- 1Department of Biology, Faculty of Science, University of Ottawa, ON, Canada
- 2Department of Zoology, Institute of Science, Banaras Hindu University, Varanasi, India
- 3Department of Biotechnology, Cochin University of Science and Technology, Kochi, India
The vertebrate nonapeptide families arginine vasopressin (AVP) and oxytocin (OXT) are considered to have evolved from a single vasopressin-like peptide present in invertebrates and termed arginine vasotocin in early vertebrate evolution. Unprecedented genome sequence availability has more recently allowed new insight into the evolution of nonapeptides and especially their receptor families in the context of whole genome duplications. In bony fish, nonapeptide homologues of AVP termed arginine vasotocin (Avp) and an OXT family peptide (Oxt) originally termed isotocin have been characterized. While reproductive roles of both nonapeptide families have historically been studied in several vertebrates, their roles in teleost reproduction remain much less understood. Taking advantage of novel genome resources and associated technological advances such as genetic modifications in fish models, we here critically review the current state of knowledge regarding the roles of nonapeptide systems in teleost reproduction. We further discuss sources of plasticity of the conserved nonapeptide systems in the context of diverse reproductive phenotypes observed in teleost fishes. Given the dual roles of preoptic area (POA) synthesized Avp and Oxt as neuromodulators and endocrine/paracrine factors, we focus on known roles of both peptides on reproductive behaviour and the regulation of the hypothalamic-pituitary-gonadal axis. Emphasis is placed on the identification of a gonadal nonapeptide system that plays critical roles in both steroidogenesis and gamete maturation. We conclude by highlighting key research gaps including a call for translational studies linking new mechanistic understanding of nonapeptide regulated physiology in the context of aquaculture, conservation biology and ecotoxicology.
1 Introduction
Members of the arginine vasopressin (AVP)/oxytocin (OXT) molecular family and their receptors are phylogenetically ancient, originating in a common ancestor evolved from a common ancestor of the Bilateria (1). As conserved cyclic nonapeptides, they exert pleiotropic functions in reproduction, sociosexual behavior, energy balance, osmoregulation, and the cardiovascular system, among others. In this review we take a focussed approach and examine their importance in the reproductive physiology of teleost fishes.
1.1 The teleost nonapeptide system
1.1.1 Nonapeptide structure and evolution
In basal cephalochordates, such as the Florida lancelet, Branchiostoma floridae, and urochordates such as the sea vase, Ciona intestinalis, single nonapeptides, [Ile4]-VP and Ciona (ciVP) have been reported (2–4). The presence of a single AVP family peptide extends to basal vertebrate agnathans, including lampreys such as the Japanese lamprey, Lethenteron japonicum, and hagfishes such as Eptatretus burgeri (5, 6). It is believed that two rounds of whole genome duplication (2R WGD), one before and one after the separation of agnathans and gnathostomes (7), led to a duplication of the ancestral arginine vasotocin (avp) gene. This duplication gave rise to two nonapeptide families conserved in vertebrates; the AVP and OXT family peptides (8). In vertebrates, both genes code for precursor proteins which include a 5’ signal sequence, a highly conserved nonapeptide and neurophysin, and, in the case of the AVP family, a C-terminal glycoprotein termed copeptin without known biological function (9). Processing into the mature nonapeptides occurs via prohormone convertases, and the acidic neurophysins I (OXT family) and neurophysin II (AVP family) associate with mature nonapeptides acting as carrier molecules within the neurosecretory system (9).
In teleost fishes, vasotocin (Avp) was originally isolated and characterized in pout, Gadus luscus (10), pollock, Polacchius virens (11), and European hake, Mercluccius mercluccius (12), and has since been identified in genomes of all teleost fishes studied to date (3, 6). Avp occurs in all verebrates except for mammals, where it appears to have mutated to give rise to vasopressin (AVP) in which 3Ile was substituted for 3Phe (3, 5, 6). All AVP family members are basic nonapeptides due to a basic amino acid (AA) at position 8.
In contrast to the AVP family peptides, the duplicated nonapeptide gene appears to have accumulated more mutations giving rise to the OXT family (3, 5, 6). The OXT nonapeptides are characterized by a neutral AA at position 8 (Leu, Ile, Gln or Val). In fishes alone, as many as 12 Oxt family peptides are known and likely arose due to lineage-specific duplications followed by substitutions in AA positions 3, 4 and 5 in the ring structure and position 8 in the tail structure (Table 1). With reference to mammalian OXT, the substitutions are Tyr2 by Phe2, Ile3 by Phe3, Gln4 by Ser4 or Asp4, and Leu8 by Ile8 or Val8. In teleost fishes, a single OXT family peptide (Oxt; [Ser4-Ile8]-OXT) was first isolated in pout, pollock, and the European hake and originally termed isotocin due to the presence of Ile in position 8 (18).
While position 8 mutations are very common in cartilaginous fishes (19) specific Oxt family peptides have also been reported in Sarcopterygii, such as the Australian lungfish, Neoceratodus forsteri, which expresses [Phe2-Ile3] OXT (20). Based on more recent genomic data, it has become evident that non-teleost Actinopterygians also express specific Oxt peptides (6). Indeed, in the spotted gar, Lepisosteus oculatus, which possess a pre-3R genome, two gene paralogues coding for Oxt were identified - one coding for a pro-[Ser4-Ile8]-OXT with a long C-terminal (NCBI Accession No. XM_006626499.1) and the other coding for a novel pro-[Phe2, Ser4]-OXT, which has a short C-terminal like other vertebrate neutral OXT family peptide precursors (NCBI Accession no. XM_006626523.1). An analysis of tissue expression profiles based on a fish RNA-seq expression database (21) does not reveal clear-cut differential expression of both oxt paralogues in spotted gar (Figure 1A).
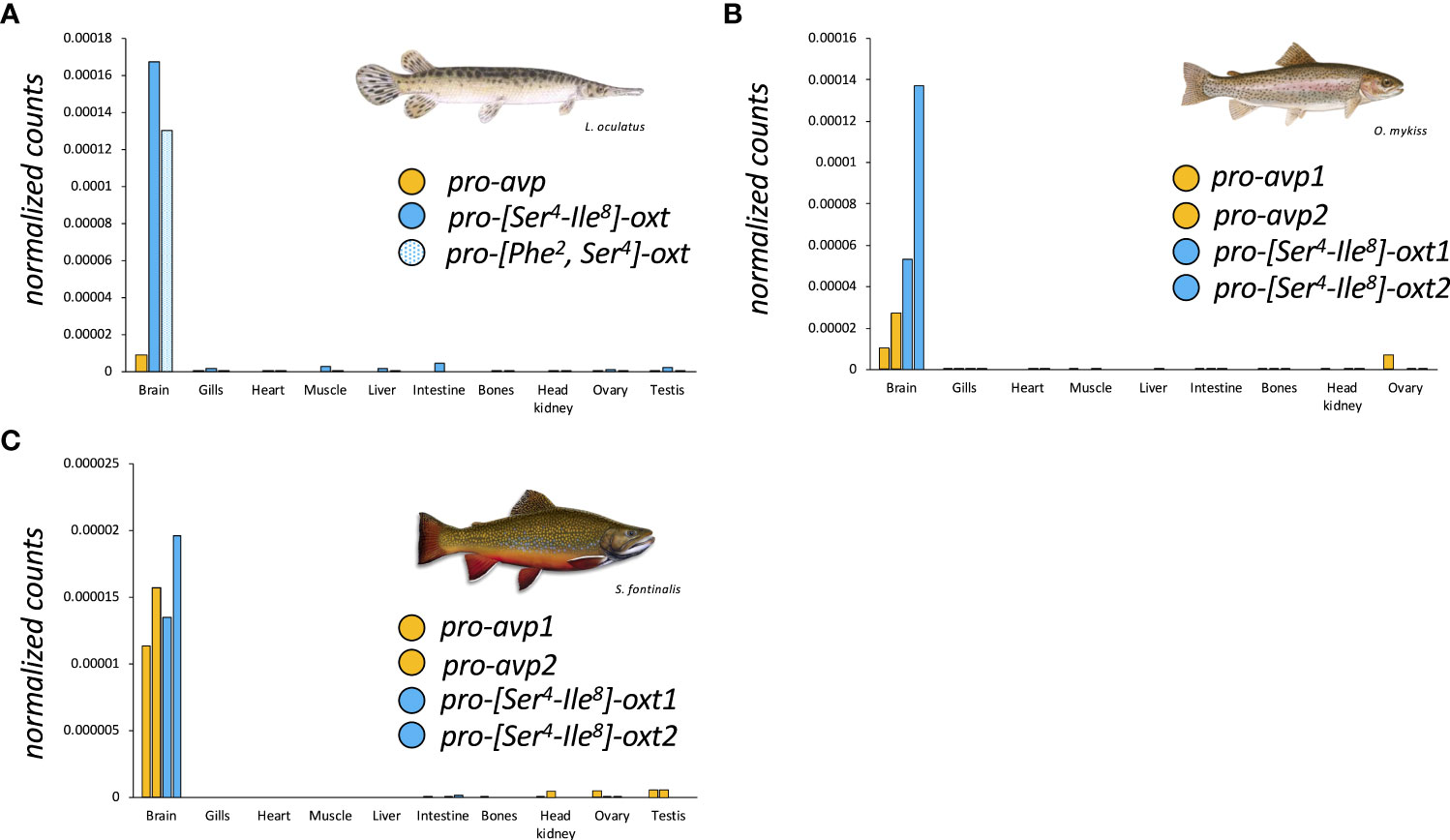
Figure 1 Tissue-specific expression profiles of nonapeptide precursor coding genes in spotted gar (A), rainbow trout, Oncorhynchus mykiss (B), and brook trout, Salvelinus fontinalis (C) derived from the Phylofish RNA-seq database (www.phylofish.signae.org) queried on June 1st, 2022 (20).
In teleosts, characterized by a 3R genome condition, occurrence of two copies each of pro-avp (pro-avp1 and pro-avp2) and pro-oxt (pro-oxt1 and pro-oxt2) have been reported in the blind cave fish, Astyanax mexicanus, based on genomic information and in salmonids and catastomids based on cloning studies (6, 22–24). In the blind cave fish, a diploid fish, synteny analysis suggests that the gene duplications may be due to 3R without subsequent gene loci losses (6). In salmonids and catostomids, the multiple oxt copies may be due to tetraploidization (4R WGD) and/or gene conversion (22–24). Regarding tissue expression profiles of paralogous oxt genes in salmonids, analysis of RNA-seq data (21) suggests similar expression profiles, at least in, rainbow trout, Oncorhynchus mykiss (Figure 1B), and brook trout, Salvelinus fontinalis (Figure 1C).
More recently however, the paradigm that teleost fishes, while encoding multiple gene loci for neutral nonapeptides in some cases, nevertheless exclusively express Oxt ([Ser4-Ile8]-OXT) was challenged by the cloning and discovery of two different neutral Oxt family peptides in the Asian stinging catfish, Heteropneustes fossilis, and the walking catfish, Clarias batrachus (6). In both species, a conventional oxt gene coding for a [Ser4-Ile8]-OXT and a second oxt gene coding for the novel [Ser4-Val8]-OXT, coined sevatocin, are expressed in addition to a single avt gene (6). Like pro-[Ser4-Ile8]-OXT, the peptide precursor encoding for [Ser4-Val8]-OXT has a similar organization (Table 2), with an extended C-terminal and a Leu-rich region. The functional implications of the oxt genes in these catfish species deserve special mention. The substitutions in the hormone moiety (Ile8/Val8) may lead to altered receptor‐ligand interaction with possible sub- or neo-functionalization. Both oxt-like genes are similarly expressed in the preoptic area (POA), and functional studies show that both synthetic [Ser4-Ile8]-OXT and [Ser4-Val8]-OXT similarly regulate fshb, lhb and gpa expression in the catfish pituitary (6, 24). It is possible that both Oxt peptides bind to the same receptors, but receptor characterization has not yet been undertaken.

Table 2 General features of the cDNAs of an encoded precursor proteins of Oxta, Oxtb and Avp in the catfish Heteropneustes fossilis.
For the purpose of this review, we follow the recent Zebrafish Information Network (ZFIN, www.zfin.org) nomenclature, which uses avp/Avp and oxt/Oxt to designate teleost genes and their protein products. The reasoning for this recent change in the literature is to highlight the homologous nature of avp and oxt family genes and their products in vertebrates. Consequently, this nomenclature no longer uses the historical distinction between teleost and mammalian nonapeptides (arginine vasotocin/arginine vasopressin and isotocin/oxytocin) which is reflective of their AA composition. Thus, while the historically widely used nomenclature is not used in this review, it is implicitly understood that the teleost avp/Avp and oxt/Oxt differ from mammalian and other vertebrate nonapeptide homologues in their AA residues as described.
1.1.2 Anatomy of the nonapeptide system in the context of reproduction
The distribution of Avp and Oxt has been examined in many teleosts and will not be covered in detail here. Controversies arise largely because of differing sensitivities of the neuroanatomical methods used, variable control experiments and other technical challenges (25, 26). Nevertheless, the use of transgenic approaches is helping to firmly establish the key locations of neuronal soma, and a new appreciation for their wide projection fields (27–30). In teleosts, Avp and Oxt neurons are intermingled and have been classified into three populations in the POA based on soma size. These are the giganto-, magno- and parvocellular neurons. Preoptic Avp and Oxt neurons send their fibers into diverse regions of the brain such as the hypothalamus, ventral telencephalon, mesencephalon and diencephalon, as well as the hindbrain and the spinal cord (20–30, amongst many others). Through the latter two systems, a role for POA-derived nonapeptides in modulating motor output related to reproductive behaviour and gamete release has been postulated in some, but not all teleost species (31–33).
In fish species in which specific sensory modalities have been shown to play a key role in reproductive behaviours, nonapeptide innervation has been described for distinct brain regions involved in sending and receiving sensory information. For example, in male zebrafish, Danio rerio, known to respond to female sex pheromones (34), fibers positive for Oxt have been identified in the olfactory bulb (35). In the plainfin midshipman, Porichtys notatus, which relies on vocalization as part of their courtship behaviour (36), especially Avp but also Oxt innervation was found in fore- and mid-brain regions involved in vocalization, and diencephalic regions of the ascending auditory pathway (37, 38). In the weakly electric gymnotiform bluntnose knifefish, Brachyhypopomus gauderio, which uses electric organ discharge (EOD) signals for mate selection (39), Avp innervation in the medulla was found to be in proximity of the pacemaker nucleus that controls EOD (40). Together, neuroanatomical evidence thus suggests a role for nonapeptides in modulating both emitting and receiving pathways of diverse sensory signals linked to reproductive behaviours in various teleost fishes.
In all teleost species studied to date, prominent preoptic and ventral hypothalamic projections terminating in the posterior pituitary for release to the pituitary vasculature have been reported (41, 42). In some species, such as the dwarf gourami, Colisa lalia, a colocalization of parvocellular Oxt with gonadotropin releasing hormone (Gnrh) has been reported (43). In the goldfish, Carassius auratus, Oxt colocalizes with secretoneurin a (44), an important hypophysiotropic stimulator of the hypothalamo-pituitary-gonadal (HPG) axis (45, 46). Such neuroanatomical data suggest that nonapeptides may regulate the HPG axis by co-release with other neuropeptides.
Detailed neuroanatomical studies of the pituitary in the sailfin molly, Poecilia lattipinna, the European bass, Dicentrarchus labrax, and the African sharptooth catfish, Clarias gariepinus, revealed that nonapeptidergic innervation principally forms contact with pituitary vasculature in the form of terminal release buttons which highlights an endocrine role of the nonapeptides (41, 42, 47, 48). Central and circulating Avp and Oxt concentrations in the nM range have been reported to be sex-specific, correlated, and reproductive stage-dependent in at least some species, such as the round goby, Neogobius melanostomus (49), and the air-breathing catfish, Heteropneustes fossilis (50). In addition to pituitary release, nonapeptide fibers innervating gonadotrophs in the proximal pars distalis through gaps in the basement lamina have been demonstrated in the sailfin molly, Poecilia latipinna (41, 48). While there are far fewer contact sites of nonapeptide innervation of gonadotrophs compared to pituitary blood vessels, this evidence does nevertheless suggest that the neuronal organization of nonapeptide fibers in the pituitary also provides a basis for paracrine effects on gonadotrophs. Whether a single nonapeptide neuron originating from the teleost POA can be both encephalotropic and hypophysiotropic, and thus simultaneously regulate brain function as a neuromodulator and peripheral function as a paracrine factor or hormone, remains an open question. Evidence to-date suggests that this may be species-dependent. While single nonapeptide neurons originating in the POA have been shown to extend to both extrahypothalamic brain regions and the pituitary in Atlantic salmon, Salmo salar (51), clearly distinct Avp and Oxt neurons originating in the POA have been shown to innervate either extrahypothalamic brain regions or the pituitary, but not both, in zebrafish, Danio rerio (28, 29).
Transcript and/or protein abundance of nonapeptides in female and, to a lesser extent, male gonads has also been reported in several species. In rainbow trout, Onchorhynchus mykiss, ovarian expression of both avp and oxt has been reported (52). High oxt expression is also noted for whole zebrafish ovaries (53). In the air-breathing catfish, high-performance liquid chromatography (HPLC) analysis indicated the presence of Avp in ovaries and, albeit to a much lower extent, in testes (50). Immunohistochemistry approaches localized Avp to the ovarian follicular cell layer, with positive staining in both theca and granulosa cells, but failed to locate Avp in testes (50). Conversely, Avp has been located to interstitial cells in the testes of the chanchita, Cichlasoma dimerus (54). The expression of gonadal nonapeptide systems, and especially avp, appears to be a more widespread feature in teleosts, as suggested by RNA-seq data mined from the Phylofish database (20) and presented here (Figures 1A-C). Together, evidence of expression of a gonadal nonapeptide system in female and male gonads provides the anatomical basis for an additional reproductive role of nonapeptides thorough paracrine modulation of processes such as gametogenesis, steroidogenesis, and gamete release.
1.1.3 The teleost nonapeptide receptor repertoire
Nonapeptide systems can regulate reproductive physiology via central neuromodulatory and/or peripheral endocrine and/or paracrine pathways via G-protein coupled membrane-bound AVP and OXT peptide family receptors (AVPRs and OXTRs). Since newly available genome sequences have recently allowed for a comprehensive description of the vertebrate nonapeptide receptor repertoires and their evolution resulting in proposed nomenclature changes (55–59), we here review the repertoire of teleost receptors considering these findings in detail. For the purpose of this review, we once again follow the ZFIN nomenclature, which is largely reflective of these changes. This focused review provides the basis to critically review relevant information regarding specific teleost Avpr and Oxtr function in mediating central and peripheral nonapeptide effects on teleost reproduction.
The teleost nonapeptide receptor repertoire consists of five distinct gene family members, although not all are present in every teleost species. These receptors include avpr1a, avpr2, avprl as well as oxtr and their paralogues. A fifth vertebrate nonapeptide receptor family member, avpr1b is present in basal ray-finned fishes as well as tetrapods but is not present in elasmobranchs and teleost fishes (55–59) (Figures 2A-C). With regard to intracellular signaling mechanisms, Avprs and Oxtrs use diacylglycerol (DAG), inositol triphosphate (IP3) and calcium (Ca2+) as second-messengers with the exception of Avpr2l, which uses cyclic adenosine monophosphate (cAMP) (60). Avpr2b signaling has not directly been assessed in teleost fishes but may be similar to Avpr2aa, based on intracellular domain AA sequence similarities (57). For avpr1, all teleost genomes analyzed to date possess two paralogues, termed avpr1aa and avpr1ab (Figure 2A). Additionally, all teleost fishes appear to possess avpr2aa and avpr2ab paralogues (Figure 2B). The presence and retention of these paralogues is in line with the teleost-specific genome duplication, as single avpr1a and avpr2a genes exist in early non-teleost fishes (Figures 2A, B). Conversely, the situation appears to be more complex with regard to avpr2b and avpr2l genes which are retained in different paralogue numbers and lost in some but not all teleost fishes in no obvious evolutionary pattern (Figures 2A, B). The oxtra and oxtrb paralogues are found in all teleosts assessed to date, again indicative of a retention of paralogues following genome duplication at the base of teleost evolution (Figure 2C). Teleost-specific changes in the nonapeptide receptor repertoires have been reported, especially regarding possible intracellular AA sequences involved in cell signalling. For example, the teleost Avpr2aa AA sequence harbours extensions of the intracellular loop 3 region, which include relatively well-conserved Tyr, Ser and Thr residues which can be phosphorylated by a variety of intracellular kinases to alter intracellular signaling cascades as well as G-protein coupled receptor attenuation/desensitization, endocytosis, and intracellular trafficking (59). Lineage-specific changes in the nonapeptide repertoires have also been reported even within the infraclass of teleost fishes. For example, otocephalans lack an avpr2bb paralogue found in early teleosts as well as euteleosts (59), as shown in Figure 2B. In our current microsynteny analysis, we found additional otocephalan-specific changes for the oxtrb nonapeptide receptor locus (Figure 3A). These microsynteny changes translate into C-terminal AA sequence changes in an intracellular domain which, based on annotation from functional mutation studies in the human OXTR (61), is linked to Gq-protein recruitment and cell signaling (Figure 3B). Otocephala, which diverged from Euteleostei during the Jurassic ~220 million years ago, are characterized by innovations in communication and sensing, notably through Schreckstoff, the alarm substance released through skin injury from conspecifics, and auditory capacity through a link between the swim bladder and inner ear (otophysic link) (62). It is thus tempting to speculate that such rearrangements, with possible functional implications, represent changes which facilitated sub- or neo-functionalization linked to increased social cues relevant to reproduction in otocephalans. In the case of otocephalan Oxtrb, a loss of highly conserved intracellular domain AA residues was observed (Figure 3B). These are specifically located in the C-terminal sequence and have been shown to be required for Gq/11 and subsequent IP3, DAG and Ca2+ signaling (61). They also include consecutive Cys residues, which through palmitoylation, have been shown to anchor the C-terminal region to the membrane (61). Finally, it includes consecutive Ser residues believed to be involved in receptor retention for receptors following ligand-dependent internalization (61). Investigation of oxtra and oxtrb expression using an RNA-seq-based fish tissue database (21) reveal that in the otocephalans Allis shad, Alosa alosa, and Mexican cavefish, expression pattern for both oxtra and oxtrb receptor paralogues are similar in most tissues, apart from the brain where oxtra expression exceeded oxtrb expression (Figure 3C). In zebrafish ovary, oxtrb appears to be more strongly expressed compared to oxtra (53), suggesting possible differential roles in regulating ovarian function (53).
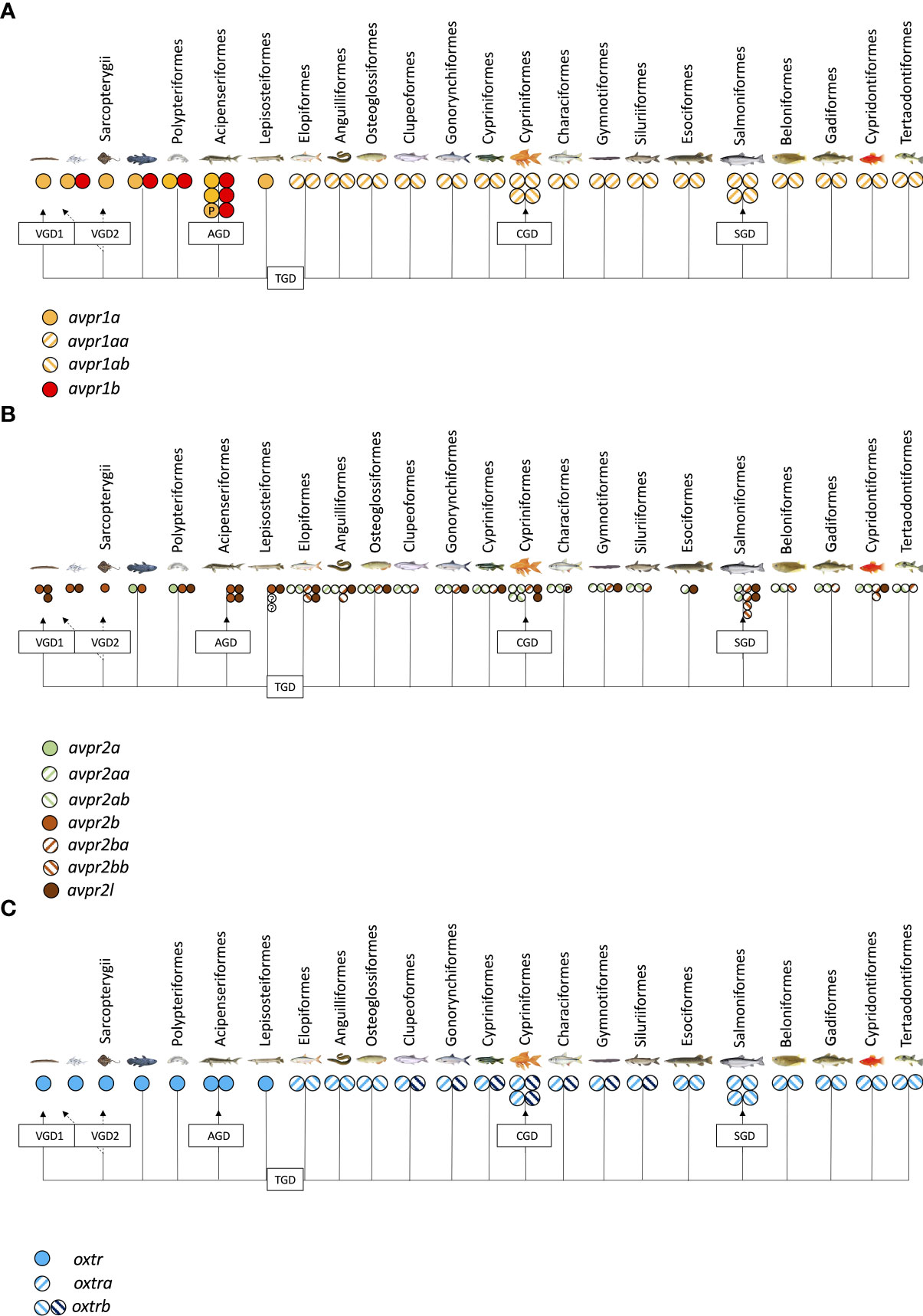
Figure 2 Presence of avpr1 (A), avpr2/avpr2l (B) and oxtr (C) genes in published fish genomes accessed on NCBI (www.pubmed.com) on June 1st, 2022.
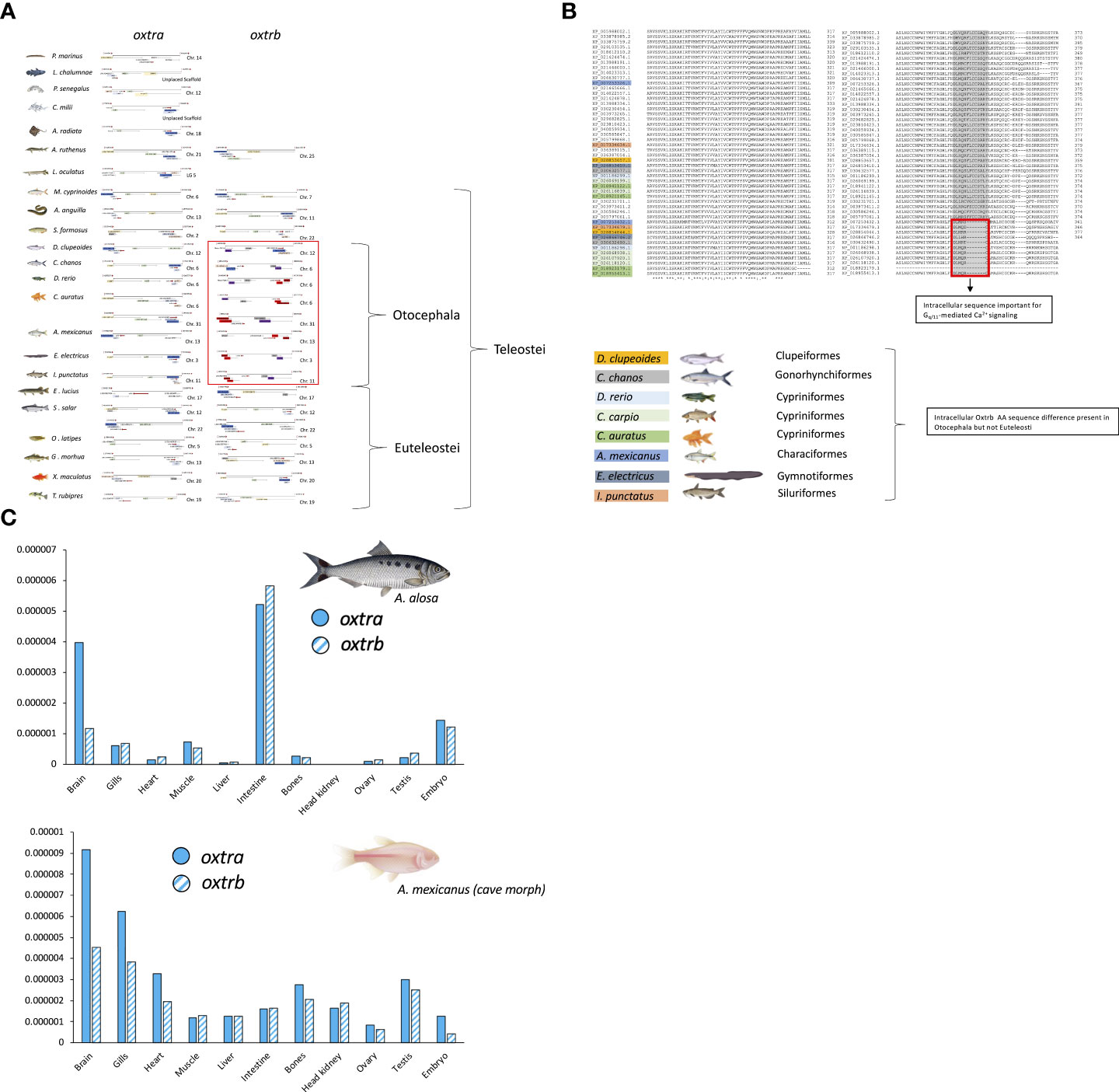
Figure 3 Microsynteny analysis (A), predicted AA sequence alignment (B) and RNA-seq based tissue expression profiles (C) of oxtra and oxtrb paralogues in select teleost fishes. Micro-synteny analysis of oxtr paralogue gene loci was manually conducted on NCBI (www.pubmed.com) derived genome sequences retrieved on June 1st, 2022, while oxtr gene paralogue sequence-predicted AA sequences were aligned using Clustal Omega (https://www.ebi.ac.uk/Tools/msa/clustalo/). RNA-seq based tissue expression profiles of oxtr paralogues was created derived from the Phylofish RNA-seq database queried on June 1st, 2022 (20).
In addition to oxtr paralogues, the expression of other teleost nonapeptide receptors and in some instances specific paralogues have been localized to tissues relevant to teleost reproduction (63–73) and are summarized in Table 3. Much of our current information on reproductive roles of nonapeptides has been derived from studies of otocephalan species including the air-breathing catfish (4, 5, 24, 84), goldfish (85–87), and zebrafish (35). Given that the nonapeptide repertoire is characterized by genomic rearrangements and potentially important AA sequence changes in this clade, caution is warranted when attempting to generalize functionality across all teleosts.
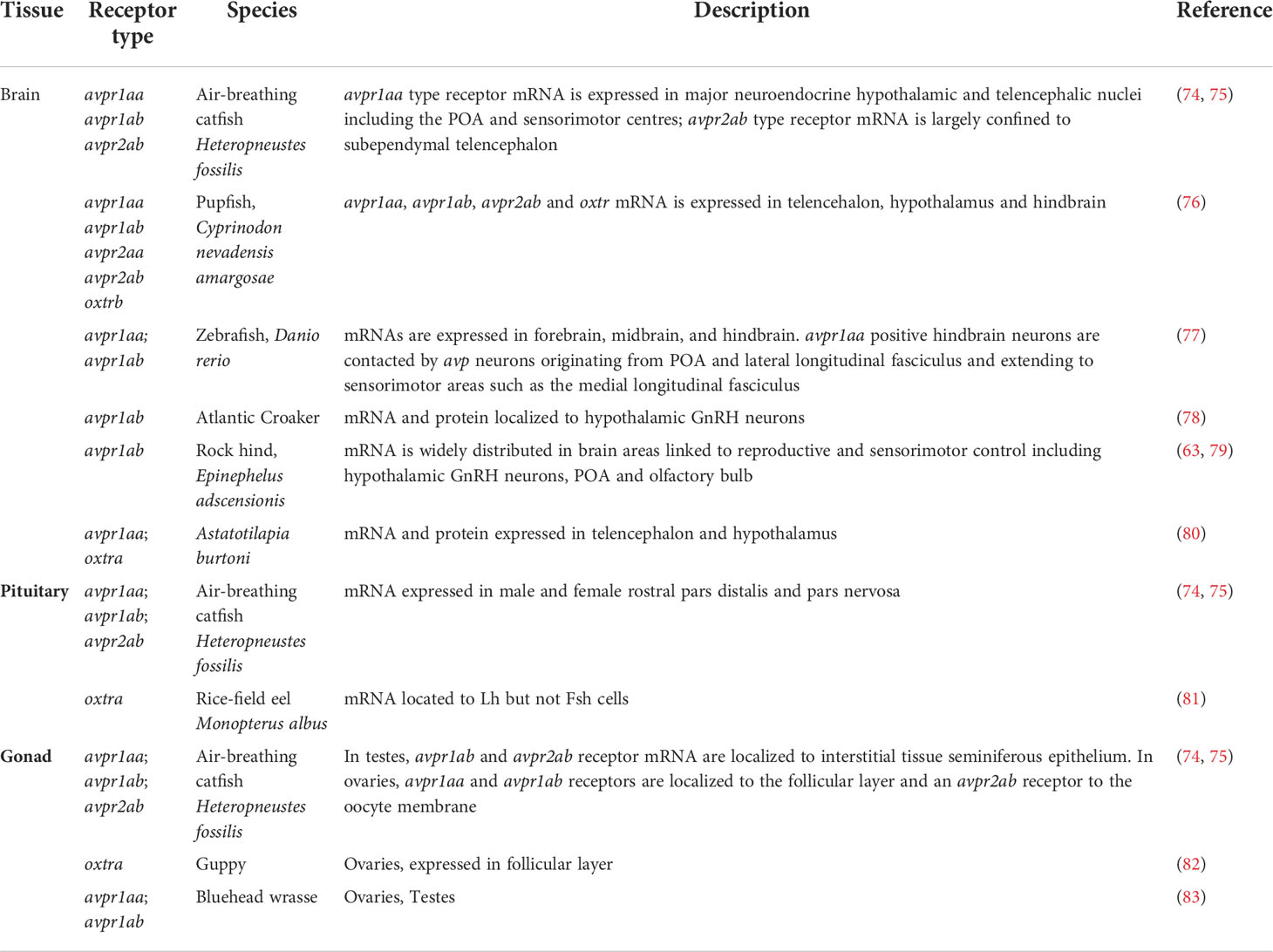
Table 3 Nonapeptide receptor expression in central and peripheral teleost tissues relevant to reproduction.
In contrast to the detailed evolutionary history, the functional characterization of nonapeptide receptors remains limited, and in some instances, such as for avpr2l, virtually unexplored in teleost fishes. Early studies in a cyprinid, the white sucker, Catostomus commersonii, investigated binding kinetics and specificity of both Avpr (88, 89) and Oxtr (90). Based on teleost genome sequences available today, these can retroactively be classified as Avpr1ab and Oxtra, respectively. These studies revealed that the Avpr1ab is highly selective for Avp (EC50 13 nM ± 6 nM) over Oxt. Both nonapeptides bound to and activated cell signaling of a heterologously expressed Oxtra receptor with 3- to 4-fold higher affinity for Oxt (80 ± 30 nM) compared to Avp (300 ± 90 nM). More recent studies in zebrafish assessed specificity of nonapeptides and a mammalian OXT receptor antagonist (L-368,899) in heterologous expression assays (91). It was reported that Oxt exhibits similar affinities for both Oxtr parlogues with EC50 values of 2.99 ± 0.93 nM for Oxtra and 3.14 ± 1.10 nM for Oxtrb, respectively. In comparison, Avp exhibited slightly lower affinities to Oxtrs with EC50 values of 11.0 ± 3.0 nM for Oxtra and 27.0 ± 9.5 nM for Oxtrb, respectively. Both Avp and Oxt had low affinities for Avpr1aa (EC50 727 ± 338 nM and 317 ± nM, respectively) and high affinities for Avpr1ab (2.79 ± 1.4 nM and 3.52 ± 0.94 nM, respectively). The guppy Oxtra was shown to be activated by both Oxt and Avp (82). While Oxt induced a strong dose-response (10 nM-1 μM) induction of co-transfected luciferase CRE-element, Avp induced a weaker, yet significant response at all concentrations tested (82). Together these data show that at physiological concentrations, it is likely that some degree of cross activation between Avp and Oxt ligands and the nonapeptides receptors occurs. It is therefore important to consider that reproductive roles of Avp and Oxt may, at least in part, be dependent on receptor cross-activation.
2 External and internal reproductive cues regulate teleost central nonapeptide systems
In several seasonal teleost fishes, nonapeptide expression and/or protein abundance in the POA is positively correlated with mature reproductive status (49, 50), which in turn, is dependent on environmental cues such as photoperiod and temperature (92). In female goldfish, hypothalamic oxt mRNA abundance peaks in the seasonal breeding period when fish have maximal gonadosomatic index (GSI) and is likely photoregulated (93). In male goldfish, exposure to the female releaser pheromone PGF2α, an important olfactory reproductive cue for males, increases oxt and avp mRNA levels in the telencephalon while stimulating circulating testosterone (T) concentrations and strippable milt volumes (94). Together, such association of nonapeptide expression in the neuroendocrine brain and enhanced pituitary and gonadal activity during seasonal cycles and after pheromone exposure is supportive of a physiological role in reproduction. Exposure to androstenedione, another pheromone known to play an important role in male-male competition in a reproductive context in goldfish (95), increases parvocellular avp (96). Thus, it appears that reproductive pheromones represent important environmental cues to regulate male goldfish nonapeptide expression. In male and female round goby, Neogobius melanostomus, maximal seasonal brain Avp concentration was observed just before spawning in March-April, whereas that of Oxt peaked during spawning in May-June (97). The lowest brain Avp level was noted in the non-breeding season from November to January, while the level of Oxt decreased immediately at the end of the spawning. The results show that high Avp levels correlate with pre-spawning period whereas the highest Oxt levels correspond to spawning. In female round gobies, these increases appear, at least in part, to be dependent on estrogens acting via genomic (nuclear receptors), and genomic and non-genomic mechanisms in the case of Avp and Oxt, respectively (98). This suggests that season-dependent gonadally-derived positive sex steroid feedback mechanisms may reinforce the seasonal activation of hypothalamic nonapeptide systems. In the half-spotted goby, Asterropteryx semipunctata, Avp and Gnrh protein abundances were positively correlated and exhibited significant peaks in their abundances in sexually mature females (99). In male sticklebacks, the highest brain concentrations of Avp were observed in the most aggressive males that cared for eggs and nuptial-colored subordinates that fought to change their social status. Oxt was significantly higher in brains of aggressive dominant males (100). In the stickleback, the highest Avp levels were found in brains of females that did not deposit eggs, regardless of whether they were kept with courting or non-courting males and whether they had a nest or not. The highest Oxt levels were observed in females that did not deposit eggs but were kept with a courting male. The presence of courting or non-courting males that somehow activate Oxt- or/and Avp-producing neurones may be decisive for both behaviour and/or final oocyte maturation or egg deposition, because brain levels of both nonapeptides decreased sharply after egg deposition (101).
In the anadromous chum salmon, Oncorhynchus keta, dynamic changes in nonapeptide transcript and protein abundance have been reported during the reproductive migration period (102, 103). Lower transcript but higher immunoreactivity of both Oxt and Avp in the POA of fish collected upstream in a freshwater system compared to those caught in the marine bay, which serves as an entry point of the reproductive migration (102, 103). Based on these results it is tempting to speculate that due to their well-described role in osmoregulation, nonapeptide changes may act to integrate relevant environmental signals, such as the change in salinity, to subsequently change aspects of reproductive physiology in these anadromous fish. It has, however, been shown that salinity changes are not consistently the principal factor mediating changes in the nonapeptide systems of migrating salmonids and temperature and endogenous sex steroid concentrations also act as important regulators of these systems (104). Nevertheless, these data demonstrate a reproductive phase-dependent regulation of the nonapeptide system in chum salmon. Within the context of salmonid reproduction and migration, it is important to note that alternative reproductive tactics exist among individuals. In addition to males migrating to the ocean to grow and mature, some precocious parr achieve sexual maturity quickly before migrating to the ocean and can thus fertilize female eggs as ‘sneaker males’ (105, 106). Two transcriptomic and targeted gene expression studies investigating differential gene expression in the whole brain of maturing males and precocious parr of Atlantic salmon identified nonapeptides as being differentially regulated (107). The functional relevance of the differential expression with regard to reproduction remains, however, unknown.
In some group-living cichlid species in which HPG axis activity and reproductive behaviour is linked to social dominance, avp and Avp abundance has been investigated (108). The results show differential effects on avp mRNA levels and Avp neuron size in different POA subpopulations, with higher levels in gigantocellular neurons and, conversely, lower levels in parvocellular neurons of dominant fish. While underlining the responsiveness of the nonapeptide system to social status, a determinant of reproductive status in at least some cichlids, these findings also highlight the importance of considering potentially differential effects on nonapeptide subpopulations in the POA. However, the finding that avp expression in the POA of dominant and reproductively active African cichlid fish, Astatotilapia burtoni, is significantly reduced compared to subordinates who are reproductively supressed (109), clearly suggests caution is warranted to avoid simplified and global paradigms regarding the role of nonapeptides in species in which social dominance is linked to increased reproductive capacity.
Teleost fish exhibit significant plasticity in terms of their reproductive biology and life history traits. Some species are sequential hermaphrodites, beginning life as one sex, and changing sometime later to the other. Such species are capable of protandrous (male-to-female), protogynous (female-to-male), or serial (bidirectional) sex change (110). Changes in nonapeptide systems have been observed in response to social context-induced sexual plasticity. For example, the bluehead wrasse, Thalassoma bifasciatum, exhibits specific increases in Avp immunoreactivity in the magnocellular POA when undergoing behavioural female-to-male sex change that occurs rapidly following the removal of a large terminal colour male and is independent of the gonads (111). The increase in magnocellular avp expression coincides with a rapid increase in dominant and male courting behaviour, suggesting a functional link (111, 112). Serial adult sex change in the marine goby, Trimma okinawae, is associated with significant and reversible changes in the size of Avp-producing forebrain cells, which are higher in males and coincide with increased male mating behaviour (113). In overcrowded single sex groups of female black mollies, Poecilia sphenops, which do not form social hierarchies, masculinization of reproductive behaviour occurs and is linked to a decrease of higher, female-typical Avp concentrations in this species to male typical concentrations (114). In a transcriptomic analysis of the bluehead wrasse forebrain, increased oxt transcript abundance was one of the few statistically significant changes detected across female-to-male sex change (115). The opposite trend was observed in the bluebanded goby, Lythrypnus dalli, where lower Oxt immunoreactivity was observed in the POA of males and late-stage female-to-male sex-changers compared to females (116).
Overall, these studies reveal context-dependent regulation of central nonapeptide systems across several species with diverse reproductive strategies. This strongly implicates nonapeptides in the regulation of teleost reproductive physiology. However, caution is clearly warranted to avoid oversimplified paradigms for specific roles of nonapeptides across all teleost species (117), as at least some species-specific roles are likely to have evolved among teleosts with such varied reproductive strategies.
3 Reproductive function of nonapeptides in teleost fishes
3.1 Nonapeptide-dependent regulation of reproductive behaviour
The plainfin midshipman fish, Porychthys notatus, displays sex- and morph-specific vocalization during mating. Oxt and Avp regulate these sex- and morph-specific effects on the vocal circuitry (37). Type I males mating call are stimulated by Avp, whereas female and type II males’ grunting sounds are stimulated by Oxt. Using homozygous Japanese medaka, Oryzias latipes, knockout mutants, an essential role for Avp in male mate-guarding behaviours in this non-monogamous species has been demonstrated (118). For example, under natural conditions, two medaka males kept in triads with a female are in competition and the dominant medaka male that is ‘guarding’ the female exhibits increased reproductive success measured as increased paternity in offspring. Males harbouring mutations in avp and avpr1aa exhibit significantly reduced male guarding behaviour indicating a key role for avp in dominant status-dependent reproductive success (118). Similarly, oxt and oxtra, exert sex-specific effects in Japanese medaka: mutant female fish exhibit a lack of mate preference for familiar males and mutant male fish have reduced courtships displays to unfamiliar females, but exhibit increased mate-guarding behaviour towards familiar females (64). Since the potential effects on the HPG axis were not quantified in these studies, it is not clear whether these effects are entirely mediated by the nonapeptides, or whether altered HPG axis regulation also contributes to the behavioural observations.
In male bluehead wrasse, Thalassoma bifasciatum, a species with alternate male reproductive tactics (territorial and non-territorial), Avp intraperitoneal injection increased courtship behaviour in the field irrespective of male reproductive tactic and promoted a territorial-like phenotype in non-territorial males (65). An opposite effect was observed following administration of Manning’s compound, a mammalian AVPR1 receptor antagonist, suggesting that this effect is mediated via this nonapeptide receptor subtype (65). In male white perch, Morone americana, intracerebroventricular but not intraperitoneal administration of Avp significantly stimulated an important courtship behavior termed ‘attending’ without affecting whole body or circulating androgens (119). These data suggest that central rather than peripheral HPG axis actions are involved in mediating the effects of Avp on the male white perch courtship behaviour. In male beaugregory damselfish, Stegastes leucostictus, Manning’s compound significantly lowered male courtship behaviour, while exogenous Avp administration did not affect male courtship behaviour (120). Similarly, administration of Manning’s compound significantly reduced male reproductive courtship behaviour and reproductive success in mating assays with female zebrafish, Danio rerio without affecting whole body androgen (T and 11-keto-testosterone; 11-KT) levels, suggesting Avp acutely regulates male zebrafish courtship behaviour including chasing, nudging, and circling via central Avpr1a receptors and independently of HPG axis regulation (35). In the weakly electric fish, Brachyhypopomus gauderio, male courtship behaviour observed in male-female dyads resulted in a higher degree of Avp neuron activation in the nucleus preopticus ventricularis anterior compared to isolated males (121). In the same species, Avp increases dominance in part via direct modulation of the EOD rate (122). Together, these findings raise the possibility that male reproductive behaviour via electric signaling may be under Avp control in this species. Despite the reviewed evidence, a universal Avp-dependent stimulation of male reproductive behaviour in teleost fishes is unlikely, as the reproductive phenotype of females and sneaker males, but not dominant males, is sensitive to Avp in the peacock blenny, Salaria pavo (123). There is a need for detailed comparative studies of the roles of nonapeptides in teleosts which exhibit the most diverse reproductive strategies and behaviours amongst the vertebrates (117).
Several studies have provided evidence for regulatory roles of nonapeptides in teleost species with parental care. In the primarily paternal teleost the common clownfish, Amphiprion ocellaris for example, administration of an OXTR antagonist abolished paternal behaviours such as nips, fanning the eggs, and proportion of time in the nest, without affecting aggressive behaviours in paired non-reproductive fish (124). This suggests a specific action of Oxt in controlling male common clownfish parental care behaviours (124); however, whether the high selectivity of antagonist (desGly-NH2-d(CH2)5[D-Tyr2,Thr4]OVT) for mammalian OXTR also applies to teleosts has not been formally investigated. When introducing domino damselfish, Dascyllus trimaculatus, as non-conspecific intruders, administration of the OXTR antagonist reduced paternal care behaviour in clownfish, but increased aggression towards the non-conspecific intruder, demonstrating the importance of social context in behavioural responses (125). Conversely, administration of an AVTR1 receptor antagonist increased male parental behaviours while reducing aggression towards intruders (125), thus demonstrating antagonistic roles of the nonapeptide systems in common clownfish (124, 125). These data suggest a role for nonapeptides in paternal care. In the monogamous convict cichlid, Amatitlania nigrofasciata, single fathers increase paternal care behaviours quickly after removal of the female partner, and this increase coincides with increased activation of parvocellular Oxt neurons in the POA (126). Administration of a mammalian OXTR antagonist in biparental males inhibited paternal care behaviour, indicating a functional role for Oxt neurons (126).
3.2 A role for central nonapeptides in regulating saliency to reproductive cues in teleost fishes
In addition to the direct modulation of teleost reproductive behaviours, central roles for nonapeptides may not be restricted to the role of transducing and integrating reproductive cues, but also to act as a filter and/or amplifier of exogenous or endogenous cues. This latter concept has recently gained more traction as the ‘salience hypothesis’, especially in higher vertebrate species, including humans. The salience hypothesis is based on the notion that an individual is being constantly inundated with sensory information in its environment and therefore needs to be able to filter the information to identify what is relevant and important (i.e., signal) from that which is unimportant (i.e., background noise). The nonapeptides OXT and AVP have been proposed to serve as two important neuromodulators in the central nervous system that can help to increase the salience of sensory information, such as conspecific olfactory cues, to induce effects on endocrine and behavioural responses (127). In fish, however, this concept remains comparatively poorly explored, especially in the context of the diverse reproductive strategies in different environmental conditions and cues. Olfactory stimuli in particular play important roles in at least some groups of teleost fish such as cyprinids (35, 94, 95). Future studies exploring sensitizing roles of nonapeptides to reproductive pheromonal and visual cue detection are thus clearly warranted. A recent study in zebrafish identified Oxt-like innervation in the male olfactory bulb (35), providing a neuroanatomical basis for functional studies. Similarly, the widely studied electrical and vocal communication systems in some teleost fishes (128) should provide excellent models to investigate possible modulatory roles of nonapeptides in the reception and integration rather than production of reproductive signals.
3.3 Nonapeptide-dependent regulation of the HPG axis
3.3.1 Nonapeptides are an integral part in the hypothalamic circuitry controlling the HPG axis
Both stimulatory and inhibitory factors regulating the teleost HPG axis have been well described (92). Nonapeptides may affect the HPG axis via modulation of stimulatory and/or inhibitory hypophysiotropic systems. To date, little evidence exists for potential roles of nonapeptides in directly affecting Gnrh in teleost fish. In rock hind, Epinephelus adscensionis, and in Atlantic Croaker, Micropogonias undulatus, Avpr1ab receptors are co-localized with Gnrh1 in preoptic anterior hypothalamic neurons. The functional relevance of this crosstalk other than reported concordant regulation of gene expression between avpr1ab and gnrh1 (78, 79, 99) must be investigated. Studies in female goldfish show that serotonin neurons, known to stimulate pituitary Lh release in this species (129), are found in proximity to Oxt neurons in the POA and Oxt fibres in the pars nervosa of the pituitary gland, suggesting a possible interaction between them (87). However, the functional relevance of possible Oxt dependent modulation of serotonin-dependent gonadotropin release has not been investigated.
Nonapeptides may ostensibly also act to promote pituitary gonadotropin release via reduction of potent dopaminergic inputs on Lh release (92). This is supported by work in the walking catfish, Clarias batrachus, which suggests that Oxt may stimulate Lh release via the inhibition of dopaminergic blockage (66). In walking catfish Oxt immunoreactivity was greatly enhanced in the POA in female pre-spawning and spawning fish, and superfusion of brain slices with Oxt resulted in a ~50% reduction of tyrosine hydroxylase staining, suggesting rapid inhibitory effects on dopamine or other catecholaminergic neurons (66).
In addition to hypothalamic interaction between nonapeptide systems and the neuronal circuitry involved in HPG axis regulation in several teleost models (66, 86, 87), a few lines of evidence also demonstrated co-expression of nonapeptides with neuropeptides with known stimulatory function on gonadotropin release. For example, Oxt and secretoneurin were found to be colocalized in the POA and fibers innervating the pituitary in goldfish, Carassius auratus (44), while colocalization of Oxt and GnRH was reported in the dwarf gourami, Colisa lalia (43). Together, these studies suggest that potential co-release of nonapeptides with other neuropeptides known to stimulate gonadotropin release represent an understudied aspect of HPG axis regulation.
In addition to targeting neuronal circuitry involved in the regulation of the HPG axis, POA nonapeptide neurons in teleost fish themselves receive neuronal input from reproductive neuropeptides. These include Gnrh, which contact Oxt neurons in rainbow trout, Oncorhynchus mykiss (130), and kisspeptin, which contact Oxt and Avp neurons in Japanese medaka (131) and striped bass, Morone saxatillis (132). Together, this neuroanatomical evidence points to potential neuromodulatory roles for nonapeptides in HPG stimulation in the context of multimodal signaling systems regulating gonadotrophs in teleosts (133). Given the reported roles of nonapeptides in teleost sociosexual and courtship behaviour, neuroanatomical evidence may also reflect synchronization of HPG axis activation stimulation with nonapeptidergic behavioural pathways to maximize reproductive success.
Several studies have also demonstrated the sensitivity of both Oxt and Avp neuronal populations to sex steroids. Administration of both low (0.1 μg/g body weight) and high (0.5 μg/g body weight) estradiol (E2) doses (0.5 μg/g body weight) normalizes ovariectomy-induced decreases in brain and plasma Avp concentrations in Asian stinging catfish (134). This effect appears to be, at least in part, indirectly mediated via the modulation of dopaminergic control, as treatment with α-methyl-para-tyrosine, a tyrosine hydroxylase inhibitor, partially abolished the restorative effect of the low E2 dose on Avp abundance in the ovariectomized fish (134). A male-specific stimulatory effect of androgens on parvocellular Oxt neurons in the medaka POA has also been reported (135). This regulation also appears to be indirect, as androgen receptor expression was not found in Oxt neurons but on kisspeptin neurons known to stimulate Oxt neurons in this species (131, 135). In female round gobies, circulating E2 levels are higher in the spawning phase compared to non-spawning phase and coincide with high circulating Avp and Oxt concentrations (98). Brain explant exposure to E2 in spawning and non-spawning phases stimulated Avp and Oxt release in this species; however, pharmacological studies using the estrogen receptor (ER) antagonist fulvestrant and the transcription inhibitor actinomycin D showed that the effect of E2 on Avp and Oxt release was mediated by different signaling pathways (98). E2-dependent Avp release was mediated by ERs via both genomic and non-genomic pathways, while Oxt release was mediated through ERs via a genomic pathway only (98). Whether E2 acted directly on nonapeptide neurons in female gobies was not resolved, as the study did not investigate whether nonapeptide neurons express ERs. Oxt neurons in the POA of goldfish, Carassius auratus, are, at least based on immunohistochemical evidence, direct targets for estrogen actions, as they express the membrane estrogen receptor Gper1 (96) and are surrounded by radial glia, the only cells in the teleost brain expressing cyp19a1b and capable of producing neuroestrogens (136). While these studies provide evidence for effects of steroids on nonapeptide systems, future studies are warranted to delineate direct and indirect mechanisms of action, and whether effects are mediated by gonadal steroids and feedback regulation and/or local neurosteroids.
In sum, POA nonapeptide systems have been shown to be integrated into neuronal circuits involved in the regulation of the HPG axis in several teleosts. Additionally, recent evidence suggests that nonapeptide neurons are also responsive to sex steroids, suggesting the potential for endocrine feedback and/or modulation via neurosteroids. Additional studies investigating nonapeptide crosstalk with other hypothalamic regulators of the HPG axis are clearly warranted, as are careful studies investigating the direct or indirect regulation of hypothalamic nonapeptide systems to sex steroids, the critical endogenous reproductive signals.
3.3.2 Nonapeptides exert direct hypophysiotropic effects on gonadotrophs in several species
In the goldfish, hypothalamic expression of oxt was found to peak seasonally in reproductively mature females (93). Pharmacological investigations demonstrate that hypothalamic induction of goldsfish oxt is dependent on GABAergic and dopaminergic signaling (137), in line with an integration of this nonapeptide system with seasonally-regulated HPG neurocircuitry in this species (92). Intraperitoneal injection of 1 μg/g body weight Oxt in sexually recrudescent female goldfish significantly increased circulating Lh by 167% 5h post-injection (86) with subsequent increases in circulating E2 12h post injection (137). Unfortunately, Oxt-dependent stimulation of Lh release in sexually mature female goldfish was not investigated. Subsequent examination of potential direct effects of Oxt on gonadotrophs were investigated using primary goldfish dispersed pituitary cell cultures (87). In these preparations, Oxt significantly stimulated Lh release without affecting lhb or fshb subunit mRNAs, suggesting direct, transcription-independent stimulation of Lh release (87). These findings are in line with reported neuroanatomical evidence in other teleosts, such as the sailfin molly, Poecilia latipinna, the European bass, Dicentrarchus labrax, and the African sharptooth catfish, Clarias gariepinus, in which nonapeptidergic innervation of gonadotrophs has been reported (41, 48). Similar to Oxt, a stimulatory effect of Avp on Lh release has been demonstrated in at least two teleosts: in the sailfin molly 18 h pituitary incubation with Avp stimulated Lh synthesis and release, with lower dose- responsiveness and more consistent effects in male compared to female pituitaries (41). In the Asian stinging catfish, Avp, and to a much lesser extent Oxt and servatocin, stimulated gpa, fshb and lhb in pituitary cultures in a sex- and reproductive stage-dependent manner (24). The less potent effects of Oxt and servatocin where largely limited to lhb stimulation in pre-spawning females, with no effect on fshb. In female walking catfish, Clarias batrachus, pituitaries superfused with 20 nM Oxt for 1h displayed a significant decrease in Lhb staining reflective of increased Lh release was reported (138). In the ricefield eel, Monopterus albus, Oxt-stimulated Lh release from dispersed pituitaries via an Oxtra-activated IP3/Ca2+ pathway (81). In males of the Chanchita, Cichlasoma dimerus, Avp-stimulated gonadotropin secretion in single pituitary culture, with biphasic stimulation of Lh release at the lowest (0.1 μM) and highest (10 μM) concentration of Avp tested (54) and a stimulation of Fsh release at the highest Avp concentration tested.
These data establish a stimulatory role of nonapeptides on gonadotrophs, with sex-, reproductive stage- and species-dependent differences in potency and gonadotropin specificity. While it is important to keep in mind that observations are limited to only a few teleost species, data to date suggest that hypophysiotropic nonapeptide systems stimulate Lh release in teleost fishes similar to the situation reported in rodents and humans (139, 140).
3.3.3 Endocrine and paracrine roles of nonapeptides in gonads
Both nonapeptides and their receptors have also been identified in ovaries and testes, and roles for nonapeptides in the regulation key gonadal functions via endocrine and paracrine signaling have been reported.
3.3.3.1 Steroidogenesis
Effects of nonapeptides on male steroidogenesis have been reported in testicular cultures of the rainbow trout (141). Testosterone production stimulated by Avp and Oxt has been observed in immature but not mature testes in vitro. Exposure to Avp elicited a stronger maximal response in T production compared baseline production (6-fold) than Oxt (4-fold). The maximally active concentration of 100 nM Avp was furthermore found to augment dose-dependent Lh-stimulated T production, suggesting a synergistic role. In a similar study in chanchita, Avp-stimulated T production in testes incubated in vitro dose-dependently, reaching a significant, 2-fold increase at 50 nM (54). A limitation of these two studies is that the authors measured T, which is the prohormone for the more potent teleost sex steroids: 11-KT and E2. Unfortunately, nonapeptide receptor antagonists were not used in these experiments to probe specific receptor involvement. Since recent gene expression and in-situ hybridization data suggests a role for avpr1aa and avpr2aa receptors (Table 3), additional studies with teleost-validated antagonists are warranted to probe the molecular mechanistic basis involved in Avp-induced T production in testes.
Several studies have investigated the role of nonapeptides on ovarian steroidogenesis. While a stimulatory role for Oxt on circulating E2 has been reported in female goldfish in vivo (87), it was not investigated whether these effects are linked to prior increases in Lh (86) or mediated via direct action at the ovary. In contrast, a comprehensive study determining the role of Avp and Oxt on in vitro ovarian steroidogenesis at different seasonal reproductive developmental stages was conducted in the air-breathing catfish (142). Dose-dependent, biphasic stimulatory effects of Avp on E2 production for pre-vitellogenic ovarian tissue were reported. In contrast, Avp produced a dose- and time-dependent inhibition of E2 production in early postvitellogenic ovaries (142). In comparison, Oxt produced a low, yet significant, stimulation of E2 production without any dose effect in the previtellogenic ovaries, and a dose- and time-dependent inhibition like Avp in the early postvitellogenic ovary (142). The inhibitory effect of Avp on the E2 synthesis in the postvitellogenic ovary may be part of a trigger for the steroidogenic shift to decreased E2 in favour of synthesis of the maturation-inducing steroid (MIS) 17, 20β-dihydroxy-4-pregnen-3, 20-dione (17, 20β-DP) in air-breathing catfish (143). The MIS reinitiates oocyte meiosis up to the second metaphase (144).
Concurrent with the modulation of E2 synthesis, Avp and, to a lesser extent, Oxt also stimulated progesterone (P4) production (142). The Avp-stimulated increase in P4 was generally dose-dependent in pre- and early post-vitellogenic ovaries, reaching approximately a 30% increase in production compared to baseline at high doses (142). In late postvitellogenic ovaries, concentrations as low as 1 nM Avp induced a 60% increase over baseline concentrations (142). This effect was similar to that of hCG, and combined administration of 20 IU hCG and Oxt was found to be additive at least after 16 h incubation. Avp stimulated 17-hydroxyprogesterone (17-OHP4) synthesis in the previtellogenic phase ovaries following both 8 h and 16 h incubation (142). Avp stimulated the production of 17,20β-Dihydroxy-4-pregnen-3-one (17,20β-DP), which acts as maturation induced steroid in this species, about 2-fold more in the spawning phase than pre-spawning phase, similar to hCG. The stimulatory effect of Oxt was several-fold lower compared to Avp and occurred at higher concentrations (142). The combination of Avp and hCG elicited a cumulative effect on the 17,20β-DP level especially after 16 h of incubation in the spawning phase (142). The authors concluded that Avp was more potent than Oxt to stimulate the progestin pathway, and that Avp paralleled the actions of hCG. The finding that hCG and steroid hormones (E2, P4 and 17, 20β-DP) stimulate ovarian Avp production suggests a positive feedback loop (145) underscoring the functional significance of Avp in follicular growth, maturation, and ovulation. The stimulatory effect of Avp on ovarian P4 secretion is conserved as similar actions for AVP family peptides have been reported for chicken, mouse, and cow in vitro (146).
3.3.3.2 Gametogenesis, gamete release and parturition
In the air-breathing catfish ovaries, Avp induces germinal vesicle breakdown (GVBD) and ovulation in a dose- and time-dependent manner (145). In this experiment, postvitellogenic follicles were co-incubated with Avp and an AVPR1 antagonist (deamino-Pen1, O-Me-Try2, Arg8 vasopressin), an AVPR2 antagonist (1-adamantane acetyl O-Et-D-Try2Val4, Abu6, Arg8,9 vasopressin), or both. GVBD, ovulation and 17,20β-DP concentration were inhibited or reduced by 92-94% after 24h co-incubation with both antagonists. The AVPR1 antagonist inhibited GVBD and ovulation by 82-83%, and the MIS concentration by 70%. The AVPR2 antagonist inhibited GVBD, ovulation and MIS concentration by 29%, 26% and 15%, respectively. The results show that the effects of Avp are mediated mainly by Avpr1 receptors with a minor role for Avpr2 receptors.
Prostaglandins (PGs) have a critical role in diverse aspects of reproduction in vertebrates (147). The cyclooxygenase inhibitor indomethacin can block MIS-induced final oocycte maturation and ovulation in yellow perch, Perca flavescens, and Atlantic croaker, indicating dependence on PGs (148, 149) The functional relationship between Avp and PGs was investigated in air-breathing catfish (150): Avp stimulated PGF2α and PGE2 levels in a dose- and time-dependent manner in vitro and the effects were similar to that produced by hCG. Both Avp and hCG-induced stimulation of PG levels were inhibited by indomethacin, supporting involvement of cyclooxygenase. The Avp stimulation of PG levels was strongly inhibited by the AVPR1 receptor antagonist but not by the AVPR2 receptor antagonist. Indomethacin inhibited the Avp and hCG-induced GVBD and ovulation. Both PGF2α and PGE2 stimulated GVBD and ovulation in a dose- and time-dependent manner and PGF2α was more effective than PGE2. Taken together, these observations highlight a relationship between Avp and PGs, and their interaction in the control of oocyte maturation and ovulation.
Follicular or oocyte hydration is a phenomenon conspicuous and widespread in marine and catadromous fish eggs associated with follicular and oocyte maturation (FOM) and ovulation (151), and this process is retained to some extent in freshwater and anadromous fishes. Singh and Joy (152) reported a 23% rise in oocyte water content during the FOM and ovulation in H. fossilis with Avp eliciting a significant effect on oocyte water content, diameter, volume, osmolality, Na+K+ ATPase activity, Na+, K+, Mg2+, Ca2+ concentration, GVBD and ovulation, similar to hCG. The combination of Avp and hCG produced a higher effect. In a further study, Acharjee et al. reported that Avp regulates aqp1ab, (ovary-specific aquaporin 1ab) expression through an Avpr2 receptor (153, 154), which is linked to the cAMP-PKA pathway, similar to AVP in mammalian kidney tubules.
The involvement of nonapeptides in sperm release was first demonstrated in the killifish, Fundulus heteroclitus, in which fish and mammalian neurohypophyseal preparations as well as synthetic OXT initiated a spawning reflex response (155). The relative effectiveness of the nonapeptides to induce spawning reflex in the killifish was estimated to be the highest and equipotent for AVP and Avp, followed by Oxt and OXT (155). However, concentrations used in these original studies were high compared to physiological concentrations. In male African sharptooth catfish testes slices 30-min incubation with OXT (at 10 IU), but not Oxt, AVP, epinephrine, PGF2α, LH and pituitary extracts increased milt release (156). In male walking catfish, nonapeptides and their nanotube composites designed for slower release were tested for their efficacy to promote stripping of milt by abdominal massage (157). Both naked or nano-conjugated nonapeptides increased strippable milt concentrations without altering reproductive success of fertilized eggs and increased the expression of the steroidogenesis pathway enzymes star, 3bhsd, 17bhsd, cyp17a1a, ands cyp11a1a (157).
Regarding parturition in teleosts, nonapeptides have been reported to stimulate premature parturition in the guppy, Poecilia reticulata, an ovoviviparous teleost (82, 158). The injection of Avp, Oxt and PGs to guppy, Poecilia reticulata, a live-bearing teleost, induced premature parturition (157). Both Avp and Oxt stimulated cox2 mRNA expression in guppy ovaries in vitro, which in the case of Avp, but not Oxt, translated into increased PG concentrations (82). Together this data suggests that the nonapeptide-dependent stimulation of premature parturition in guppies is mediated by PG. Interestingly, both Avp and Oxt exposure upregulated a guppy oxtr paralogue, suggesting interaction between ovarian nonapeptide systems in the guppy ovary (82). In zebrafish, a recent study investigating downstream effectors linked to a reduced ovulation phenotype observed in female chromosome 23 miR-200 cluster KO mutants showed that co-injection of hCG, Avp and Oxt, but not injection of synthetic human GnRH and LH analogues were able to partially rescue the phenotype (159). Together, this data suggests a Gnrh-independent role for Avp and Oxt in zebrafish ovulation. Nevertheless, early comparative evidence from teleost fishes has demonstrated that roles for nonapeptides on male and female spawning cannot be generalized in teleost fish and are possibly indirect following application of supraphysiological concentrations (160).
While we acknowledge a generally high degree of evolutionary conservation of nonapeptide genes and the gross (neuro)anatomical distribution of their expression, differences in reproductive function of nonapeptides between teleost species certainly exist. We have integrated the current state of knowledge of nonapeptides on central and HPG axis components of teleost reproduction in Figure 4. It is anticipated, however, that additional detailed comparative studies will uncover diversity of nonapeptide-dependent regulation of teleost reproductive physiology.
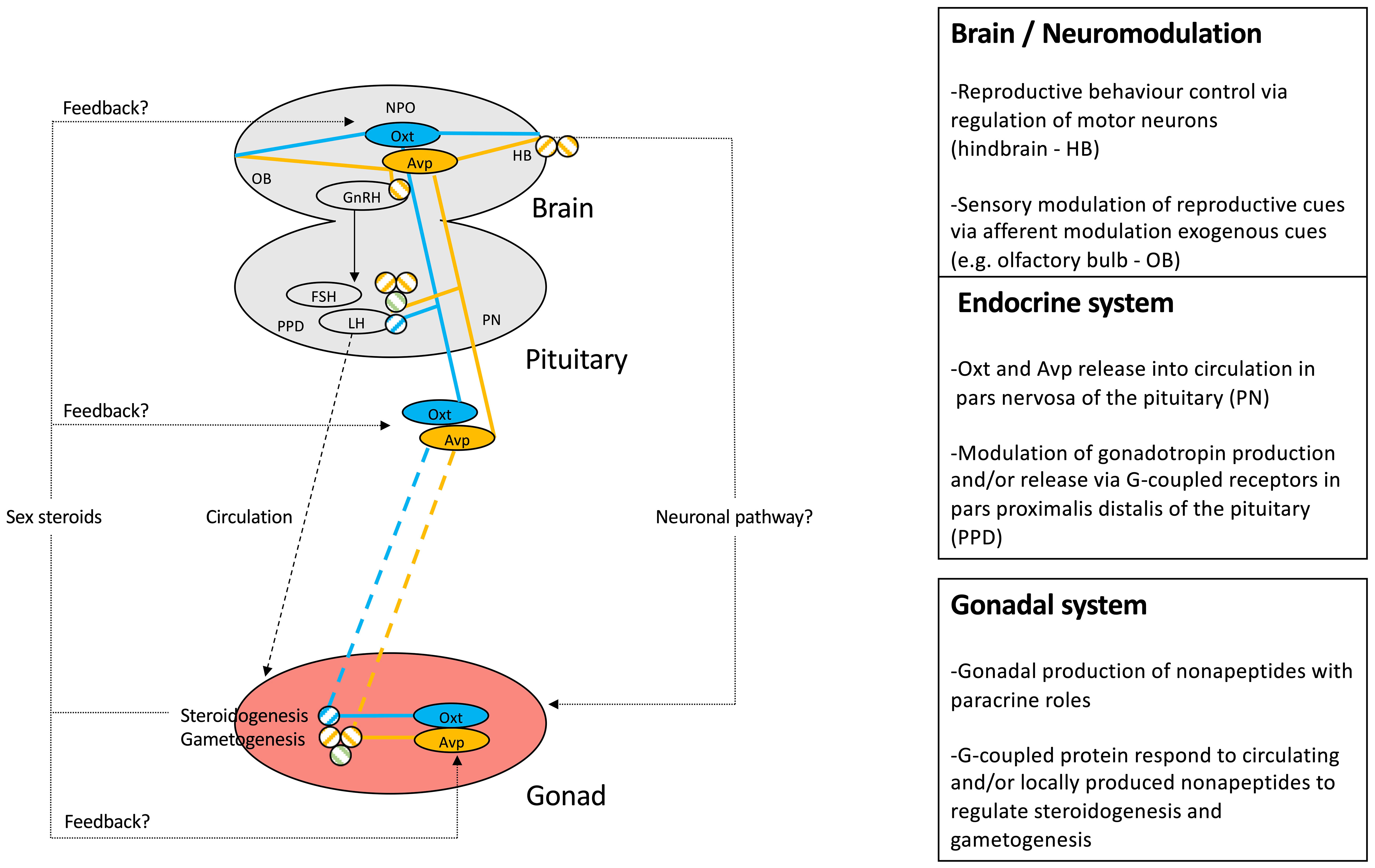
Figure 4 Schematic representation of mechanistic knowledge of nonapeptide roles in teleost reproduction. The Avp system is highlighted in yellow, while the Oxt system is represented in blue. Circled nonapeptides indicate sites of synthesis, while connecting lines represent neuronal, endocrine, and paracrine pathways. Specific receptor involvement in regulatory function of HPG axis components is symbolized as defined in Figure 2.
4 Translational aspects
As evolutionarily conserved systems regulating reproduction (3), research investigating roles of nonpeptides in teleost fish reproduction in detail have translational relevance in the areas of aquaculture and species conservation; both of which rely on methods informed by mechanistic understanding of reproductive physiology. As such, we anticipate that modulation of nonapeptide function in teleost species has significant potential to stimulate and possibly coordinate behavioural and endocrine processes necessary to promote reproduction for species in captivity (161). A third area of translational relevance is ecotoxicology. We review emerging evidence suggesting that neuroendocrine disruption of teleost nonapeptides may be linked to decreased reproductive success in teleost fishes, an ecologically meaningful endpoint (162).
4.1 Neuroendocrine disruption
In teleost fish, relatively recent studies have investigated Avp and Oxt nonapeptide systems as targets of different groups of aquatic contaminants. Histological studies of POA magnocellular neurons revealed that a six-month exposure of channel catfish, Channa punctatus, to inorganic mercury at a concentration of 10 µg/L resulted in smaller and less active Avp‐secreting neurons (163). Persistent organic pollutants have also been shown to affect nonapeptide systems in teleost fishes: in Atlantic Croaker, a four week daily dietary exposure (2 and 8 μg/g body weight) to the planar polychlorinated biphenyl congener 3,3′,4,4′-tetrachlorobiphenyl (PCB-77) significantly reduced hypothalamic expression of avpr1a mRNA and Avpr1a protein levels, as well as (co-localized) gnrh1 mRNA levels in the brain (78).
Pharmaceuticals and plasticizers are other major environmental contaminants with reported effects on nonapeptide systems. Repeated intraperitoneal injections of pharmacological doses (5 µg/g) of the selective serotonin reuptake inhibitor and aquatic contaminant fluoxetine (FLX) significantly reduced oxt mRNA levels in female goldfish telencephalon and hypothalamus, an effect that was linked to reduced circulating E2 concentrations (85). Subsequent waterborne FLX exposure studies in both female and male goldfish revealed that oxt transcript abundance in the same tissues was not affected by a two- week exposure to FLX at 540 ng/L and 54 μg/L concentrations, but that the same two-week exposure to waterborne fluoxetine significantly diminished releaser pheromone PGF2α-induced increases in oxt mRNA (94, 164). Similarly, targeted gene expression analysis of zebrafish larvae acutely exposed to 50 and 500 ng/L FLX for 96 h (165) as well as transcriptomic screens of whole brains collected from a wild zebrafish population exposed to 100 μg/L FLX at a concentration for two weeks (166) identified oxt transcripts as being differentially expressed in FLX-exposed fish compared to unexposed control fish. Together, these studies reveal that the Oxt system is responsive to FLX at both early developmental and adult life-stages, raising the possibility of mediating organisational as well as activational effects. Repeated injection of pharmacological concentrations of 6 μg/g body weight FLX over a period of two weeks significantly reduced avt transcript abundance in giganto-, magno- and parvocellular neurons of the POA in male bluehead wrasses, an effect that correlated with decreased territorial aggression (167). The responsiveness of teleost nonapeptide systems to FLX corresponds to several observations in mammalian models (168, 169) and suggests an evolutionarily conserved serotonin-dependent regulation of these systems (87, 170, 171).
In Japanese medaka chronically exposed to environmentally relevant and high concentrations of waterborne metamphetamine for a period of 90 days, a dose-dependent, significant increase in whole brain oxtr mRNA and Oxt peptide were observed (172). Because both FLX and metamphetamine affect neurotransmitter systems and neuroactive contaminants may exert reproductive effects, at least in part, via nonapeptidergic systems, these findings support the concept of neuroendocrine disruption (173). In line with this interpretation, a meta-analysis of transcriptomic screens of the goldfish hypothalamus identified oxt as the single transcript affected by drugs modulating serotonergic, dopaminergic, and GABAergic systems, all of which have established roles in goldfish reproduction (137). Concurrent with previously described responsiveness of teleost POA nonapeptide systems to sex steroids, zebrafish chronically exposed to 1,10 and 30 μg/L Bisphenol A (BPA), a weakly estrogenic compound, as well as 1 μg/L E2, exhibited complex dose-dependent and sex-specific effects on whole brain nonapeptide and nonapeptide receptor gene expression levels, which corresponded with alterations in social but not overall locomotor behaviour (174, 175). Similarly, developmental (2-5dpf) exposure to BPA and its replacement compound Bisphenol S (BPS) in the low μM range revealed non-linear alteration of oxt and Oxt protein abundance in association with quantifiable behavioural disruptions at 21dpf (176, 177). There is a need to study the involvement of nonapeptide systems in mediating organizational and/or activational effects of endocrine disrupting chemicals. This is an area understudied in teleost fish (178) compared to rodent models (179, 180), and will inform the possible development of teleost nonapeptides as functional biomarkers relevant to reproductive function in teleost fishes.
5 The state of the art and current limitations
Having critically reviewed the current state of knowledge regarding reproductive roles of teleost nonapeptides, we conclude by briefly discussing key insights and current limitations in the field. We furthermore suggest conceptual and technical approaches in the hope of stimulating collaborative research in the field.
1. It is well-appreciated that teleosts are champions of reproductive plasticity and diversity. There is a high degree of evolutionary conservation of nonapeptides and their gross (neuro)anatomical distribution. However, novel resources such as genomic sequences and detailed comparative investigation increasingly reveal areas of plasticity of teleost nonapeptide systems. A point in case is the nonapeptide receptor inventory, which exhibits differences between teleost fishes and other vertebrates, but, importantly, also within teleost fish lineages such as the otocephala and euteleosti (59). It will thus be important to address functional differences in nonapeptide receptor paralogue expression, regulation, and function to assess their potential roles in mediating reproductive functions in a reproductively diverse fishes.
2. While increasing comparative studies to delineate plasticity of nonapeptides in regulating reproduction in the diverse group of teleost fishes will be important, it will be equally crucial to comprehensively study the reproductive roles of nonapeptides within single teleost fish species. In reviewing the current knowledge of the role of nonapeptides in teleost reproduction, it has become clear that, apart from detailed studies in the Asian stinging catfish (50, 84) and, to a lesser degree, the canchita cichlid (54), few if any studies have investigated the role of nonapeptides across the different levels of the HPG axis within a single teleost species. The need for detailed studies within single fish species becomes even more clear when considering that research investigating behavioural and endocrine roles of nonpeptides in teleost reproduction has largely co-existed in isolation focussing on separate fish species. However, there is a need to consider (nonapeptide-regulated) courtship behaviour in the context of HPG axis function (165). Investigation of the salience hypothesis and possible roles for nonapeptides in the integration of reproductive cues are also warranted.
3. The recent generation of nonapeptide and/or nonapeptide receptor knock-out models in genetically-tractable model systems such as zebrafish (67) and medaka (64, 118) may hold particular promise. However, neither of these models have, to-date, been used to explore nonapeptide effects on the HPG axis. Receptor-specific knock-outs may furthermore prove fruitful to probe the role of virtually uncharacterized nonapeptide receptors such as avtr2l and generally circumvent possible specificity issues linked to the use of mammalian nonapeptide agonists and antagonists. Furthermore, GFP-reporter lines have been described in zebrafish, at least for Oxt (68, 69). While such lines represent powerful tools to investigate regulation and activation of central and peripheral nonapeptide systems in response to environmental and endogenous cues relevant to reproduction (35), few studies have been conducted in this area. Transgenic reporter lines may also allow endocrine disrupting) chemical screening for effects on nonapeptide systems. Adult-specific transgenic ablation studies and optogenetic approaches may in the future permit specific investigation of organisational and activational reproductive effects of the nonapeptides.
4. Previous co-localization experiments (43, 44) and more recent single-cell techniques (28) have demonstrated co-localization of nonapeptides with other neuropeptide regulators of reproduction in neuroendocrine neurons. Thus, future functional studies should investigate co-release and interactions between nonapeptides and co-expressed reproductive regulators to quantify potential combinatorial effects on courtship behaviour and HPG axis function.
Author contributions
JM, DR, KS,RC, KJ, and VLT contributed to conception and design of the review. DR conducted in silico analyses. JM wrote the first draft of the manuscript. DR, KS, RC, KJ and VLT wrote sections of the manuscript. All authors contributed to manuscript revision, read, and approved the submitted version.
Funding
The authors gratefully acknowledge the following funding sources: NSERC-Discovery Grants (Grant no. 147476 to JM, and to VLT), an NSERC-CGS-D (to KS), and partial funding from the Institute of Eminence of the Banaras Hindu University (to RC).
Conflict of interest
The authors declare that the research was conducted in the absence of any commercial or financial relationships that could be construed as a potential conflict of interest.
Publisher’s note
All claims expressed in this article are solely those of the authors and do not necessarily represent those of their affiliated organizations, or those of the publisher, the editors and the reviewers. Any product that may be evaluated in this article, or claim that may be made by its manufacturer, is not guaranteed or endorsed by the publisher.
References
1. Odekunle EA, Elphick MR. Comparative and evolutionary physiology of Vasopressin/Oxytocin type neuropeptide signaling in invertebrates. Front Endocrinol (2020) 11:225. doi: 10.3389/fendo.2020.00225
2. Kawada T, Sekiguchi T, Itoh Y, Ogasawara M, Satake H. Characterization of a novel vasopressin/oxytocin superfamily peptide and its receptor from an ascidian, ciona intestinalis. Peptides (2008) 29:1672–8. doi: 10.1016/j.peptides.2008.05.030
3. Gwee P-C, Tay B-H, Brenner S, Venkatesh B. Characterization of the neurohypophysial hormone gene loci in elephant shark and the Japanese lamprey: origin of the vertebrate neurohypophysial hormone genes. BMC Evol Biol (2009) 9:47. doi: 10.1186/1471-2148-9-47
4. Matsubara S, Shiraishi A, Osugi T, Kawada T, Satake H. The regulation of oocyte maturation and ovulation in the closest sister group of vertebrates. Elife (2019) 8:e49062. doi: 10.7554/eLife.49062
5. Banerjee P, Chaube R, Joy KP. Molecular cloning, sequencing, and tissue expression of vasotocin and isotocin precursor genes from ostariophysian catfishes: phylogeny and evolutionary considerations in teleosts. Front Neurosci (2015) 9:166. doi: 10.3389/fnins.2015.00166
6. Banerjee P, Chaube R, Joy KP. Molecular cloning and characterisation of an isotocin paralogue ([V8] isotocin) in catfishes (superorder ostariophysi): Origin traced likely to the fish-specific whole genome duplication. J Neuroendocrinol (2018) 30:e12647. doi: 10.1111/jne.12647
7. Panopoulou G, Poustka AJ. Timing and mechanism of ancient vertebrate genome duplications – the adventure of a hypothesis. Trends Genet (2005) 21:559–67. doi: 10.1016/j.tig.2005.08.004
8. Acher R. Molecular evolution of fish neurohypophysial hormones: Neutral and selective evolutionary mechanisms. Gen Comp Endocrinol (1996) 102:157–72. doi: 10.1006/gcen.1996.0057
9. Acher R, Chauvet J. Structure, processing, and evolution of the neurohypophysial hormone-neurophysin precursors. Biochimie (1988) 70:1197–207. doi: 10.1016/0300-9084(88)90185
10. Acher R, Chauvet J, Chauvet MT, Crepy D. Neurohypophysial hormones from fish: isolation of a vasotocin from the whiting-pour (Gadus luscus l.). Biochim Biophys Acta (1961) 51:419–20. doi: 10.1016/0006-3002(61)90198-6
11. Heller H, Pickering BT. Neurohypophysial hormones of non-mammalian vertebrates. J Physiol (1961) 155:98–114. doi: 10.1113/jphysiol.1961.sp006616
12. Chauvet J, Chauvet-Lenci MT, Acher R. Some observations on the neurohypophysial hormones of a teleostfish, the hake (Merluccius merluccius l.). C R Seances Soc Biol Fil (1961) 252:2145–7.
13. Acher R, Chauvet J, Chauvet MT. Phylogeny of the neurohypophysial hormones. Two New active pept isolated cartilaginous fish Squalus acanthias. Eur J Biochem (1972) 29:12–9. doi: 10.1111/j.1432-1033.1972.tb01951.x
14. Chauvet J, Rouille Y, Chauveau C, Chauvet MT, Acher R. Special evolution of neurohypophysial hormones in cartilaginous fishes: asvatocin and phasvatocin, two oxytocin-like peptides isolated from the spotted dogfish (Scyliorhinus caniculus). PNAS (1994) 91:11266–70. doi: 10.1073/pnas.91.23.11266
15. Hyodo S, Tsukada T, Takei Y. Neurohypophysial hormones of dogfish, triakis scyllium: structures and salinity-dependent secretion. Gen Comp Endocrinol (2004) 138:97–104. doi: 10.1016/j.ygcen.2004.05.009
16. Buchholz H, Schönrock C, Fehr S, Richter D. Sequence analysis of a cDNA encoding an isotocin precursor and localization of the corresponding mRNA in the brain of the cartilaginous fish torpedo marmorata. Mol Mar Biol Biotechnol (1995) 4:179–84.
17. Gwee P-C, Amemiya CT, Brenner S, Venkatesh B. Sequence and organization of coelacanth neurohypophysial hormone genes: evolutionary history of the vertebrate neurohypophysial hormone gene locus. BMC Evol Biol (2008) 8:93. doi: 10.1186/1471-2148-8-93
18. Acher R, Chauvet J, Chauvet MT, Crepy D. Isolation of a new neurohypophysial hormone, isotocin, present in bony fish. Biochim Biophys Acta (1962) 58:624–5. doi: 10.1016/0006-3002(62)90085-9
19. Acher R, Chauvet J, Chauvet MT, Rouille Y. Unique evolution of neurohypophysial hormones in cartilaginous fishes: possible implications for urea-based osmoregulation. J Exp Zool (1999) 284:475–84. doi: 10.1002/(sici)1097-010x
20. Hyodo S, Ishii S, Joss JMP. Australian Lungfish neurohypophysial hormone genes encode vasotocin and [Phe2] mesotocin precursors homologous to tetrapod-type precursors. Proc Natl Acad Sci USA (1997) 94:13339–44. doi: 10.1073/pnas.94.24.13339
21. Pasquier J, Cabau C, Nguyen T, Jouanno E, Severac D, Braasch I, et al. Gene evolution and gene expression after whole genome duplication in fish: the PhyloFish database. BMC Genomics (2016) 17:368. doi: 10.1186/s12864-016-2709-z
22. Suzuki M, Hyodo S, Urano A. Cloning and sequence analyses of vasotocin and isotocin precursor cDNAs in the masu salmon, oncorhynchus masou: evolution of neurohypophysial hormone precursors. Zoolog Sci (1992) 9:157–67. doi: 10.1006/gcen.1993.1165
23. Heierhorst J, Morley SD, Figueroa J, Krentler C, Lederis K, Richter D. Vasotocin and isotocin precursors from the white sucker, catostomus commersoni: cloning and sequence analysis of the cDNAs. Proc Natl Acad Sci USA (1989) 86:5242–6. doi: 10.1073/pnas.86.14.5242
24. Acharjee A, Chaube R, Joy KP. Reproductive stage- and sex-dependant effects of neurohypophyseal nonapeptides on gonadotropin subunit mRNA expression in the catfish heteropneustes fossilis: An in vitro study. Gen Comp Endocrinol (2018) 260:80–9. doi: 10.1016/j.ygcen.2018.01.001
25. Rodriguez-Santiago M, Nguyen J, Winton LS, Weitekamp CA, Hofmann HA. Arginine vasotocin preprohormone is expressed in surprising regions of the teleost forebrain. Front Endocrinol (Lausanne) (2017) 8:195. doi: 10.3389/fendo.2017.00195
26. Loveland JL, Hu CK. Commentary: Arginine vasotocin preprohormone is expressed in surprising regions of the teleost forebrain. Front Endocrinol (Lausanne) (2018) 9:63. doi: 10.3389/fendo.2018.00063
27. Gutnick A, Blechman J, Kaslin J, Herwig L, Belting H-G, Affolter M, et al. The hypothalamic neuropeptide oxytocin is required for formation of the neurovascular interface of the pituitary. Dev Cell (2011) 21:642–54. doi: 10.1016/j.devcel.2011.09.004
28. Herget U, Ryu S. Coexpression analysis of nine neuropeptides in the neurosecretory preoptic area of larval zebrafish. Front Neuroanat (2015) 9:2. doi: 10.3389/fnana.2015.00002
29. Herget U, Gutierrez-Triana JA, Salazar Thula O, Knerr B, Ryu S. Single-cell reconstruction of oxytocinergic neurons reveals separate hypophysiotropic and encephalotropic subtypes in larval zebrafish. eNeuro (2017) 4:1–16. doi: 10.1523/ENEURO.0278-16.2016
30. Kagawa N, Honda A, Zenno A, Omoto R, Imanaka S, Takehana Y, et al. Arginine vasotocin neuronal development and its projection in the adult brain of the medaka. Neurosci Lett (2016) 613:47–53. doi: 10.1016/j.neulet.2015.12.049
31. Demski LS, Sloan HE. A direct magnocellular-preopticospinal pathway in goldfish: Implications for control of sex behavior. Neurosci Lett (1985) 55:283–8. doi: 10.1016/0304-3940(85)90449-5
32. Walton JC, Waxman B, Hoffbuhr K, Kennedy M, Beth E, Scangos J, et al. Behavioral effects of hindbrain vasotocin in goldfish are seasonally variable but not sexually dimorphic. Neuropharmacology (2010) 58:126–34. doi: 10.1016/j.neuropharm.2009.07.018
33. Macey MJ, Pickford GE, Peter RE. Forebrain localization of the spawning reflex response to exogenous neurohypophysial hormones in the killifish. Fundulus heteroclitus. J Exp Zool (1974) 190:269–79. doi: 10.1002/jez.1401900303
34. Yabuki Y, Koide T, Miyasaka N, Wakisaka N, Masuda M, Ohkura M, et al. Olfactory receptor for prostaglandin F2α mediates male fish courtship behavior. Nat Neurosci (2016) 19:897–904. doi: 10.1038/nn.4314
35. Altmieme Z, Jubouri M, Touma K, Coté G, Fonseca M, Julian T, et al. A reproductive role for the nonapeptides vasotocin and isotocin in male zebrafish (Danio rerio). Comp Biochem Physiol B Biochem Mol Biol (2019) 238:110333. doi: 10.1016/j.cbpb.2019.110333
36. Forlano PM, Bass AH. Neural and hormonal mechanisms of reproductive-related arousal in fishes. Horm Behav (2011) 59:616–29. doi: 10.1016/j.yhbeh.2010.10.006
37. Goodson JL, Bass AH. Forebrain peptides modulate sexually polymorphic vocal circuitry. Nature (2000) 403:769–72. doi: 10.1038/35001581
38. Goodson JL, Evans AK, Bass AH. Putative isotocin distributions in sonic fish: Relation to vasotocin and vocal-acoustic circuitry. J Comp Neurol (2003) 462:1–14. doi: 10.1002/cne.10679
39. Silva A, Perrone R, Macadar O. Environmental, seasonal, and social modulations of basal activity in a weakly electric fish. Physiol Behav (2007) 90:525–36. doi: 10.1016/j.physbeh.2006.11.003
40. Perrone R, Migliaro A, Comas V, Quintana L, Borde M, Silva A. Local vasotocin modulation of the pacemaker nucleus resembles distinct electric behaviors in two species of weakly electric fish. J Physiol Paris (2014) 108:203–12. doi: 10.1016/j.jphysparis.2014.07.007
41. Batten Trevor FC. Ultrastructural characterization of neurosecretory fibres immunoreactive for vasotocin, isotocin, somatostatin, LHRH and CRF in the pituitary of a teleost fish, poecilia latipinna. Cell Tissue Res (1986) 244: 661–72. doi: 10.1007/BF00212547
42. Groves DJ, Batten TFC. Direct control of the gonadotroph in a teleost, poecilia latipinna. Gen Comp Endocrinol (1986) 62:315–26. doi: 10.1016/0016-6480(86)90122-X
43. Maejima K, Oka Y, Park MK, Kawashima S. Immunohistochemical double-labeling study of gonadotropin-releasing hormone (GnRH)-immunoreactive cells and oxytocin-immunoreactive cells in the preoptic area of the dwarf gourami, colisa lalia. J Neurosci Res (1994) 20:189–93. doi: 10.1016/0168-0102(94)90037-X
44. Canosa LF, Lopez GC, Scharrig E, Lesaux-Farmer K, Somoza GM, Kah O, et al. Forebrain mapping of secretoneurin-like immunoreactivity and its colocalization with isotocin in the preoptic nucleus and pituitary gland of goldfish. J Comp Neurol (2011) 519:3748–65. doi: 10.1002/cne.22688
45. Zhao E, Basak A, Wong AOL, Ko W, Chen A, López GC, et al. The secretogranin II-derived peptide secretoneurin stimulates luteinizing hormone secretion from gonadotrophs. Endocrinology (2009) 150:2273–82. doi: 10.1210/en.2008-1060
46. Mitchell K, Zhang WS, Lu C, Tao B, Chen L, Hu W, et al. Targeted mutation of secretogranin-2 disrupts sexual behavior and reproduction in zebrafish. Proc Natl Acad Sci USA (2020) 117:12772–83. doi: 10.1073/pnas.2002004117
47. Batten TFC, Cambre ML, Moons L, Vandesande F. Comparative distribution of neuropeptide-immunoreactive systems in the brain of the green molly, poecilia latipinna. J Comp Neurol (1990) 302:893–919. doi: 10.1002/cne.903020416
48. Batten TFC, Moons L, Vandesande F. Innervation and control of the adenohypophysis by hypothalamic peptidergic neurons in teleost fishes: EM immunohistochemical evidence. Microsc Res Tech (1999) 44:19–35. doi: 10.1002/(SICI)1097-0029(19990101)44:1<19::AID-JEMT4>3.0.CO;2-L
49. Sokołowska E, Gozdowska M, Kulczykowska E. Nonapeptides arginine vasotocin and isotocin in fishes: Advantage of bioactive molecules measurement. Front Mar Sci (2020) 7:610. doi: 10.3389/fmars.2020.00610
50. Singh V, Joy KP. Immunocytochemical localization, HPLC characterization, and seasonal dynamics of vasotocin in the brain, blood plasma and gonads of the catfish heteropneustes fossilis. Gen Comp Endocrinol (2008) 159:214–25. doi: 10.1016/j.ygcen.2008.09.003
51. Saito D, Komatsuda M, Urano A. Functional organization of preoptic vasotocin and isotocin neurons in the brain of rainbow trout: central and neurohypophysial projections of single neurons. Neuroscience (2004) 124:973–84. doi: 10.1016/j.neuroscience.2003.12.038
52. Bobe J, Montfort J, Nguyen T, Fostier A. Identification of new participants in the rainbow trout (Oncorhynchus mykiss) oocyte maturation and ovulation processes using cDNA microarrays. Reprod Biol Endocrinol (2006) 4:39. doi: 10.1186/1477-7827-4-39
53. Chou M-Y, Hung J-C, Wu L-C, Hwang S-PL, Hwang P-P. Isotocin controls ion regulation through regulating ionocyte progenitor differentiation and proliferation. Cell Mol Life Sci (2011) 68:2797–809. doi: 10.1007/s00018-010-0593-2
54. Ramallo MR, Grober M, Cánepa MM, Morandini L, Pandolfi M. Arginine-vasotocin expression and participation in reproduction and social behavior in males of the cichlid fish cichlasoma dimerus. Gen Comp Endocrinol (2012) 179:221–31. doi: 10.1016/j.ygcen.2012.08.015
55. Banerjee P, Joy KP, Chaube R. Structural and functional diversity of nonapeptide hormones from an evolutionary perspective: A review. Gen Comp Endocrinol (2017) 241:4–23. doi: 10.1016/j.ygcen.2016.04.025
56. Mayasich SA, Clarke BL. Vasotocin and the origins of the vasopressin/oxytocin receptor gene family. Vitam Horm (2020) 113:1–12. doi: 10.1016/bs.vh.2019.08.018
57. Theofanopoulou C. Reconstructing the evolutionary history of the oxytocin and vasotocin receptor gene family: Insights on whole genome duplication scenarios. Dev Biol (2021) 479:99–106. doi: 10.1016/j.ydbio.2021.07.012
58. Theofanopoulou C, Gedman G, Cahill JA, Boeckx C, Jarvis ED. Universal nomenclature for oxytocin–vasotocin ligand and receptor families. Nature (2021) 592:747–55. doi: 10.1038/s41586-020-03040-7
59. Ocampo Daza D, Bergqvist CA, Larhammar D. The evolution of oxytocin and vasotocin receptor genes in jawed vertebrates: A clear case for gene duplications through ancestral whole-genome duplications. Front Endocrinol (2022) 12:792644. doi: 10.3389/fendo.2021.792644
60. Yamaguchi Y, Kaiya H, Konno N, Iwata E, Miyazato M, Uchiyama M, et al. The fifth neurohypophysial hormone receptor is structurally related to the V2-type receptor but functionally similar to V1-type receptors. Gen Comp Endocrinol (2012) 178:519–28. doi: 10.1016/j.ygcen.2012.07.008
61. Gimpl G, Fahrenholz F. The oxytocin receptor system: Structure, function, and regulation. Physiol Rev (2001) 81:629–83. doi: 10.1152/physrev.2001.81.2.629
62. Helfman G, Collette B, Facey D, Bowen BW. The diversity of fishes: biology, evolution, and ecology. 2nd ed. Chichester: Blackwell (2009).
63. Kline RJ, O’Connell LA, Hofmann HA, Holt GJ, Khan IA. The distribution of an AVT V1a receptor in the brain of a sex changing fish, epinephelus adscensionis. J Chem Neuroanat (2011) 42:72–88. doi: 10.1016/j.jchemneu.2011.06.005
64. Yokoi S, Naruse K, Kamei Y, Ansai S, Kinoshita M, Mito M, et al. Sexually dimorphic role of oxytocin in medaka mate choice. Proc Natl Acad Sci USA (2020) 117:4802–8. doi: 10.1073/pnas.1921446117
65. Semsar K, Kandel FLM, Godwin J. Manipulations of the AVT system shift social status and related courtship and aggressive behavior in the bluehead wrasse. Horm Behav (2001) 40:21–31. doi: 10.1006/hbeh.2001.1663
66. Singh O, Kumar S, Singh U, Bhute Y, Singru PS. Role of isotocin in the regulation of the hypophysiotropic dopamine neurones in the preoptic area of the catfish, clarias batrachus. J Neuroendocrinol (2016) 28:1–11. doi: 10.1111/jne.12441
67. Wee CL, Song E, Nikitchenko M, Herrera KJ, Wong S, Engert F, et al. Social isolation modulates appetite and avoidance behavior via a common oxytocinergic circuit in larval zebrafish. Nat Commun (2022) 13:2573. doi: 10.1038/s41467-022-29765-9
68. Blechman J, Amir-Zilberstein L, Gutnick A, Ben-Dor S, Levkowitz G. The metabolic regulator PGC-1α directly controls the expression of the hypothalamic neuropeptide oxytocin. J Neurosci (2011) 31:14835–40. doi: 10.1523/JNEUROSCI.1798-11.2011
69. Coffey CM, Solleveld PA, Fang J, Roberts AK, Hong S-K, Dawid IB, et al. Novel oxytocin gene expression in the hindbrain is induced by alcohol exposure: transgenic zebrafish enable visualization of sensitive neurons. PloS One (2013) 8:e53991. doi: 10.1371/journal.pone.0053991
70. Canosa LF, Chang JP, Peter RE. Neuroendocrine control of growth hormone in fish. Gen Comp Endocrinol (2007) 151:1–26. doi: 10.1016/j.ygcen.2006.12.010
71. Pouso P, Perrone R, Silva A. Immunohistochemical description of isotocin neurons and the anatomo-functional comparative analysis between isotocin and vasotocin systems in the weakly electric fish, gymnotus omaroum. Gen Comp Endocrinol (2021) 313:113886. doi: 10.1016/j.ygcen.2021.113886
72. Pouso P, Radmilovich M, Silva A. An immunohistochemical study on the distribution of vasotocin neurons in the brain of two weakly electric fish, gymnotus omarorum and brachyhypopomus gauderio. Tissue Cell (2017) 49:257–69. doi: 10.1016/j.tice.2017.02.003
73. Arias Padilla LF, Castañeda-Cortés DC, Rosa IF, Moreno Acosta OD, Hattori RS, Nóbrega RH, et al. Cystic proliferation of germline stem cells is necessary to reproductive success and normal mating behavior in medaka. Elife (2021) 10:e62757. doi: 10.7554/eLife.62757
74. Rawat A, Chaube R, Joy KP. In situ localization of vasotocin receptor gene transcripts in the brain-pituitary-gonadal axis of the catfish heteropneustes fossilis: a morpho-functional study. Fish Physiol Biochem (2019) 45:885–905. doi: 10.1007/s10695-018-0590-1
75. Rawat A, Chaube R, Joy KP. Molecular cloning, sequencing, and phylogeny of vasotocin receptor genes in the air-breathing catfish heteropneustes fossilis with sex dimorphic and seasonal variations in tissue expression. Fish Physiol Biochem (2015) 41:509–32. doi: 10.1007/s10695-015-0026-0
76. Lema SC, Sanders KE, Walti KA. Arginine vasotocin, isotocin and nonapeptide receptor gene expression link to social status and aggression in sex-dependent patterns. J Neuroendocrinol (2015) 27:142–57. doi: 10.1111/jne.12239
77. Iwasaki K, Taguchi M, Bonkowsky JL, Kuwada JY. Expression of arginine vasotocin receptors in the developing zebrafish CNS. Gene Expr Patterns (2013) 13:335–42. doi: 10.1016/j.gep.2013.06.005
78. Rahman MS, Kline RJ, Vázquez OA, Khan IA, Thomas P. Molecular characterization and expression of arginine vasotocin V1a2 receptor in Atlantic croaker brain: Potential mechanisms of its downregulation by PCB77. J Biochem Mol Toxicol (2020) 34: 1–12. doi: 10.1002/jbt.22500
79. Kline RJ, Holt GJ, Khan IA. Arginine vasotocin V1a2 receptor and GnRH-I co-localize in preoptic neurons of the sex changing grouper, epinephelus adscensionis. Gen Comp Endocrinol (2016) 225:33–44. doi: 10.1016/j.ygcen.2015.07.013
80. Huffman LS, O’Connell LA, Kenkel CD, Kline RJ, Khan IA, Hofmann HA. Distribution of nonapeptide systems in the forebrain of an African cichlid fish, astatotilapia burtoni. J Chem Neuroanat (2012) 44:86–97. doi: 10.1016/j.jchemneu.2012.05.002
81. Yang W, Zhang N, Wu Y, Zhang L, Zhang L, Zhang W. Oxytocin-like signal regulates lh cells directly but not fsh cells in the ricefield eel Monopterus albus †. Biol Reprod (2021) 104:399–409. doi: 10.1093/biolre/ioaa202
82. Lyu LK, Li JS, Wang XJ, Yao YJ, Li JF, Li Y, et al. Arg-vasotocin directly activates isotocin receptors and induces COX2 expression in ovoviviparous guppies. Front Endocrinol (2021) 12:617580. doi: 10.3389/fendo.2021.617580
83. Lema SC, Slane MA, Salvesen KE, Godwin J. Variation in gene transcript profiles of two V1a-type arginine vasotocin receptors among sexual phases of bluehead wrasse (Thalassoma bifasciatum). Gen Comp Endocrinol (2012) 179:451–64. doi: 10.1016/j.ygcen.2012.10.001
84. Joy KP, Chaube R. Vasotocin – a new player in the control of oocyte maturation and ovulation in fish. Gen Comp Endocrinol (2015) 221:54–63. doi: 10.1016/j.ygcen.2015.02.013
85. Mennigen JA, Martyniuk CJ, Crump K, Xiong H, Zhao E, Popesku J, et al. Effects of fluoxetine on the reproductive axis of female goldfish (Carassius auratus). Physiol Genomics (2008) 35:273–82. doi: 10.1152/physiolgenomics.90263.2008
86. Popesku JT, Mennigen JA, Chang JP, Trudeau VL. Dopamine d 1 receptor blockage potentiates AMPA-stimulated luteinising hormone release in the goldfish: D 1 r blockage potentiates AMPA-stimulated LH release. J Neuroendocrinol (2011) 23:302–9. doi: 10.1111/j.1365-2826.2011.02114.x
87. Mennigen JA, Volkoff H, Chang JP, Trudeau VL. The nonapeptide isotocin in goldfish: Evidence for serotonergic regulation and functional roles in the control of food intake and pituitary hormone release. Gen Comp Endocrinol (2017) 254:38–49. doi: 10.1016/j.ygcen.2017.09.008
88. Mahlmann S, Meyerhof W, Hausmann H, Heierhorst J, Schönrock C, Zwiers H, et al. Structure, function, and phylogeny of [Arg8] vasotocin receptors from teleost fish and toad. PNAS (1994) 91:1342–5. doi: 10.1073/pnas.91.4.1342
89. Hausmann H, Richters A, Kreienkamp HJ, Meyerhof W, Mattes H, Lederis K, et al. Mutational analysis and molecular modeling of the nonapeptide hormone binding domains of the [Arg8] vasotocin receptor. Proc Natl Acad Sci USA (1996) 93:6907–12. doi: 10.1073/pnas.93.14.6907
90. Hausmann H, Meyerhof W, Zwiers H, Lederis K, Richter D. Teleost isotocin receptor: structure, functional expression, mRNA distribution and phylogeny. FEBS Lett (1995) 370:227–30. doi: 10.1016/0014-5793(95)00832-T
91. Landin J, Hovey D, Xu B, Lagman D, Zettergren A, Larhammar D, et al. Oxytocin receptors regulate social preference in zebrafish. Sci Rep (2020) 10:5435. doi: 10.1038/s41598-020-61073-4
92. Trudeau V. Neuroendocrine regulation of gonadotrophin II release and gonadal growth in the goldfish, carassius auratus. Rev Reprod (1997) 2:55–68. doi: 10.1530/ror.0.0020055
93. Zhang D, Xiong H, Mennigen JA, Popesku JT, Marlatt VL, Martyniuk CJ, et al. Defining global neuroendocrine gene expression patterns associated with reproductive seasonality in fish. PloS One (2009) 4:e5816. doi: 10.1371/journal.pone.0005816
94. Mennigen JA, Lado WE, Zamora JM, Duarte-Guterman P, Langlois VS, Metcalfe CD, et al. Waterborne fluoxetine disrupts the reproductive axis in sexually mature male goldfish, carassius auratus. Aquat Toxicol (2010) 100:354–64. doi: 10.1016/j.aquatox.2010.08.016
95. Sorensen PW, Pinillos M, Scott AP. Sexually mature male goldfish release large quantities of androstenedione into the water where it functions as a pheromone. Gen Comp Endocrinol (2005) 140:164–75. doi: 10.1016/j.ygcen.2004.11.006
96. Mangiamele LA, Keeney ADT, D’Agostino EN, Thompson RR. Pheromone exposure influences preoptic arginine vasotocin gene expression and inhibits social approach behavior in response to rivals but not potential mates. Brain Behav Evol (2013) 81:194–202. doi: 10.1159/000350589
97. Sokołowska E, Kleszczyńska A, Nietrzeba M, Kulczykowska E. Annual changes in brain concentration of arginine vasotocin and isotocin correspond with phases of reproductive cycle in round goby, neogobius melanostomus. Chronobiol Int (2015) 32:917–24. doi: 10.3109/07420528.2015.1052142
99. Maruska KP, Mizobe MH, Tricas TC. Sex and seasonal co-variation of arginine vasotocin (AVT) and gonadotropin-releasing hormone (GnRH) neurons in the brain of the halfspotted goby. Comp Biochem Physiol Part A Mol Integr (2007) 147:129–44. doi: 10.1016/j.cbpa.2006.12.019
100. Kleszczyńska A, Sokołowska E, Kulczykowska E. Variation in brain arginine vasotocin (AVT) and isotocin (IT) levels with reproductive stage and social status in males of three-spined stickleback (Gasterosteus aculeatus). Gen Comp Endocrinol (2012) 175:290–6. doi: 10.1016/j.ygcen.2011.11.022
101. Kulczykowska E, Kleszczyńska A. Brain arginine vasotocin and isotocin in breeding female three-spined sticklebacks (Gasterosteus aculeatus): The presence of male and egg deposition. Gen Comp Endocrinol (2014) 204:8–12. doi: 10.1016/j.ygcen.2014.04.039
102. Ota Y, Ando H, Ban M, Ueda H, Urano A. Sexually different expression of neurohypophysial hormone genes in the preoptic nucleus of pre-spawning chum salmon. Zool Sci (1996) 13:593–601. doi: 10.2108/zsj.13.593
103. Hiraoka S, Ando H, Ban M, Ueda H, Urano A. Changes in expression of neurohypophysial hormone genes during spawning migration in chum salmon, oncorhynchus keta. J Mol Endocrinol (1997) 18:49–55. doi: 10.1677/jme.0.0180049
104. Makino K, Onuma TA, Kitahashi T, Ando H, Ban M, Urano A. Expression of hormone genes and osmoregulation in homing chum salmon: A minireview. Gen Comp Endocrinol (2007) 152:304–9. doi: 10.1016/j.ygcen.2007.01.010
105. Aubin-Horth N, Letcher BH, Hofmann HA. Gene-expression signatures of Atlantic salmon’s plastic life cycle. Gen Comp Endocrinol (2009) 163:278–84. doi: 10.1016/j.ygcen.2009.04.021
106. Guiry A, Flynn D, Hubert S, O’Keeffe AM, LeProvost O, White SL, et al. Testes and brain gene expression in precocious male and adult maturing Atlantic salmon (Salmo salar). BMC Genomics (2010) 11:211. doi: 10.1186/1471-2164-11-211
107. Ota Y, Ando H, Ueda H, Urano A. Differences in seasonal expression of neurohypophysial hormone genes in ordinary and precocious Male masu salmon. Gen Comp Endocrinol (1999) 116:40–8. doi: 10.1006/gcen.1999.7344
108. Maruska KP. Social regulation of reproduction in male cichlid fishes. Gen Comp Endocrinol (2014) 207:2–12. doi: 10.1016/j.ygcen.2014.04.038
109. Greenwood AK, Wark AR, Fernald RD, Hofmann HA. Expression of arginine vasotocin in distinct preoptic regions is associated with dominant and subordinate behaviour in an African cichlid fish. Proc R Soc B (2008) 275:2393–402. doi: 10.1098/rspb.2008.0622
110. Bass AH, Grober MS. Social and neural modulation of sexual plasticity in teleost fish. Brain Behav Evol (2001) 57:293–300. doi: 10.1159/000047247
111. Semsar K, Godwin J. Social influences on the arginine vasotocin system are independent of gonads in a sex-changing fish. J Neurosci (2003) 23:4386–93. doi: 10.1523/JNEUROSCI.23-10-04386.2003
112. Godwin J, Sawby R, Warner RR, Crews D, Grober MS. Hypothalamic arginine vasotocin mRNA abundance variation across sexes and with sex change in a coral reef fish. Brain Behav Evol (2000) 55:77–84. doi: 10.1159/000006643
113. Grober MS, Sunobe T. Serial adult sex change involves rapid and reversible changes in forebrain neurochemistry. Neuroreport (1996) 7:2945–9. doi: 10.1097/00001756-199611250-00029
114. Kulczykowska E, Kalamarz-Kubiak H, Nietrzeba M, Gozdowska M. Brain nonapeptide and gonadal steroid responses to deprivation of heterosexual contact in the black molly. Biol Open (2015) 4:69–78. doi: 10.1242/bio.20149597
115. Liu H, Lamm MS, Rutherford K, Black MA, Godwin JR, Gemmell NJ. Large-Scale transcriptome sequencing reveals novel expression patterns for key sex-related genes in a sex-changing fish. Biol Sex Differ (2015) 6:26. doi: 10.1186/s13293-015-0044-8
116. Black MP, Reavis RH, Grober MS. Socially induced sex change regulates forebrain isotocin in lythrypnus dalli. NeuroReport (2004) 15:185–9. doi: 10.1097/00001756-200401190-00036
117. Godwin J, Thompson R. Nonapeptides and social behavior in fishes. Horm Behav (2012) 61:230–8. doi: 10.1016/j.yhbeh.2011.12.016
118. Yokoi S, Okuyama T, Kamei Y, Naruse K, Taniguchi Y, Ansai S, et al. An essential role of the arginine vasotocin system in mate-guarding behaviors in triadic relationships of medaka fish (Oryzias latipes). PloS Genet (2015) 11:e1005009. doi: 10.1371/journal.pgen.1005009
119. Salek SJ, Sullivan CV, Godwin J. Arginine vasotocin effects on courtship behavior in male white perch (Morone americana). Behav Brain Res (2002) 133:177–83. doi: 10.1016/S0166-4328(02)00003-7
120. Santangelo N, Bass AH. Individual behavioral and neuronal phenotypes for arginine vasotocin mediated courtship and aggression in a territorial teleost. Brain Behav Evol (2010) 75:282–91. doi: 10.1159/000316867
121. Pouso P, Cabana Á, Goodson JL, Silva A. Preoptic area activation and vasotocin involvement in the reproductive behavior of a weakly pulse-type electric fish, brachyhypopomus gauderio. Front Integr Neurosci (2019) 13:37. doi: 10.3389/fnint.2019.00037
122. Perrone R, Silva A. Vasotocin increases dominance in the weakly electric fish brachyhypopomus gauderio. J Physiol-Paris (2016) 110:119–26. doi: 10.1016/j.jphysparis.2016.12.004
123. Carneiro LA, Oliveira RF, Canário AVM, Grober MS. The effect of arginine vasotocin on courtship behaviour in a blenniid fish with alternative reproductive tactics. Fish Physiol Biochem (2003) 28:241–3. doi: 10.1023/B:FISH.0000030542.31395.8a
124. DeAngelis R, Gogola J, Dodd L, Rhodes JS. Opposite effects of nonapeptide antagonists on paternal behavior in the teleost fish amphiprion ocellaris. Horm Behav (2017) 90:113–9. doi: 10.1016/j.yhbeh.2017.02.013
125. DeAngelis R, Dodd L, Rhodes J. Nonapeptides mediate trade-offs in parental care strategy. Horm Behav (2020) 121:104717. doi: 10.1016/j.yhbeh.2020.104717
126. O’Connell LA, Matthews BJ, Hofmann HA. Isotocin regulates paternal care in a monogamous cichlid fish. Horm Behav (2012) 61:725–33. doi: 10.1016/j.yhbeh.2012.03.009
127. Wacker DW, Ludwig M. Vasopressin, oxytocin, and social odor recognition. Horm Behav (2012) 61(3):259–65. doi: 10.1016/j.yhbeh.2011.08.014
128. Dunlap KD, Koukos HM, Chagnaud BP, Zakon HH, Bass AH. Vocal and electric fish: Revisiting a comparison of two teleost models in the neuroethology of social behavior. Front Neural Circuits (2021) 15:713105. doi: 10.3389/fncir.2021.713105
129. Somoza GM, Yu KL, Peter RE. Serotonin stimulates gonadotropin release in female and male goldfish, Carassius auratus l. Gen Comp Endocrinol (1988) 72:374–82. doi: 10.1016/0016-6480(88)90159-1
130. Saito D, Hasegawa Y, Urano A. Gonadotropin-releasing hormones modulate electrical activity of vasotocin and isotocin neurons in the brain of rainbow trout. Neurosci Lett (2003) 351:107–10. doi: 10.1016/j.neulet.2003.08.017
131. Kanda S, Akazome Y, Mitani Y, Okubo K, Oka Y. Neuroanatomical evidence that kisspeptin directly regulates isotocin and vasotocin neurons. PloS One (2013) 8:e62776. doi: 10.1371/journal.pone.0062776
132. Zmora N, Stubblefield JD, Wong T-T, Levavi-Sivan B, Millar RP, Zohar Y. Kisspeptin antagonists reveal kisspeptin 1 and kisspeptin 2 differential regulation of reproduction in the teleost, morone saxatilis. Biol Reprod (2015) 93:1–12. doi: 10.1095/biolreprod.115.131870
133. Trudeau VL, Somoza GM. Multimodal hypothalamo-hypophysial communication in the vertebrates. Gen Comp Endocrinol (2020) 293:113475. doi: 10.1016/j.ygcen.2020.113475
134. Chaube R, Singh RK, Joy KP. Estrogen regulation of brain vasotocin secretion in the catfish heteropneustes fossilis: An interaction with catecholaminergic system. Gen Comp Endocrinol (2012) 175:206–13. doi: 10.1016/j.ygcen.2011.11.012
135. Yamashita J, Kawabata Y, Okubo K. Expression of isotocin is male-specifically up-regulated by gonadal androgen in the medaka brain. J Neuroendocrinol (2017) 29:e12545. doi: 10.1111/jne.12545
136. Da Fonte DF, Xing L, Mikwar M, Trudeau VL. Secretoneurin-a inhibits aromatase b (cyp19a1b) expression in female goldfish (Carassius auratus) radial glial cells. Gen Comp Endocrinol (2018) 257:106–12. doi: 10.1016/j.ygcen.2017.04.014
137. Popesku JT, Martyniuk CJ, Mennigen J, Xiong H, Zhang D, Xia X, et al. The goldfish (Carassius auratus) as a model for neuroendocrine signaling. Mol Cell Endocrinol (2008) 293:43–56. doi: 10.1016/j.mce.2008.06.017
138. Singh U, Kumar S, Singru PS. Interaction between dopamine- and isotocin-containing neurones in the preoptic area of the catfish, clarias batrachus: role in the regulation of luteinising hormone cells. J Neuroendocrinol (2012) 24:1398–411. doi: 10.1111/j.1365-2826.2012.02350.x
139. Robinson G, Evans JJ, Catt KJ. Oxytocin stimulates LH production by the anterior pituitary gland of the rat. J Endocrinol (1992) 132:277–83. doi: 10.1677/joe.0.1320277
140. Evans JJ, Robinson G, Catt KJ. Luteinizing hormone response to oxytocin is steroid-dependent. Neuroendocrinology (1992) 55:538–43. doi: 10.1159/000126167
141. Rodríguez M, Specker JL. In vitro effects of arginine vasotocin on testosterone production by testes of rainbow trout (Oncorhynchus mykiss). Gen Comp Endocrinol (1991) 83:249–57. doi: 10.1016/0016-6480(91)90028-5
142. Singh V, Joy KP. Relative in vitro seasonal effects of vasotocin and isotocin on ovarian steroid hormone levels in the catfish heteropneustes fossilis. Gen Comp Endocrinol (2009) 162:257–64. doi: 10.1016/j.ygcen.2009.03.024
143. Mishra A, Joy KP. Effects of gonadotrophin in vivo and 2-hydroxyoestradiol-17β in vitro on follicular steroid hormone profile associated with oocyte maturation in the catfish heteropneustes fossilis. J Endocrinol (2006) 189:341–53. doi: 10.1677/joe.1.06686
145. Singh V, Joy KP. Vasotocin induces final oocyte maturation and ovulation through the production of a maturation-inducing steroid in the catfish heteropneustes fossilis. Gen Comp Endocrinol (2011) 174(1):15–21. doi: 10.1016/j.ygcen.2011.07.009
146. Sirotkin AV, Gerasimova GG, Golubev AK, Dmitriev VB. The effect of arginine-vasotocin on the production of steroid hormones by mouse, cow, and chicken ovarian tissues. vitro. BioMed Sci (1990) 1:308–10.
147. Lister AL, van der Kraak G. An investigation into the role of prostaglandins in zebrafish oocyte maturation and ovulation. Gen Comp Endocrinol (2008) 159:46–57. doi: 10.1016/j.ygcen.2008.07.017
148. Bradley JA, Goetz FW. The inhibitory effects of indomethacin, nordihydroguaiaretic acid, and pyrrolidinedithiocarbamate on ovulation and prostaglandin synthesis in yellow perch (Perca flavescens) follicle incubates. Prostaglandins (1994) 48:11–20. doi: 10.1016/0090-6980(94)90092-2
149. Patiño R, Yoshizaki G, Bolamba D, Thomas P. Role of arachidonic acid and protein kinase c during maturation-inducing hormone-dependent meiotic resumption and ovulation in ovarian follicles of Atlantic croaker. Biol Reprod (2003) 68:516–23. doi: 10.1095/biolreprod.102.009662
150. Joy KP, Singh V. Functional interactions between vasotocin and prostaglandins during final oocyte maturation and ovulation in the catfish heteropneustes fossilis. Gen Comp Endocrinol (2013) 186:126–35. doi: 10.1016/j.ygcen.2013.02.043
151. Cerdà J. Molecular pathways during marine fish egg hydration: the role of aquaporins. J Fish Biol (2009) 75:2175–96. doi: 10.1111/j.1095-8649.2009.02397.x
152. Singh V, Joy KP. An involvement of vasotocin in oocyte hydration in the catfish heteropneustes fossilis: A comparison with effects of isotocin and hCG. Gen Comp Endocrinol (2010) 166:504–12. doi: 10.1016/j.ygcen.2010.02.020
153. Chaube R, Chauvigné F, Tingaud-Sequeira A, Joy KP, Acharjee A, Singh V, et al. Molecular and functional characterization of catfish (Heteropneustes fossilis) aquaporin-1b: changes in expression during ovarian development and hormone-induced follicular maturation. Gen Comp Endocrinol (2011) 170:162–71. doi: 10.1016/j.ygcen.2010.10.002
154. Acharjee A, Chaube R, Joy KP, Cerda J. Hormonal regulation of aquaporin-1ab in heteropneustes fossilis oocytes in vitro. Indian J Sci Technol (2011) 4: 165–166.
155. Wilhelmi AE, GE P, Sawyer WH. Initiation of the spawning reflex response in fundulus by the administration of fish and mammalian neurohypophysial preparations and synthetic oxytocin. Endocrinology (1955) 57:243–52. doi: 10.1210/endo-57-2-243
156. Viveiros ATM, Jatzkowski A, Komen J. Effects of oxytocin on semen release response in African catfish (Clarias gariepinus). Theriogenology (2003) 59:1905–17. doi: 10.1016/s0093-691x(02)01290-6
157. Wisdom KS, Bhat IA, Pathan MA, CT I, Kumar P, Babu PG, et al. Teleost nonapeptides, isotocin and vasotocin administration released the milt by abdominal massage in Male catfish, clarias magur. Front Endocrinol (2022) 13:899463. doi: 10.3389/fendo.2022.899463
158. Venkatesh B, Tan CH, Lam TJ. Prostaglandins and teleost neurohypophyseal hormones induce premature parturition in the guppy, poecilia reticulata. Gen Comp Endocrinol (1992) 87:28–32. doi: 10.1016/0016-6480(92)90146-b
159. Xiong S, Tian J, Ge S, Li Z, Long Z, Guo W, et al. The microRNA-200 cluster on chromosome 23 is required for oocyte maturation and ovulation in zebrafish. Biol Reprod (2020) 103:769–78. doi: 10.1093/biolre/ioaa125
160. Peter RE. The preoptic nucleus in fishes: A comparative discussion of function-activity relationships. Amer Zool (1977) . 17:775–85. doi: 10.1093/icb/17.4.775
161. Zohar Y. Fish reproductive biology - reflecting on five decades of fundamental and translational research. Gen Comp Endocrinol (2021) 300:113544. doi: 10.1016/j.ygcen.2020.113544
162. Arcand-Hoy LD, Benson WH. Fish reproduction an ecologically relevant indicator of fish endocrine disruption. Env Toxicol Chem (1998) 17:49–57. doi: 10.1002/etc.5620170108
163. Ram RN, Joy KP. Mercurial induced changes in the hypothalamo-neurohypophysical complex in relation to reproduction in the teleostean fish, channa punctatus (Bloch). Bull Environ Contam Toxicol (1988) 41:329–36. doi: 10.1007/BF01688875
164. Mennigen JA, Zamora JM, Chang JP, Trudeau VL. Endocrine disrupting effects of waterborne fluoxetine exposure on the reproductive axis of female goldfish, carassius auratus. Comp Biochem Physiol Part-C: Toxicol Pharmacol (2017) 202:70–8. doi: 10.1016/j.cbpc.2017.08.003
165. Parolini M, Ghilardi A, De Felice B, Del Giacco L. Environmental concentration of fluoxetine disturbs larvae behavior and increases the defense response at molecular level in zebrafish (Danio rerio). Environ Sci pollut Res Int (2019) 26:34943–52. doi: 10.1007/s11356-019-06619-4
166. Wong RY, Oxendine SE, Godwin J. Behavioral and neurogenomic transcriptome changes in wild-derived zebrafish with fluoxetine treatment. BMC Genomics (2013) 14:348. doi: 10.1186/1471-2164-14-348
167. Semsar K, Perreault HAN, Godwin J. Fluoxetine-treated male wrasses exhibit low AVT expression. Brain Res (2004) 1029:141–7. doi: 10.1016/j.brainres.2004.09.030
168. Cantor JM, Binik YM, Pfaus JG. Chronic fluoxetine inhibits sexual behavior in the male rat: reversal with oxytocin. Psychopharmacol (Berl) (1999) 144:355–62. doi: 10.1007/s002130051018
169. de Jong TR, Veening JG, Olivier B, Waldinger MD. Oxytocin involvement in SSRI-induced delayed ejaculation: a review of animal studies. J Sex Med (2007) 4:14–28. doi: 10.1111/j.1743-6109.2006.00394.x
171. Mennigen JA, Stroud P, Zamora JM, Moon TW, Trudeau VL. Pharmaceuticals as neuroendocrine disruptors: lessons learned from fish on Prozac. J Toxicol Environ Health B Crit Rev (2011) 14:387–412. doi: 10.1080/10937404.2011.578559
172. Wang Z, Mao K, Du W, Cai M, Zhang Z, Li X. Diluted concentrations of methamphetamine in surface water induce behavior disorder, transgenerational toxicity, and ecosystem-level consequences of fish. Water Res (2020) 184:116164. doi: 10.1016/j.watres.2020.116164
173. León-Olea M, Martyniuk CJ, Orlando EF, Ottinger MA, Rosenfeld C, Wolstenholme J, et al. Current concepts in neuroendocrine disruption. Gen Comp Endocrinol (2014) 203:158–73. doi: 10.1016/j.ygcen.2014.02.005
174. Salahinejad A, Naderi M, Attaran A, Meuthen D, Niyogi S, Chivers DP. Effects of chronic exposure to bisphenol-s on social behaviors in adult zebrafish: Disruption of the neuropeptide signaling pathways in the brain. Environ pollut (2020) 262:113992. doi: 10.1016/j.envpol.2020.113992
175. Salahinejad A, Attaran A, Meuthen D, Rachamalla M, Chivers DP, Niyogi S. Maternal exposure to bisphenol s induces neuropeptide signaling dysfunction and oxidative stress in the brain, and abnormal social behaviors in zebrafish (Danio rerio) offspring. Sci Total Environ (2022) 830:154794. doi: 10.1016/j.scitotenv.2022.154794
176. Naderi M, Puar P, JavadiEsfahani R, Kwong RWM. Early developmental exposure to bisphenol a and bisphenol s disrupts socio-cognitive function, isotocin equilibrium, and excitation-inhibition balance in developing zebrafish. Neurotoxicology (2022) 88:144–54. doi: 10.1016/j.neuro.2021.11.009
177. Naderi F, Míguez JM, Soengas JL, López-Patiño MA. SIRT1 mediates the effect of stress on hypothalamic clock genes and food intake regulators in rainbow trout, oncorhynchus mykiss. Comp Biochem Physiol A Mol Integr Physiol (2019) 235:102–11. doi: 10.1016/j.cbpa.2019.05.021
178. Kalamarz-Kubiak H. Endocrine-disrupting compounds in fish physiology, with emphasis on their effects on the arginine Vasotocin/Isotocin system. Endocr Metab Immune Disord Drug Targets (2021) 22(7):738–47. doi: 10.2174/1871530321666210202150947
179. Patisaul HB. Endocrine disruption of vasopressin systems and related behaviors. Front Endocrinol (Lausanne) (2017) 8:134. doi: 10.3389/fendo.2017.00134
Keywords: endocrine system, hormone, hypothalamic–pituitary–gonadal axis, isotocin, neuromodulator, courtship behaviour, paracrine, vasotocin
Citation: Mennigen JA, Ramachandran D, Shaw K, Chaube R, Joy KP and Trudeau VL (2022) Reproductive roles of the vasopressin/oxytocin neuropeptide family in teleost fishes. Front. Endocrinol. 13:1005863. doi: 10.3389/fendo.2022.1005863
Received: 28 July 2022; Accepted: 23 September 2022;
Published: 13 October 2022.
Edited by:
Shan He, Huazhong Agricultural University, ChinaReviewed by:
Christian W. Gruber, Medical University of Vienna, AustriaPaula Pouso, University of the Republic, Uruguay
Copyright © 2022 Mennigen, Ramachandran, Shaw, Chaube, Joy and Trudeau. This is an open-access article distributed under the terms of the Creative Commons Attribution License (CC BY). The use, distribution or reproduction in other forums is permitted, provided the original author(s) and the copyright owner(s) are credited and that the original publication in this journal is cited, in accordance with accepted academic practice. No use, distribution or reproduction is permitted which does not comply with these terms.
*Correspondence: Jan A. Mennigen, amFuLm1lbm5pZ2VuQHVvdHRhd2EuY2E=