- 1Global Centre for Environmental Remediation (GCER), College of Science, Engineering and Environment, The University of Newcastle, Callaghan, NSW, Australia
- 2Cooperative Research Centre for Contamination Assessment and Remediation of the Environment (CRC CARE), The University of Newcastle, Callaghan, NSW, Australia
- 3Department of Biotechnology, Sher-e-Bangla Agricultural University, Dhaka, Bangladesh
- 4Institute of Medical and Biomedical Education, St George’s University of London, London, United Kingdom
Farmers use wastewater for irrigation in many developing countries, for example Bangladesh, India, China, Sri Lanka and Vietnam because they have limited access to clean water. This study explored cadmium (Cd) bioaccumulation in two spring wheat cultivars (cv. Mustang and Lancer), which were grown in different concentrations of Cd (0,1, 2, 4, and 8 mg kg−1) in agricultural soils. The half maximum inhibitory concentration (IC50) values were 4.21 ± 0.29 and 4.02 ± 0.95, respectively, whereas the maximum health risk index (HRI) was 3.85 ± 0.049 and 5.33 ± 0.271, respectively, for Mustang and Lancer. In other words, the malondialdehyde content increased significantly in Mustang (around five-fold) and Lancer (around four-fold) compared with the control treatment. Results revealed that Cd content was well above the acceptable limit (HRI >1) in the two cultivars when exposed to different levels of Cd stress. The tolerant cultivar (Mustang) has potential to chelate Cd in the nonedible parts of plants in variable fractions and can be used efficiently to improve growth and macro- and micro-nutrients content while reducing Cd concentration in plants in Cd-contaminated soil. It can also diminish the HRI, which may help to protect humans from Cd risks. The two cultivars’ nutrient availability and sorption capacity significantly shape their survival and adaptability under Cd stress. Based on what is documented in the current study, we can conclude that Mustang is more tolerant and poses fewer health hazards to people than Lancer because of its capacity to maintain grain macro- and micro-nutrients under Cd stress.
Introduction
Irrigation with wastewater is a concern for farmers due to limited access to clean water for farming in many developing countries, for instance Bangladesh, India, China, Sri Lanka and Vietnam (Ansari and Malik, 2007; Qadir et al., 2010; Rezapour et al., 2019). One consequence of the Industrial Revolution’s effect on farming is that agricultural modernization has dangerously polluted agricultural soils with heavy metals including cadmium (Cd) (Singh et al., 2021). Cadmium is an unessential heavy metal, is highly toxic to plants and represents one of the most dangerous environmental challenges worldwide. The European Food Safety Authority recommended the tolerable daily intake is 0.358 μg Cd kg−1 body weight, which is lower than the World Health Organization’s (WHO’s) recommended value (25 µg kg−1 BW month−1) (Jinadasa et al., 2019). Cadmium is a toxic trace metal that is detrimental to different cereal crops including wheat since it can typically block the essential plant nutrients transport in different plant organs, such as roots, shoots and grain during seedlings’ emergence to through to their growth and developments stages (Mahawar et al., 2021). The bread wheat (Triticum aestivum L.) is the third most cultivable and earliest domesticated cereal crop worldwide, and it is grown in around 20% of cultivable agricultural soil globally (Mehta and Bharat, 2019). Hence, wheat, as a food consumed by most of the world’s population, if contaminated with Cd might be hazardous to humans. When Cd accumulates in the human body it poses a very serious cancer risk, kidney diseases and renal dysfunction (Hayat et al., 2019).
The concentrations of Cd in cultivated soils ranged from 0.11 to 6.37 mg kg−1, whereas in the background soil it ranged from 0.02 to 1.99 mg kg−1 in the Sydney region (Gray et al., 2019). The ANZ environmental investigation guideline for soil Cd is 3 mg kg−1 (Siddique et al., 2021). Ecological urban soil investigation level is 3 mg kg−1 although background Cd in soil is 1 mg kg−1 (Kandic et al., 2019). Moreover, wheat grain Cd concentrations exceed the Australian and New Zealand food standards for Cd when it is grown in soil with 0.6 mg Cd kg−1 soil (Gray et al., 2019). The low-Cd accumulating wheat cultivars with high macro- and micro-nutrients contents in plant biomass are the best way to diminish Cd concentration in wheat grain.
Cadmium could be available in the plant-soil interface because it is easily available with pore water and transported by plants’ roots from rhizosphere soil, then to the other vegetative and reproductive parts of the plant, and finally to the grains (Seyfferth et al., 2018). The small Cd concentrations can compromise wheat yield and quality when exposed for long periods of time to one of the most toxic contaminants in soil that can inhibit plant growth. The protease inhibitor, mitosis disruptions, discourages cell proliferation and division and mitochondrial disintegration which triggers the negative influence on chelators detoxification, cellular antioxidants activity, and signaling in reactive oxygen species (ROS). These in turn wield some effect on chlorophyll and MDA contents directly in wheat plants (Kaya et al., 2019; Qiao et al., 2019). The antagonistic effect of Cd on plant growth is a result of photosynthesis malfunctioning, which reduces antioxidants and increases lipid peroxidase, causing biomass reduction. Furthermore, resulting chlorosis impairs the uptake of nutrients, and ultimately causes yield decline and crop death (Hassan et al., 2019; Kabir et al., 2021). As well, crop plants generate free radicals and reactive oxygen species under Cd stress by antioxidant enzymes via different mechanisms inside the cell (Gill and Tuteja, 2010; Li et al., 2019). Plants can cope with Cd stress by various mechanisms, such as chelation and complexation of Cd with different protein and non-protein thiols and plant hormones such as auxin and cytokinin which minimize Cd toxicity in plants (Pál et al., 2018).
The plants can develop tolerance to Cd toxicity by developing morphophysiological parameters and adaptation to Cd stress. For example, a crop can prevent Cd absorption by blocking the elemental transport pathway and uptake of more antagonistic nutrients elements such as calcium (Ca), magnesium (Mg), potassium (K), zinc (Zn), iron (Fe), manganese (Mn) and copper (Cu) in its roots, shoots and grain. This process may decrease Cd uptake from polluted soil (Ondrasek et al., 2021). So for this reason it is crucial to recognize the mechanism of bread wheat cultivar responses and resistance to Cd stress, in order to improve cultivars’ growth and grain quality. By selecting Cd-tolerant and high nutrient-containing wheat grain, wheat-based foodstuffs have the potential to be Cd safe, thus protecting people’s health.
Understanding the tolerance level of wheat under different plant soil conditions is crucial to curtail Cd bioaccumulation in wheat grain and hence develop effective remediation strategies. It is hypothesized that potentiality of bread wheat cultivar can be achieved by accumulating higher macro- and micro-nutrients contents in grains to reduce Cd bioaccumulation. Most studies have been done on hydroponics to explore Cd bioaccumulation and tolerance concentration. To the best of our knowledge, no study has yet been conducted on soil to discover Cd inhibitory concentration trends in response to grain macro- and micro-nutrients under different levels of Cd. In this study, two commonly grown Australian spring wheat cultivars (Mustang and Lancer) were investigated to identify Cd tolerance levels and bioaccumulation in the plant soil ecosystem. In addition, the HRI was used to assess how toxic Cd was to humans under different Cd treatments of the examined wheat varieties.
Materials and Methods
Experimental Soil Preparation
For pot experiment, soil (0–20 cm) was collected from the Patterson and Hunter regions of New South Wales, Australia. Soil physicochemical properties were determined by standard procedures including pH, electrical conductivity (EC); cation exchange capacity (CEC); carbon (C), nitrogen (N) and sulphur (S) contents; and aqua regia extractable total metals (Cd, Ca, Mg, K, Zn, Fe, Mn, and Cu). Results are listed in Table 1.
Plant Growth Study
Two commonly cultivated Australian spring wheat varieties (Mustang and Lancer), usually cultivated in northern and south-eastern zones (New South Wales and Queensland) of Australia were chosen for this study. Seeds were disinfected by 0.5% NaOCl (20 min) and rinsed carefully with ultrapure water. NPK fertilizers (i.e., N = 60 mg kg−1 soil, P2O5 = 30 mg kg−1 soil and K2O = 60 mg kg−1 soil) were applied as urea (46%N), triple superphosphate (TSP, 48% P2O5) and potassium chloride (KCl, 60% K2O), respectively. Uniform seeds were potted in 1.9 L plastic pots (five seeds per pot) filled with 1.5 kg of soil per pot. The soil was spiked with Cd to generate different concentration gradients of 0 (control), 1, 2, 4, and 8 mg/kg (120 days with Cd (NO3)2.4H2O). These were given the codes CK, Cd1, Cd2, Cd4 and Cd8, respectively. Soil Cd was extracted using 10 ml of 5 mmol L−1 diethylenetriaminepentaacetic acid, 100 mmol L−1 triethanolamine, and 10 mmol L−1 CaCl2 (DTPA) (pH 7.3) (Li et al., 2021) and aqua regia (Aqua regia extracted Cd) (Supplementary Table S1 in Supplementary Information). Plants were grown in pots in a glasshouse during October 2018 to February 2019 with a day and night temperature of day/night 25/16 ± 2°C and 65 ± 5% relative humidity. Each treatment had three replicates. Plant seedlings were grown to maturity stage, then plants were collected and stored for biomass and elemental (Cd, Ca, Mg, K, Zn, Fe, and Cu) analysis. Fresh leaves were collected at 50% anthesis stage (65 days after seedling emergence, DASE) for measuring the physiological parameters (stored at −20°C after treating with liquid nitrogen) such as chlorophyll content and lipid peroxidase (malondialdehyde MDA).
Plant Growth Parameters and Cd Bioaccumulation
The fresh harvested plant parts (roots, shoots and spikes) were soaked in distilled water to remove attached soil, rinsed with 20 mM Na2-EDTA (15 min) (Song et al., 2016), then washed thoroughly with ultrapure water (three times) to remove attached contaminants from root surfaces, and then split into roots, shoots, and spikes. Subsequently, plant samples were oven dried for 72 h at 70°C to generate completely dried samples. These dried samples were weighed (DW), ground and digested by following the digestion protocol for plant materials (Rahman et al., 2009). Cadmium, Zn and Cu were analyzed using ICP-MS (NEXION 350, Perkin Elmer, United States) while Ca, Mg, K, and Fe contents was analyzed using ICP-OES (Avio 200, Perkin Elmer). Growth parameters such as bioconcentration factors (BCFs), tolerance indices (TIs), translocation factors (TFs), and Cd bio-accumulation were determined as stated below (Khanna et al., 2019):
Analysis of Photosynthetic Products in Wheat Seedlings
The bread wheat flag leaves were harvested at 50% anthesis stage (65 DASE) and immediately immersed in liquid nitrogen for analysis of photosynthetic products.
Measurement of Chlorophyll (Chl a, Chl b and Total Chl) Contents
The determination of chlorophyll (Chl a, Chl b and total Chl) content was done using Arnon’s (Arnon, 1949) procedure. Briefly, 1,000 mg of fresh leaves were macerated in 4 ml acetone (80%). Following that, they were centrifuged at 16128 g for 15–20 min at 4°C. The supernatant was taken to determine chlorophyll content. Absorbance was measured at 649 and 655 nm. Chlorophyll a (Chl a) and Chlorophyll b (Chl b) were estimated using the following equations (Xu et al., 2021), respectively:
Analysis of Lipid Peroxidation
The lipid peroxidase (MDA) levels in the fresh leaves were estimated based on MDA content, which was measured following the method of Shalata and Neumann (Shalata and Neumann, 2001). Briefly, 200 mg of collected and stored fresh leaf samples were homogenized in trichloroacetic acid (TCA, 10% w/v), centrifuged at 4,000 rpm (10 min) and then monitored for homogenized supernatants. Then, the supernatant (2 ml) was interspersed with 2 ml 0.6% (w/v) TCA and incubated for 15 min in boiling water. MDA content was measured by the absorbance at 600 nm, 532 and 450 nm and determined based on a fresh weight (mmol g−1 FW) basis. MDA was measured using following formula:
Estimation of Health Risk Index
The HRI of Cd was estimated by calculating daily intake of Cd (DIC) and dividing it with oral reference doses (RfD) of Cd. The RfD value for Cd was 0.003 mg kg−1 body weight day−1, respectively (Epa, 1989; Arisekar et al., 2020).
Daily intake of Cd was estimated by Equation (2).
Where, CCd, Cfactor, Dfood-intake and Baverage-weight represent Cd concentration in grains (mg kg−1), conversion factor (0.085) (Rizwan et al., 2017b), wheat grains consumption daily (g day−1 person−1) and average body weight (kg), respectively. An average body weight of 70 kg and consumption of wheat flour was 219 g day−1 per person−1 (Du et al., 2013).
Quality Control and Assurance
Montana soil (SRM 2711a) and spinach leaves (SRM 1570a) were used as standard reference materials (SRMs) from the NIST [National Institute of Standard and Technology, United States of America (USA)] to validate the elemental concentrations for soil and wheat samples using the same extraction techniques employed for samples. The standards studied were consistent with the standard values, with recovery rates ranging from 93 to 103%.
Statistical Analysis
Statistical evaluations for comparing mean significant difference were conducted by a two-way analysis of variance (ANOVA) using JMP Pro 14.0 (SPSS Inc. Chicago, IL, United States). Mean comparison was conducted utilizing Duncan’s multiple range test among the different treatments at p < 0.05. Pearson’s correlation analysis was followed among TIs, BCF, TFs, Cd bioaccumulation and total chlorophyll for the two cultivars tested. Inhibition concentration of Cd (IC) for the dose-dependent nonlinear model using the fitted line equation, namely Y = a * X + b and graphs was plotted using Origin 2020b (Tisi et al., 2021).
Results
Consequences of Cd on Biomass of Two Australian Spring Wheat Varieties
The growth of wheat cultivars (during harvesting) at different levels of Cd is presented in Supplementary Figure S1 (Supplementary Information, SI). The data shows that the two cultivars were significantly affected by increasing Cd treatments. The root biomass for Lancer declined significantly (up to 40%) using different Cd treatments compared with the control, whereas for Mustang, it fell by up to 25% (Figure 1A). Under the treatments of Cd4 and Cd8, the root biomass in Mustang was significantly higher (p < 0.05) compared to Lancer. Under different levels of Cd stress, the variation of shoot biomass was significantly higher for Mustang (5–30%) than for Lancer (Figure 1B). Referring to the spike biomass, Mustang showed significantly higher biomass than Lancer and the variation was 10–65% with different Cd treatments (Figure 1C). The grain was affected severely by varying levels of Cd for both cultivars. However, Lancer was more seriously affected than Mustang except for the Cd8 treatment (Figure 1D). When the plants were exposed to different concentrations of Cd, the plant biomass diminished significantly compared with the control treatment. Generally, higher soil Cd affected plant biomass severely in both cultivars. Result of plant biomass revealed that the Mustang cultivar was less affected by increasing the Cd concentration in soil compared with Lancer (Figure 1).
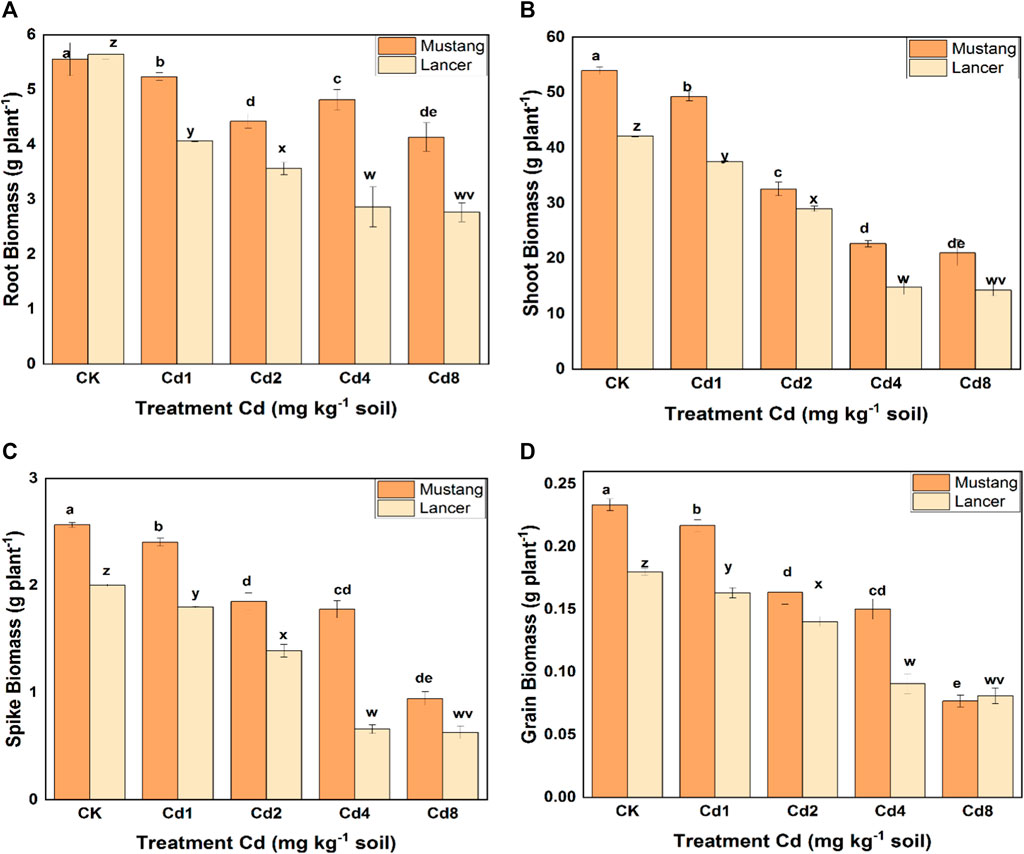
FIGURE 1. Plant root, shoot, spikelets, and grain biomass dry weight under different levels of soil cadmium (Cd) concentrations in two wheat cultivars (Mustang and Lancer). Data are mean ± standard error of three replicates. Different letters indicate the significant differences by letters (a, b, c, d, e for Mustang and z, y, x, w, v for Lancer) among treatments at (p ≤ 0.05) as per Duncan’s multiple range test.
Cd Bioaccumulation in Different Plant Parts
Cd bioaccumulation varied significantly between the two cultivars, shown in Figure 2A and Table 2. Cd concentration in different plant materials varied significantly among treatments (p < 0.05). Regarding the different parts of wheat, the root bioaccumulated significantly higher (p < 0.05) Cd concentrations compared with the control treatment. Mustang bioaccumulated Cd more in shoot compared with Lancer (Table 2). Cadmium concentrations in wheat grains of both cultivars in Cd treatment groups demonstrated an upward trend compared with the control treatment.
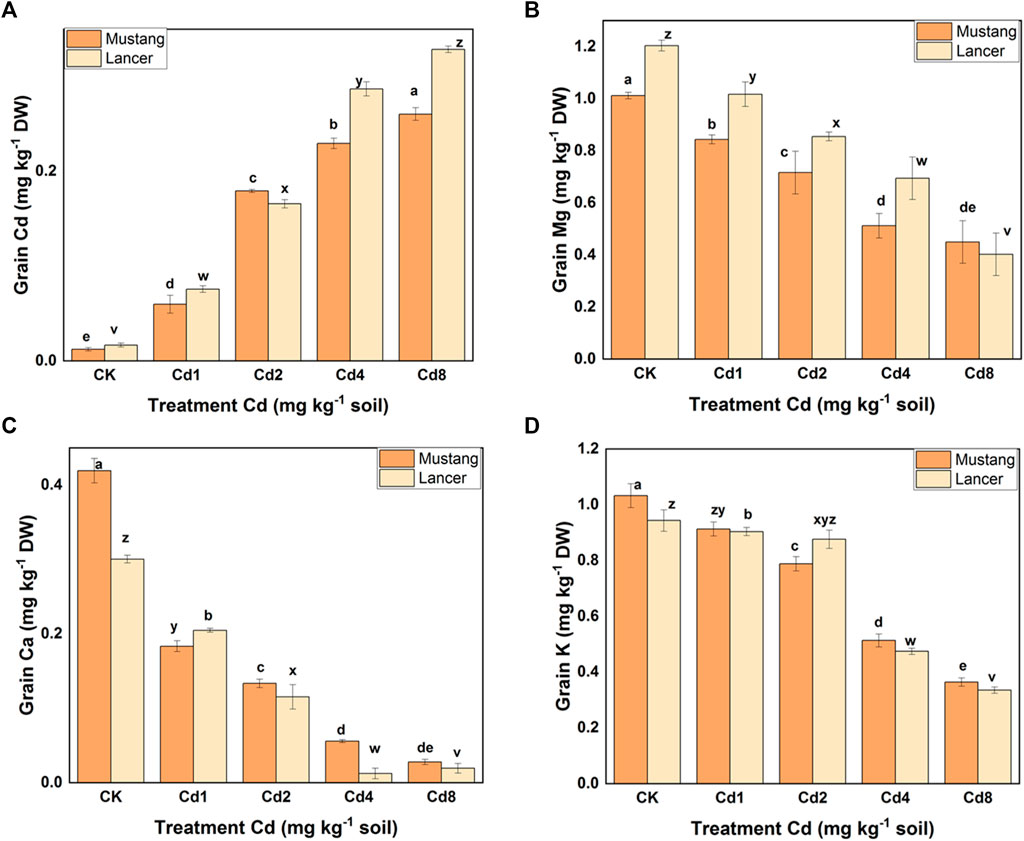
FIGURE 2. Cadmium and macro-nutrient element (Mg, K, and Ca) concentrations in the grain. Data are mean ± standard error of three replicates. Different letters indicate significant differences by letters (a, b, c, d, e for Mustang and z, y, x, w, v for Lancer) among treatments at (p ≤ 0.05) as per Duncan’s multiple range test.
The Cd concentrations in grains rose by 29–65% and 35–72% in Lancer and Mustang, respectively, compared to control and the highest Cd concentrations in wheat grain were detected at Cd8 treatment (Figure 2A). Cd concentrations in the spike of Mustang increased by 46.7–66.1% and those in the shoots increased by 41.0–70.7% compared to the control, which was significantly higher in shoot than Lancer. Cd concentrations in the roots of Mustang and Lancer increased by 43.8–58.9% and 50.7–70.3%, respectively, compared with the controls (0.004 and 0.033 mg kg−1 for Mustang and Lancer, respectively). Overall, the results demonstrated that the Cd concentrations in shoot are statistically significant between cultivars in all Cd treatments (Table 2). For grain, Lancer accumulated more Cd than Mustang (Figure 2A). However, no noticeable trend was observed for root and spikes under different Cd treatments.
Bioaccumulation of Macro-Nutrients in Different Plant Parts
Macro-nutrients (Mg, Ca, and K) bioaccumulation in the wheat plant parts was significantly influenced by different levels of soil Cd compared to the control, as shown in Figures 2B–D and Table 3. When exposed to Cd8 treatment, Mg content in roots, shoots, spike and grain fell by around 62, 55, 41 and 62.5%, respectively, for Mustang, and 68, 66, 20 and 60%, respectively, for Lancer compared with the control treatment. Ca contents decreased by up to 48–94% and 25–95% for Mustang and Lancer, respectively, for all plant parts undergoing Cd8 treatment compared with the control treatment. Again, under the same Cd8 treatment, K content decreased by more than 90% in all plant parts except for grain where it was around 64% for both cultivars compared with the control treatment. Under different levels of Cd stress, grain Ca, Mg and K concentrations in both wheat cultivars were not significant (Figures 2B–D).

TABLE 3. Plant essential macro-nutrients (Mg, Ca, K) in different plant parts under different Cd stress.
Bioaccumulation of Micro-nutrients in Different Plant Parts
Micro-nutrient (Zn, Fe, Mn, and Cu) bioaccumulation in wheat plant parts was significantly (p ≤ 0.05) affected by different levels of soil Cd compared to the control, as presented in Figure 3 and Table 4. Under Cd8 treatment, Zn content in plant parts such as roots, shoots, spike and grain reduced by 42, 59, 60 and 64%, respectively, in Mustang. The percentages for Lancer were 39, 62, 93 and 76%, compared with the control treatment (CK). In the case of iron (Fe) content, Mustang indicated a 64, 63, 52 and 64% and Lancer showed 68, 55, 38 and 58% decrease in roots, shoots, spike and grain, respectively. However, the content of Mn exhibited 79, 67, 46 and 68% decrease in root, shoot, spike and grain, respectively, for Mustang. Conversely for the Lancer cultivars the figures were 80, 67, 38 and 79% decrease in root, shoot, spike and grain. Again, the element Cu contents reduced by 59, 78, 70, and 48% in root, shoot, spike and grain, respectively, for Mustang and 60, 79, 81 and 55% for in root, shoot, spike and grain, respectively, for Lancer. The experimental data confirmed that micro-nutrient (Zn, Fe, Mn, and Cu) contents in the grain significantly decreased in Lancer compared with the Mustang cultivar.
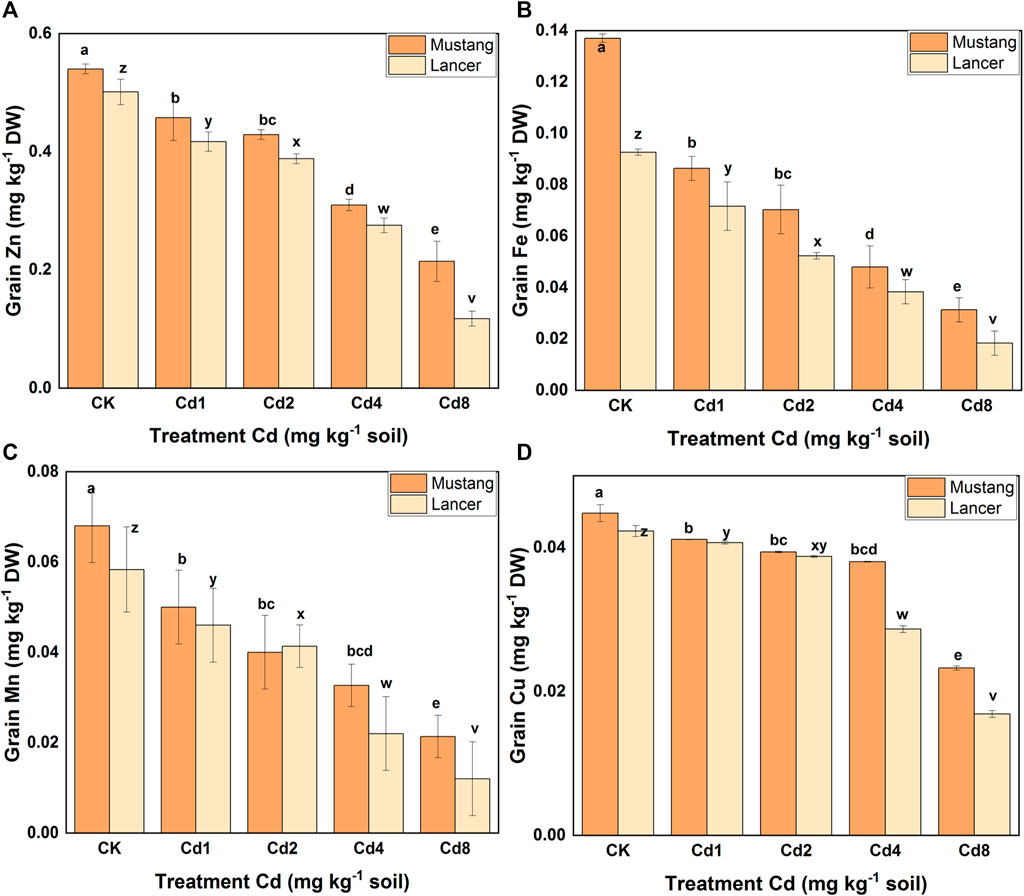
FIGURE 3. Micro-nutrient element (Zn, Fe, Mn, and Cu) concentration the grain. Data are mean ± standard error (SE) of three replicates. p < 0.005. Different letters indicate the significant differences by letters (a, b, c, d, e for Mustang and z, y, x, w, v for Lancer) among treatments at (p ≤ 0.05) as per Duncan’s multiple range test (DMRT).
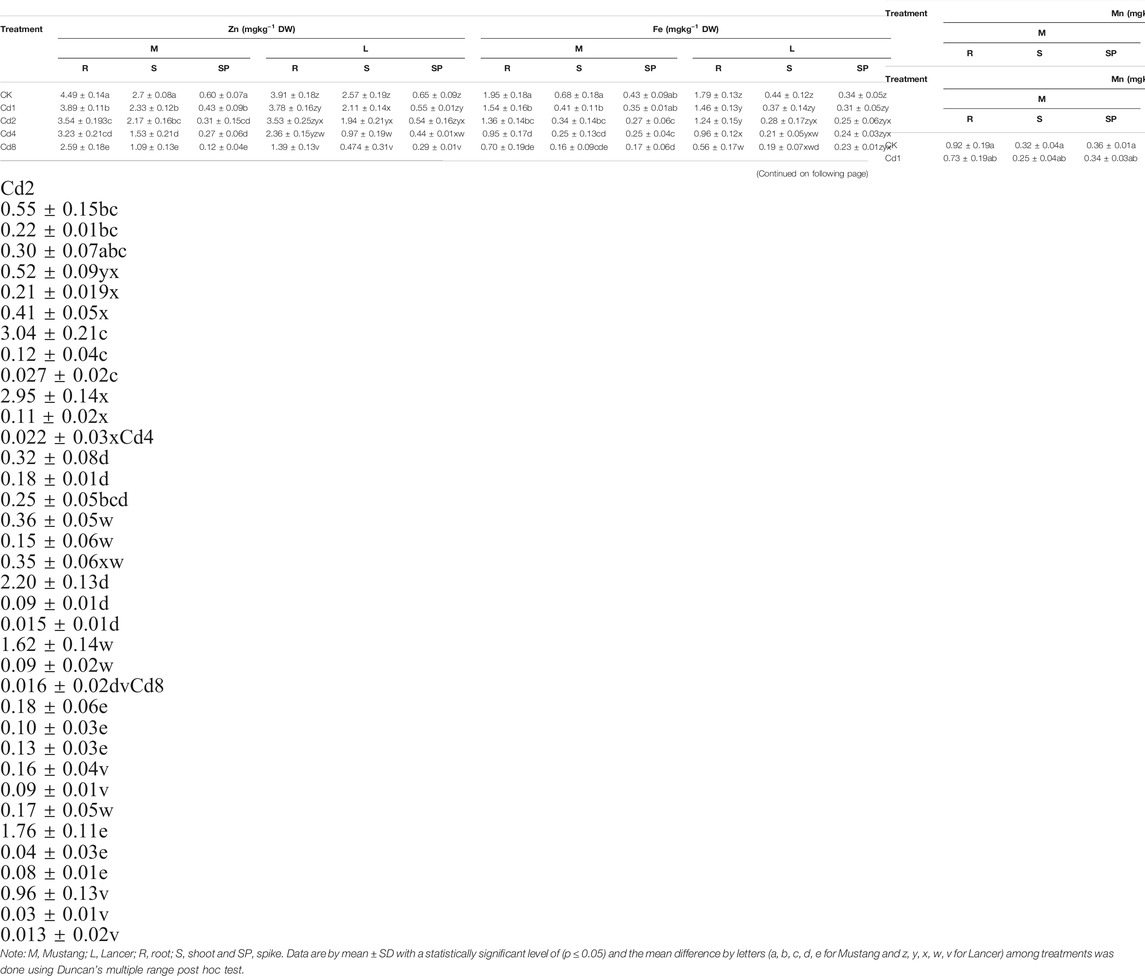
TABLE 4. Plant essential micro-nutrients (Zn, Fe, Mn and Cu) in different plant parts under different level of soil Cd stress.
Photosynthetic Pigment Under Cd Stress
Chlorophyll content was severely decreased by the Cd treatments, and a statistically significant difference was noted among treatments (Figure 4A). Chla, Chlb and total Chl declined by 80, 67 and 76% in the Mustang cultivar and by 89, 91 and 90% in the Lancer cultivar, respectively, when subjected to Cd8 treatment. Chlorophyll content decreased with increasing Cd levels for both varieties. These results demonstrated higher amounts of Chl in leaves in Mustang regardless of Cd stress levels compared with Lancer (Figure 4A). The higher the Cd stress which occurred under Cd4 and Cd8 treatments, the more the chlorophyll content of the Lancer cultivar was affected compared with the Mustang. This was evident judging by the decrease in total Chl in the leaves (Figure 4A).
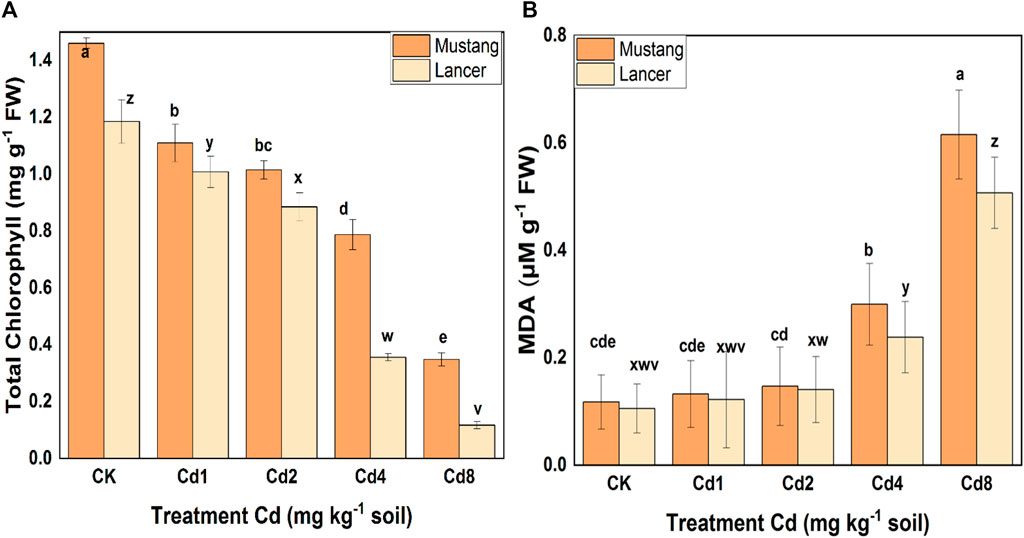
FIGURE 4. Photosynthetic pigment (total chlorophyll) (A) and malondialdehyde (MDA) (B) content in leaves of two Australian wheat varieties under Cd stress. Data are mean ± standard error of three replicates. Different letters indicate significant differences by letters (a, b, c, d, e for Mustang and z, y, x, w, v for Lancer) among treatments at (p ≦ 0.05) as per Duncan's multiple range test.
Lipid Peroxidase Under Cd Stress
Oxidative membrane damage is correlated with variations in H2O2 which manifest as changes in MDA content in plant leaves tissues. MDA content was significantly different for higher levels of Cd stress, and the data are presented in Figure 4B. MDA content increased with rising Cd stress levels for both cultivars: 0.12 ± 0.05 and 0.62 ± 0.08 µg kg−1 FW for Mustang and 0.10 ± 0.04 and 0.51 ± 0.06 µg kg−1 FW for cv. Lancer when grown in CK and Cd8 treatments, respectively. Very minor variation in MDA content at Cd1 and Cd2 treatments was noted compared with the control treatment. However, at Cd4 and Cd8 mg kg−1, MDA content significantly increased in Mustang (around five-fold) and Lancer (around four-fold) leaves compared with the control treatment. Physiological levels of MDA were virtually the same when comparing the two wheat cultivars and they were the lowest at Cd0 and Cd1 in both cultivars (Figure 4B). Under high Cd stress levels, enhanced oxidative membrane damage occurred in both cultivars, which appeared to be relatively milder in Mustang compared to Lancer. In general, MDA levels in both cultivars are very similar in all Cd treatments.
Toxicity Concentration of Cd in Wheat Cultivars
The inhibition concentration of Cd (IC) was used to demonstrate biomass reduction efficiency of Cd for the two spring wheat cultivars evaluated under different Cd treatments as presented in Table 5. IC10, IC50 and IC90 represent concentrations of Cd responsible for 10, 50 and 90% reductions of biomass, respectively. IC10 was higher for the Mustang variety than for the Lancer whereas IC90 was higher for the Lancer cultivar in terms of plant biomass. However, IC50 in both cultivars was not statistically different (Table 5). The Mustang cultivar showed a higher tolerance index compared with the Lancer under different Cd stress levels, which may reduce plant biomass (Supplementary Figure S2 in Supplementary Information).
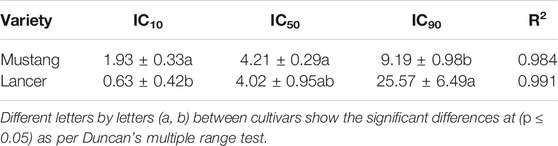
TABLE 5. Inhibitory concentration (IC) of (DTPA extractable) Cd for two spring wheat cultivars grown in different levels of Cd contaminated soil.
Correlation Between Grain Cd Concentration and Agronomic Traits
Correlations among grain Cd concentration and agronomic traits of wheat were analyzed using Spearman correlation tests and are presented in Supplementary Figure S3 (Supplementary Information). Results revealed that DTPA Cd concentration in soil highlighted significant negative correlations with the tolerance index, shoot TF and grain TF (p < 0.05). There was also a significant positive correlation with Cd bioaccumulation (p < 0.05) in both wheat cultivars.
Health Risk Assessment From Exposure to Cd in Wheat Grain
Estimated values of DIC and HRI by consumption of the two wheat cultivar grains grown on Cd-polluted soils are given in Table 6. Cd concentrations in the two wheat cultivars were statistically different at the p < 0.05 level of significance. DIC values varied from 0.00066 to 0.0154 for Mustang and 0.00079 to 0.02131 for Lancer at CK to Cd8 treatment levels. Meanwhile, HRI values for Cd ranged from 0.53 to 12.34 and 0.64 to 17.17 for Mustang and Lancer cultivars, respectively. The results demonstrate that DIC and HRI values of Cd increased for the two spring wheat cultivars with increasing soil Cd pollution. At the Cd8 treatment, the HRI for Cd increased 20 times and 34 times for Mustang and Lancer, respectively, compared with the control treatment.

TABLE 6. Estimated dietary intake of Cd (DIC) and hazardous human risk index (HRI) for Cd by consumption of flour from wheat grains grown on Cd-polluted soil for two well-known spring wheat varieties in Australian soil.
Discussion
These results are supported by different studies that soil Cd significantly (p ≤ 0.05)reduced the biomass in different wheat species (Cai et al., 2020). Plants may develop resistance and as a result may survive in Cd contaminated soils. In several studies it was reported that leaf mesophyll damage, cell wall disruption, golgi-body diffusion, and stunted root tip growth could be caused by Cd toxicity leading to plant biomass reduction (Arshad et al., 2016; Rizwan et al., 2017a; Kamran et al., 2019). The findings from the current study reveal that the two different spring varieties of wheat responded differently under Cd stress. Higher Cd in the soil lowers the cultivars’ biomass production, which might be due to the deadly impact of Cd since it has been widely reported that Cd is toxic to crops specifically to cereal crops such as wheat, rice, maize and sorghum (Lehman et al., 2015).
The dry biomass of roots, shoots, spikes, and grain was 4.13 ± 0.25, 21.01 ± 2.31, 0.94 ± 0.06 and 0.07 ± 0.04 g/plant, respectively, for Mustang, whereas it was 2.76 ± 0.16, 14.24 ± 1.09, 0.63 ± 0.05 and 0.081 ± 0.06 g/plant, respectively, for Lancer when grown in Cd8 treated soil. Studies have demonstrated that when plants are exposed to Cd stress it reduces the biomass dry weight (Hou et al., 2020). For instance, Cd reduces the length and weight of roots and shoots in various plants, while the adverse effects of Cd have detrimental effects on plants at the maturity stage (Ding et al., 2016). In another study it was found that root development was poor under Cd stress (Bahmani et al., 2019). Photosynthesis, macro- and micro-nutrients are the major reasons for plants biomass reduction under Cd stress27-29, which were also investigated in the present study. In the current study we found that Mustang accumulated significantly higher dry biomass in comparison to Lancer under different levels of soil Cd (Figure 1).
The Cd content in roots, shoots, husks and grain was 2.625 ± 0.049, 0.967 ± 0.023, 0.259 ± 0.025 and 0.261 ± 0.06 mg kg−1, respectively, for Mustang, whereas these parameters were 2.781 ± 0.023, 0.674 ± 0.029, 0.534 ± 0.024 and 0.328 ± 0.09 mg kg−1, respectively, for Lancer under Cd8 treatment (Figure 2 and Table 2). These findings reveal that Lancer can bioaccumulate more Cd than Mustang in different plant parts. The higher Cd content in different organs of wheat might be due to lower macro- and micro-nutrients content in plant parts since Cd and macro- and micro-nutrients have antagonistic effects during bioaccumulation. They may also compete for symport in the plant root cell wall and membrane, which has been reported previously (Mourato et al., 2019).
Again, essential macro- and micro-nutrients are positively correlated with root and grain biomass which was restricted by Cd stress in the current studies. Essential nutrients such as K, Ca, Mg, Zn, Fe, Mn and Cu strongly influenced plant growth. These results are supported by Rahman et al. (2009), Maurato et al. (2019) and Islam et al. (2021) (Mourato et al., 2019; Islam et al., 2021; Ur Rahman et al., 2021). For instance, cell wall and membrane performance is predominantly influenced by Ca whereas K influences enzyme activation, photosynthesis pigmentation and protein synthesis, which builds up the organic structures in a plant. In addition, Cd changes the ultra-structure, induces oxidative damage and decreases essential macro- and micro-nutrients in cereal crops (Rizwan et al., 2017b). The macro- and micro-nutrients concentration declined significantly in wheat biomass when subjected to Cd treatment (Arshad et al., 2016). The Cd has been shown to hamper the status of essential nutrients in many plant species. The decrease in major element concentrations with the addition of Cd may be attributed to probable competition at adsorption sites and transporters, those with the same charge. Other studies reported that micro-nutrients such as Ca, Mg and Fe could alleviate the detrimental effects of Cd stress and improve photosynthetic performance under Cd stress (Liu et al., 2015; Singh et al., 2018). Overall, it could be hypothesized that an increase in wheat biomass could be explained by: firstly, the role of macro- and micro-nutrients in controlling the antioxidant activities; and secondly, its influence on the physiological and biochemical processes in wheat tissues (Wang et al., 2020; Moussa et al., 2021).
Mustang was more tolerant to Cd than was Lancer which can be seen from the IC50 inhibitory concentration since Mustang exhibited high macro- and micro-nutrients as well photosynthesis pigmentation and MDA content in leaves compared with Lancer. Varietal Cd tolerance levels impair the morphophysiological parameters of different wheat cultivars, such as Pingan 8 (3), Aikang58 (1.98 ± 0.22), Yangmai 20 (2.20), Zhoumai-32 (0.421 ± 0.026), Sceptre (0.105) mg kg−1 soil Cd (Grant and Bailey, 1998; Ci et al., 2010; Arshad et al., 2016; Guo et al., 2019; Zhang et al., 2019; Huang et al., 2020). Furthermore, it was shown that macro- and micro-nutrients in grain uptake by varietal composition was compromised by Cd toxicity, perhaps due to chelating activities inside plants and rhizosphere (Halim et al., 2020). The greater biomass production in the Mustang cultivar might be due to high uptake of macro- and micro-nutrients of crops under Cd stress. The presence of Cd in soils decreased roots, shoots, and grains of the cultivars compared with the control treatment in the current study as presented in Table 2. It was reported that foliar application of Ca, K and Zn was effective in reducing grain Cd content in wheat grain (Abedi and Mojiri, 2020). Increased macro- and micro-nutrient concentrations in plants may increase competition with Cd for translocation to grains since a strong negative relationship was observed in leaf macro- and micro-nutrients and grain Cd content of wheat. Higher Ca, K, Mg, Zn and Fe content in wheat indicate biofortification which may enhance the quality of cereal grains (Aziz et al., 2019; Yaseen and Hussain, 2021).
In addition, the toxic effect of higher levels of Cd in plants induces redox imbalance in plants (Mir et al., 2018). The current reported that higher MDA levels were found when more Cd toxicity to plants was evident. Higher oxidative damage is the result of larger Cd concentrations in plants’ parts. In addition, oxidative stress negatively disturbs the metabolic process and structural alterations in cells (Castelli et al., 2019; Małkowski et al., 2020). Chlorophyll content and MDA attributes were affected by the toxic effect of Cd in plants compared with the control treatment (Younis et al., 2016). It was evident that chelation and complexation among amino acids and micro-nutrients might increase photosynthesis pigments in crop plants’ leaves under Cd stress. The reduction of chlorophyll content might have occurred because of the decrease in crop nutrients under increasing Cd concentration or increased Cd toxicity (Ali et al., 2019)in the two spring wheat varieties examined in the current study.
Our results showed that the tolerant wheat variety had a lower HRI value for Cd. Moreover, the tolerant variety had significantly higher macro- and micro-nutrients in the grains. Hence, such wheat variety will not only increase the quality of food but also reduce health risks that are due to consumption of wheat-based food produced in Cd-contaminated soils (Zakaria et al., 2021). However, the HRI value of Cd was >1 with increasing soil Cd in plants which may increase owing to consumption of contaminated food for longer periods. Thus, attention must be given to such areas, and the Cd tolerant variety might be helpful for minimizing Cd risk to the consumer.
Conclusion
The current study revealed that the wheat cultivars examined were significantly affected by Cd stress, which is expressed by IC50 and HRI index based on grain Cd exposure to human. In conclusion, under Cd stress two spring wheat cultivars were significantly affected, showing an improved lipid peroxidase defense system and reduced photosynthesis. Cd content was well above the acceptable limits (HRI >1) in two cultivars when exposed to high Cd contaminated soil. We conclude that, when compared with the Lancer cultivar, the Mustang cultivar is more tolerant, poses less risk to human health and contains higher grain macro- and micro-nutrients under Cd stress compared to Lancer. Finally, tolerant cultivar has the potential to chelate Cd in plants’ nonedible parts in variable fractions and improve plant growth and grain macro- and micro-nutrient content. So, despite growing in Cd-contaminated soil, it leads to a reduction in its HRI and might help to protect people from Cd risks. Further research is needed to explore how soil amendment could reduce human health hazards and improve macro- and micro-nutrients in Mustang cultivars grains under Cd stress.
Data Availability Statement
The raw data supporting the conclusion of this article will be made available by the authors, without undue reservation.
Author Contributions
MAH: Conceptualization, Formal analysis, Investigation, Methodology, Writing - original draft, Software; MMR: Supervision, Writing - review and editing; DM: Writing - review and editing; MM: Supervision, Writing - review and editing; RN: Project Administration, Supervision, Writing - review and editing. All authors have read and agreed to the published version of the manuscript.
Funding
This work is supported by the Cooperative Research Centre for Contamination Assessment and Remediation of the Environment (CRC CARE), Australia. The Authority of Sher-e-Bangla Agricultural University is highly appreciated for granting study leave for the first author.
Conflict of Interest
The authors declare that the research was conducted in the absence of any commercial or financial relationships that could be construed as a potential conflict of interest.
Publisher’s Note
All claims expressed in this article are solely those of the authors and do not necessarily represent those of their affiliated organizations, or those of the publisher, the editors and the reviewers. Any product that may be evaluated in this article, or claim that may be made by its manufacturer, is not guaranteed or endorsed by the publisher.
Acknowledgments
The authors kindly acknowledge the support from Global Center for Environmental Remediation (GCER), The University of Newcastle, Australia through the postgraduate research scholarship (UNIPRS) and University of Newcastle Research Scholarship Central (UNRSC). The authors are thankful to “AusWest seed”, Australia for providing us wheat seed for this experiment.
Supplementary Material
The Supplementary Material for this article can be found online at: https://www.frontiersin.org/articles/10.3389/fenvs.2021.779588/full#supplementary-material
References
Abedi, T., and Mojiri, A. (2020). Cadmium Uptake by Wheat (Triticum aestivum L.): An Overview. Plants 9, 500. doi:10.3390/plants9040500
Ali, S., Rizwan, M., Hussain, A., Zia ur Rehman, M., Ali, B., Yousaf, B., et al. (2019). Silicon Nanoparticles Enhanced the Growth and Reduced the Cadmium Accumulation in Grains of Wheat (Triticum aestivum L.). Plant Physiol. Biochem. 140, 1–8. doi:10.1016/j.plaphy.2019.04.041
Ansari, M. I., and Malik, A. (2007). Biosorption of Nickel and Cadmium by Metal Resistant Bacterial Isolates from Agricultural Soil Irrigated with Industrial Wastewater. Bioresour. Technol. 98, 3149–3153. doi:10.1016/j.biortech.2006.10.008
Arisekar, U., Shakila, R. J., Shalini, R., and Jeyasekaran, G. (2020). Human Health Risk Assessment of Heavy Metals in Aquatic Sediments and Freshwater Fish Caught from Thamirabarani River, the Western Ghats of South Tamil Nadu. Mar. Pollut. Bull. 159, 111496. doi:10.1016/j.marpolbul.2020.111496
Arnon, D. I. (1949). Copper Enzymes in Isolated Chloroplasts. Polyphenoloxidase in Beta Vulgaris. Plant Physiol. 24, 1–15. doi:10.1104/pp.24.1.1
Arshad, M., Ali, S., Noman, A., Ali, Q., Rizwan, M., Farid, M., et al. (2016). Phosphorus Amendment Decreased Cadmium (Cd) Uptake and Ameliorates Chlorophyll Contents, Gas Exchange Attributes, Antioxidants, and mineral Nutrients in Wheat (Triticum aestivumL.) under Cd Stress. Arch. Agron. Soil Sci. 62, 533–546. doi:10.1080/03650340.2015.1064903
Aziz, M. Z., Yaseen, M., Abbas, T., Naveed, M., Mustafa, A., Hamid, Y., et al. (2019). Foliar Application of Micronutrients Enhances Crop Stand, Yield and the Biofortification Essential for Human Health of Different Wheat Cultivars. J. Integr. Agric. 18, 1369–1378. doi:10.1016/s2095-3119(18)62095-7
Bahmani, R., Kim, D., Na, J., and Hwang, S. (2019). Expression of the Tobacco Non-symbiotic Class 1 Hemoglobin Gene Hb1 Reduces Cadmium Levels by Modulating Cd Transporter Expression through Decreasing Nitric Oxide and ROS Level in Arabidopsis. Front. Plant Sci. 10, 201. doi:10.3389/fpls.2019.00201
Cai, Y., Zhang, S., Cai, K., Huang, F., Pan, B., and Wang, W. (2020). Cd Accumulation, Biomass and Yield of rice Are Varied with Silicon Application at Different Growth Phases under High Concentration Cadmium-Contaminated Soil. Chemosphere 242, 125128. doi:10.1016/j.chemosphere.2019.125128
Castelli, V., Benedetti, E., Antonosante, A., Catanesi, M., Pitari, G., Ippoliti, R., et al. (2019). Neuronal Cells Rearrangement during Aging and Neurodegenerative Disease: Metabolism, Oxidative Stress and Organelles Dynamic. Front. Mol. Neurosci. 12, 132. doi:10.3389/fnmol.2019.00132
Ci, D., Jiang, D., Wollenweber, B., Dai, T., Jing, Q., and Cao, W. (2010). Cadmium Stress in Wheat Seedlings: Growth, Cadmium Accumulation and Photosynthesis. Acta Physiol. Plant 32, 365–373. doi:10.1007/s11738-009-0414-0
Ding, Y., Ye, Y., Jiang, Z., Wang, Y., and Zhu, C. (2016). MicroRNA390 Is Involved in Cadmium Tolerance and Accumulation in rice. Front. Plant Sci. 7, 235. doi:10.3389/fpls.2016.00235
Du, Y., Hu, X.-F., Wu, X.-H., Shu, Y., Jiang, Y., and Yan, X.-J. (2013). Affects of Mining Activities on Cd Pollution to the Paddy Soils and rice Grain in Hunan Province, Central South China. Environ. Monit. Assess. 185, 9843–9856. doi:10.1007/s10661-013-3296-y
Epa, U. (1989). Risk Assessment Guidance for Superfund Vol. I Human Health Evaluation Manual. Available at: EPA/540/1-89/002. Office of Solid Waste and Emergency Response.
Gill, S. S., and Tuteja, N. (2010). Reactive Oxygen Species and Antioxidant Machinery in Abiotic Stress Tolerance in Crop Plants. Plant Physiol. Biochem. 48, 909–930. doi:10.1016/j.plaphy.2010.08.016
Grant, C. A., and Bailey, L. D. (1998). Nitrogen, Phosphorus and Zinc Management Effects on Grain Yield and Cadmium Concentration in Two Cultivars of Durum Wheat. Can. J. Plant Sci. 78, 63–70. doi:10.4141/p96-189
Gray, C. W., Yi, Z., Munir, K., Lehto, N. J., Robinson, B. H., and Cavanagh, J.-A. E. (2019). Cadmium Concentrations in New Zealand Wheat: Effect of Cultivar Type, Soil Properties, and Crop Management. J. Environ. Qual. 48, 701–708. doi:10.2134/jeq2018.12.0430
Guo, J., Qin, S., Rengel, Z., Gao, W., Nie, Z., Liu, H., et al. (2019). Cadmium Stress Increases Antioxidant Enzyme Activities and Decreases Endogenous Hormone Concentrations More in Cd-Tolerant Than Cd-Sensitive Wheat Varieties. Ecotoxicology Environ. Saf. 172, 380–387. doi:10.1016/j.ecoenv.2019.01.069
Halim, M. A., Rahman, M. M., Megharaj, M., and Naidu, R. (2020). Cadmium Immobilization in the Rhizosphere and Plant Cellular Detoxification: Role of Plant-Growth-Promoting Rhizobacteria as a Sustainable Solution. J. Agric. Food Chem. 68, 13497–13529. doi:10.1021/acs.jafc.0c04579
Hassan, M. U., Chattha, M. U., Khan, I., Chattha, M. B., Aamer, M., Nawaz, M., et al. (2019). Nickel Toxicity in Plants: Reasons, Toxic Effects, Tolerance Mechanisms, and Remediation Possibilities-A Review. Environ. Sci. Pollut. Res. 26, 12673–12688. doi:10.1007/s11356-019-04892-x
Hayat, M. T., Nauman, M., Nazir, N., Ali, S., and Bangash, N. (2019). “Environmental Hazards of Cadmium: Past, Present, and Future,” in Cadmium Toxicity and Tolerance in Plants (Elsevier), 163–183. doi:10.1016/b978-0-12-814864-8.00007-3
Hou, L., Yu, J., Zhao, L., and He, X. (2020). Dark Septate Endophytes Improve the Growth and the Tolerance of Medicago Sativa and Ammopiptanthus Mongolicus under Cadmium Stress. Front. Microbiol. 10, 3061. doi:10.3389/fmicb.2019.03061
Huang, X., Wang, C., Hou, J., Du, C., Liu, S., Kang, J., et al. (2020). Coordination of Carbon and Nitrogen Accumulation and Translocation of winter Wheat Plant to Improve Grain Yield and Processing Quality. Sci. Rep. 10, 1–11. doi:10.1038/s41598-020-67343-5
Islam, M. S., Magid, A. S. I. A., Chen, Y., Weng, L., Ma, J., Arafat, M. Y., et al. (2021). Effect of Calcium and Iron-Enriched Biochar on Arsenic and Cadmium Accumulation from Soil to rice Paddy Tissues. Sci. Total Environ. 785, 147163. doi:10.1016/j.scitotenv.2021.147163
Jinadasa, B. K. K. K., Chathurika, G. S., Jayaweera, C. D., and Jayasinghe, G. D. T. M. (2019). Mercury and Cadmium in Swordfish and Yellowfin Tuna and Health Risk Assessment for Sri Lankan Consumers. Food Additives & Contaminants: B 12, 75–80. doi:10.1080/19393210.2018.1551247
Kabir, A. H., Akther, M. S., Skalicky, M., Das, U., Gohari, G., Brestic, M., et al. (2021). Downregulation of Zn-Transporters along with Fe and Redox Imbalance Causes Growth and Photosynthetic Disturbance in Zn-Deficient Tomato. Sci. Rep. 11, 1–12. doi:10.1038/s41598-021-85649-w
Kamran, M., Malik, Z., Parveen, A., Zong, Y., Abbasi, G. H., Rafiq, M. T., et al. (2019). Biochar Alleviates Cd Phytotoxicity by Minimizing Bioavailability and Oxidative Stress in Pak Choi (Brassica Chinensis L.) Cultivated in Cd-Polluted Soil. J. Environ. Manag. 250, 109500. doi:10.1016/j.jenvman.2019.109500
Kandic, S., Tepe, S. J., Blanch, E. W., De Silva, S., Mikkonen, H. G., and Reichman, S. M. (2019). Quantifying Factors Related to Urban Metal Contamination in Vegetable Garden Soils of the West and north of Melbourne, Australia. Environ. Pollut. 251, 193–202. doi:10.1016/j.envpol.2019.04.031
Kaya, C., Okant, M., Ugurlar, F., Alyemeni, M. N., Ashraf, M., and Ahmad, P. (2019). Melatonin-mediated Nitric Oxide Improves Tolerance to Cadmium Toxicity by Reducing Oxidative Stress in Wheat Plants. Chemosphere 225, 627–638. doi:10.1016/j.chemosphere.2019.03.026
Khanna, K., Jamwal, V. L., Gandhi, S. G., Ohri, P., and Bhardwaj, R. (2019). Metal Resistant PGPR Lowered Cd Uptake and Expression of Metal Transporter Genes with Improved Growth and Photosynthetic Pigments in Lycopersicon esculentum under Metal Toxicity. Sci. Rep. 9, 1–14. doi:10.1038/s41598-019-41899-3
Lehman, R., Cambardella, C., Stott, D., Acosta-Martinez, V., Manter, D., Buyer, J., et al. (2015). Understanding and Enhancing Soil Biological Health: the Solution for Reversing Soil Degradation. Sustainability 7, 988–1027. doi:10.3390/su7010988
Li, Q., Wang, G., Wang, Y., Dan Yang, D., Guan, C., and Ji, J. (2019). Foliar Application of Salicylic Acid Alleviate the Cadmium Toxicity by Modulation the Reactive Oxygen Species in Potato. Ecotoxicology Environ. Saf. 172, 317–325. doi:10.1016/j.ecoenv.2019.01.078
Li, X., Jia, R., Lu, X., Xu, Y., Liang, X., Shen, L., et al. (2021). The Use of Mercapto-Modified Palygorskite Prevents the Bioaccumulation of Cadmium in Wheat. J. Hazard. Mater. 417, 125917. doi:10.1016/j.jhazmat.2021.125917
Liu, W., Shang, S., Feng, X., Zhang, G., and Wu, F. (2015). Modulation of Exogenous Selenium in Cadmium-Induced Changes in Antioxidative Metabolism, Cadmium Uptake, and Photosynthetic Performance in the 2 Tobacco Genotypes Differing in Cadmium Tolerance. Environ. Toxicol. Chem. 34, 92–99. doi:10.1002/etc.2760
Mahawar, L., Popek, R., Shekhawat, G. S., Alyemeni, M. N., and Ahmad, P. (2021). Exogenous Hemin Improves Cd2+ Tolerance and Remediation Potential in Vigna Radiata by Intensifying the HO-1 Mediated Antioxidant Defence System. Sci. Rep. 11, 2811–2812. doi:10.1038/s41598-021-82391-1
Małkowski, E., Sitko, K., Szopiński, M., Gieroń, Ż., Pogrzeba, M., Kalaji, H. M., et al. (2020). Hormesis in Plants: The Role of Oxidative Stress, Auxins and Photosynthesis in Corn Treated with Cd or Pb. Int. J. Mol. Sci. 21, 2099.
Mehta, S., and Bharat, R. (2019). Effect of Integrated Use of Nano and Non-nano Fertilizers on Yield and Yield Attributes of Wheat (Triticum aestivum L.). Int. J. Curr. Microbiol. App. Sci. 8, 598–606. doi:10.20546/ijcmas.2019.812.078
Mir, M. A., Sirhindi, G., Alyemeni, M. N., Alam, P., and Ahmad, P. (2018). Jasmonic Acid Improves Growth Performance of Soybean under Nickel Toxicity by Regulating Nickel Uptake, Redox Balance, and Oxidative Stress Metabolism. J. Plant Growth Regul. 37, 1195–1209. doi:10.1007/s00344-018-9814-y
Mourato, M., Pinto, F., Moreira, I., Sales, J., Leitão, I., and Martins, L. L. (2019). “The Effect of Cd Stress in mineral Nutrient Uptake in Plants,” in Cadmium Toxicity and Tolerance in Plants (Elsevier), 327–348. doi:10.1016/b978-0-12-814864-8.00013-9
Moussa, M. G., Sun, X., Ismael, M. A., Elyamine, A. M., Rana, M. S., Syaifudin, M., et al. (2021). Molybdenum-Induced Effects on Grain Yield, Macro–Micro-Nutrient Uptake, and Allocation in Mo-Inefficient Winter Wheat. J. Plant Growth Regul., 1–16. doi:10.1007/s00344-021-10397-0
Ondrasek, G., Rengel, Z., Maurović, N., Kondres, N., Filipović, V., Savić, R., et al. (2021). Growth and Element Uptake by Salt-Sensitive Crops under Combined NaCl and Cd Stresses. Plants 10, 1202. doi:10.3390/plants10061202
Pál, M., Janda, T., and Szalai, G. (2018). Interactions between Plant Hormones and Thiol-Related Heavy Metal Chelators. Plant Growth Regul. 85, 173–185. doi:10.1007/s10725-018-0391-7
Qadir, M., Wichelns, D., Raschid-Sally, L., Mccornick, P. G., Drechsel, P., Bahri, A., et al. (2010). The Challenges of Wastewater Irrigation in Developing Countries. Agric. Water Manag. 97, 561–568. doi:10.1016/j.agwat.2008.11.004
Qiao, K., Liang, S., Wang, F., Wang, H., Hu, Z., and Chai, T. (2019). Effects of Cadmium Toxicity on Diploid Wheat (Triticum Urartu) and the Molecular Mechanism of the Cadmium Response. J. Hazard. Mater. 374, 1–10. doi:10.1016/j.jhazmat.2019.04.018
Rahman, M. M., Owens, G., and Naidu, R. (2009). Arsenic Levels in rice Grain and Assessment of Daily Dietary Intake of Arsenic from rice in Arsenic-Contaminated Regions of Bangladesh-implications to Groundwater Irrigation. Environ. Geochem. Health 31, 179–187. doi:10.1007/s10653-008-9238-x
Rezapour, S., Atashpaz, B., Moghaddam, S. S., Kalavrouziotis, I. K., and Damalas, C. A. (2019). Cadmium Accumulation, Translocation Factor, and Health Risk Potential in a Wastewater-Irrigated Soil-Wheat (Triticum aestivum L.) System. Chemosphere 231, 579–587. doi:10.1016/j.chemosphere.2019.05.095
Rizwan, M., Ali, S., Hussain, A., Ali, Q., Shakoor, M. B., Zia-Ur-Rehman, M., et al. (2017b). Effect of Zinc-Lysine on Growth, Yield and Cadmium Uptake in Wheat (Triticum aestivum L.) and Health Risk Assessment. Chemosphere 187, 35–42. doi:10.1016/j.chemosphere.2017.08.071
Rizwan, M., Ali, S., Zaheer Akbar, M., Shakoor, M. B., Mahmood, A., Ishaque, W., et al. (2017a). Foliar Application of Aspartic Acid Lowers Cadmium Uptake and Cd-Induced Oxidative Stress in rice under Cd Stress. Environ. Sci. Pollut. Res. 24, 21938–21947. doi:10.1007/s11356-017-9860-1
Seyfferth, A. L., Limmer, M. A., and Dykes, G. E. (2018). On the Use of Silicon as an Agronomic Mitigation Strategy to Decrease Arsenic Uptake by rice. Adv. Agron. 149, 49–91. doi:10.1016/bs.agron.2018.01.002
Shalata, A., and Neumann, P. M. (2001). Exogenous Ascorbic Acid (Vitamin C) Increases Resistance to Salt Stress and Reduces Lipid Peroxidation. J. Exp. Bot. 52, 2207–2211. doi:10.1093/jexbot/52.364.2207
Siddique, A. B., Rahman, M. M., Islam, M. R., Shehzad, M. T., Nath, B., and Naidu, R. (2021). Influence of Iron Plaque on Accumulation and Translocation of Cadmium by Rice Seedlings. Sustainability 13, 10307. doi:10.3390/su131810307
Singh, H., Sharma, A., Bhardwaj, S. K., Arya, S. K., Bhardwaj, N., and Khatri, M. (2021). Recent Advances in the Applications of Nano-Agrochemicals for Sustainable Agricultural Development. Environ. Sci. Process. Impacts 23, 213–239. doi:10.1039/d0em00404a
Singh, R., Parihar, P., and Prasad, S. M. (2018). Sulfur and Calcium Simultaneously Regulate Photosynthetic Performance and Nitrogen Metabolism Status in As-Challenged Brassica Juncea L. Seedlings. Front. Plant Sci. 9, 772. doi:10.3389/fpls.2018.00772
Song, Y. L., Dong, Y. J., Tian, X. Y., Kong, J., Bai, X. Y., Xu, L. L., et al. (2016). Role of Foliar Application of 24-epibrassinolide in Response of Peanut Seedlings to Iron Deficiency. Biol. Plant. 60, 329–342. doi:10.1007/s10535-016-0596-4
Tisi, R., Spinelli, M., Palmioli, A., Airoldi, C., Cazzaniga, P., Besozzi, D., et al. (2021). The Multi-Level Mechanism of Action of a Pan-Ras Inhibitor Explains its Antiproliferative Activity on Cetuximab-Resistant Cancer Cells. Front. Mol. Biosciences 8, 21. doi:10.3389/fmolb.2021.625979
Ur Rahman, S., Xuebin, Q., Zhao, Z., Du, Z., Imtiaz, M., Mehmood, F., et al. (2021). Alleviatory Effects of Silicon on the Morphology, Physiology, and Antioxidative Mechanisms of Wheat (Triticum aestivum L.) Roots under Cadmium Stress in Acidic Nutrient Solutions. Sci. Rep. 11, 1–12. doi:10.1038/s41598-020-80808-x
Wang, J., Chatzidimitriou, E., Wood, L., Hasanalieva, G., Markellou, E., Iversen, P. O., et al. (2020). Effect of Wheat Species (Triticum aestivum vs T. Spelta), Farming System (Organic vs Conventional) and Flour Type (Wholegrain vs white) on Composition of Wheat Flour - Results of a Retail Survey in the UK and Germany - 2. Antioxidant Activity, and Phenolic and mineral Content. Food Chem. X 6, 100091. doi:10.1016/j.fochx.2020.100091
Xu, H.-S., Zhu, L., and Mei, Y. (2021). Effects of High Levels of Nitrogen and Phosphorus on Perennial Ryegrass (Lolium Perenne L.) and its Potential in Bioremediation of Highly Eutrophic Water. Environ. Sci. Pollut. Res. 28, 9475–9483. doi:10.1007/s11356-020-11458-9
Yaseen, M. K., and Hussain, S. (2021). Zinc-biofortified Wheat Required Only a Medium Rate of Soil Zinc Application to Attain the Targets of Zinc Biofortification. Arch. Agron. Soil Sci. 67, 551–562. doi:10.1080/03650340.2020.1739659
Younis, U., Malik, S. A., Rizwan, M., Qayyum, M. F., Ok, Y. S., Shah, M. H. R., et al. (2016). Biochar Enhances the Cadmium Tolerance in Spinach (Spinacia Oleracea) through Modification of Cd Uptake and Physiological and Biochemical Attributes. Environ. Sci. Pollut. Res. 23, 21385–21394. doi:10.1007/s11356-016-7344-3
Zakaria, Z., Zulkafflee, N. S., Mohd Redzuan, N. A., Selamat, J., Ismail, M. R., Praveena, S. M., et al. (2021). Understanding Potential Heavy Metal Contamination, Absorption, Translocation and Accumulation in Rice and Human Health Risks. Plants 10, 1070. doi:10.3390/plants10061070
Keywords: cadmium, chlorophyll, malondialdehyde, macronutrients and micronutrients, health risk index, IC50
Citation: Halim MA, Rahman MM, Mondal D, Megharaj M and Naidu R (2021) Bioaccumulation and Tolerance Indices of Cadmium in Wheat Plants Grown in Cadmium-Spiked Soil: Health Risk Assessment. Front. Environ. Sci. 9:779588. doi: 10.3389/fenvs.2021.779588
Received: 19 September 2021; Accepted: 24 November 2021;
Published: 23 December 2021.
Edited by:
Nsikak U. Benson, Covenant University, NigeriaReviewed by:
Clare Alexandra Wilson, University of Stirling, United KingdomPatompong Johns Saengwilai, Mahidol University, Thailand
Copyright © 2021 Halim, Rahman, Mondal, Megharaj and Naidu. This is an open-access article distributed under the terms of the Creative Commons Attribution License (CC BY). The use, distribution or reproduction in other forums is permitted, provided the original author(s) and the copyright owner(s) are credited and that the original publication in this journal is cited, in accordance with accepted academic practice. No use, distribution or reproduction is permitted which does not comply with these terms.
*Correspondence: Mohammad Mahmudur Rahman, mahmud.rahman@newcastle.edu.au; Ravi Naidu, ravi.naidu@newcastle.edu.au