- 1Department of Epidemiology & Data Science, Amsterdam University Medical Centre (UMC), University of Amsterdam, Amsterdam, Netherlands
- 2Department of Reproductive Medicine, Amsterdam University Medical Centre (UMC), Vrije Universiteit Amsterdam, Amsterdam, Netherlands
- 3Amsterdam Reproduction & Development Research Institute, Amsterdam, Netherlands
- 4Department of Paediatric Endocrinology, Amsterdam University Medical Centre (UMC), Vrije Universiteit Amsterdam, Emma Children’s Hospital, Amsterdam, Netherlands
- 5Department of Medical Informatics, Amsterdam University Medical Centre (UMC), University of Amsterdam, Amsterdam, Netherlands
- 6Department of Obstetrics & Gynaecology, Amsterdam University Medical Centre (UMC), University of Amsterdam, Amsterdam, Netherlands
Background: Early-life exposures during gestation may permanently alter thyroid physiology and health in adulthood. We investigated whether exposure to the Dutch Famine (1944-1945) in late, mid, or early gestation influences thyroid function (i.e., incidence of thyroid disease, thyroid autoantibodies, thyroid stimulating hormone (TSH), and free thyroxine (FT4) levels) in adulthood. We specifically assessed whether potential effects of famine differed for men and women.
Methods: This study includes 910 men and women born as term singletons in the Wilhelmina Gasthuis in Amsterdam, the Netherlands, shortly before, during, or after the Dutch Famine. We evaluated medical histories for previous diagnosis or current treatment for thyroid dysfunction. At age 50 blood samples were drawn from 728 individuals for tests of thyroid function. We studied the prevalence of overt hypo- and hyperthyroidism and thyroid autoimmunity using medical histories, and measurements of TSH, FT4, anti-TPO and anti-TG, comparing participants exposed to famine at different pregnancy trimesters or born before or conceived after the famine. Additionally, we studied associations of TSH and FT4 levels with in utero famine exposure in a subsample of men and women free of thyroid disease that were exposed in late, mid, or early gestation.
Results: There were no differences in thyroid dysfunction diagnosis or current treatment between participants at age 50 years who been exposed to famine during different periods of gestation and those born before or conceived after. There was no association between famine exposure and overt hypo- or hyperthyroidism or thyroid autoantibody positivity. Women who had been exposed to famine in mid gestation had slightly lower TSH levels than women who had not been exposed to famine prenatally (b=-0.06; 95%; CI=[-0.11,-0.02]; p<0.01). No differences in TSH levels were observed in men, and no differences in FT4 levels were observed in men or women.
Conclusions: There are no differences in adult thyroid disease at age 50 years according to prenatal famine exposure. However, the lower TSH levels in women exposed to famine in the second trimester suggest that there may be sex-specific effects of famine exposure during a critical period of thyroid development on hypothalamic-pituitary-thyroid axis regulation in adulthood.
Introduction
Thyroid disease is among the most common endocrine disorders, yet little is known about the effect of adverse conditions during early development on thyroid function regulation in adulthood (1). Thyroid dysfunction groups with obesity-related non-communicable diseases such as cardiovascular disease, type 2 diabetes mellitus, and polycystic ovary syndrome (2–4). Slightly elevated TSH levels are frequently found in individuals with metabolic syndrome, which refers to the clustering of visceral adiposity, insulin resistance, hypertension, and dyslipidaemia (5, 6). These metabolic conditions have all previously been associated with prenatal undernutrition or foetal growth restriction followed by affluence (7, 8). The framework of Development Origins of Health and Disease (DOHaD) offers the perspective that there are critical periods of development when physiological systems are highly flexible and environmental conditions play an important role in the long-term programming of human health (9–11). During this window of developmental plasticity endocrine, nutritional, and stress-related cues may alter an individual’s physiological make-up and affect the epigenetic programming of key metabolic pathways (9, 11). This might include the hypothalamic-pituitary-thyroid (HPT) axis, which through a negative feedback loop via the anterior pituitary’s release of thyroid stimulating hormone (TSH), regulates the production of thyroid hormones that contribute to the regulation of metabolism, reproduction, and growth (1). Yet, the importance of timing of severe malnutrition at different pregnancy trimesters on adult thyroid function has not been studied previously in humans, despite possible implications for the occurrence of metabolic syndrome.
Research from the Netherlands Twin Register suggests that between 22-27% of variance in thyroxine (T4) in neonates is due to shared environment in the womb and a further 33-47% due to environmental factors (12). This suggests a role for foetal environment in the development of HPT axis setpoints (12). The thyroid becomes active after the 12th week of gestation (1), and during the second trimester of gestation undergoes a large increase in size (13). Famine exposure during critical development could disrupt these processes, leading to slightly altered thyroid physiology in later life. Studies in rats have found that undernutrition during gestation starting at the day of mating predisposed towards a pattern of elevated TSH and lower plasma free T4 in adulthood (14). Conversely, a study in sheep found that undernutrition in the last six weeks of pregnancy followed by overnutrition postnatally predisposed towards adult hyperthyroidism (15). Similarly, in rats protein-restricted maternal diets during lactation led to lower TSH levels and hyperthyroidism in adult offspring (16). In humans exposed to the Chinese famine of 1959-1961, one study found that individuals exposed in utero had significantly higher TSH levels (within the normal range) in adulthood, in contrast to those born before or after the famine (17). Another study on individuals exposed to the Chinese famine in early development did not find altered thyroid function in individuals exposed in utero, instead finding lower free T4 and higher TSH levels in adults postnatally exposed to the famine (18). Overall, these studies suggests that exposure to famine during early life may affect thyroid function depending on the timing of the exposure; specifically, studies on the Chinese famine suggest that exposure to famine conditions from early pregnancy onwards may be associated with a subtle downregulation of the HPT axis and higher TSH levels in adulthood (17, 18). Amongst famine-exposed Chinese, there was not a higher incidence of overt thyroid dysfunction (17, 18), suggesting that in humans the overall effect of undernutrition in early life on thyroid function regulation is subtle.
In contrast to the Chinese famine, which lasted between 1959 and 1961 (17, 18), the Dutch famine of 1944-1945 lasted for a shorter period of time, had an abrupt beginning and end in an otherwise affluent and well-nourished population (19). Because of its well-defined exposure periods and the conservation of the original birth records of the main maternity hospital in Amsterdam (19), the Dutch Famine Birth Cohort (DFBC) offers a unique opportunity to consider whether timing of famine exposure during different pregnancy trimesters may lead to differences in adult thyroid function. The DFBC is a prospective birth cohort that looks at the health of individuals born shortly before, during, or after the Dutch Famine of 1944-1945, also known as the ‘Hunger Winter’, in the Wilhelmina Gasthuis in Amsterdam (19). During this period, a ban of food transport into the occupied Western region of the Netherlands, as a retaliation against the local population for a railroad strike in support of the Allied forces, led to an acute famine, which in combination with a harsh winter resulted in extreme nutritional deprivation in an otherwise affluent population (19, 20). Daily food rations, which were recorded in detail since 1941, declined to only 500-600 calories a day, which abruptly ended when the Netherlands was liberated on the 5th of May 1945 (19, 20). Nutritional status afterwards rapidly improved and by June 1945 food rations included 2,130 calories a day (21). Thus, the events of the 1944-1945 winter offer a historic opportunity, as a time-limited, well characterised ‘natural experiment’ to understand the long-term effects of undernutrition during early development followed by more prosperous conditions during child- and adulthood in post-World War Netherlands on thyroid (dys)function in later life. Making use of thyroid function tests at age 50 in combination with extensive medical histories, the DFBC offers the opportunity to further explore the long-term effect of the timing of prenatal famine exposure during pregnancy on the programming of the HPT axis. This study aims to investigate whether overt hypo- and hyperthyroidism is more common amongst those exposed to the famine during gestation, and whether there is any difference in normal variation in thyroid function (TSH and FT4) in men and women depending on the timing of exposure to famine in utero.
Methods
Study Population
This study is based on data collected in 1996-1997, when the participants were 50 years old, by the DFBC study team at the Academisch Medisch Centrum (AMC), now the Amsterdam University Medical Center (UMC). The study received ethical approval from the Medical-Ethical Committee of the Academic Medical Centre of the University of Amsterdam. The study design and selection procedures of the DFBC have extensively been described elsewhere (19). In short, all singleton babies born at term in the Wilhelmina Gasthuis in Amsterdam between the 1st of November 1943 and 28th of February 1947 were eligible for inclusion in (n=5,425) (22, 23). The cohort thus included 1,380 individuals that were exposed to famine in utero based on their birth date (1 November 1944 and 28 February 1946). Additionally, a random sample of babies born the year before or conceived after this pre-defined period were selected. Exclusion criteria included premature birth (i.e., gestational age <37 weeks), individuals with missing medical records, or multiple pregnancies. Of the 2,414 liveborn singletons it was possible to trace 2,155 individuals (89.2%) using Amsterdam’s population registry (24). 910 Individuals were visited at home and interviewed by the research team. Medical histories were recorded, including any previous diagnosis of thyroid dysfunction and current treatment of thyroid disease.
Ultimately 741 individuals were included in the first clinical data collection wave at age 50 and attended the follow-up clinic at the AMC. Exclusion criteria at this stage included death, migration, and inability to attend the clinic in Amsterdam (22). Of these participants that attended the follow-up clinic, 728 individuals provided blood samples from which thyroid function (at least TSH as well as free T4) could be determined. Participants are divided up into the following groups based on birth date as an indication of trimester of famine exposure (19, 22): i) those born shortly before the famine (born 11/1943-1/1944); ii) those mainly exposed in late gestation (born 1/1945-4/1945); iii) those mainly exposed in mid gestation (born 4/1945-8/1945); iv) those mainly exposed in early gestation (born 8/1945-12/1945); v) those conceived after famine exposure (12/1945-2/1947). Of the 728 participants, 291 were in utero whilst being exposed to the famine, whereas another 437 were born shortly before or after the famine.
Laboratory Analyses
Blood samples were drawn in the morning. Thyroid function tests included assessment of thyroid stimulating hormone (TSH), free thyroxine (FT4), and antibodies against thyroid peroxidase (TPO) and thyroglobulin (TG). TSH was measured using an immunofluorimetric assay (IFMA) by Delfia, Wallac - Perkin Elmer (cut-off: 0,4 – 4,0 mE/L). FT4 was measured with a fluorescent immunoassay by Delfia, Wallac - Perkin Elmer (cut-off: 10 – 23 pmol/L). Anti-TPO and anti-TG were measured using radioimmunoassay by Brahms and in house of the AMC respectively. For the autoantibody positivity the cut-off for positivity was set at >100 kU/L. These thyroid function tests were analysed in batch in the endocrinology laboratory of the Amsterdam university hospital in 1997 shortly after collection of the data in 1994-1996.
Definitions of Thyroid Disease
Using medical histories collected during interviews at home (n=910), we identified anyone with a previous diagnosis of thyroid dysfunction, being treated for a thyroid condition, or taking medication for hypothyroidism. Based on the thyroid function tests in combination with the medical histories of the participants for whom blood samples were available (n=728), we report of rates of overt hypo- and hyperthyroidism in early, mid, and late gestation exposure groups and those born before or after the famine occurred. For the purpose of this study, we defined overt hypothyroidism as i) previous diagnosis of hypothyroidism (either of autoimmune origin or as a result of post-thyroidectomy) as self-reported in the medical history, ii) taking thyroid medication (prescribed as eltroxin, euthyrox, thyrax, thyrofix, tirosint, or as generic levothyroxine in the Netherlands), iii) a TSH >10 mE/L or a combination of TSH>4mE/L and FT4<10 pmol/L at the time of blood collection. Hyperthyroidism was defined as i) current diagnosis of hyperthyroidism (due to Graves’ disease or another reason), ii) TSH levels <0.4 mE/L in combination with FT4 >23 pmol/L. Anti-TPO and anti-TG positivity were used to determine thyroid autoimmunity.
Statistical Analyses
Firstly, we reported on sample characteristics according to timing of famine exposure in utero, differentiating between early, late, and mid gestation, as well as those born before or conceived after the famine in the complete cohort of 910 individuals. Using thyroid function tests in 728 individuals from which blood samples were taken, we then assessed significance of any differences in overt thyroid dysfunction or thyroid autoantibodies according to timing of famine exposure and sex. We used binary logistic regression models to estimate the adjusted odds ratios (OR) with 95% confidence interval (95%CI).
Excluding participants with overt hypo- or hyperthyroidism based on thyroid function tests, previous diagnosis of thyroid dysfunction as recorded in the medical history, currently taking thyroid medication or other medication that may affect thyroid function such as amiodarone, we report the sex- and trimester-specific effect of famine exposure. One participant with an abnormally high FT4 level (>50 mE/L) but normal TSH level was also excluded from this subsample as this measurement was attributed to a measurement artefact. This created a subsample free from overt thyroid disease with thyroid function tests in the normal range. Next, TSH levels were log-transformed and estimates were back transformed, to ease interpretation. The following formulas were used for the log-transformation and the back transformation: Log10(1+TSH) and Exp(TSH)-1. Using a Two Sample T-test, we first determined whether there were any significant differences in TSH or FT4 levels between individuals who were born shortly before or conceived shortly after the famine, pooling these into one group of individuals not exposed to famine during gestation when no significant difference was found. We then used linear regression models to investigate the interaction between sex and famine exposure. In model 1 the association between timing of famine exposure and TSH and FT4 levels were assessed by entering exposure groups in the model as dummies and setting the group that was not exposed to famine in utero as the reference category. Model 2 was besides famine exposure further adjusted for sex. In model 3, interaction terms between sex and famine exposure groups (early, mid, late) were added. In case of a sex effect, where an interaction was deemed significant for p-values smaller than 0.1, the sample was sex-stratified.
The following possible confounders were selected by examining their (bivariate) association with TSH and FT4 levels, at a significance level of p<0.1 for either TSH or FT4. Smoking was defined as those who reported at the time of the interview that they had been smoking longer than 1 month (23). Menopausal status was calculated as the last menstrual period being more than 12 months in the past from the date of the study interview. Socioeconomic status was based on the International Socio-Economic Index (ISEI-92) which is a scale of socioeconomic status between 16 (low) and 87 (high) and was constructed from the highest socioeconomic status between study participant and their partner (25). All statistical analyses were conducted in RStudio (version 1.3.9590).
Results
Of the 910 participants included, 4.9% had previously been diagnosed with a thyroid dysfunction, and 1.8% were currently under treatment for the condition (Table 1). Based on the blood samples taken from 728 participants, 2.3% had thyroid autoantibodies (anti-TPO or anti-TG) above the clinical threshold. The incidence of overt hypothyroidism in this sample based on thyroid function tests and medical histories was 4.4%, whereas the incidence of overt hyperthyroidism was 2.2% (Table 2). There was no significant difference in prevalence of hypothyroidism, hyperthyroidism, or thyroid antibodies between early, mid, and late gestation famine exposed groups and those born before or after the famine. However, the odds of hypothyroidism were greater amongst women than men (OR=2.16, 95%CI [1.04,4.84], p<0.05), as were the odds of hyperthyroidism (OR= 4.24, 95%CI [1.35-18.60], p<0.01). Furthermore, more women than men had thyroid autoantibody levels (anti-TPO and/or anti-TG) above the clinical threshold (antibody positivity OR= 4.54, 95%CI [1.47-19.81], p<0.02).
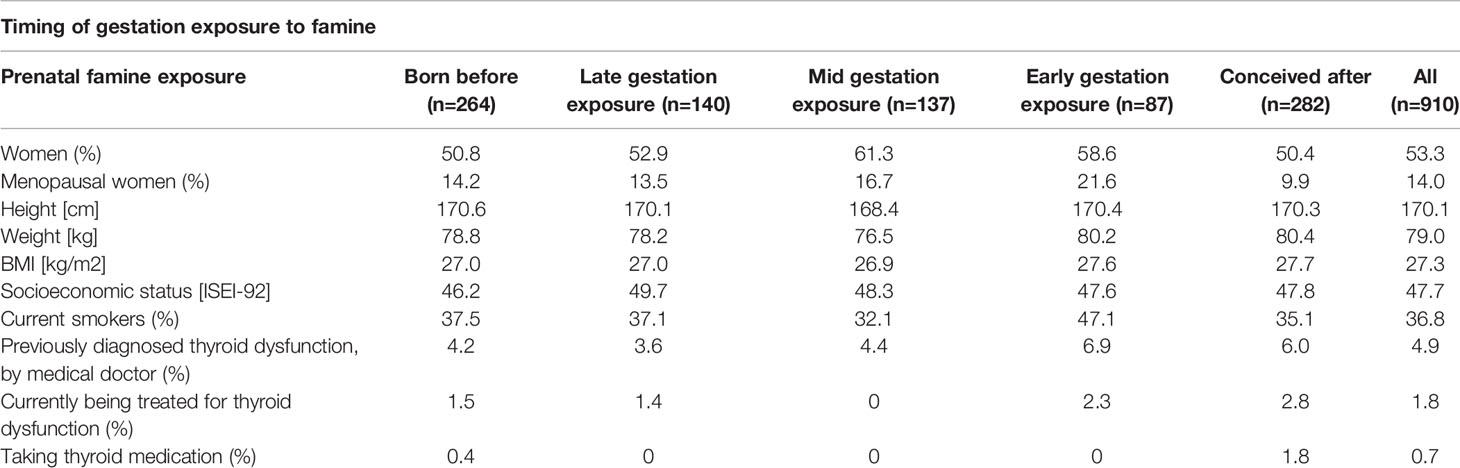
Table 1 Mean descriptives of the DFBC clinical visit cohort that had thyroid function tests taken at age 50 years old, according to timing of famine exposure in early life (n=910).
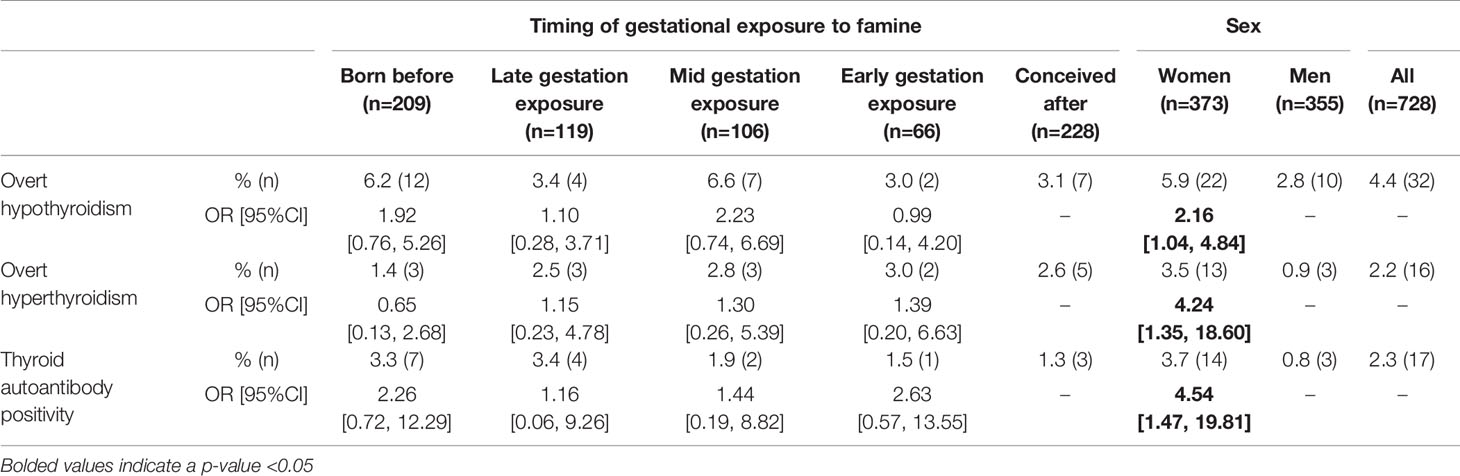
Table 2 Prevalence and odds ratio of thyroid dysfunction in the DFBC depending on sex and famine exposure group (born before, late, mid, or early gestation, or conceived after) as well as sex in a subsample for which thyroid function tests were available (n=728).
Individuals with overt hyper- or hypothyroidism were excluded from subsequent analyses, as was anyone previously diagnosed by a medical doctor with thyroid dysfunction or currently being treated for a thyroid condition, leading to a final sample of 675 participants. We did not find significant differences between the TSH and FT4 levels of participants born before or conceived after the famine (TSH t=0.58, p=0.56; FT4 t=-0.14, p=0.89) (results not shown), therefore individuals not in utero during the famine were grouped to form the unexposed group. Supplementary Table 1 shows the levels of TSH (geometric mean and interquartile range (IQR)) and FT4 (mean and standard deviation (SD)) of the early, mid, and late gestation exposed groups as well as the unexposed. Table 3 presents models 1, 2, and 3 in the sample including both sexes and in the sex-stratified subsamples. Results of model 1 indicate that exposure group alone is not associated with TSH or FT4 levels, however sex was significantly associated with both TSH and FT4 levels (model 2). It was found in model 3 that adding sex and famine exposure as interaction terms, there were significant differences (p<0.1) in TSH within the early and mid exposed groups. Sex-stratified models revealed a significant trend in women towards lower TSH levels as a result of famine exposure in the second trimester of gestation, but not in men. Sex, smoking, and socioeconomic status were significantly associated with log-transformed TSH and FT4 levels in bivariate analyses, therefore we adjusted for these variables in the final analyses (Supplementary Table 2; Table 4). These final models were separated by sex based on the finding in bivariate analysis that famine exposure might affect TSH levels in the sexes differently. In these final models it was found that when correcting for confounding variables, mid-famine exposure was significantly associated with lower TSH levels in women but not men. There was no significant association between famine exposure and FT4 levels in either sex.
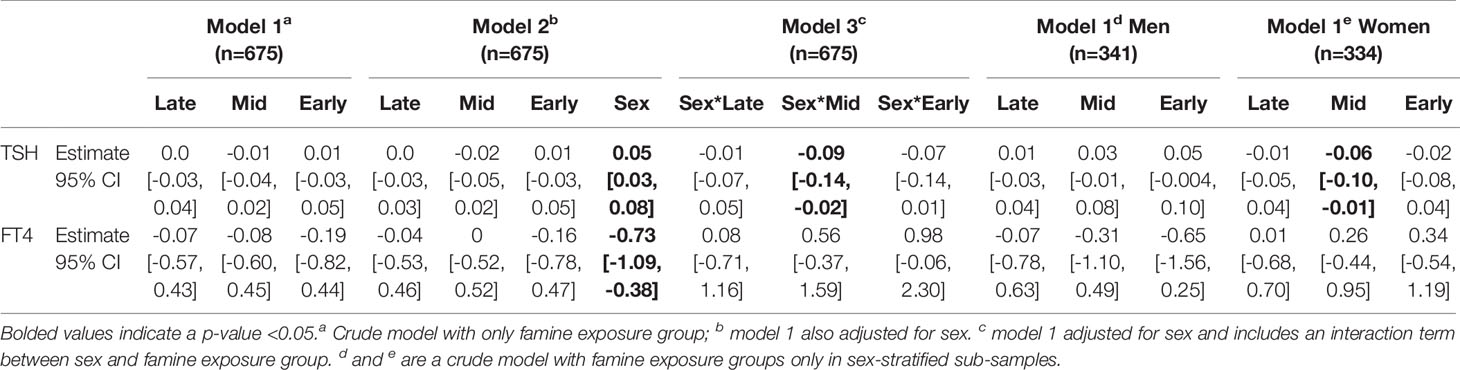
Table 3 Estimates of the association between in utero famine exposure group (late, mid, early) compared to participants that were not exposed to famine during gestation and thyroid function (TSH and FT4) tests in a thyroid disease free subsample (n=675).

Table 4 Adjusted Estimates, 95% CIs, and p-values of TSH levels and FT4 levels in men (n=341) and women (n=334) exposed to famine in utero compared to those not exposed to famine during gestation.
Discussion
In this study it was found that incidence of thyroid dysfunction in adulthood was not greater in men and women prenatally exposed to the Dutch Famine of 1944-1945 than individuals born shortly before or conceived after the famine. However, mid gestation famine exposure in utero affected women’s thyroid function 50 years later, but not men’s. Indeed, amongst mid gestation famine exposed women without overt thyroid dysfunction we found significantly lower TSH values within the normal range. No differences were observed in the FT4 levels of either sex.
The finding that prenatal famine exposure may affect the HPT axis in adulthood is in accordance with previous studies in animals (14, 15) and survivors of the Chinese famine (17, 18), which also found such effects, but did not assess trimester-specific effects or the role of sex therein. Particularly, we found an effect of famine exposure mid-gestation on TSH levels in women. From an ontogenic point of view the second trimester marks the greatest growth of the foetal thyroid during development and is the time that the HPT axis first becomes active (after the 12th week of gestation approximately) (13). Our findings align with previous studies in other organ systems in the DFBC, which found that famine exposure during the second trimester disrupts the normal growth of the lungs and kidneys, which also develop and expand sizably during mid gestation (26, 27).
Previous studies within the DFBC have found that prenatal famine exposure leads to considerable differences in health outcomes, and that timing plays an important role in determining what organ systems are affected (19). Various findings within the DFBC suggest that famine exposure in utero affects other biomarkers of metabolism in adulthood as those exposed to famine are more likely to have impaired glucose tolerance (28, 29), a more atherogenic lipid profile (22, 30), and suffer from higher rates of obesity (23, 31), even up to more than threequarters of a century later (19). These effects seem to be sex-specific and timing of exposure during gestation causes significant differences in metabolic phenotypes (23, 30). Those exposed to famine early in gestation, during the time which organogenesis takes place, have a higher chronic disease risk, for example having a higher prevalence of coronary heart disease risk (19, 24). Men exposed to famine in early gestation, but not women, were found to have smaller intracranial and total brain volume compared to unexposed controls (32). Early exposed individuals of both sexes perform worse on selective attention tests (33) and showed signs of premature brain aging in late adulthood (34). Individuals exposed to famine mid gestation, when the respiratory and renal systems undergo rapid growth and development, are more likely to suffer from obstructive airway disease (27) or microalbuminuria (26) in later life. Finally, famine exposure particularly in mid-late gestation is associated with impaired glucose tolerance compared to unexposed individuals (19, 35). This suggests that prenatal famine exposure at the time of the development of vital organs such as the heart, brain, lungs, kidneys, pancreas as well as the HPT axis, and may have long term impacts on the regulation of these physiological systems.
The reduced TSH levels at age 50 in women exposed to famine conditions in mid gestation might be the result of epigenetic alterations within the regulation of the HPT axis. It has been suggested that tissue-specific changes in the epigenetic expression of deiodinase enzymes, which activate and deactivate thyroid hormones, may be a putative mechanism by which such changes in the regulation of the HPT axis can occur (1, 36, 37). Research by Anselmo et al. (2019) on the intergenerational effect of foetal exposure to abnormally high maternal thyroid hormone levels in an Azorean population has shown that even in the third generation, reduced sensitivity to administered thyroid hormones is found (37). They speculated that the pituitary expression of the deiodinase 3 enzyme, which inactivates thyroid hormones by deiodination of the inner ring of both T4 and T3, is increased after exposure to maternal hyperthyroidism during pregnancy (36). The opposite might be true in the context of maternal malnutrition during pregnancy, which has been shown to lead to decreased thyroid hormone levels, specifically T3, compared to well-nourished women (38). Based on this research, we postulate that central feedback suppression in female offspring may be permanently enhanced due to decreased pituitary expression of deiodinase 3, although free T4 levels in the peripheral circulation as measured from fasting blood samples remain unaffected. On the level of the thyroid, prenatal undernutrition in late gestation followed by postnatal overnutrition has been shown to lead to a higher expression of TSH receptors in the thyroid tissue of female sheep, supporting the hypothesis that the thyroid axis is more sensitive to the effects of TSH and thyroid hormones after maternal dietary restrictions during gestation (15). Further research is also needed to elucidate why women but not men experience alterations in the HPT axis due to famine exposure at mid gestation. Interestingly, the second trimester is also when the hypothalamic-pituitary-gonadal (HPG) axis becomes active, which also happens in a sexually dimorphic manner (39). In future research it may be of interest to understand how the sexually dimorphic activation of the HPG axis during this pregnancy trimester interacts with the programming of the HPT axis regulation.
This study has several limitations. Firstly, it is important to consider the general limitations of the DFBC, such as selective fertility in women exposed to the famine of 1944-1945, high rates of perinatal deaths amongst famine exposed pregnancies, and selective survival and participation in the cohort before the follow-up clinics were conducted (19). Secondly, only a single measurement was taken to determine thyroid function, despite evidence that thyroid hormones can fluctuate (40) and are influenced by factors such as seasonality (41), time of the day (42, 43), and menstrual cycle phase for premenopausal women (44). However, all blood samples were taken during the morning. Moreover, only the levels of TSH and FT4 were measured, precluding an estimate of local T4 to T3 conversion, which is known to be affected by starvation in utero (38). Thirdly, the thyroid autoantibody levels in this study were slightly lower than reported in more recent studies, but this may be due to more stringent cut-offs being used in the 1990s and/or lower sensitivity of the tests at the time. Finally, iodine status of the mother at the time of gestation remains unknown because of the war circumstances under which the famine took place. There is some evidence from Belgian monks, who persisted during the war by eating tulip bulbs and subsequently developed goitres, suggestive of severe iodine deficiency (45). Severe iodine deficiency among pregnant women (46–48) may impact thyroid function not only in themselves but also in their offspring, through altered thyroid hormone levels in their mothers or decreased transplacental iodine transport.
In this study we showed that the regulation of the HPT axis, which is closely related to metabolic phenotype, may be subtly altered as a result of famine exposure in the second trimester when vital thyroid development and growth take place. However, future research should focus on why the disruption by famine during this period of growth might affect female foetuses differently from male ones. Using the Dutch Famine of 1944-1945 as a historical ‘natural experiment’ this study points towards the existence of subtle sex-specific and trimester-specific effects of severe malnutrition in utero on the thyroid physiology of adult survivors.
Conclusion
In this study of survivors of the Dutch Famine (1944-1945) we found that there are no differences in prevalence of adult thyroid disease at age 50 according to prenatal famine exposure. However, in mid gestation exposed women but not men we find lower TSH levels, which suggest that there may be subtle sex-specific effects of famine exposure during a critical period of thyroid development on the regulation of the HPT axis in adulthood.
Data Availability Statement
The data that support the findings of this study are available on request from the corresponding author by submitting a formal project outline. The data are not publicly available due to participant privacy restrictions. Code is available upon request from the corresponding author.
Ethics Statement
The studies involving human participants were reviewed and approved by Medical-Ethical Committee of the Academic Medical Centre of the University of Amsterdam. The patients/participants provided their written informed consent to participate in this study.
Author Contribution
AR and TR collected the data in 1996-1997. IM is responsible for data management. SK conducted the statistical analyses under supervision of TR, IM, and MF. SK wrote the first draft of the manuscript. All authors contributed to the revisions of the manuscript and approved the final version of the manuscript as submitted.
Funding
The Dutch famine birth cohort study has been funded by the Medical Research Council (UK), the Diabetes Fonds (Netherlands), Netherlands Heart Foundation grants (NHS2001B087, NHS2007B083; Netherlands), WellBeing (UK), the Diabetes UK, The European Science Foundation (EUROSTRESS-DOME project), the European Commission (Brainage (Seventh Framework Programme Project 279281)), Dynahealth (Horizon 2020 Project 633595), Longitools (Horizon 2020 Project 874739), Well-being (UK, Grant Number NA), the Medical Research Council (UK, Grant Number NA), the Dutch Research Council (NWO Aspasia Project 015014039) and the Academic Medical Centre (Amsterdam, The Netherlands, Grant number NA). SK is supported by a MD/PhD scholarship from the Amsterdam University Medical Centre (Netherlands) and a Moving Forward grant from Amsterdam Reproduction and Development (Netherlands).
Conflict of Interest
The authors declare that the research was conducted in the absence of any commercial or financial relationships that could be construed as a potential conflict of interest.
Publisher’s Note
All claims expressed in this article are solely those of the authors and do not necessarily represent those of their affiliated organizations, or those of the publisher, the editors and the reviewers. Any product that may be evaluated in this article, or claim that may be made by its manufacturer, is not guaranteed or endorsed by the publisher.
Acknowledgments
We thank all the men and women of the Dutch Famine Birth Cohort who participated in the study; Marjan Loep, Mieneke Vaas, Lydia Stolwijk, Yvonne Graafsma, Jokelies Knopper, Maartje De Ley, and the nurses at the Special Research Unit for collecting the data; the Gemeentearchief of Amsterdam for tracing the birth records; and the Bevolkingsregister of Amsterdam for tracing the subjects. Additionally, we would like to thank Prof. dr A. Boelen of the Endocrinologisch Laboratorium Amsterdam UMC for providing the reference values that were used for the interpretation of the thyroid function tests. Finally, S.K. is grateful for the help of Austin Argentieri of the University of Oxford for advice on the data analysis.
Supplementary Material
The Supplementary Material for this article can be found online at: https://www.frontiersin.org/articles/10.3389/fendo.2022.836245/full#supplementary-material
References
1. Keestra S, Tabor VH, Alvergne A. Reinterpreting Patterns of Variation in Human Thyroid Function An Evolutionary Ecology Perspective. Evol Med Public Health (2021) 9(1):93–112. doi: 10.1093/emph/eoaa043
2. Kachuei M, Jafari F, Kachuei A, Keshteli AH. Prevalence of Autoimmune Thyroiditis in Patients With Polycystic Ovary Syndrome. Arch Gynecol Obstet (2012) 285:853–6. doi: 10.1007/s00404-011-2040-5
3. Rodondi N, Elzen WPJD, Bauer DC, Cappola AR, Bremner A, Maisonneuve P, et al. Subclinical Hypothyroidism and the Risk of Coronary Heart Disease and Mortality. JAMA - J Am Med Assoc (2010) 304:1365–74. doi: 10.1001/jama.2010.1361
4. Biondi B. Thyroid and Obesity: An Intriguing Relationship. J Clin Endocrinol Metab (2010) 95:3614–7. doi: 10.1210/jc.2010-1245
5. Saklayen MG. The Global Epidemic of the Metabolic Syndrome. Curr Hyperten Rep 2018 20:2 (2018) 20:1–8. doi: 10.1007/S11906-018-0812-Z
6. Meher, SK R, SK K, Sarangi J, Jali SN. Prevalence of Hypothyroidism in Patients With Metabolic Syndrome. Thyroid Res Pract (2013) 10:60. doi: 10.4103/0973-0354.110583
7. Kuzawa CW, Gluckman PD, Hanson MA, Beedle AS. Evolution, Developmental Plasticity, and Metabolic Disease. In: Stearns SC, Koella JC, editors. Evolution in Health & Disease. Oxford: University Press (2008). p. 253–64.
8. Gaberšček S, Zaletel K, Schwetz V, Pieber T, Obermayer-Pietsch B, Lerchbaum E. Thyroid and Polycystic Ovary Syndrome. Eur J Endocrinol (2015) 172:9–21. doi: 10.1530/EJE-14-0295
9. Hochberg Z, Feil R, Constancia M, Fraga M, Junien C, Carel J-C, et al. Child Health, Developmental Plasticity, and Epigenetic Programming. Endocr Rev (2011) 32:159–224. doi: 10.1210/er.2009-0039
10. Barker DJP. The Origins of the Developmental Origins Theory. J Internal Med (2007) 261:412–7. doi: 10.1111/j.1365-2796.2007.01809.x
11. Lea AJ, Tung J, Archie EA, Alberts SC. Developmental Plasticity Bridging Research in Evolution and Human Health. Evol Med Public Health (2017) 2017:162–75. doi: 10.1093/emph/eox019
12. Zwaveling-Soonawala N, van Beijsterveldt CEM, Mesfum ET, Wiedijk B, Oomen P, Finken MJJ, et al. Fetal Environment is a Major Determinant of the Neonatal Blood Thyroxine Level: Results of a Large Dutch Twin Study. J Clin Endocrinol Metab (2015) 100:2388–95. doi: 10.1210/jc.2015-1429
13. Radaelli T, Cetin I, Zamperini P, Ferrazzi E, Pardi G. Intrauterine Growth of Normal Thyroid. (2009) 16(6):427–30. doi: 10.1080/gye.16.6.427.430
14. Ayala-Moreno R, Racotta R, Anguiano B, Aceves C, Quevedo L. Perinatal Undernutrition Programmes Thyroid Function in the Adult Rat Offspring. Br J Nutr (2013) 110:2207–15. doi: 10.1017/S0007114513001736
15. Johnsen L, Kongsted AH, Nielsen MO. Prenatal Undernutrition and Postnatal Overnutrition Alter Thyroid Hormone Axis Function in Sheep. J Endocrinol (2013) 216:389–402. doi: 10.1530/JOE-12-0389
16. Lisboa PC, Fagundes ATS, Denolato ATA, Oliveira E, Bonomo IT, Alves SB, et al. Neonatal Low-Protein Diet Changes Deiodinase Activities and Pituitary TSH Response to TRH in Adult Rats. https://doi.org/103181/0705-RM-146 (2008) 233:57–63. doi: 10.3181/0705-RM-146
17. Guo J, Teng D, Shi X, Li Y, Ba J, Chen B, et al. Exposure to the Chinese Great Famine in Early Life and Thyroid Function and Disorders in Adulthood: A Cross-Sectional Study. Thyroid (2021) 31(4):563–71. doi: 10.1089/thy.2020.0325
18. Zheng X, Long J, Ren W, Liu C, Wei Q, Luo R, et al. Exposure to the Chinese Famine in Early Life and the Thyroid Function and Nodules in Adulthood | Elsevier Enhanced Reader. Endocr Pract (2019) 25:598–603. doi: 10.4158/EP-2019-0004
19. Bleker LS, de RSR, RC P, Ravelli ACJ, Roseboom TJ. Cohort Profile: The Dutch Famine Birth Cohort (DFBC), a Prospective Birth Cohort Study in the Netherlands. BMJ Open (2021) 11:e042078. doi: 10.1136/bmjopen-2020-042078
20. Lumey LH, Van Poppel FWA. The Dutch Famine of 1944-45: Mortality and Morbidity in Past and Present Generations. Soc History Med (1994) 7:229–46. doi: 10.1093/shm/7.2.229
21. Ravelli ACJ. Nutritional Intake During the Dutch Famine. Amsterdam (1999). Available at: https://pure.uva.nl/ws/files/3358789/5585_UBA003000061_005.pdf.
22. Roseboom TJ, van der Meulen JH, Osmond C, Barker DJ, Ravelli AC, Bleker OP. Plasma Lipid Profiles in Adults After Prenatal Exposure to the Dutch Famine. Am J Clin Nutr (2000) 72:1101–6. doi: 10.1093/ajcn/72.5.1101
23. Ravelli AC, van der Meulen JH, Osmond C, Barker DJ, Bleker OP. Obesity at the Age of 50 Y in Men and Women Exposed to Famine Prenatally. Am J Clin Nutr (1999) 70:811–6. doi: 10.1093/ajcn/70.5.811
24. Roseboom TJ, van der Meulen JHP, Osmond C, Barker DJP, Ravelli ACJ, Schroeder-Tanka JM, et al. Coronary Heart Disease After Prenatal Exposure to the Dutch Famine, 1944-45. Heart (2000) 84:595–8. doi: 10.1136/heart.84.6.595
25. Ginty AT, Phillips AC, Roseboom TJ, Carroll D, DeRooij SR. Cardiovascular and Cortisol Reactions to Acute Psychological Stress and Cognitive Ability in the Dutch Famine Birth Cohort Study. Psychophysiology (2012) 49:391–400. doi: 10.1111/j.1469-8986.2011.01316.x
26. Painter RC, Roseboom TJ, van Montfrans GA, Bossuyt PMM, Krediet RT, Osmond C, et al. Microalbuminuria in Adults After Prenatal Exposure to the Dutch Famine. J Am Soc Nephrol (2005) 16:189–94. doi: 10.1681/ASN.2004060474
27. Lopuhaä CE, Roseboom TJ, Osmond C, Barker DJP, Ravelli ACJ, Bleker OP, et al. Atopy, Lung Function, and Obstructive Airways Disease After Prenatal Exposure to Famine. Thorax (2000) 55:555–61. doi: 10.1136/thorax.55.7.555
28. de Rooij SR, Painter RC, Phillips DIW, Osmond C, Michels RPJ, Godsland IF, et al. Impaired Insulin Secretion After Prenatal Exposure to the Dutch Famine. (2006) 29(8):1897–901. doi: 10.2337/dc06-0460
29. de Rooij SR, Painter RC, Roseboom TJ, Phillips DIW, Osmond C, Barker DJP, et al. Glucose Tolerance at Age 58 and the Decline of Glucose Tolerance in Comparison With Age 50 in People Prenatally Exposed to the Dutch Famine. Diabetologia (2006) 49:637–43. doi: 10.1007/s00125-005-0136-9
30. Lumey L, Stein AD, Kahn HS, Romijn J. Lipid Profiles in Middle-Aged Men and Women After Famine Exposure During Gestation: The Dutch Hunger Winter Families Study. Am J Clin Nutr (2009) 89:1737–43. doi: 10.3945/ajcn.2008.27038
31. Ravelli G-P, Stein ZA, Susser MW. Obesity in Young Men After Famine Exposure in Utero and Early Infancy. New Engl J Med (1976) 295:349–53. doi: 10.1056/nejm197608122950701
32. de Rooij SR, Caan MW, Swaab DF, Nederveen AJ, Majoie CB, Schwab M, et al. Prenatal famine exposure has sex-specific effects on brain size. Brain (2016) 139(Pt 8):2136–42 doi: 10.1093/brain/aww132
33. de Rooij SR, Wouters H, Yonker JE, Painter RC, Roseboom TJ. Prenatal undernutrition and cognitive function in late adulthood. Proceedings of the National Academy of Sciences of the United States of America. (2010) 107(39):16881–6. doi: 10.1073/pnas.1009459107
34. Franke K, Gaser C, Roseboom TJ, Schwab M, de Rooij SR. Premature Brain Aging in Humans Exposed to Maternal Nutrient Restriction During Early Gestation. Neuroimage (2018) 173:460–71. doi: 10.1016/J.NEUROIMAGE.2017.10.047
35. Ravelli ACJ, van der Meulen JHP, Michels RPJ, Osmond C, Barker JP, Hales CN, et al. Glucose Tolerance in Adults After Prenatal Exposure to Famine. (1998) 351(9097):173–7. doi: 10.1016/S0140-6736(97)07244-9
36. Anselmo J, Chaves CM. (2020). Physiologic Significance of Epigenetic Regulation of Thyroid Hormone Target Gene Expression. Eur Thyroid J (2020) 9(3):114–23. doi: 10.1159/000506423
37. Anselmo J, Scherberg NH, Dumitrescu AM, Refetoff S. Reduced Sensitivity to Thyroid Hormone as a Transgenerational Epigenetic Marker Transmitted Along the Human Male Line. Thyroid (2019) 29:778–82. doi: 10.1089/thy.2019.0080
38. Mahajan SD, Aalinkeel R, Singh S, Shah P, Gupta N, Kochupillai N. Thyroid Hormone Dysregulation in Intrauterine Growth Retardation Associated With Maternal Malnutrition and / or Anemia. Hormone Metab Res (2005) 37:633–40. doi: 10.1055/s
39. Bizzarri C, Cappa M. Ontogeny of Hypothalamus-Pituitary Gonadal Axis and Minipuberty: An Ongoing Debate? Front Endocrinol (2020) 11:187. doi: 10.3389/FENDO.2020.00187
40. Ehrenkranz J, Bach PR, Snow GL, Schneider A, Lee JL, Ilstrup S, et al. Circadian and Circannual Rhythms in Thyroid Hormones: Determining the TSH and Free T4 Reference Intervals Based Upon Time of Day, Age, and Sex. Thyroid (2015) 25:954–61. doi: 10.1089/thy.2014.0589
41. de Grande LAC, Goossens K, van Uytfanghe K, Halsall I, Yoshimura Noh J, Hens K, et al. Using “Big Data” to Describe the Effect of Seasonal Variation in Thyroid-Stimulating Hormone. Clin Chem Lab Med (2017) 55:e34–6. doi: 10.1515/cclm-2016-0500
42. Ikegami K, Refetoff S, van Cauter E, Yoshimura T. Interconnection Between Circadian Clocks and Thyroid Function. Nat Rev Endocrinol (2019) 15:590–600. doi: 10.1038/s41574-019-0237-z
43. Jensen E, Blaabjerg O, Petersen PH, Hegedüs L. Sampling Time is Important But may be Overlooked in Establishment and Use of Thyroid-Stimulating Hormone Reference Intervals. Clin Chem (2007) 53:355–6. doi: 10.1373/clinchem.2006.078964
44. Benvenga S, di Bari F, Granese R, Antonelli A. Serum Thyrotropin and Phase of the Menstrual Cycle. Front Endocrinol (2017) 8:250. doi: 10.3389/fendo.2017.00250
46. Luck M. “The Thyroid Gland.,”. In: Hormones - A Very Short Introduction. Oxford: Oxford University Press (2014). p. 88–96.
47. de Leo S, Pearce EN, Braverman LE. Iodine Supplementation in Women During Preconception, Pregnancy, and Lactation: Current Clinical Practice by U.S. Obstetricians and Midwives. Thyroid (2017) 27:434–9. doi: 10.1089/thy.2016.0227
Keywords: famine, thyroid, DOHaD (Developmental origins of health and disease), TSH (thyroid stimulating hormone), thyroxine (FT4)
Citation: Keestra SM, Motoc I, Ravelli ACJ, Roseboom TJ and Finken MJJ (2022) Thyroid Function at Age Fifty After Prenatal Famine Exposure in the Dutch Famine Birth Cohort. Front. Endocrinol. 13:836245. doi: 10.3389/fendo.2022.836245
Received: 30 December 2021; Accepted: 18 May 2022;
Published: 30 June 2022.
Edited by:
Francesco Vermiglio, University of Messina, ItalyReviewed by:
Arturo Hernandez, Maine Medical Center, United StatesHaixia Guan, Guangdong Provincial People’s Hospital, China
Copyright © 2022 Keestra, Motoc, Ravelli, Roseboom and Finken. This is an open-access article distributed under the terms of the Creative Commons Attribution License (CC BY). The use, distribution or reproduction in other forums is permitted, provided the original author(s) and the copyright owner(s) are credited and that the original publication in this journal is cited, in accordance with accepted academic practice. No use, distribution or reproduction is permitted which does not comply with these terms.
*Correspondence: Sarai M. Keestra, cy5tLmtlZXN0cmFAYW1zdGVyZGFtdW1jLm5s