- Department of Urology, Tianjin Medical University General Hospital, Tianjin, China
Background: Although studies on the effects of diet on fertility has progressed, some cumulative evidence has piled against popular hypotheses. The aim of our study was to investigate the effects of 31 diets including 23 individual dietary intakes and 8 dietary habits on infertility in men and women.
Methods: The datas of diets and infertility were collected from genome-wide association studies (GWAS). Mendelian randomization (MR) methods were used to analyze causal relationships. Multivariate MR (MVMR) adjusted for the effects of other exposures on causality. And MR-Egger, Cochran’s Q, radial MR, and MR-PRESSO tests were employed to assess heterogeneity and horizontal pleiotropy.
Results: Our study found that coffee intake (OR, 3.6967; 95% CI, 1.0348 – 13.2065; P = 0.0442) and cooked vegetable intakes (OR, 54.7865; 95% CI, 2.9011 – 1030.5500; P = 0.0076) increased the risk of male infertility. For women, beer was a risk factor for infertility (OR, 4.0932; 95% CI, 1.8728 – 8.9461; P = 0.0004); but processed meat was negatively associated with infertility (OR, 0.5148; 95% CI, 0.2730 – 0.9705; P = 0.0401). MVMR demonstrated selenium as a protective factor against female infertility (OR, 7.4474e-12; 95% CI, 5.4780e-22 – 1.0125e-01; P = 0.0314).
Conclusion: We found the causal relationships between four diets and infertility. We look forward to more high-quality epidemiologic studies to prove our conclusions.
Introduction
Infertility is defined as the failure to achieve a clinically recognized pregnancy after 12 months or more of regular unprotected sexual intercourse (1). Globally, infertility affects 15% of couples at reproductive age (2). According to reports, 33–41% of cases of infertility are caused exclusively by a female factor, 25–39% by a male factor, and 9–39% by a combination of both male and female factors (3). Infertility can cause severe psychological distress to the patient, as well as great distress and economic burden to society (4).
Current treatment options for infertility include assisted reproductive technologies (ART) including ovarian stimulation with or without intrauterine insemination (IUI) and in vitro fertilization (IVF). Despite increased ART use, the results for amelioration of infertility are still poor, with an average of only one in five cycles resulting in a live birth (5). Given the high prevalence of impaired fertility, coupled with the high financial cost of infertility treatment, the impact of lifestyle factors on infertility is increasingly being considered. Being overweight or obese plays a significant role in the fertility status of a couple (6). Although diet and obesity are undoubtedly related, there is growing evidence that diet may independently influence fertility. This effect depends on the quantitative and qualitative impact of diet, including calories per macronutrient, and specific proteins, carbohydrates, and certain acid-spectrum fatty acids (7, 8). For men, for example, high-fat diets not only have a strong negative impact on sperm parameters, but are also associated with smaller testes, lower seminal vesicle and epididymal quality. Most importantly, high-fat diets are associated with lower fertilization success rates (9, 10). In addition, following a “fertility diet” may favorably impact the fertility of otherwise healthy women, as well as preventing most cases of infertility caused by ovulatory disorders (11). Although the impact of diet on reproductive performance has been increasingly recognized in recent years, there are no dietary guidelines for fertility (12). The aim of our study was to explore the causal link between 31 different dietary plans and infertility using Mendelian randomization (MR) methods. In addition, we explored the effects of diet on infertility in men and women, with a view to providing guidance on dietary choices for couples of childbearing age; and to provide a basis for investigating the mechanisms of sex differences while probing the relationship between diet and infertility.
MR is an epidemiological method that uses genetic variants to simulate an exposure and predict its causal association with an outcome. The main limitation of observational studies and randomized trials is the inadequate validation of confounders, leading to biased estimates of causality. In contrast, genetic variation is a lifetime constant, independent of confounding effects of the environment (13, 14).
Methods
Data sources
For the causal analysis, diet was considered as the exposure variable, and infertility in men and women as the outcome variable. The genetic variants of dietary intake comprised output from the genome-wide association studies (GWAS) pipeline using Phenome-wide Scan Analysis Tools (PheSANT)-derived variables of the UK Biobank cohort of about 500,000 individuals (15). The list of 31 dietary intakes included in the analysis consisted of 23 individual dietary intakes and 8 dietary habits. 23 individual dietary intakes include bacon, beef, bread, cereal, cheese, coffee, cooked vegetable, dried fruit, fresh fruit, lamb, milk, non-oily fish, oily fish, pork, poultry, processed meat, raw vegetable, salted nuts, salted peanuts, tea, unsalted nuts, unsalted peanuts, and yogurt; and 8 dietary habits include average weekly beer intake, average weekly red wine intake, average weekly spirits intake, never eat dairy products, never eat eggs or foods containing eggs, never eat sugar or foods/drinks containing sugar, never eat wheat products, and eat eggs, dairy, wheat, and sugar. Upon participant recruitment, a touchscreen questionnaire used in UK Biobank asked twenty-nine questions about diet, most of which gathered information about the mean frequency of consumption of food and food groups over the past year.
Genetic data for the outcome phenotype including male infertility (680 cases and 72,799 controls) and female infertility (6,481 cases and 68,969 controls) were collected from the FinnGen Consortium.
Instrumental variables selection
A reliable MR study must fulfill three basic assumptions: (1) the genetic variations and the exposure of interest are closely associated; (2) the genetic instruments have no impact on the outcome and are unaffected by any confounding factors; and (3) the exposures of interest are the only factors that mediate the effects of instrumental variables (IVs) on the outcomes. We specified strict single-nucleotide polymorphisms (SNPs) filtering criteria. SNPs that were associated with diet and infertility were extracted with a GWAS threshold (p<5×10-8). Due to the low number of SNPs at the original threshold after screening for the intake of bacon, milk, yogurt, salted peanuts, unsalted peanuts, salted nuts, spirits intake, unsalted nuts, never eat dairy, never eat wheat, and never eat eggs, we chose a relaxed threshold (p<5×10-6). Using the linkage disequilibrium (LD) clustering method, SNPs with LD were identified and removed (clumping: r2 = 0.001, kb = 10,000). We also searched the PhenoScanner website (http://www.phenoscanner.medschl.cam.ac.uk/information/) to identify IVs included in the study and to exclude SNPs associated with outcomes. Lastly, SNPs with weak IV bias were assessed using F statistics. The formula used to compute F statistics was F=beta2/se2. In the MR analysis, only SNPs with F statistic values greater than 10 were considered present.
Statistical analysis
Inverse variance-weighted (IVW), weighted median (WM), and MR-Egger tests were used for two-sample Mendelian randomization (TSMR) analysis. For IVW data, we used meta-analysis to combine the Wald estimates for each SNP and obtain an overall estimate of the effect of exposure on outcome. This was considered the strongest MR analysis method (16). And the multivariate MR (MVMR) method adjusts for the effect of other exposures on outcomes. In sensitivity analysis, Cochran’s Q test was used to quantify the heterogeneity between IVs. The MR-Egger intercept test uses the intercept term to evaluate pleiotropy, ensuring genetic variants independently linked to exposure and outcome are employed for the computations. Additionally, we employed the MR pleiotropy residual sum and outlier (MR-PRESSO) and radial MR tests to identify horizontal pleiotropy and outliers. A distortion test was used to determine the results before and after correcting for outliers. Finally, a “leave-one-out” analysis was performed to assess the stability of the results, implying that one SNP was sequentially excluded to estimate whether it biased the results.
Results
Diet and infertility in men
The F-statistic values for all IVs were higher than 10, avoiding the risk of weak instrumental bias (Table 1). The results of the TSMR analysis indicated that coffee intake increased the risk of male infertility (Odds Ratio [OR], 3.6967; 95% Confidence Interval [CI], 1.0348 – 13.2065; P = 0.0442). Furthermore, there was a significant positive correlation between cooked vegetable intake and male infertility (OR, 54.7865; 95% CI, 2.9011 – 1030.5500; P = 0.0076) (Figures 1;, 2A; Supplementary Table 1).
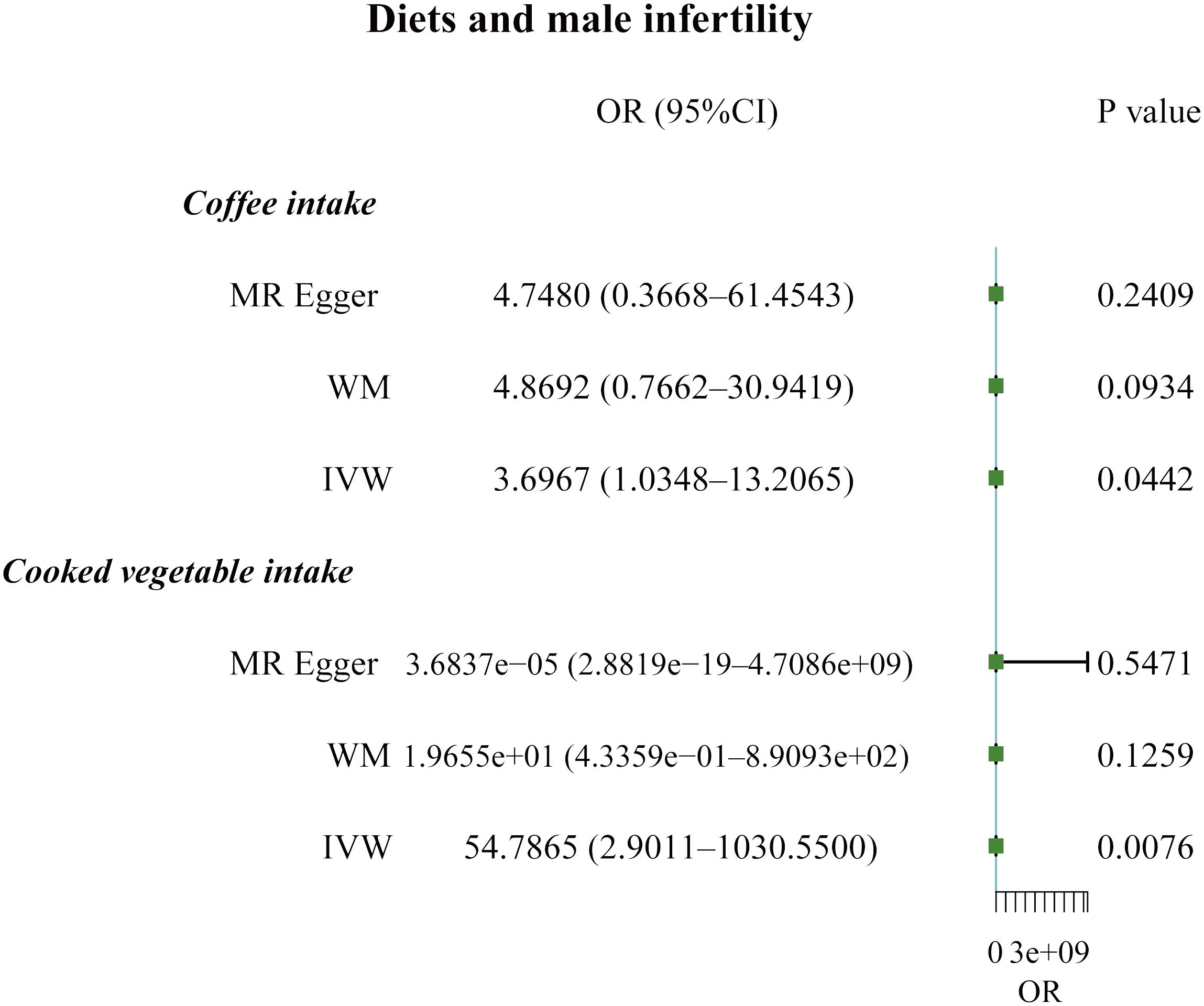
Figure 1 Forest plot of the causal associations between coffee and cooked vegetable intakes, and male infertility.
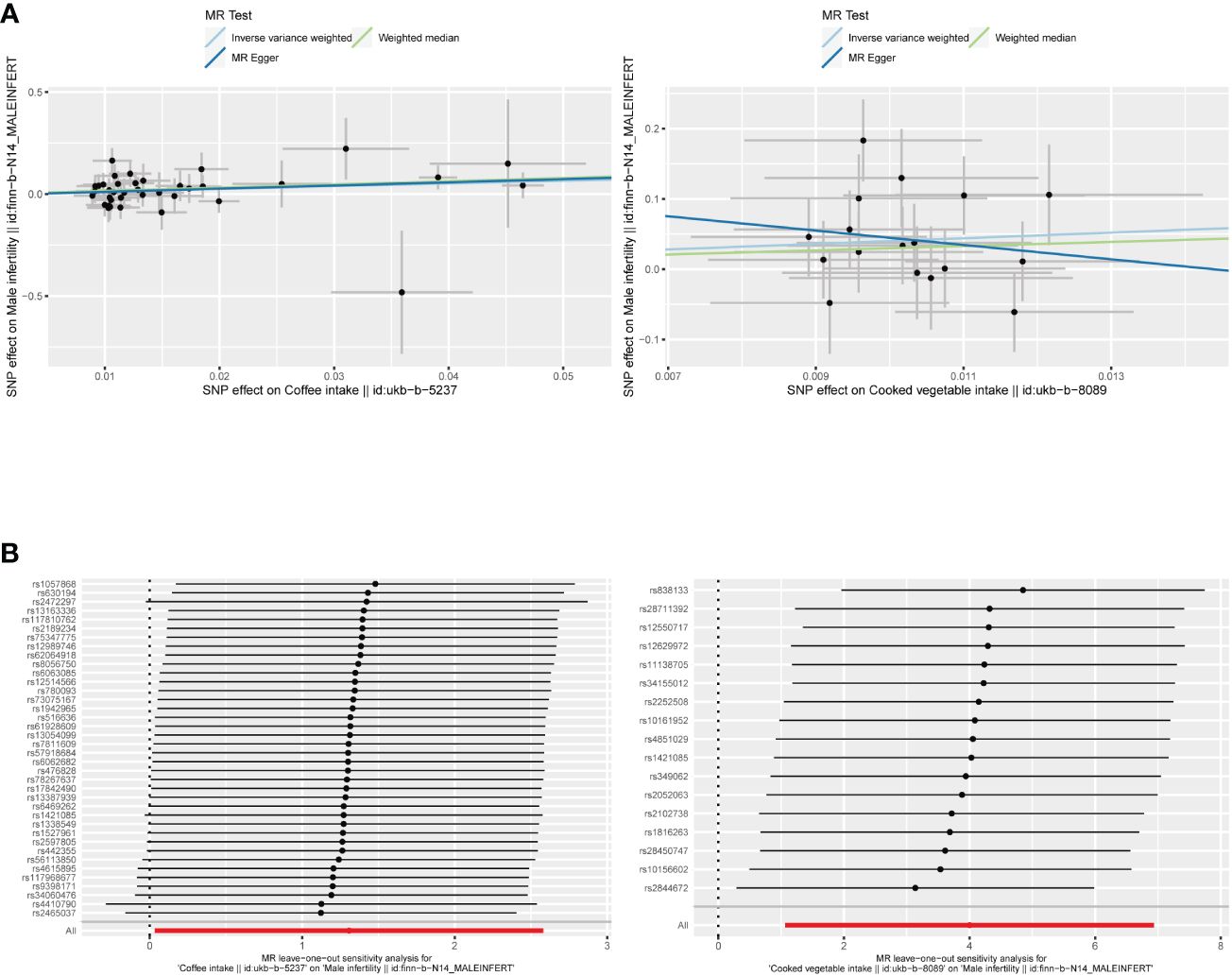
Figure 2 (A) Scatter plots of the causal relationship between coffee and cooked vegetable intakes, and male infertility. (B) The leave-one-out plots of the causal relationship between coffee and cooked vegetable intakes, and male infertility.
In sensitivity analysis, neither the IVW test nor the MR-Egger test showed any heterogeneity in the results of causality between all diets and male infertility (Supplementary Table 2). Additionally, the MR-Egger intercept test did not detect horizontal pleiotropy of the causality results, except for the relationship between processed meat intake and male infertility (P = 0.0262). The MR-PRESSO and radial MR tests found pleiotropy in lamb intake (P = 0.0460) alone (Figure 3, Table 2), and no significant outliers. Based on the leave-one-out analysis, no single SNP had a large impact on the robustness of the results after the individual removal tests (Figure 2B).
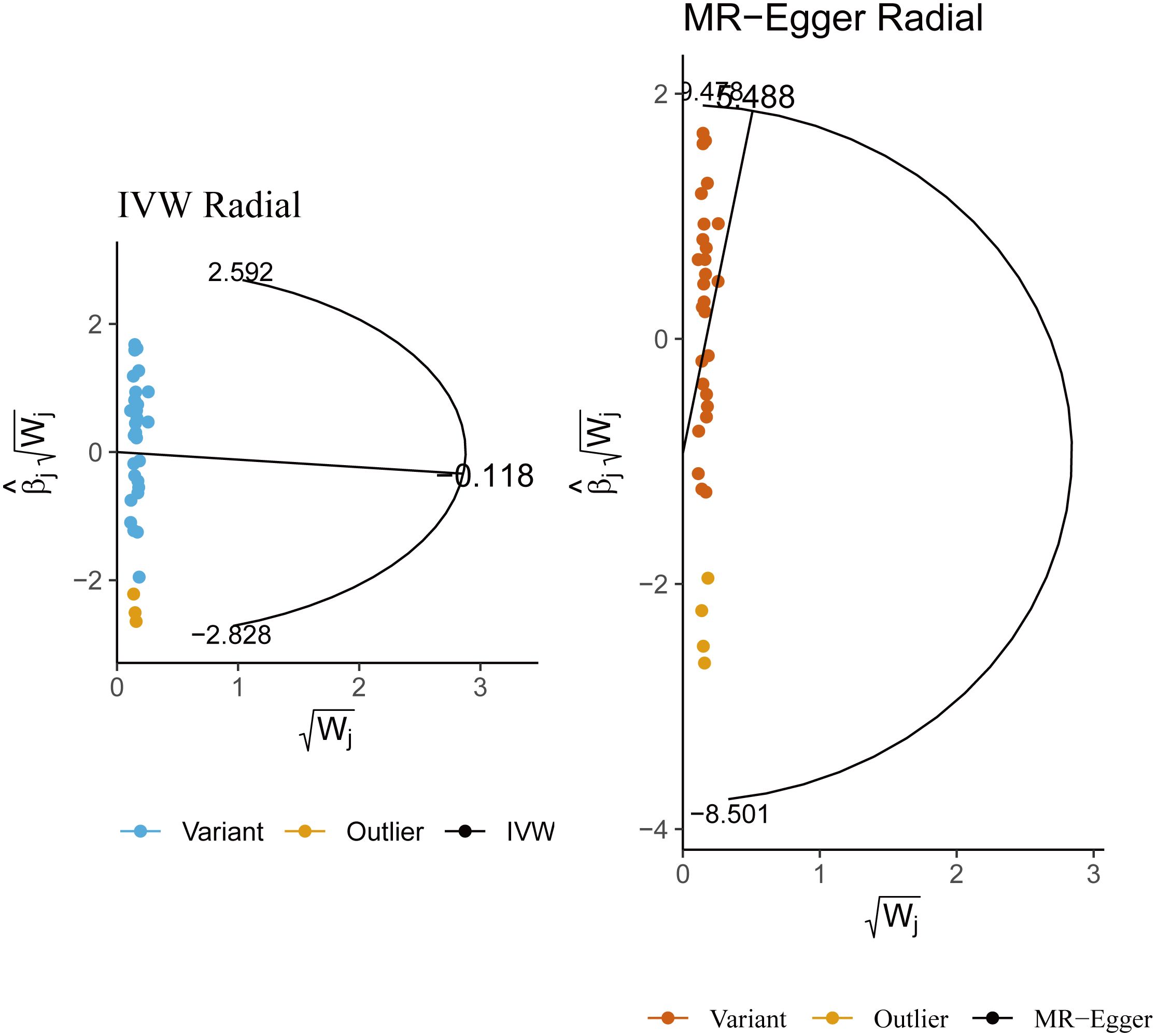
Figure 3 Radial Mendelian randomization analysis of the causal relationship between lamb intake and male infertility.
Diet and infertility in women
The F-statistic values for all IVs were higher than 10, avoiding the risk of weak instrumental bias (Table 1). TSMR analysis revealed a negative association between processed meat intake and female infertility, suggesting that processed meat reduced the risk of female infertility (OR, 0.5148; 95% CI, 0.2730 – 0.9705; P = 0.0401). Additionally, beer has been found to be a risk factor for female infertility (OR, 4.0932; 95% CI, 1.8728 – 8.9461; P = 0.0004) (Figures 4, 5A; Supplementary Table 3).
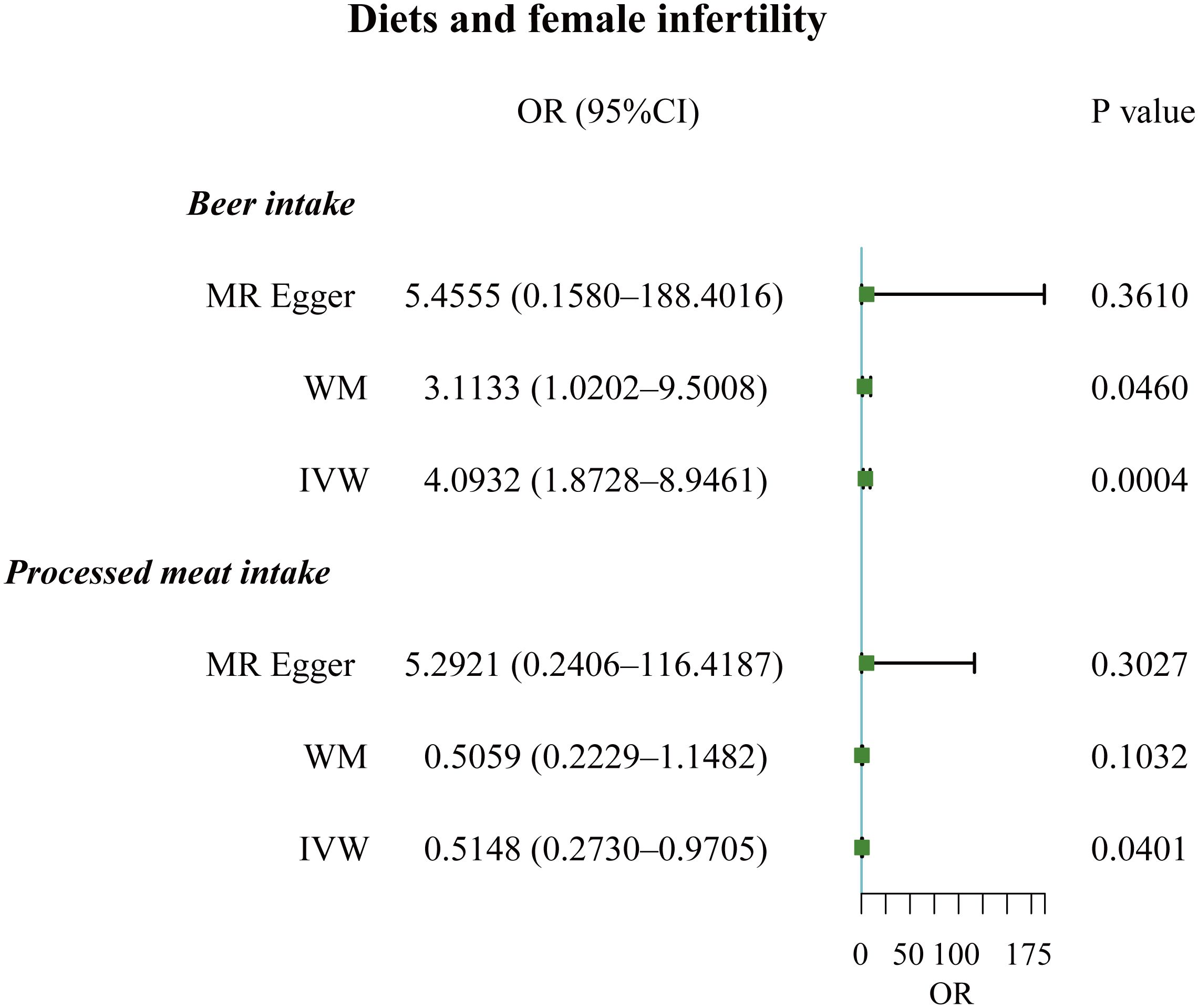
Figure 4 Forest plot of the causal associations between beer and processed meat intakes, and female infertility.
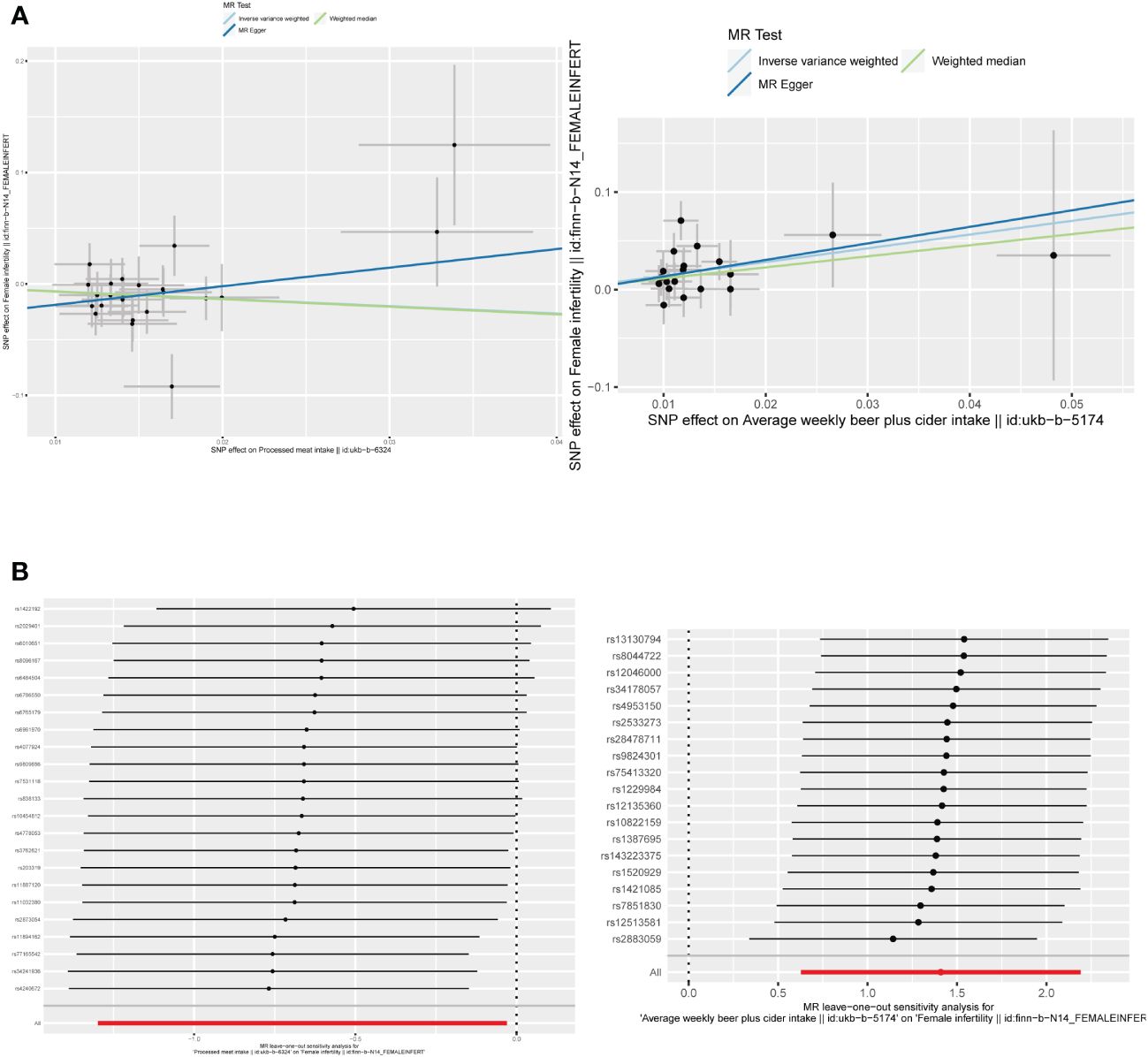
Figure 5 (A) Scatter plots of the causal relationship between beer and processed meat intakes, and female infertility. (B) The leave-one-out plots of the causal relationship between beer and processed meat intakes, and female infertility.
By employing Cochran’s Q test, we found evidence of heterogeneity in bread, cereal, coffee, salted peanuts, and red wine intakes. No horizontal pleiotropy was detected in the MR-Egger intercept test (Supplementary Table 4). Additionally, radial MR and MR-PRESSO global test found outliers in coffee and red wine intakes (Figure 6, Table 2). After excluding outliers (rs13163336 for coffee intake; rs62573521 for red wine intake), the original causality did not change (Supplementary Table 5). Based on the leave-one-out analysis, no single SNP had a large impact on the robustness of the results after the individual removal tests (Figure 5B).
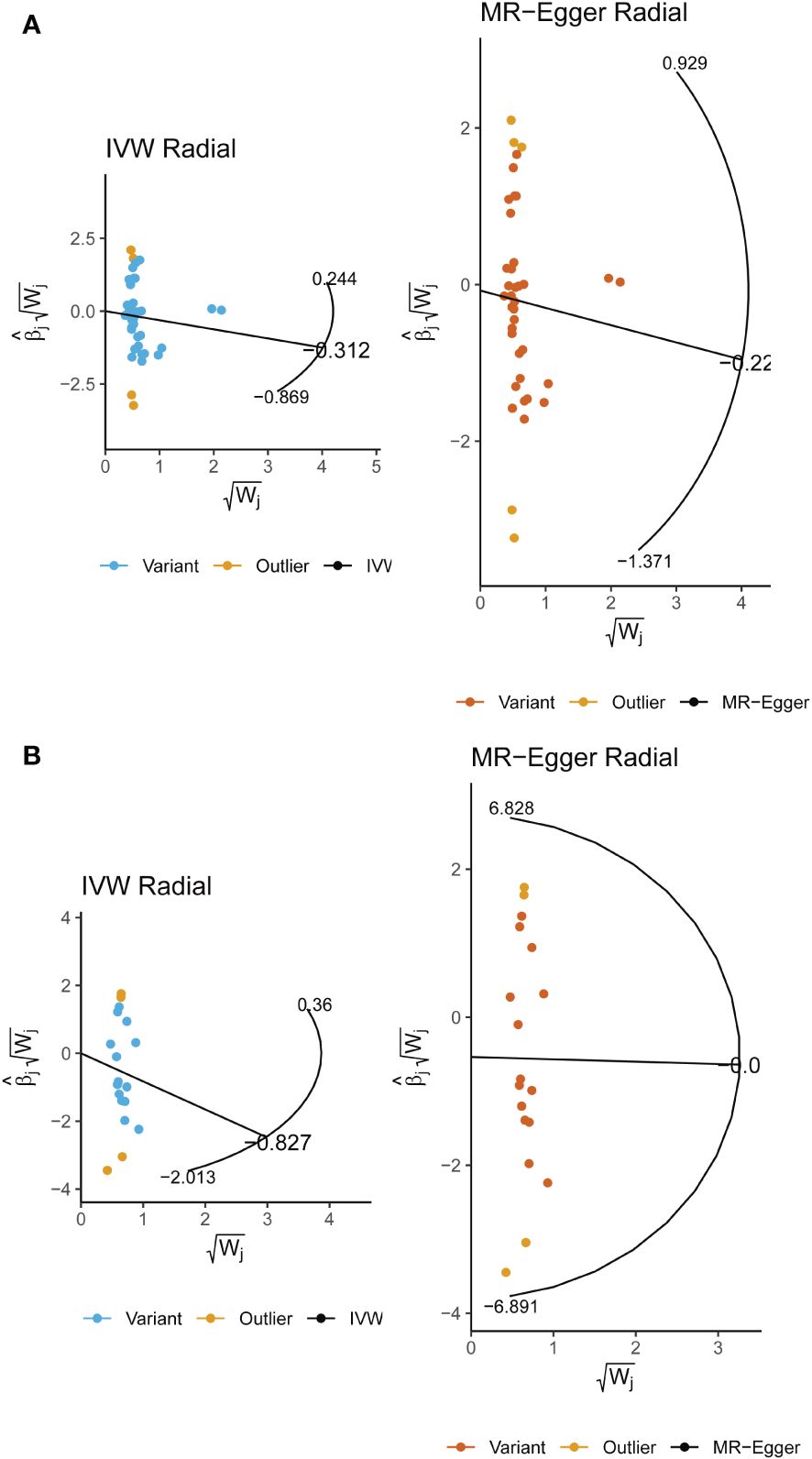
Figure 6 (A) Radial Mendelian randomization analysis of the causal relationship between coffee intake and female infertility. (B) Radial Mendelian randomization analysis of the causal relationship between red wine intake and female infertility.
Multivariate mendelian randomization
To examine whether diet impacts infertility by working through other factors, we performed MVMR analyses (Supplementary Table 6). After adjusting for zinc and selenium, cooked vegetable intake remained as having a significant negative effect on male infertility (OR, 34.3751; 95% CI, 2.1094 – 560.1708; P = 0.0130). For women, we found that processed meat (OR, 0.3718; 95% CI, 0.1876 – 0.7367; P = 0.0046) and selenium (OR, 7.4474e-12; 95% CI, 5.4780e-22 – 1.0125e-01; P = 0.0314) reduced the risk of female infertility after adjustments. When adjusted for monounsaturated fatty acids, polyunsaturated fatty acids, and saturated fatty acids, processed meat intake still displayed a protective effect against female infertility (OR, 0.4875; 95% CI, 0.2600 – 0.9142; P = 0.0251).
Discussion
The present study investigated the impact of diet on male and female infertility using TSMR, MVMR, and radial MR methods. Our data showed that coffee and cooked vegetables increased the risk of male infertility while beer intake positively associated with female infertility. Processed meat intake was found to be protective against female infertility. Additionally, selenium may improve female fertility.
Reproduction, nutrition, and energy metabolism are closely linked and regulate each other (17). Nutritional imbalance such as excessive calorie intake and malnutrition, coupled with lack of exercise, can cause an accumulation of body fat that in turn may lead to non-communicable diseases such as diabetes, cancer, and cardiovascular disease (18, 19). These lifestyle factors can negatively impact male fertility by decreasing sperm quality, including semen volume and sperm motility (20). For women, an unhealthy diet can negatively affect menstruation, fertility, and maternal and fetal health (21).
Coffee is widely consumed around the world, and whether its effects on fertility are large or small, it may have serious public health implications. Current research disputes an impact of coffee or caffeine on fertility. A systematic review involving 19,967 men found that semen parameters were unaffected by caffeine intake, primarily from coffee, tea, and cocoa beverages, in most studies (22). Another study supported this idea by observing no association between moderate caffeine (≤800mg/d) or cola (≤1L/d) intake and decreased semen quality (23). However, Belloc et al. found that nearly 76% of caffeine consumers (3.0 ± 1.8 cups of coffee/day) noticed a slight increase in semen volume; and sperm viability was higher in men of childbearing age who drank six or more cups of coffee per day (24, 25). Most of the current research has focused solely on the effects of caffeine on fertility, but caffeine is widely available in tea, cola, and cocoa drinks that contain many other ingredients besides caffeine (26, 27). This means that it is difficult to separate spurious associations from potential causal associations, and that caffeine may not be the only factor in the effect of these beverages on fertility. Our study avoided confounders as much as possible and revealed the potentially harmful effects of coffee, but not caffeine, on male infertility. The mechanism by which coffee intake impairs male reproductive function is related to double-stranded DNA breaks and sperm aneuploidy, but not DNA adducts (28).
Vegetables are high in water content, certain minerals (like magnesium and potassium) that have antioxidant qualities, fiber, folate, and antioxidant-rich vitamins (like Vitamin A, Vitamin C, polyphenols, and β-carotene). Vegetable intake is known to be a reproductively beneficial part of diet. A cross-sectional study has confirmed that elevated vegetable consumption is associated with higher sperm concentration, motility, and total and progressive semen motility (29). In another case-control study, total vegetable intake was associated with a lower risk of idiopathic asthenozoospermia (30). Vegetables are rich in antioxidants that are used to reverse the negative effects of high reactive oxygen species (ROS) concentrations on sperm viability, vitality and concentration, as well as on miscarriages and abnormalities during offspring development (31). Moreover, folate that is found primarily in green leafy vegetables plays an important role in DNA maintenance as well as transfer RNA and protein synthesis. Given that DNA synthesis is an important component of spermatogenesis, it is likely that folic acid affects this process (32). However, few studies have distinguished between the effects of different cooking methods on vegetable efficacy. In the previously reported cooking-affected relationships between vegetables and noncommunicable diseases, for example, the causality of some vegetables and blood pressure can change with cooking style (33). Also, of the 11 studies on raw and cooked vegetables, nine showed a statistically significant negative association between these cancers and raw vegetable intake, but only four reports showed a negative association with cooked vegetables (34). These differences may stem from changes in food structure and nutrient availability, and a disruption of digestive enzymes (34). Our results demonstrated that an increased cooked vegetable intake resulted in infertility, and in conjunction with another similar study, that raw vegetables maintained or improved semen quality (35). Advising couples trying to conceive or people with infertility to use more raw vegetables appears to be beneficial.
A case-control study showed an increase in infertility due to ovulatory factors or endometriosis and moderate to high levels of alcohol use (36). In another prospective study, alcohol intake was associated with reduced fertility, even among women who drank five or fewer drinks per week (37). A recent dose-response meta-analysis confirmed this idea, with a linear association between reduced fertility and each 12.5 g/day increase in alcohol consumption (38). Many past publications have revealed an association between alcohol consumption and fertility; however, the results are largely controversial (39, 40). This inconsistency may stem from the type of alcohol consumption, the dose of alcohol consumed, sample characteristics, and study design. Furthermore, alcohol consumption has been found to negatively affect sperm quality (41–43). Heavy drinkers have increased rates of chromatin de-condensation and sperm DNA fragmentation (44, 45). It is also important to emphasize the effects of alcohol consumption on the offspring, as women who consume alcohol during pregnancy cause the immature fetal brain exposed to ethanol to experience massive neuronal death, resulting in fetal alcohol spectrum disorders that are one of the leading causes of intellectual disability in western countries (46). Paternal alcohol consumption not only produces severe brain alterations in their offspring but is also associated with restricted reproductive development in the offspring and reduced placental efficiency (47–49).
Despite the inevitable loss of nutrients (such as zinc) from meat because of the cooking process, as well as the possible formation of unfavorable heterocyclic amines during processing leading to cell damage and loss of biological function, resulting in processed meat being reported to have adverse effects on male reproduction (50, 51). However, we found that processed meat may have a protective effect against female infertility. It is possible that as consumer demand for the health benefits of food has increased, the processed meat industry has developed low-fat, healthy meat products reformulated by substituting vegetable oils for animal fats, and that this trend has led to a modest improvement in human fertility (52). Moreover, we have demonstrated the application of zinc in promoting reproductive health, while meat, especially liver and kidney, is particularly high in selenium; this may also explain the reproductive benefits of processed meat (53).
The role of subjective confounding factors in the effect of diet on infertility is still not negligible. Inadequate diets, whether low calorie or unhealthy excess calorie intake, negatively affects physiological reproductive function and greatly increases the risk of infertility (54–56). High body mass index (BMI) interferes with reproductive potential. Men with obesity have decreased total and bioavailable testosterone levels (57); and central, peripheral, and testicular factors affect the hypothalamic-pituitary-gonadal (HPG) axis, contributing to the secondary hypogonadism, which may lead to reduced sexual desire, erectile dysfunction, and impaired spermatogenesis (57). In women, excess adipose tissue leads to negative feedback on the hypothalamic-pituitary-ovarian (HPO) axis and affects gonadotropin production (58). This manifests as menstrual abnormalities and ovulatory dysfunction (59). Moreover, polycystic ovary syndrome (PCOS) is a common endocrine disorder in women, and patients are usually overweight and have menstrual irregularities. And the triad of obesity, infertility and PCOS is reported to be inextricably linked, and its prevalence is rising (60). Obesity can lead to insulin resistance that exacerbates the symptoms of PCOS, in that the adverse effects of PCOS are more prevalent in obese women (61). Additionally, insulin resistance contributes to ovulation disorders and abnormal endometrial structure (62, 63). As discussed above, factors such as metabolic disorders and hormonal imbalancement still play a role in the effects of diet on infertility, suggesting that the use of causal language is necessary when discussing the link between diet and infertility. Although the results of the analysis based on the three assumptions of MR are stable and reliable, given the variety of confounders with large sample sizes, we do not have assessment parameters available for these factors. Therefore, it is essential for clinicians to consider the triad of diet, the above factors and infertility when developing prevention or treatment options. This also suggests that individualized diets should be tailored to the patients, rather than specific recipes. Our study has some limitations. First, the participants of the GWAS data we utilized were predominantly from Europe, and the applicability of the causal relationships in the study to populations in other regions still requires further validation. Second, the level of food intake is not known, and the amount of intake may bias the results. Finally, not all participants were of childbearing age, and the effect of age on fertility may outweigh dietary effects. Our study revealed the impact of diet on infertility in men and women and provided clinicians with an inexpensive and concise option for guiding couples of childbearing age to prevent infertility. In the future, we hope to design better prospective studies with larger sample sizes to validate our conclusions.
Conclusion
We utilized TSMR and MVMR methods to explore the effect of diet on infertility. We found that coffee and cooked vegetable intakes increased the risk of male infertility. And there was a positive correlation between beer intake and female infertility. But processed meat was negatively associated with female infertility. Moreover, MVMR found selenium to be a protective factor for female infertility. We expect prospective studies with larger samples to justify our conclusions in the future.
Data availability statement
The datasets presented in this study can be found in online repositories. The names of the repository/repositories and accession number(s) can be found in the article/Supplementary Material.
Ethics statement
Ethical approval was not required for the study involving humans in accordance with the local legislation and institutional requirements. Written informed consent to participate in this study was not required from the participants or the participants’ legal guardians/next of kin in accordance with the national legislation and the institutional requirements.
Author contributions
XC: Data curation, Formal analysis, Methodology, Resources, Software, Visualization, Writing – original draft, Writing – review & editing. CR: Software, Writing – original draft. CW: Methodology, Writing – original draft. XL: Methodology, Writing – original draft, Writing – review & editing.
Funding
The author(s) declare that no financial support was received for the research, authorship, and/or publication of this article.
Acknowledgments
We appreciate the support of Tianjin Medical University.
Conflict of interest
The authors declare that the research was conducted in the absence of any commercial or financial relationships that could be construed as a potential conflict of interest.
Publisher’s note
All claims expressed in this article are solely those of the authors and do not necessarily represent those of their affiliated organizations, or those of the publisher, the editors and the reviewers. Any product that may be evaluated in this article, or claim that may be made by its manufacturer, is not guaranteed or endorsed by the publisher.
Supplementary material
The Supplementary Material for this article can be found online at: https://www.frontiersin.org/articles/10.3389/fendo.2024.1376800/full#supplementary-material
References
1. Minhas S, Bettocchi C, Boeri L, Capogrosso P, Carvalho J, Cilesiz NC, et al. European association of urology guidelines on male sexual and reproductive health: 2021 update on male infertility. Eur Urol. (2021) 80:603–20. doi: 10.1016/j.eururo.2021.08.014
2. Vander Borght M, Wyns C. Fertility and infertility: Definition and epidemiology. Clin Biochem. (2018) 62:2–10. doi: 10.1016/j.clinbiochem.2018.03.012
3. Agarwal A, Baskaran S, Parekh N, Cho C-L, Henkel R, Vij S, et al. Male infertility. Lancet. (2021) 397:319–33. doi: 10.1016/S0140-6736(20)32667-2
4. Wu AK, Elliott P, Katz PP, Smith JF. Time costs of fertility care: the hidden hardship of building a family. Fertil Steril. (2013) 99:2025–30. doi: 10.1016/j.fertnstert.2013.01.145
5. Fauser BC. Towards the global coverage of a unified registry of IVF outcomes. Reprod Biomed Online. (2019) 38:133–7. doi: 10.1016/j.rbmo.2018.12.001
6. Sundaram R, Mumford SL, Buck Louis GM. Couples’ body composition and time-to-pregnancy. Hum Reprod. (2017) 32:662–8. doi: 10.1093/humrep/dex001
7. Skoracka K, Eder P, Łykowska-Szuber L, Dobrowolska A, Krela-Kaźmierczak I. Diet and nutritional factors in male (in) fertility—underestimated factors. J Clin Med. (2020) 9:1400. doi: 10.3390/jcm9051400
8. Ferramosca A, Zara V. Diet and male fertility: The impact of nutrients and antioxidants on sperm energetic metabolism. Int J Mol Sci. (2022) 23:2542. doi: 10.3390/ijms23052542
9. Crean AJ, Senior AM. High-fat diets reduce male reproductive success in animal models: A systematic review and meta-analysis. Obes Rev. (2019) 20:921–33. doi: 10.1111/obr.12827
10. Nematollahi A, Kazeminasab F, Tavalaee M, Marandi SM, Ghaedi K, Nazem MN, et al. Effect of aerobic exercise, low-fat and high-fat diet on the testis tissue and sperm parameters in obese and nonobese mice model. Andrologia. (2019) 51:e13273. doi: 10.1111/and.13273
11. Chavarro JE, Rich-Edwards JW, Rosner BA, Willett WC. Diet and lifestyle in the prevention of ovulatory disorder infertility. Obstet Gynecol. (2007) 110:1050–8. doi: 10.1097/01.AOG.0000287293.25465.e1
12. Firns S, Cruzat VF, Keane KN, Joesbury KA, Lee AH, Newsholme P, et al. The effect of cigarette smoking, alcohol consumption and fruit and vegetable consumption on IVF outcomes: a review and presentation of original data. Reprod Biol Endocrinol. (2015) 13:1–13. doi: 10.1186/s12958-015-0133-x
13. Davies NM, Holmes MV, Smith GD. Reading Mendelian randomisation studies: a guide, glossary, and checklist for clinicians. Bmj. (2018) 362:k601. doi: 10.1136/bmj.k601
14. Gagliano Taliun SA, Evans D. M. J. P. C. B. Ten simple rules for conducting a mendelian randomization study. CA USA: Public Library of Science San Francisco (2021). doi: 10.1371/journal.pcbi.1009238
16. Burgess S, Butterworth A, Thompson SG. Mendelian randomization analysis with multiple genetic variants using summarized data. Genet Epidemiol. (2013) 37:658–65. doi: 10.1002/gepi.21758
17. Torre SD, Benedusi V, Fontana R, Maggi A. Energy metabolism and fertility—a balance preserved for female health. Nat Rev Endocrinol. (2014) 10:13–23. doi: 10.1038/nrendo.2013.203
18. Sharma R, Biedenharn KR, Fedor JM, Agarwal A. Lifestyle factors and reproductive health: taking control of your fertility. Eproductive Biol Endocrinol. (2013) 11:1–15. doi: 10.1186/1477-7827-11-66
19. Ilacqua A, Izzo G, Emerenziani GP, Baldari C, Aversa A. Lifestyle and fertility: the influence of stress and quality of life on male fertility. Reprod Biol Endocrinol. (2018) 16:1–11. doi: 10.1186/s12958-018-0436-9
20. Bisconti M, Simon J-F, Grassi S, Leroy B, Martinet B, Arcolia V, et al. Influence of risk factors for male infertility on sperm protein composition. Int J Mol Sci. (2021) 22:13164. doi: 10.3390/ijms222313164
21. Stewart DE, Robinson GE, Goldbloom DS, Wright C. Infertility and eating disorders. Am J Obstet Gynecol. (1990) 163:1196–9. doi: 10.1016/0002-9378(90)90688-4
22. Ricci E, Viganò P, Cipriani S, Somigliana E, Chiaffarino F, Bulfoni A, et al. Coffee and caffeine intake and male infertility: a systematic review. Nutr J. (2017) 16:1–14. doi: 10.1186/s12937-017-0257-2
23. Jensen TK, Swan SH, Skakkebæk NE, Rasmussen S, Jørgensen N. Caffeine intake and semen quality in a population of 2,554 young Danish men. Am J Epidemiol. (2010) 171:883–91. doi: 10.1093/aje/kwq007
24. Sobreiro BP, Lucon AM, Pasqualotto FF, Hallak J, Athayde KS, Arap S. Semen analysis in fertile patients undergoing vasectomy: reference values and variations according to age, length of sexual abstinence, seasonality, smoking habits and caffeine intake. Sao Paulo Med J. (2005) 123:161–6. doi: 10.1590/S1516-31802005000400002
25. Belloc S, Cohen-Bacrie M, Dalleac A, Amar E, Hazout A, De Mouzon J. Caffeine intake and sperm parameters. Analysis of a cohort of 4474 consecutive semen samples. Fertil Steril. (2013) 100:S212. doi: 10.1016/j.fertnstert.2013.07.1331
26. Li Y, Lin H, Li Y, Cao J. Association between socio-psycho-behavioral factors and male semen quality: systematic review and meta-analyses. Fertil Steril. (2011) 95:116–23. doi: 10.1016/j.fertnstert.2010.06.031
27. Karmon AE, Toth T, Chiu YH, Gaskins A, Tanrikut C, Wright D, et al. Male caffeine and alcohol intake in relation to semen parameters and in vitro fertilization outcomes among fertility patients. Andrology. (2017) 5:354–61. doi: 10.1111/andr.12310
28. Schmid T, Eskenazi B, Baumgartner A, Marchetti F, Young S, Weldon R, et al. The effects of male age on sperm DNA damage in healthy non-smokers. Hum Reprod. (2007) 22:180–7. doi: 10.1093/humrep/del338
29. Madej D, Granda D, Sicinska E, Kaluza J. Influence of fruit and vegetable consumption on antioxidant status and semen quality: a cross-sectional study in adult men. Front Nutr. (2021) 8:753843. doi: 10.3389/fnut.2021.753843
30. Eslamian G, Amirjannati N, Rashidkhani B, Sadeghi M-R, Hekmatdoost A. Intake of food groups and idiopathic asthenozoospermia: a case–control study. Hum Reprod. (2012) 27:3328–36. doi: 10.1093/humrep/des311
31. Ross C, Morriss A, Khairy M, Khalaf Y, Braude P, Coomarasamy A, et al. A systematic review of the effect of oral antioxidants on male infertility. Reprod Biomed Online. (2010) 20:711–23. doi: 10.1016/j.rbmo.2010.03.008
32. Koury MJ, Ponka P. New insights into erythropoiesis: the roles of folate, vitamin B12, and iron. Annu Rev Nutr. (2004) 24:105–31. doi: 10.1146/annurev.nutr.24.012003.132306
33. Chan Q, Stamler J, Brown IJ, Daviglus ML, Van Horn L, Dyer AR, et al. Relation of raw and cooked vegetable consumption to blood pressure: the INTERMAP Study. J Hum Hypertension. (2014) 28:353–9. doi: 10.1038/jhh.2013.115
34. Link LB, Potter JD. Raw versus cooked vegetables and cancer risk. Cancer Epidemiol Biomarkers Prev. (2004) 13:1422–35. doi: 10.1158/1055-9965.1422.13.9
35. Mendiola J, Torres-Cantero AM, Moreno-Grau JM, Ten J, Roca M, Moreno-Grau S, et al. Food intake and its relationship with semen quality: a case-control study. Fertil Steril. (2009) 91:812–8. doi: 10.1016/j.fertnstert.2008.01.020
36. Grodstein F, Goldman MB, Cramer DW. Infertility in women and moderate alcohol use. Am J Public Health. (1994) 84:1429–32. doi: 10.2105/AJPH.84.9.1429
37. Jensen TK, Hjollund NHI, Henriksen TB, Scheike T, Kolstad H, Giwercman A, et al. Does moderate alcohol consumption affect fertility? Follow up study among couples planning first pregnancy. Bmj. (1998) 317:505–10. doi: 10.1136/bmj.317.7157.505
38. Fan D, Liu L, Xia Q, Wang W, Wu S, Tian G, et al. Female alcohol consumption and fecundability: a systematic review and dose-response meta-analysis. Sci Rep. (2017) 7:13815. doi: 10.1038/s41598-017-14261-8
39. Lopez-Del Burgo C, Gea A, De Irala J, Martínez-González MA, Chavarro JE, Toledo E. Alcohol and difficulty conceiving in the SUN cohort: a nested case-control study. Nutrients. (2015) 7:6167–78. doi: 10.3390/nu7085278
40. Mikkelsen EM, Riis AH, Wise LA, Hatch EE, Rothman KJ, Cueto HT, et al. Alcohol consumption and fecundability: prospective Danish cohort study. Bmj. (2016) 354:i4262. doi: 10.1136/bmj.i4262
41. Borges JE, Braga D. P. D. A. F., Provenza RR, Figueira RDCS, Iaconelli A Jr., Setti AS. Paternal lifestyle factors in relation to semen quality and in vitro reproductive outcomes. Andrologia. (2018) 50:e13090. doi: 10.1111/and.2018.50.issue-9
42. Boeri L, Capogrosso P, Ventimiglia E, Pederzoli F, Cazzaniga W, Chierigo F, et al. Heavy cigarette smoking and alcohol consumption are associated with impaired sperm parameters in primary infertile men. Asian J Androl. (2019) 21:478. doi: 10.4103/aja.aja_110_18
43. Bai S, Wan Y, Zong L, Li W, Xu X, Zhao Y, et al. Association of alcohol intake and semen parameters in men with primary and secondary infertility: a cross-sectional study. Front Physiol. (2020) 11:566625. doi: 10.3389/fphys.2020.566625
44. Anifandis G, Bounartzi T, Messini C, Dafopoulos K, Sotiriou S, Messinis I. The impact of cigarette smoking and alcohol consumption on sperm parameters and sperm DNA fragmentation (SDF) measured by Halosperm®. Arch Gynecol Obstet. (2014) 290:777–82. doi: 10.1007/s00404-014-3281-x
45. Aboulmaouahib S, Madkour A, Kaarouch I, Sefrioui O, Saadani B, Copin H, et al. Impact of alcohol and cigarette smoking consumption in male fertility potential: Looks at lipid peroxidation, enzymatic antioxidant activities and sperm DNA damage. Andrologia. (2018) 50:e12926. doi: 10.1111/and.2018.50.issue-3
46. Granato A, Dering B. Alcohol and the developing brain: why neurons die and how survivors change. Int J Mol Sci. (2018) 19:2992. doi: 10.3390/ijms19102992
47. Ceccanti M, Coccurello R, Carito V, Ciafrè S, Ferraguti G, Giacovazzo G, et al. Paternal alcohol exposure in mice alters brain NGF and BDNF and increases ethanol-elicited preference in male offspring. Addict Biol. (2016) 21:776–87. doi: 10.1111/adb.2016.21.issue-4
48. Xia R, Jin L, Li D, Liang H, Yang F, Chen J, et al. Association between paternal alcohol consumption before conception and anogenital distance of offspring. Alcoholism: Clin Exp Res. (2018) 42:735–42. doi: 10.1111/acer.13595
49. Chang RC, Wang H, Bedi Y, Golding MCJE, Chromatin. Preconception paternal alcohol exposure exerts sex-specific effects on offspring growth and long-term metabolic programming. Epigenet Chromatin. (2019) 12:1–17. doi: 10.1186/s13072-019-0254-0
50. Badiani A, Stipa S, Bitossi F, Gatta P, Vignola G, Chizzolini R. Lipid composition, retention and oxidation in fresh and completely trimmed beef muscles as affected by common culinary practices. Meat Sci. (2002) 60:169–86. doi: 10.1016/S0309-1740(01)00119-X
51. Carvalho A, Miranda A, Santos F, Loureiro APDM, Fisberg RM, Marchioni D. High intake of heterocyclic amines from meat is associated with oxidative stress. Br J Nutr. (2015) 113:1301–7. doi: 10.1017/S0007114515000628
52. Badar IH, Liu H, Chen Q, Xia X, Kong B. Future trends of processed meat products concerning perceived healthiness: A review. Compr Rev Food Sci Food Saf. (2021) 20:4739–78. doi: 10.1111/1541-4337.12813
53. Mojadadi A, Au A, Salah W, Witting P, Ahmad G. Role for selenium in metabolic homeostasis and human reproduction. Nutrients. (2021) 13:3256. doi: 10.3390/nu13093256
54. Silvestris E, De Pergola G, Rosania R, Loverro G. Obesity as disruptor of the female fertility. Reprod Biol Endocrinol. (2018) 16:1–13. doi: 10.1186/s12958-018-0336-z
55. Guo D, Xu M, Zhou Q, Wu C, Ju R, Dai J. Is low body mass index a risk factor for semen quality? A PRISMA-compliant meta-analysis. Medicine. (2019) 98(32):e16677. doi: 10.1097/MD.0000000000016677
56. Carson SA, Kallen AN. Diagnosis and management of infertility: a review. Jama. (2021) 326:65–76. doi: 10.1001/jama.2021.4788
57. Du Plessis SS, Cabler S, Mcalister DA, Sabanegh E, Agarwal A. The effect of obesity on sperm disorders and male infertility. Nat Rev Urol. (2010) 7:153–61. doi: 10.1038/nrurol.2010.6
58. Rachoń D, Teede H. Ovarian function and obesity—interrelationship, impact on women’s reproductive lifespan and treatment options. Mol Cell Endocrinol. (2010) 316:172–9. doi: 10.1016/j.mce.2009.09.026
59. Jungheim ES, Moley KH. Current knowledge of obesity’s effects in the pre-and periconceptional periods and avenues for future research. Am J Obstet Gynecol. (2010) 203:525–30. doi: 10.1016/j.ajog.2010.06.043
60. Talmor A, Dunphy B. Female obesity and infertility. Best Pract Res Clin Obstet Gynaecol. (2015) 29:498–506. doi: 10.1016/j.bpobgyn.2014.10.014
61. Moran LJ, Norman RJ, Teede HJ. Metabolic risk in PCOS: phenotype and adiposity impact. Trends Endocrinol Metab. (2015) 26:136–43. doi: 10.1016/j.tem.2014.12.003
62. Chen W, Pang Y. Metabolic syndrome and PCOS: Pathogenesis and the role of metabolites. Metabolites. (2021) 11:869. doi: 10.3390/metabo11120869
Keywords: DIETS, male infertility, female infertility, mendelian randomization, GWAS
Citation: Chen X, Ren C, Wu C and Liu X (2024) Mendelian randomization reveals the impact of diet on infertility in men and women. Front. Endocrinol. 15:1376800. doi: 10.3389/fendo.2024.1376800
Received: 26 January 2024; Accepted: 10 April 2024;
Published: 23 April 2024.
Edited by:
Yulei Wei, China Agricultural University, ChinaReviewed by:
Marco Ghezzi, Independent Researcher, Albignasego, ItalyYuxuan Song, Peking University People’s Hospital, China
Copyright © 2024 Chen, Ren, Wu and Liu. This is an open-access article distributed under the terms of the Creative Commons Attribution License (CC BY). The use, distribution or reproduction in other forums is permitted, provided the original author(s) and the copyright owner(s) are credited and that the original publication in this journal is cited, in accordance with accepted academic practice. No use, distribution or reproduction is permitted which does not comply with these terms.
*Correspondence: Xiaoqiang Liu, eGlhb3FpYW5nbGl1MUAxNjMuY29t; Y3h5MTcxNzRAdG11LmVkdS5jbg==
†These authors contributed equally to this work and share first authorship