- 1Department of Zoology and Entomology, Forestry and Agricultural Biotechnology Institute (FABI), University of Pretoria, Pretoria, South Africa
- 2Department of Natural Resources and the Environment, College of Life Sciences and Agriculture (COLSA), University of New Hampshire, Durham, NH, United States
- 3Silviculture and Phytosanitary Protection Division, Bioforest, Concepción, Chile
- 4Forest Research Institute, The University of the Sunshine Coast, Sippy Downs, QLD, Australia
- 5Department of Biochemistry, Genetics and Microbiology, Forestry and Agricultural Biotechnology Institute, University of Pretoria, Pretoria, South Africa
Glycaspis brimblecombei is an invasive insect pest of Eucalyptus that has spread rapidly around the world since its first report in California in 1998. The pest now occurs on at least four continents where Eucalyptus is grown as a non-native plantation species. To characterize global routes of invasion for this insect, we characterized the sequences of a portion of the Cytochrome Oxidase 1 (COI) gene from 105 individuals from the invasive and native range, including from Australia, Brazil, Chile, La Réunion, Mauritius, South Africa and the United States. In addition, we developed 13 polymorphic microsatellite markers, of which we used 11 to characterize the diversity in the same 105 specimens. Our results suggest that there have been two independent introduction events from Australia, which is assumed to be the origin, to distinct parts of the adventive range. The first introduction was into the United States, from where it appears to have spread to South America and eventually to South Africa. This finding highlights the threat of bridgehead populations to accelerate pest invasions in Eucalyptus, even if those populations are on widespread non-commercial populations of Eucalyptus (as in California). A second introduction appears to have occurred on the islands of Mauritius and La Réunion and provides another example of the establishment of independent lineages of invasive global insect pests. This complex invasion pattern mirrors that found in other Eucalyptus pests.
Introduction
Invasive insects threaten biodiversity and ecosystem function in both natural and highly managed ecosystems such as forestry and agriculture (Hoffmann et al., 2008; Wingfield et al., 2015; Hurley et al., 2016). Increasingly, such threats are global in scope. Together with climate change and increasing volumes of global trade, the homogenization of ecosystems, in part due to the widespread planting of common species for agriculture or forestry, have increased the rate of insect spread and establishment worldwide (Ali et al., 2014; Garnas et al., 2016). Although the invasion mechanisms and patterns of movement vary among organisms, the increase in accidental insect spread is strongly linked to anthropogenic pathways (Estoup and Guillemaud, 2010; Foucaud et al., 2010; Garnas et al., 2016). Understanding these patterns of movement is important for managing existing invasive populations, as well as avoiding future introductions.
Eucalyptus species are native to Australasia and have become one of the most widely planted hardwood species worldwide, now occurring in more than 100 countries and on all continents with the exception of Antarctica and covering over 20 million hectares (Brockerhoff et al., 2013). Since the first report of invasive Eucalyptus pests reported outside their native range, the number of invasive insect pest outbreaks has been continuously rising, with a sharp increase in the number of emergences since the 1980’s (Wingfield et al., 2015; Hurley et al., 2016). This number is expected to continue to increase rapidly in future given the consistent pattern over the last 20 years.
Glycaspis brimblecombei Moore, 1964 (Hemiptera, Psylloidea, Aphalaridae), commonly known as the red gum lerp psyllid, has become an important threat to Eucalyptus plantations worldwide (de Queiroz et al., 2013). It was first reported outside its native range in 1998 in California, United States (Brennan et al., 2001) and has since rapidly spread to several countries (Garnas et al., 2016; Makunde et al., 2020). Within 10 years after its first report from California, it was noted in at least seven countries in the Americas (de Queiroz et al., 2013; Tsagkarakis et al., 2014), Mauritius and Madagascar (Laudonia and Garonna, 2010). Subsequently, G. brimblecombei has also been observed from several countries in Europe and Africa (Laudonia and Garonna, 2010; Bella and Rapisarda, 2013; Frasconi et al., 2013; Ben Attia and Rapisarda, 2014; Laudonia et al., 2014; Spodek et al., 2015; Ndlela et al., 2018; Makunde et al., 2020).
Heavy infestations of G. brimblecombei on Eucalyptus trees have the potential to cause premature leaf fall (including total defoliation in severe cases), dieback and reduction of the tree growth (de Queiroz et al., 2013). Physiological stresses caused by G. brimblecombei on the tree leads to increased susceptibility of the tree to other pests and diseases and can result in the death of susceptible varieties (Frasconi et al., 2013). Although G. brimblecombei mainly attack species such as E. camaldulensis Dehnh and E. tereticornis Smith, it also affects hybrids such as E. grandis× E. tereticornis, and E. grandis × E. camaldulensis (Brennan et al., 2001; de Queiroz et al., 2013; Ben Attia and Rapisarda, 2014; Lucia et al., 2016; Bush et al., 2020). Classic biological control, pesticides and the planting of tolerant tree species and/or hybrid clones are currently being used as management strategies for G. brimblecombei (Gonçalves et al., 2013; Garnas et al., 2016; Ndlela et al., 2018; Kojwang, 2019). Biological control using the psyllid-specific parasitic wasp Psyllaephagus bliteus Riek (Hymenoptera: Encyrtidae) has been attempted in a few countries (Dahlsten et al., 2005; Huerta et al., 2011). However, the effectiveness of P. bliteus varies geographically and studies have concluded that these differences are linked to habitat and temperature (Dahlsten et al., 2005; Gonçalves et al., 2013; Margiotta et al., 2017). Similarly, studies have shown the dependence on environmental factors also of development and invasion capacity of Glycaspis brimblecombei (Laudonia et al., 2014; Caleca et al., 2018; Junk et al., 2020).
The impact of G. brimblecombei as an invasive pest, the need to increase the efficiency of biological control management options, as well as the history of complex invasions (including cryptic species and establishment of multiple independent lineages) in other Eucalyptus pests, inspired this study to characterize the diversity of and invasion history of global populations of G. brimblecombei. We use reports of the emergence of G. brimblecombei, together with nuclear and mitochondrial DNA markers to characterize the genetic diversity within and between populations from its native (Australia) and invasive (including the United States, various countries in South America, South Africa, La Réunion and Mauritius) range to suggest the historical pattern of the invasion.
Materials and Methods
Glycaspis brimblecombei Sample Collection and DNA Extraction
Whole specimens of Glycaspis brimblecombei were obtained from across the invasive range from Brazil (N = 11), Chile (Valparaiso Region; Santiago) (N = 16), La Réunion (N = 15), Mauritius (N = 13), South Africa (Gauteng; KwaZulu-Natal; Limpopo; Mpumalanga) (N = 30), the United States (California—Laguna Grande; Riverside) (N = 11), and Australia (Queensland) (N = 9) and were stored in 95% ethanol at –20°C until used for DNA extraction. The sampling was carried out from 2012 to 2014. A modified phenol-chloroform DNA extraction protocol was used to obtain genomic DNA from individual insects (Nishiguchi et al., 2002). Prior to DNA extraction, specimens were preserved in 95% ethanol.
Glycaspis brimblecombei Mitochondrial Diversity
A portion of the Cytochrome Oxidase subunit I (COI) of the mitochondrial gene from a total of 105 individuals was amplified using the universal primers C1-N-2191 (5’-CCC GGT AAA ATT AAA ATA TAA ACT TC-3’) (alias Nancy) and C1-J-1718 (5’-GGA GGA TTT GGA AAT TGAT TAG TTC C-3’) (alias Ron) (Simon et al., 1994). PCR amplifications were performed using the Veriti Thermal Cycler (Applied Biosystems, Foster City, California, Unites States) in a total volume of 25 μl which contained 5 μl 5X MyTaq Reaction Buffer consisting of dNTPs, MgCl2, and ready to use enhancers (Bioline, London, United Kingdom), 2.5 U MyTaq™ DNA Polymerase (Bioline, London, United Kingdom), 10 μM of each C1-N-2191 and C1-J-1718 primer, 16.5 μl SABAX pure water (Adcock Ingram, Midrand, South Africa) and a minimum of 10 ng/μl of DNA. The PCR cycling parameters included an initial denaturation step of 95°C for 3 min., followed by 35 cycles of denaturation at 95°C for 20 s, annealing at 54°C for 30 s, and a final extension step of at 72°C for 7 min. The PCR product was visualized on a 2% agarose gel using a BioRad Gel Doc™ Ez Imager and the software Image Lab v4.0 build 16 (Hercules, California, United States).
The PCR products were purified using Sephadex G-50 (Sigma, St. Louis, Missouri, United States) columns for 3 min. at 800 rcf in a desktop centrifuge (Eppendorf, Hamburg, Germany). Subsequently the PCR products were visualized on a 2% agarose gel using a BioRad Gel Doc™ Ez Imager and the software Image Lab v4.0 build 16 (Hercules, California, United States). Purified PCR amplicons were used for cycle sequencing incorporating 6.4 μl sterile SABAX water (Adcock Ingram, Midrand, South Africa), 2.1 μl of 5 X sequencing buffer (Thermo Fisher Scientific, Waltham, Massachusetts, United States), 0.5 μl of BigDye terminator 3.1, 10 μM of each primer. The cycle sequencing conditions included 96°C for 2 min., followed by 30 cycles of 96°C for 30 s, 54°C for 15 min. and 60°C for 4 min. Subsequently, the cycle sequencing product was cleaned using Ethanol/NaAc precipitation protocol, following guidelines from the ABI manual (2010).1 Samples were sequenced using the ABI PRISM™ 3100 Genetic Analyzer (Applied Biosystems, Foster City, California, Unites States).
MEGA version 7 (Kumar et al., 2016) was used to edit individual sequences and create contigs. AliView Version 1.17.1 (Larsson, 2014) was used to translate nucleotide sequences to amino acid sequences to check for the presence of stop codons. Nucelotide diversity and haplotype diversity (Nei, 1987) were computed using DnaSP version 6.12.03 (Rozas et al., 2003). A multiple sequence alignment was conducted using ClustalΩ (Sievers and Higgins, 2002) with default parameters. A COI haplotype network was generated using Network Version 10.1.0.0 package (Polzin and Daneschmand, 2003).2 A neighbor Joining analysis, using the Kimura-2 parameter substitution model, was conducted using MEGA version 7 (Kumar et al., 2016) with 1000 bootstrap replicates. Blastopsylla occidentalis (GenBank number MN272142.1) was used as an outgroup. Uncorrected percentage pairwise sequence divergence was also calculated using MEGA version 7 (Kumar et al., 2016).
Glycaspis brimblecombei Microsatellite Development, Amplification, and Genotyping
Low-coverage sequencing of the G. brimblecombei genome was performed using the Illumina MiSeq platform. Sequence reads for G. brimblecombei were assembled using VelvetOptimiser v.2.2.5 (Zerbino and Birney, 2008). All contigs larger than 1000 base pairs (bp) were used to search for microsatellites using MsatCommander 1.0.8 (Faircloth, 2008). Selection criteria included a minimum of seven and a maximum of 23 repeats. Microsatellites from di-, tri-, tetra-, and pentanucleotide classes were selected with product sizes ranging from 100 to 377 bp. Subsequently, Primer3 version 2.5.0 (Untergrasser et al., 2012) was used to design twenty primers which were evaluated for suitability.
Polymorphism were confirmed by PCR using three individuals per country (Australia, Brazil, Chile, La Réunion, Mauritius, South Africa and United States). Thirteen of the 20 microsatellite primers showed polymorphisms. The forward primers of these 13 primer pairs were fluorescently labeled (Thermo Fischer Scientific, Waltham, Massachusetts, United States) and subsequently used for PCR. The same 105 specimens used for the mitochondrial analysis were used for the microsatellite analysis. Microsatellite amplification was conducted in a 96-well plate with a total reaction volume of 11 μl comprising 1 μl PCR product, 0.15 μl GeneScan 500 LIZ dye Size Standard (Applied Biosystems, Foster City, California, Unites States) and 10.27 μl Formamide. Scoring was conducted using GeneMapper software (Version 4.1, Applied Biosystems, Foster City, California, Unites States).
Genetic Diversity Analysis
For each of the seven populations (Australia, Brazil, Chile, La Réunion, Mauritius, South Africa, and United States) genetic diversity indices were generated using Arlequin version 3.5.1.3 (Excoffier et al., 2005). An analysis of molecular variance (AMOVA) was conducted, with 100 000 permutations using Arlequin version 3.5.1.3 (Excoffier et al., 2005), to compare the genetic variance among regions (native and invasive), among populations within regions, and within populations. Pairwise Fst values were calculated for specimens in each of the seven populations using Arlequin version 3.5.1.3 (Excoffier et al., 2005).
Genetic Relatedness, Population Structure, and Scenario Testing
A Principle Coordinate Analysis (PCoA) was conducted using GenAlEx version 6.503 (Peakall and Smouse, 2012) to quantify and visualize genetic groupings in our data. In addition, a one-way PERMANOVA was conducted using PAST (PAleontological STatistics Version 3.20) with the Bray-Curtis similarity index and 9,999 permutations (Hammer et al., 2001). STRUCTURE v2.3.4 (Pritchard et al., 2000) was used to group specimens into populations based on genetic similarity. Default parameters were used, with the burn-in length set to 100,000 iterations and 100,000 simulations. The K-values tested included K = 1 to K = 10, with 20 repetitions. To define the most likely partition of the number of clusters ΔK was calculated (Evanno et al., 2005) using Structure Harvester (Earl and von Holdt, 2012). Cluster Markov Packager Across K (CLUMPAK) was used to visualize the results (Kopelman et al., 2015).
Approximate Bayesian Computation (ABC) using DIYABC software version 2.0.4 beta (Cornuet et al., 2008) was used to test different scenarios which hypotheses of the global movement of G. brimblecombei. The genetic diversity data from the microsatellites and historical records were used to infer the most likely dispersal route of G. brimblecombei between Australia, Africa and America. Eight scenarios were tested using 1,000,000 simulated data sets for each scenario. One and two sample summary statistics included Mean Genic Diversity and Mean Size Variance, and Mean Number of Alleles, Mean Size Variance, Classification Index, and Distance between two samples, respectively. The most likely scenario was chosen based on two criteria, namely that the simulated scenario was amongst the 1% comprising the most likely scenarios, and that the scenario had the highest posterior probability, calculated by using a logistic regression, of all the scenarios tested. Several assumptions were made in the construction of tested scenarios: Australia (AUS) was defined as the native range, South America (SAM) represented by Brazil and Chile, and Mauritius and La Réunion (ISL: islands) were combined into one population due to their genetic similarity at the COI mtDNA locus. Finally, the United States and South Africa (RSA) were regarded as separate populations. All scenarios have been included, in a visual format in the Supplementary Material.
Scenario 1 tested the hypothesis that three independent introductions of G. brimblecombei occurred from the native range—an introduction from Australia to the United States with the South American population establishing as a result of a bottleneck occurring during the movement from the United States to South America, a separate introduction from Australia to the ISL (Mauritius and La Réunion), and from Australia to South Africa. Scenario 2 tested the hypothesis that a direct introduction from Australia to the United States occurred, with a subsequent bottleneck event and three independent radiation events (South America, ISL and South Africa) from the United States. Scenario 3 tested the hypothesis that all four populations, the United States, South America, South Africa and the ISL, originated directly but independently from the origin. Scenario 4 tested the hypothesis that two introductions occurred, one from the origin to the ISL (Mauritius and La Réunion) and another from the origin to the Unites States, followed by movement from the United States to South America and the emergence of the South African population from the United States population. Scenario 5 tested the hypothesis of a step-wise introduction from the origin to the United States and the ISL (i.e., from the United States to South America and then to South Africa). Scenario 6 tested the hypothesis of an introduction from Australia to the United States, with a subsequent introduction from the United States into South America and then from South America to South Africa. Subsequently an introduction from the United States to both Mauritius and La Réunion occurred. Scenario 7 tested the hypothesis of a direct introduction from Australia to the United States, then from the United States to South America, and from South America to the ISL and from the ISL to South Africa. Scenario 8 tested the hypothesis that a direct introduction of G. brimblecombei occurred from the origin to the United States and to the ISL followed by secondary introductions from ISL to South Africa and from the United States to South America.
Results
Mitochondrial DNA Sequence Diversity
A 502 bp portion of the cytochrome oxidase I region (COI) of the mitochondrial DNA was amplified for 105 specimens (GenBank accession OK335882-OK335986) and used to generate a neighbor joining tree and a haplotype network (Figure 1). The sequences contained no stop codons. Three haplotypes were present. A single haplotype was present in all introduced populations, as well as in seven specimens (77%) from the native range. The remaining two haplotypes were present only in the native range of Australia (Table 1). The percent sequence divergence between the invasive haplotype and specimens in the Australian only group ranged from 0 to 0.2%. These divergent haplotypes differed by a single base pair each and were represented by a total of two individuals.
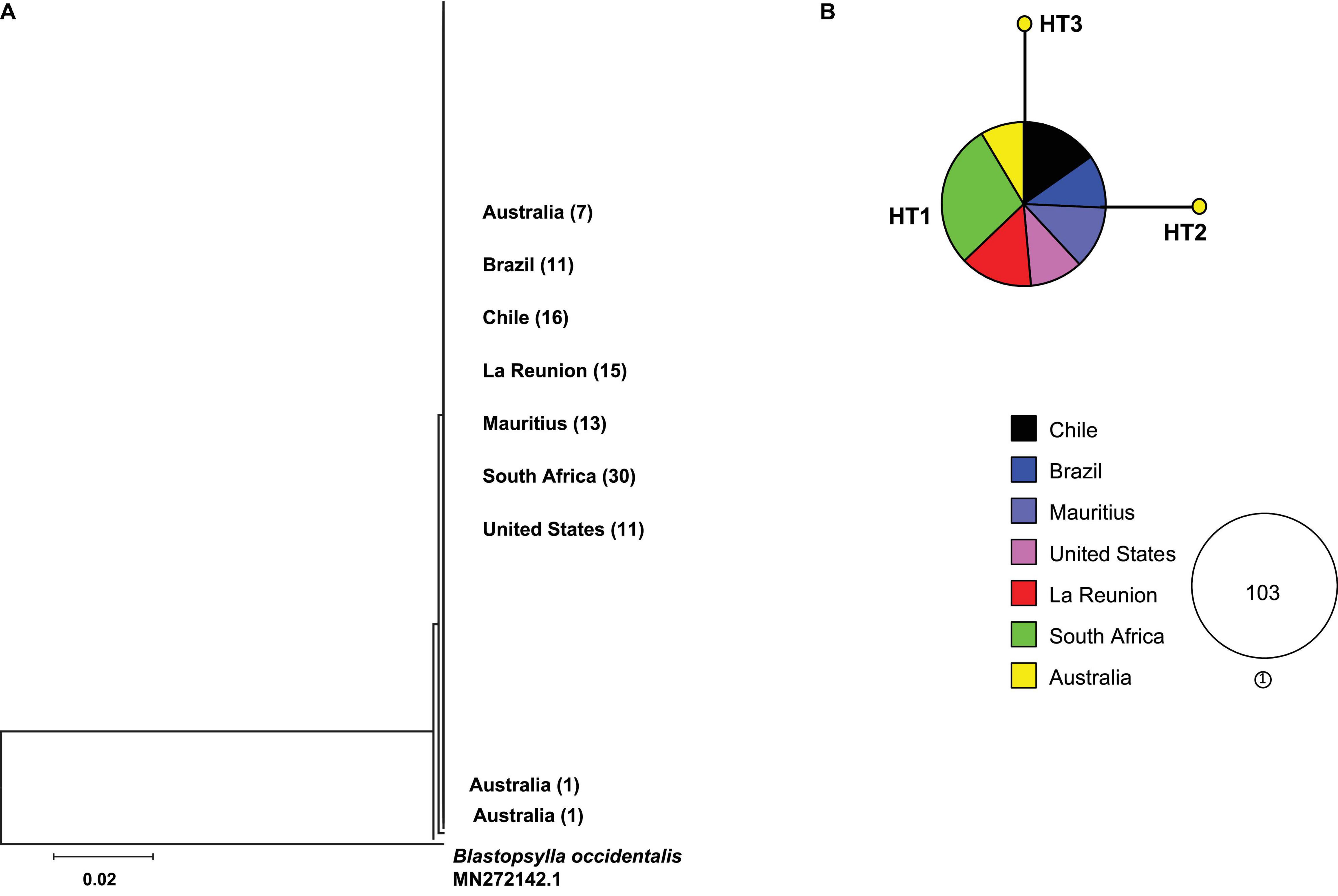
Figure 1. Neighbor joining tree based on 106 mitochondrial DNA sequences based on 502 bp of the cytochrome oxidase I (COI) mitochondrial gene. The psyllid Blastopsylla occidentalis is used as an outgroup (A). Haplotype network depicting the geographic distribution of three dominant mitochondrial haplotypes (B). Circles are proportional to the number of individuals represented. The number of individuals are shown in the empty circles next to the haplotype network. Each circle represents a single haplotype. Slices are proportional to the number of individuals per geographic location. Each line connecting a circle represents one mutational step.
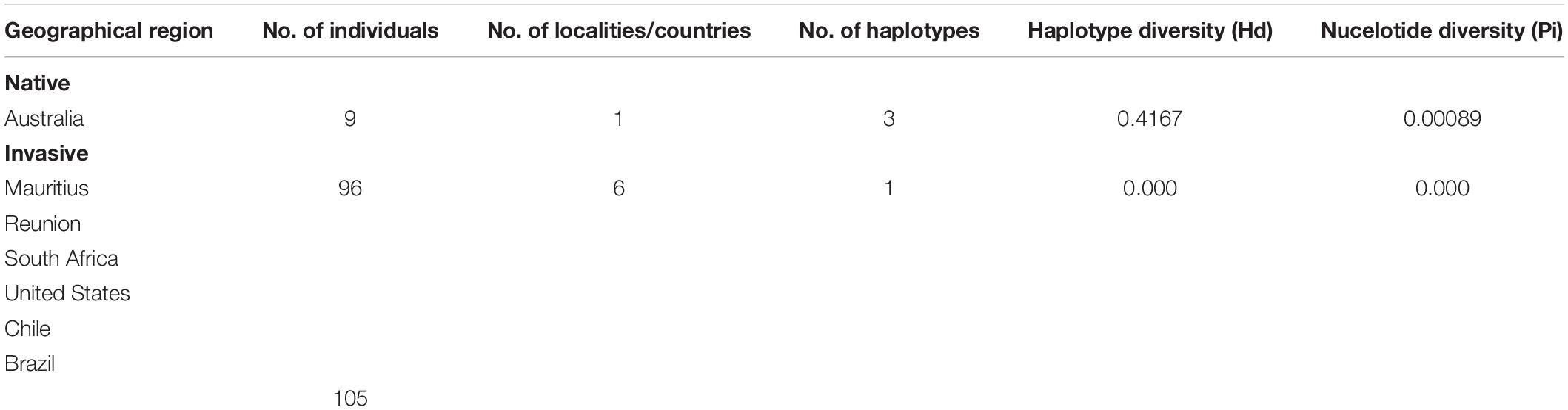
Table 1. Haplotype and nucleotide diversity of Glycaspis brimblecombei based on mitochondrial DNA data.
Microsatellite Diversity
The genome of G. brimblecombei as assembled using Illumina MiSeq reads indicated a genome size of 138 Mb, an N50 of 584 bp, contained 278,167 contigs, and a GC content of 31.7%. Of the initial twenty primers, 13 produced polymorphic fragments/alleles using a PCR analysis of three individuals; these were fluorescently labeled. Two of these primers were discarded due to scoring difficulties. The eleven remaining primers were used to produce length polymorphism data for the 105 specimens on which SSR diversity estimates are based.
Of the invaded geographical areas, the United States had the highest neutral genetic (SSR) diversity (calculated using allele frequency per locus) (0.66 ± 0.36), followed by Chile (0.57 ± 0.32), Brazil (0.56 ± 0.32), La Réunion (0.54 ± 0.30), Mauritius (0.49 ± 0.29), and South Africa (0.20 ± 0.15). The total number of alleles (A), mean number of alleles and average gene diversity was highest in the native range (Australia), as expected (Table 2). Pairwise Fst values for the seven sampled populations ranged from 0.53 ± 0.02 to 1.000 (where Fst = 1 indicates no shared alleles) and did not differ significantly from zero for any population pairs. The AMOVA analysis indicated that 30.8% variation occurred among populations within regions (df = 5; P < 0.001) while within population variation was 67.6% (df = 203; P < 0.001; Table 3). Only 1.6% of variation was attributed to region (native v. invasive; df = 1; P = 0.57).

Table 3. Analysis of the molecular variance (AMOVA) of the native (Australia) and introduced (Brazil, Chile, Mauritius, Reunion, South Africa, United States) populations of Glycaspis brimblecombei using SSR data.
The Principal Component Analysis (PCoA) suggested two clusters, one for the island group (La Réunion and Mauritius) and the another for the rest of the samples, with South Africa comprising a mini-cluster within this second cloud (Figure 2). PC 1 and PC 2 explained 22.23% and 8.99% of variation, respectively. The PERMANOVA indicated that there were significant differences (P < 0.05) between all clusters except Mauritius and La Réunion, and Brazil and Chile (Table 4).
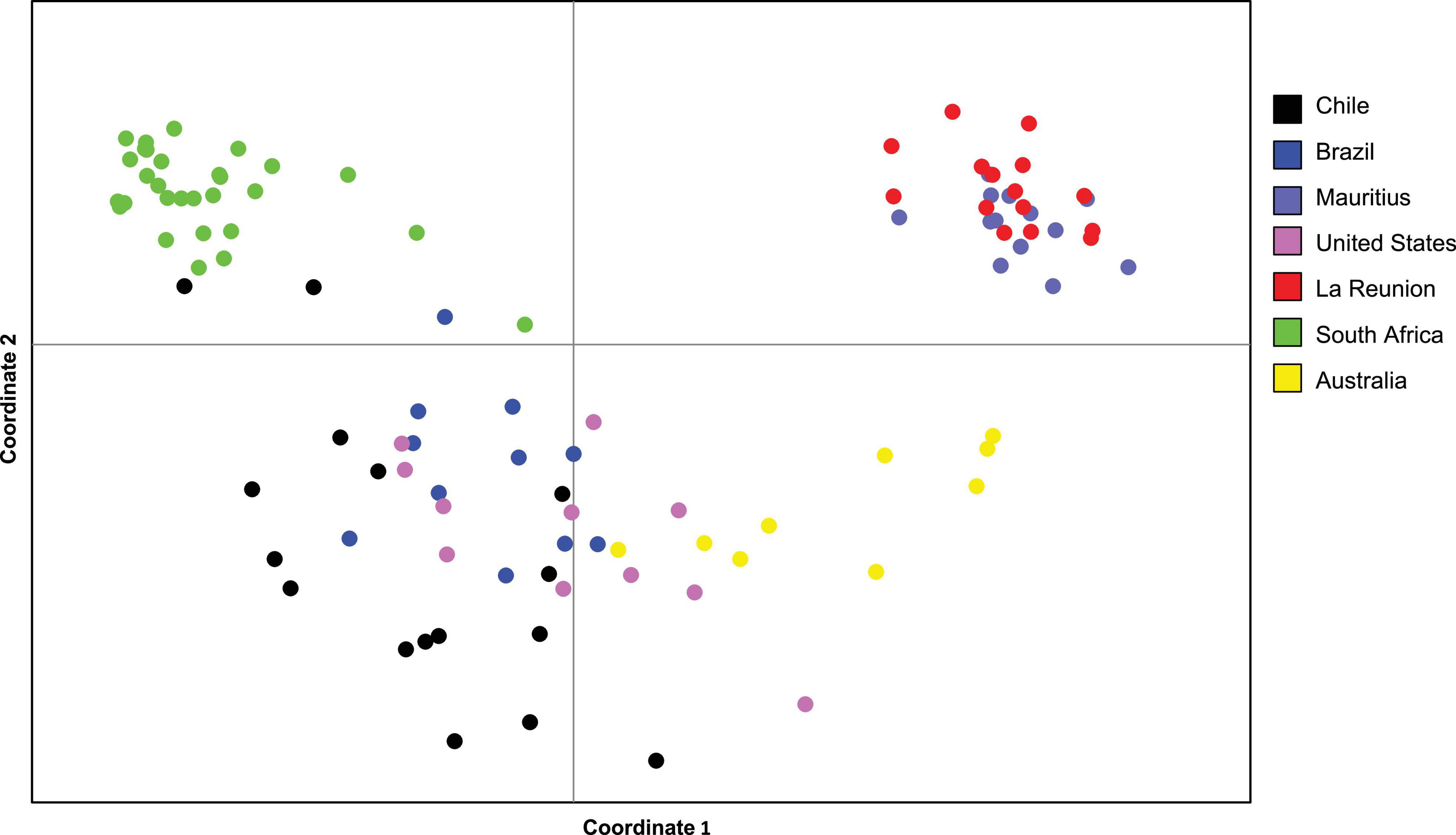
Figure 2. Principal Coordinate Analysis (PCoA) using the mean population genetic distance among populations indicating the separation of Glycaspis brimlecombei specimens from different countries.

Table 4. One-way PERMANOVA, conducted using the Bray-Curtis similarity index, indicating p-values (uncorrected significance) for Glycaspis brimblecombei using SSR data.
Analysis of population structure using STRUCTURE and STRUCTURE harvester, indicated the highest likelihood for two clusters (K = 2; Figure 3). At K = 2, one group consisted of samples from South America (Brazil and Chile), South Africa and the United States and the rest Australia and the ISL.
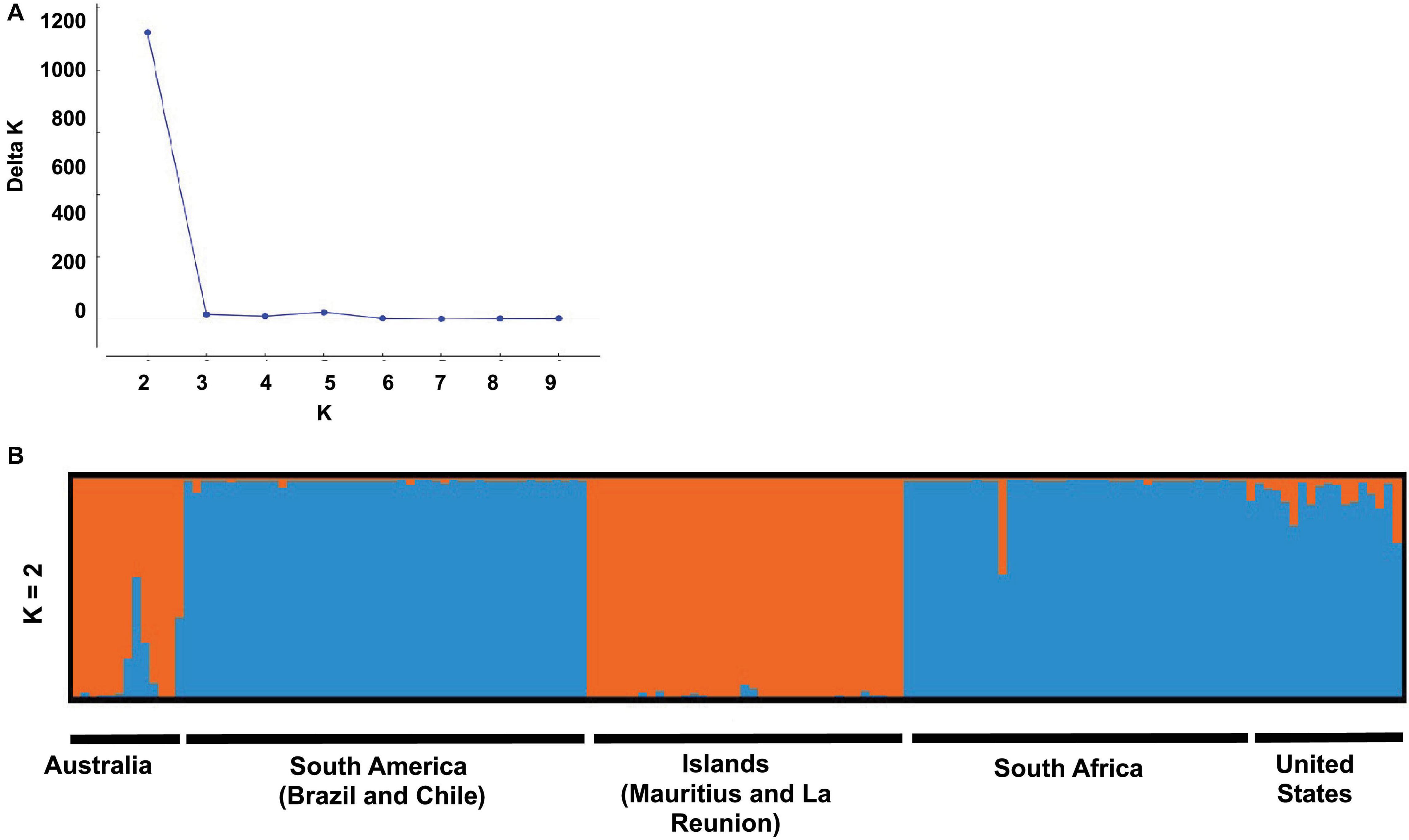
Figure 3. Population structure based on the optimal K-value (K = 2) as determined by the Evanno method (A). STRUCTURE plot indicating the grouping of specimens into populations based on genetic similarity (B).
Hypothesis testing of eight scenarios intended to identify the most likely global pattern of movement of G. brimblecombei, showed highest support for Scenario 5 representing one independent introduction from Australia to the ISL (Mauritius and La Réunion) and another from Australia to the United States, followed by a secondary introduction from the United States to the southern hemisphere adventive range (i.e., South America and South Africa; Bayesian probability value [95% confidence interval] = 0.76 [0.64; 0.87]; Figure 4 and Supplementary Material).
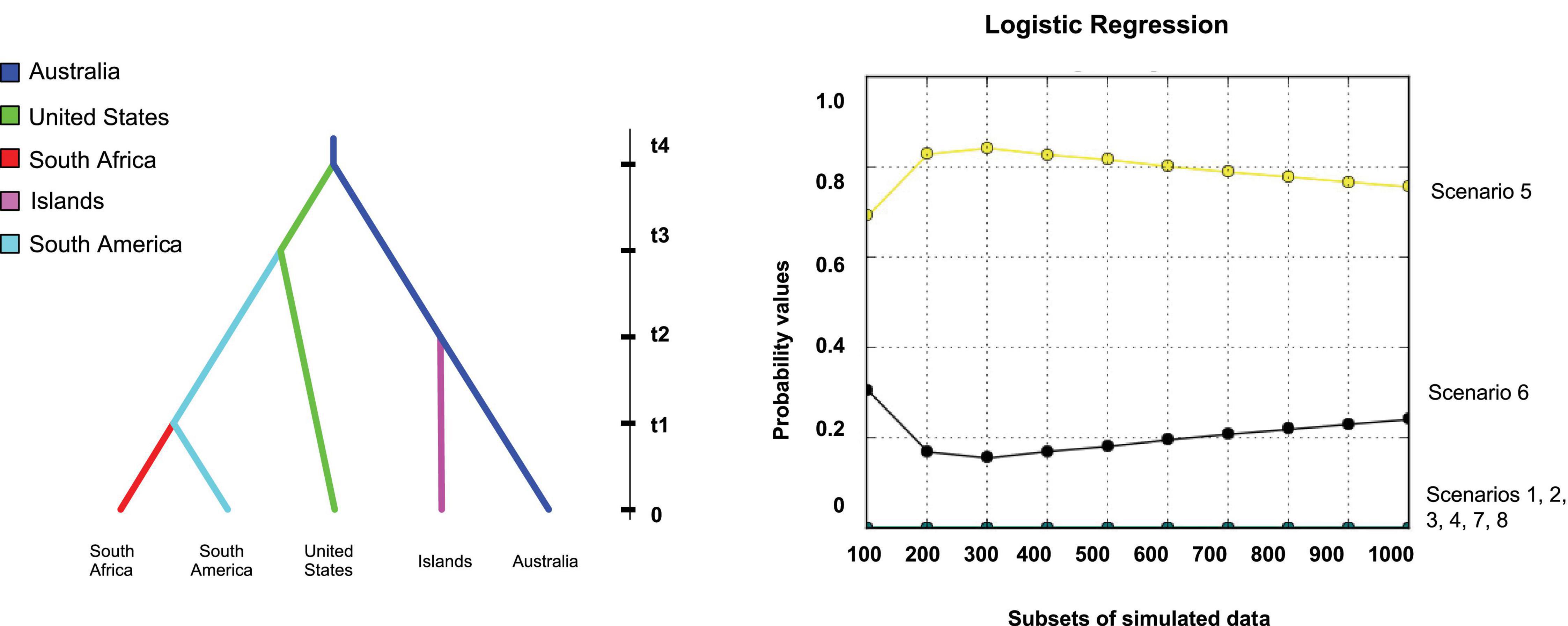
Figure 4. Global pathway of spread (Scenario 5) has been identified as the most highly supported invasive pathway of G. brimblecombei. The “Islands” refers to individuals from Mauritius and La Réunion and “South America” refers to individuals from Brazil and Chile.
Discussion
The current study is the first to characterize the genetic diversity of the red gum lerp psyllid, Glycaspis brimblecombei, across multiple regions and to evaluate among hypothesized invasion routes. We inferred the invasion routes using neutral genetic diversity from across the genome of the insect, using mitochondrial (CO1) diversity and historical records of introduction to guide which hypotheses to be tested. The study shows an invasion history outside the native range, as reported in other invasive insect pests associated with forestry (Nadel et al., 2009; Boissin et al., 2012), starting from a population established in the United States, and then spreading to South America and Africa. It also indicates that there has been a second, independent introduction into Mauritius and La Réunion. The small sample size used in this study was adequate to analyze the genetic structure of this insect population. There was only a single haplotype present in the invaded range, which would indicate that all the diversity in the invaded range had been captured in these samples. A similar study, using specimens from a number of different regions indicated no genetic diversity in the invasive range, even though the sample size was substantially larger (Dittrich-Schröder et al., 2018).
Glycaspis brimblecombei is native to Australia and this region is also confirmed as the likely source population of the invasions using both mitochondrial and nuclear data. In addition, the mitochondrial and nuclear data suggest the occurrence of a bottleneck in the invasive populations. Evidence for a bottleneck event include (i) the absence of diversity at the CO1 mtDNA locus in the introduced range, (ii) a decrease in heterozygosity in the invaded range, and (iii) a decrease in the number of alleles in the invaded range (Roderick and Navajas, 2003; Arca et al., 2015). Comparison of the genetic diversity between and within populations is a method regularly used to identify the source population (Zepeda-Paulo et al., 2010). However, these methods do not consider specific invasion scenarios, and hence additional methods are required.
We found three COI haplotypes across the native and introduced range. Haplotype 1 was observed throughout the invasive range as a whole as well as in the origin. Two haplotypes (Haplotype 2 and Haplotype 3) were each represented by a single individual from Australia. The low sequence divergence observed between the three haplotypes (0–0.2%) is typical of specimens of the same species, as is expected in this case with all collections identified as G. brimblecombei. Previous studies on psyllid species delimitation have indicated that a 3% uncorrected p-distance threshold for cytochrome oxidase I (COI) divergence is a likely guideline for psyllid species identification (Taylor et al., 2016; Percy, 2017; Wonglersak et al., 2017; Martoni et al., 2018).
The Australian population of G. brimblecombei contained the highest genetic diversity, consistent with other studies that have shown more diversity in populations collected from the native range (Cifuentes et al., 2011). Of the invaded geographical areas, the United States had the highest genetic diversity followed by Chile, Brazil, La Réunion, Mauritius, and South Africa, respectively. The South African group had the lowest genetic diversity among the invaded populations, which could be due to a founder effect or genetic drift in this population following introduction. This pattern could reflect a genetic bottleneck if the number of colonizing individuals was low or genetic drift occurred during establishment. Reduced genetic variation is frequently observed within invasive ranges, though this pattern can be ameliorated by multiple introductions, admixture, and rapid population growth (Rius and Darling, 2014; Garnas et al., 2016). Similarly, reduced genetic variation has been observed in a number of other recently introduced invasive species in their invaded range [e.g., Thaumastocoris peregrinus (Nadel et al., 2009; Mutitu et al., 2020); Anoplophora glabripennis (Carter et al., 2010); Halyomorpha halys (Cesari et al., 2018); Vespula germanica (Eloff et al., 2020); amongst many others]. There is little evidence, however, that low genetic diversity represents a major constraint on adaptive evolution (Garnas, 2018).
Approximate Bayesian Computation (ABC) scenario testing supported two independent introduction events from the native Australian range; one into the United States with subsequent, secondary introductions into South America and South Africa, and another from Australia into Mauritius and La Réunion. The STRUCTURE analysis also provides support for two independent groups with the Australian, Mauritian and La Réunion populations grouping together, and the South American (Brazil and Chile), South African and United States populations grouping together. Similar patterns of multiple independent introductions from species’ native ranges have been shown for various other plantation insect pests, such as Thaumastocoris peregrinus (Nadel et al., 2009), Sirex noctilio (Boissin et al., 2012), three species of Gonipterus weevils (Mapondera et al., 2012), and Leptocybe invasa (Dittrich-Schröder et al., 2018). In some studies, multiple introductions were speculated to result from increases in the population of the introduced organism within the native range likely to have acted as a proximal source of invasive propagules. This suggests that monitoring of insect pests in native ranges of major crops, such as Eucalyptus in this case, could help predict potential future invasions. Little is known about G. brimblecombei in its native range (Santos et al., 2020).
The invasive United States population was the first to be detected outside of Australia and has served as a source of a number of subsequent introductions. Numerous examples exist where invasive species have become a source for further introductions and this process is referred to as the bridgehead effect (Lombaert et al., 2010; Ascunce et al., 2011; Yang et al., 2012). If this population emerges as a disproportionate driver of global spread, it could plausibly be considered a “bridgehead” population (Lombaert et al., 2010; Ascunce et al., 2011; Yang et al., 2012; Santos et al., 2020). One explanation of this phenomenon is that individuals in these bridgehead populations acquire traits associated with successful establishment and spread (Bertelsmeier and Keller, 2018). Such populations are important to monitor for the emergence of new genotypes and/or evidence of adaptive evolution. Alternatively, this effect could simply reflect larger population sizes or greater connectivity via global trade and travel (Garnas et al., 2012, 2016).
Scenario testing and the STRUCTURE results suggest that the G. brimblecombei invasion occurred from Australia to La Réunion and Mauritius (both islands), and not between these islands and South Africa, which could have been predictable due to their geographic proximity. Projections based on historical invasion rates of insect pests in Mauritius suggest that one new insect pest is expected to be recorded each year, perhaps increasing with time (Russel et al., 2017; Mauremootoo et al., 2019). Islands have been shown to be more susceptible to invasion by non-native species (Sol, 2000; Simberloff, 2003; Gimeno et al., 2006), including forest pests and pathogens (Brockerhoff and Liebhold, 2017). The high invasibility of islands makes them a potential hub for future invasion elsewhere globally.
Understanding invasion pathways of insect pests is important in order to control spread as well as to prevent future escapes from the native range (Beggs et al., 2011; Masciocchi and Corley, 2013; Eloff et al., 2020). This study demonstrates how detailed knowledge of spread pathways can be obtained using neutral molecular markers and surveillance data (Eloff et al., 2020). Our results provide an additional example of multiple independent introductions followed by spread within the adventive range that would be difficult to predict without the use of molecular tools and associated analytical approaches. Further elucidation of these pathways is crucial to prevent additional introductions in future (Eloff et al., 2020). While genetic diversity of G. brimblecombei and other pests of forests and forestry is moderately reduced relative to the native range, this does not appear to impact population fitness across a range of environments nor the potential for future spread.
Data Availability Statement
The datasets presented in this study can be found in online repositories. The names of the repository/repositories and accession number(s) can be found below: NCBI (accessions: OK335882–OK335986).
Author Contributions
GD-S, JG, DA-C, RA, BH, SL, and BS designed the research. GD-S and DA-C performed the research. GD-S, JG, DA-C, and BS analyzed the data. JG, RA, BH, SL, and BS provided material and contributed to interpretation of the results. All authors contributed to the writing of the manuscript.
Funding
Members of the Tree Protection Co-operative Programme (TPCP) and the DSI NRF Centre of Excellence in Plant Health Biotechnology were acknowledged for providing funding.
Conflict of Interest
The authors declare that the research was conducted in the absence of any commercial or financial relationships that could be construed as a potential conflict of interest.
Publisher’s Note
All claims expressed in this article are solely those of the authors and do not necessarily represent those of their affiliated organizations, or those of the publisher, the editors and the reviewers. Any product that may be evaluated in this article, or claim that may be made by its manufacturer, is not guaranteed or endorsed by the publisher.
Acknowledgments
We thank Timothy Paine (University of California, Riverside) and Carlos Wilcken (Sâo Paulo State University) for supplying specimens, in addition to those provided collected by the authors, without which this study would not have been possible. Renate Zipfel (FABI, University of Pretoria) is thanked for assistance with the microsatellite-related work.
Supplementary Material
The Supplementary Material for this article can be found online at: https://www.frontiersin.org/articles/10.3389/ffgc.2021.783603/full#supplementary-material
Supplementary Figure 1 | The eight scenarios tested to determine the most likely route of introduction of Glycaspis brimblecombei. The “Islands” refers to individuals from Mauritius and La Réunion and “South America” refers to individuals from Brazil and Chile.
Footnotes
References
Ali, S., Gladieux, P., Leconte, M., Gautier, A., Justesen, A., HovmØller, M., et al. (2014). Origin, migration routes and worldwide population genetic structure of the wheat yellow rust pathogen Puccinia striiformis f.sp. tritici. PLoS Pathog. 10:e1003903. doi: 10.1371/journal.ppat.1003903
Arca, M. A., Mougel, F., Guillemaud, T., Dupas, S., Rome, Q., Perrard, A., et al. (2015). Reconstructing the invasion and the demographic history of the yellowlegged hornet, Vespa velutina, in Europe. Biol. Invas. 17, 2357–2371.
Ascunce, M. S., Yang, C. C., Oakey, J., Calcaterra, L., Wu, W. J., and Shih, C. J. (2011). Global invasion history of the fire ant Solenopsis invicta. Science 331, 1066–1068. doi: 10.1126/science.1198734
Beggs, J. R., Brockerhoff, E. G., Corley, J. C., Kenis, M., Masciocchi, M., Muller, F., et al. (2011). Ecological effects and management of invasive alien Vespidae. Biocontrol 56, 505–526.
Bella, S., and Rapisarda, C. (2013). Forst record from Greece of the invasive red gum lerp psyllid Glycaspis brimblecombei Moore (Hemiptera Psyllidae) and its associated parasitoid Psyllaephagus bliteus Riek (Hymenoptera Encyrtidae). Redia XCVI, 33–35.
Ben Attia, S., and Rapisarda, C. (2014). First record of the red gum lerp psyllid, Glycaspis brimblecombei Moore (Hemiptera Psyllidae), in Tunisia. Phytoparasitica 42, 535–539.
Bertelsmeier, C., and Keller, L. (2018). Bridgehead effects and role of adaptive evolution in invasive Populations. Trends Ecol. Evol. 7, 527–534. doi: 10.1016/j.tree.2018.04.014
Boissin, E., Hurley, B., Wingfield, M. J., Vasaitis, R., Stenlid, J., Davis, C., et al. (2012). Retracing the routes of introduction of invasive species: the case of the Sirex noctilio woodwasp. Mol. Ecol. 21, 5728–5744. doi: 10.1111/mec.12065
Brennan, E., Hrusa, F., Weinbaum, S., and Levison, W. (2001). Resistance of Eucalyptus species to Glycaspis brimblecombei (Homoptera: Psyllidae) in the San Francisco Bay area. Pan Pac. Entomol. 77, 249–253.
Brockerhoff, E. G., and Liebhold, A. M. (2017). Ecology of forest insect invaions. Biol. Invas. 19, 3141–3159.
Brockerhoff, E. G., Jactel, H., Parrotta, J. A., and Ferraz, S. F. B. (2013). Role of eucalypt and other planted forsts in biodiversity conservation and the provision of biodiversity-related ecosystem services. Forest Ecol. Manag. 301, 43–50. doi: 10.1016/j.foreco.2012.09.018
Bush, S. J., Slippers, B., and Hurley, B. P. (2020). Eucalypt susceptibility towards the invasive Glycaspis brimblecombei Moore (Hemiptera : Aphalaridae) in South Africa. South. Forests 82, 243–252. doi: 10.2989/20702620.2020.1824556
Caleca, V., Bella, S., La Pergola, A., Lombardo, A., Lo Verde, G., Maltese, M., et al. (2018). Environmental factors and incidence of parasitism of Psyllaephagus bliteus Riek (Hymenoptera, Encyrtidae) on populations of Glycaspis brimblecombei Moore (Hemiptera, Aphalaridae) in Mediterranean climatic areas. Redia 1010, 89–100. doi: 10.19263/redia-101.18.12
Carter, M., Smith, M., and Harrison, R. (2010). Genetic analyses of the Asian longhorn beetle (Coleoptera, Cerambycidae, Anoplophora glabripennis), in North America, Europe and Asia. Biol. Invas. 12, 1165–1182. doi: 10.1007/s10530-009-9538-9
Cesari, M., Maistrello, L., Piemontese, L., Bonini, R., Diolo, P., Lee, W., et al. (2018). Genetic diversity of the brown marmorated stink bug Halyomorpha halys in the invaded territories of Europe and its patterns of diffusion in Italy. Biol. Invas. 20, 1073–1092. doi: 10.1007/s10530-017-1611-1
Cifuentes, D., Chynoweth, R., and Bielza, P. (2011). Genetic study of Mediterranean and South American populations of tomato leafminer Tuta absoluta (Povolny, 1994) (Lepidoptera: Gelechiidae) using ribosomal and mitochondrial markers. Pest Manag. Sci. 67, 1155–1162. doi: 10.1002/ps.2166
Cornuet, J. M., Santos, F., Beaumont, M. A., Robert, C. P., Marin, J. M., Balding, D. J., et al. (2008). Inferring population history with DIY ABC: a user-friendly approach to approximate Bayesian computation. Bioinformatics 24, 2713–2719. doi: 10.1093/bioinformatics/btn514
Dahlsten, D. L., Daane, K. M., Paine, T. D., Sime, K. R., Lawson, A. B., Rowney, D. L., et al. (2005). Imported parasitic wasp helps control red gum lerp psyllid. Calif. Agric. 59, 229–234.
de Queiroz, D. L., Majer, J., Burckhardt, D., Zanetti, R., Fernandez, J. I. R., de Queiroz, E. C., et al. (2013). Predicting the geographical distribution of Glycaspis brimblecombei (Hemiptera: Psylloidea) in Brazil. Austr. J. Entomol. 52, 20–30.
Dittrich-Schröder, G., Hoareau, T. B., Hurley, B. P., Wingfield, M. J., Lawson, S., Nahrung, H. F., et al. (2018). Population genetic analyses of complex global insect invasions in managed landscapes: a Leptocybe invasa (Hymenoptera) case study. Biol. Invas. 20, 2395–2420. doi: 10.1007/s10530-018-1709-0
Earl, D., and von Holdt, B. (2012). STRUCTURE HARVESTER: a website and program for visualizing STRUCTURE output and implementing the nnonno method. Conserv. Genet. Resour. 4, 359–361.
Eloff, J., Veldtman, R., Bulgarella, M., and Lester, P. J. (2020). Population genetics of the invasive wasp Vespula germanica in South Africa. Insectes Soc. 67, 229–238. doi: 10.1007/s00040-020-00752-x
Estoup, A., and Guillemaud, T. (2010). Reconstructing routes of invasion using genetic data: why, how and so what? Mol. Ecol. 19, 4113–4130. doi: 10.1111/j.1365-294X.2010.04773.x
Evanno, G., Regnaut, S., and Goudet, J. (2005). Detecting the number of clusters of individuals using the software STRUCRURE: a simulation study. Mol. Ecol. 14, 2611–2620. doi: 10.1111/j.1365-294X.2005.02553.x
Excoffier, L., Laval, G., and Schneider, S. (2005). ARLEQUIN version 3.0: an integrated software package for population genetics data analysis. Evol. Bioinform. Online 1, 47–50.
Faircloth, B. C. (2008). MSATCOMMANDER: detection of microsatellite repeat arrays and automated, locus-specific primer design. Mol. Ecol. Resour. 8, 92–94. doi: 10.1111/j.1471-8286.2007.01884.x
Foucaud, J., Orivel, J., Loiseau, A., Delabie, J. H. C., Jourdan, H., Konghouleux, D., et al. (2010). Worldwide invasion by the little fire ant: routes of introduction and eco-evolutionary pathways. Evol. Appl. 3, 363–374. doi: 10.1111/j.1752-4571.2010.00119.x
Frasconi, C., Rossi, E., Antonelli, R., and Loni, A. (2013). First record of the red gum lerp psyllid Glycaspis brimblecombei Moore (Hemiptera: Psyllidae) in Tuscany (Italy). EPPO Bull. 43, 167–168.
Garnas, J. R. (2018). Rapid evolution of insects to global environmental change: conceptual issues and empirical gaps. Curr. Opin. Insect Sci. 29, 93–101. doi: 10.1016/j.cois.2018.07.013
Garnas, J. R., Auger-Rozenberg, M. A., Roques, A., Bertelsmeier, C., Wingfield, M. J., Saccaggi, D., et al. (2016). Complex patterns of global spread in invasive insects: eco-evolutionary and management consequences. Biol. Invas. 18, 935–952.
Garnas, J. R., Hurley, B. P., Slippers, B., and Wingfield, M. J. (2012). Biological control of forest plantation pests in an interconnected world requires greater international focus. Int. J. Pest Manag. 58, 211–223. doi: 10.1080/09670874.2012.698764
Gimeno, I., Vila, M., and Hulme, P. E. (2006). Are islands more susceptible to plant invasion than continents? A test using Oxalis pes-caprae L. in the western Mediterranean. J. Biogeogr. 33, 1559–1565. doi: 10.1111/j.1365-2699.2006.01525.x
Gonçalves, J. L. M., Alvares, C. A., Higa, A. R., Silva, L. D., Alfenas, A. C., Stahl, J., et al. (2013). Integrating genetic and silvicultural strategies to minimize abiotic and biotic constraints in Brazilian eucalypt plantations. For. Ecol. Manag. 301, 6–27.
Hammer, Ø, Harper, D. A. T., and Ryan, P. D. (2001). PAST: paleontological statistics software package for education and data analysis. Palaeontol. Electron. 4, 1–9.
Hoffmann, A. A., Reynolds, K. T., Nash, M. A., and Week, A. R. (2008). A high incidence of parthenogenesis in agricultural pests. Proc. R. Soc. Lond. B 275, 2473–2481. doi: 10.1098/rspb.2008.0685
Huerta, A., Jaramillo, J., and Araya, J. E. (2011). Establishment of the red gum psyllid parasitoid. For. Syst. 20, 339–347.
Hurley, B. P., Garnas, J., Wingfield, M. J., Branco, M., Richardson, D. M., and Slippers, B. (2016). Increasing numbers and intercontinental spread of invasive insects on eucalypts. Biol. Invas. 18, 921–933. doi: 10.1007/s10530-016-1081-x
Junk, J., Eickermann, M., Milenovic, M., Suma, P., and Rapisarda, C. (2020). Re-visiting the incidence of environmental factors on a pre-imaginal population of the red gum lerp psyllid Glycaspis brimblecombei Moore. Insects 11:860. doi: 10.3390/insects11120860
Kojwang, H. O. (2019). Forest pests and diseases of Southern Africa: a review. Afr. J. Rural Dev. 4, 65–91.
Kopelman, N. M., Mayzel, J., Jakobsson, M., Rosenberg, N. A., and Mayrose, I. (2015). CLUMPAK: a program for identifying clustering modes and packaging population structure inferences across K. Mol. Ecol. Resour. 15, 1179–1191. doi: 10.1111/1755-0998.12387
Kumar, S., Stecher, G., and Tamura, K. (2016). MEGA7: molecular evolutionary genteics analysis version7.0 for bigger datasets. Mol. Biol. Evol. 33, 1870–1874. doi: 10.1093/molbev/msw054
Larsson, A. (2014). AliView: a fast and lightweight alignment viewer and editor for large data sets. Bioinformatics 30, 3276–3278. doi: 10.1093/bioinformatics/btu531
Laudonia, S., and Garonna, A. P. (2010). The red gum lerp psyllid, Glycaspis brimblecombei, a new exotic pest of Eucalyptus camaldulensis in Italy. Bull. Insectol. 63, 233–236.
Laudonia, S., Margiotta, M., and Sasso, R. (2014). Seasonal ocurrence and adaptaion of the exotic Glycaspis brimblecombei Moore (Hemiptera: Aphalaridae) in Italy. J. Nat. Hist. 48, 675–689.
Lombaert, E., Guillemaud, T., Cornuet, J. M., Malausa, T., Facon, B., and Estoup, A. (2010). Bridgehead Effect in the worldwide invasion of the biocontrol harlequin ladybird. PLoS One 5:e9743. doi: 10.1371/journal.pone.0009743
Lucia, A., Naspi, C., Zerba, E., and Masuh, H. (2016). Infestation of Glycaspis brimblecombei Moore on thirteen Eucalyptus species and their relationship with the chemical composition of essential oils. J. Insects 2016, 1–7.
Makunde, P. T., Slippers, B., Burckhardt, D., de Quieroz, D. L., Lawson, S. A., and Hurley, B. P. (2020). Current and potential threat of psyllids (Hemiotera: Psylloidea) on eucalypts. South. For. 82, 233–242.
Mapondera, T. S., Burgess, T., Matsuki, M., and Oberprieler, R. G. (2012). Identification and molecular phylogenetics of the cryptic species of the Gonipterus scutellatus complex (Coleoptera: Curculionidae: Gonipterini). Austral Entomol. 51, 175–188. doi: 10.1111/j.1440-6055.2011.00853.x
Margiotta, M., Bella, S., Buffa, F., Caleca, V., Floris, I., Giorno, V., et al. (2017). Modeling environmental influences in the Psyllaephagus bliteus (Hymenoptera: Encyrtidae) – Glycaspis brimblecombei (Hemiptera: Apohalaridae) parasitoid – host system. J. Econ. Entomol. 110, 491–501. doi: 10.1093/jee/tow253
Martoni, F., Bulman, S., Pitman, A., Taylor, G., and Armstrong, K. (2018). DNA barcoding highlights cryptic diversity in the New Zealand Psylloidea (Hemiptera:Sternoeehyncha). Diversity 10:50.
Masciocchi, M., and Corley, J. (2013). Distribution, dispersal and spread of the invasive social wasp (Vespula germanica) in Argentina. Austral Ecol. 38, 162–168. doi: 10.1111/1744-7917.12374
Mauremootoo, J. R., Pandoo, S., Bachraz, V., Buldawoo, I., and Cole, N. C. (2019). “Invasive species management in Mauritius: from the reactive to the proactive – the National invasive species management strategy and its inplementation,” in Island Invasives: Scaling up to Meet the Challenge: Occasional Paper SSC no. 62, eds C. R. Veitch, M. N. Clout, A. R. Martin, J. C. Russell, and C. J. West (Gland: IUCN), 503–509.
Mutitu, E., Hoareau, T. B., Hurley, B. P., Garnas, J. R., Wingfield, M. J., and Slippers, B. (2020). Reconstructing early routes of invasion of the bronze bug Thaumastocoris peregrinus (Hemiptera: Thaumastocoridae): cities as bridgeheads for global pest invasions. Biol. Invas. 22, 2325–2338. doi: 10.1007/s10530-020-02258-w
Nadel, R. L., Slippers, B., Scholes, M. C., Lawson, S. A., Noack, A. E., Wilcken, C. F., et al. (2009). DNA bar-coding reveals source and patterns of Thaumastocoris peregrinus invasions in South Africa and South America. Biol. Invas. 12, 1067–1077. doi: 10.1007/s10530-009-9524-2
Ndlela, S., Manyangadze, T., Sachisuko, A., van der Lingen, S., and Makowe, I. A. (2018). The distribution and management of two invasive pests of Eucalyptus: the red gum lerp psyllid, Glycaspis brimblecombei (Hemiptera: Psylloidea), and the blue gum chalcid wasp, Leptocybe invasa (Hymenoptera: Eulophidae), in Zimbabwe. Afr. Entomol. 26, 104–115.
Nishiguchi, M. K., Doukakis, P., Egan, M., Kizirian, D., Phillips, A., Prendini, L., et al. (2002). “DNA isolation procedures,” in Techniques in Molecular Systematics and Evolution. Methods and Tools in Biosciences and Medicine, eds R. DeSalle, G. Giribet, and W. Wheeler (Basel: Birkhäuser).
Peakall, R., and Smouse, P. E. (2012). GenAlEx 6.5: genetic analysis in Excel. Population genetic software for teaching and research-an update. Bioinformatics 28, 2537–2539. doi: 10.1093/bioinformatics/bts460
Percy, D. M. (2017). Making the mosts of your host: the Metrosideros-feeding psyllids (Hemiptera: Psylloidea) of the Hawaiian Islands. ZooKeys 649, 1–163. doi: 10.3897/zookeys.649.10213
Polzin, T., and Daneschmand, S. V. (2003). On Steiner trees and minimum spanning trees in hypergraphs. Oper. Res. Lett. 31, 12–20. doi: 10.1016/s0167-6377(02)00185-2
Pritchard, J. K., Stephens, M., and Donnelly, P. (2000). Inference of population structure using multilocus genotype data. Genetics 155, 945–959. doi: 10.1093/genetics/155.2.945
Rius, M., and Darling, J. A. (2014). How important is intraspecific genetic admixture to the success of colonising populations? Trends Ecol. Evol. 29, 233–242. doi: 10.1016/j.tree.2014.02.003
Roderick, G. K., and Navajas, M. (2003). Genes in new environments: genetics and evolution in biological control. Nat. Rev. Genet. 4, 889–899. doi: 10.1038/nrg1201
Rozas, J., Sánches-DelBarrio, J. C., Messeguer, X., and Rozas, R. (2003). DnaSP, DNA polymorphism analyses by the coalescent and other methods. Bioinformatics 18, 2496–2497. doi: 10.1093/bioinformatics/btg359
Russel, J. C., Meyer, J. Y., Holmes, N. D., and Pagad, S. (2017). Invasive Alien speceis on islands: impacts, distribution, interactions and management. Environ. Conserv. 44, 359–370. doi: 10.1017/s0376892917000297
Santos, F. A., Correa, A. S., Nanini, F., Nascimento, D. A., Junqueira, L. R., and Wilcken, C. F. (2020). Genetic diversity of Glycaspis brimblecombei (Hemiptera: Aphalaridae) and its parasitoid Psyllaephagus bliteus (Hymenoptera: Encyrtidae) in Brazil. Braz. J. Biol. 8, 838–841. doi: 10.1590/1519-6984.230486
Simberloff, D. (2003). How much information on population biology is needed to manage introduced species? Conserv. Biol. 17, 83–92. doi: 10.1046/j.1523-1739.2003.02028.x
Simon, C., Frati, F., Beckenbach, A., Crespi, B., Liu, H., and Flook, P. (1994). Evolution, weighting numbers, and phylogenetic utility of mitochondrial gene sequences and a compilation of conserved polymerase chain reaction primers. Ann. Entomol. Soc. Am. 87, 651–701.
Sol, D. (2000). Are islands more susceptible to be invaded than continents? Birds say no. Ecography 23, 687–692.
Spodek, M., Burckhardt, D., Protasov, A., and Mendel, Z. (2015). Firest record of two invasive eucalypt psyllids (Hemiptera: Psylloidea) in Israel. Phytoparasitica 43, 401–406. doi: 10.1007/s12600-015-0465-2
Taylor, G. S., Fagan-Jeffries, E. P., and Austin, A. D. (2016). A new genus and twenty new species of Australian jumping plant-lice (Psylloidea: Triozidae) from Eremophila and Myoporum (Scrophulariaceae: Myoporeae). Zootaxa 4073, 1–84. doi: 10.11646/zootaxa.4073.1.1
Tsagkarakis, A. E., Kalaitzaki, A. P., and Balotis, G. N. (2014). Note on Glycaspis brimblecombei Moore (Hemiptera Psyllidae): a new pest of Eucalyptus in Greece. Adv. Entomol. 2, 57–59.
Untergrasser, A., Cutcutache, I., Koressaar, T., Ye, J., Faircloth, B. C., Remm, M., et al. (2012). Primer3 – new capabilities and interfaces. Nucleic Acids Res. 40:e115. doi: 10.1093/nar/gks596
Wingfield, M. J., Brocherhoff, E., Wingfield, B. D., and Slippers, B. (2015). Planted forest health: the need for a global strategy. Science 349, 832–836. doi: 10.1126/science.aac6674
Wonglersak, R., Cronk, Q. C., and Percy, D. (2017). Salix transect of Europe: structured genetic variation and isolation-by-distance in the nettle psyllid, Trioza urticae (Psylloidea, Hemiptera), from Greece to Arctic Norway. Biodiv. Data J. 5:e10824. doi: 10.3897/BDJ.5.e10824
Yang, X. M., Sun, J. T., Xue, X. F., Li, J. B., and Hong, X. Y. (2012). Invasion genetics of the western flower thrips in China: evidence for genetic bottleneck, hybridization and bridgehead effect. PLoS One 7:e34567. doi: 10.1371/journal.pone.0034567
Zepeda-Paulo, F., Simon, J., Ramírez, C., Fuentes-Contreras, E., Margaritopoulos, J., Wilson, A., et al. (2010). The invasion route for an insect pest species: the tobacco aphid in the New World. Mol. Ecol. 19, 4738–4752. doi: 10.1111/j.1365-294X.2010.04857.x
Keywords: invasion pathways, invasive insect pest, Eucalyptus spp., population genetics, forest health
Citation: Dittrich-Schröder G, Garnas JR, Arriagada-Cares D, Ahumada R, Hurley BP, Lawson SA and Slippers B (2021) Diversity and Introduction History of Glycaspis brimblecombei Reflects a History of Bridgeheads and Distinct Invasions. Front. For. Glob. Change 4:783603. doi: 10.3389/ffgc.2021.783603
Received: 26 September 2021; Accepted: 26 November 2021;
Published: 22 December 2021.
Edited by:
Casper Nyamukondiwa, Botswana International University of Science and Technology, BotswanaReviewed by:
Carmelo Rapisarda, University of Catania, ItalyMário Marcos Do Espírito-Santo, Unimontes, Brazil
Copyright © 2021 Dittrich-Schröder, Garnas, Arriagada-Cares, Ahumada, Hurley, Lawson and Slippers. This is an open-access article distributed under the terms of the Creative Commons Attribution License (CC BY). The use, distribution or reproduction in other forums is permitted, provided the original author(s) and the copyright owner(s) are credited and that the original publication in this journal is cited, in accordance with accepted academic practice. No use, distribution or reproduction is permitted which does not comply with these terms.
*Correspondence: Gudrun Dittrich-Schröder, Gudrun.Dittrich@fabi.up.ac.za