- 1Victor Babes National Institute of Pathology, Bucharest, Romania
- 2Carol Davila University of Medicine and Pharmacy, Bucharest, Romania
- 3Brigham and Women’s Hospital and Harvard Medical School, Boston, MA, United States
- 4Viron Molecular Medicine Institute, Boston, MA, United States
Immune checkpoint inhibitors, namely anti-CTLA-4, anti-PD-1 and anti-PD-L1 monoclonal antibodies, have emerged in the last decade as a novel form of cancer treatment, promoting increased survival in patients. As they tamper with the immune response in order to destroy malignant cells, a new type of adverse reactions has emerged, known as immune-related adverse events (irAEs), which frequently target the endocrine system, especially the thyroid and hypophysis. Thyroid irAEs include hyperthyroidism, thyrotoxicosis, hypothyroidism and a possibly life-threatening condition known as the “thyroid storm”. Early prediction of occurrence and detection of the thyroid irAEs should be a priority for the clinician, in order to avoid critical situations. Moreover, they are recently considered both a prognostic marker and a means of overseeing treatment response, since they indicate an efficient activation of the immune system. Therefore, a multidisciplinary approach including both oncologists and endocrinologists is recommended when immune checkpoint inhibitors are used in the clinic.
Introduction
The influence of the immune system over tumor development is of high importance, since the immune system can recognize and destroy tumor cells. This is not the case for every type of tumor: different mechanisms are involved in a process called immune escape, helping malignant cells to avoid being killed (1). Our immune system could also respond against healthy tissues, leading to autoimmunity, which is normally prevented through activation of inhibitory pathways known as immune checkpoints. When these pathways are not properly regulated, immune escape of cancer cells may occur (2).
Immune checkpoint inhibitors (ICIs) have emerged as a new class of drugs used for in treatment of various types of malignancies, since their first U.S. Food and Drugs Administration (FDA) approval, in 2011 of ipilimumab (3, 4). Anti-cytotoxic T-lymphocyte-associated antigen 4 (CTLA-4) monoclonal antibodies (e.g. ipilimumab), anti-programmed death 1 (PD-1) monoclonal antibodies (e.g. pembrolizumab, nivolumab, cemiplimab) and anti-PD-1 ligand (PD-L1) monoclonal antibodies (e.g. avelumab, atezolizumab, durvalumab) are the types of ICIs used at the moment (5). They have improved overall survival for patients suffering from neoplasms, such as melanoma, non-small cell lung cancer (NSCLC) and renal cancer. However, ICIs are also the cause of specific adverse reactions known as immune-related adverse events (irAEs) (6). IrAEs are usually phenotypically similar to autoimmune conditions, even though it is not certain that the underlying mechanisms are similar. Some predisposing factors that might lead to irAE are genetic and environmental, or a combination of both (7).
Common irAEs associated with ICI therapy include thyroid dysfunctions, both hypothyroidism and hyperthyroidism or thyrotoxicosis, especially while administering anti-PD-1 or anti-PD-L1 antibodies. Therefore, this form of cancer treatment challenges both oncologists and endocrinologists and it should involve a multi-disciplinary approach (8).
Immune checkpoint inhibitors: Mechanism of action
The therapy involving modulation of/tampering with the immune system in order to treat malignant tumors is called immunotherapy. It involves cancer vaccines, an oncolytic virus, a bi-specific T-cell and ICIs (9). ICIs have emerged in the last decade, changing the outlook on treatment for at least 17 different types of cancer (3).
Immune checkpoints are responsible for self-tolerance, while inhibitory checkpoint molecules such as CTLA-4, PD-1 and its ligands PD-L1 and PD-L2, regulate this process. Inflammation is said to upregulate these molecules through interferon gamma. Antibodies targeting CTLA-4 or PD-1 and its ligands ensure the enhancement of immune reactions against malignant cells, while also promoting autoimmunity (3, 6, 9).
CTLA-4 (also known as CD152) is a glycoprotein expressed by CD4+ and CD8+ T-cells (10), acting as a negative regulator for T-cell activation (11). This inhibitory effect is based on its similarities to CD28, which enable binding of CTLA-4 to B7 glycoproteins (B7.1 or CD80 and B7.2 or CD86) with 20-fold higher affinity than CD28 (11, 12). This results in limitation of activated T-cell proliferation (8), promoting the immune escape of cancer cells (see Figure 1).
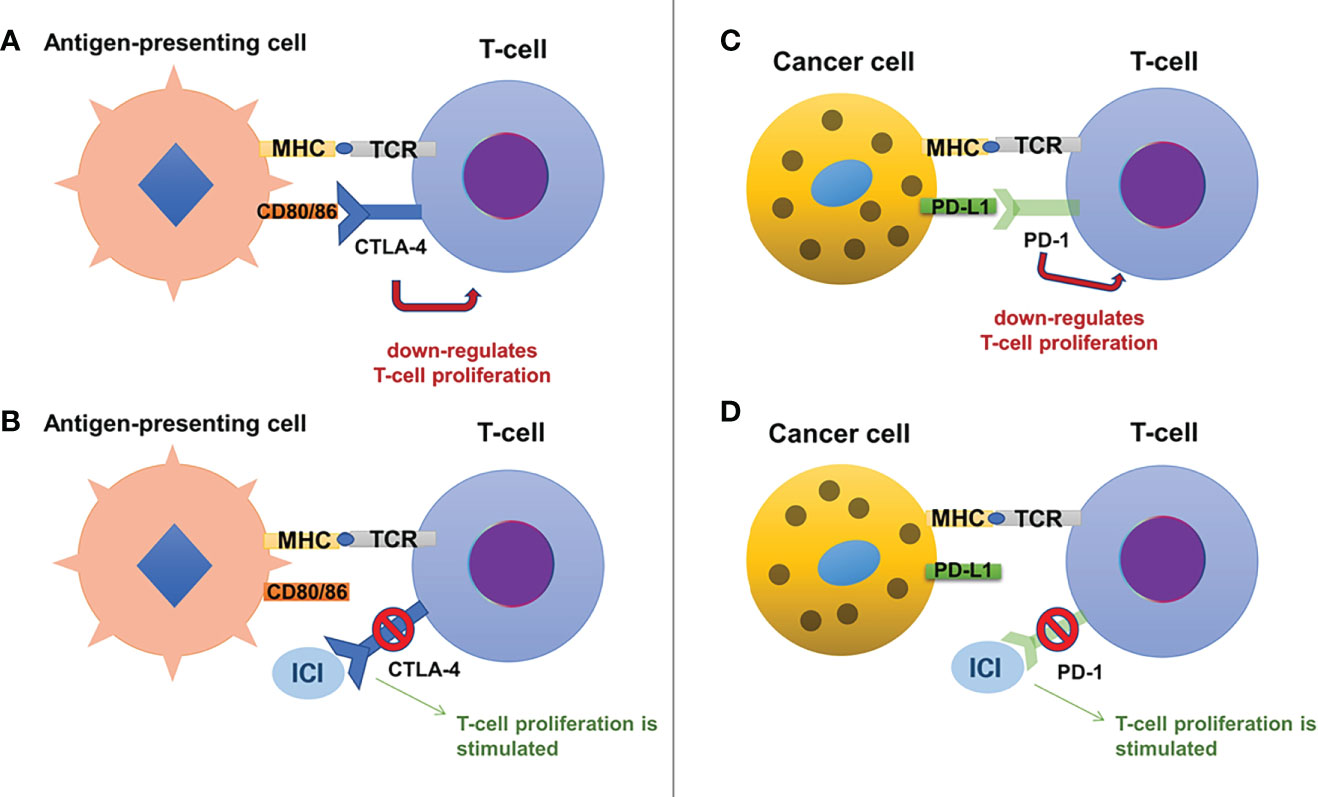
Figure 1 The mechanisms of action for immune checkpoint inhibitors include the CTLA-4 pathway (on the left, panels (A) and (B)) and the PD-1/PD-L1 pathway (on the right, panels (C) and (D)). Normally, CTLA-4 is present on the surface of T-cells and by binding to its ligands, CD80 and CD86 (expressed on antigen-presenting cells), it exerts an inhibitory effect on the T-cell proliferation (A). Anti-CTLA-4 antibodies (a variety of ICIs) bind to CTLA-4 and block its inhibitory effects, stimulating T-cell proliferation (B). Similarly, T-cells express PD-1, which binds to its ligand, PD-L1, present on cancer cells, suppressing T-cell proliferation (C). Anti-PD-1 antibodies bind to PD-1 blocking its inhibitory effects and stimulating T-cell proliferation (D).
PD-1 (also known as CD279) is mainly expressed on the activated CD8+ T-cells (13), although it is also present on the surface of macrophages, dendritic cells, B-cells, and while it is also similar to CD28, it binds to its specific ligands: PD-L1 (expressed on immune cells, hepatocytes, pancreatic islet cells, endothelial cells, myocytes, thyroid cells and many other cells, including various tumor cells) and PD-L2 (only expressed on macrophages and dendritic cells) (12). The inhibitory effect on T-cell activity is obtained through binding of PD-1 to its ligands, which results in a decrease of glucose uptake and gluconeogenesis of T-cells and apoptosis, while also interrupting the co-stimulatory pathway of CD28-CD80/86 (11, 12). Only regulatory T-cells will have an increased survival, as they have the ability to suppress cytotoxic CD8+ T-cell proliferation, in favor of immune escape of cancer cells (11, 14). The PD-1 ligands seem to be present mostly in inflammatory settings as they are strongly regulated by interferon gamma (15): this could be a reason why chronic inflammation which surrounds tumors limits the destruction of cancer cells (16). Also, the high expression of PD-L1 which promotes immune escape was linked with greater tumor aggressiveness (17).
Considering the fact that CTLA-4 and PD-1 regulate different stages of the immune response (CTLA-4 regulates the early stages of T-cell activation, while PD-1 is expressed after the T-cells are activated) and that they exert their actions at different sites (draining lymph nodes for CTLA-1 and tumor microenvironment for PD-1 and its ligands), it is understandable that their effects and adverse reactions are different (18–20). PD-1 inhibitors seem to have a more specific effect, associating less severe adverse reactions (20–23).
Both CTLA-4 and PD-1 are also involved in development of autoimmune disorders. CTLA-4 seems to have a role in the development of Graves’ disease (24) and immune dysregulation syndrome, through autosomal dominant mutations (25). PD-L1 expression is increased in Hashimoto’s thyroiditis (26), while PD-1 blockade might stimulate B-cells to produce thyroid autoantibodies and thyroid dysfunction, especially in patients with higher baseline levels of these thyroid antibodies prior to the treatment (1, 27, 28).
The importance of prognostic markers and routine laboratory investigation before deciding to use a specific cancer treatment option has been previously established (29). Potential prognostic markers with either proven efficacy or in-line for future studies are: tumor mutational burden, PD-L1 expression, fraction of copy number alteration, human leukocyte antigen (HLA)-I evolutionary divergence, the loss of heterozygosity status in HLA-I25, microsatellite instability, body mass index, sex, blood neutrophil-to-lymphocyte ratio (NLR), tumor stage, immunotherapy drug agent, age, cancer type, whether the patient received chemotherapy before immunotherapy, blood levels of albumin, platelets and hemoglobin (the last three providing information about the presence of tumor-promoting inflammation), other molecular features of the tumor immune microenvironment (infiltration levels of myeloid cells or lymphocytes), composition of the microbiome, T-cell receptor diversity, mutations associated with resistance to ICIs and the expression of ADORA1 or p62 (30–47).
Currently, the main FDA-approved prognostic marker for ICI response is the tumor mutation burden, which measures the number of mutations present in the tumor, proportional to the number of neo-antigens and to the probability of triggering a T-cell response. It performs well on its own, but the efficacy can be increased by associating the detection of PD-L1 expression (48, 49) or NLR (33). Regarding PD-L1 expression, it has been hypothesized that the presence of tumor infiltrating lymphocytes might increase its accuracy in predicting the ICI treatment response (35). NLR is an accessible marker of systemic inflammation, negatively correlated to cancer prognosis and response to immunotherapy (32).
FDA-approved immune checkpoint inhibitors
The first ICI approved by the FDA for treating metastatic melanoma (2011) was ipilimumab, an anti-CTLA-4 monoclonal antibody, and it was followed by the FDA-approval of three anti-PD-1 (pembrolizumab, nivolumab, cemiplimab) and three anti-PD-L1 monoclonal antibodies (atezolizumab, durvalumab, avelumab) (5). Along with ipilimumab, another anti-CTLA-4 antibody known as tremelimumab has entered clinical trials, but its antineoplastic activity was low while it was administered as a single drug, and it has also shown substantial side effects (the incidence of thyroid disorders is 0-5.2%). However, its association with durvalumab for treating mesothelioma, melanoma, NSCLC, gastroesophageal or colorectal tumors could be promising, even though some studies have not shown a substantial benefit added by tremelimumab compared to durvalumab alone (50–52). A phase 3 clinical trial (CheckMate 037) has shown that anti-PD-1 antibodies have both higher efficacy and a superior safety profile compared to ipilimumab (53). Another association of ICIs considered to be successful is the combination of ipilimumab with nivolumab, which has shown 57% intracranial central nervous system response for patients suffering from melanoma brain metastases (54, 55). ICIs can also be associated with chemotherapy agents to treat certain types of cancer: in 2019, FDA has approved the combination of atezolizumab and paclitaxel for treating patients with advanced metastatic triple negative breast cancer expressing PD-L1, without previous systemic treatment for metastatic disease (56, 57). Also, radiation therapy might be useful alongside ICI treatment, as it can sensitize melanoma brain metastases to ICIs (55, 58).
While most of the studies about ICIs involved solid tumors, their promising effects have paved the way for treating hematological malignancies as well: nivolumab and pembrolizumab were approved by the FDA in 2016 and 2017 for the treatment of the relapsed/refractory Hodgkin’s lymphoma (5, 59, 60).
The anti-CTLA-4, anti-PD-1 and anti-PD-L1 antibodies belong to different categories: ipilimumab, atezolizumab, durvalumab and avelumab belong to the IgG1 subclass, activating the classical complement pathway and initiating antibody-dependent cell-mediated cytotoxicity (ADCC), while pembrolizumab, nivolumab and cemiplimab are part of the IgG4 subclass, which is not able to activate the classical complement pathway and has a lower potency in inducing ADCC (10).
Ipilimumab (Yervoy®) is a CTLA-4 inhibitor belonging to the IgG1 subclass, approved by the FDA in March 2011 (4, 9). It is used for the treatment of melanoma, renal cell carcinoma and colorectal cancer (13), while also being responsible for development of irAE, usually after 9 weeks of therapy (hypophysitis, hypothyroidism, hyperthyroidism are the most frequent) (9, 61, 62).
Pembrolizumab (Keytruda®) is a PD-1 inhibitor belonging to the IgG4 subclass, approved by the FDA in September 2014 (9, 63). It is used for the treatment of melanoma, small-cell lung cancer (SCLC), NSCLC, Hodgkin’s lymphoma, squamous cell carcinoma of the head and neck, urothelial carcinoma, gastroesophageal and colorectal cancer, primary mediastinal large B cell lymphoma, hepatocellular carcinoma, Merkel cell carcinoma, cervical and renal cell cancer (13). The most frequent irAEs induced by pembrolizumab are hypothyroidism and hyperthyroidism (64), which have a particular immune phenotype demonstrated by studies: elevation of CD56+CD16+ natural killer (NK) cells along with decrease of CD56brCD16- NK cells and CD14+HLA-DRlo/neg monocytes. PD-1 is not expressed on T-cells from pembrolizumab-induced thyroiditis, which supports a T-cell mediated mechanism, rather than a B-cell mediated one (65).
Nivolumab (Opdivo®) is a PD-1 inhibitor belonging to the IgG4 subclass, approved by the FDA in 2015 as a second-line treatment for NSCLC (grades IIIB and IV) (66, 67). Currently, it is used for the treatment of melanoma, SCLC, NSCLC, Hodgkin’s lymphoma, squamous cell carcinoma of the head and neck, urothelial carcinoma, microsatellite instability-high or mismatch repair deficient colorectal cancer, hepatocellular and renal cell carcinoma (10, 52, 68). The combination of nivolumab and ipilimumab is the most frequent cause of hypothyroidism (CheckMate 069 trial has shown an incidence of 17%) (9, 69, 70). Nivolumab-induced thyrotoxicosis shows similarities to autoimmune thyroiditis (predominance of CD8+ T-cells), while also showing granulomas, destruction of thyroidal follicles and chronic thyroid lymphocytic inflammation, which are not present in autoimmune thyroiditis (71).
Cemiplimab (Libtayo®) is a PD-1 inhibitor belonging to the IgG4 subclass, approved by the FDA in September 2018, for the treatment of metastatic or locally advanced cutaneous squamous cell carcinoma not amenable to surgery or radiation (72, 73). Clinical studies are conducted for evaluating its efficacy in NSCLC, renal, ovarian and uterine carcinomas (74). It is responsible for the following irAEs: hypothyroidism (a research study has found an 8% incidence of hypothyroidism in a cohort of 59 patients treated with cemiplimab) (75), hyperthyroidism, type 1 diabetes mellitus (T1 DM), adrenal insufficiency and hypophysitis (76).
Atezolizumab (Tecentriq®) is a PD-L1 inhibitor belonging to the IgG1 subclass, approved by the FDA in 2016 for the treatment of urothelial carcinoma (77). It has also shown efficacy in SCLC, NSCLC, triple-negative breast cancer and metastatic squamous cell carcinoma of the head and neck (13, 78–81). It can induce hypothyroidism as irAE (64).
Durvalumab (Imfinzi®) is a PD-L1 inhibitor belonging to the IgG1 subclass, approved by the FDA in 2017 for the treatment of urothelial carcinoma and NSCLC (13, 82), while inducing hypothyroidism and hyperthyroidism as irAEs (64).
Avelumab (Bavencio®) is a PD-L1 inhibitor from the IgG1 subclass, approved by the FDA in 2017 for urothelial carcinoma, Merkel cell carcinoma and renal cell carcinoma (13, 83), while inducing hypothyroidism as irAE (64).
The information about the ICIs described above is synthetized in Table 1.
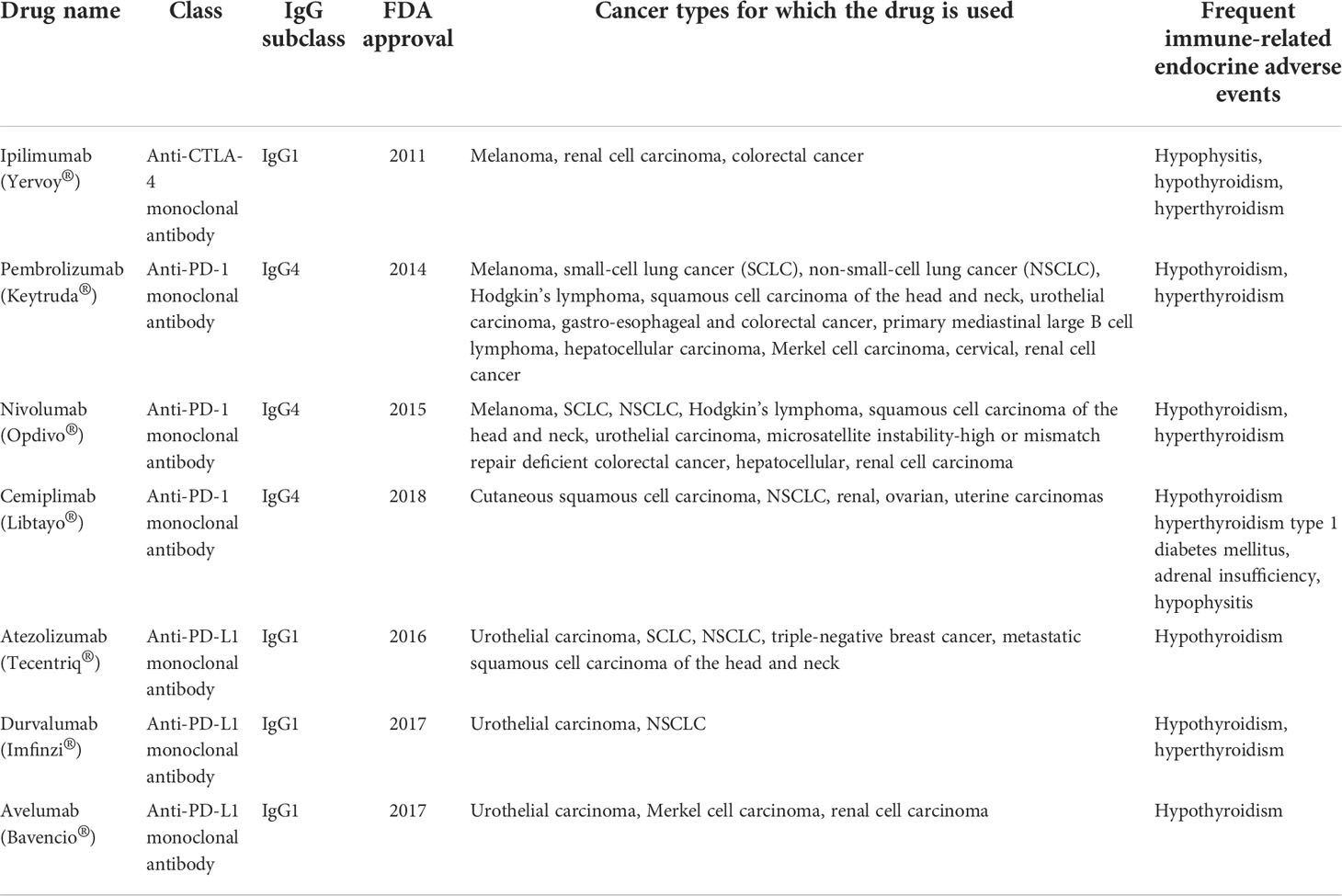
Table 1 Immune checkpoint inhibitors, cancer types for which they are used and the most frequently induced immune-related endocrine adverse events.
Immune checkpoint inhibitors: Immune-related endocrine adverse events
The immunologic tolerance can be altered after the administration of ICIs, therefore the risk of targeting self-antigens is high (84). A new category of adverse events has emerged as a consequence of the augmented immune response - they are known as immune-related adverse events (6) and they are present in the majority of the patients treated with anti-CTLA-4 and anti-PD-1 antibodies (a clinical trial has identified 86% occurrence of irAEs after ipilimumab administration, 82% after nivolumab and 96% after combination therapy) (70, 85), the most frequent being the thyroid dysfunctions (86).
Common Terminology Criteria for Adverse Events (CTCAE) are used for grading the irAEs induced by ICIs. There are 5 grades of severity, grade 1 representing mild toxicity and grade 5 representing death caused by the adverse reaction (see Table 2) (87). CTLA-4 inhibitors are responsible for causing grade 3 or 4 irAEs in >34% of the patients, while PD-1 inhibitors induce grade 3 or 4 irAEs in 15% of the patients. ICIs combinations are the most frequent elicitors of severe irAES (58-68%) (70, 88–91). Nivolumab and pembrolizumab are considered to be more easily tolerated than ipilimumab in terms of irAE severity (6, 70, 92).
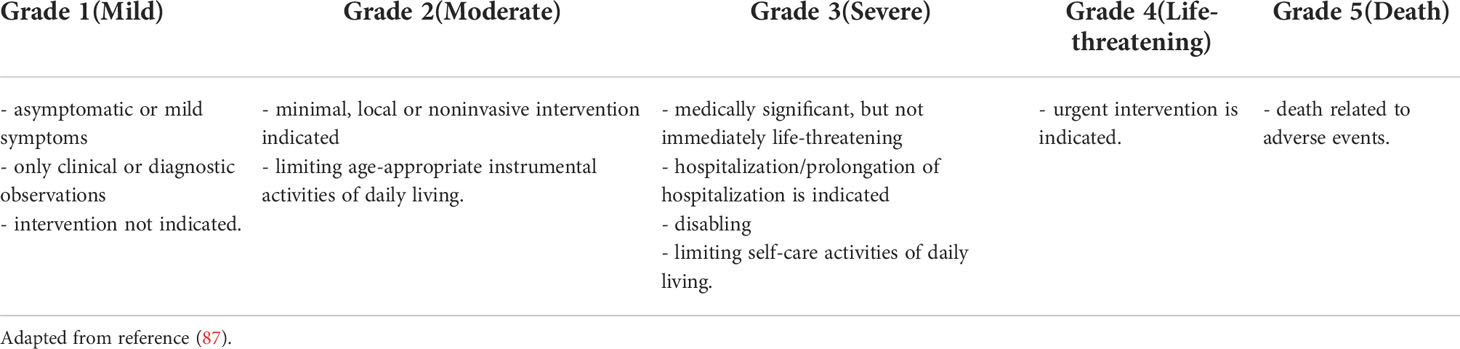
Table 2 Grading guidelines proposed by Common Terminology Criteria for Adverse Events (CTCAE) (applicable for immune-related adverse events induced by immune checkpoint inhibitors).
IrAEs can involve the following sites: skin, gastrointestinal tract, liver, endocrine system, cardiovascular system, lungs, kidneys, pancreas, bone marrow, musculoskeletal system, nervous system, ocular system (see Figure 2). The endocrine related adverse events are the most frequent, as they are present in up to 40% of the patients treated with different ICIs – in descending order, the affected organs are: thyroid, hypophysis (both of these having a rich vascularization, ensuring contact with activated T-lymphocytes), adrenals, beta cells of the pancreatic islets (3, 68, 93–96) and parathyroid glands. Generalized lipodystrophy, autoimmune polyglandular syndrome (97, 98), hypercalcemia (99), low testosterone levels (in the absence of hypophysitis) and a single case of “suspicious” adrenocorticotropic hormone (ACTH)-independent cortisol secretion (in the absence of exogenous cortisol administration) after ICIs’ administration, have also been described (76, 100).
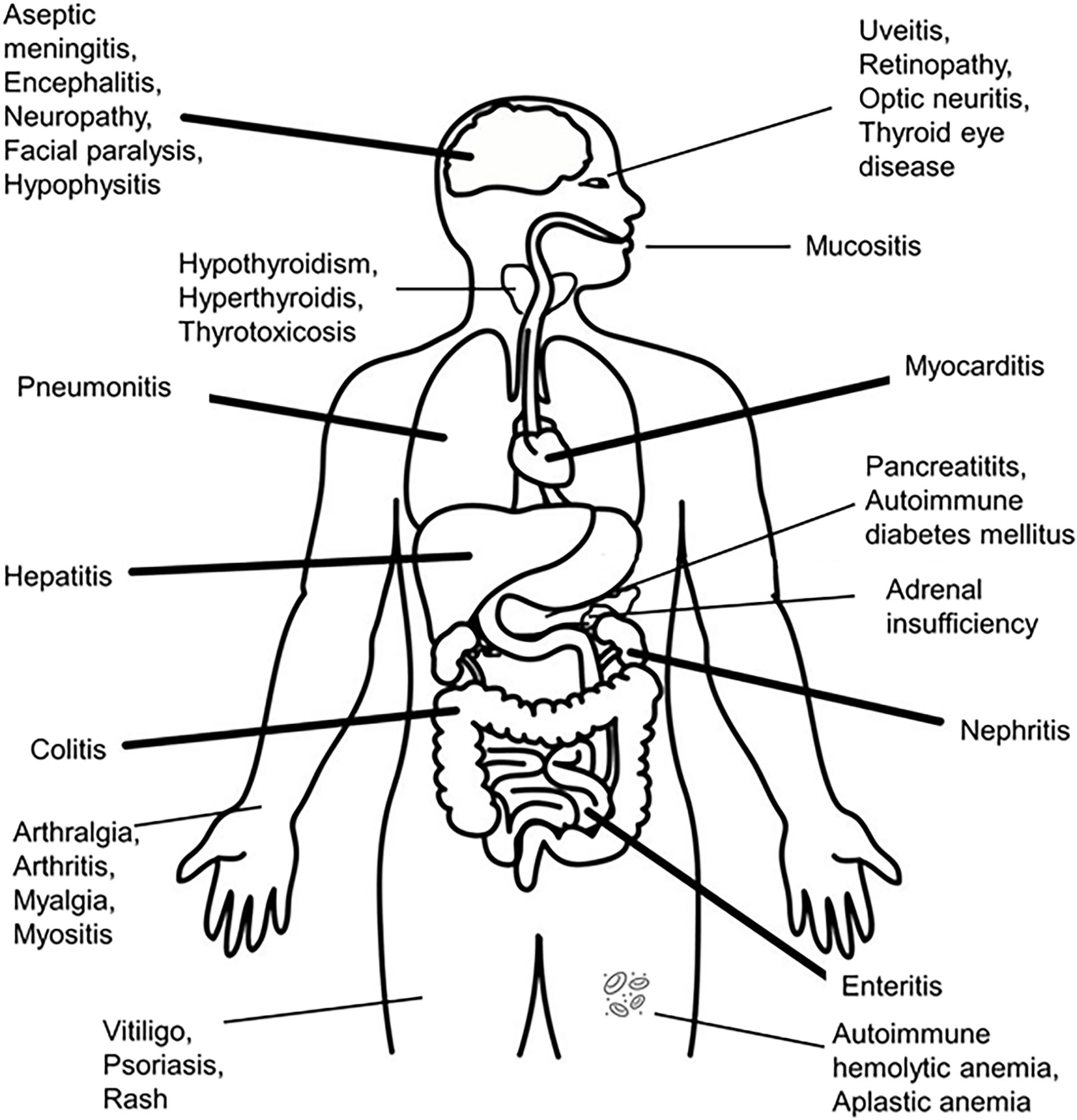
Figure 2 Organs suffering from immune-related adverse events after administration of immune checkpoint inhibitors.
Although the pathogenesis is not clearly elucidated, there are many mechanisms that have been incriminated in irAEs development: genetic susceptibility which triggers autoimmunity, cross-reactivity of neoantigens released from tumoral and normal cells, or production of cytokines (101). In addition, different ICIs might involve different mechanisms and sites, seeing that the anti-CTLA-4 antibodies target the hypophysis more frequently, while the anti-PD-1 antibodies are usually linked to thyroid dysfunctions (9): this is also linked to the presence of CTLA-4 expressed in the pituitary gland [mostly on cells secreting prolactin or thyroid stimulating hormone (TSH)] and the expression of PD-1 and PD-L1 in the thyroid (68, 102).
Unfortunately, endocrinopathies induced by ICIs are usually irreversible (50% recovery of the pituitary-thyroid axis, 50-60% recovery of the pituitary-gonadal axis) (9, 62, 70, 103). They can occur at any time after the ICI administration. They usually tend to develop early after therapy initiation. However, seeing that the benefic, anti-cancer effects are still present after discontinuation of ICIs, there is also a risk of late development of adverse reactions (104, 105).
Risk factors for developing irAEs are the administration of high doses of ICI (only while using CTLA-4 inhibitors), preexisting autoimmune diseases (3, 106) and obesity (through the proinflammatory metabolic state) (107, 108). It is not known whether certain races may develop irAEs more frequently than others, which is why more studies need to be conducted on minorities and differentiated populations (101).
Clinicians should be vigilant in order to detect irAEs as early as possible. Before initiating treatment with ICIs, a few initial tests are recommended: thyroid function tests (TSH, free T4, free T3), hypophysis function tests (early morning cortisol levels), fasting glucose, adrenal function tests (ACTH), gonadal function tests (testosterone, luteinizing hormone – LH, and follicle-stimulating hormone – FSH), brain magnetic resonance imaging (MRI) (1, 93, 94). Patients should be monitored after each course during the first semester, every two courses over the next 6 months, and afterwards more rarely, as needed, considering the clinical suspicion (68, 109).
The management of the endocrine irAEs does not usually involve stopping the administration of ICIs (97, 103). Mild irAEs need low/moderate doses of steroids (0.5 mg/kg of prednisone or equivalent) and supportive therapy for 4-6 weeks, with the possibility of using other immunosuppressants the patient does not respond to steroids (3, 93).
Several research studies suggest that the occurrence of irAEs could predict a favorable response to treatment with ICIs, increased survival and progression-free survival, especially linked to PD-1 or PD-L1 inhibitors administration (5, 110).
Hypophysitis is the most frequent irAE found in patients treated with anti-CTLA-4 antibodies (9) (around 5% of the patients) (111), with higher incidence in patients treated with combination therapy involving ipilimumab and nivolumab, even though the mechanisms are not fully understood (96, 97). While hypophysitis generally affects women, ICI-related hypophysitis seems to be more frequently found in male patients. It tends to appear either within the first 2-3 months of therapy or even 19 months after (12, 112, 113). The supposedly direct proportional relationship between the doses of ipilimumab and the incidence of hypophysitis has been discussed in several studies, without a consensus being reached (64, 100, 114). Symptoms associated with hypophysitis are: fatigue, muscle weakness, headache, anorexia, nausea, weight loss, visual changes, intolerance of temperatures, arthralgias, mental status alteration. Hyponatremia, low ACTH or low TSH might be present (9, 100, 114). The suspicion of hypophysitis appears when two of the symptoms are identified, and the diagnosis is confirmed by hypophysis enlargement found on the brain MRI (which subsides after steroid treatment) (100, 111, 115). Given that the vast majority of the symptoms are unspecific, they can be overlooked, and the patient could develop life threatening complications. Usually, the hypopituitarism developed during hypophysitis is permanent and it does not respond to high doses of steroids (70, 97, 103), leading to infertility among other consequences (96, 100). Treatment with ICIs should not be discontinued, and the patient should receive replacing pituitary hormones (84).
Immune checkpoint inhibitor-related diabetes mellitus has been reported after treatment with PD-1/PD-L1 inhibitors (10, 12). Patients should be instructed regarding the associated symptoms of this irAE, in order to avoid life-threatening complications (93, 116). Diabetes mellitus can appear a few weeks after the ICI treatment initiation, or belatedly, after more than a year (97, 117), mostly in patients with no prior prediabetes, diabetes or other autoimmune diseases. However, islet autoantibodies have been identified in 50% of the patients that have been tested (10), and genetic susceptibility through HLA variants could be involved in diabetes development (fulminant type 1 diabetes mellitus was associated with HLA DRB1*04:05- DQB1*04:01) (10, 117). The average blood glucose level at diagnosis is higher than 550 mg/dL (116, 118), the patients accusing polyuria, polydipsia, weight loss and sometimes nausea, vomiting, abdominal pain, lethargy or even coma if diabetic ketoacidosis occurs (119). The exocrine component of the pancreas could be affected, with increased lipase levels (120). Monitoring blood glucose is crucial for a prompt diagnosis. High-dose corticosteroids are not able to reverse the ICI-related diabetes mellitus (97, 121), therefore the treatment is centered on administering insulin analogs in order to maintain the glycated hemoglobin below 8% (68, 109), followed by long-term insulin therapy (10, 12, 81).
Immune checkpoint inhibitor-related primary adrenal insufficiency presents itself by elevated ACTH levels and low glucocorticoids and mineralocorticoids, usually installed 10 weeks after initiating the treatment with ICIs (97). It seems to be more frequent in men and after administration of ipilimumab, nivolumab and pembrolizumab. The patient can report fatigue, ortostatic hypertension and abdominal disconfort (12, 122). Tests should be conducted in order to differentiate primary adrenal insufficiency from secondary adrenal insufficiency (as the first requires both glucocorticoid and mineralocorticoid administration). In the case of adrenal crisis, the treatment should include high doses of glucocorticoids (100 mg hydrocortisone administered intravenously, followed by 50 mg hydrocortisone, every 6 hours) (10, 97).
Immune checkpoint inhibitor-related primary hypoparathyroidism involves acute hypocalcemia along with low levels of parathormone (68). In some cases, autoantibodies against the calcium-sensing receptor have been identified (123, 124). In most cases, the parathyroid dysfunction is irreversible, requiring continuous calcium and active vitamin D administration (97, 124).
Acquired generalized lipodystrophy represents the loss of subcutaneous fat alongside central obesity and insulin resistance after initiating treatment with ICIs; the level of leptin is low (97, 108).
Autoimmune polyendocrine syndrome type II has been stated as a rare endocrine irAE (125). It is defined by the presence of two out of the following three elements: autoimmune thyroid disease, type 1 diabetes mellitus and Addison’s disease (126). Mutations in CTLA-4 or HLA DR3-DQ2 and HLA DR4-DQ8 variants might increase the risk of developing this syndrome after the administration of ICIs (125, 127).
Thyroid-related adverse events linked to immune checkpoint inhibitors
Thyroid dysfunctions, such as hypothyroidism, thyrotoxicosis, painless thyroiditis or the “thyroid storm” are present in 1-6% of the patients treated with ICIs (9, 114, 128) (other authors suggest the percentage amounts to over 10%) (86). Painless thyroiditis is probably under-reported, since it is not clinically suggestive, while hypothyroidism ranges between 1-15% and hyperthyroidism between 1-10%, in ICI-treated patients (pembrolizumab alone, nivolumab alone or combinations) (6, 53, 70, 92, 129).
There are certain risk factors linked to the development of thyroid-relates irAEs after ICI administration: gender (females seem to have a higher risk), age (younger patients are at risk) (97, 130), race (Caucasian and Hispanic races are associated with hypothyroidism, while African Americans are linked to thyrotoxicosis) (101), elevated anti-thyroid peroxidase antibodies (TPOAb) or anti-thyroglobulin-antibodies (TgAb) (27, 131), higher baseline TSH, a higher number of treatment cycles, usage of PD-1 and PD-L1 inhibitors rather than CTLA-4 inhibitors, obesity (linked to earlier occurrence of thyroid irAEs – every increase of 1 kg/m2 of the body mass index rises the risk of symptomatic thyrotoxicosis to 10%) (107, 132), increased fluorodeoxyglucose (18FDG) uptake in the thyroid before initiating treatment (65, 97), and renal cell carcinoma (probably due to the fact that before treatment with ICI, sunitinib is usually used for treating these patients and it can affect thyroid function as well) (133).
The thyroid irAEs usually develop 6 weeks after starting the treatment (3, 134), but they can also appear years after treatment initiation (19, 135, 136); therefore, frequent monitoring of patients treated with ICIs is important even after therapy completion (137). ICI combinations are linked to earlier development of thyroid dysfunctions (135, 136).
Many theories have emerged regarding the pathogenesis of thyroid-related irAEs. However, the most accepted current theory involves an interplay between genetic factors, cellular autoimmunity and humoral immunity, supported by T-cells cross-reactivity, increased levels of interferon gamma-inducible chemokines (which attract T-cells), the contribution of ADCC and the HLA-DR allele which is involved in autoimmunity (5, 138). Anti-PD-1 antibody-induced thyroiditis seems to be primarily a T-cell mediated process, supported by the presence of CD8+ T-cells in the thyroid, and CD4-CD8- T-cells in the thyroid and blood of the patients (139, 140). However, T-cell dependent activation of B-cells has been reported, resulting in production of autoantibodies which could serve as biomarkers for ICI-induced immunogenicity and therapeutic response (141). A modified Th1/Th2 balance has been reported in favor of Th1, with increased levels of IL-2 (which might stimulate autoreactive lymphocytes), IL-1β, GM-CSF and decrease of Il-8, G-CSF and MCP-1 (111, 142).
Two clinical patterns of thyroid dysfunction related to the administration of ICIs have been described: 1. hyperthyroidism, followed by hypothyroidism; 2. de novo hypothyroidism. There is also the triphasic pattern of presentation in some of the patients (hyperthyroidism, followed by hypothyroidism and euthyroidism), while the remaining patients experience isolated hypothyroidism or hyperthyroidism, followed by euthyroidism (88, 143). However, the distinction between hypo-, hyper- and euthyroidism should not be radical, seeing that they are part of the same process (129). Another classification of thyroid irAEs has been made regarding the duration of therapy needed for managing the thyroid dysfunction – there are thyroid irAEs which need continuous therapy for thyroid dysfunction (linked to maximum levels of thyroglobulin and thyroglobulin antibodies while administering ICIs) and those in need of temporal treatment only (144).
The patients with thyroid irAEs developed after treatment with ICIs can have three different clinical presentations: 1. newly onset hypothyroidism (TSH ≥ 4.3 mIU/L, free T4 ≤ 0.8 ng/dL) or subclinical hypothyroidism (TSH ≥ 4.3 mIU/L, free T4: 0.9-1.7 ng/dL); 2. symptomatic (overt) thyrotoxicosis (TSH ≤ 0.2 mIU/L, free T4 ≥ 1.8 ng/dL) or subclinical hyperthyroidism (TSH ≤ 0.2 mIU/L and free T4: 0.9-1.7 ng/dL); 3. acute elevation of TSH in patients previously diagnosed with hypothyroidism, requiring a 50% increase of the levothyroxine dose (145). Low levels of TSH accompanied by low or normal levels of free T4 and free T3 appear in the context of euthyroid sick syndrome, which is a differential diagnosis for thyroid irAEs (146).
Patients can be asymptomatic, or they might show nonspecific symptoms, such as tachycardia or palpitations (11, 103). However, there are 5 grades of severity for thyroid irAEs according to CTCAE, similar to all the other endocrine adverse events related to ICI administration: grade 1 – mild, grade 2 – moderate, grade 3 – severe, but not immediately life-threatening, grade 4 – life-threatening, grade 5 – death (see Table 2 for the general criteria) (87).
The thyroid workup involves measuring TSH, free T4 (which seems to successfully predict recovery, because it is less sensitive than TSH to variations of the thyroid hormone feedback), and also free T3 can be measured in cases of thyrotoxicosis (93, 94, 147).
Studies have linked irAEs to improved survival in cancer patients treated with ICIs (148, 149). Therefore, it was hypothesized that thyroid dysfunction could be seen as a predictive biomarker of treatment response (139) (patients with acute symptomatic thyrotoxicosis seem to have a better prognosis than patients without thyroid irAEs) (139, 145, 150). The presence of thyroglobulin antibodies has also been correlated with increased survival (as they are markers of a potent activation of the immune system) (151). Variations of thyroid volume might serve as imagistic markers of therapeutic response as well (due to the increase in volume after administration of a PD-1 inhibitor) (152).
Thyrotoxicosis and hyperthyroidism
“Thyrotoxicosis” refers to the excess of thyroid hormones of any cause, while “hyperthyroidism” is a more specific term, referring to an excess of thyroid hormones caused only by overactivity of the thyroid. Despite the terminology, many studies use both words as equivalents, leading to an underestimation of ICI-triggered Graves’ disease (which results in hyperthyroidism) (5). Hyperthyroidism is less common than hypothyroidism among patients under ICI treatment, with an incidence of 1-7% (6, 53, 70, 92), which is probably underestimated. CTLA-4 inhibitors cause hyperthyroidism in 0.2-1.7% of the patients, while PD-1/PD-L1 inhibitors affect 0.6-3.7% and combinations of ICIs are responsible for 8-11% of the cases (96, 97, 153).
Hyperthyroidism usually appears after 21 days of combination therapy, or after 47 days when a PD-1 inhibitor is administered alone (8, 129, 153). Graves’ disease induced by ICI appears mostly at the beginning of the treatment. However, in some cases it can occur many weeks later (90). After 3-6 weeks of the initial phase of thyrotoxicosis, hypothyroidism is frequently developed (96, 136).
The pathogenesis is variable, hyperthyroidism/thyrotoxicosis may occur either in the context of thyroiditis (when thyroid follicles are destroyed and the hormones are spilled into the bloodstream), or because of autoantibody development (e.g. Graves’ disease) (3, 6). ICI-induced thyroiditis is considered a T-cell mediated process, with CD8+ and CD4-CD8- T-cells found in the thyroid (140), as well as clusters of necrotic cells and CD163+ histiocytes (154).
The patients usually present with palpitations, heat intolerance, tremor, anxiety, emotional lability, weight loss in the presence of increased appetite, atrial fibrillation, hyperdefecation, oligo/amenorrhea in women and erectile dysfunction in men (5, 155). A phenomenon called ‘thyroid storm’ can sometimes occur, which involves fever, tachycardia or atrial fibrillation, signs of congestive heart failure and diarrhea, which poses a great threat (97, 156, 157).
When the clinician observes these symptoms, thyroid function tests should be recommended: the patient suffering from hyperthyroidism will have low levels of TSH and a high free T4 (96). Afterwards, thyroid autoantibodies should be measured (97) (pembrolizumab increases anti-thyroid peroxidase antibodies and anti-thyroglobulin antibodies in 80% of the patients with ICI-induced thyroid dysfunction) (158). Thyroid ultrasound (which can distinguish Graves’ disease from thyroiditis by vascularity), scintigraphy, technetium or iodine scanning, positron emission tomography scan (PET) might also be used for differential diagnosis. Increased uptake of 18FDG on PET scan is suggestive for destructive thyroiditis (65, 96, 97).
Graves’ disease which developed after treatment with ICIs, consists of hyperthyroidism, diffuse goiter and sometimes ophthalmopathy (159, 160). However, there are also cases of ophthalmopathy in the absence of elevated thyroid hormones (161–163). This clinical entity has been named thyroid eye disease (TED)-like orbital inflammatory syndrome, and it involves eye pain, conjunctival redness, periorbital edema, proptosis and ophthalmoplegia (5, 160). Considering that allelic variants of CTLA-4 are involved in Graves’ disease development, CTLA-4 and PD-1 gene polymorphisms might be involved in the occurrence of TED (21, 164–166). 60-70% of the Graves’ disease cases evolve over a long period of time, with numerous relapses and remissions, while 30-40% of the patients have only one episode of hyperthyroidism. It is possible that patients experience spontaneous remission (5, 167, 168).
Hypothyroidism
Hypothyroidism is the most frequent manifestation of thyroid irAEs, and it usually occurs 6 weeks after subclinical hyperthyroidism, in the context of destructive thyroiditis (in 30-40% of the patients treated with anti-PD-1/PD-L1 antibodies). Therefore, patients diagnosed with ICI-related thyroiditis should be monitored for the development of hypothyroidism (3, 6, 134, 145). CTLA-4 inhibitors cause hypothyroidism in 2.5-5.2% of the patients, while PD-1/PD-L1 inhibitors affect 3.9-8.5% and combinations of ICIs are responsible for 10.2-16.4% of the cases (96, 97, 153). Hypothyroidism usually occurs ~63 days after initiation of ICI-combination treatment, and ~70 days after intake of PD-1 inhibitors alone. Risk factors do not include age or gender (8, 155). However. they comprise preexistent autoimmune diseases (e.g. type 1 diabetes mellitus, gastric atrophy) and certain HLA-DR alleles (1, 65, 169).
The debut is often nonspecific, especially when hypothyroidism is classified as a grade 1 or 2 (97), the patients presenting weight gain, fatigue, cold intolerance, constipation, dryness of the skin, bradycardia, periorbital edema and tongue swelling (10, 100, 153, 155). The TSH levels are high, while free T4 is decreased (97). Rarely, hypothyroidism-associated myositis might occur (described in a patient after administration of nivolumab), which involves severe myalgia, arthralgia and high levels of creatine kinase (170, 171). Fortunately, this condition is reversible after levothyroxine replacement therapy. Myxedema crisis was also reported in a few cases (11, 97, 172).
It is important that patients who suffer from preexistent hypothyroidism are closely monitored with thyroid function tests at all times after initiation of ICis in order to adjust the levothyroxine doses (10, 65). Hypothyroidism developed as irAE is oftentimes permanent and it requires levothyroxine replacement therapy (10, 97).
Management of thyroid adverse events linked to immune checkpoint inhibitors
The American Society of Clinical Oncology (ASCO) has established a set of guidelines for performing the thyroid function tests: TSH and free T4 should be monitored every 4-6 weeks from starting the ICIs. TSH is considered to be the optimal screening test, accompanied by free T4 when its values are modified (93).
The treatment with ICIs should not be interrupted if thyroid irAEs occur, except when severe symptoms occur and in cases of TED, when ICIs are stopped until the symptoms disappear (8, 93, 97). Severe irAEs also require administration of steroids in order to limit the inflammation, or infliximab (anti-TNFα antibody), mycofenolate mofetil, tacrolimus, cyclosporine in cases of lack of response to steroids (6, 173). The endocrinopathies developed after ICI therapy are generally managed by the treating oncologists, but collaborations with endocrinologists should be considered in complicated or more severe cases (9, 103).
Management of thyrotoxicosis and hyperthyroidism
Prevention is key for avoiding severe irAEs. Therefore, monitoring the patient through thyroid function tests is very important throughout the treatment with ICIs (174): testing should be performed before therapy initiation, and after every 2 months (9, 93). Ultrasonographic monitoring could be useful in decision-making, considering that variations of thyroid volume related to the thyroid dysfunction have been discovered (first, the thyroid grows in volume after initiation of ICI treatment, and afterwards it starts shrinking, indicating the need of continuous thyroid hormone replacement after stopping the ICIs) (175).
Treatment options are chosen considering the CTCAE grading. Because hyperthyroidism is most frequently of grade 1 or 2 (mild or moderate), ICI therapy does not usually need to be interrupted and patients should only receive symptomatic treatment with beta blockers (8, 9, 93). If the patient is asymptomatic, she/he should only be observed and monitored thoroughly until symptoms develop, TSH decreases (5-10 mIU/L or lower) or TPO antibodies increase (93, 97). ICIs should be interrupted if the patient suffers from a grade 3 thyroid irAE and, in these cases, in general, corticotherapy should be initiated: 1-2 mg/kg/day of oral prednisolone. In grade 4 hyperthyroidism, corticotherapy is needed and it consists of 1-2 mg/kg/day intravenous methylprednisolone first, followed by oral prednisolone (1-2 mg/kg/day), decreasing the dose after one month (9, 93). However, some studies have indicated that doses of prednisone (or equivalent) higher than 7.5 mg/day have not shown benefits compared to no administration of glucocorticoids whatsoever, in terms of the duration of thyrotoxicosis, time until onset of hypothyroidism and dose of levothyroxine needed for hormonal replacement (5, 176). Depending on whether the patient suffers from thyrotoxicosis in the context of destructive thyroiditis or hyperthyroidism in the context of Graves’ disease, anti-thyroid therapy with methimazole or propylthiouracil might be considered (it is only effective for hyperthyroidism, and not for the thyrotoxicosis caused by thyroiditis) (93, 97). Therefore, patients developing Graves’ disease after the administration of ICIs should receive anti-thyroid drugs (which are usually able to maintain the euthyroidism), and only if the hyperthyroidism is not manageable through drug administration, radioiodine or thyroidectomy should be considered (158, 177). There is also a chance of spontaneous remission of hyperthyroidism caused by Graves’ disease, even if no treatment is administered (5, 168). While speaking about TED developed in the context of ICI treatment, there might be mild cases which are usually treated topically (with artificial tears, for instance), but also dramatic cases which require high doses of steroids that are usually able to stop the inflammation and lead to symptoms resolution (84, 160).
Management of hypothyroidism
For early detection of hypothyroidism as an irAE developed after ICI treatment, ASCO recommends measuring TSH and free T4 every 4–6 weeks after initiation of treatment, while also monitoring the patient after stopping the ICIs (93), while the European Society for Medical Oncology (ESMO) additionally proposes screening before starting therapy (measuring TSH, free T4 and free T3) (94).
Serum TSH levels determine what doses of levothyroxine are needed for thyroid hormonal replacement therapy. When TSH is mildly elevated (5-10 mIU/L), hormonal replacement therapy can be initiated, with low doses of levothyroxine (25-50 µg/day). If the patient is an elder with cardiovascular comorbidities, lower doses should be considered (12.5-25 µg/day), while a young, healthy patient could receive doses of 1.6 µg/kg (full estimated replacement dose) from the start (84, 97, 178). However, a recent research study has shown that in a murine model of lung carcinoma, levothyroxine increased tumor growth, while the administration of liothyronine, which is a form of T3, has prolonged survival by suppressing tumor growth. Therefore, more studies regarding the use of liothyronine instead of levothyroxine for hormonal replacement in the context of neoplasia should be conducted (179).
Discussion and conclusion
While cancer is on the rise, novel treatments are required for a better management of the disease. ICIs are a new class of drugs, emerged in the last decade, which show promising results and increased survival in cancer patients. Since ICIs are classified as a subtype of immunotherapy, their main mechanism is removing the brakes on the immune system, thus facilitating the destruction of the malignant cells. This comes bundled with a new category of adverse reactions, known as irAEs, which can affect the whole body through autoimmunity. Endocrine irAEs are particularly found in patients treated with ICIs, and among them the thyroid irAEs (including hyperthyroidism, thyrotoxicosis, hypothyroidism, ‘thyroid storm’) are the most frequent.
At this time, the exact mechanisms of thyroid irAEs induction by each of the ICIs is not fully understood. Many theories have emerged, and the most accepted current theory involves an interplay between genetic factors, cellular autoimmunity and humoral immunity, supported by T-cells cross-reactivity, increased levels of interferon gamma-inducible chemokines (which attract T-cells), the contribution of ADCC and the HLA-DR allele which is involved in autoimmunity (5, 138). The PD-1 inhibitors such as pembrolizumab, nivolumab and cemiplimab, are IgG4 antibodies and do not activate classical complement pathway, thus having less potency to initiate ADCC. Anti-PD-1 antibody-induced thyroiditis seems to be primarily a T-cell mediated process, supported by the presence of CD8+ T-cells in the thyroid, and CD4-CD8- T-cells in the thyroid and blood of the patients (139, 140). However, T-cell dependent activation of B-cells has been reported, resulting in production of autoantibodies which could serve as biomarkers for ICI-induced immunogenicity and therapeutic response (141). A modified Th1/Th2 balance has been reported in favor of Th1, with increased levels of IL-2 (which might stimulate autoreactive lymphocytes), IL-1β, GM-CSF and decrease of Il-8, G-CSF and MCP-1 (111, 142). In contrast, PD-L1, such as atezolizumab, durvalumab and avelumab and anti-CTLA-4 inhibitors, such as ipilimumab, activate the classical complement pathway and initiate the ADCC.
While all of the ICIs have somewhat overlapping thyroid irAEs, PD-L1 inhibitors, such as atezolizumab and avelumab, have a predilection in inducing hypothyroidism, while the PD-1 and CTLA-4 inhibitors can cause both hypothyroidism and hyperthyroidism (Table 1). CTLA-4 inhibitors cause hyperthyroidism in 0.2-1.7% of the patients, while PD-1/PD-L1 inhibitors affect 0.6-3.7% and combinations of ICIs are responsible for 8-11% of the cases (96, 97, 153). Anti-CTLA-4 antibodies are known to have hypophysitis as the most frequent irAE (9) (around 5% of the patients) (111). Immune checkpoint inhibitor-related diabetes mellitus has been reported after treatment with PD-1/PD-L1 inhibitors (10,12, Table 1).
A major inconvenience is that many thyroid irAEs developed in the context of ICI therapy are irreversible and in need of long-term hormonal replacement. However, there is a positive side: thyroid irAEs have been associated with better cancer outcomes, with increased survival and better prognosis for the patient developing these adverse reactions. This is probably due to efficient activation of the immune system. A better understanding of the irAEs pathogenesis through further studies is required.
Monitoring the thyroid irAEs is firstly important for preventing the life-threatening situations that might occur, such as thyroid storm, and secondly as a means of overseeing treatment response and estimating the prognosis of the patient. A close collaboration between the treating oncologist and an endocrinologist is crucial, and also raising awareness of the existence of these adverse reactions should be a priority among both clinicians and patients, as the former could recognize early symptoms of hyper- or hypothyroidism and might seek help from the medical community sooner, receiving an optimal treatment and increasing their quality of life.
Author contributions
AC and OB designed and organized the review. AC analyzed, summarized the information, and wrote the manuscript. AS contributed to the writing and improvement of the review. OB supervised the work and contributed to the writing and improvement of the manuscript. All authors contributed to the article and approved the submitted version.
Funding
OB is funded by a grant of the Romanian Ministry of Education and Research, CNCS - UEFISCDI, project number PN-III-P4-ID-PCE-2020-2027, within PNCDI III. Authors would also like to acknowledge the funding from Ministry of Research, Innovation and Digitization in Romania, under Program 1 – The Improvement of the National System of Research and Development, Subprogram 1.2 – Institutional Excellence – Projects of Excellence Funding in RDI, Contract No. 31PFE/30.12.2021.
Acknowledgments
Authors would like to acknowledge the extraordinary environment and support from our host institutions.
Conflict of interest
The authors declare that the research was conducted in the absence of any commercial or financial relationships that could be construed as a potential conflict of interest.
Publisher’s note
All claims expressed in this article are solely those of the authors and do not necessarily represent those of their affiliated organizations, or those of the publisher, the editors and the reviewers. Any product that may be evaluated in this article, or claim that may be made by its manufacturer, is not guaranteed or endorsed by the publisher.
References
1. Zhan L, Feng HF, Liu HQ, Guo LT, Chen C, Yao XL, et al. Immune checkpoint inhibitors-related thyroid dysfunction: Epidemiology, clinical presentation, possible pathogenesis, and management. Front Endocrinol (2021) 12:649863. doi: 10.3389/fendo.2021.649863
2. Shroff GS, Shroff S, Ahuja J, Truong MT, Vlahos I. Imaging spectrum of adverse events of immune checkpoint inhibitors. Clin Radiol (2021) 76(4):262–72. doi: 10.1016/j.crad.2020.11.117
3. Wright JJ, Powers AC, Johnson DB. Endocrine toxicities of immune checkpoint inhibitors. Nat Rev Endocrinol (2021) 17:389–99. doi: 10.1038/s41574-021-00484-3
4. FDA. FDA Approves yervoy for late-stage melanoma (2011). Available at: https://www.webmd.com/melanoma-skin-cancer/news/20110325/fda-approves-new-melanoma-treatment-yervoy (Accessed August 19, 2022).
5. Deligiorgi MV, Sagredou S, Vakkas L, Trafalis DT. The continuum of thyroid disorders related to immune checkpoint inhibitors: Still many pending queries. Cancers (2021) 13:5277. doi: 10.3390/cancers13215277
6. Spain L, Diem S, Larkin J. Management of toxicities of immune checkpoint inhibitors. Cancer Treat Rev (2016) 44:51–60. doi: 10.1016/j.ctrv.2016.02.001
7. Luo J, Martucci VL, Quandt Z, Groha S, Murray MH, Lovly CM, et al. Immunotherapy-mediated thyroid dysfunction: Genetic risk and impact on outcomes with PD-1 blockade in non–small cell lung cancer. Clin Cancer Res (2021) 27:5131–40. doi: 10.1158/1078-0432.CCR-21-0921
8. Sabbagh R, Azar NS, Eid AA, Azar ST. Thyroid dysfunctions due to immune checkpoint inhibitors: A review. Int J Gen Med (2020) 13:1003–9. doi: 10.2147/IJGM.S261433
9. Ferrari SM, Fallahi P, Galetta F, Citi E, Benvenga S, Antonelli A, et al. Thyroid disorders induced by checkpoint inhibitors. Rev Endocrine Metab Disord (2018) 19:325–333. doi: 10.1007/s11154-018-9463-2
10. Chang LS, Barroso-Sousa R, Tolaney SM, Hodi FS, Kaiser UB, Min L. Endocrine toxicity of cancer immunotherapy targeting immune checkpoints. Endocrine Rev (2018) 40:17–65. doi: 10.1210/er.2018-00006
11. Ferrari SM, Fallahi P, Elia G, Ragusa F, Ruffilli I, Patrizio A, et al. Autoimmune endocrine dysfunctions associated with cancer immunotherapies. Int J Mol Sci (2019) 20:2560. doi: 10.3390/ijms20102560
12. Paschou SA, Stefanaki K, Psaltopoulou T, Liontos M, Koutsoukos K, Zagouri F, et al. How we treat endocrine complications of immune checkpoint inhibitors. ESMO Open (2021) 6:100011. doi: 10.1016/j.esmoop.2020.100011
13. Elia G, Ferrari SM, Galdiero MR, Ragusa F, Paparo SR, Ruffilli I, et al. New insight in endocrine-related adverse events associated to immune checkpoint blockade. Best Pract Research: Clin Endocrinol Metab (2020) 34:101370. doi: 10.1016/j.beem.2019.101370
14. Chye A, Allen I, Barnet M, Burnett DL. Insights into the host contribution of endocrine associated immune-related adverse events to immune checkpoint inhibition therapy. Front Oncol (2022) 12:894015. doi: 10.3389/fonc.2022.894015
15. Garcia-Diaz A, Shin DS, Moreno BH, Saco J, Escuin-Ordinas H, Rodriguez GA, et al. Interferon receptor signaling pathways regulating PD-L1 and PD-L2 expression. Cell Rep (2017) 19:1189–201. doi: 10.1016/j.celrep.2017.04.031
16. Imblum BA, Baloch ZW, Fraker D, LiVolsi VA. Pembrolizumab-induced thyroiditis. Endocrine Pathol (2019) 30:163–7. doi: 10.1007/s12022-019-9579-2
17. Chowdhury S, Veyhl J, Jessa F, Polyakova O, Alenzi A, Macmillan C, et al. Programmed death-ligand 1 overexpression is a prognostic marker for aggressive papillary thyroid cancer and its variants. Oncotarget (2016) 7:32318–28. doi: 10.18632/oncotarget.8698
18. Varricchi G, Loffredo S, Marone G, Modestino L, Fallahi P, Ferrari SM, et al. The immune landscape of thyroid cancer in the context of immune checkpoint inhibition. Int J Mol Sci (2019) 20:3934. doi: 10.3390/ijms20163934
19. Guaraldi F, la Selva R, Samà MT, D’Angelo V, Gori D, Fava P, et al. Characterization and implications of thyroid dysfunction induced by immune checkpoint inhibitors in real-life clinical practice: a long-term prospective study from a referral institution. J Endocrinological Invest (2018) 41:549–56. doi: 10.1007/s40618-017-0772-1
20. Pardoll DM. The blockade of immune checkpoints in cancer immunotherapy. Nat Rev Cancer (2012) 12:252–64. doi: 10.1038/nrc3239
21. Chau CYC, Shih KC, Chow LLW, Lee VHF. Considerations for use of immune checkpoint inhibitors in cancer therapy for patients with Co-existing thyroid eye disease. Ophthalmol Ther (2021) 10:5–12. doi: 10.1007/s40123-020-00317-y
22. Ribas A. Tumor immunotherapy directed at PD-1. New Engl J Med (2012) 366:2517–9. doi: 10.1056/NEJMe1205943
23. Okazaki T, Honjo T. PD-1 and PD-1 ligands: From discovery to clinical application. Int Immunol (2007) 19:813–24. doi: 10.1093/intimm/dxm057
24. Patel NS, Oury A, Daniels GA, Bazhenova L, Patel SP. Incidence of thyroid function test abnormalities in patients receiving immune-checkpoint inhibitors for cancer treatment. Oncologist. (2018) 23:1236–41. doi: 10.1634/theoncologist.2017-0375
25. Schubert D, Bode C, Kenefeck R, Hou TZ, Wing JB, Kennedy A, et al. Autosomal dominant immune dysregulation syndrome in humans with CTLA4 mutations. Nat Med (2014) 20:1410–6. doi: 10.1038/nm.3746
26. Lubin D, Baraban E, Lisby A, Jalali-Farahani S, Zhang P, Livolsi V. Papillary thyroid carcinoma emerging from hashimoto thyroiditis demonstrates increased PD-L1 expression, which persists with metastasis. Endocrine Pathol (2018) 29:317–23. doi: 10.1007/s12022-018-9540-9
27. Kobayashi T, Iwama S, Yasuda Y, Okada N, Tsunekawa T, Onoue T, et al. Patients with antithyroid antibodies are prone to develop destructive thyroiditis by nivolumab: A prospective study. J Endocrine Soc (2018) 2:241–51. doi: 10.1210/js.2017-00432
28. Kimbara S, Fujiwara Y, Iwama S, Ohashi K, Kuchiba A, Arima H, et al. Association of antithyroglobulin antibodies with the development of thyroid dysfunction induced by nivolumab. Cancer Sci (2018) 109:3583–90. doi: 10.1111/cas.13800
29. Kannaujia AK, Gupta A, Verma S, Srivastava U, Haldar R, Jasuja S. Importance of routine laboratory investigations before elective surgery. Discoveries (2020) 8(3):e114. doi: 10.15190/d.2020.11
30. Chowell D, Yoo SK, Valero C, Pastore A, Krishna C, Lee M, et al. Improved prediction of immune checkpoint blockade efficacy across multiple cancer types. Nat Biotechnol (2022) 40:499–506. doi: 10.1038/s41587-021-01070-8
31. Chan TA, Yarchoan M, Jaffee E, Swanton C, Quezada SA, Stenzinger A, et al. Development of tumor mutation burden as an immunotherapy biomarker: Utility for the oncology clinic. Ann Oncol (2019) 30(1):44–56. doi: 10.1093/annonc/mdy495
32. Li Y, Meng Y, Sun H, Ye L, Zeng F, Chen X, et al. The prognostic significance of baseline neutrophil-to-Lymphocyte ratio in melanoma patients receiving immunotherapy. J Immunotherapy (2021) 45:43–50. doi: 10.1097/CJI.0000000000000392
33. Valero C, Lee M, Hoen D, Weiss K, Kelly DW, Adusumilli PS, et al. Pretreatment neutrophil-to-lymphocyte ratio and mutational burden as biomarkers of tumor response to immune checkpoint inhibitors. Nat Commun (2021) 12:729. doi: 10.1038/s41467-021-20935-9
34. Chowell D, Krishna C, Pierini F, Makarov V, Rizvi NA, Kuo F, et al. Evolutionary divergence of HLA class I genotype impacts efficacy of cancer immunotherapy. Nat Med (2019) 25:1715–20. doi: 10.1038/s41591-019-0639-4
35. Tumeh PC, Harview CL, Yearley JH, Shintaku IP, Taylor EJM, Robert L, et al. PD-1 blockade induces responses by inhibiting adaptive immune resistance. Nature (2014) 515:568–71. doi: 10.1038/nature13954
36. Rizvi NA, Hellmann MD, Snyder A, Kvistborg P, Makarov V, Havel JJ, et al. Cancer immunology. mutational landscape determines sensitivity to PD-1 blockade in non-small cell lung cancer. Science (2015) 348:124–8. doi: 10.1126/science.aaa1348
37. Davoli T, Uno H, Wooten EC, Elledge SJ. Tumor aneuploidy correlates with markers of immune evasion and with reduced response to immunotherapy. Science (2017) 355:eaaf8399. doi: 10.1126/science.aaf8399
38. Gopalakrishnan V, Spencer CN, Nezi L, Reuben A, Andrews MC, Karpinets TV, et al. Gut microbiome modulates response to anti-PD-1 immunotherapy in melanoma patients. Science (2018) 359:97–103. doi: 10.1126/science.aan4236
39. Jiang P, Gu S, Pan D, Fu J, Sahu A, Hu X, et al. Signatures of T cell dysfunction and exclusion predict cancer immunotherapy response. Nat Med (2018) 24:1550–8. doi: 10.1038/s41591-018-0136-1
40. Cristescu R, Mogg R, Ayers M, Albright A, Murphy E, Yearley J, et al. Pan-tumor genomic biomarkers for PD-1 checkpoint blockade-based immunotherapy. Science (2018) 362:eaar3593. doi: 10.1126/science.aar3593
41. Mandal R, Samstein RM, Lee KW, Havel JJ, Wang H, Krishna C, et al. Genetic diversity of tumors with mismatch repair deficiency influences anti-PD-1 immunotherapy response. Science (2019) 364:485–491. doi: 10.1126/science.aau0447
42. Valero C, Lee M, Hoen D, Wang J, Nadeem Z, Patel N, et al. The association between tumor mutational burden and prognosis is dependent on treatment context. Nat Genet (2021) 53:11–5. doi: 10.1038/s41588-020-00752-4
43. Peng D, Zhang CJ, Gong YQ, Hao H, Guan B, Li XS, et al. Prognostic significance of HALP (hemoglobin, albumin, lymphocyte and platelet) in patients with bladder cancer after radical cystectomy. Sci Rep (2018) 8:794. doi: 10.1038/s41598-018-19146-y
44. Kuai J, Yang F, Li GJ, Fang XJ, Gao BQ. In vitro-activated tumor-specific T lymphocytes prolong the survival of patients with advanced gastric cancer: A retrospective cohort study. OncoTargets Ther (2016) 9:3763–70. doi: 10.2147/OTT.S102909
45. Sanchez A, Furberg H, Kuo F, Vuong L, Ged Y, Patil S, et al. Transcriptomic signatures related to the obesity paradox in patients with clear cell renal cell carcinoma: a cohort study. Lancet Oncol (2020) 21:283–93. doi: 10.1016/S1470-2045(19)30797-1
46. Ikeguchi A, Machiorlatti M, Vesely SK. Disparity in outcomes of melanoma adjuvant immunotherapy by demographic profile. Melanoma Manage (2020) 7:MMT43. doi: 10.2217/mmt-2020-0002
47. Van Allen EM, Miao D, Schilling B, Shukla SA, Blank C, Zimmer L, et al. Genomic correlates of response to CTLA-4 blockade in metastatic melanoma. Science (2015) 350:207–11. doi: 10.1126/science.aad0095
48. Jardim DL, Goodman A, de Melo Gagliato D, Kurzrock R. The challenges of tumor mutational burden as an immunotherapy biomarker. Cancer Cell (2021) 39:154–73. doi: 10.1016/j.ccell.2020.10.001
49. Ott PA, Bang YJ, Piha-Paul SA, Razak ARA, Bennouna J, Soria JC, et al. T-Cell-Inflamed gene-expression profile, programmed death ligand 1 expression, and tumor mutational burden predict efficacy in patients treated with pembrolizumab across 20 cancers: KEYNOTE-028. . J Clin Oncol (2018) 37:318–27. doi: 10.1200/JCO.2018.78.2276
50. Ralph C, Elkord E, Burt DJ, O’Dwyer JF, Austin EB, Stern PL, et al. Modulation of lymphocyte regulation for cancer therapy: A phase II trial of tremelimumab in advanced gastric and esophageal adenocarcinoma. Clin Cancer Res (2010) 16:1662–72. doi: 10.1158/1078-0432.CCR-09-2870
51. Arru C, de Miglio MR, Cossu A, Muroni MR, Carru C, Zinellu A, et al. Durvalumab plus tremelimumab in solid tumors: A systematic review. Adv Ther (2021) 38:3674–93. doi: 10.1007/s12325-021-01796-6
52. Fernandes S, Varlamov EV, McCartney S, Fleseriu M. A novel etiology of hypophysitis: Immune checkpoint inhibitors. Endocrinol Metab Clinics North Am (2020) 49:387–99. doi: 10.1016/j.ecl.2020.05.002
53. Weber JS, D’Angelo SP, Minor D, Hodi FS, Gutzmer R, Neyns B, et al. Nivolumab versus chemotherapy in patients with advanced melanoma who progressed after anti-CTLA-4 treatment (CheckMate 037): A randomised, controlled, open-label, phase 3 trial. Lancet Oncol (2015) 16:375–84. doi: 10.1016/S1470-2045(15)70076-8
54. Tawbi HA, Forsyth PA, Algazi A, Hamid O, Hodi FS, Moschos SJ, et al. Combined nivolumab and ipilimumab in melanoma metastatic to the brain. New Engl J Med (2018) 379:722–30. doi: 10.1056/NEJMoa1805453
55. Fernandes GNC. Immunotherapy for melanoma brain metastases. Discoveries (2019) 7:e93. doi: 10.15190/d.2019.6
56. Soare GR, Soare CA. Immunotherapy for breast cancer: First FDA approved regimen. Discoveries (2019) 7:e91. doi: 10.15190/d.2019.4
57. U.S. Food and Drug Administration. FDA Approves atezolizumab for PD-L1 positive unresectable locally advanced or metastatic triple-negative breast cancer (2019). Available at: www.fda.gov/drugs/drug-approvals-and-databases/fda-approves-atezolizumab-pd-l1-positive-unresectable-locally-advanced-or-metastatic-triple-negative (Accessed August 16, 2022).
58. Postow MA, Callahan MK, Barker CA, Yamada Y, Yuan J, Kitano S, et al. Immunologic correlates of the abscopal effect in a patient with melanoma. New Engl J Med (2012) 366:925–31. doi: 10.1056/NEJMoa1112824
59. U.S. Food and Drug Administration. Nivolumab (Opdivo) for Hodgkin lymphoma (2016). Available at: www.fda.gov/drugs/resources-information-approved-drugs/nivolumab-opdivo-hodgkin-lymphoma (Accessed August 16, 2022).
60. U.S. Food and Drug Administration. Pembrolizumab (KEYTRUDA) for classical Hodgkin lymphoma (2017). Available at: www.fda.gov/drugs/resources-information-approved-drugs/pembrolizumab-keytruda-classical-hodgkin-lymphoma (Accessed August 16, 2022).
61. Wolchok JD, Neyns B, Linette G, Negrier S, Lutzky J, Thomas L, et al. Ipilimumab monotherapy in patients with pretreated advanced melanoma: a randomised, double-blind, multicentre, phase 2, dose-ranging study. Lancet Oncol (2010) 11:155–64. doi: 10.1016/S1470-2045(09)70334-1
62. Weber JS, Kähler KC, Hauschild A. Management of immune-related adverse events and kinetics of response with ipilimumab. J Clin Oncol (2012) 30:2691–7. doi: 10.1200/JCO.2012.41.6750
63. FDA Approves pembrolizumab for advanced melanoma (2014). Available at: https://www.esmo.org/oncology-news/archive/fda-approves-pembrolizumab-for-advanced-melanoma (Accessed August 16, 2022).
64. Okura N, Asano M, Uchino J, Morimoto Y, Iwasaku M, Kaneko Y, et al. Endocrinopathies associated with immune checkpoint inhibitor cancer treatment: A review. J Clin Med (2020) 9:1–12. doi: 10.3390/jcm9072033
65. Delivanis DA, Gustafson MP, Bornschlegl S, Merten MM, Kottschade L, Withers S, et al. Pembrolizumab-induced thyroiditis: Comprehensive clinical review and insights into underlying involved mechanisms. J Clin Endocrinol Metab (2017) 102:2770–80. doi: 10.1210/jc.2017-00448
66. Thuillier P, Joly C, Alavi Z, Crouzeix G, Descourt R, Quere G, et al. Thyroid dysfunction induced by immune checkpoint inhibitors is associated with a better progression-free survival and overall survival in non-small cell lung cancer: an original cohort study. Cancer Immunol Immunother (2021) 70:2023–33. doi: 10.1007/s00262-020-02802-6
67. Kazandjian D, Suzman DL, Blumenthal G, Mushti S, He K, Libeg M, et al. FDA Approval summary: Nivolumab for the treatment of metastatic non-small cell lung cancer with progression on or after platinum-based chemotherapy. Oncologist (2016) 21:634–42. doi: 10.1634/theoncologist.2015-0507
68. Deligiannis NG, Sosa S, Danilowicz K, Rizzo LFL. Endocrine dysfunction induced by immune checkpoint inhibitors. Medicina (2021) 81:269–78.
69. Hodi FS, Chesney J, Pavlick AC, Robert C, Grossmann KF, McDermott DF, et al. Combined nivolumab and ipilimumab versus ipilimumab alone in patients with advanced melanoma: 2-year overall survival outcomes in a multicentre, randomised, controlled, phase 2 trial. Lancet Oncol (2016) 17:1558–68. doi: 10.1016/S1470-2045(16)30366-7
70. Larkin J, Chiarion-Sileni V, Gonzalez R, Grob JJ, Cowey CL, Lao CD, et al. Combined nivolumab and ipilimumab or monotherapy in untreated melanoma. New Engl J Med (2015) 373:23–34. doi: 10.1056/NEJMoa1504030
71. Neppl C, Kaderli RM, Trepp R, Schmitt AM, Berger MD, Wehrli M, et al. Histology of nivolumab-induced thyroiditis. Thyroid (2018) 28:1727–8. doi: 10.1089/thy.2018.0418
72. Gambale E, Fancelli S, Caliman E, Petrella MC, Doni L, Pillozzi S, et al. Immune checkpoint blockade with anti-programmed cell death 1 (PD-1) monoclonal antibody (mAb) cemiplimab: Ongoing and future perspectives in rare genital cancers treatment. J Immunother Cancer (2022) 10:e003540. doi: 10.1136/jitc-2021-003540
73. U.S. Food and Drug Administration. FDA Approves cemiplimab-rwlc for metastatic or locally advanced cutaneous squamous cell carcinoma (2018). Available at: https://www.fda.gov/drugs/drug-approvals-and-databases/fda-approves-cemiplimab-rwlc-metastatic-or-locally-advanced-cutaneous-squamous-cell-carcinoma (Accessed August 19, 2022).
74. National Institute of Diabetes and Digestive and Kidney Diseases. LiverTox: Clinical and research information on drug-induced liver injury. Cemiplimab. Bethesda: National Institute of Diabetes and Digestive and Kidney Diseases (2022). Available at: https://www.ncbi.nlm.nih.gov/books/NBK548288/.
75. Migden MR, Rischin D, Schmults CD, Guminski A, Hauschild A, Lewis KD, et al. PD-1 blockade with cemiplimab in advanced cutaneous squamous-cell carcinoma. N Engl J Med (2018) 379:341–51. doi: 10.1056/NEJMoa1805131
76. Deligiorgi MV, Panayiotidis MI, Trafalis DT. Endocrine adverse events related with immune checkpoint inhibitors: An update for clinicians. Immunother (2020) 12:481–510. doi: 10.2217/imt-2019-0132
77. U.S. Food and Drug Administration. FDA Approves new, targeted treatment for bladder cancer (2016). Available at: https://www.fda.gov/news-events/press-announcements/fda-approves-new-targeted-treatment-bladder-cancer (Accessed August 19, 2022).
78. Horn L, Mansfield AS, Szczęsna A, Havel L, Krzakowski M, Hochmair MJ, et al. First-line atezolizumab plus chemotherapy in extensive-stage small-cell lung cancer. N Engl J Med (2018) 379:2220–9. doi: 10.1056/NEJMoa1809064
79. Socinski MA, Jotte RM, Cappuzzo F, Orlandi F, Stroyakovskiy D, Nogami N, et al. Atezolizumab for first-line treatment of metastatic nonsquamous NSCLC. N Engl J Med (2018) 378:2288–301. doi: 10.1056/NEJMoa1716948
80. Emens LA, Cruz C, Eder JP, Braiteh F, Chung C, Tolaney SM, et al. Long-term clinical outcomes and biomarker analyses of atezolizumab therapy for patients with metastatic triple-negative breast cancer: A phase 1 study. JAMA Oncol (2019) 5:74–82. doi: 10.1001/jamaoncol.2018.4224
81. Ntali G, Kassi E, Alevizaki M. Endocrine sequelae of immune checkpoint inhibitors. Hormones (2017) 16:341–50. doi: 10.14310/horm.2002.1754
82. U.S. Food and Drug Administration. Durvalumab (Imfinzi) (2017). Available at: https://www.fda.gov/drugs/resources-information-approved-drugs/durvalumab-imfinzi (Accessed August 19, 2022).
83. U.S. Food and Drug Administration. Avelumab (BAVENCIO) (2017). Available at: https://www.fda.gov/drugs/resources-information-approved-drugs/avelumab-bavencio (Accessed August 19, 2022).
84. González-Rodríguez E, Rodríguez-Abreu D. Immune checkpoint inhibitors: Review and management of endocrine adverse events. Oncologist (2016) 21:804–16. doi: 10.1634/theoncologist.2015-0509
85. Esfahani K, Meti N, Miller WH, Hudson M. Adverse events associated with immune checkpoint inhibitor treatment for cancer. Can Med Assoc J (2019) 191:E40–6. doi: 10.1503/cmaj.180870
86. Muir CA, Menzies AM, Clifton-Bligh R, Tsang VHM. Thyroid toxicity following immune checkpoint inhibitor treatment in advanced cancer. Thyroid (2020) 30:1458–69. doi: 10.1089/thy.2020.0032
87. National Cancer Institute. Common terminology criteria for adverse events (CTCAE) v5.0 (2017). Available at: https://ctep.cancer.gov/protocoldevelopment/electronic_applications/docs/CTCAE_v5_Quick_Reference_5x7.pdf (Accessed August 19, 2022).
88. Olsson-Brown A, Lord R, Sacco J, Wagg J, Coles M, Pirmohamed M. Two distinct clinical patterns of checkpoint inhibitor-induced thyroid dysfunction. Endocrine Connections (2020) 9:318–25. doi: 10.1530/EC-19-0473
89. Postow MA, Chesney J, Pavlick AC, Robert C, Grossmann K, McDermott D, et al. Nivolumab and ipilimumab versus ipilimumab in untreated melanoma. New Engl J Med (2015) 372:2006–17. doi: 10.1056/NEJMoa1414428
90. Peiffert M, Cugnet-Anceau C, Dalle S, Chikh K, Assaad S, Disse E, et al. Graves’ disease during immune checkpoint inhibitor therapy (A case series and literature review). Cancers (2021) 13:1944. doi: 10.3390/cancers13081944
91. Arnaud-Coffin P, Maillet D, Gan HK, Stelmes JJ, You B, Dalle S, et al. A systematic review of adverse events in randomized trials assessing immune checkpoint inhibitors. Int J Cancer (2019) 145:639–48. doi: 10.1002/ijc.32132
92. Robert C, Schachter J, v. LG, Arance A, Grob JJ, Mortier L, et al. Pembrolizumab versus ipilimumab in advanced melanoma. New Engl J Med (2015) 372:2521–32. doi: 10.1056/NEJMoa1503093
93. Brahmer JR, Lacchetti C, Schneider BJ, Atkins MB, Brassil KJ, Caterino JM, et al. Management of immune-related adverse events in patients treated with immune checkpoint inhibitor therapy: American society of clinical oncology clinical practice guideline. J Clin Oncol (2018) 36:1714–68. doi: 10.1200/JCO.2017.77.6385
94. Haanen JBAG, Carbonnel F, Robert C, Kerr KM, Peters S, Larkin J, et al. Management of toxicities from immunotherapy: ESMO clinical practice guidelines for diagnosis, treatment and follow-up. Ann Oncol (2017) 28:iv119–42. doi: 10.1093/annonc/mdx225
95. Martins F, Sofiya L, Sykiotis GP, Lamine F, Maillard M, Fraga M, et al. Adverse effects of immune-checkpoint inhibitors: epidemiology, management and surveillance. Nat Rev Clin Oncol (2019) 16:563–80. doi: 10.1038/s41571-019-0218-0
96. De Filette J, Andreescu CE, Cools F, Bravenboer B, Velkeniers B. A systematic review and meta-analysis of endocrine-related adverse events associated with immune checkpoint inhibitors. Hormone Metab Res (2019) 51:145–56. doi: 10.1055/a-0843-3366
97. Stelmachowska-Banaś M, Czajka-Oraniec I. Management of endocrine immune-related adverse events of immune checkpoint inhibitors: An updated review. Endoc Connect (2020) 9:R207–28. doi: 10.1530/EC-20-0342
98. Jehl A, Cugnet-Anceau C, Vigouroux C, Legeay AL, Dalle S, Harou O, et al. Acquired generalized lipodystrophy: A new cause of anti-PD-1 immune-related diabetes. Diabetes Care (2019) 42:2008–10. doi: 10.2337/dc18-2535
99. Del Rivero J, Cordes LM, Klubo-Gwiezdzinska J, Madan RA, Nieman LK, Gulley JL. Endocrine-related adverse events related to immune checkpoint inhibitors: Proposed algorithms for management. Oncologist (2020) 25:290–300. doi: 10.1634/theoncologist.2018-0470
100. Ryder M, Callahan M, Postow MA, Wolchok J, Fagin JA. Endocrine-related adverse events following ipilimumab in patients with advanced melanoma: A comprehensive retrospective review from a single institution. Endocrine-Related Cancer (2014) 21:371–81. doi: 10.1530/ERC-13-0499
101. D’Aiello A, Lin J, Gucalp R, Tabatabaie V, Cheng H, Bloomgarden NA, et al. Thyroid dysfunction in lung cancer patients treated with immune checkpoint inhibitors (ICIs): Outcomes in a multiethnic urban cohort. Cancers (2021) 13:1464. doi: 10.3390/cancers13061464
102. Iwama S, de Remigis A, Callahan MK, Slovin SF, Wolchok JD, Caturegli P. Pituitary expression of CTLA-4 mediates hypophysitis secondary to administration of CTLA-4 blocking antibody. Sci Trans Med (2014) 6:230ra45. doi: 10.1126/scitranslmed.3008002
103. Sznol M, Postow MA, Davies MJ, Pavlick AC, Plimack ER, Shaheen M, et al. Endocrine-related adverse events associated with immune checkpoint blockade and expert insights on their management. Cancer Treat Rev (2017) 58:70–6. doi: 10.1016/j.ctrv.2017.06.002
104. Jin KT, Wang SB, Ying XJ, Lan HR, Lv JQ, Zhang LH, et al. Immune-mediated adverse effects of immune-checkpoint inhibitors and their management in cancer. Immunol Lett (2020) 221:61–71. doi: 10.1016/j.imlet.2020.02.008
105. Schadendorf D, Wolchok JD, Hodi FS, Chiarion-Sileni V, Gonzalez R, Rutkowski P, et al. Efficacy and safety outcomes in patients with advanced melanoma who discontinued treatment with nivolumab and ipilimumab because of adverse events: A pooled analysis of randomized phase II and III trials. J Clin Oncol (2017) 35:3807–14. doi: 10.1200/JCO.2017.73.2289
106. Johnson DB, Sullivan RJ, Ott PA, Carlino MS, Khushalani NI, Ye F, et al. Ipilimumab therapy in patients with advanced melanoma and preexisting autoimmune disorders. JAMA Oncol (2016) 2:234–40. doi: 10.1001/jamaoncol.2015.4368
107. Pollack R, Ashash A, Cahn A, Rottenberg Y, Stern H, Dresner-Pollak R. Immune checkpoint inhibitor-induced thyroid dysfunction is associated with higher body mass index. J Clin Endocrinol Metab (2020) 105:1–8. doi: 10.1210/clinem/dgaa458
108. Wang Z, Aguilar EG, Luna JI, Dunai C, Khuat LT, Le CT, et al. Paradoxical effects of obesity on T cell function during tumor progression and PD-1 checkpoint blockade. Nat Med (2019) 25:141–51. doi: 10.1038/s41591-018-0221-5
109. Albarel F, Castinetti F, Brue T. Management of endocrine disease immune check point inhibitors-induced hypophysitis. Eur J Endocrinol (2019) 181:R107–18. doi: 10.1530/EJE-19-0169
110. Hussaini S, Chehade R, Boldt RG, Raphael J, Blanchette P, Maleki Vareki S, et al. Association between immune-related side effects and efficacy and benefit of immune checkpoint inhibitors – a systematic review and meta-analysis. Cancer Treat Rev (2021) 92:102134. doi: 10.1016/j.ctrv.2020.102134
111. Corsello SM, Barnabei A, Marchetti P, de Vecchis L, Salvatori R, Torino F. Endocrine side effects induced by immune checkpoint inhibitors. J Clin Endocrinol Metab (2013) 98:1361–75. doi: 10.1210/jc.2012-4075
112. Faje AT, Sullivan R, Lawrence D, Tritos NA, Fadden R, Klibanski A, et al. Ipilimumab-induced hypophysitis: A detailed longitudinal analysis in a large cohort of patients with metastatic melanoma. J Clin Endocrinol Metab (2014) 99:4078–85. doi: 10.1210/jc.2014-2306
113. Caturegli P, di Dalmazi G, Lombardi M, Grosso F, Larman HB, Larman T, et al. Hypophysitis secondary to cytotoxic T-Lymphocyte–associated protein 4 blockade: Insights into pathogenesis from an autopsy series. Am J Pathol (2016) 186:3225–35. doi: 10.1016/j.ajpath.2016.08.020
114. Byun DJ, Wolchok JD, Rosenberg LM, Girotra M. Cancer immunotherapy-immune checkpoint blockade and associated endocrinopathies. Nat Rev Endocrinol (2017) 13:195–207. doi: 10.1038/nrendo.2016.205
115. Torino F, Corsello SM, Salvatori R. Endocrinological side-effects of immune checkpoint inhibitors. Curr Opin Oncol (2016) 28:278–87. doi: 10.1097/CCO.0000000000000293
116. Quandt Z, Young A, Perdigoto AL, Herold KC, Anderson MS. Annual review of medicine autoimmune endocrinopathies: An emerging complication of immune checkpoint inhibitors. Annu Rev Med (2021) 72:313–30. doi: 10.1146/annurev-med-050219-034237
117. Okamoto M, Okamoto M, Gotoh K, Masaki T, Ozeki Y, Ando H, et al. Fulminant type 1 diabetes mellitus with anti-programmed cell death-1 therapy. J Diabetes Invest (2016) 7:915–8. doi: 10.1111/jdi.12531
118. Akturk HK, Kahramangil D, Sarwal A, Hoffecker L, Murad MH, Michels AW. Immune checkpoint inhibitor-induced type 1 diabetes: a systematic review and meta-analysis. Diabetic Med (2019) 36:1075–81. doi: 10.1111/dme.14050
119. De Filette JMK, Pen JJ, Decoster L, Vissers T, Bravenboer B, van der Auwera BJ, et al. Immune checkpoint inhibitors and type 1 diabetes mellitus: A case report and systematic review. Eur J Endocrinol (2019) 181:363–74. doi: 10.1530/EJE-19-0291
120. Marchand L, Thivolet A, Dalle S, Chikh K, Reffet S, Vouillarmet J, et al. Diabetes mellitus induced by PD-1 and PD-L1 inhibitors: description of pancreatic endocrine and exocrine phenotype. Acta Diabetologica (2019) 56:441–8. doi: 10.1007/s00592-018-1234-8
121. Aleksova J, Lau PKH, Soldatos G, McArthur G. Glucocorticoids did not reverse type 1 diabetes mellitus secondary to pembrolizumab in a patient with metastatic melanoma. BMJ Case Rep (2016) 2016:bcr2016217454. doi: 10.1136/bcr-2016-217454
122. Grouthier V, Lebrun-Vignes B, Moey M, Johnson DB, Moslehi JJ, Salem JE, et al. Immune checkpoint inhibitor-associated primary adrenal insufficiency: WHO VigiBase report analysis. Oncologist (2020) 25:696–701. doi: 10.1634/theoncologist.2019-0555
123. Piranavan P, Li Y, Brown E, Kemp EH, Trivedi N. Immune checkpoint inhibitor-induced hypoparathyroidism associated with calcium-sensing receptor-activating autoantibodies. J Clin Endocrinol Metab (2018) 104:550–6. doi: 10.1210/jc.2018-01151
124. Dadu R, Rodgers TE, Trinh VA, Kemp EH, Cubb TD, Patel S, et al. Calcium-sensing receptor autoantibody-mediated hypoparathyroidism associated with immune checkpoint inhibitor therapy: diagnosis and long-term follow-up. J Immunotherapy Cancer (2020) 8:e000687. doi: 10.1136/jitc-2020-000687
125. Zhao Z, Wang X, Bao X-Q, Ning J, Shang M, Zhang D. Autoimmune polyendocrine syndrome induced by immune checkpoint inhibitors: a systematic review. Cancer Immunology Immunotherapy (2021) 70:1527–40. doi: 10.1007/s00262-020-02699-1
126. Eisenbarth GS. Autoimmune polyendocrine syndromes. Adv Exp Med Biol (2004) 552:204–18. doi: 10.1056/NEJMra030158
127. Lanzolla G, Coppelli A, Cosottini M, del Prato S, Marcocci C, Lupi I. Immune checkpoint blockade anti-PD-L1 as a trigger for autoimmune polyendocrine syndrome. J Endocrine Soc (2019) 3:496–503. doi: 10.1210/js.2018-00366
128. Hodi FS, O’Day SJ, McDermott DF, Weber RW, Sosman JA, Haanen JB, et al. Improved survival with ipilimumab in patients with metastatic melanoma. New Engl J Med (2010) 363:711–23. doi: 10.1056/NEJMoa1003466
129. Iyer PC, Cabanillas ME, Waguespack SG, Hu MI, Thosani S, Lavis VR, et al. Immune-related thyroiditis with immune checkpoint inhibitors. Thyroid (2018) 28:1243–51. doi: 10.1089/thy.2018.0116
130. Campredon P, Mouly C, Lusque A, Bigay-Game L, Bousquet E, Mazières J, et al. Incidence of thyroid dysfunctions during treatment with nivolumab for non-small cell lung cancer: Retrospective study of 105 patients. Presse Medicale (2019) 48:e199–207. doi: 10.1016/j.lpm.2018.10.019
131. Inaba H, Ariyasu H, Takeshima K, Iwakura H, Akamizu T. Comprehensive research on thyroid diseases associated with autoimmunity: autoimmune thyroid diseases, thyroid diseases during immune-checkpoint inhibitors therapy, and immunoglobulin-G4-associated thyroid diseases. Endocrine J (2019) 66:843–52. doi: 10.1507/endocrj.EJ19-0234
132. Pollack RM, Kagan M, Lotem M, Dresner-Pollak R. Baseline TSH level is associated with risk of anti–PD-1–induced thyroid dysfunction. Endocrine Pract (2019) 25:824–9. doi: 10.4158/EP-2018-0472
133. Percik R, Liel Y, Urban D, Bar J, Ben-Ami E, Abu Tailakh M. Thyroid dysfunction and survival in cancer patients treated with immune checkpoint inhibitors: analyses from a large single tertiary cancer center database. Acta Oncol (2021) 60:1466–71. doi: 10.1080/0284186X.2021.1958006
134. Tan MH, Iyengar R, Mizokami-Stout K, Yentz S, MacEachern MP, Shen LY, et al. Spectrum of immune checkpoint inhibitors-induced endocrinopathies in cancer patients: a scoping review of case reports. Clin Diabetes Endocrinol (2019) 5:1. doi: 10.1186/s40842-018-0073-4
135. Jannin A, Penel N, Ladsous M, Christine Vantyghem M, do Cao C, Arnaud J, et al. Tyrosine kinase inhibitors and immune checkpoint inhibitors-induced thyroid disorders. Crit Rev Oncology/Hematology (2019) 141:23–35. doi: 10.1016/j.critrevonc.2019.05.015
136. De Filette J, Jansen Y, Schreuer M, Everaert H, Velkeniers B, Neyns B, et al. Incidence of thyroid-related adverse events in melanoma patients treated with pembrolizumab. J Clin Endocrinol Metab (2016) 101:4431–9. doi: 10.1210/jc.2016-2300
137. Zhou N, Velez MA, Bachrach B, Gukasyan J, Fares CM, Cummings AL, et al. Immune checkpoint inhibitor induced thyroid dysfunction is a frequent event post-treatment in NSCLC. Lung Cancer (2021) 161:34–41. doi: 10.1016/j.lungcan.2021.08.009
138. Chan KK, Bass AR. Autoimmune complications of immunotherapy: pathophysiology and management. The BMJ (2020) 369:m736. doi: 10.1136/bmj.m736
139. Lima Ferreira J, Costa C, Marques B, Castro S, Victor M, Oliveira J, et al. Improved survival in patients with thyroid function test abnormalities secondary to immune-checkpoint inhibitors. Cancer Immunology Immunotherapy (2021) 70:299–309. doi: 10.1007/s00262-020-02664-y
140. Kotwal A, Gustafson MP, Bornschlegl S, Kottschade L, Delivanis DA, Dietz AB, et al. Immune checkpoint inhibitor-induced thyroiditis is associated with increased intrathyroidal T lymphocyte subpopulations. Thyroid (2020) 30:1440–50. doi: 10.1089/thy.2020.0075
141. De Moel EC, Rozeman EA, Kapiteijn EH, Verdegaal EME, Grummels A, Bakker JA, et al. Autoantibody development under treatment with immune-checkpoint inhibitors. Cancer Immunol Res (2019) 7:6–11. doi: 10.1158/2326-6066.CIR-18-0245
142. Kurimoto C, Inaba H, Ariyasu H, Iwakura H, Ueda Y, Uraki S, et al. Predictive and sensitive biomarkers for thyroid dysfunctions during treatment with immune-checkpoint inhibitors. Cancer Sci (2020) 111:1468–77. doi: 10.1111/cas.14363
143. Caturegli P, de Remigis A, Rose NR. Hashimoto thyroiditis: Clinical and diagnostic criteria. Autoimmun Rev (2014) 13:391–7. doi: 10.1016/j.autrev.2014.01.007
144. Inaba H, Ariyasu H, Iwakura H, Kurimoto C, Takeshima K, Morita S, et al. Distinct clinical features and prognosis between persistent and temporary thyroid dysfunctions by immune-checkpoint inhibitors. Endocrine J (2021) 68:231–41. doi: 10.1507/endocrj.EJ20-0371
145. Kotwal A, Kottschade L, Ryder M. PD-L1 inhibitor-induced thyroiditis is associated with better overall survival in cancer patients. Thyroid (2020) 30:177–84. doi: 10.1089/thy.2019.0250
146. Goyal I, Pandey MR, Sharma R, Chaudhuri A, Dandona P. The side effects of immune checkpoint inhibitor therapy on the endocrine system. Indian J Med Res (2021) 154:559–70. doi: 10.4103/ijmr.IJMR_313_19
147. Al Mushref M, Guido PA, Collichio FA, Moore DT, Clemmons DR. Thyroid dysfunction, recovery, and prognosis in melanoma patients treated with immune checkpoint inhibitors: A retrospective review. Endocrine Pract (2020) 26:36–42. doi: 10.4158/EP-2019-0244
148. Shankar B, Zhang J, Naqash AR, Forde PM, Feliciano JL, Marrone KA, et al. Multisystem immune-related adverse events associated with immune checkpoint inhibitors for treatment of non-small cell lung cancer. JAMA Oncol (2020) 6:1952–6. doi: 10.1001/jamaoncol.2020.5012
149. Maher VE, Fernandes LL, Weinstock C, Tang S, Agarwal S, Brave M, et al. Analysis of the association between adverse events and outcome in patients receiving a programmed death protein 1 or programmed death ligand 1 antibody. J Clin Oncol (2019) 37:2730–7. doi: 10.1200/JCO.19.00318
150. Baek HS, Jeong C, Shin K, Lee J, Suh H, Lim DJ, et al. Association between the type of thyroid dysfunction induced by immune checkpoint inhibitors and prognosis in cancer patients. BMC Endocrine Disord (2022) 22:89. doi: 10.1186/s12902-022-01004-8
151. De Remigis A, de Gruijl TD, Uram JN, Tzou SC, Iwama S, Talor MV, et al. Development of thyroglobulin antibodies after GVAX immunotherapy is associated with prolonged survival. Int J Cancer (2015) 136:127–37. doi: 10.1002/ijc.28973
152. Itonaga T, Saito K, Ikeda N, Mikami R. Morphological changes of the thyroid gland as a new radiographic marker for lung cancer treatment efficacy of immune checkpoint inhibitors. Acta Radiologica (2021) 62:42–50. doi: 10.1177/0284185120916197
153. Barroso-Sousa R, Barry WT, Garrido-Castro AC, Hodi FS, Min L, Krop IE, et al. Incidence of endocrine dysfunction following the use of different immune checkpoint inhibitor regimens a systematic review and meta-analysis. JAMA Oncol (2018) 4:173–82. doi: 10.1001/jamaoncol.2017.3064
154. Angell TE, Min L, Wieczorek TJ, Hodi FS. Unique cytologic features of thyroiditis caused by immune checkpoint inhibitor therapy for malignant melanoma. Genes Dis (2018) 5:46–8. doi: 10.1016/j.gendis.2017.11.002
155. Lee H, Hodi FS, Giobbie-Hurder A, Ott PA, Buchbinder EI, Haq R, et al. Characterization of thyroid disorders in patients receiving immune checkpoint inhibition therapy. Cancer Immunol Res (2017) 5:1133–40. doi: 10.1158/2326-6066.CIR-17-0208
156. Yu C, Chopra IJ, Ha E. A novel melanoma therapy stirs up a storm: ipilimumab-induced thyrotoxicosis. Endocrinology Diabetes Metab Case Rep (2015) 2015:140092. doi: 10.1530/EDM-14-0092
157. McMillen B, Dhillon MS, Yong-Yow S. A rare case of thyroid storm. BMJ Case Rep (2016) 2016:bcr2016214603. doi: 10.1136/bcr-2016-214603
158. Osorio JC, Ni A, Chaft JE, Pollina R, Kasler MK, Stephens D, et al. Antibody-mediated thyroid dysfunction during T-cell checkpoint blockade in patients with non-small cell lung cancer. Ann Oncol (2017) 28(3):583–9. doi: 10.1093/annonc/mdw640
159. Brancatella A, Viola N, Brogioni S, Montanelli L, Sardella C, Vitti P, et al. Graves’ disease induced by immune checkpoint inhibitors: A case report and review of the literature. Eur Thyroid J (2019) 8:192–5. doi: 10.1159/000501824
160. Min L, Vaidya A, Becker C. Thyroid autoimmunity and ophthalmopathy related to melanoma biological therapy. Eur J Endocrinol (2011) 164:303–7. doi: 10.1530/EJE-10-0833
161. Campredon P, Imbert P, Mouly C, Grunenwald S, Mazières J, Caron P. Severe inflammatory ophthalmopathy in a euthyroid patient during nivolumab treatment. Eur Thyroid J (2018) 7:84–7. doi: 10.1159/000485742
162. Sagiv O, Kandl TJ, Thakar SD, Thuro BA, Busaidy NL, Cabanillas M, et al. Extraocular muscle enlargement and thyroid eye disease-like orbital inflammation associated with immune checkpoint inhibitor therapy in cancer patients. Ophthalmic Plast Reconstructive Surg (2019) 35:50–2. doi: 10.1097/IOP.0000000000001161
163. McElnea E, Ní Mhéalóid Á, Moran S, Kelly R, Fulcher T. Thyroid-like ophthalmopathy in a euthyroid patient receiving ipilimumab. Orbit (2014) 33:424–7. doi: 10.3109/01676830.2014.949792
164. Chen DP, Chu YC, Wen YH, Lin WT, Hour AL, Wang WT. Investigation of the correlation between graves’ ophthalmopathy and CTLA4 gene polymorphism. J Clin Med (2019) 8:1842. doi: 10.3390/jcm8111842
165. Wang H, Zhu LS, Cheng JW, Cai JP, Li Y, Ma XY, et al. Meta-analysis of association between the +49A/G polymorphism of cytotoxic T-lymphocyte antigen-4 and thyroid associated ophthalmopathy. Curr Eye Res (2015) 40:1195–203. doi: 10.3109/02713683.2014.993767
166. Ban Y, Davies TF, Greenberg DA, Kissin A, Marder B, Murphy B, et al. Analysis of the CTLA-4, CD28, and inducible costimulator (ICOS) genes in autoimmune thyroid disease. Genes Immun (2003) 4:586–93. doi: 10.1038/sj.gene.6364018
167. Kahaly GJ, Bartalena L, Hegedüs L, Leenhardt L, Poppe K, Pearce SH. European Thyroid association guideline for the management of graves’ hyperthyroidism. Eur Thyroid J (2018) 7:167–86. doi: 10.1159/000490384
168. Wiersinga WM. Graves’ disease: Can it be cured? Endocrinol Metab (2019) 34:29–38. doi: 10.3803/EnM.2019.34.1.29
169. Valdés-Corona LF, Hernández-Doño S, Rodríguez-Reyna TS, García-Silva R, Jakez J, Escamilla-Tilch M, et al. Aspartic acid70 in the HLA-DRB1 chain and shared epitope alleles partially explain the high prevalence of autoimmunity in mexicans. J Trans Autoimmun (2020) 3:100057. doi: 10.1016/j.jtauto.2020.100057
170. Badovinac S, Korsic M, Zarkovic K, Mursic D, Roglic M, Jakopovic M, et al. Nivolumab-induced synchronous occurrence of myositis and hypothyroidism in a patient with squamous cell lung cancer. Immunotherapy (2018) 10:427–31. doi: 10.2217/imt-2017-0174
171. Johnson ED, Kerrigan K, Butler K, Patel SB. Nivolumab-induced hypothyoidism with consequent hypothyroid related myopathy. J Oncol Pharm Pract (2020) 26(1):224–7. doi: 10.1177/1078155219835912
172. Khan U, Rizvi H, Sano D, Chiu J, Hadid T. Nivolumab induced myxedema crisis. J Immunotherapy Cancer (2017) 5:13. doi: 10.1186/s40425-017-0213-x
173. Chmiel KD, Suan D, Liddle C, Nankivell B, Ibrahim R, Bautista C, et al. Resolution of severe ipilimumab-induced hepatitis after antithymocyte globulin therapy case report. J Clin Oncol (2011) 29:237–40. doi: 10.1200/JCO.2010.32.2206
174. Illouz F, Briet C, Cloix L, le Corre Y, Baize N, Urban T, et al. Endocrine toxicity of immune checkpoint inhibitors: Essential crosstalk between endocrinologists and oncologists. Cancer Med (2017) 6:1923–9. doi: 10.1002/cam4.1145
175. Yoon JH, Ram Hong A, Kim HK, Kang HC. Characteristics of immune-related thyroid adverse events in patients treated with PD-1/PD-L1 inhibitors. Endocrinol Metab (2021) 36:413–23. doi: 10.3803/EnM.2020.906
176. Ma C, Hodi FS, Giobbie-Hurder A, Wang X, Zhou J, Zhang A, et al. The impact of high-dose glucocorticoids on the outcome of immune-checkpoint inhibitor–related thyroid disorders. Cancer Immunol Res (2019) 7:1214–20. doi: 10.1158/2326-6066.CIR-18-0613
177. Azmat U, Liebner D, Joehlin-Price A, Agrawal A, Nabhan F. Treatment of ipilimumab induced graves’ disease in a patient with metastatic melanoma. Case Rep Endocrinol (2016) 2016:2087525. doi: 10.1155/2016/2087525
178. Garber JR, Cobin RH, Gharib H, Hennessey JV, Klein I, Mechanick JI, et al. Clinical practice guidelines for hypothyroidism in adults: cosponsored by the American association of clinical endocrinologists and the American thyroid association. Endocrine Pract (2012) 18:988–1028. doi: 10.4158/EP12280.GL
Keywords: immune checkpoint inhibitors, cancer treatment, CTLA-4, PD-1, PD-L1, immune-related adverse events, endocrine, thyroid
Citation: Chera A, Stancu AL and Bucur O (2022) Thyroid-related adverse events induced by immune checkpoint inhibitors. Front. Endocrinol. 13:1010279. doi: 10.3389/fendo.2022.1010279
Received: 02 August 2022; Accepted: 29 August 2022;
Published: 20 September 2022.
Edited by:
Sadegh Rajabi, Shahid Beheshti University of Medical Sciences, IranReviewed by:
Guofang Chen, Nanjing University of Chinese Medicine, ChinaAnupam Kotwal, University of Nebraska Medical Center, United States
Copyright © 2022 Chera, Stancu and Bucur. This is an open-access article distributed under the terms of the Creative Commons Attribution License (CC BY). The use, distribution or reproduction in other forums is permitted, provided the original author(s) and the copyright owner(s) are credited and that the original publication in this journal is cited, in accordance with accepted academic practice. No use, distribution or reproduction is permitted which does not comply with these terms.
*Correspondence: Octavian Bucur, b2N0YXZpYW4uYnVjdXJAaXZiLnJv; b2N0YXZpYW4uYnVjdXJAZ21haWwuY29t