- Department of Medicine, Pituitary Center, Cedars-Sinai Medical Center, Los Angeles, CA, United States
Molecular therapeutic targets in growth hormone (GH)-secreting adenomas range from well-characterized surface receptors that recognize approved drugs, to surface and intracellular markers that are potential candidates for new drug development. Currently available medical therapies for patients with acromegaly bind to somatostatin receptors, GH receptor, or dopamine receptors, and lead to attainment of disease control in most patients. The degree of control is variable: however, correlates with both disease aggressiveness and tumor factors that predict treatment response including somatostatin receptor subtype expression, granulation pattern, kinases and their receptors, and other markers of proliferation. A better understanding of the mechanisms underlying these molecular markers and their relationship to outcomes holds promise for expanding treatment options as well as a more personalized approach to treating patients with acromegaly.
Introduction
Acromegaly is a disease of excess growth hormone (GH) and insulin-like growth factor (IGF)-1 that is most commonly caused by a pituitary somatotroph adenoma and is associated with increased mortality (1). The pathophysiology of acromegaly, like that of other pituitary disorders, is an evolving area of research, and particularly with regard to how it informs identification of molecular therapeutic targets (2). The underlying pathogenesis likely involves disruption of cell-cycle control, alteration of signaling pathways, genetic/epigenetic changes, and abnormal hormone production. Understanding mechanisms of currently known and exploited targets, as well as other theoretical targets, allows for an intriguing opportunity for a precision approach in treating acromegaly (3).
Current pharmacological options for patients with acromegaly include somatostatin receptor ligands (SRL), growth hormone receptor agonist, and dopamine agonists (DA). These agents are mostly well tolerated, but have adverse events such as injection site reactions or gastrointestinal distress, as well as psychological/social stressors that contribute to the treatment burden (4).
Precision medicine aims to improve patient outcomes through targeted treatment employing genetic, biomarker, phenotypic, or psychosocial characteristics unique to each patient or the disease process (5). Despite challenges inherent in precision medicine, namely complexity of disease classification and expanding library of biomarker, molecular profiling of acromegaly has the potential for determining optimal drug efficacy, predicting treatment response and prognosis, and management strategies that will achieve optimal outcomes.
Here, we describe immunohistochemical, cell surface, and intracellular factors that together may comprise a subcellular basis for determining a precision medicine approach to acromegaly management (Table 1).
Pathophysiology
Differentiation of GH-producing somatotroph cells in the anterior pituitary is determined by the PIT1 (POU1F1) transcription factor. Somatotroph adenomas are almost invariably sporadic, but familial tumors occur in very rare disorders such as MEN1, Carney complex, or X-LAG acrogigantism (6). A notable cause of familial isolated pituitary adenomas is germ-line mutation in the AIP gene (7).
Tumorigenesis involves dysregulation of cell proliferation and GH production through transcriptional, hormonal, and other growth stimulating factors. GH gene transcription and secretion is mediated by intracellular cAMP, and alterations in cAMP signaling leads to dysregulated GH production (4). Activating GNAS (guanine nucleotide binding protein, alpha stimulating activity polypeptide 1 gene) mutations, present in up to 40% of sporadic tumors, leads to constitutive cAMP activation and consequent excess GH production (8). However, classic oncogene mutations are not encountered in the pathogenesis of acromegaly.
Several other non-oncogenic factors play important pathogenic roles, including stimulatory signals from central and peripheral hormones, and disruption of proteins and kinases that regulate the cell cycle. Peripheral sex steroids and hypothalamic growth hormone releasing hormone (GHRH), if dysregulated, may induce GH production through constitutive cAMP production, as well as promote tumor cell proliferation. Disruption of cell cycle regulatory factors such as cyclin-dependent kinases (CDK), CDK inhibitors (e.g., p21, p27), and pituitary tumor–transforming protein (PTTG) also allow for pituitary tumor growth (4) (Figure 1).
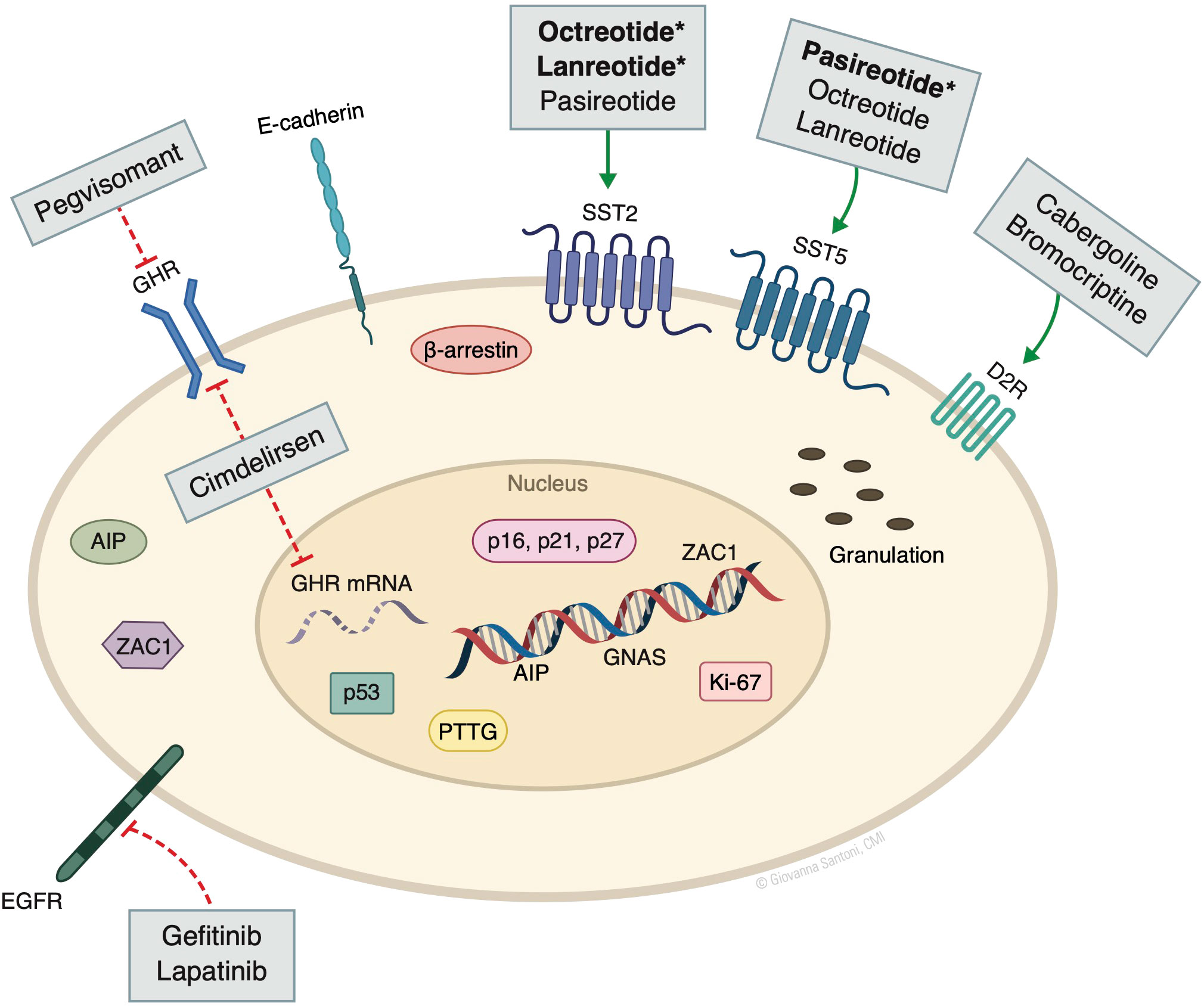
Figure 1 Molecular markers and targeted drugs. AIP, aryl hydrocarbon receptor-interacting protein; D2R, dopamine receptor subtype 2; GHR, growth hormone receptor; GNAS, guanine nucleotide binding protein, alpha stimulating activity polypeptide 1 gene. SST, somatostatin receptor; PTTG, pituitary tumor–transforming protein; ZAC1, zinc finger regulator of apoptosis and cell cycle arrest. Bolded* denotes the higher/predominant affinity of drug to receptor. The action of pegvisomant on GHR is mainly peripheral and not in the pituitary. © Giovanna Santoni, CMI. Used by permission.
Cell surface receptors for somatostatin, GHRH, and dopamine play a less direct role in pathogenesis, but are important mediators of stimulatory and inhibitory signals, and their balance is required to maintain normal GH secretory physiology.
Cell surface receptors as molecular targets
Somatostatin receptors
The hypothalamic-pituitary axis involves the interplay of tightly regulated hormone action through positive and negative signaling. Somatotroph GH production is attenuated by hypothalamic somatostatin, which suppresses timing and amplitude of GH secretory pulses by binding to somatostatin receptors (SST) (4), a family of G protein-coupled transmembrane receptors (GPCR) with five known subtypes (SST1-5), each with differing binding and hormone modulating capacity. SST2 and SST5 are most abundantly expressed receptors on somatotrophs and are the primary targets of adenoma-directed medical therapy (9, 10).
SST2 is expressed in brain, pituitary, stomach, kidney, and intestines (11). It signals to suppress mainly GH, and less compellingly thyroid-stimulating hormone (TSH), adrenocorticotrophin, and prolactin (12). The prevalent expression of SST2 in GH-secreting adenomas (9) makes it a prime target for treating acromegaly. The SRLs octreotide and lanreotide preferentially bind to SST2, thereby suppressing GH expression (1, 13). Disease control with these agents, however, can be variable with a reported mean response rate of approximately 55% (14).
SST5 is primarily expressed in the pituitary but is found as well in the spleen, intestines, and pancreas (15–17). SST5 regulates GH and TSH (12). Unlike with octreotide and lanreotide, the SRL pasireotide predominantly targets and binds with high affinity to SST5. It has shown to be more efficacious in patients resistant to other SRLs (18, 19), but it is associated with an increased risk of hyperglycemia, likely due to the role of SST5 in regulating glucose homeostasis through paracrine regulation of intestinal GLP-1 (20). Intuitively, lowering circulating GH also enhances insulin sensitivity thereby countering some of these hyperglycemic effects, but the risk remains and potentially limits its use.
Immunohistochemical expression of SST2 and SST5 may predict treatment outcomes, especially as expression is less abundant in more aggressive adenomas that are less prone to treatment responsiveness (21). For example, we showed that SRL treatment efficacy is typically high in patients with low or intermediate tumor aggressiveness, and these patients show concordant abundant SST2 expression. By contrast, SST2 expression is lower in patients with more aggressive and highly unresponsive tumors (21). Others have reported that response rate to SRL may be as high as 81% in the presence of SST2 expression compared with no response observed in the absence of SST2 expression (22, 23). Further, a higher SST5:SST2 ratio correlates with better response to pasireotide and resistance to octreotide/lanreotide (24–26). Of note, epigenetic factors, such as the natural antisense transcript SST5-AS, has been shown to alter SST5 expression, potentially influencing adenoma behavior and treatment response (27).
Although not routinely evaluated by clinical pathologists, SST expression may be a valuable molecular marker for precision-based acromegaly treatment, as decision for repeat surgery, radiotherapy, or choice of a specific SRL could be better guided with knowledge of SST2 and SST5 expression.
Growth hormone receptor
Human growth hormone receptor (GHR) is a transmembrane protein structurally related to a family of cytokine receptors (28, 29). Binding of GH leads to GHR dimerization and activation through the JAK/STAT pathway, leading to induction of IGF-1 (30). GHR is the target of the peripheral GHR antagonist pegvisomant, which is highly effective in treating acromegaly both as monotherapy and in combination with SRL (31, 32). A novel drug in development, Cimdelirsen (IONIS-GHR-LRx; ISIS 766720), which is an antisense molecule that acts by reducing GHR synthesis in the liver, has also shown promise in treating acromegaly (33). Polymorphisms in GHR, such as d3-GHR, has been studied in acromegaly but correlation with clinical features or therapeutic outcomes has not been consistent (34).
GHR expression is observed in normal somatotroph cells and to a lesser degree in somatotroph adenomas (35, 36). Pegvisomant may in part affect growth hormone production through direct action on GHR on somatotroph cells without impacting cell proliferation (37). However, the value of GHR as either a peripheral or central molecular marker for predicting treatment response is uncertain.
Dopamine receptors
Dopamine receptors (DR) comprise five GPCR subtypes numbered D1R through D5R and are present abundantly in the central nervous system, and peripherally in the pituitary, kidney, and vasculature (38, 39). D2R is present in two isoforms of equal activity and distribution, and is the predominant DR found in normal pituitary as well as in somatotroph and lactotroph adenomas (25).
The downstream cellular action of activated DR includes inhibition of adenylyl cyclase and activation of potassium channels, leading to inhibitory action of hypothalamic dopamine on prolactin production (40). In somatotroph adenomas, dopamine lowers GH production (41). This is likely the mechanism underlying the modest efficacy of DA monotherapy in the treatment of mild acromegaly (42) as expression of DR on somatotroph adenoma may have some value in predicting clinical response to SRL (25) yet serum prolactin level does not correlate with GH responses to cabergoline (42).The very limited value of using D2R as a therapeutic marker for acromegaly is limited to identifying patients with mild disease who may respond to DA as monotherapy or as an adjunct to SRL therapy.
Other cell-surface molecules
E-cadherin
E-cadherin is a transmembrane adhesion protein found on epithelial cells, and its loss is implicated in invasiveness and metastasis (43). Its expression has been suggested to be functional for somatotroph adenoma growth (44), but this association is modest, likely because other factors that promote cell senescence (45) prevent low expression of E-cadherin from causing transformation of pituitary adenomas to malignant tumors. Decreased E-cadherin expression in somatotroph tumors correlates with larger size, invasiveness, and response to SRL treatment (44). Sparsely granulated somatotroph adenomas, which are more likely to be aggressive (discussed below), also show lower E-cadherin expression compared to densely granulated tumors (46, 47). The association between low E-cadherin expression and poor SRL response seems independent of SST2 expression but may correlate inversely with SST5 expression (48). Such correlations between histological and cell surface markers provide an interesting intersection of molecular markers of tumor aggressiveness. However, direct relationships of these factors are not known, and they may simply represent the phenotypic components of more aggressive tumors.
Epidermal growth factor receptor (EGFR)
The EGFR family of tyrosine kinase receptors including EGFR (ErbB1 and HER1), p185Her2/neu (ErbB2 and HER2), ErbB3 (HER3) and ErbB4 (HER4), and their activating ligands including EGF and transforming growth factor-α are expressed in lacto-somatotroph cells and are potential targets for treatment (49). EGFR tyrosine kinase inhibitors (TKI) such as gefitinib, lapatinib, and canertinib have shown promising results in patients harboring aggressive corticotroph and lactotroph adenomas (50, 51). Although EGFR and EGF are abundantly expressed in somatotroph adenomas, trials evaluating TKI in treatment of aggressive acromegaly are lacking.
β-Arrestin
β-arrestin binds to GPCR and thereby regulates signaling (52). Results of studies on the role of β-arrestin in responsiveness of somatotroph adenomas to therapy has been mixed. In a study of 31 somatotroph adenomas, lower expression of β-arrestin-1 correlated with improved responsiveness to SRL (53), and a similarly sized study showed an inverse relationship between low β-arrestin-1/2 and SST2 expression, as well as a more favorable long-term response to SRL treatment (54). However, this relationship was not observed in another study of 40 patients, which showed no correlation of β-arrestin with SST2, SST5, or D2 expression, nor an association between β-arrestin and SRL response or tumor invasiveness (55). Therefore, the significance of β-arrestin as a molecular target for acromegaly remains unclear.
Granulation
Somatotroph adenomas are classified in two immunohistochemical subtypes - sparsely or densely granulated – with important clinical implications (56). The granulation pattern refers to the density of intracellular GH secretory granules as seen on electron microscopy, and is distinguished immunohistochemically by expression of cytokeratin expression, which is a cytoplasmic fibrous protein belonging to the family of intermediate filament proteins comprising the cytoskeleton of the cell (57, 58). As a dense granulation pattern is seen in normal somatotroph cells, densely granulated tumors more closely resemble nontumorous cells, while sparsely granulated tumors with scattered small secretory granules resemble poorly differentiated cells (59). The clinical outcomes associated with granulation pattern is generally consistent with outcomes associated with tumors harboring normal or poorly differentiated cells. Densely granulated adenomas are smaller at diagnosis, express higher levels of SST2, and are more responsive to treatment; therefore, these are less aggressive tumors. Conversely, sparsely granulated tumors are more likely to be aggressive: they are larger at diagnosis, show sparsity of SST2 expression, and are less responsive to treatment (21, 22).
Cell-cycle regulatory factors
Aryl hydrocarbon receptor-interacting protein (AIP)
Inactivating mutations of the tumor suppressor gene AIP in pituitary tumors is associated with familial acromegaly syndromes and is rarely seen in sporadic acromegaly (7). Familial acromegaly involving AIP mutation is more aggressive and less responsive to SRL (60). AIP expression has been associated with dense granulation (61), and inversely correlates with Ki-67 expression (62), suggesting a pro-proliferative state.
The mechanism of AIP mutation and tumorigenesis likely involves alterations in PDE4 phosphodiesterases as well as defective cAMP signaling. Specific PDE4 isoforms, PDE4A4 and PDE4A8, are under-expressed in normal pituitary and overexpressed in somatotroph adenomas, suggesting that disruptions of PDE4-AIP interaction play a role in tumorigenesis (63). Dysfunction in cAMP signaling through defective and decreased expression of Gai subunits, which help regulate AIP mediated cAMP signaling, also plays a key role (64). The mechanism of interplay between expression of AIP and cell-surface molecular receptors requires further study and may yield novel sub-cellular targets for treatment.
GNAS
Mutations in GNAS are present as somatic mutations in up to 40% of sporadic somatotroph adenomas and as mosaic mutations in McCune-Albright syndrome, a genetic disorder characterized by skin and bone manifestations as well as increased incidence of acromegaly (65). The impact of GNAS mutation on treatment response is uncertain. Though a meta-analysis showed a greater GH reduction in response to SRL treatment in GNAS mutations (66), a more recent study did not show a significant difference in SRL response and GNAS mutations (67). Pegvisomant is also an effective treatment option for acromegaly in patients with MAS (68); however, whether GNAS mutation influences treatment outcome between different available therapies is not known.
PTTG
PTTG is a homolog of securin proteins that prevent sister chromatin separation (69), and regulate the cell cycle through interaction with p53 (70). Over 70% of somatotroph adenomas overexpress PTTG, and this expression is an important component of cell senescence (71). PTTG expression may correlate with aggressiveness across different pituitary adenoma types (72), but it’s role as a molecular marker for clinical use in somatotroph adenoma characterization and targeted treatment warrants further investigation.
ZAC1
Zinc finger regulator of apoptosis and cell cycle arrest (ZAC1) is a tumor suppressor that attenuates cell proliferation (73). The antiproliferative property of SRL therapy may be mediated in part through increased ZAC1 gene expression (74), with evidence that suggests increased AIP expression is the linking mechanism (75). The lack of difference in ZAC1 expression between densely and sparsely granulated adenomas implies that ZAC1 does not play a significant role in tumor aggressiveness (57).
Ki-67
In the 2017 WHO Classification of Tumors of the Pituitary Gland, the term or classification of atypical adenoma, which was defined partly by Ki-67 proliferative index, was no longer recommended (76). However, Ki-67 may still hold value in predicting aggressiveness and treatment response in somatotroph adenomas.
Most non-aggressive adenomas show a Ki-67 proliferative index of <3% (21), and low Ki-67 expression has been associated with more favorable SRL response (67). Additionally, Ki-67 index correlates inversely with cavernous sinus invasion, surgical cure, and response to medical therapy (77). SRL treatment may also alter Ki-67 expression as evidenced by lower adenoma Ki-67 values observed in patients treated chronically with octreotide (78).
Cyclin-dependent kinase inhibitors
CDK and their inhibitors may serve as markers of somatotroph adenoma subtypes (4, 79). p21, a CDK inhibitor that plays a key role in cell senescence, is activated to maintain the benign nature of somatotroph adenomas (45, 71). Increased expression of p21 is associated with less aggressive somatotroph adenomas, likely exerting a dampening effect on cell proliferation (21). However, its impact in predicting curative success of surgery may be limited. In a series of 55 patients undergoing surgical adenoma resection, p21 overexpression did not correlate with biochemical remission after surgery (80), likely because the skill and volume of the surgeon, is a primary predictive factor for surgical success, more so than any one particular molecular factor (81).
The value of other CDK inhibitors such as p16 and p27 as molecular drivers in acromegaly are less certain. p16 expression is low or undetectable in all pituitary adenoma types and does not correlate with somatotroph tumor aggressiveness (21, 82). Low or absent p27 expression seems to play an especially important role in aggressive corticotroph adenomas as well as malignant pituitary tumors. Although p27 expression is also lower in somatotroph adenomas, its correlation to tumor aggressiveness or response in acromegaly is less clear (83). Despite limitations, CDK inhibitors may serve as compelling molecular predictors of tumor proliferative growth and as targets for development of novel drugs that target CDK pathways.
p53
p53-mediated tumor suppression in pituitary pathology acts through the p53/p21 senescence pathway (71). However, p53 is not reliable in predicting tumor aggressiveness. Somatotroph adenoma p53 overexpression has not shown significant association with tumor invasiveness or response to treatment (84–86).
Conclusion
The molecular profile of acromegaly affords an array of potential targets that may predict tumor aggressiveness or treatment response. Somatostatin receptors are the most well studied markers, and SST2 and SST5 are the targets of standard of care SRL treatment, and varying expression levels of these receptors also reliably predict response to specific types of SRL as well as overall resistance to treatment. The cell surface receptors GHR and DA also serve as targets for the highly effective GHR antagonist pegvisomant and for DA used primarily in an adjunctive treatment setting, respectively, but there is less evidence regarding their use as markers of tumor aggressiveness. Cell-surface molecules and cell-cycle regulatory factors involved in the complex interplay of cell signaling and cell cycle regulation in somatotroph tumors, including E-cadherin, CDK inhibitors, and EGFR TKIs, hold potential as targets for new therapies in the future.
Author contributions
AL conducted the initial literature review and prepared the first draft of the manuscript. All authors reviewed and approved the final version of the manuscript and made the decision to submit.
Funding
Supported by NIH grants R01DK113998 and T32DK007770, and by the Doris Factor Molecular Endocrinology Laboratory at Cedars-Sinai.
Conflict of interest
The authors declare that the research was conducted in the absence of any commercial or financial relationships that could be construed as a potential conflict of interest.
Publisher’s note
All claims expressed in this article are solely those of the authors and do not necessarily represent those of their affiliated organizations, or those of the publisher, the editors and the reviewers. Any product that may be evaluated in this article, or claim that may be made by its manufacturer, is not guaranteed or endorsed by the publisher.
References
1. Colao A, Grasso LFS, Giustina A, Melmed S, Chanson P, Pereira AM, et al. Acromegaly. Nat Rev Dis Primers (2019) 5(1):20. doi: 10.1038/s41572-019-0071-6
2. Melmed S. Pituitary-tumor endocrinopathies. N Engl J Med (2020) 382(10):937–50. doi: 10.1056/NEJMra1810772
3. Melmed S, Kaiser UB, Lopes MB, Bertherat J, Syro LV, Raverot G, et al. Clinical biology of the pituitary adenoma. Endocr Rev (2022). doi: 10.1210/endrev/bnac010
4. Melmed S. Acromegaly pathogenesis and treatment. J Clin Invest (2009) 119(11):3189–202. doi: 10.1172/JCI39375
5. Jameson JL, Longo DL. Precision medicine–personalized, problematic, and promising. N Engl J Med (2015) 372(23):2229–34. doi: 10.1056/NEJMsb1503104
6. Melmed S. Pituitary medicine from discovery to patient-focused outcomes. J Clin Endocrinol Metab (2016) 101(3):769–77. doi: 10.1210/jc.2015-3653
7. Vierimaa O, Georgitsi M, Lehtonen R, Vahteristo P, Kokko A, Raitila A, et al. Pituitary adenoma predisposition caused by germline mutations in the AIP gene. Science (2006) 312(5777):1228–30. doi: 10.1126/science.1126100
8. Vortmeyer AO, Gläsker S, Mehta GU, Abu-Asab MS, Smith JH, Zhuang Z, et al. Somatic GNAS mutation causes widespread and diffuse pituitary disease in acromegalic patients with McCune-albright syndrome. J Clin Endocrinol Metab (2012) 97(7):2404–13. doi: 10.1210/jc.2012-1274
9. Greenman Y, Melmed S. Heterogeneous expression of two somatostatin receptor subtypes in pituitary tumors. J Clin Endocrinol Metab (1994) 78(2):398–403. doi: 10.1210/jcem.78.2.8106629
10. Greenman Y, Melmed S. Expression of three somatostatin receptor subtypes in pituitary adenomas: evidence for preferential SSTR5 expression in the mammosomatotroph lineage. J Clin Endocrinol Metab (1994) 79(3):724–9. doi: 10.1210/jcem.79.3.7521350
11. Yamada Y, Post SR, Wang K, Tager HS, Bell GI, Seino S. Cloning and functional characterization of a family of human and mouse somatostatin receptors expressed in brain, gastrointestinal tract, and kidney. Proc Natl Acad Sci USA (1992) 89(1):251–5. doi: 10.1073/pnas.89.1.251
12. Shimon I, Taylor JE, Dong JZ, Bitonte RA, Kim S, Morgan B, et al. Somatostatin receptor subtype specificity in human fetal pituitary cultures. differential role of SSTR2 and SSTR5 for growth hormone, thyroid-stimulating hormone, and prolactin regulation. J Clin Invest (1997) 99(4):789–98. doi: 10.1172/JCI119225
13. Melmed S, Bronstein MD, Chanson P, Klibanski A, Casanueva FF, Wass JAH, et al. A consensus statement on acromegaly therapeutic outcomes. Nat Rev Endocrinol (2018) 14(9):552–61. doi: 10.1038/s41574-018-0058-5
14. Carmichael JD, Bonert VS, Nuño M, Ly D, Melmed S. Acromegaly clinical trial methodology impact on reported biochemical efficacy rates of somatostatin receptor ligand treatments: a meta-analysis. J Clin Endocrinol Metab (2014) 99(5):1825–33. doi: 10.1210/jc.2013-3757
15. Yamada Y, Kagimoto S, Kubota A, Yasuda K, Masuda K, Someya Y, et al. Cloning, functional expression and pharmacological characterization of a fourth (hSSTR4) and a fifth (hSSTR5) human somatostatin receptor subtype. Biochem Biophys Res Commun (1993) 195(2):844–52. doi: 10.1006/bbrc.1993.2122
16. Bruno JF, Xu Y, Song J, Berelowitz M. Tissue distribution of somatostatin receptor subtype messenger ribonucleic acid in the rat. Endocrinology (1993) 133(6):2561–7. doi: 10.1210/endo.133.6.8243278
17. Portela-Gomes GM, Stridsberg M, Grimelius L, Oberg K, Janson ET. Expression of the five different somatostatin receptor subtypes in endocrine cells of the pancreas. Appl Immunohistochem Mol Morphol (2000) 8(2):126–32. doi: 10.1097/00129039-200006000-00007
18. Lesche S, Lehmann D, Nagel F, Schmid HA, Schulz S. Differential effects of octreotide and pasireotide on somatostatin receptor internalization and trafficking in vitro. J Clin Endocrinol Metab (2009) 94(2):654–61. doi: 10.1210/jc.2008-1919
19. Gadelha MR, Bronstein MD, Brue T, Coculescu M, Fleseriu M, Guitelman M, et al. Pasireotide versus continued treatment with octreotide or lanreotide in patients with inadequately controlled acromegaly (PAOLA): a randomised, phase 3 trial. Lancet Diabetes Endocrinol (2014) 2(11):875–84. doi: 10.1016/S2213-8587(14)70169-X
20. Jepsen SL, Albrechtsen NJW, Windeløv JA, Galsgaard KD, Hunt JE, Farb TB, et al. Antagonizing somatostatin receptor subtype 2 and 5 reduces blood glucose in a gut- and GLP-1R-dependent manner. JCI Insight (2021) 6(4). doi: 10.1172/jci.insight.143228
21. Cuevas-Ramos D, Carmichael JD, Cooper O, Bonert VS, Gertych A, Mamelak AN, et al. A structural and functional acromegaly classification. J Clin Endocrinol Metab (2015) 100(1):122–31. doi: 10.1210/jc.2014-2468
22. Brzana J, Yedinak CG, Gultekin SH, Delashaw JB, Fleseriu M. Growth hormone granulation pattern and somatostatin receptor subtype 2A correlate with postoperative somatostatin receptor ligand response in acromegaly: a large single center experience. Pituitary (2013) 16(4):490–8. doi: 10.1007/s11102-012-0445-1
23. Plöckinger U, Albrecht S, Mawrin C, Saeger W, Buchfelder M, Petersenn S, et al. Selective loss of somatostatin receptor 2 in octreotide-resistant growth hormone-secreting adenomas. J Clin Endocrinol Metab (2008) 93(4):1203–10. doi: 10.1210/jc.2007-1986
24. Gatto F, Feelders RA, Franck SE, van Koetsveld PM, Dogan F, Kros JM, et al. In vitro head-to-Head comparison between octreotide and pasireotide in GH-secreting pituitary adenomas. J Clin Endocrinol Metab (2017) 102(6):2009–18. doi: 10.1210/jc.2017-00135
25. Neto LV, EeO M, RM L, GF T, JB M, LM C, et al. Expression analysis of dopamine receptor subtypes in normal human pituitaries, nonfunctioning pituitary adenomas and somatotropinomas, and the association between dopamine and somatostatin receptors with clinical response to octreotide-LAR in acromegaly. J Clin Endocrinol Metab (2009) 94(6):1931–7. doi: 10.1210/jc.2008-1826
26. Iacovazzo D, Carlsen E, Lugli F, Chiloiro S, Piacentini S, Bianchi A, et al. Factors predicting pasireotide responsiveness in somatotroph pituitary adenomas resistant to first-generation somatostatin analogues: an immunohistochemical study. Eur J Endocrinol (2016) 174(2):241–50. doi: 10.1530/EJE-15-0832
27. Pedraza-Arevalo S, Ibáñez-Costa A, Blázquez-Encinas R, Branco MR, Vázquez-Borrego MC, Herrera-Martínez AD, et al. Epigenetic and post-transcriptional regulation of somatostatin receptor subtype 5 (SST. Mol Oncol (2022) 16(3):764–79. doi: 10.1002/1878-0261.13107
28. Leung DW, Spencer SA, Cachianes G, Hammonds RG, Collins C, Henzel WJ, et al. Growth hormone receptor and serum binding protein: purification, cloning and expression. Nature (1987) 330(6148):537–43. doi: 10.1038/330537a0
29. Bass SH, Mulkerrin MG, Wells JA. A systematic mutational analysis of hormone-binding determinants in the human growth hormone receptor. Proc Natl Acad Sci U S A (1991) 88(10):4498–502. doi: 10.1073/pnas.88.10.4498
30. Cunningham BC, Ultsch M, De Vos AM, Mulkerrin MG, Clauser KR, Wells JA. Dimerization of the extracellular domain of the human growth hormone receptor by a single hormone molecule. Science (1991) 254(5033):821–5. doi: 10.1126/science.1948064
31. van der Lely AJ, Hutson RK, Trainer PJ, Besser GM, Barkan AL, Katznelson L, et al. Long-term treatment of acromegaly with pegvisomant, a growth hormone receptor antagonist. Lancet (2001) 358(9295):1754–9. doi: 10.1016/S0140-6736(01)06844-1
32. Bonert V, Mirocha J, Carmichael J, Yuen KCJ, Araki T, Melmed S. Cost-effectiveness and efficacy of a novel combination regimen in acromegaly: A prospective, randomized trial. J Clin Endocrinol Metab (2020) 105(9):e3236–e3245. doi: 10.1210/clinem/dgaa444
33. Bhanot S, Fleseriu M, Geary R, Hu K, Li L, Melmed S, et al. OR27-4 placebo-controlled and open-label extension study of a novel hepatic-targeted antisense cimdelirsen (IONIS-GHR-LRx) under investigation in acromegaly patients. J Endocr Soc (2022) 6(Supplement_1):A526. doi: 10.1210/jendso/bvac150.1095
34. Boguszewski CL, Barbosa EJL, Svensson PA, Johannsson G, Glad CAM. MECHANISMS IN ENDOCRINOLOGY: Clinical and pharmacogenetic aspects of the growth hormone receptor polymorphism. Eur J Endocrinol (2017) 177(6):R309–R21. doi: 10.1530/EJE-17-0549
35. Mertani HC, Pechoux C, Garcia-Caballero T, Waters MJ, Morel G. Cellular localization of the growth hormone receptor/binding protein in the human anterior pituitary gland. J Clin Endocrinol Metab (1995) 80(11):3361–7. doi: 10.1210/jcem.80.11.7593452
36. Kola B, Korbonits M, Diaz-Cano S, Kaltsas G, Morris DG, Jordan S, et al. Reduced expression of the growth hormone and type 1 insulin-like growth factor receptors in human somatotroph tumours and an analysis of possible mutations of the growth hormone receptor. Clin Endocrinol (Oxf) (2003) 59(3):328–38. doi: 10.1046/j.1365-2265.2003.01851.x
37. Cuny T, Zeiller C, Bidlingmaier M, Défilles C, Roche C, Blanchard MP, et al. In vitro impact of pegvisomant on growth hormone-secreting pituitary adenoma cells. Endocr Relat Cancer (2016) 23(7):509–19. doi: 10.1530/ERC-16-0140
38. Missale C, Nash SR, Robinson SW, Jaber M, Caron MG. Dopamine receptors: from structure to function. Physiol Rev (1998) 78(1):189–225. doi: 10.1152/physrev.1998.78.1.189
39. Weiner DM, Levey AI, Sunahara RK, Niznik HB, O'Dowd BF, Seeman P, et al. D1 and D2 dopamine receptor mRNA in rat brain. Proc Natl Acad Sci U S A (1991) 88(5):1859–63. doi: 10.1073/pnas.88.5.1859
40. Ben-Shlomo A, Liu NA, Melmed S. Somatostatin and dopamine receptor regulation of pituitary somatotroph adenomas. Pituitary (2017) 20(1):93–9. doi: 10.1007/s11102-016-0778-2
41. Miell JP, Corder R, Pralong FP, Gaillard RC. Effects of dexamethasone on growth hormone (GH)-releasing hormone, arginine- and dopaminergic stimulated GH secretion, and total plasma insulin-like growth factor-I concentrations in normal male volunteers. J Clin Endocrinol Metab (1991) 72(3):675–81. doi: 10.1210/jcem-72-3-675
42. Sandret L, Maison P, Chanson P. Place of cabergoline in acromegaly: a meta-analysis. J Clin Endocrinol Metab (2011) 96(5):1327–35. doi: 10.1210/jc.2010-2443
43. Halbleib JM, Nelson WJ. Cadherins in development: cell adhesion, sorting, and tissue morphogenesis. Genes Dev (2006) 20(23):3199–214. doi: 10.1101/gad.1486806
44. Fougner SL, Lekva T, Borota OC, Hald JK, Bollerslev J, Berg JP. The expression of e-cadherin in somatotroph pituitary adenomas is related to tumor size, invasiveness, and somatostatin analog response. J Clin Endocrinol Metab (2010) 95(5):2334–42. doi: 10.1210/jc.2009-2197
45. Chesnokova V, Zhou C, Ben-Shlomo A, Zonis S, Tani Y, Ren SG, et al. Growth hormone is a cellular senescence target in pituitary and nonpituitary cells. Proc Natl Acad Sci U S A (2013) 110(35):E3331–9. doi: 10.1073/pnas.1310589110
46. Fougner SL, Casar-Borota O, Heck A, Berg JP, Bollerslev J. Adenoma granulation pattern correlates with clinical variables and effect of somatostatin analogue treatment in a large series of patients with acromegaly. Clin Endocrinol (Oxf) (2012) 76(1):96–102. doi: 10.1111/j.1365-2265.2011.04163.x
47. Soukup J, Hornychova H, Manethova M, Michalova K, Michnova L, Popovska L, et al. Predictive and prognostic significance of tumour subtype, SSTR1-5 and e-cadherin expression in a well-defined cohort of patients with acromegaly. J Cell Mol Med (2021) 25(5):2484–92. doi: 10.1111/jcmm.16173
48. Venegas-Moreno E, Flores-Martinez A, Dios E, Vazquez-Borrego MC, Ibañez-Costa A, Madrazo-Atutxa A, et al. E-cadherin expression is associated with somatostatin analogue response in acromegaly. J Cell Mol Med (2019) 23(5):3088–96. doi: 10.1111/jcmm.13851
49. Cooper O, Vlotides G, Fukuoka H, Greene MI, Melmed S. Expression and function of ErbB receptors and ligands in the pituitary. Endocr Relat Cancer (2011) 18(6):R197–211. doi: 10.1530/ERC-11-0066
50. Ben-Shlomo A, Cooper O. Role of tyrosine kinase inhibitors in the treatment of pituitary tumours: from bench to bedside. Curr Opin Endocrinol Diabetes Obes (2017) 24(4):301–5. doi: 10.1097/MED.0000000000000344
51. Cooper O, Bonert VS, Rudnick J, Pressman BD, Lo J, Salvatori R, et al. EGFR/ErbB2-targeting lapatinib therapy for aggressive prolactinomas. J Clin Endocrinol Metab (2021) 106(2):e917–e25. doi: 10.1210/clinem/dgaa805
52. Luttrell LM, Gesty-Palmer D. Beyond desensitization: physiological relevance of arrestin-dependent signaling. Pharmacol Rev (2010) 62(2):305–30. doi: 10.1124/pr.109.002436
53. Gatto F, Feelders R, van der Pas R, Kros JM, Dogan F, van Koetsveld PM, et al. β-arrestin 1 and 2 and G protein-coupled receptor kinase 2 expression in pituitary adenomas: role in the regulation of response to somatostatin analogue treatment in patients with acromegaly. Endocrinology (2013) 154(12):4715–25. doi: 10.1210/en.2013-1672
54. Gatto F, Biermasz NR, Feelders RA, Kros JM, Dogan F, van der Lely AJ, et al. Low beta-arrestin expression correlates with the responsiveness to long-term somatostatin analog treatment in acromegaly. Eur J Endocrinol (2016) 174(5):651–62. doi: 10.1530/EJE-15-0391
55. Coelho MCA, Vasquez ML, Wildemberg LE, Vázquez-Borrego MC, Bitana L, Camacho AHDS, et al. Molecular evidence and clinical importance of β-arrestins expression in patients with acromegaly. J Cell Mol Med (2018) 22(4):2110–6. doi: 10.1111/jcmm.13427
56. Melmed S, Braunstein GD, Horvath E, Ezrin C, Kovacs K. Pathophysiology of acromegaly. Endocr Rev (1983) 4(3):271–90. doi: 10.1210/edrv-4-3-271
57. Mayr B, Buslei R, Theodoropoulou M, Stalla GK, Buchfelder M, Schöfl C. Molecular and functional properties of densely and sparsely granulated GH-producing pituitary adenomas. Eur J Endocrinol (2013) 169(4):391–400. doi: 10.1530/EJE-13-0134
58. Bakhtiar Y, Hirano H, Arita K, Yunoue S, Fujio S, Tominaga A, et al. Relationship between cytokeratin staining patterns and clinico-pathological features in somatotropinomae. Eur J Endocrinol (2010) 163(4):531–9. doi: 10.1530/EJE-10-0586
59. Larkin S, Reddy R, Karavitaki N, Cudlip S, Wass J, Ansorge O. Granulation pattern, but not GSP or GHR mutation, is associated with clinical characteristics in somatostatin-naive patients with somatotroph adenomas. Eur J Endocrinol (2013) 168(4):491–9. doi: 10.1530/EJE-12-0864
60. Daly AF, Vanbellinghen JF, Khoo SK, Jaffrain-Rea ML, Naves LA, Guitelman MA, et al. Aryl hydrocarbon receptor-interacting protein gene mutations in familial isolated pituitary adenomas: analysis in 73 families. J Clin Endocrinol Metab (2007) 92(5):1891–6. doi: 10.1210/jc.2006-2513
61. Daly AF, Cano DA, Venegas-Moreno E, Petrossians P, Dios E, Castermans E, et al. AIP and MEN1 mutations and AIP immunohistochemistry in pituitary adenomas in a tertiary referral center. Endocr Connect (2019) 8(4):338–48. doi: 10.1530/EC-19-0027
62. Jaffrain-Rea ML, Rotondi S, Turchi A, Occhi G, Barlier A, Peverelli E, et al. Somatostatin analogues increase AIP expression in somatotropinomas, irrespective of gsp mutations. Endocr Relat Cancer (2013) 20(5):753–66. doi: 10.1530/ERC-12-0322
63. Bolger GB, Bizzi MF, Pinheiro SV, Trivellin G, Smoot L, Accavitti MA, et al. cAMP-specific PDE4 phosphodiesterases and AIP in the pathogenesis of pituitary tumors. Endocr Relat Cancer (2016) 23(5):419–31. doi: 10.1530/ERC-15-0205
64. Tuominen I, Heliövaara E, Raitila A, Rautiainen MR, Mehine M, Katainen R, et al. AIP inactivation leads to pituitary tumorigenesis through defective gαi-cAMP signaling. Oncogene (2015) 34(9):1174–84. doi: 10.1038/onc.2014.50
65. Coopmans EC, Korbonits M. Molecular genetic testing in the management of pituitary disease. Clin Endocrinol (Oxf) (2022) 97(4):424–35. doi: 10.1111/cen.14706
66. Efstathiadou ZA, Bargiota A, Chrisoulidou A, Kanakis G, Papanastasiou L, Theodoropoulou A, et al. Impact of gsp mutations in somatotroph pituitary adenomas on growth hormone response to somatostatin analogs: a meta-analysis. Pituitary (2015) 18(6):861–7. doi: 10.1007/s11102-015-0662-5
67. Puig-Domingo M, Gil J, Sampedro-Nuñez M, Jordà M, Webb SM, Serra G, et al. Molecular profiling for acromegaly treatment: a validation study. Endocr Relat Cancer (2020) 27(6):375–89. doi: 10.1530/ERC-18-0565
68. Akintoye SO, Kelly MH, Brillante B, Cherman N, Turner S, Butman JA, et al. Pegvisomant for the treatment of gsp-mediated growth hormone excess in patients with McCune-albright syndrome. J Clin Endocrinol Metab (2006) 91(8):2960–6. doi: 10.1210/jc.2005-2661
69. Zou H, McGarry TJ, Bernal T, Kirschner MW. Identification of a vertebrate sister-chromatid separation inhibitor involved in transformation and tumorigenesis. Science (1999) 285(5426):418–22. doi: 10.1126/science.285.5426.418
70. Bernal JA, Luna R, Espina A, Lázaro I, Ramos-Morales F, Romero F, et al. Human securin interacts with p53 and modulates p53-mediated transcriptional activity and apoptosis. Nat Genet (2002) 32(2):306–11. doi: 10.1038/ng997
71. Chesnokova V, Zonis S, Kovacs K, Ben-Shlomo A, Wawrowsky K, Bannykh S, et al. p21(Cip1) restrains pituitary tumor growth. Proc Natl Acad Sci U S A (2008) 105(45):17498–503. doi: 10.1073/pnas.0804810105
72. Filippella M, Galland F, Kujas M, Young J, Faggiano A, Lombardi G, et al. Pituitary tumour transforming gene (PTTG) expression correlates with the proliferative activity and recurrence status of pituitary adenomas: a clinical and immunohistochemical study. Clin Endocrinol (Oxf) (2006) 65(4):536–43. doi: 10.1111/j.1365-2265.2006.02630.x
73. Spengler D, Villalba M, Hoffmann A, Pantaloni C, Houssami S, Bockaert J, et al. Regulation of apoptosis and cell cycle arrest by Zac1, a novel zinc finger protein expressed in the pituitary gland and the brain. EMBO J (1997) 16(10):2814–25. doi: 10.1093/emboj/16.10.2814
74. Theodoropoulou M, Zhang J, Laupheimer S, Paez-Pereda M, Erneux C, Florio T, et al. Octreotide, a somatostatin analogue, mediates its antiproliferative action in pituitary tumor cells by altering phosphatidylinositol 3-kinase signaling and inducing Zac1 expression. Cancer Res (2006) 66(3):1576–82. doi: 10.1158/0008-5472.CAN-05-1189
75. Chahal HS, Trivellin G, Leontiou CA, Alband N, Fowkes RC, Tahir A, et al. Somatostatin analogs modulate AIP in somatotroph adenomas: the role of the ZAC1 pathway. J Clin Endocrinol Metab (2012) 97(8):E1411–20. doi: 10.1210/jc.2012-1111
76. Lloyd RV, Osamura RY, Klöppel G, Rosai J eds. WHO classification of tumours of endocrine organs. 4th ed. Lyon: IARC Press (2017).
77. Fusco A, Zatelli MC, Bianchi A, Cimino V, Tilaro L, Veltri F, et al. Prognostic significance of the ki-67 labeling index in growth hormone-secreting pituitary adenomas. J Clin Endocrinol Metab (2008) 93(7):2746–50. doi: 10.1210/jc.2008-0126
78. Losa M, Ciccarelli E, Mortini P, Barzaghi R, Gaia D, Faccani G, et al. Effects of octreotide treatment on the proliferation and apoptotic index of GH-secreting pituitary adenomas. J Clin Endocrinol Metab (2001) 86(11):5194–200. doi: 10.1210/jcem.86.11.7986
79. Garcia-Rendueles AR, Chenlo M, Oroz-Gonjar F, Solomou A, Mistry A, Barry S, et al. RET signalling provides tumorigenic mechanism and tissue specificity for AIP-related somatotrophinomas. Oncogene (2021) 40(45):6354–68. doi: 10.1038/s41388-021-02009-8
80. Babu H, Ortega A, Nuno M, Dehghan A, Schweitzer A, Bonert HV, et al. Long-term endocrine outcomes following endoscopic endonasal transsphenoidal surgery for acromegaly and associated prognostic factors. Neurosurgery (2017) 81(2):357–66. doi: 10.1093/neuros/nyx020
81. Barker FG, Klibanski A, Swearingen B. Transsphenoidal surgery for pituitary tumors in the united states, 1996-2000: mortality, morbidity, and the effects of hospital and surgeon volume. J Clin Endocrinol Metab (2003) 88(10):4709–19. doi: 10.1210/jc.2003-030461
82. Seemann N, Kuhn D, Wrocklage C, Keyvani K, Hackl W, Buchfelder M, et al. CDKN2A/p16 inactivation is related to pituitary adenoma type and size. J Pathol (2001) 193(4):491–7. doi: 10.1002/path.833
83. Lidhar K, Korbonits M, Jordan S, Khalimova Z, Kaltsas G, Lu X, et al. Low expression of the cell cycle inhibitor p27Kip1 in normal corticotroph cells, corticotroph tumors, and malignant pituitary tumors. J Clin Endocrinol Metab (1999) 84(10):3823–30. doi: 10.1210/jcem.84.10.6066
84. Sarkar S, Chacko AG, Chacko G. An analysis of granulation patterns, MIB-1 proliferation indices and p53 expression in 101 patients with acromegaly. Acta Neurochir (Wien) (2014) 156(12):2221–30. doi: 10.1007/s00701-014-2230-6
85. Gejman R, Swearingen B, Hedley-Whyte ET. Role of ki-67 proliferation index and p53 expression in predicting progression of pituitary adenomas. Hum Pathol (2008) 39(5):758–66. doi: 10.1016/j.humpath.2007.10.004
Keywords: somatostatin receptor, epidermal growth factor receptor, tyrosine kinase inhibitor, cyclin-dependent kinase, granulation
Citation: Labadzhyan A and Melmed S (2022) Molecular targets in acromegaly. Front. Endocrinol. 13:1068061. doi: 10.3389/fendo.2022.1068061
Received: 12 October 2022; Accepted: 18 November 2022;
Published: 05 December 2022.
Edited by:
Adriana G. Ioachimescu, School of Medicine, Emory University, United StatesReviewed by:
David Clemmons, University of North Carolina at Chapel Hill, United StatesDaniel Cuevas-Ramos, Instituto Nacional de Ciencias Médicas y Nutrición Salvador Zubirán (INCMNSZ), Mexico
Copyright © 2022 Labadzhyan and Melmed. This is an open-access article distributed under the terms of the Creative Commons Attribution License (CC BY). The use, distribution or reproduction in other forums is permitted, provided the original author(s) and the copyright owner(s) are credited and that the original publication in this journal is cited, in accordance with accepted academic practice. No use, distribution or reproduction is permitted which does not comply with these terms.
*Correspondence: Artak Labadzhyan, QXJ0YWsuTGFiYWR6aHlhbkBjc2hzLm9yZw==