- 1Instituto de Medicina Molecular, Faculdade de Medicina, Universidade de Lisboa, Lisbon, Portugal
- 2Centro de Imunodeficiência Primárias de Lisboa, Lisbon, Portugal
- 3Hospital de Santa Maria, Centro Hospitalar Lisboa Norte, Lisbon, Portugal
- 4Cytokines and Viral Infections, Immunology Infection and Inflammation Department, Institut Cochin, INSERM, U1016, Paris, France
- 5CNRS, UMR8104, Paris, France
- 6Université Paris Descartes, Paris, France
Chronic granulomatous disease (CGD) results from primary defects in phagocytic reactive oxygen species (ROS) production. T-cell evaluation is usually neglected during patients’ follow-up, although T-cell depletion has been reported in CGD through unknown mechanisms. We describe here a 36-year-old patient with X-linked CGD with severe CD4 T-cell depletion <200 CD4 T-cells/μl, providing insights into the mechanisms that underlie T-cell loss in the context of oxidative burst defects. In addition to the typical infections, the patient featured a progressive T-cell loss associated with persistent lymphocyte activation, expansion of interleukin (IL)-17-producing CD4 T-cells, and impaired thymic activity, leading to a reduced replenishment of the T-cell pool. A relative CD4 depletion was also found at the gut mucosal level, although no bias to IL-17-production was documented. This immunological pattern of exhaustion of immune resources favors prompt, potentially curative, therapeutic interventions in CGD patients, namely, stem-cell transplantation or gene therapy. Moreover, this clinical case raises new research questions on the interplay of ROS production and T-cell homeostasis and immune senescence.
Introduction
Chronic granulomatous disease (CGD) is the most common primary immunodeficiency affecting phagocytes. It is due to genetic defects in nicotinamide adenine dinucleotide phosphate (NADPH) oxidase (1, 2), leading to impaired reactive oxygen species (ROS) production by monocytes and neutrophils, defective microorganism clearance, and chronic inflammation (1–4). Reduced T-cell numbers were reported in an American CGD cohort, although the underlying mechanisms remain unclear (5). The defective ROS production by myeloid cells indirectly contributes to the T-cell loss through the promotion of inflammation (1, 2). On the other hand, T-cells have been shown to harbor NADPH (6), and T-cell intrinsic defects in ROS production are associated with disturbances in T-cell effector differentiation and regulatory function (6–11). Notably, CGD patients feature expansion of the interleukin (IL)-17-producing T helper cell subset, which is thought to contribute to their heightened immune activation state (12). T-cell alterations in susceptibility to apoptosis have also been described (13), as well as impairments in autophagy (14), which may also impact on T-cell development and function.
We report here long-lasting severe CD4 lymphopenia in a 36-year-old patient with CGD that allowed us to investigate the pathways involved in T-cell production and peripheral homeostasis in the context of markedly impaired ROS production. Our findings are consistent with an overall scenario of immunological exhaustion and are of foremost clinical relevance in the context of the increased life expectancy of CGD patients, as well as to the ongoing debate regarding aggressive early therapeutic intervention (15–17).
Case Report
X-linked CGD was only diagnosed at the age of 13 (G897A mutation at the extreme end of exon 8 of the CYBB gene), although the patient had a clinical history typical of this disorder, namely, neonatal Staphylococcus aureus sepsis, Salmonella enteritidis sepsis (at 7 years of age), multiple liver abscesses due to S. aureus requiring partial hepatectomy (at 10 years of age), and recurrent suppurative lymphadenitis (axillary and cervical), as well as subcutaneous abscesses. ROS production was undetectable using a flow cytometry-based oxidative burst assay (<1% positive monocytes or neutrophils; BD Biosciences). Prophylactic antimicrobials were started with an effective control of major infections, except Pseudomonas aeruginosa keratoconjunctivitis (at age 16).
The patient featured a progressive loss of T-cells with sustained CD4 T-cell counts <200 cells/μl (Figure 1A) and an inverse CD4/CD8 ratio (varying between 0.6 and 0.09).
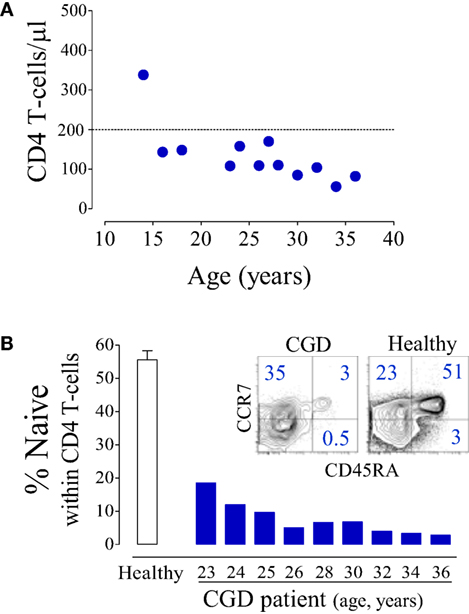
Figure 1. Major CD4 T-cell depletion in a chronic granulomatous disease (CGD) patient. (A) Longitudinal CD4 T-cell counts. (B) Proportion of naïve (CD45RA+CD27+) CD4 T-cells in the CGD patient; open bar represents mean ± SEM of healthy subjects (n = 25), and dot plots illustrate CCR7/CD45RA expression within CD4 T-cells.
In parallel with the T-cell depletion, the phenotypic analysis revealed marked loss of naïve cells within both CD4 (Figure 1B) and CD8 T-cells (6.2% of total CD8 T-cells, 100 cells/μl at 36 years of age). This was in agreement with an impaired replenishment of the T-cell pool by recently produced cells. Indeed, we found evidence of compromised thymopoiesis via the quantification of by-products of T-cell receptor (TCR) rearrangement that are generated during thymic T-cell development [signal joint (sj) and DβJβ TCR rearrangement circles, T-cell receptor rearrangement excision circle (TREC)] and progressively decline during age-associated thymus involution. Both sjTREC frequency and the sj/βTREC ratio, which are considered to reflect intra-thymic precursor T-cell proliferation and directly correlate with thymic output (18, 19), were markedly low for the patient’s age (Figure 2A). Of note, reduced thymic activity was observed despite the levels of IL-7, an essential homeostatic cytokine, being highly enhanced (Figure 2B), even in comparison with those of untreated HIV-1-infected individuals. Thus, impaired thymopoiesis seemed to significantly contribute to naïve T-cell loss. The risks inherent to the reduced thymic activity in conjunction with the patient’s age contributed to the decision to not undergo hematopoietic stem-cell transplantation (HSCT).
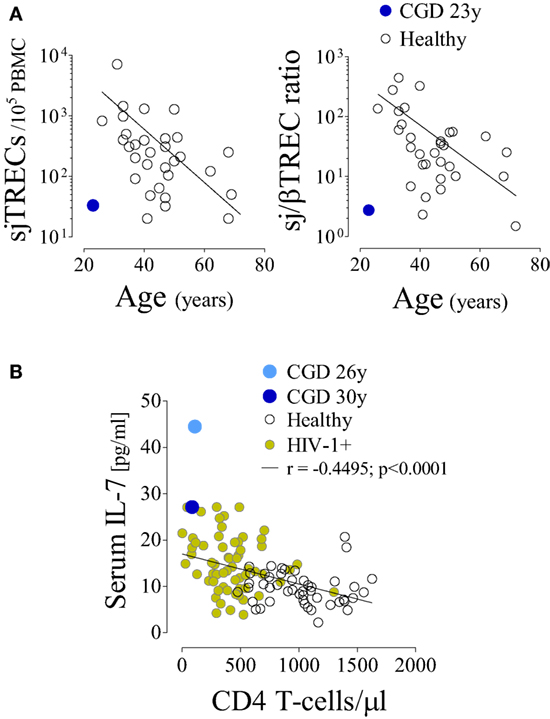
Figure 2. Impaired T-cell production despite increased circulating interleukin (IL)-7 levels in a chronic granulomatous disease (CGD) patient. (A) Signal joint T-cell receptor rearrangement excision circle (sjTREC) frequency and sj/βTREC ratio in total T-cells in relation to age in the CGD patient and healthy subjects. (B) IL-7 levels and CD4 counts in the CGD patient (blue), healthy subjects (black, n = 47), and untreated HIV-1-infected individuals (green, n = 61); each dot represents one individual/time point. P-value and Spearman’s correlation coefficient are shown.
Naïve CD4 T-cell loss was accompanied by upregulation of activation markers and by increased frequency of cycling cells (Figure 3A). The analysis of cytokine production by T-cells revealed an effector differentiation profile with a significant production of pro-inflammatory cytokines, particularly IL-17 (Figure 3B), as has been previously reported (12, 20). Of note, the majority of IL-17-producing T-cells were CD4+ (96%). There was also an increased frequency of CD4 T-cells producing IL-22 (Figure 3B), of which 42% concomitantly produced IL-17. Notably, there was a parallel overrepresentation of regulatory T-cells (Treg) expressing high levels of CD25 and CD39, markers associated with suppressive function (Figures 3A,B). CD8 T-cells featured an activated and terminally differentiated phenotype, as illustrated by their high levels of perforin and IFN-γ production (Figure 3C). In agreement with these findings, both CD4 and CD8 T-cells, irrespective of their degree of differentiation, featured markedly reduced telomere length, further supporting persistent immune stimulation and increased cell cycling in parallel with reduced de novo T-cell replenishment (Figure 3D).
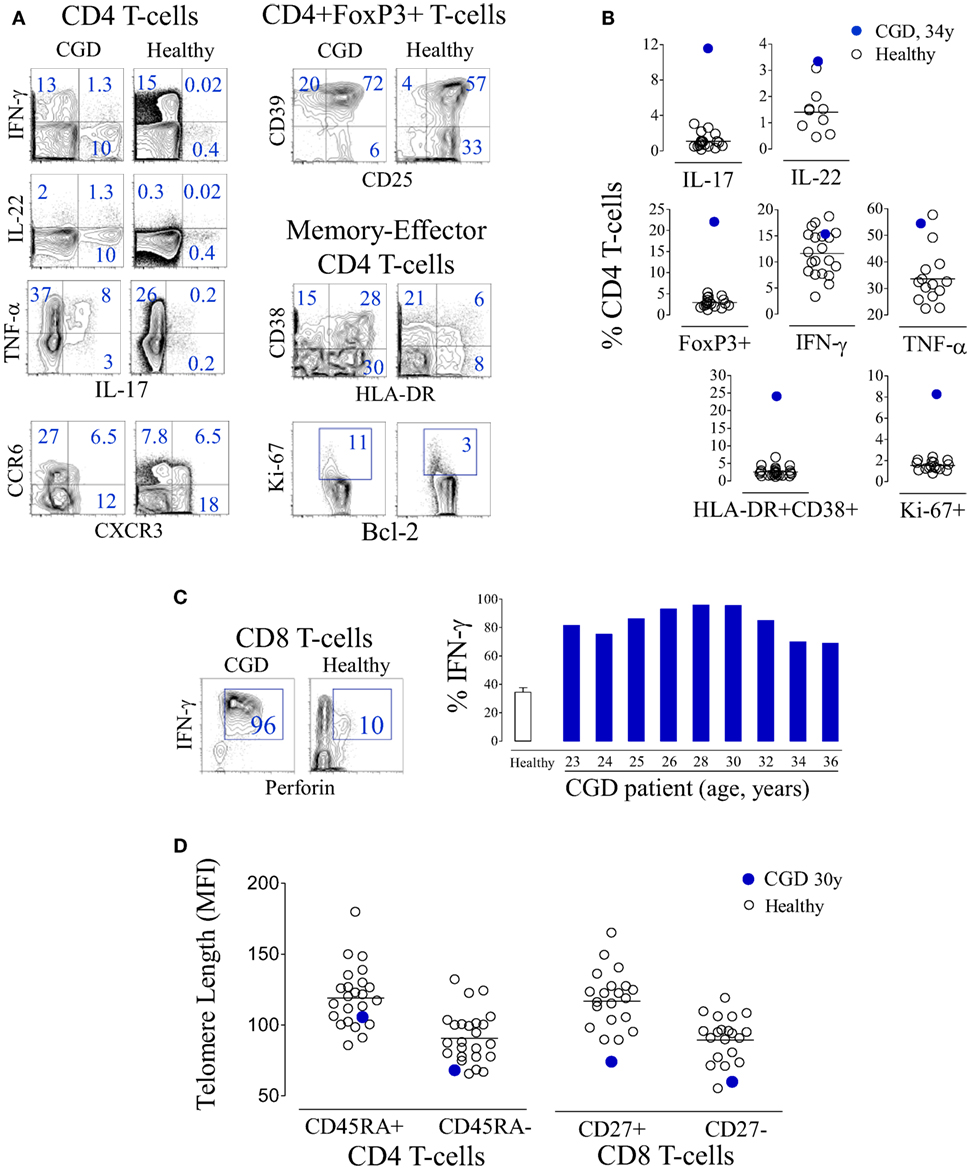
Figure 3. T-cell activation and terminal differentiation in a chronic granulomatous disease (CGD) patient. (A) Representative dot plots of the analysis of cytokine production and chemokine expression within CD4 T-cells, regulatory T-cell-associated markers within CD4+FoxP3+ cells, as well as activation and cycling markers within memory-effector CD4 T-cells from the CGD patient at 36 years of age and a representative age-matched healthy control. (B) Frequency of interleukin (IL)-17-, IL-22-, IFN-γ-, or TNF-α-producing cells, as well as the frequency of FoxP3+, HLA-DR+CD38+, or Ki-67+ cells within the CD4 subset, in the peripheral blood of the CGD patient and healthy subjects. (C) Representative dot plots showing the co-expression of IFN-γ and perforin within CD8 T-cells in the CGD patient and an age-matched control, and a graph showing the frequency of IFN-γ-producing cells within CD8 T-cells of the CGD patient at different ages and of healthy adults (bar represents mean ± SEM, n = 24). (D) Telomere length of naïve (CD45RA+) and memory (CD45RA−) CD4 T-cells as well as CD8 T-cells defined according to CD27 expression from the CGD patient and controls.
At 34 years of age, colonoscopy was performed due to an episode of prolonged diarrhea, with watery stool four to five times a day without mucus or blood, with no apparent microbial cause, accompanied by hypoalbuminemia without other evidence of exudative enteropathy or significant malabsorption. The gastrointestinal symptoms subsided spontaneously after 4 weeks. The mucosa was macroscopically normal with an overall preserved structure, with areas of mild inflammatory infiltrates with lymphoid aggregates in the gut biopsies. Cell suspensions from sigmoid biopsies were analyzed by flow cytometry, and patient data were compared with those of healthy subjects submitted to routine colon cancer screening colonoscopy. The CGD patient featured a decreased frequency of CD4+ cells within total T-lymphocytes in the sigmoid mucosa (Figure 4A) and a very low CD4/CD8 ratio (0.14), although we were unable to quantify the absolute counts in the histological material to confirm the CD4 loss. Importantly, in contrast to the circulating CD4 subset, the relative proportions of cells producing IL-17, IL-22, or IFN-γ, as well as FoxP3-expressing Treg, within sigmoid CD4 cells were within the range of healthy subjects (Figure 4B). Moreover, there were no alterations in the levels of expression of genes implicated in the regulation of these populations, namely, IL-17, IL-22, IL-23, IDO1, and AHR, in the sigmoid biopsies (Figure 4C). To the best of our knowledge, this is the first report of T-cell imbalances illustrating the occurrence of CD4 depletion at the gut mucosa in CGD. The apparent lack of Th17 expansion at the mucosal level warrants further investigation, given the known role of IL-17 in inflammatory bowel disorders (21, 22) and the importance of gut pathology in CGD patients.
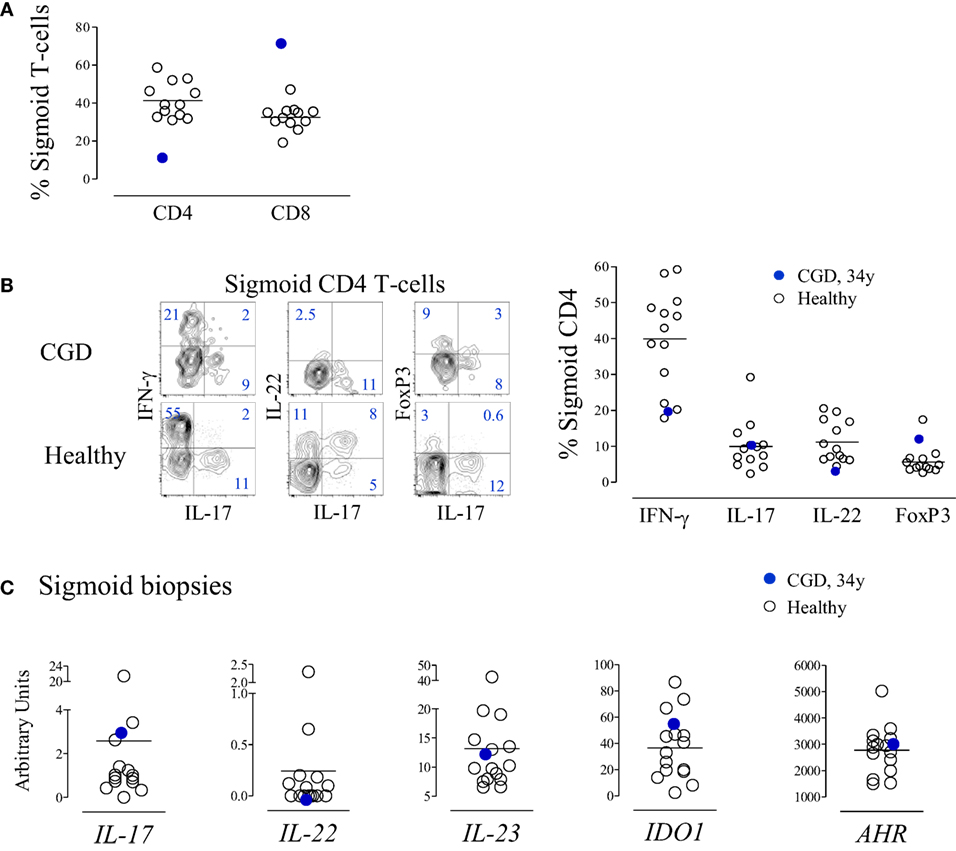
Figure 4. T-cell subsets in the gut mucosa of the chronic granulomatous disease (CGD) patient. (A) Frequency of CD4 and CD8 T-cells within CD3+ lymphocytes in cell suspensions of sigmoid biopsies from the CGD patient at 34 years of age and from healthy subjects (n = 12). (B) Representative dot plots of the expression of IFN-γ, interleukin (IL)-17, IL-22, and FoxP3 within sigmoid CD4+ cells from the CGD patient and an age-matched control; the graph indicates the frequency of IFN-γ-, IL-17-, and IL-22-producing cells, as well as FoxP3+ cells within sigmoid CD4+ cells from the CGD patient and healthy subjects. (C) IL-17, IL-22, IL-23, IDO1, and AHR mRNA levels of expression; total RNA extracted from sigmoid biopsies of the CGD patient and healthy controls and mRNA levels expressed in relative units, normalized to the mean Ct levels of GAPDH and r18S. Each dot represents one individual. Bars represent mean of values obtained in healthy controls.
Methods
Patient and Controls
Longitudinal data obtained from a 36-year-old Caucasian patient with X-linked CGD were compared with results obtained from healthy donors (23, 24) and a previously described cohort of untreated HIV-1-infected individuals (25), which featured a wide CD4 T-cell loss range. All individuals gave written informed consent. The study was approved by the ethical board of the Faculty of Medicine of University of Lisbon.
Cell Isolation
Fresh peripheral blood mononuclear cells (PBMCs) were isolated from heparinized venous blood immediately after collection by standard Ficoll-Hypaque density gradient centrifugation (Amersham Pharmacia Biotech, Uppsala, Sweden). Approximately 7–10 sigmoid biopsies collected from the patient and from individuals performing colonoscopy in the context of routine clinical follow-up with macroscopically normal mucosa (24) were digested with collagenase B (10 mg/ml; Roche, Penzberg, Germany), at 37°C, immediately after collection; mechanically macerated; and the lymphocytes separated using a Percoll gradient (GE Healthcare, Uppsala, Sweden; 40% over 80%), as previously described (24).
Flow Cytometry Studies
Lymphocyte phenotypic analysis was performed in whole blood after surface staining with monoclonal antibodies (mAbs) (26). The following anti-human mAbs were used: FITC-conjugated HLA-DR (clone L243) and CD45RA (clone HI100); PE-conjugated CD4 (clone RPA-T4), CD27 (clone O323), and CD38 (clone HB7); PerCP-conjugated CD4 (clone RPA-T4), CD8 (clone RPA-T8), and CD3 (clone OKT3); and APC-conjugated CD25 (clone 2A3), purchased from either BD Biosciences (San Jose, CA, USA) or eBiosciences (San Diego, CA, USA). Intracellular staining for FoxP3 (clone PCH101 from eBiosciences), Bcl-2 (clone 124 from Dako), Ki-67 (clone B56 from BD Biosciences), or perforin (clone 27-37 from BD Biosciences) was performed in PBMC using eBiosciences’s kit after surface staining, as previously described (26). At least 100,000 events were acquired on FACSCalibur or LSRFortessa cytometers (BD Biosciences), and data were analyzed using FlowJo software (Tree Star Inc., Ashland, OR, USA). The absolute numbers of lymphocyte subsets were calculated by multiplying their representative frequency by the absolute lymphocyte count obtained at the clinical laboratory.
Cytokine Production
Cytokine production by PBMC or mucosal lymphocytes was assessed at the single-cell level after 4-h culture with 50 ng/ml phorbol 12-myristate 13-acetate (Sigma-Aldrich, St. Louis, MO, USA) plus 500 ng/ml ionomycin (Calbiochem; Merck Millipore) in the presence of 10 µg/ml brefeldin A (Sigma-Aldrich), as previously described (27). The following anti-human mAbs were used for intracellular staining: IFN-γ (clone 4S.B3), IL-17 (clone eBio64DEC17), TNF-α (clone MAb11), and IL-22 (clone 22URTI), all from eBiosciences.
TREC Analysis
Signal joint and DJ TREC analyses were conducted as described (18, 19). Briefly, triplicate multiplex PCR amplification for sjTREC, DJβ1TRECs (Dβ1-Jβ1.1 to 1.6), or DJβ2TRECs (Dβ2-Jβ2.1 to 2.7), together with the CD3γ chain, was performed on lysed PBMC. TREC and CD3γ quantifications were then performed using a LightCycler™ in independent experiments, with the same first-round serial dilution standard curve. This highly sensitive nested quantitative PCR assay allowed detection of one copy in 105 cells for any excision circle. The sj/βTREC ratio was calculated [sjTREC/105cells/(DJβ1TRECs/105cells + DJβ2TRECs/105cells)], as described (18).
IL-7 Quantification
Serum IL-7 levels were measured using Quantikine HS ELISA kit (R&D Systems, Minneapolis, MN, USA), according to the manufacturer’s instructions (25). All samples were assayed in duplicate.
Telomere Length Measurement by Flow Fluorescent In Situ Hybridization Coupled to Flow Cytometry (Flow-FISH)
Telomere length was assessed by Flow-FISH technique on thawed PBMCs, as previously described (23, 28). Briefly, after surface staining with CD45RA-FITC, CD27-FITC, and biotin-conjugated CD4 or CD8, PBMCs were fixed for 30 min at 4°C with 1 mM bis(sulfosuccinimidyl)suberate. The reaction was quenched using 1 ml of 50 mM Tris pH7.2 for 20 min at room temperature. After washing in PBS, followed by hybridization buffer containing 70% formamide, 20 mM Tris-HCl, 1% BSA, and 1.5 M NaCl, cells were incubated with the protein nucleic acid telomeric probe (C3TA2)3 conjugated to Cy5. After heating for 10 min at 82°C, samples were left to hybridize for 60–90 min, washed in post-hybridization buffer followed by PBS, and analyzed immediately by flow cytometry. All samples were run in triplicate.
mRNA Extraction from the Gut and Transcripts Expression
One sigmoid biopsy was stored in RLT buffer (Qiagen, Valencia, CA, USA) immediately post collection. RNA was extracted using Allprep RNA/DNA mini kit (Qiagen), and 250 ng was used to synthesize cDNA (SuperScript III Reverse Transcriptase; Life Technologies), as described (24). Expression levels of IL-17A, IL-22, IL-23, indoleamine 2,3-dioxygenase (IDO) 1, and aryl hydrocarbon receptor (AHR) were measured after pre-amplification with TaqMan PreAmp Master Mix, using TaqMan gene expression assays with an Applied Biosystems 7500 Fast Real-Time PCR System (all from Life Technologies), as previously described (24). Results were expressed as ΔCt normalized to the medium Ct levels of glyceraldehyde 3-phosphate dehydrogenase (GAPDH) and r18S.
Discussion
Our finding of sustained severe long-term CD4 T-cell lymphopenia in X-linked CGD calls attention to the occurrence of major T-cell imbalances in the context of burst oxidative defects and raises the possibility of T-cell immunological exhaustion. To our knowledge, this is the first report of CD4 depletion at the gut mucosal level in CGD, in agreement with peripheral blood data. Therefore, increasing effort should be devoted to the understanding of the mechanisms underlying T-cell loss in CGD and their clinical implications.
This immune senescent profile has been considered of immunological risk for infections and shown to be an independent predictor of death in aged subjects (29). Moreover, it resembles the profile of immunosenescence documented in individuals thymectomized early in life during corrective cardiac surgery (26, 30, 31).
Our case suggests that CGD-associated T-cell depletion may worsen with age, which further supports early therapy potentially curative interventions such as HSCT and gene therapy (2, 4, 15–17, 32).
Of note, the persistent immune activation of our patient featured an AIDS-like immunological profile (33–35). CGD is typically associated with an inflammatory and hyper-activated state (3, 32, 36, 37). In CGD mouse models, inflammation has been linked to a defective production of the immunosuppressive molecule l-kynurenine (20), a tryptophan catabolism intermediate generated by the enzyme IDO, leading to increased IL-17 production. However, recent data showed that CGD patients have preserved IDO function in circulating leukocytes (38, 39). Nevertheless, our patient featured a relative expansion of IL-17-producing CD4 T-cells as compared to other CD4 T-cell subsets, in agreement with previous reports (3, 12, 20), which likely contributed to the hyperactivated state. Notably, the Th17 overrepresentation was not found at the gut mucosa level, a finding that warrants further investigation given the importance of gut pathology in CGD and the known role of IL-17 in inflammatory bowel disorders (21, 22).
The observed massive IL-7 levels may also contribute to the exaggerated immune stimulation. The increase in IL-7 levels in lymphopenic settings is thought to be mostly related to lack of consumption due to the reduction in available targets (25, 40). However, in our case, they were much above those found in untreated HIV-infected individuals with comparable T-cell depletion (25). This finding raises the possibility of IL-7 being partially produced as an acute-phase response in the liver, as reported upon toll-like receptors signaling in mouse models (41).
Of note, in spite of the high levels of IL-7, there was marked impairment of thymopoiesis and a consequent defective replenishment of the naïve compartment, as attested by the reduced sj/βTREC ratio and T-cell telomere length.
Interestingly, it has also been suggested that T-cells have intrinsic defects in ROS production in CGD, which may compromise their thymic development as well as their peripheral survival and function (6–11).
Concluding Remarks
Long-lasting severe CD4 lymphopenia may occur in CGD, likely due to both persistent immune activation and impaired T-cell production. This profile of early immune senescence represents a further argument in favor of timely curative therapeutic interventions in CGD, namely, stem-cell transplantation or gene therapy. Additionally, in a broader perspective, our results open new areas of research regarding the interplay of ROS production and T-cell homeostasis and immune senescence.
Ethics Statement
The study was approved by the ethical board of the Faculty of Medicine of University of Lisbon. All individuals gave written informed consent.
Author Contributions
AA, RV, and AS designed the study; AA, SF, RT, RC, and SS performed research; ML, SS, and RV collected clinical data; AS supervised the study; and AA and AS wrote the paper.
Conflict of Interest Statement
The authors declare that the research was conducted in the absence of any commercial or financial relationships that could be construed as a potential conflict of interest.
Acknowledgments
We acknowledge Dirk Roos for the genetic diagnosis of the CGD patient; Luís Correia for performing the colonoscopy; the technical assistance of David Gautier for TREC quantification; Alcinda Melo and Catarina Cortesão for flow cytometry studies; Ana R. Pires, Cristina Ferreira, and Emília Oliveira for immunohistochemistry studies; and Russell B. Foxall for discussions and critical review of the manuscript.
Funding
AA, SF, RT, and SS received scholarships from Fundação para a Ciência e Tecnologia (FCT), Portugal.
References
2. Holland SM. Chronic granulomatous disease. Hematol Oncol Clin North Am (2013) 27(1):89–99, viii. doi:10.1016/j.hoc.2012.11.002
3. Bylund J, MacDonald KL, Brown KL, Mydel P, Collins LV, Hancock RE, et al. Enhanced inflammatory responses of chronic granulomatous disease leukocytes involve ROS-independent activation of NF-kappa B. Eur J Immunol (2007) 37(4):1087–96. doi:10.1002/eji.200636651
4. Martire B, Rondelli R, Soresina A, Pignata C, Broccoletti T, Finocchi A, et al. Clinical features, long-term follow-up and outcome of a large cohort of patients with chronic granulomatous disease: an Italian multicenter study. Clin Immunol (2008) 126(2):155–64. doi:10.1016/j.clim.2007.09.008
5. Heltzer M, Jawad AF, Rae J, Curnutte JT, Sullivan KE. Diminished T cell numbers in patients with chronic granulomatous disease. Clin Immunol (2002) 105(3):273–8. doi:10.1006/clim.2002.5291
6. Jackson SH, Devadas S, Kwon J, Pinto LA, Williams MS. T cells express a phagocyte-type NADPH oxidase that is activated after T cell receptor stimulation. Nat Immunol (2004) 5(8):818–27. doi:10.1038/ni1096
7. Shatynski KE, Chen H, Kwon J, Williams MS. Decreased STAT5 phosphorylation and GATA-3 expression in NOX2-deficient T cells: role in T helper development. Eur J Immunol (2012) 42(12):3202–11. doi:10.1002/eji.201242659
8. Liu Q, Yi L, Sadiq-Ali S, Koontz SM, Wood A, Zhu N, et al. PP2A-dependent control of transcriptionally active FOXO3a in CD8(+) central memory lymphocyte survival requires p47(phox). Cell Death Dis (2012) 3:e375. doi:10.1038/cddis.2012.118
9. Padgett LE, Tse HM. NADPH oxidase-derived superoxide provides a third signal for CD4 T cell effector responses. J Immunol (2016) 197(5):1733–42. doi:10.4049/jimmunol.1502581
10. Kwon BI, Kim TW, Shin K, Kim YH, Yuk CM, Yuk JM, et al. Enhanced Th2 cell differentiation and function in the absence of Nox2. Allergy (2017) 72(2):252–65. doi:10.1111/all.12944
11. Wen Z, Shimojima Y, Shirai T, Li Y, Ju J, Yang Z, et al. NADPH oxidase deficiency underlies dysfunction of aged CD8+ Tregs. J Clin Invest (2016) 126(5):1953–67. doi:10.1172/JCI84181
12. Horvath R, Rozkova D, Lastovicka J, Polouckova A, Sedlacek P, Sediva A, et al. Expansion of T helper type 17 lymphocytes in patients with chronic granulomatous disease. Clin Exp Immunol (2011) 166(1):26–33. doi:10.1111/j.1365-2249.2011.04449.x
13. Montes-Berrueta D, Ramirez L, Salmen S, Berrueta L. Fas and FasL expression in leukocytes from chronic granulomatous disease patients. Invest Clin (2012) 53(2):157–67.
14. de Luca A, Smeekens SP, Casagrande A, Iannitti R, Conway KL, Gresnigt MS, et al. IL-1 receptor blockade restores autophagy and reduces inflammation in chronic granulomatous disease in mice and in humans. Proc Natl Acad Sci U S A (2014) 111(9):3526–31. doi:10.1073/pnas.1322831111
15. Kaufmann KB, Chiriaco M, Siler U, Finocchi A, Reichenbach J, Stein S, et al. Gene therapy for chronic granulomatous disease: current status and future perspectives. Curr Gene Ther (2014) 14(6):447–60. doi:10.2174/1566523214666140918113201
16. Ahlin A, Fasth A. Chronic granulomatous disease – conventional treatment vs. hematopoietic stem cell transplantation: an update. Curr Opin Hematol (2015) 22(1):41–5. doi:10.1097/MOH.0000000000000097
17. Soncini E, Slatter MA, Jones LB, Hughes S, Hodges S, Flood TJ, et al. Unrelated donor and HLA-identical sibling haematopoietic stem cell transplantation cure chronic granulomatous disease with good long-term outcome and growth. Br J Haematol (2009) 145(1):73–83. doi:10.1111/j.1365-2141.2009.07614.x
18. Dion ML, Poulin JF, Bordi R, Sylvestre M, Corsini R, Kettaf N, et al. HIV infection rapidly induces and maintains a substantial suppression of thymocyte proliferation. Immunity (2004) 21(6):757–68. doi:10.1016/j.immuni.2004.10.013
19. Dion ML, Sekaly RP, Cheynier R. Estimating thymic function through quantification of T-cell receptor excision circles. Methods Mol Biol (2007) 380:197–213. doi:10.1007/978-1-59745-395-012
20. Romani L, Fallarino F, De Luca A, Montagnoli C, D’Angelo C, Zelante T, et al. Defective tryptophan catabolism underlies inflammation in mouse chronic granulomatous disease. Nature (2008) 451(7175):211–5. doi:10.1038/nature06471
21. Fujino S, Andoh A, Bamba S, Ogawa A, Hata K, Araki Y, et al. Increased expression of interleukin 17 in inflammatory bowel disease. Gut (2003) 52(1):65–70. doi:10.1136/gut.52.1.65
22. Eastaff-Leung N, Mabarrack N, Barbour A, Cummins A, Barry S. Foxp3+ regulatory T cells, Th17 effector cells, and cytokine environment in inflammatory bowel disease. J Clin Immunol (2010) 30(1):80–9. doi:10.1007/s10875-009-9345-1
23. Tendeiro R, Albuquerque AS, Foxall RB, Cavaleiro R, Soares RS, Baptista AP, et al. Preserved CD4 T-cell telomere length during long-lasting HIV-2 infection. AIDS (2013) 27(2):289–92. doi:10.1097/QAD.0b013e32835ab234
24. Fernandes SM, Pires AR, Ferreira C, Foxall RB, Rino J, Santos C, et al. Enteric mucosa integrity in the presence of a preserved innate interleukin 22 compartment in HIV type 1-treated individuals. J Infect Dis (2014) 210(4):630–40. doi:10.1093/infdis/jiu126
25. Albuquerque AS, Cortesao CS, Foxall RB, Soares RS, Victorino RM, Sousa AE. Rate of increase in circulating IL-7 and loss of IL-7Ralpha expression differ in HIV-1 and HIV-2 infections: two lymphopenic diseases with similar hyperimmune activation but distinct outcomes. J Immunol (2007) 178(5):3252–9. doi:10.4049/jimmunol.178.5.3252
26. Silva SL, Albuquerque AS, Serra-Caetano A, Foxall RB, Pires AR, Matoso P, et al. Human naive regulatory T-cells feature high steady-state turnover and are maintained by IL-7. Oncotarget (2016) 7(11):12163–75. doi:10.18632/oncotarget.7512
27. Sousa AE, Chaves AF, Loureiro A, Victorino RM. Comparison of the frequency of interleukin (IL)-2-, interferon-gamma-, and IL-4-producing T cells in 2 diseases, human immunodeficiency virus types 1 and 2, with distinct clinical outcomes. J Infect Dis (2001) 184(5):552–9. doi:10.1086/322804
28. Plunkett FJ, Soares MV, Annels N, Hislop A, Ivory K, Lowdell M, et al. The flow cytometric analysis of telomere length in antigen-specific CD8+ T cells during acute Epstein-Barr virus infection. Blood (2001) 97(3):700–7. doi:10.1182/blood.V97.3.700
29. Boraschi D, Del Giudice G, Dutel C, Ivanoff B, Rappuoli R, Grubeck-Loebenstein B. Ageing and immunity: addressing immune senescence to ensure healthy ageing. Vaccine (2010) 28(21):3627–31. doi:10.1016/j.vaccine.2010.03.035
30. Sauce D, Larsen M, Fastenackels S, Duperrier A, Keller M, Grubeck-Loebenstein B, et al. Evidence of premature immune aging in patients thymectomized during early childhood. J Clin Invest (2009) 119(10):3070–8. doi:10.1172/JCI39269
31. Silva SL, Albuquerque AS, Matoso P, Charmeteau-de-Muylder B, Cheynier R, Ligeiro D, et al. IL-7-induced proliferation of human naive CD4 T-cells relies on continued thymic activity. Front Immunol (2017) 8:20. doi:10.3389/fimmu.2017.00020
32. Segal BH, Veys P, Malech H, Cowan MJ. Chronic granulomatous disease: lessons from a rare disorder. Biol Blood Marrow Transplant (2011) 17(1 Suppl):S123–31. doi:10.1016/j.bbmt.2010.09.008
33. Albuquerque AS, Foxall RB, Cortesao CS, Soares RS, Doroana M, Ribeiro A, et al. Low CD4 T-cell counts despite low levels of circulating HIV: insights from the comparison of HIV-1 infected patients with a discordant response to antiretroviral therapy to patients with untreated advanced HIV-2 disease. Clin Immunol (2007) 125(1):67–75. doi:10.1016/j.clim.2007.07.001
34. Grossman Z, Meier-Schellersheim M, Sousa AE, Victorino RM, Paul WE. CD4+ T-cell depletion in HIV infection: are we closer to understanding the cause? Nat Med (2002) 8(4):319–23. doi:10.1038/nm0402-319
35. Sousa AE, Carneiro J, Meier-Schellersheim M, Grossman Z, Victorino RM. CD4 T cell depletion is linked directly to immune activation in the pathogenesis of HIV-1 and HIV-2 but only indirectly to the viral load. J Immunol (2002) 169(6):3400–6. doi:10.4049/jimmunol.169.6.3400
36. Meissner F, Seger RA, Moshous D, Fischer A, Reichenbach J, Zychlinsky A.Inflammasome activation in NADPH oxidase defective mononuclear phagocytes from patients with chronic granulomatous disease. Blood (2010) 116(9):1570–3. doi:10.1182/blood-2010-01-264218
37. Hatanaka E, Carvalho BT, Condino-Neto A, Campa A. Hyperresponsiveness of neutrophils from gp 91phox deficient patients to lipopolysaccharide and serum amyloid A. Immunol Lett (2004) 94(1–2):43–6. doi:10.1016/j.imlet.2004.04.016S0165247804001178
38. De Ravin SS, Zarember KA, Long-Priel D, Chan KC, Fox SD, Gallin JI, et al. Tryptophan/kynurenine metabolism in human leukocytes is independent of superoxide and is fully maintained in chronic granulomatous disease. Blood (2010) 116(10):1755–60. doi:10.1182/blood-2009-07-233734
39. Jurgens B, Fuchs D, Reichenbach J, Heitger A. Intact indoleamine 2,3-dioxygenase activity in human chronic granulomatous disease. Clin Immunol (2010) 137(1):1–4. doi:10.1016/j.clim.2010.05.007
40. Napolitano LA, Grant RM, Deeks SG, Schmidt D, De Rosa SC, Herzenberg LA, et al. Increased production of IL-7 accompanies HIV-1-mediated T-cell depletion: implications for T-cell homeostasis. Nat Med (2001) 7(1):73–9. doi:10.1038/83381
Keywords: primary immunodeficiency, chronic granulomatous disease, genetic phagocytic defect, reactive oxygen species, CD4 T-cell lymphopenia, immune senescence, gut mucosa, interleukin-17
Citation: Albuquerque AS, Fernandes SM, Tendeiro R, Cheynier R, Lucas M, Silva SL, Victorino RMM and Sousa AE (2017) Major CD4 T-Cell Depletion and Immune Senescence in a Patient with Chronic Granulomatous Disease. Front. Immunol. 8:543. doi: 10.3389/fimmu.2017.00543
Received: 17 February 2017; Accepted: 24 April 2017;
Published: 11 May 2017
Edited by:
Andrew Gennery, Newcastle University, UKCopyright: © 2017 Albuquerque, Fernandes, Tendeiro, Cheynier, Lucas, Silva, Victorino and Sousa. This is an open-access article distributed under the terms of the Creative Commons Attribution License (CC BY). The use, distribution or reproduction in other forums is permitted, provided the original author(s) or licensor are credited and that the original publication in this journal is cited, in accordance with accepted academic practice. No use, distribution or reproduction is permitted which does not comply with these terms.
*Correspondence: Ana E. Sousa, YXNvdXNhQG1lZGljaW5hLnVsaXNib2EucHQ=