in the short term, and it could also result in various health problems in the long term, such as neurodevelopment and cardiovascular disease (5, 6).
Pregnant women are in a critically important state for susceptibility to metal effects because of hemodynamics, hormone changes, and immature immune systems (7), and metals could further affect fetal growth after passing through the placental barrier (8). Studies have found that prenatal exposure to certain ranges of manganese (Mn) (9), copper (Cu) (10), lead (Pb) (11), zinc (Zn) (12), and magnesium (Mg) (13) is linked to adverse birth outcomes, including small-for-gestational-age (SGA) births, low birth weight, and preterm delivery. Similarly, low-level prenatal Pb exposure, as well as elevated Mn and Zn levels, might increase the risk of preterm birth and SGA (14). Moreover, increasing concentrations of Mn have been linked to decreasing birth weight (15). However, the associations between these metals and birth outcomes have been inconsistent. Increasing placental Mn could slightly increase neonatal head circumference in females (16). Pb has been shown to elevate the risk of SGA in infants (11) and was inversely associated with birth weight, birth length, and head circumference in observational studies (17). Furthermore, Mg supplementation during pregnancy might increase birth weight (13). Moreover, prenatal exposure to Pb and Mn has been assessed as showing no relation to birth weight and length (8). Additionally, there seems to be an optimal range of exposure to some metals; for instance, both low and high levels of Mn are associated with lower birth weight and smaller head circumference (18). Meanwhile, an optimum level of Zn and Cu in pregnant women could reduce the risk of low birth weight (19).
Fasting plasma glucose (FPG) is predominantly produced following the decomposition of liver glycogen, while basal insulin secretion can inhibit the output of liver glycogen and prevent higher FPG. Therefore, the level of fasting blood glucose can objectively reflect the secretion level of basal insulin in patients. Elevated metal levels can accumulate in pancreatic tissue where they may contribute to insulin resistance or damage islet β cells and impair insulin secretion (20). Mn has been shown to inhibit glucose-stimulated insulin secretion in β cells (21), while higher urinary Zn or Pb could increase FPG of Chinese adults (22). In addition, serum Mg levels have been negatively associated with fasting insulin, and higher copper concentrations could increase the risk of glucose dysregulation during pregnancy (23, 24). Our previous study (25) also found that metals including Mn, Cu, Pb, Zn, and Mg in early pregnancy were prospectively related to later FPG in pregnant women. Moreover, high FPG levels have been linked to macrosomia and large-for-gestational-age infants (26). It has also been revealed that correlations between postprandial blood glucose and primary outcomes are weaker than for FPG (27). Nevertheless, we found no relevant studies that clarify the relationship among metals, FPG, and birth outcomes, especially in a longitudinal direction of complete pregnancy.
Therefore, after combining our previous study with those of others, we hypothesized that earlier metal exposure in pregnant women was prospectively associated with final birth outcome, and later FPG mediated their relationship. Here, we tested this hypothesis in a retrospective and longitudinal population-based cohort study to explore i) whether maternal early metal exposure including Mn, Cu, Pb, Zn, and Mg before 24 weeks was related to birth outcomes and ii) whether a maternal FPG of 75 g oral glucose tolerance test (OGTT) at 24–28 weeks mediated the relationship between metal exposure and birth outcomes.
Methods
Study Population
The retrospective cohort study was conducted between January 2017 and December 2018 in Foshan, China. Pregnant women were recruited at the first obstetric clinic visit in the Southern Medical University Affiliated Foshan Women and Children Hospital. The study was approved by the Human Subjects Committee of our hospital. The inclusion criteria were as follows: 1) singleton pregnancy, 2) no diabetes prior to pregnancy, and 3) gestational age in the first trimester (<14 weeks). The exclusion criteria were as follows: 1) no available data on metal detection before 24 weeks of pregnancy (<24 weeks), 2) missing data of OGTT at 24–28 weeks of gestation, and 3) stillbirth or without delivery data in our hospital. Of 11,845 women who initially met the above inclusion criteria, 7,646 were ultimately included for the study (Figure S1).
Data Collection and Definition of Variables
Medical data were collected from hospital computerized databases or clinical charts. The information of maternal demographic characteristics included maternal age, employment status, maternity insurance, family history of diabetes, family history of hypertension, smoking habit, and drinking habit. The clinical history information included parity, and prenatal visit records consisted of gestational age and BMI at the first prenatal examination in the first trimester; gestational age for metal detection; serum levels of the metals Mn, Cu, Pb, Zn, and Mg; OGTT results at 24–28 weeks; diagnosis of gestational diabetes mellitus (GDM) and hypertensive disorders of pregnancy (HDP); and weight gain during the whole pregnancy. The delivery records (e.g., gestational age at delivery, neonatal sex, birth weight, birth length, head and chest circumference) were retrieved. All identifying patient information was anonymized to protect patient identity.
The diagnostic criteria for GDM referred to the criteria of the International Association for Diabetes in Pregnancy Study Group (IADPSG): pregnant women were given OGTT at 24–28 weeks. The results were classified based on one of the following cutoff points: fasting plasma glucose (FPG, OGTT0) ≥5.1 mmol/L or 1-h PG (1-h postprandial plasma glucose, OGTT1) ≥10.0 mmol/L or 2-h PG (2-h postprandial plasma glucose, OGTT2) ≥8.5 mmol/L (28). First-trimester BMI (kg/m2) was divided into categories according to Chinese BMI criteria for general adults: BMI < 18.5 kg/m2, 18.5 kg/m2 ≤ BMI < 24 kg/m2, 24 kg/m2 ≤ BMI < 28 kg/m2, and BMI ≥ 28 kg/m2 (29). Hypertensive disorders of pregnancy (HDP) included gestational hypertension, preeclampsia, eclampsia, superimposed preeclampsia on chronic hypertension, and chronic hypertension in pregnancy (30).
Measurement of Serum Metal Concentration
Metal detection was performed according to the methods described in our previous article (25). Maternal serum metal concentration was assessed only once before 24 weeks of pregnancy in our hospital. Peripheral venous blood samples (2 ml) were drawn in the obstetric clinic and then transported to the Department of Laboratory Medicine of the hospital within 1 h for serum metal detection, using the polarography method (AS-9000 C, AWSA, Wuhan, China). The detection limit of the polarographic channel was ≤1 × 10−8 mol/L. All of the metal measurements were above the limit. The coefficient of variation (CV) of detection was ≤1%, and the relative error (B) of detection was ≤1%.
Statistical Analysis
Baseline characteristics were described as mean ± SD or number (%). In the analysis of birth outcomes, maternal serum metal levels were categorized into three parts according to tertiles. Comparisons for birth outcomes among three groups of metals used ANOVA, and the pairwise comparisons were conducted using Dunnett with a control category of the 1st tertile. The analyses were conducted using SPSS 24.0 software.
Quantile regression was performed to detect the distribution shifts of neonatal size (e.g., head circumference) and to explore the associations with metal levels primarily occurring at the tails of neonatal size. In particular, the 10th, 50th, and 90th percentiles of neonatal size were focused on. The metal linear regression was established for each percentile and the mean of neonatal size. Metal level was log-transformed based on a 1.5 logarithm function, assuring that 1-unit change on the transformed scale was close to or within the IQR. One-unit difference on the natural log-transformed scale corresponded to a much wider range than the IQR on the original range (24). The models were adjusted for the following factors: maternal age, employment status, parity, maternity insurance, family history of diabetes, family history of hypertension, HDP, GDM, BMI, gestational age for metal detection, weight gain during pregnancy, infant sex, and gestational age at delivery. These analyses were performed in R 3.5.2, using the packages quantreg and forestplot in quantile regression.
In our previous study (25), we found significant associations between Mn, Cu, Pb, Zn, and Mg and OGTT0. Thus, we aimed to further explore the mediating effect of OGTT0 on metals and birth outcomes. Mediation analysis, one model of the structural equation modeling, was performed using the robust maximum-likelihood estimation. Standardized coefficients of direct, indirect, and total effects were estimated, and the 95% CI was measured using the bootstrap method with 2,000 resamplings. The model was adjusted for the following factors: maternal age, employment status, parity, maternity insurance, family history of diabetes, family history of hypertension, HDP, BMI, gestational age for metal detection, weight gain during pregnancy, infant sex, and gestational age at delivery. The mediation proportion of indirect effect was calculated as follows: Estimated mediated = Indirect effect/Total effect × 100%. Total effect = Direct effect + Indirect effect. The data were analyzed using Mplus 7.4 (Muthén and Muthén). A two-sided P-value <0.05 was considered to indicate statistical significance.
Results
Baseline Characteristics
Tables 1 and S1 show the characteristics of our study population. The mean maternal age was 30.13 years. Of the pregnant participants, 55.45% were employed and 67.51% of those had maternity insurance. None of the participants had smoking or drinking habits. Only 0.47% of women had a family history of diabetes. However, the incidence of GDM was 15.93%. The mean BMI in the first trimester was 21.38 kg/m2, and the mean weight gain during the whole of pregnancy was 12.70 kg. The mean gestational age at delivery was 38.47 weeks. The mean birth weight, birth length, head circumference, and chest circumference of the newborns were 3,155.13 g, 49.12 cm, 32.89 cm, and 32.70 cm, respectively.
Distribution of Metals and the Association With Birth Outcomes
The mean gestational age of metal detection was 16.56 weeks (Table 1). Table S2 shows the geometric mean and percentiles of the levels of five metals. Results from metals classified into tertiles indicated that head circumference in the second and third tertiles of Mn or Zn was significantly larger than that in the first tertile (P < 0.05) (Table 2). Chest circumference in the second and third tertiles of Mn was also significantly larger than that in the first tertile (P < 0.05). No significant difference of gestational age, birth weight, or birth length was observed among the three tertiles of all five metals.
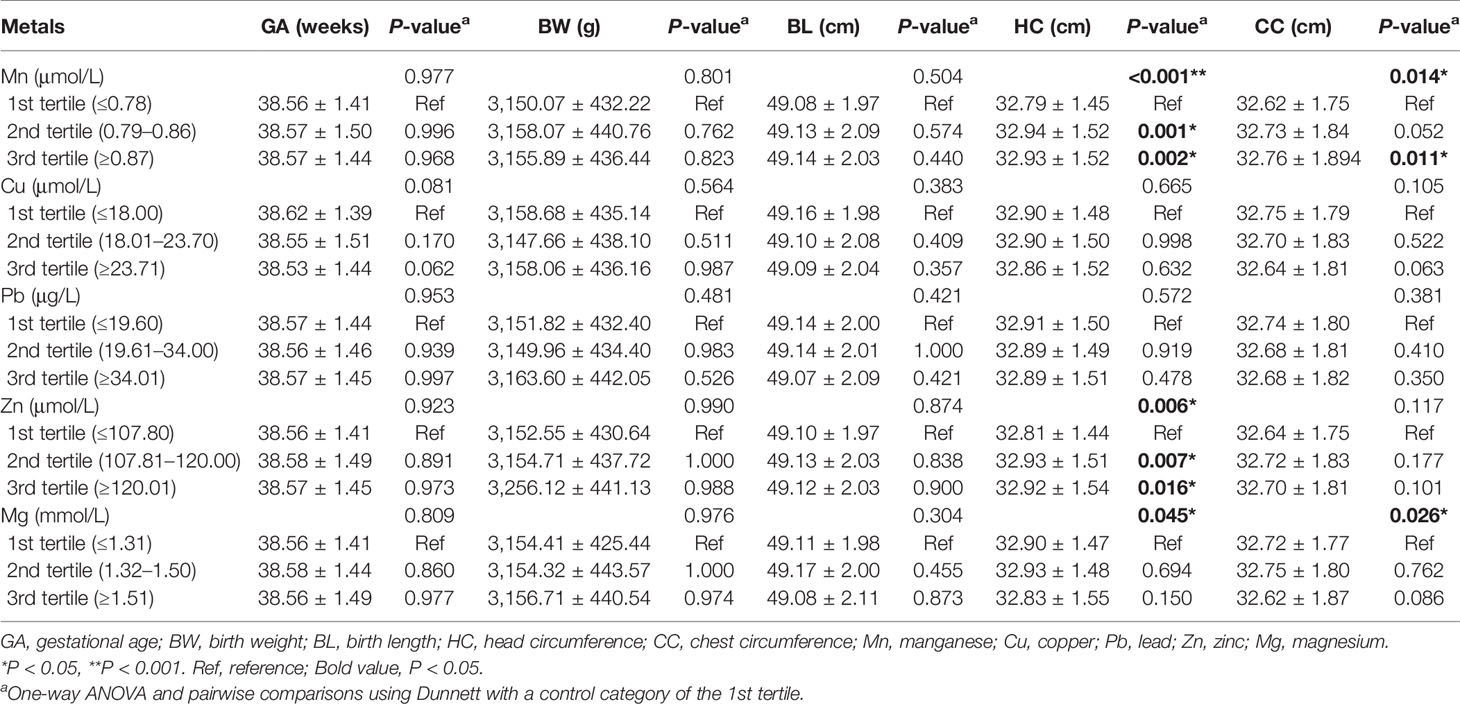
Table 2 Association between metal concentrations and birth outcomes based on three different metal levels.
In the further analysis, we explored the associations between metals and the distribution shifts of head and chest circumference. As shown in Figure 1, a 50% increase in Mn and Zn levels was related to a 0.136-cm (95% CI: 0.067–0.205) and 0.120-cm (95% CI: 0.046–0.193) increase in mean head circumference after adjustment, respectively. Based on the 10th, 50th, and 90th percentiles of head circumference distribution, the magnitude of the association with Mn was smaller at the upper tail, while the magnitude of correlation with Zn was greater at the upper tail. However, no significant association was found between Pb, Cu, and Mg levels and head circumference distribution.
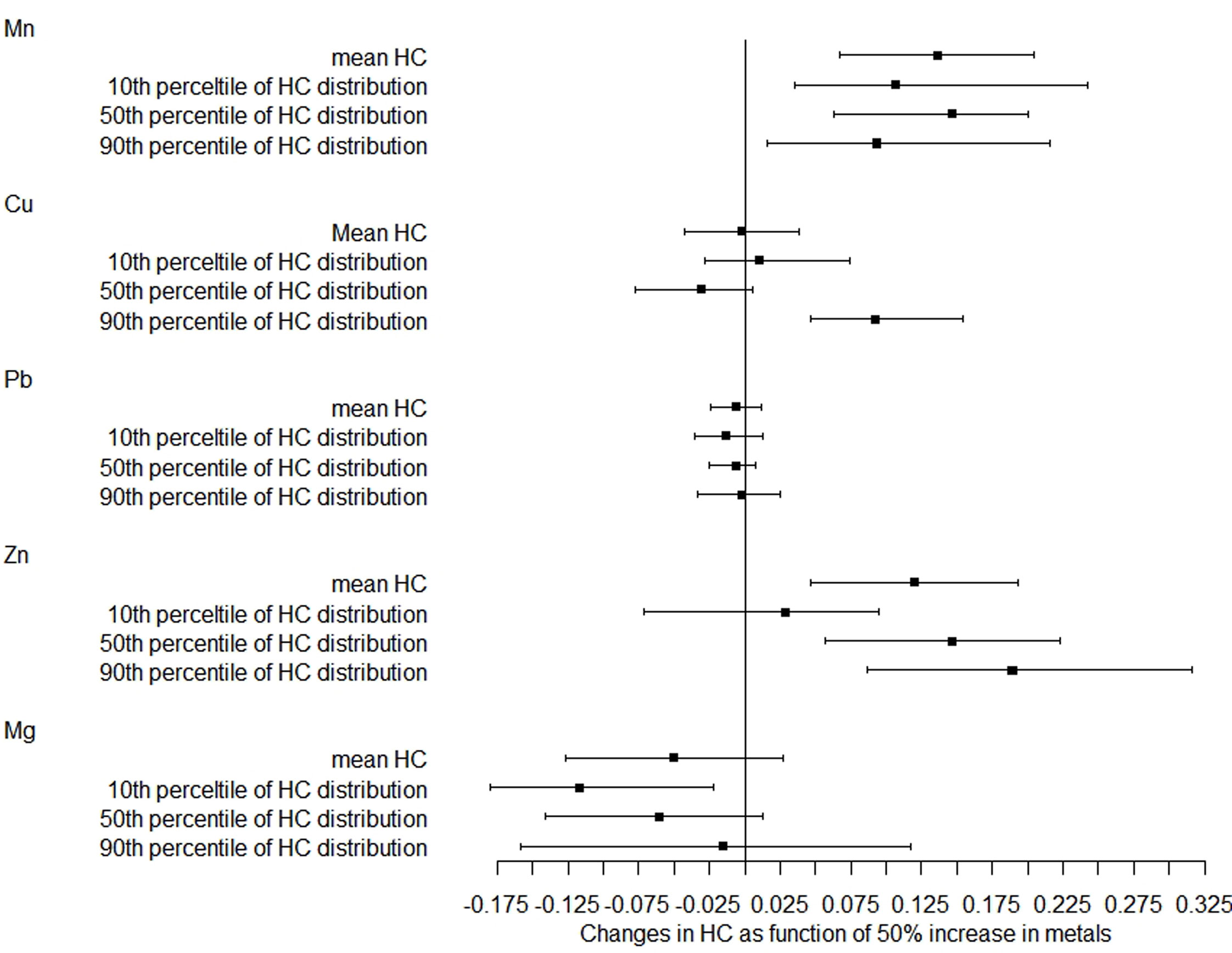
Figure 1 Distribution changes in head circumference as a function of 50% increase in metals. The models were adjusted for maternal age, employment status, parity, maternity insurance, family history of diabetes, family history of hypertension, HDP, GDM, BMI, gestational age for metal detection, weight gain during pregnancy, infant sex, and gestational age at delivery. Mn, manganese; Cu, copper; Pb, lead; Zn, zinc; Mg, magnesium; HC, head circumference.
The correlations of metals with chest circumference were similar to those of head circumference (Figure 2). After adjustment, a 50% increase in Mn and Zn levels was related to a 0.135-cm (95% CI: 0.058–0.212) and 0.095-cm (95% CI: 0.013–0.178) increase in mean chest circumference, respectively. The magnitude of the association with Mn was increased with the increase of the 10th, 50th, and 90th percentiles of chest circumference distribution, while the magnitude of correlation with Zn was decreased with the increase of the distribution shifts. There was also no relationship between Pb, Cu, and Mg concentrations and chest circumference distribution.
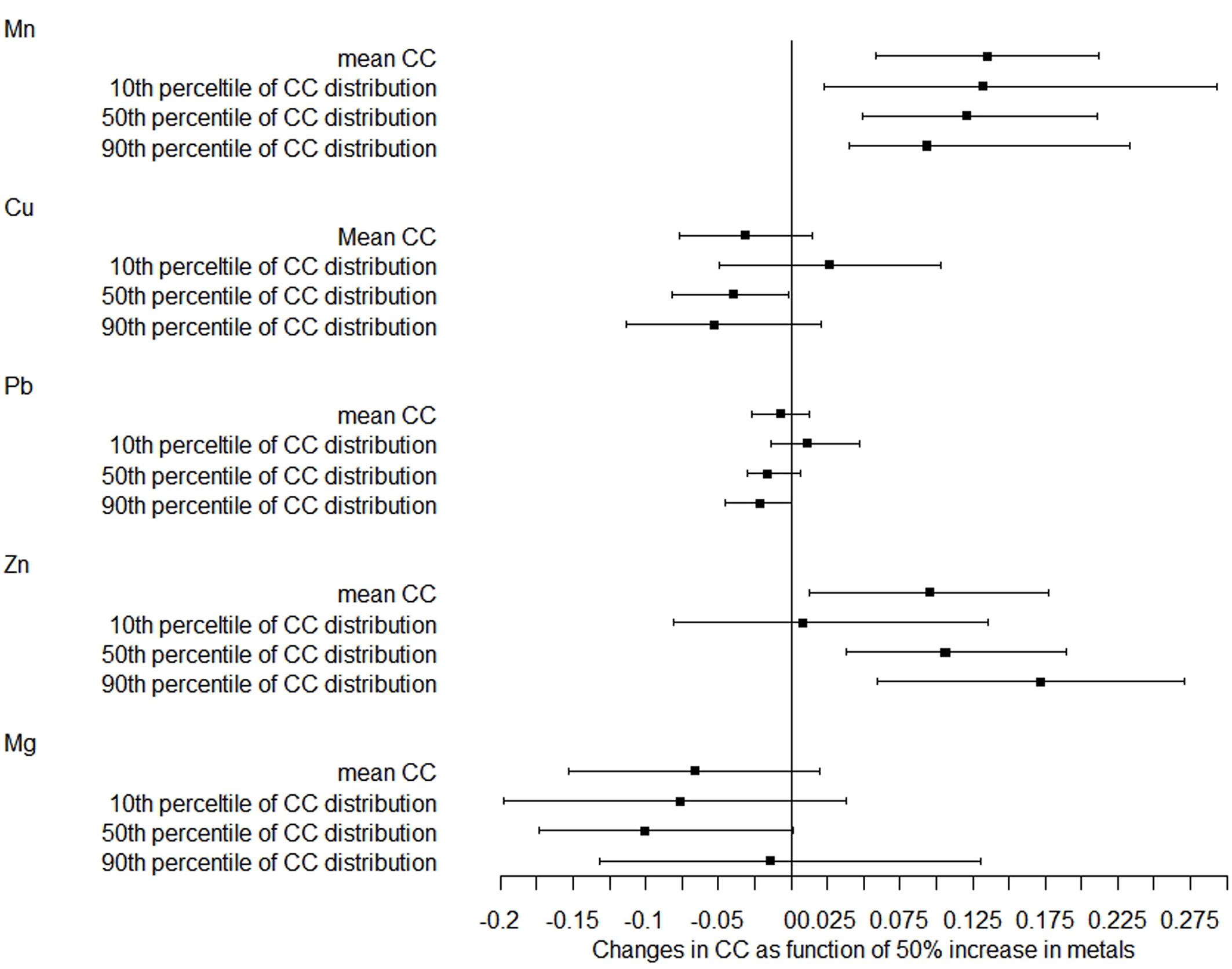
Figure 2 Distribution changes in chest circumference as a function of 50% increase in metals. The models were adjusted for maternal age, employment status, parity, maternity insurance, family history of diabetes, family history of hypertension, HDP, GDM, BMI, gestational age for metal detection, weight gain during pregnancy, infant sex, and gestational age at delivery. Mn, manganese; Cu, copper; Pb, lead; Zn, zinc; Mg, magnesium; CC, chest circumference.
Mediating Effects of OGTT0 on Metals and Head and Chest Circumference
Because of the significant correlations of Mn/Zn and head/chest circumference, mediation analysis was performed to explore the mediating effects of OGTT0 (Figure 3). After controlling for the influence of covariates, the results showed that OGTT0 level explained 10.00% (indirect effect: 0.004, 95% CI: 0.003–0.007, P < 0.001) and 17.65% (indirect effect: 0.006, 95% CI: 0.004–0.009, P < 0.001) for the associations of serum Mn concentration with head and chest circumference. A positive indirect effect of Zn association with head circumference (0.004, 95% CI: 0.002–0.006, P < 0.001) and chest circumference (0.005, 95% CI: 0.003–0.008, P < 0.001) through OGTT0 level was also observed, and the estimated proportions of the mediating effect were 13.79% and 26.32%, respectively. In conclusion, there were positive correlations between maternal serum Mn and Zn levels and neonatal head and chest circumference, and these positive correlations were mediated by OGTT0 glucose level.
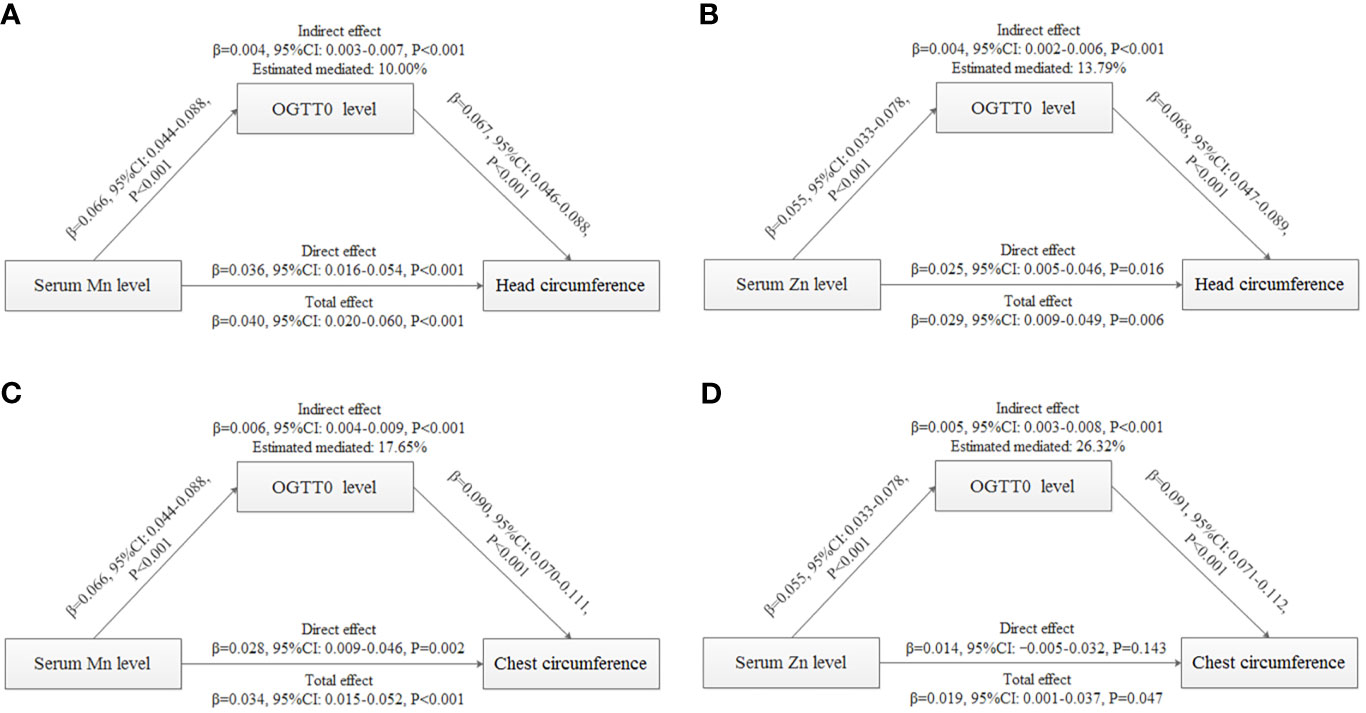
Figure 3 Mediation analysis with standardized coefficients of metals, OGTT0, and birth outcomes. (A) Analysis of serum Mn level, OGTT0 level, and head circumference. (B) Analysis of serum Zn level, OGTT0 level, and head circumference. (C) Analysis of serum Mn level, OGTT0 level, and chest circumference. (D) Analysis of serum Zn level, OGTT0 level, and chest circumference. The model was adjusted for maternal age, employment status, parity, maternity insurance, family history of diabetes, family history of hypertension, HDP, BMI, gestational age for metal detection, weight gain during pregnancy, infant sex, and gestational age at delivery. All models were found to be statistically significant (P < 0.001).
Discussion
The current study showed that maternal serum Mn and Zn levels before 24 weeks of gestation could prospectively increase neonatal head and chest circumference. For the head circumference, the magnitude of the association with Mn was smaller at the upper tail, while the magnitude of correlation with Zn was greater at the upper tail. For the chest circumference, the magnitude of the association with Mn was greater at the upper tail, while the magnitude of correlation with Zn was smaller at the upper tail. In addition, FPG at 24–28 weeks mediated the positive effect of Mn or Zn exposure on head or chest circumference. No relationships between Mn and Zn and other birth outcomes including gestational age, birth weight, and birth length were found. No correlation between Pb, Cu, and Mg and birth outcomes was observed.
To date, several studies have explored the association between Mn exposure and birth outcome. However, only a few articles report a prospective and positive relationship between early maternal Mn level and neonatal birth size and the mediating effect of FPG on them. Maternal blood Mn has previously been shown to be positively related to neonatal head circumference, but not to birth weight and birth length (31), which is consistent with our findings. However, our present results were contrary to some other published studies, as follows: maternal Mn level in erythrocytes in the second trimester has been demonstrated to be negatively linked to birth weight and head and chest circumferences (32). This might have contributed to a slightly higher exposure to environmental Mn among pregnant women living in this region (geometric mean Mn in our study: 0.81 μmol/L vs. 0.72 μmol/L in theirs). Furthermore, elevated umbilical cord blood Mn may decrease birth weight, but was not related to head circumference and birth length (15). Moreover, maternal serum Mn level was not associated with birth weight, but was negatively linked to head circumference (33). Although some studies have also found a positive correlation between metals and birth size, the results were different from ours. A previous report described that maternal Mn was related to higher birth length (34). Also, maternal hair but not blood Mn concentrations showed a positive linear association with infant chest circumference (35). This may be because the amount of Mn might vary in different parts of the body, leading to different correlations between Mn and birth outcomes. In addition, considering that the rapid increase in fetal body weight during late pregnancy is associated with an accelerated growth of fetal peripheral muscle and fat deposition, neonatal birth weight might be more affected by the intrauterine environment, including nutritional supplementation from the mother during pregnancy, than neonatal head and chest circumference (36). Neonatal head and chest growth might be more susceptible to metal exposure in early pregnancy than birth weight and length; however, birth weight and length might be more susceptible to the combined effects of maternal nutrition and maternal and paternal height and genes in late pregnancy. We cautiously assumed that the combined effects in late pregnancy might be stronger than the effect of Mn in early pregnancy and, thus, masked the effect of metals. In future studies, it will be important to further explore the relationship between neonatal birth size and metals by integrating nutrition, genes, and other factors during pregnancy.
The results of the current study further showed that maternal Mn might affect the birth outcome by influencing FPG during pregnancy. Mn, both an essential element and a potential toxicant, is a component of several enzymes and an activator for enzymes. Deficiency of Mn might affect growth and development of the body and glucose metabolism, and excessive intake of Mn could cause poisoning (37). Mn is suggested to inhibit glucose-stimulated insulin secretion in β cells by impairing mitochondrial function (21). One population-based study has shown a positive relationship of urinary Mn with hyperglycemia risk in Chinese coke oven workers (38). Meanwhile, our previous study found that early maternal serum Mn could increase FPG at 24–28 weeks (25).
Larger offspring size has been attributed mainly to higher pregnancy glucose levels. We made a cautious hypothesis that the long-term effects of fetal glucose exposure could affect fetal β-cell capacity, especially exposure to higher FPG, and thus, higher maternal FPG might indirectly affect neonatal birth outcomes. Therefore, FPG might mediate the effect of Mn level on birth outcome. However, there are currently no metal requirement guidelines for pregnant women. The Mn level of our study population was slightly higher than that found in other populations, and serum Mn levels in most pregnant women in our study were below the lower limit of the reference range (0.15–0.22 μmol/L) for healthy adults (39). Mn requirements for pregnant women greatly increase as the fetus grows (40); however, this high demand might impair the function of the islets and cause elevated blood glucose. Further research is needed to investigate the balance between maternal blood glucose and neonatal size.
Zn is a component of zinc-containing metalloenzymes involved in the activity of more than 80 enzymes. Zn is also an important immunomodulator and growth cofactor and plays an important role in anti-oxidation, anti-apoptosis, and anti-inflammation. About 90% of Zn in the body is distributed in muscles and bone (19). Blood Zn concentration is about 0.1~0.15 mmol/L in the general population (41), and our study population showed similar levels. Previous studies have reported that higher Zn levels in early pregnancy could increase head circumference (34), and prenatal Zn has been associated with greater chest circumference (42), which are consistent with our findings. Zn supplementation is also beneficial to head growth rate in infants (43). However, one report also states that maternal Zn level was inversely related to birth weight (12). It could be argued that the discordant results are owing to the different levels of original Zn exposure and different study populations with different underlying diseases affecting fetal growth. Head circumference in early life is an important indicator of brain development. Furthermore, it could affect neuronal growth and brain function (19). Brain development is incredibly complex and takes place in two major stages: the first 20 weeks involve fetal organogenesis and neurogenesis, and during weeks 20–40, there is continued neuronal growth, neural migration, and maturation. Brain development is very rapid, from 32 to 39 weeks when the brain can come to weigh 30% as much as an adult (44). In our study, metal exposure before 24 weeks of gestation had significant implications for the two stages of fetal brain development. Rapid brain growth increases vulnerability to unfavorable environmental conditions. In our study, although the head circumference increased slightly with the increase of Zn concentration, it also had some clinical significance. Large population studies are needed to explore the relationship between small differences in neonatal head circumference and brain function. In addition, Zn and Mn were related to birth head and chest circumference but had no relationship with birth weight and length. This might be associated with neonatal birth size as Zn has the same effect as described for Mn above. Zn is also an essential trace element for insulin synthesis, storage, and release (45). However, excess levels of Zn during embryogenesis could disturb biochemical processes and could be teratogenic or ultimately fatal (19). We found that Zn prospectively increased late second-trimester FPG in our previous study (25) and Zn could further increase the head and chest circumference through higher levels of FPG. The relationship of Zn among the three (Zn, FPG, and birth outcome) might be similar to that of Mn. These findings require further epidemiological and molecular studies in both the fetal stage and the childhood stage.
Our current study found no association between Pb and birth outcomes, which is consistent with a previous study (15). However, Pb has been reported to decrease birth weight and head circumference following fetal lead exposure during pregnancy and lactation (46, 47). The normal range of Pb in the general population is <50 μg/L (48), and the Pb levels of our participants were within the normal range. Therefore, Pb level in the normal range might not be associated with birth outcomes, but higher levels above the normal range might have toxic effects on neonatal birth size.
Excess Cu is harmful to human health, but it is rarely excessive in the general population (49), and the demand for Cu is significantly increased in pregnant women (19). Cu deficiency during pregnancy might result in oxidative stress that can reduce fetal growth (19). Serum Cu <350 μg/L (5.51 μmol/L) is linked to conditions of Cu deficiency (50). The minimum concentration in our study population was 7.60 μmol/L. Therefore, we speculated with caution that a significant association between maternal Cu exposure and adverse birth outcomes might not be observed at normal concentrations. The underlying mechanisms need further exploration.
The reference range for serum Mg in adults is 0.75 mmol/L (5% CI: 0.45, 1.05) (51); our study population had a slightly higher level. There is an increasing need for Mg throughout pregnancy, and Mg supplementation might have neuroprotective effects on preterm infants (52). However, limited studies have reported the association between Mg and birth size. Most prenatal Mg supplementation is given in the third trimester, in order to protect neurologic function in preterm infants. Although we found that Mg in early pregnancy was not associated with birth outcome, an appropriate Mg range should be established for pregnant women. Further studies are needed to determine whether Mg in early pregnancy is prospectively related to neurodevelopment of newborns in the long term.
The current study was the first study to show that metal exposure in early pregnancy could affect FPG in the late second trimester and, thus, affect neonatal head and chest circumference. This information may promote new regimens for the personalized control and management of birth size during pregnancy. The study sample size was also relatively large, which increased the accuracy of the findings and made them more applicable to other populations. However, some limitations in the study should be noted. First, despite the large sample size, none of the pregnant women in our study had a smoking or drinking habit. This limits the value of the study. Smoking and drinking habits were investigated in each pregnant woman during prenatal visits as they are risk factors for adverse pregnancy and birth outcomes, not just for the birth size of the newborn. This could be a good habit of women in southern China, although it is impossible to rule out a few cases of concealment. Second, our research was a single-center study; multicenter studies are needed in the future to make the results more precise and reliable. Third, data related to trace element supplementation during pregnancy in this study were not available, which might be a confounding factor affecting the results. We are currently conducting a prospective birth cohort study, aiming to collect more detailed and accurate data. Fourth, the study only examined maternal metal exposure in early pregnancy before 24 weeks, not in the third trimester, placenta, or umbilical cord blood. Metal requirements for pregnant women might vary in different trimesters, and the placental barrier might support different metal effects between mother and offspring. Further epidemiological and molecular studies should be conducted.
In conclusion, our study suggests that maternal serum Mn and Zn levels in early pregnancy may prospectively increase neonatal head and chest circumference. FPG in the late second trimester could positively mediate the association of Mn and Zn exposure with head or chest circumference. However, maternal Mn and Zn levels in early pregnancy were not associated with birth weight and birth length. Further studies should explore the relationship between neonatal birth size and metals by integrating maternal nutrition, living habits, the genes of both parents, and other factors throughout pregnancy. In addition, further research is needed to find a balance between maternal blood glucose and neonatal birth size; the corresponding epidemiological and molecular studies need to explore and reveal the molecular mechanisms.
Data Availability Statement
The raw data supporting the conclusions of this article will be made available by the authors, without undue reservation.
Ethics Statement
The studies involving human participants were reviewed and approved by the Ethics Committee of the Southern Medical University Affiliated Foshan Women and Children Hospital (FSFYMEC-2019-024). Written informed consent for participation was not required for this study in accordance with the national legislation and the institutional requirements.
Author Contributions
ZZ, DS, FW and ZL conceived and designed the study. ZZ, DY, GC, PL, LW, JY, DL, JR, DF, XG, HW and XG carried out data collection. ZZ carried out the statistical analyses and drafted the manuscript. All authors contributed to the article and approved the submitted version.
Funding
This work was supported by the Basic and Applied Basic Research Foundation of Guangdong Province (Nos. 2019A1515110163 and 2019A1515111011), the Medical Science and Technology Foundation of Guangdong Province (No. C2019090), the Medical Research Foundation of Guangdong Province (No. A2019214), and the Foundation of Science and Technology Agency of Foshan City (Nos. 1920001000248, 1920001000294, and 20200027).
Conflict of Interest
The authors declare that the research was conducted in the absence of any commercial or financial relationships that could be construed as a potential conflict of interest.
Publisher’s Note
All claims expressed in this article are solely those of the authors and do not necessarily represent those of their affiliated organizations, or those of the publisher, the editors and the reviewers. Any product that may be evaluated in this article, or claim that may be made by its manufacturer, is not guaranteed or endorsed by the publisher.
Supplementary Material
The Supplementary Material for this article can be found online at: https://www.frontiersin.org/articles/10.3389/fendo.2021.763693/full#supplementary-material
Supplementary Figure 1 | Follow charts of study population.
References
1. Stratakis N, Golden-Mason L, Margetaki K, Zhao Y, Valvi D, Garcia E, et al. In Utero Exposure to Mercury Is Associated With Increased Susceptibility to Liver Injury and Inflammation in Childhood. Hepatology (2021) 74(3):1546–59. doi: 10.1002/hep.31809
2. Liu J, Martin LJ, Dinu I, Field CJ, Dewey D, Martin JW. Interaction of Prenatal Bisphenols, Maternal Nutrients, and Toxic Metal Exposures on Neurodevelopment of 2-Year-Olds in the APrON Cohort. Environ Int (2021) 155:106601. doi: 10.1016/j.envint.2021.106601
3. Nordberg GF, Fowler BA, Nordberg M. Handbook on the Toxicology of Metals. London, London: Elsevier B.V (2014).
4. Barker DJP. The Developmental Origins of Adult Disease. Eur J Epidemiol (2003) 18(8):733–6. doi: 10.1023/a:1025388901248
5. Valeri L, Mazumdar MM, Bobb JF, Claus Henn B, Rodrigues E, Sharif OIA, et al. The Joint Effect of Prenatal Exposure to Metal Mixtures on Neurodevelopmental Outcomes at 20-40 Months of Age: Evidence From Rural Bangladesh. Environ Health Perspect (2017) 125(6):067015. doi: 10.1289/EHP614
6. Eriksson JG, Forsén T, Tuomilehto J, Osmond C, Barker DJ. Early Growth and Coronary Heart Disease in Later Life: Longitudinal Study. BMJ (Clinical Res ed.) (2001) 322(7292):949–53. doi: 10.1136/bmj.322.7292.949
7. Shih YH, Chen HY, Christensen K, Handler A, Turyk ME, Argos M. Prenatal Exposure to Multiple Metals and Birth Outcomes: An Observational Study Within the National Children's Study Cohort. Environ Int (2021) 147:106373. doi: 10.1016/j.envint.2020.106373
8. Li A, Zhuang T, Shi J, Liang Y, Song M. Heavy Metals in Maternal and Cord Blood in Beijing and Their Efficiency of Placental Transfer. J Environ Sci (China) (2019) 80:99–106. doi: 10.1016/j.jes.2018.11.004
9. Ashley-Martin J, Dodds L, Arbuckle TE, Ettinger AS, Shapiro GD, Fisher M, et al. Maternal and Cord Blood Manganese (Mn) Levels and Birth Weight: The MIREC Birth Cohort Study. Int J Hyg Environ Health (2018) 221(6):876–82. doi: 10.1016/j.ijheh.2018.05.015
10. Kim SS, Meeker JD, Carroll R, Zhao S, Mourgas MJ, Richards MJ, et al. Urinary Trace Metals Individually and in Mixtures in Association With Preterm Birth. Environ Int (2018) 121(Pt 1):582–90. doi: 10.1016/j.envint.2018.09.052
11. Wang H, Li J, Hao JH, Chen YH, Liu L, Yu Z, et al. High Serum Lead Concentration in the First Trimester Is Associated With an Elevated Risk of Small-for-Gestational-Age Infants. Toxicol Appl Pharmacol (2017) 332:75–80. doi: 10.1016/j.taap.2017.07.020
12. Luo J, Wu W, Zhang P, Chen X, Feng Y, Ma N, et al. Zinc Levels and Birth Weight in Pregnant Women With Gestational Diabetes Mellitus: A Matched Cohort Study in China. J Clin Endocrinol Metab (2020) 105(7). doi: 10.1210/clinem/dgaa171
13. Makrides M, Crosby DD, Bain E, Crowther CA. Magnesium Supplementation in Pregnancy. Cochrane Database Syst Rev (2014) 2014(4):Cd000937. doi: 10.1002/14651858.CD000937.pub2
14. Ashrap P, Watkins DJ, Mukherjee B, Boss J, Richards MJ, Rosario Z, et al. Maternal Blood Metal and Metalloid Concentrations in Association With Birth Outcomes in Northern Puerto Rico. Environ Int (2020) 138:105606. doi: 10.1016/j.envint.2020.105606
15. Lee MS, Eum KD, Golam M, Quamruzzaman Q, Kile ML, Mazumdar M, et al. Umbilical Cord Blood Metal Mixtures and Birth Size in Bangladeshi Children. Environ Health Perspect (2021) 129(5):57006. doi: 10.1289/EHP7502
16. Freire C, Amaya E, Gil F, Murcia M, Llop S, Casas M, et al. Placental Metal Concentrations and Birth Outcomes: The Environment and Childhood (INMA) Project. Int J hygiene Environ Health (2019) 222(3):468–78. doi: 10.1016/j.ijheh.2018.12.014
17. Luo Y, McCullough LE, Tzeng J-Y, Darrah T, Vengosh A, Maguire RL, et al. Maternal Blood Cadmium, Lead and Arsenic Levels, Nutrient Combinations, and Offspring Birthweight. BMC Public Health (2017) 17(1):354. doi: 10.1186/s12889-017-4225-8
18. Eum J-H, Cheong H-K, Ha E-H, Ha M, Kim Y, Hong Y-C, et al. Maternal Blood Manganese Level and Birth Weight: A MOCEH Birth Cohort Study. Environ Health Global Access Sci Source (2014) 13(1):31. doi: 10.1186/1476-069X-13-31
19. Grzeszczak K, Kwiatkowski S, Kosik-Bogacka D. The Role of Fe, Zn, and Cu in Pregnancy. Biomolecules (2020) 10(8):1176. doi: 10.3390/biom10081176
20. Hectors TLM, Vanparys C, van der Ven K, Martens GA, Jorens PG, Van Gaal LF, et al. Environmental Pollutants and Type 2 Diabetes: A Review of Mechanisms That can Disrupt Beta Cell Function. Diabetologia (2011) 54(6):1273–90. doi: 10.1007/s00125-011-2109-5
21. Dover EN, Patel NY, Stýblo M. Impact of In Vitro Heavy Metal Exposure on Pancreatic β-Cell Function. Toxicol Lett (2018) 299:137–44. doi: 10.1016/j.toxlet.2018.09.015
22. Hribal ML, Feng W, Cui X, Liu B, Liu C, Xiao Y, et al. Association of Urinary Metal Profiles With Altered Glucose Levels and Diabetes Risk: A Population-Based Study in China. PloS One 10(4):e0123742. doi: 10.1371/journal.pone.0123742
23. Spiga R, Mannino GC, Mancuso E, Averta C, Paone C, Rubino M, et al. Are Circulating Mg(2+) Levels Associated With Glucose Tolerance Profiles and Incident Type 2 Diabetes? Nutrients (2019) 11(10):2460. doi: 10.3390/nu11102460
24. Zheng Y, Zhang C, Weisskopf M, Williams PL, Parsons PJ, Palmer CD, et al. A Prospective Study of Early Pregnancy Essential Metal(loid)s and Glucose Levels Late in the Second Trimester. J Clin Endocrinol Metab (2019) 104(10):4295–303. doi: 10.1210/jc.2019-00109
25. Zhou Z, Chen G, Li P, Rao J, Wang L, Yu D, et al. Prospective Association of Metal Levels With Gestational Diabetes Mellitus and Glucose: A Retrospective Cohort Study From South China. Ecotoxicol Environ Saf (2021) 210:111854. doi: 10.1016/j.ecoenv.2020.111854
26. Mary Helen B, Sacks DA, Xiang AH, Lawrence JM. Clinical Outcomes of Pregnancies Complicated by Mild Gestational Diabetes Mellitus Differ by Combinations of Abnormal Oral Glucose Tolerance Test Values. Diabetes Care (2010) 33(12):2524–30. doi: 10.2337/dc10-1445
27. Farrar D, Fairley L, Santorelli G, Tuffnell D, Sheldon TA, Wright J, et al. Association Between Hyperglycaemia and Adverse Perinatal Outcomes in South Asian and White British Women: Analysis of Data From the Born in Bradford Cohort. Lancet Diabetes Endocrinol (2015) 3(10):795–804. doi: 10.1016/s2213-8587(15)00255-7
28. Weinert LS. International Association of Diabetes and Pregnancy Study Groups Recommendations on the Diagnosis and Classification of Hyperglycemia in Pregnancy: Comment to the International Association of Diabetes and Pregnancy Study Groups Consensus Panel. Diabetes Care (2010) 33(7):e97; author reply e98. doi: 10.2337/dc10-0544
29. Zhou B-F. Predictive Values of Body Mass Index and Waist Circumference for Risk Factors of Certain Related Diseases in Chinese Adults–Study on Optimal Cut-Off Points of Body Mass Index and Waist Circumference in Chinese Adults. Biomed Environ Sci BES (2002) 15(1):83–96.
30. Sibai BM. Diagnosis and Management of Gestational Hypertension and Preeclampsia. Obstet Gynecol (2003) 102(1):181–92. doi: 10.1016/s0029-7844(03)00475-7
31. Yamamoto M, Sakurai K, Eguchi A, Yamazaki S, Nakayama SF, Isobe T, et al. Association Between Blood Manganese Level During Pregnancy and Birth Size: The Japan Environment and Children's Study (JECS). Environ Res (2019) 172:117–26. doi: 10.1016/j.envres.2019.02.007
32. Tsai MS, Liao KW, Chang CH, Chien LC, Mao IF, Tsai YA, et al. The Critical Fetal Stage for Maternal Manganese Exposure. Environ Res (2015) 137:215–21. doi: 10.1016/j.envres.2014.12.010
33. Eguchi A, Yanase K, Yamamoto M, Sakurai K, Watanabe M, Todaka E, et al. The Relationship of Maternal PCB, Toxic, and Essential Trace Element Exposure Levels With Birth Weight and Head Circumference in Chiba, Japan. Environ Sci Pollut Res Int (2019) 26(15):15677–84. doi: 10.1007/s11356-019-05009-0
34. Rahman ML, Oken E, Hivert M-F, Rifas-Shiman S, Lin P-ID, Colicino E, et al. Early Pregnancy Exposure to Metal Mixture and Birth Outcomes - A Prospective Study in Project Viva. Environ Int (2021) 156:106714. doi: 10.1016/j.envint.2021.106714
35. Mora AM, van Wendel de Joode B, Mergler D, Cordoba L, Cano C, Quesada R, et al. Maternal Blood and Hair Manganese Concentrations, Fetal Growth, and Length of Gestation in the ISA Cohort in Costa Rica. Environ Res (2015) 136:47–56. doi: 10.1016/j.envres.2014.10.011
36. Institute of Medicine (US) and National Research Council (US) Committee to Reexamine IOM Pregnancy Weight Guidelines. Weight Gain During Pregnancy: Reexamining the Guidelines. In: Rasmussen KM, Yaktine AL, editors. Washington DC: National Academies Press (US) (2009).
37. Wasserman GA, Liu X, Parvez F, Ahsan H, Levy D, Factor-Litvak P, et al. Water Manganese Exposure and Children's Intellectual Function in Araihazar, Bangladesh. Environ Health Perspect (2006) 114(1):124–9. doi: 10.1289/ehp.8030
38. Liu B, Feng W, Wang J, Li Y, Han X, Hu H, et al. Association of Urinary Metals Levels With Type 2 Diabetes Risk in Coke Oven Workers. Environ Pollut (Barking Essex 1987) (2016) 210:1–8. doi: 10.1016/j.envpol.2015.11.046
39. Hardy IJ, Gillanders L, Hardy G. Is Manganese an Essential Supplement for Parenteral Nutrition? Curr Opin Clin Nutr Metab Care (2008) 11(3):289–96. doi: 10.1097/MCO.0b013e3282f9e889
40. Takser L, Lafond J, Bouchard M, St-Amour G, Mergler D. Manganese Levels During Pregnancy and at Birth: Relation to Environmental Factors and Smoking in a Southwest Quebec Population. Environ Res (2004) 95(2):119–25. doi: 10.1016/j.envres.2003.11.002
41. Zhou C, Yao L. Biochemistry and Molecular Biology. Beijing: People's Daily Publishing Corporation (2018).
42. Iannotti LL, Zavaleta N, León Z, Shankar AH, Caulfield LE. Maternal Zinc Supplementation and Growth in Peruvian Infants. Am J Clin Nutr (2008) 88(1):154–60. doi: 10.1093/ajcn/88.1.154
43. Surkan PJ, Shankar M, Katz J, Siegel EH, Leclerq SC, Khatry SK, et al. Beneficial Effects of Zinc Supplementation on Head Circumference of Nepalese Infants and Toddlers: A Randomized Controlled Trial. Eur J Clin Nutr (2012) 66(7):836–42. doi: 10.1038/ejcn.2012.42
44. Hansen D, Lou HC. Brain Development, Head Circumference and Medication. Acta Paediatr (Oslo Norway 1992) (2000) 89(5):505–7. doi: 10.1111/j.1651-2227.2000.tb00326.x
45. Chimienti F. Zinc, Pancreatic Islet Cell Function and Diabetes: New Insights Into an Old Story. Nutr Res Rev (2013) 26(1):1–11. doi: 10.1017/S0954422412000212
46. Mikelson CK, Troisi J, LaLonde A, Symes SJK, Thurston SW, DiRe LM, et al. Placental Concentrations of Essential, Toxic, and Understudied Metals and Relationships With Birth Outcomes in Chattanooga, Tn. Environ Res (2019) 168:118–29. doi: 10.1016/j.envres.2018.09.006
47. Perng W, Tamayo-Ortiz M, Tang L, Sánchez BN, Cantoral A, Meeker JD, et al. Early Life Exposure in Mexico to ENvironmental Toxicants (ELEMENT) Project. BMJ Open (2019) 9(8):e030427. doi: 10.1136/bmjopen-2019-030427
48. Soomro MH, Baiz N, Huel G, Yazbeck C, Botton J, Heude B, et al. Exposure to Heavy Metals During Pregnancy Related to Gestational Diabetes Mellitus in Diabetes-Free Mothers. Sci Total Environ (2019) 656:870–6. doi: 10.1016/j.scitotenv.2018.11.422
49. Gaetke LM, Chow-Johnson HS, Chow CK. Copper: Toxicological Relevance and Mechanisms. Arch Toxicol (2014) 88(11):1929–38. doi: 10.1007/s00204-014-1355-y
50. Bhatia J, Griffin I, Anderson D, Kler N, Domellöf M. Selected Macro/Micronutrient Needs of the Routine Preterm Infant. J Pediatr (2013) 162(3, Supplement):S48–55. doi: 10.1016/j.jpeds.2012.11.053
51. Duncanson GO, Worth HG. Determination of Reference Intervals for Serum Magnesium. Clin Chem (1990) 36(5):756–8. doi: 10.1093/clinchem/36.5.756
Keywords: metal, fasting plasma glucose, birth outcome, mediating effect, cohort study
Citation: Zhou Z, Yu D, Chen G, Li P, Wang L, Yang J, Rao J, Lin D, Fan D, Wang H, Gou X, Guo X, Suo D, Huang F and Liu Z (2021) Fasting Plasma Glucose Mediates the Prospective Effect of Maternal Metal Level on Birth Outcomes: A Retrospective and Longitudinal Population-Based Cohort Study. Front. Endocrinol. 12:763693. doi: 10.3389/fendo.2021.763693
Received: 24 August 2021; Accepted: 22 October 2021;
Published: 16 November 2021.
Edited by:
Ihtisham Bukhari, Fifth Affiliated Hospital of Zhengzhou University, ChinaReviewed by:
Masahide Hamaguchi, Kyoto Prefectural University of Medicine, JapanMisbah Sultana, University of Lahore, Pakistan
Copyright © 2021 Zhou, Yu, Chen, Li, Wang, Yang, Rao, Lin, Fan, Wang, Gou, Guo, Suo, Huang and Liu. This is an open-access article distributed under the terms of the Creative Commons Attribution License (CC BY). The use, distribution or reproduction in other forums is permitted, provided the original author(s) and the copyright owner(s) are credited and that the original publication in this journal is cited, in accordance with accepted academic practice. No use, distribution or reproduction is permitted which does not comply with these terms.
*Correspondence: Zhengping Liu, bGl1enBobGs4MUBvdXRsb29rLmNvbQ==; Fang Huang, ZnNndW94bEAxNjMuY29t; Dongmei Suo, ZnNndW94bEAxNjMuY29t