- 1School of Natural Resources and the Environment, University of Arizona, Tucson, AZ, United States
- 2Department of Family and Community Medicine, University of New Mexico Health Sciences Center, University of New Mexico, Albuquerque, NM, United States
- 3O’Neill School of Public and Environmental Affairs, Indiana University, Bloomington, IN, United States
- 4Department of Natural Resources and Environmental Science, Program in Ecology, Evolution, and Conservation Biology, University of Nevada, Reno, NV, United States
- 5Department of Biology, University of North Carolina, Chapel Hill, NC, United States
- 6School of Environmental and Conservation Sciences, Murdoch University, Murdoch, WA, Australia
- 7Biodiversity and Conservation Science, Department of Biodiversity, Conservation and Attractions, Kensington, WA, Australia
- 8Grupo de Investigación en Ecología Aplicada, Facultad de Ingeniería, Universidad de Antioquia, Medellín, Colombia
Mortality of tree species around the globe is increasingly driven by hotter drought and heat waves. Tree juveniles are at risk, as well as adults, and this will have a negative effect on forest dynamics and structure under climate change. Novel management options are urgently needed to reduce this mortality and positively affect forest dynamics and structure. Potential drought-ameliorating soil amendments such as nanochitosan – a biopolymer upcycled from byproducts of the seafood industry – may provide an additional set of useful tools for reducing juvenile mortality during hotter droughts. Nanochitosan promotes water and nutrient absorption in plants but has not been tested in the context of drought and heat stress. We evaluated factors affecting mortality risk and rate for dryland Pinus edulis juveniles (2–3 years old) in a growth chamber using a factorial experiment that included ambient and +4°C warmer base temperatures, with and without a 10 day +8°C heat wave, and with and without a nanochitosan soil amendment. The nanochitosan treatment reduced the relative risk of mortality, emphasizing a protective function of this soil amendment, reducing the relative risk of mortality by 37%. Importantly, the protective effects of nanochitosan soil amendment in delaying tree mortality under hotter drought and heat waves provides a new, potentially positive management treatment for tree juveniles trying to survive in the climate of the Anthropocene.
Introduction
Drought accompanied by hotter temperatures is driving an increase in tree mortality worldwide (Allen et al., 2010; Hammond et al., 2022; McDowell et al., 2022). Global tree mortality (recorded from field observations) is being documented in response to a combination of metrics related to the extent or severity of drought and to warmer temperatures, such as maximum temperature of the hottest month (Hammond et al., 2022). Although factors such as catastrophic wildfire and pathogen outbreaks cause tree mortality (e.g., Hicke et al., 2016), earlier life stages—juveniles as well as seedlings—are particularly vulnerable to extreme heat and drought (e.g., Kolb and Robberecht, 1996). Tree species distribution and population persistence are highly dependent on seed dispersal and appropriate, favorable site conditions for germination, seedling establishment and survival, and for established individuals to develop as understory juveniles that can later become overstory adult trees (Grubb, 1977; Dey et al., 2019).
As temperature increases during drought, at least up to +8°C warming over base as evaluated in previous studies (e.g., Adams et al., 2017), juveniles of two widely distributed species of Pinus (P. edulis and P. ponderosa) die at a linearly increasing rate (Adams et al., 2017). When this relationship is combined with a drought frequency distribution—for which there are many more short droughts than long droughts—the frequency of lethal events for these tree species increases nonlinearly with warming (Adams et al., 2017). Further, heat waves can occur up to subcontinental scales (Ruthrof et al., 2018), which can trigger widespread tree mortality (Matusick et al., 2012, 2013). Some tree species, such as shade-tolerant temperate broad-leaved trees, are more sensitive to thermal stress from heat waves than non-shade-tolerant species and are at greater risk of accelerated forest mortality (Kunert and Hajek, 2022). Consequently, there is substantial concern about the future of forests and their likelihood of transitioning into shorter structural configurations (McDowell et al., 2020) due to extreme climatic events such as hotter drought and heat waves. Heat waves are projected to increase in frequency, intensity, and duration over the next several decades (Meehl and Tebaldi, 2004; IPCC, 2013; Perkins-Kirkpatrick and Gibson, 2017; Guerreiro et al., 2018; Oliver et al., 2018). The spatial extent of land impacted by these events is expected to quadruple by 2040 (Coumou and Robinson, 2013). As heat waves become more frequent and widespread, model projections indicate, with high confidence, increasing frequency of concurrent heat waves and droughts, creating potential compound hazards associated with simultaneous disturbances (e.g., fire, Enright et al., 2015; Stevens-Rumann et al., 2018).
Understanding the influence of environmental stressors such as concurrent heat waves and drought on rates of juvenile survival and mortality is critical to predicting future forest dynamics. Seedling recruitment is particularly important in the face of rapid anthropogenic warming, where range shifts upslope or poleward will be necessary for some species persistence. The survival and mortality of juveniles also influences the structure (Smith et al., 2009) and function (Bansal and Germino, 2010) of forests and their composition (Suarez and Kitzberger, 2008). Thus, forest resilience to global-change induced disturbances, including droughts and heat waves, as well as wildfires, depends on juvenile survival (Davis et al., 2019). Healthy adult individuals from many species can withstand multiple years of drought and heat without suffering mortality (e.g., Adams et al., 2015). Yet, drought under hotter conditions during the juvenile stage hastens mortality (Adams et al., 2017) at what is likely the most sensitive and vulnerable stage. Interactions between ontogeny and drought are expected (Niinemets, 2010), and some projections, based on results of juvenile experiments, have been consistent with those projections for overall tree species mortality (Adams et al., 2017). Moreover, relative responses among juveniles to adverse conditions are similar to those for adult trees (McDowell et al., 2013). Controlled experiments using juveniles offer great promise to rapidly advance understanding of heat wave-induced mortality and aid predictions of future forest dynamics.
Management options are needed to help increase juvenile survival under the harsher conditions of the Anthropocene, both for naturally recruited juveniles as well as planted ones. In addition to traditional forest management practices of selective thinning, contouring, mulching, pest, and wildfire control (Millar et al., 2007; Millar and Stephenson, 2015; Andrews et al., 2020), recent advances in nanoscience and nanotechnology may provide an additional set of useful tools for forest managers to reduce the risk of tree mortality during extreme drought and heat waves (Field et al., 2020). Nanochitosan is a biopolymer plant elicitor derived by the deacetylation of chitin—a commercially produced byproduct acquired by upcycling waste from the seafood industry. Unlike chitosan, which has been used for decades in agricultural and horticultural applications (e.g., Sharp, 2013; Sharif et al., 2018), nanochitosan has unique physicochemical properties and is highly water soluble, making it more biologically reactive and effective than chitosan (e.g., Sabbour and Solieman, 2016; Li et al., 2019) and more sustainable. Nanochitosan enhances the organic carbon of the soil and stabilizes the micro and macro aggregates (Aminiyan et al., 2015). A growing number of studies, particularly in the agricultural literature, have demonstrated the effectiveness of nanochitosan applications to soil for significantly improving plant responses including growth and yield, drought tolerance, establishment, resistance to diseases and pathogens, and production of secondary metabolites to help mitigate stressors including temperature extremes and phytophagous insect attacks such as bark beetles (e.g., Sharp, 2013; Katiyar et al., 2015; Pichyangkura and Chadchawan, 2015; Zayed et al., 2017; Behboudi et al., 2018; Kumaraswamy et al., 2018; Alaghmand et al., 2020).
Our primary focus was on testing whether or not a nanochitosan soil amendment would have a protective effect overall reducing both drought- and heat-related juvenile mortality. This study evaluates the combined effects of drought and heat on mortality of tree juveniles and does not focus on drought or heat in isolation, as these stressors often occur in tandem throughout many dryland forests. In this context, we also evaluated whether or not hotter drought (i.e., warmer mean temperature and dry conditions) combined with a heat wave synergistically affects tree juvenile mortality, as suggested by adult tree mortality under field conditions (Matusick et al., 2018; Lawal et al., 2024). For this, we tested the previously hypothesized relationship that tree juvenile mortality increased progressively from ambient base temperature, to ambient temperature with a heat wave, to hotter base temperature, and hotter temperature with a heat wave (Breshears et al., 2021). We focused on the piñon pine, Pinus edulis, one of the most intensively studied species regarding drought- and heat-related tree mortality (Breshears et al., 2018) and widespread across the dryland western USA. One approach for studying drought and heat wave effects has been to focus on short-term physiological responses that may include mortality of sensitive individuals but does not follow plants through protracted drought to quantify population-level mortality. Here we focus on population-level mortality of tree juveniles under controlled climate and growth media conditions rather than on intermediate physiological measures; this precludes us inferring direct mechanistic responses but is germane to assessing population-level mortality that is relevant to recruitment dynamics.
Methods
Experimental design and measurements
We conducted a multifactorial growth chamber experiment to investigate the impacts of temperature, heat waves, and nanochitosan soil amendment treatments on survival of potted P. edulis juveniles (2–3 years old) during drought. The plants were repotted into 7.5 × 7.5 × 30 cm pots. Soil mixture was prepared to approximate an intermediate soil texture using a sieved sandy loam soil with less than 2 mm gravel size (Acme Sand and Gravel, Tucson, AZ, United States). The mineral soil was amended with 30% fine sand and 25% sphagnum peat moss based potting soil (Sunshine Mix #4, Sungro Horticulture, Agawam, MA, United States). To simulate a drought, juveniles were given a one-time watering at the beginning of the study period and did not receive additional water throughout the rest of the study period. The experiment included two base temperature treatments: ‘Ambient’ (ambient temperatures), and ‘Hotter’ (+4° C temperatures). Ambient diurnal temperatures were set to mimic ambient summer (June) conditions comparable to a relatively low elevation P. edulis ecotone (near White Rock, New Mexico, USA), with mean minimum and mean maximum diurnal temperature of ~15° C and ~ 30° C, respectively (30-year mean from 1991 to 2020, PRISM Climate Group, Oregon State University1, data created and accessed April 2018). A 12-h photoperiod was maintained in the growth chambers with ambient CO2 concentrations. Hotter treatments followed the same diurnal temperature cycle but with elevated temperatures. The temperature treatments were further subdivided into juveniles experiencing a 10-day heat wave (‘HW’) and those without a heat wave (‘No HW’). The heat wave was +8° C warmer than the base temperature (‘Ambient’ or ‘Hotter’). Finally, each of the four base temperature by heat wave treatments were further split into juveniles whose soil was treated with nanochitosan (‘Nano’) and those that were not (‘No Nano’). We implemented this design in four growth chambers: an ambient temperature chamber, a ~ 4° C ‘Hotter’ than ambient chamber, an ambient plus a 10-day heat wave (~8° C warmer than ambient) chamber, and a ‘Hotter’ plus 10-day heat wave (~12° C warmer than ambient) chamber.
Following 3 months of acclimation (Supplemental Material S1), 96 juveniles were randomly divided (April 30, 2018) among the growth chambers assigned to one of four base temperature by heatwave treatments (n = 24 each for Ambient, Ambient + HW, Hotter and Hotter + HW), split further into a nanochitosan treatment within each temperature treatment (‘No Nano’ n = 12; ‘Nano’ n = 12). Larger sample sizes were precluded by growth chamber space limitations; also, ideally it would be best to have replicate chambers for each treatment. Juveniles in nanochitosan treatments received a soil surface drenching using approximately 50 mL of nanochitosan solution (4% by weight) twice before starting the experiment and were not given water once the experiment started.
Soil water loss was measured via gravimetric analysis on a weekly time schedule. A soil water release curve (Supplementary Figure S1) was created using a WP4 dewpoint water potential meter (METER Group, Inc. Pullman, Washington, USA). The 10-day heat wave period was started in the heat wave chambers when plant available soil water reached approximately 50% (estimated based on gravimetric soil water content), such that water was becoming limiting when the soil water potential was roughly −1.0 MPa. Juveniles were visually monitored for percent brown foliage (assessed at days since start = 9, 33, 40, 47, 68, 86, 110, 121, 135, 147, 160, 172, 190, 213) and were declared dead when foliage reached 90% brown, consistent with prior studies (Adams et al., 2009, 2017; Law et al., 2019).
Analyses
The study aimed to assess the impact of warming, heat waves, and treatment with nanochitosan on time to mortality. One approach to assess mortality is through aggregated mortality rates, calculated as the number of deaths divided by number of juveniles in each treatment. These data can be analyzed using contingency tables or logistic regression (Harrell, 2001) with fixed effects for randomized treatment factors and other seedling characteristics that might put some seedlings at greater risk of mortality. However, if mortality rates are high and follow-up is long enough, most trees will die in all treatments, resulting in small differences between treatments. An alternative approach to assess mortality is through individual survival times, which allow for analysis and visualization of the course of mortality over the study period.
Two methods were used to analyze individual survival times: Kaplan–Meier estimation of survival functions and Proportional Hazards Cox model regression (Cox, 1972; Cox and Oakes, 1984). Kaplan–Meier analyses were used to evaluate the progression of mortality over the study period, and the Cox proportional hazard regression modeled the hypothesized ordinal effects of chronic warming and heat wave treatments, as well as effects of the nanochitosan soil amendment. The study followed all seedlings up to 213 days and determined survival times, which were defined as the length of time from the start of the study until death or the end of the study period. Survival times that were only partially observed, when a seedling was still alive at the end of the study, were labeled as censored survival times, while known survival times were labeled as event times. In standard survival analysis (Landes et al., 2020), “censored” means that the event of interest (in this case, mortality) has not occurred for that individual at the time of the last observation.
Kaplan–Meier estimation of survival functions
To evaluate the effect of nanochitosan application, we compared it across the four temperature treatments: Ambient, Ambient + HW, Hotter, and Hotter + HW. Kaplan–Meier analyses (Kleinbaum and Klein, 2012) were used to estimate survival functions that show the timing of mortality over the study. The time (in days) from when juveniles were first placed in their treatments until declared dead was used in the estimation of survival functions. Juveniles that did not die had a survival time set to the last observation date and were treated as censored in the analysis. Log-rank tests were used to test for differences in survival distributions among the treatment groups. When more than two survival curves were compared, we also report the Dunnett-Hsu adjusted p-values. We chose not to display 95% confidence intervals for survival curves, because with our sample size they were very wide, which made graphs difficult to interpret.
Proportional hazards Cox model regression
The Cox model allows us to estimate the effects of our individual factors (Nano, Hotter, HW) and their interactions; as well as test our hypothesized ordinal ranking of the relative risk of mortality. Base warming (Hotter), HW, and the Nano treatment were modeled as single factors and in multivariable models using Cox proportional hazards regression (Cox, 1972; SAS v9,4), in a similar manner to using ANOVA or regression for a continuous dependent variable. The Cox models estimate the log(hazard) rate, where the hazard rate represents the risk of mortality given a juvenile has survived up to a specific time. Regression coefficients from the Cox models estimate the effect of a one unit change in the covariate relative to an arbitrary baseline hazard function. The hazard ratios, which are exponentiated coefficients, estimated the effect size of covariates relative to baseline (e.g., heat wave vs. no heat wave; Cox and Oakes, 1984). We also present 95% confidence intervals for hazard ratios to represent their uncertainty.
Hazard ratios greater than 1 indicate factors that increase the risk of mortality; those less than 1 indicate factors that reduce the risk of mortality. We created an ordinal variable to test our a priori ranking for relative risk: Ambient <Ambient + HW < Hotter < Hotter + HW (Breshears et al., 2021). This ordinal variable was coded as Ambient = 0, Ambient + HW = 1, Hotter = 2 and Hotter + HW = 3 and entered into models as a continuous covariate.
We fitted three models to test effects of experimental treatments on the relative risk of mortality: a four-category Hotter & HW model, a multivariable with Hotter and HW + Nano as categorical factors (Model A) and a multivariable model with Hotter and HW as an ordinal covariate + Nano (Model B). Cox proportional hazards models assume that hazards for different treatments have the same proportionality over the duration of the study. We tested this assumption using the approach of adding time interactions with treatment factors. We used the AIC (Akaike information criterion; Akaike, 1974) to compare strength of evidence for models.
Results
Mortality progression and Kaplan–Meier estimates of survival functions
Plant available soil water content reached ~50% by day 23, which initiated the start of the 10-day HW treatment. The first mortalities were recorded on day 40, which was 7 days after the heat wave ended. Experimental conditions simulating hotter drought and heat waves led to more extreme mortality, and 100% of juveniles without Nano in all temperature by heatwave treatments, and those in Hotter or HW treatments, died by day 213 (Supplementary Table S1). When juveniles were treated with nanochitosan, mortality rates were 92% for Ambient + HW, 92% for Hotter and 100% for Hotter + HW. Mortality rates for Ambient juveniles were 83% for No Nano and 79% for Nano. Overall, there was no significant difference in the relative frequency of mortality among the eight treatment combinations (Fisher exact test, p = 0.130).
Mortality rate for all experimental conditions was highest between days 40 and 47 (large steps, Figure 1), followed by a more gradual decline in mortality. Log-rank tests for overall main effect comparisons (Figure 1) show a small effect for Nano (p = 0.045). We next assessed Nano effects stratified by the four Hotter × HW combinations (Figure 2). Survival curves showed the biggest distinction between Nano and No Nano for juveniles in the Hotter treatment, but with only n = 12 per group, the curves were not significantly different (p = 0.078). Survival curves for the one-way combination of Hotter × HW treatments were not significantly different (Supplementary Figure S2, p = 0.279). The greatest separation between Nano and No-Nano survival curves was for Hotter + HW compared to Ambient (Supplementary Figure S2, p = 0.060, Dunnett-Hsu adjusted p = 0.143). In each comparison, the condition with less overall temperature stress (assumed a priori to be Ambient <Ambient + HW < Hotter < Hotter + HW; Breshears et al., 2021) had lower mortality compared to the condition with more overall temperature stress, as shown in the survival curves where lower overall temperature stress cases lie above conditions with more overall temperature stress. We did not detect overall effects for Hotter/Ambient (p = 0.200) and HW/No HW (p = 0.150, Supplementary Figure S3).
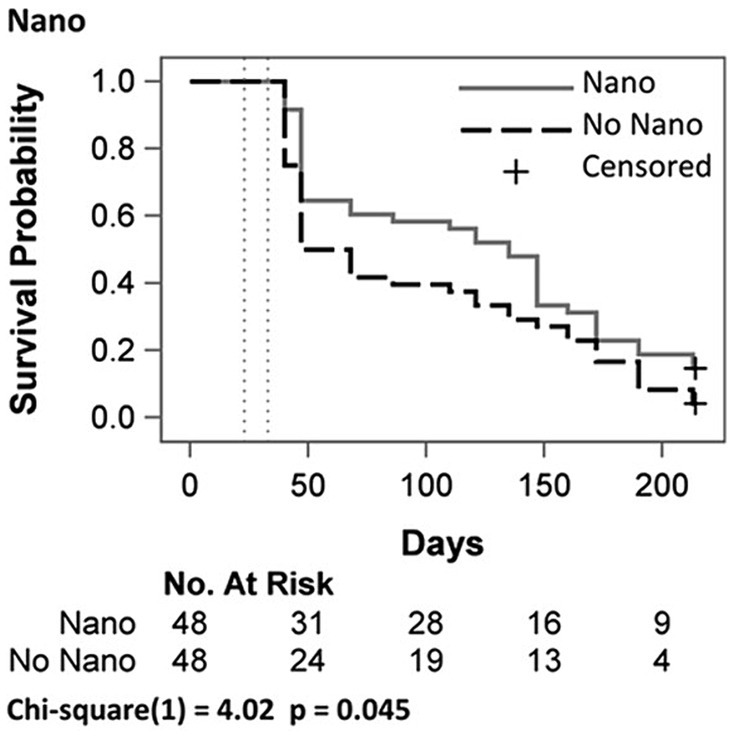
Figure 1. Survival probability curves of Pinus edulis juveniles by Nanochitosan (Nano) treatment pooling over all Hotter and Heat Wave (Hotter & HW) treatments combined. Vertical dashed lines indicate periods with experimental heat waves. Homogeneity of the curves were tested using log-rank tests; Censored indicates juveniles that survived to the end of the study.
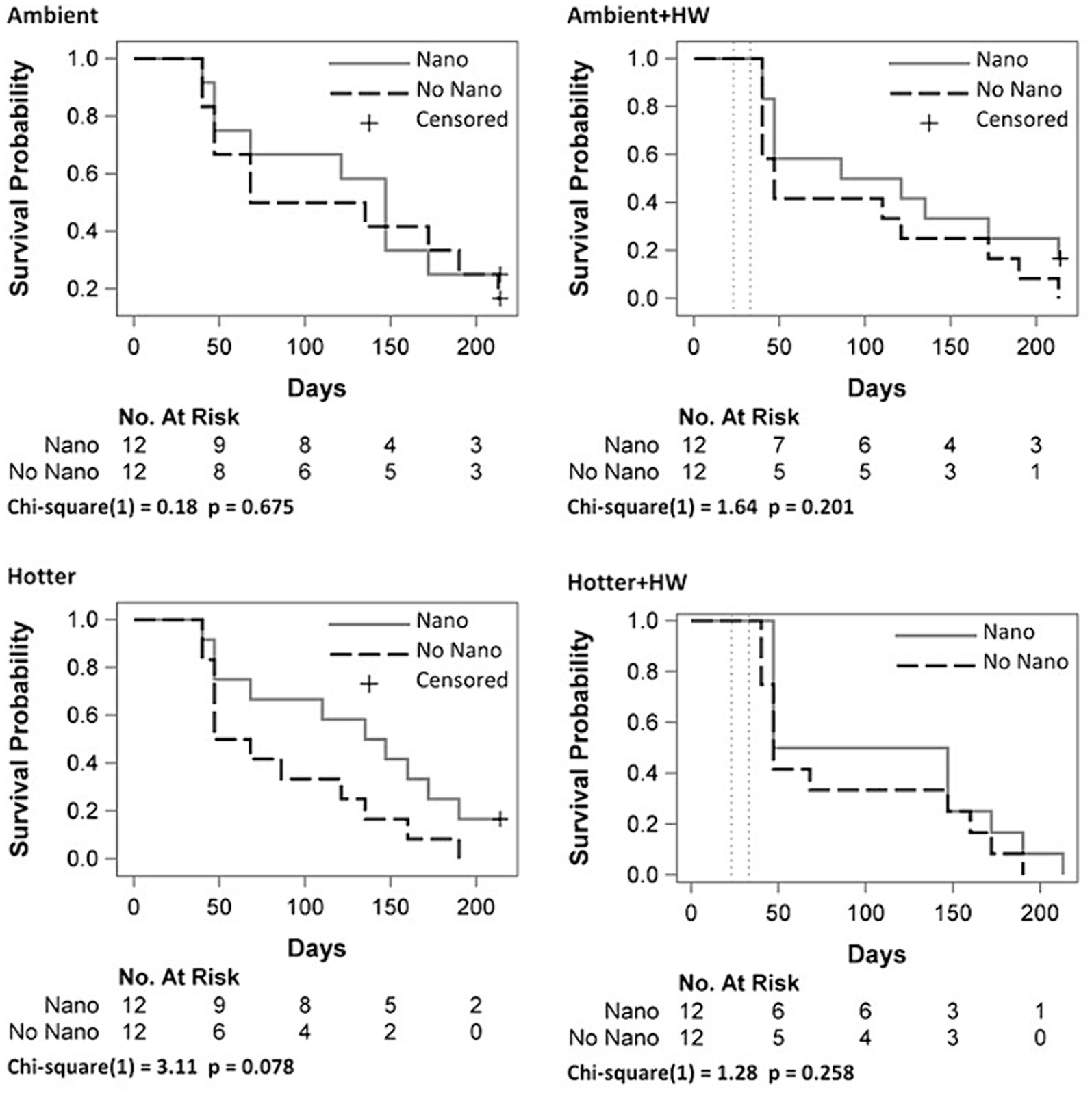
Figure 2. Survival probability curves of Pinus edulis juveniles by Nanochitosan (Nano) treatments stratified by the four Hotter and Heat Wave (Hotter & HW) combinations. Vertical dashed lines indicate periods with experimental heat waves. Homogeneity of the curves were tested using log-rank tests.
Proportional hazards Cox model regression
When a heat wave was superimposed on hotter chronic temperatures, there was a significantly increased risk of mortality, as evidenced by the elevated hazard ratio in the unadjusted Cox regression model for Hotter and HW Unadjusted (Table 1; HR = 1.85 95% CI 1.00–3.41, p = 0.049). The model that additionally incorporated Nano vs. No Nano, Model A (Hotter and HW + Nano), also shows a significant effect of Hotter + HW (p = 0.036). This model also shows a 37% reduction in relative risk of mortality with nanochitosan treatment based on its low hazard ratio (0.63 95% CI 0.41–0.96, p = 0.037). Our third model, Model B (Hotter and HWordinal + Nano) included both the nanochitosan treatment, and the four temperature by heatwave treatment combinations as an ordinal variable. Taken together, progression of ordinal overall temperature stress levels (Ambient, Ambient + HW, Hotter, Hotter + HW) was associated with a significant increase in mortality (p = 0.045, HR = 1.21 for each added treatment level), and the nanochitosan treatment had an additional positive effect on survival (p = 0.036). We found no significant evidence of non-proportional hazards.
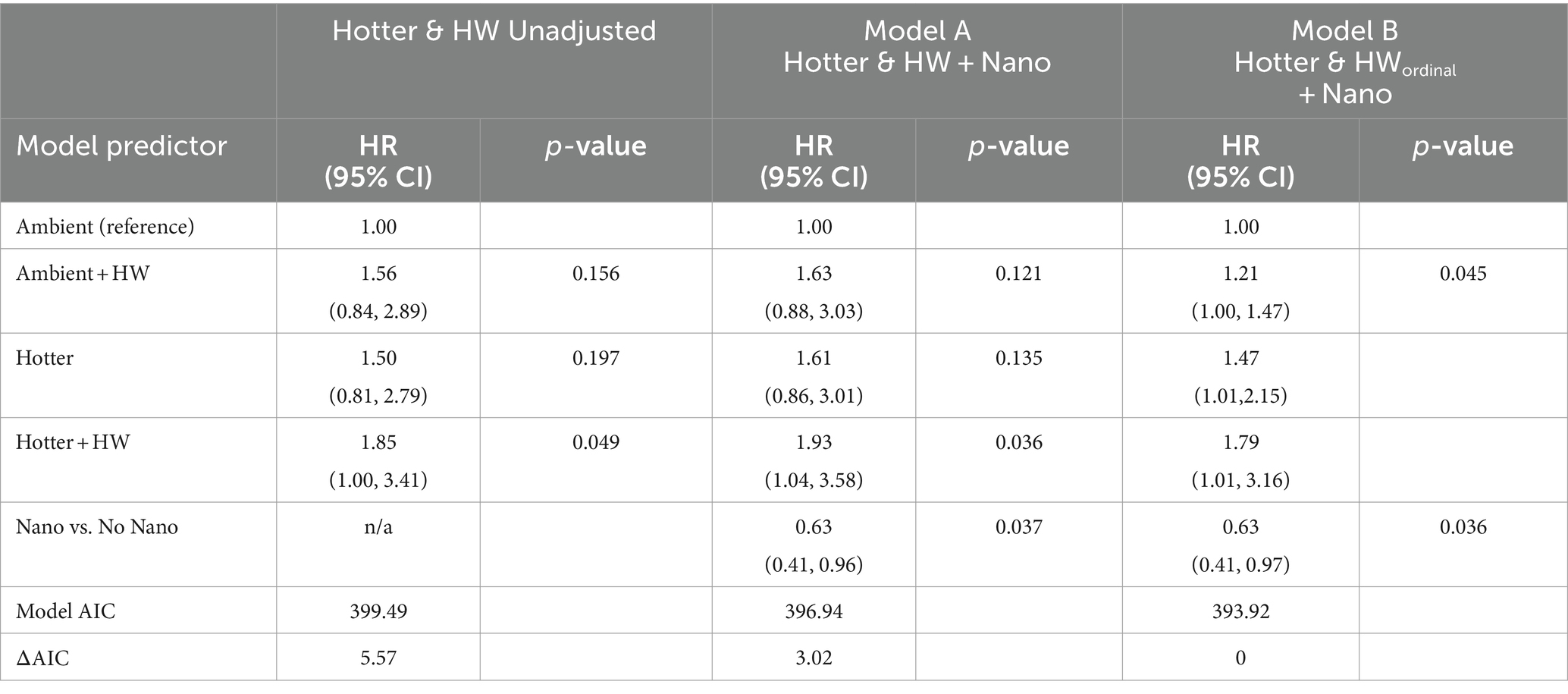
Table 1. Cox model regression results for Hotter, heat wave (HW) and nanochitosan (Nano) effects on juvenile Pinus edulis mortality: a four-category Hotter and Heat Wave (Hotter & HW) model, a multivariable with Hotter & HW + Nano as categorical factors (Model A) and a multivariable model with Hotter & HW as an ordinal covariate + Nano (Model B).
Discussion
Overall, our results suggest that the addition of a soil amendment nanochitosan can aid in increasing juvenile survival under extreme heat and drought. In addition, our results highlight that warming and heat waves accelerated juvenile P. edulis mortality during protracted drought.
Nanochitosan had a significant protective effect (Table 1) in delaying juvenile mortality under heat waves and hotter drought, reflected in the reduced hazard ratio (Table 1; although nanochitosan effect was not detectable in the survival curves (Supplementary Figure S2), likely due to small sample size). Thus, nanochitosan addition may provide a new potential management treatment for juvenile tree survival in the climate of the Anthropocene. This builds on other research showing protective effects of nanochitosan under other conditions. For example, nanochitosan, applied as a soil amendment or a foliar application, has been shown to increase drought and heat tolerance by enhancing natural defense responses such as increased cell wall synthesis, generation of reactive oxygen species, and accumulation of phenolic compounds (Katiyar et al., 2015; Kumaraswamy et al., 2018), which have been shown as effective allelochemicals against bark beetle-associated fungi and other pathogens (Nagy et al., 2000; Klepzig and WaIkinshaw, 2003). Nanochitosan has been shown to increase plant nutrient extraction from soil (Ashraf et al., 2022); however, we added fertilizer to all treatments prior to starting the experiment to ensure nutrient limitation was not a factor. Our treatment was a soil amendment only, so we do not attribute any of the responses to foliar effects of nanochitosan.
In addition, our results highlight that warming and heat waves accelerated juvenile mortality during protracted drought. These results build on prior research showing that heat waves in combination with moderate drought can increase tree mortality in Pinus (Birami et al., 2018), and in other genera (Marchin et al., 2022), as well as other research showing hotter drought accelerates tree mortality in Pinus (Adams et al., 2017). Our results also demonstrate that warming and HW both increase mortality (HR = 1.6 for each), but the combined Hotter + HW effect (HR = 1.93) was less than the expected combination of individual Hotter and HW effects (1.63*1.61 = 2.62). With a synergistic HW effect we would expect the Hotter + HW effect to be greater than 2.62, suggesting that there is not any evidence for a synergistic HW effect. Despite small sample sizes (n = 12), which limited our statistical power to detect differences, these results highlight the negative effects of combined hotter drought and heat waves on juvenile tree mortality, consistent with observed adult tree mortality occurring in response to these two factors (Matusick et al., 2018). Although additional work is needed to evaluate functional traits associated with intermediate physiological responses prior to mortality, such as conducted on Eucalyptus juveniles (Drake et al., 2018; Aspinwall et al., 2019) and on P. halepensis (Birami et al., 2018), our results nonetheless provide complimentary insights on the consequences of hotter drought combined with heat waves on tree juvenile mortality. Future work needs to further evaluate the relative influences of different magnitudes and durations of heatwaves in conjunction with different levels of baseline warming (Breshears et al., 2021). The future survival trajectories of forests following extreme events of hotter drought combined with heat waves depend on successful juvenile recruitment, which will be altered by the extreme events.
Our results provide a first step in developing a potential soil amendment treatment to mitigate the effects of hotter drought and heat waves on dryland juvenile trees. Future additional tests are needed to further assess protective effects under field conditions for both naturally recruited and planted juveniles, as well as tests of the amount, timing, and repetition of the application. Planting of juveniles (“outplanting”) is a common forestry practice following wildfire (Stevens et al., 2021) and could also be applied following large scale tree die-off events, as managers deal with rapid disturbance-induced ecosystem changes. The juveniles we used are typical size for those used in outplanting. Nanochitosan could be readily applied to such juveniles to increase their chances of surviving drought and accompanying warmer baseline temperatures, along with potential heat waves. Nanochitosan can be applied directly to the plant foliage or to the soil as a broadcast application for forest stands. It can also be utilized as a soil drench treatment at the base of individuals, from seedlings to adults. The application of nanaochitosan could potentially provide other beneficial effects to trees in addition to enhancing drought and heat tolerance, such as enhancing seedling establishment, nutrient use efficiency, resin production, and resistance to diseases and pathogens (Sharp, 2013; Katiyar et al., 2015; Kumaraswamy et al., 2018). Nanochitosan is commercially available with an approximate application cost per hectare comparable to the cost of standard fertilizer applications (Li et al., 2019).
As climate conditions become more stressful to plants, including the potential for multi-year to multi-decadal “megadroughts,” management actions will need to become more intensive and focused finer spatial scales (Field et al., 2020). The potential utility of nanochitosan proposed previously (Field et al., 2020) is supported by this study. Nanochitosan is produced by upcycling marine waste byproducts that contain chitin, a biopolymer commonly found in the shells of crustaceans. The application of nanochitosan not only helps in waste management and environmental sustainability but also serves as a potential tool for forest managers to reduce the risk of tree mortality during extreme heat and drought. Although the scope of our experimental design was not broad enough in scale or sample size to assess the expected range of reduction and delay of mortality in field conditions, our results nonetheless provide encouraging insights suggesting that significant reductions in mortality can be achieved with only one application of nanochitosan. Our study focuses on mortality of tree juveniles and supports the need for additional studies to evaluate intermediate physiological responses that could provide insights into the mechanisms behind mortality and enhance our understanding of how nanochitosan influences tree responses. Further research is needed to help provide useful data to practitioners to assess the cost/benefit of using nanochitosan as a potential forest management tool. In conclusion, our results build on prior results highlighting risk from hotter drought to illustrate synergies with heat wave extremes. In a management context, we show protective effects of nanochitosan soil amendment in delaying tree mortality under heat waves and hotter drought, thereby providing a new potential management treatment for juvenile trees in the climate of the Anthropocene.
Data availability statement
The raw data supporting the conclusions of this article will be made available by the authors, without undue reservation.
Author contributions
JPF, DL, and DB designed the experiment. JPF, DL, and KA conducted the experiment. OM and MB lead analyses. JPF, DL, OM, MB, and DB lead the drafting and revisioning of the manuscript. XF, JBF, KR, and JV provided design input, related feedback, and editing of the manuscript. All authors contributed to the article and approved the submitted version.
Funding
This study was supported by NSF EF-1340624 and USDA NIFA McIntire Stennis ARZT-1390130-M12-222; USGS Southwest Climate Adaptation Science Center G23AC00675; preliminary work related to this study was supported by University of Arizona and UNAM collaborative funding under CAZMEX to JPF, DL, and DB. DB was also supported by a Sir Walter Murdoch Distinguished Visiting Scholar award, Murdoch 403 University.
Acknowledgments
We thank the following colleagues for related comments and collaboration: Greg A. Barron-Gafford, Joseph R. Burger, Alberto Burquez, Enriquena Bustamante, Angelina Martinez-Yrizar, David J. P. Moore, Scott R. Saleska, Scott C. Stark, and Abigail L. S. Swann.
Conflict of interest
The authors declare that the research was conducted in the absence of any commercial or financial relationships that could be construed as a potential conflict of interest.
Publisher’s note
All claims expressed in this article are solely those of the authors and do not necessarily represent those of their affiliated organizations, or those of the publisher, the editors and the reviewers. Any product that may be evaluated in this article, or claim that may be made by its manufacturer, is not guaranteed or endorsed by the publisher.
Supplementary material
The Supplementary material for this article can be found online at: https://www.frontiersin.org/articles/10.3389/ffgc.2024.1215051/full#supplementary-material
Footnotes
References
Adams, H. D., Barron-Gafford, G. A., Minor, R. L., Gardea, A. A., Bentley, L. P., Law, D. J., et al. (2017). Temperature response surfaces for mortality risk of tree species with future drought. Environ. Res. Lett. 12:115014. doi: 10.1088/1748-9326/aa93be
Adams, H. D., Collins, A. D., Briggs, S. P., Vennetier, M., Dickman, L. T., Sevanto, S. A., et al. (2015). Experimental drought and heat can delay phenological development and reduce foliar and shoot growth in semiarid trees. Glob. Chang. Biol. 21, 4210–4220. doi: 10.1111/gcb.13030
Adams, H. D., Guardiola-Claramonte, M., Barron-Gafford, G. A., Villegas, J. C., Breshears, D. D., Zou, C. B., et al. (2009). Temperature sensitivity of drought-induced tree mortality portends increased regional die-off under global-change-type drought. Proc. Natl. Acad. Sci. U. S. A. 106, 7063–7066. doi: 10.1073/pnas.0901438106
Akaike, H. (1974). A new look at the statistical model identification. IEEE Trans. Autom. Control 19, 716–723. doi: 10.1109/TAC.1974.1100705
Alaghmand, A., Khaghani, S., Bihamta, M. R., Gomarian, M., and Ghorbanpour, M. (2020). Effect of chitosan and nano-chitosan on agronomic properties and omega-3, 6 and 9 fatty acids in some cultivars of Nigella sativa L. under drought stress conditions. Eco-phytochem. J. Med. Plant. 7, 83–96. doi: 10.32474/sjfn.2020.02.000141
Allen, C. D., Macalady, A. K., Chenchouni, H., Bachelet, D., McDowell, N., Vennetier, M., et al. (2010). A global overview of drought and heat- induced tree mortality reveals emerging climate change risks for forests. For. Ecol. Manag. 259, 660–684. doi: 10.1016/j.foreco.2009.09.001
Aminiyan, M. M., Sinegani, A. A. S., and Sheklabadi, M. (2015). Aggregation stability and organic carbon fraction in a soil amended with some plant residues, nanozeolite, and natural. Zeolite. Int. J. Recycl. Org. Waste Agric. 4, 11–22. doi: 10.1007/s40093-014-0080-0
Andrews, C. M., D'Amato, A. W., Fraver, S., Palik, B., Battaglia, M. A., and Bradford, J. B. (2020). Low stand density moderates growth declines during hot droughts in semi-arid forests. J. Appl. Ecol. 57, 1089–1102. doi: 10.1111/1365-2664.13615
Ashraf, U., Zafar, S., Ghaffar, R., Sher, A., Mahmood, S., Noreen, Z., et al. (2022). “Impact of nano chitosan-NPK fertilizer on field crops” in Nanomaterial-plant interactions. eds. S. Kumar and S. V. Madihally (Cambridge, MA, USA: Academic Press, Imprint of Elsevier), 165–183.
Aspinwall, M. J., Pfautsch, S., Tjoelker, M. G., Vårhammar, A., Possell, M., Drake, J. E., et al. (2019). Range size and growth temperature influence Eucalyptus species responses to an experimental heatwave. Glob. Chang. Biol. 25, 1665–1684. doi: 10.1111/gcb.14590
Bansal, S., and Germino, M. J. (2010). Unique responses of respiration, growth, and non-structural carbohydrate storage in sink tissue of conifer seedlings to an elevation gradient at timberline. Environ. Exp. Bot. 69, 313–319. doi: 10.1016/j.envexpbot.2010.05.002
Behboudi, F., Sarvestani, Z. T., Kassaee, M. Z., Sanavi, S. A. M. M., Sorooshzadeh, A., and Ahmadi, S. B. (2018). Evaluation of chitosan nanoparticles effects on yield and yield components of barley (Hordeum vulgare L.) under late season drought stress. J. Water Environ. Nanotechnol. 3, 22–39. doi: 10.22090/jwent.2018.01.003
Birami, B., Gattmann, M., Heyer, A. G., Grote, R., Arneth, A., and Ruehr, N. K. (2018). Heat waves alter carbon allocation and increase mortality of Aleppo pine under dry conditions. Front. For. Glob. Change 27, 1–8. doi: 10.3389/ffgc.2018.00008
Breshears, D. D., Carroll, C. J., Redmond, M. D., Wion, A. P., Allen, C. D., Cobb, N. S., et al. (2018). A dirty dozen ways to die: metrics and modifiers of mortality driven by drought and warming for a tree species. Front. For. Glob. Change 1:4. doi: 10.3389/ffgc.2018.00004
Breshears, D. D., Fontaine, J. B., Ruthrof, K. X., Field, J. P., Feng, X., Burger, J. R., et al. (2021). Underappreciated plant vulnerabilities to heat waves. New Phytol. 231, 32–39. doi: 10.1111/nph.17348
Coumou, D., and Robinson, A. (2013). Historic and future increase in the global land area affected by monthly heat extremes. Environ. Res. Lett. 8:34018. doi: 10.1088/1748-9326/8/3/034018
Cox, D. R. (1972). Regression models and life-tables. J. R. Stat. Soc. Series B Stat. Methodol. 34, 187–202. doi: 10.1007/978-1-4612-4380-9_37
Davis, K. T., Dobrowski, S. Z., Higuera, P. E., Holden, Z. A., Veblen, T. T., Rother, M. T., et al. (2019). Wildfires and climate change push low-elevation forests across a critical climate threshold for tree regeneration. Proc. Natl. Acad. Sci. U. S. A. 116, 6193–6198. doi: 10.1073/pnas.1815107116
Dey, D. C., Knapp, B. O., Battaglia, M. A., Deal, R. L., Hart, J. L., O’Hara, K. L., et al. (2019). Barriers to natural regeneration in temperate forests across the USA. New For. 50, 11–40. doi: 10.1007/s11056-018-09694-6
Drake, J. E., Tjoelker, M. G., Vårhammar, A., Medlyn, B. E., Reich, P. B., Leigh, A., et al. (2018). Trees tolerate an extreme heatwave via sustained transpirational cooling and increased leaf thermal tolerance. Glob. Chang. Biol. 24, 2390–2402. doi: 10.1111/gcb.14037
Enright, N. J., Fontaine, J. B., Bowman, D. M., Bradstock, R. A., and Williams, R. J. (2015). Interval squeeze: altered fire regimes and demographic responses interact to threaten woody species persistence as climate changes. Front. Ecol. Environ. 13, 265–272. doi: 10.1890/140231
Field, J. P., Breshears, D. D., Bradford, J. B., Law, D. J., Feng, X., and Allen, C. D. (2020). Forest management under megadrought: urgent needs at finer scale and higher intensity. Front. For. Glob. Change 3:502669. doi: 10.3389/ffgc.2020.502669
Grubb, P. J. (1977). The maintenance of species-richness in plant communities: the importance of the regeneration niche. Biol. Rev. 52, 107–145. doi: 10.1111/j.1469-185X.1977.tb01347.x
Guerreiro, S. B., Dawson, R. J., Kilsby, C., Lewis, E., and Ford, A. (2018). Future heat-waves, droughts and floods in 571 European cities. Environ. Res. Lett. 13:034009. doi: 10.1088/1748-9326/aaaad3
Hammond, W. M., Williams, A. P., Abatzoglou, J. T., Adams, H. D., Klein, T., López, R., et al. (2022). Global field observations of tree die-off reveal hotter-drought fingerprint for Earth’s forests. Nat. Commun. 13:1761. doi: 10.1038/s41467-022-29289-2
Harrell, F. E. (2001). Regression modeling strategies: With applications to linear models, logistic regression, and survival analysis. New York: Springer, 1–474.
Hicke, J. A., Meddens, A. J., and Kolden, C. A. (2016). Recent tree mortality in the western United States from bark beetles and forest fires. For. Sci. 62, 141–153. doi: 10.5849/forsci.15-086
IPCC (2013). “Summary for policymakers” in Climate change 2013: the physical science basis. Contribution of working group I to the fifth assessment report of the intergovernmental panel on climate change. eds. T. F. Stocker, D. Qin, G.-K. Plattner, M. Tignor, S. K. Allen, and J. Boschung, et al. (Cambridge UK and New York, NY: Cambridge University Press).
Katiyar, D., Hemantaranjan, A., and Singh, B. (2015). Chitosan as a promising natural compound to enhance potential physiological responses in plant: a review. Indian J. Plant Physiol. 20, 1–9. doi: 10.1007/s40502-015-0139-6
Kleinbaum, D. G., and Klein, M. (2012). Survival analysis: A self-learning text. 3rd Edn. New York: Springer.
Klepzig, K. D., and WaIkinshaw, C. H. (2003). Cellular response of loblolly pine to wound inoculation with bark beetle-associated fungi and chitosan. Asheville, NC: USDA, Forest Service, Southern Research Station, 9p.
Kolb, P. F., and Robberecht, R. (1996). High temperature and drought stress effects on survival of Pinus ponderosa seedlings. Tree Physiol. 16, 665–672. doi: 10.1093/treephys/16.8.665
Kumaraswamy, R. V., Kumari, S., Choudhary, R. C., Pal, A., Raliya, R., Biswas, P., et al. (2018). Engineered chitosan based nanomaterials: bioactivities, mechanisms and perspectives in plant protection and growth. Int. J. Biol. Macromol. 113, 494–495. doi: 10.1016/j.ijbiomac.2018.02.130
Kunert, N., and Hajek, P. (2022). Shade-tolerant temperate broad-leaved trees are more sensitive to thermal stress than light-demanding species during a moderate heatwave. Trees Forests People 10:100282. doi: 10.1016/j.tfp.2022.100282
Landes, J., Engelhardt, S. C., and Pelletier, F. (2020). An introduction to event history analyses for ecologists. Ecosphere 11:e03238. doi: 10.1002/ecs2.3238
Law, D. J., Adams, H. D., Breshears, D. D., Cobb, N. S., Bradford, J. B., Zou, C. B., et al. (2019). Bioclimatic envelopes for individual demographic events driven by extremes: plant mortality from drought and warming. Int. J. Plant Sci. 180, 53–62. doi: 10.1086/700702
Lawal, S., Costanza, J., Koch, F. H., and Scheller, M. S. (2024). Modeling the impacts of hot drought on forests in Texas. Front. For. Glob. Change. 7, 1280254 doi: 10.3389/ffgc.2024.1280254
Li, R., He, J., Xie, H., Wang, W., Bose, S. K., Sun, Y., et al. (2019). Effects of chitosan nanoparticles on seed germination and seedling growth of wheat (Triticum aestivum L.). Int. J. Biol. Macromol. 126, 91–100. doi: 10.1016/j.ijbiomac.2018.12.118
Marchin, R. M., Backes, D., Ossola, A., Leishman, M. R., Tjoelker, M. G., and Ellsworth, D. S. (2022). Extreme heat increases stomatal conductance and drought-induced mortality risk in vulnerable plant species. Glob. Chang. Biol. 28, 1133–1146. doi: 10.1111/gcb.15976
Matusick, G., Ruthrof, K. X., Brouwers, N. C., Dell, B., and Hardy, G. S. (2013). Sudden forest canopy collapse corresponding with extreme drought and heat in a mediterranean-type eucalypt forest in southwestern Australia. Eur. J. Forest Res. 132, 497–510. doi: 10.1007/s10342-013-0690-5
Matusick, G., Ruthrof, K. X., and Hardy, G. S. (2012). Drought and heat triggers sudden and severe dieback in a dominant Mediterranean-type woodland species. Open J. For. 2, 183–186. doi: 10.4236/ojf.2012.24022
Matusick, G., Ruthrof, K. X., Kala, J., Brouwers, N. C., Breshears, D. D., and Hardy, G. E. (2018). Chronic historical drought legacy exacerbates tree mortality and crown dieback during acute heatwave-compounded drought. Environ. Res. Lett. 13:095002. doi: 10.1088/1748-9326/aad8cb
McDowell, N. G., Allen, C. D., Anderson-Teixeira, K., Aukema, B. H., Bond-Lamberty, B., Chini, L., et al. (2020). Pervasive shifts in forest dynamics in a changing world. Science 368:6494. doi: 10.1126/science.aaz94
McDowell, N. G., Ryan, M. G., Zeppel, M. J., and Tissue, D. T. (2013). Improving our knowledge of drought-induced forest mortality through experiments, observations, and modeling. New Phytol. 200, 289–293. doi: 10.1111/nph.12502
McDowell, N. G., Sapes, G., Pivovaroff, A., Adams, H. D., Allen, C. D., Anderegg, W. R., et al. (2022). Mechanisms of woody-plant mortality under rising drought, CO2 and vapour pressure deficit. Nat. Rev. Earth Environ. 3, 294–308. doi: 10.1038/s43017-022-00272-1
Meehl, G. A., and Tebaldi, C. (2004). More intense, more frequent, and longer lasting heat waves in the 21st century. Science 305, 994–997. doi: 10.1126/science.1098704
Millar, C. I., and Stephenson, N. L. (2015). Temperate forest health in an era of emerging megadisturbance. Science 349, 823–826. doi: 10.1126/science.aaa9933
Millar, C. I., Stephenson, N. L., and Stephens, S. L. (2007). Climate change and forests of the future: managing in the face of uncertainty. Ecol. Appl. 17, 2145–2151. doi: 10.1890/06-1715.1
Nagy, N. E., Franceschi, V. R., Solheim, H., Krekling, T., and Christiansen, E. (2000). Wound-induced traumatic resin duct development in stems of Norway spruce (Pinaceae): anatomy and cytochemical traits. Am. J. Bot. 87, 302–313. doi: 10.2307/2656626
Niinemets, Ü. (2010). Responses of forest trees to single and multiple environmental stresses from seedlings to mature plants: past stress history, stress interactions, tolerance and acclimation. For. Ecol. Manag. 260, 1623–1639. doi: 10.1016/j.foreco.2010.07.054
Oliver, E. C., Donat, M. G., Burrows, M. T., Moore, P. J., Smale, D. A., Alexander, L. V., et al. (2018). Longer and more frequent marine heatwaves over the past century. Nat. Commun. 9:1324. doi: 10.1038/s41467-018-03732-9
Perkins-Kirkpatrick, S. E., and Gibson, P. B. (2017). Changes in regional heatwave characteristics as a function of increasing global temperature. Sci. Rep. 7:12256. doi: 10.1038/s41598-017-12520-2
Pichyangkura, R., and Chadchawan, S. (2015). Biostimulant activity of chitosan in horticulture. Sci. Hortic. 196, 49–65. doi: 10.1016/j.scienta.2015.09.031
Ruthrof, K. X., Breshears, D. D., Fontaine, J. B., Froend, R. H., Matusick, G., Kala, J., et al. (2018). Subcontinental heat wave triggers terrestrial and marine, multi-taxa responses. Sci. Rep. 8:13094. doi: 10.1038/s41598-018-31236-5
Sabbour, M. M., and Solieman, N. Y. (2016). The efficacy effect of using chitosan and nano-chitosan against Tuta absoluta (Lepidoptera: Gelechiidae). J. Chem. Pharm. Res. 8, 548–554. doi: 10.1016/j.biocontrol.2021.104699
Sharif, R., Mujtaba, M., Ur Rahman, M., Shalmani, A., Ahmad, H., Anwar, T., et al. (2018). The multifunctional role of chitosan in horticultural crops; a review. Molecules 23:872. doi: 10.3390/molecules23040872
Sharp, R. G. (2013). A review of the applications of chitin and its derivatives in agriculture to modify plant-microbial interactions and improve crop yields. Agronomy 3, 757–793. doi: 10.3390/agronomy3040757
Smith, G. F., Gittings, T., Wilson, M., French, L., Oxbrough, A., O’Donoghue, S., et al. (2009). Identifying practical indicators of biodiversity for stand-level management of plantation forests. Plant. Forests Biodivers., 67–91. doi: 10.1007/978-90-481-2807-5_4
Stevens, J. T., Haffey, C. M., Coop, J. D., Fornwalt, P. J., Yocom, L., Allen, C. D., et al. (2021). Tamm review: Postfire landscape management in frequent-fire conifer forests of the southwestern United States. For. Ecol. Manag. 502:119678. doi: 10.1016/j.foreco.2021.119678
Stevens-Rumann, C. S., Kemp, K. B., Higuera, P. E., Harvey, B. J., Rother, M. T., Donato, D. C., et al. (2018). Evidence for declining forest resilience to wildfires under climate change. Ecol. Lett. 21, 243–252. doi: 10.1111/ele.12889
Suarez, M. L., and Kitzberger, T. (2008). Recruitment patterns following a severe drought: long-term compositional shifts in Patagonian forests. Can. J. For. Res. 38, 3002–3010. doi: 10.1139/X08-149
Keywords: hotter drought, forest management, nanochitosan, soil amendment, tree mortality, climate change adaptation
Citation: Field JP, Law DJ, Myers OB, Barnes ML, Breshears DD, Acuña KM, Feng X, Fontaine JB, Ruthrof KX and Villegas JC (2024) Soil amendment mitigates mortality from drought and heat waves in dryland tree juveniles. Front. For. Glob. Change. 7:1215051. doi: 10.3389/ffgc.2024.1215051
Edited by:
Barry Alan Gardiner, Institut Européen De La Forêt Cultivée (IEFC), FranceReviewed by:
Giulio Curioni, Forest Research, United KingdomBognounou Fidèle, Canadian Forest Service, Canada
Copyright © 2024 Field, Law, Myers, Barnes, Breshears, Acuña, Feng, Fontaine, Ruthrof and Villegas. This is an open-access article distributed under the terms of the Creative Commons Attribution License (CC BY). The use, distribution or reproduction in other forums is permitted, provided the original author(s) and the copyright owner(s) are credited and that the original publication in this journal is cited, in accordance with accepted academic practice. No use, distribution or reproduction is permitted which does not comply with these terms.
*Correspondence: Jason P. Field, jpfield@arizona.edu