- Thyroid Autoimmune Disease Unit, Cedars-Sinai Medical Center, UCLA School of Medicine, Los Angeles, CA, United States
Evidence for original antigenic sin in spontaneous thyroid autoimmunity is revealed by autoantibody interactions with immunodominant regions on thyroid autoantigens, thyroglobulin (Tg), thyroid peroxidase (TPO), and the thyrotropin receptor (TSHR) A-subunit. In contrast, antibodies induced by immunization of rabbits or mice recognize diverse epitopes. Recognition of immunodominant regions persists despite fluctuations in autoantibody levels following treatment or over time. The enhancement of spontaneously arising pathogenic TSHR antibodies in transgenic human thyrotropin receptor/NOD.H2h4 mice by injecting a non-pathogenic form of TSHR A-subunit protein also provides evidence for original antigenic sin. From other studies, antigen presentation by B cells, not dendritic cells, is likely responsible for original antigenic sin. Recognition of restricted epitopes on the large glycosylated thyroid autoantigens (60-kDa A-subunit, 100-kDa TPO, and 600-kDa Tg) facilitates exploring the amino acid locations in the immunodominant regions. Epitope spreading has also been revealed by autoantibodies in thyroid autoimmunity. In humans, and in mice that spontaneously develop autoimmunity to all three thyroid autoantigens, autoantibodies develop first to Tg and later to TPO and the TSHR A-subunit. The pattern of intermolecular epitope spreading is related in part to the thyroidal content of Tg, TPO and TSHR A-subunit and to the molecular sizes of these proteins. Importantly, the epitope spreading pattern provides a rationale for future antigen-specific manipulation to block the development of all thyroid autoantibodies by inducing tolerance to Tg, first in the autoantigen cascade. Because of its abundance, Tg may be the autoantigen of choice to explore antigen-specific treatment, preventing the development of pathogenic TSHR antibodies.
Introduction
The concept of “original antigenic sin” arose from findings in humans and mice infected with influenza virus (1, 2) and in mice responding to Chlamydia proteins (3). For example, in sequential immunization of mice with two antigenically related but different strains of influenza A virus, antibodies induced by the second infection reacted more strongly with the primary than with the secondary virus (1). Similarly, humans infected with a novel influenza virus expanded antibodies against a viral strain of a previous infection and failed to develop antibody responses to epitopes on the new viral strain (4). Unlike in viral infections, the presently held authoritative opinion is that autoimmunity involves the converse of the “doctrine” of original antigenic sin, thereby facilitating “an unforseen platform for immune therapy” (5). In this review, we present contrary evidence supporting the concept of an original antigenic sin component occurring for autoantibodies in thyroid autoimmunity and perhaps for other autoantibody-mediated diseases.
A phenomenon interlinked with original antigenic sin is epitope spreading, a well-recognized feature of some autoimmune conditions such as type 1 diabetes mellitus and multiple sclerosis, as well as for the animal models of these diseases, namely NOD mice and experimental autoimmune encephalomyelitis (EAE). Spreading can involve increasing the number of epitopes recognized on the same autoantigen (intramolecular) and subsequent recognition of additional autoantigens (intermolecular) over time. An example of intermolecular spreading, in EAE, SWX mice immunized with myelin proteolipid protein (PLP) develop the (as expected) T cell reactivity to determinants on PLP and to determinants on myelin basic protein and myelin oligodendrocyte glycoprotein (6). Similarly, in type 1 diabetes that develops spontaneously in NOD mice, T cell recognition of islet autoantigens spreads from proinsulin to other islet autoantigens such as islet-specific glucose-6-phosphatase catalytic subunit-related protein (IGRP) [for example, Ref. (7, 8)].
Thyroid autoimmune disease, Hashimoto’s thyroiditis, and Graves’ disease are the most common organ-specific autoimmune diseases affecting humans, far more common than type 1 diabetes mellitus and multiple sclerosis. In particular, approximately 1% of the population will develop Graves’ disease in their lifetime and ~15% of adult females have autoimmune thyroiditis, although usually subclinical (9, 10). Animal models (induced and spontaneous) are available that provide insight into thyroid autoimmunity in humans. Three thyroid-specific autoantigens are targeted by the immune system: abundant soluble thyroglobulin (Tg), the much less abundant membrane-bound protein thyroid peroxidase (TPO), and the thyrotropin receptor (TSHR) [reviewed in Ref. (11)]. Although the great majority of Hashimoto patients have autoantibodies to TPO and many have Tg autoantibodies, hypothyroid patients with seronegative Hashimoto’s disease have been reported (12).
Whether autoantibodies to TPO and Tg play a role in thyroid cell destruction is unclear, but they are excellent markers of the immune response to the thyroid. Moreover, B cells are increasingly recognized as powerful antigen-presenting cells by means of their membrane-bound antibodies that capture small amount of antigen for processing and presentation to T cells [for example, Ref. (13)]. TPO autoantibody-mediated and -modulated presentation to T cells has been reported (14, 15). Also, the enhancing or suppressing effects of Tg antibodies on the processing of a pathogenic T cell epitope on Tg have been described (16). Importantly, the successful treatment of Graves’ ophthalmopathy patients with a monoclonal antibody to B cells (ritixumab) was suggested to involve antibody presentation by B cells (17). Turning to responses to the TSHR, stimulating TSHR autoantibodies are the direct cause of hyperthyroidism in Graves’ disease [reviewed in Ref. (18)] and blocking TSHR autoantibodies are responsible for hypothyroidism in rare patients [for example, Ref. (19)].
Here, we examine evidence involving thyroid autoantibodies for both intramolecular original antigenic sin and intermolecular epitope spreading in autoimmune thyroid disease. Our findings have important implications for understanding disease pathogenesis and for developing novel antigen-specific therapeutic approaches to control the development of thyroid autoantibodies and thereby prevent thyroid autoimmunity rather than treating the clinical disease.
Autoantibody Recognition in Humans of an Immunodominant Region (IDR)—A Reflection of Original Antigenic Sin
Antibody Recognition of an IDR on Tg and TPO
It has long been recognized that antibodies induced experimentally to Tg interact with multiple, widely diverse epitopes on the large (600 kDa) dimeric Tg molecule, whereas human Tg autoantibodies interact with a restricted number of epitopes (Figure 1) (20–23). Similar observations have been made for TPO (24, 25). Panels of human monoclonal TPO and Tg autoantibodies isolated from combinatorial immunoglobulin libraries and expressed as recombinant Fab confirmed restricted epitope recognition [for example (26, 27)]. Recombinant Fab provided the tools to characterize the IDRs on TPO and Tg recognized by antibodies in patients and some euthyroid controls with subclinical disease (Table 1). Characterization of the epitopes recognized by TPO and Tg autoantibodies has also been performed by competition assays using mouse monoclonal antibodies generated (for example) to Tg polypeptides (28), to purified TPO (25), or to TPO peptides (25, 29).
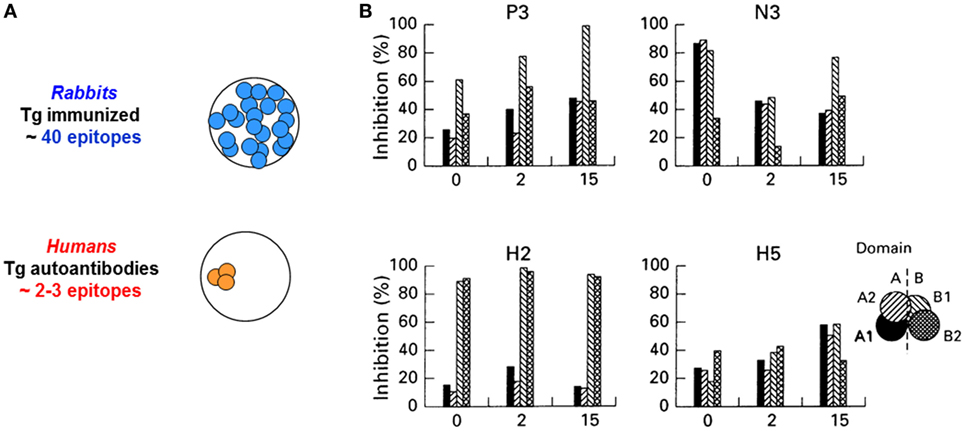
Figure 1. (A) Diverse antibody epitopic recognition in rabbits immunized with human thyroglobulin (Tg) versus restricted antibody recognition by human Tg autoantibodies in humans. Schematic illustration of the concept described in Ref. (20–23). (B) Recognition of thyroid peroxidase autoantibody epitopes (“fingerprints”) is stable over 15 years. The inset provides the key to the A and B domains (and subdomains) recognized by autoantibodies in the sera. Adapted from Ref. (30).
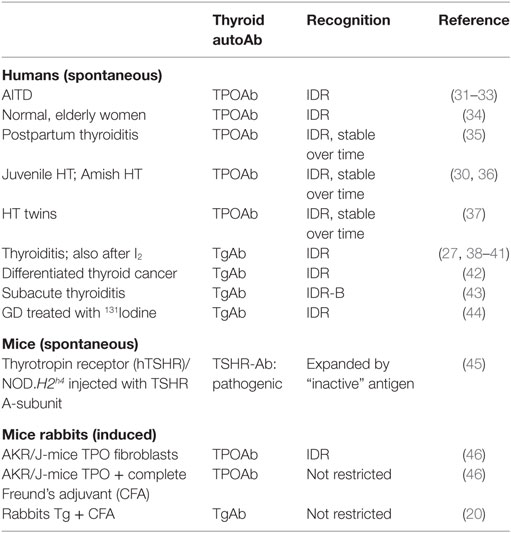
Table 1. Recognition of an autoantibody immunodominant region (IDR) on thyroglobulin and thyroid peroxidase (TPO) in spontaneous thyroid autoimmunity (humans and a mouse model) versus thyroid antibodies induced in rabbits or mice that are either restricted or not restricted to an IDR.
It should be emphasized that binding by the majority of patients’ autoantibodies to TPO is decreased following protein denaturation (47, 48), demonstrating that most TPO autoantibodies interact with epitopes on conformationally intact protein. However, some studies have shown interaction between serum autoantibodies and reduced and denatured TPO [for example, Ref. (49, 50)], suggesting that not all TPO epitopes are conformational. Further, several linear epitopes (51, 52) and polypeptide fragments (53–56) are recognized by some patients’ TPO autoantibodies. Similarly, Tg autoantibodies predominantly recognize native protein [for example, Ref. (57)]. However, recognition of peptide fragments by some Tg autoantibodies has been reported [for example, Ref. (38, 58)].
In this review, because of their dominance in the patient repertoire, we will focus on autoantibodies to TPO and Tg that interact with conformational epitopes.
Autoantibody Recognition of TPO and Tg Is Stable over Time
TPOAb epitopic “fingerprints” of the IDR are stable over time, that is without intramolecular epitope spreading, for example, in families in which the proband has juvenile Hashimoto’s thyroiditis (30), women who develop postpartum thyroiditis (35), and for TgAb in most patients on iodine supplementation (39) (Table 1). Similarly, after iodine-131 treatment in Graves’ disease, there was no evidence of TgAb intramolecular antibody spreading (44). There is some evidence that TPOAb epitopic patterns are inherited in families (36, 37).
TSHR Autoantibody Recognition
Changes are rare in the epitopes recognized by TSHR antibodies, namely thyroid-stimulating antibodies (TSAbs), which are responsible for hyperthyroidism in Graves’ disease, and TSH-blocking antibodies (TBAbs), which cause hypothyroidism. In rare patients, TSHRAb switching from TSAb to TBAb (or vice versa) has been observed [reviewed in Ref. (59)]. The derivation of monoclonal TSAb and TBAb from one blood sample of an unusual patient who alternated between hyperthyroidism and hypothyroidism (60) demonstrated that the contrasting serum biological activities were due to two distinct antibodies.
IDR Recognition by TPOAb Induced in Mice
It is of interest that injecting mice with TPO expressed together with MHC class II on a fibroblast line induced TPOAb that resembled autoantibodies from Hashimoto or Graves’ patients in terms of a high affinity for TPO and recognition of an IDR (46). In the same mouse strain, conventional immunization with TPO protein and adjuvant induced antibodies with lower affinity that recognized diverse epitopes on TPO (Table 1).
Other Autoantibody Recognition of an IDR
Autoantibodies interact with epitopes in an IDR in other autoantibody-mediated diseases [reviewed in Ref. (61)]. For example, in myasthenia gravis, autoantibodies to the acetylcholine receptor are restricted to a major IDR on the α1 subunit (62). Similarly, in the skin blistering diseases such as pemphigus vulgaris and pemphigus foliaceous, autoantibodies interact with an IDR on desmoglein (63).
Overall, the stability of human thyroid autoantibody recognition of an IDR is suggestive of “original antigenic sin.” It is possible that this concept may also apply to other human autoantibodies directed to an IDR on their respective autoantigens.
Evidence for Original Antigenic Sin in a Mouse Model of Thyroid Autoimmunity
In mice induced to develop Graves’ disease by immunization with an adenovirus encoding the TSHR A-subunit gene, pretreatment with a non-pathogenic (or “inactive”) form of TSHR A-subunit protein attenuated hyperthyroidism by diverting pathogenic TSHR antibodies to a non-functional variety (64). Subsequently, pathogenic TSHR antibody diversion was attempted using the same approach in a mouse model that spontaneously develops pathogenic TSHR autoantibodies, human thyrotropin receptor (hTSHR)/NOD.H2h4 mice with the human TSHR A-subunit transgene targeted to the thyroid (65). Unexpectedly, in an example of original antigenic sin, rather than attenuating the pre-existing pathogenic TSHRAb level, injecting “inactive” TSHR A-subunit protein into hTSHR/NOD.H2h4 mice enhanced the levels of pathogenic TSH-binding inhibition and TSAbs, as well as increasing the levels of non-pathogenic antibodies detected by ELISA (45). This effect was TSHR specific as spontaneously occurring autoantibodies to Tg and TPO were unaffected.
In hTSHR/NOD.H2h4 mice, the original antigenic sin is the initial selection of B cells for the transgenically expressed TSHR protein, namely precursors specific for both non-functional antibodies (detectable only by ELISA) as well as pathogenic TSHR antibodies (detectable only in functional assays) (Figure 2). B cells with affinity for self antigens (like the transgenic hTSHR) are tolerized by a number of mechanisms including receptor editing and anergy (functional unresponsiveness) rather than deletion as for self-reactive T cells (66). By using transgenic hen egg lysozyme-specific transgenic mouse models, it was demonstrated that self-reactive B cells were not eliminated when this antigen was expressed by thyroid cells (67). Similarly, in the spontaneous hTSHR/NOD.H2h4 model, two types of precursor B cells for pathogenic and non-pathogenic TSHRAb remain in the repertoire and both can be expanded by the “cross-reacting” antigen, the non-pathogenic TSHR A-subunit protein.
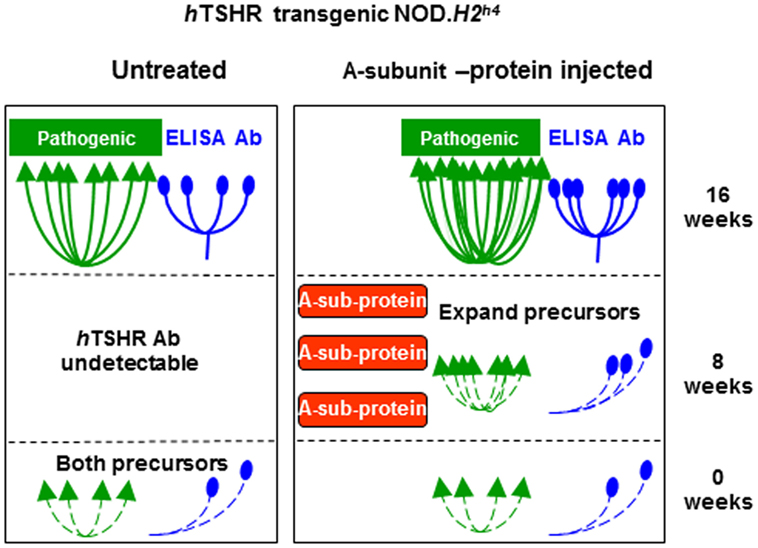
Figure 2. “Original antigenic sin” observed in human thyrotropin receptor (hTSHR)/NOD.H2h4 mice injected with an inappropriate form of thyrotropin receptor A-subunit or saline (as control). See text for explanation. Adapted with permission from Ref. (45).
Mechanisms and Implications of Original Antigenic Sin in Thyroid Autoimmunity
Mechanisms of Original Antigenic Sin
The mechanisms responsible for original antigenic sin are not fully understood. However, because of the problems caused for vaccination against novel viral strains, approaches have been used to overcome original antigenic sin. These studies provide insight into the basis for this phenomenon, at least from the perspective of T cell epitopes.
One approach involves eliciting cross-reactive responses by immunization with multiple peptide variants (68) or injecting yeasts carrying diverse virus-like particles (69). It has also been suggested that, at least for CD8+ T cell responses, original antigen sin can be overcome by treatment with neutralizing interleukin 10 together with linked T cell epitopes (70). A different strategy involves immunization with adjuvants (such as Bordetella pertussis toxin) that activate dendritic cells (71). The latter approach is of interest in the context of comments by Kim et al. (4) concerning viral antibodies, namely that antigen presentation by B cells favors the activation of memory B cells specific for the first virus rather than naive B cells specific for the subsequent cross-reacting virus. Finally, a simple mechanistic explanation proposes that original antigenic sin occurs because regulatory T cells induced by the first antigen decrease the amount of the second antigen on dendritic cells that activate naive B cells (72).
Implications of Original Antigenic Sin in Thyroid Autoimmunity
The most likely explanation for original antigenic sin in thyroid autoantibodies appears to involve antigen presentation by B cells or (in the case of TPO) thyroid cells, rather than dendritic cells. It should be noted that a restricted antibody response focused on the TPO IDR (as in humans) occurs in AKR/J-mice injected with cells expressing human TPO but not in mice of the same strain immunized with human TPO protein and adjuvant (46). The restricted response is likely related to the much lower concentration of cell-associated TPO than TPO injected with adjuvant. Because of their efficiency as specific antigen-presenting cells [for example, Ref. (13)], B cells are likely the antigen-presenting cells in spontaneously arising autoantibodies to Tg and the TSHR A-subunit. Via their specific immunoglobulin receptors, B cells may also capture conformationally intact thyroid autoantigens, a critical factor for the induction of pathogenic TSHR antibodies [reviewed in Ref. (73)].
In addition to suggesting the likely cells involved in autoantigen presentation, IDR recognition simplifies what would otherwise be a gargantuan task, namely exploring the regions recognized by autoantibodies on large, glycosylated thyroid autoantigens:
(i) Tg, the largest thyroid autoantigen comprising a homodimer of 300 kDa molecules, poses a major challenge. Iodination of Tg alters recognition by human autoantibodies (74), possibly by denaturing the antigen. However, epitope mapping using Tg fragments (fusion proteins or digestion products) suggests that some Tg autoantibody epitopes are located in the central region (75) or at the C-terminal end of the molecule (76, 77).
(ii) TPO is a homodimer (each 110 kDa) inserted in the plasma membrane, and TPO autoantibodies are directed against the ectodomain. The TPO epitope of an human autoantibody (expressed as a recombinant Fab) was identified using footprinting technology (78). In addition, progress has been made in delineating the amino acids targeted by other autoantibodies [for example, Ref. (79–81)]. However, definitive mapping of autoantibodies epitopes will require crystallization of a TPO monoclonal autoantibody with TPO protein.
(iii) The TSHR, like TPO, is also membrane bound but pathogenic TSHR autoantibodies (as in Graves’ disease) are induced spontaneously to the heavily glycosylated TSHR A-subunit (~60 kDa) shed after cleavage of the membrane-bound receptor [reviewed in Ref. (73)]. Amino acid residues involved in the binding sites of TSAb were initially explored using chimeric TSHR-luteinizing hormone receptors together with mutagenesis [for example, Ref. (82)]. More recently, the epitopes for monoclonal human (M22) and a monoclonal human TBAb (K1-70) have been determined by co-crystalizing each antibody with the major portion of the TSHR A-subunit (83, 84).
Epitope Spreading of Thyroid Autoantibodies
Intermolecular Spreading
Induced and spontaneous intermolecular spreading for thyroid autoantibodies has been demonstrated for thyroid autoimmunity (Table 2). For example, rabbits immunized with human Tg and complete Freund’s adjuvant develop antibodies to Tg, as expected, as well as antibodies to TPO and antibodies that bind to both Tg and TPO (85, 86). It should be noted that searching for previously postulated bispecific human autoantibodies that recognize both Tg and TPO (“TgPO antibodies”) [for example, Ref. (87)] in a phage display immunoglobulin gene combinatorial, constructed from thyroid-infiltrating B cells of a patient with library serum TgPO-like autoantibody activity, led to multiple antibodies specific for either Tg or TPO but none had TgPOAb activity (88).
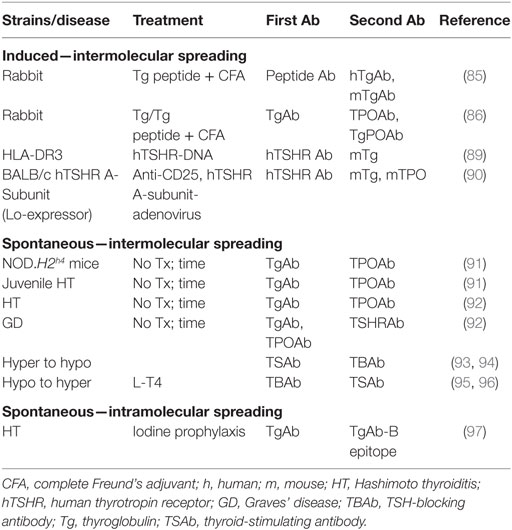
Table 2. Thyroid antibodies: induced or spontaneous intermolecular or intramolecular antigenic spreading.
Returning to intermolecular epitope spreading, transgenic HLA-DR3 mice immunized with hTSHR DNA develop TSHR antibodies and, in a few mice, mild thyroiditis in association with antibodies to Tg (89). Transgenic BALB/c mice expressing low intrathyroidal levels of the human TSHR A-subunit, depleted of regulatory T cells (CD25 positive) before immunization with human TSHR-A-subunit adenovirus, developed TSHR antibodies and (unexpectedly) TgAb- and TPOAb-associated with massive thyroiditis and hypothyroidism (90). Incidentally, anti-CD25-treated BALB/c mice transplanted with TUBO tumor cells developed antitumor responses together with antithyroid immunity (98).
Of particular interest are observations of spontaneous antibody epitope spreading. NOD.H2h4 mice develop thyroid autoimmunity, a process that is enhanced by iodide in the drinking water. The first autoantibodies to appear are directed against Tg (99–101), and subsequently, TPO antibodies appear (91). A similar pattern was observed in siblings of probands with juvenile Hashimoto thyroiditis (91). Moreover, TSHR antibodies develop spontaneously in NOD.H2h4 mice transgenically expressing the human TSHR A-subunit (65).
Combining published data from published studies (45, 102) studies, the development of autoantibodies in female hTSHR/NOD.H2h4 mice permits comparing the appearance of thyroid autoantibodies over time: TgAbs are present in some 4-month-old mice, and TSHRAb and TPOAb are detectable after the age of 6 months, and all three thyroid autoantibodies were present in more mice after 10 months (Figure 3A). Turning to humans, in a study of prediagnostic markers in Graves’ patients, TPOAb and TgAb were detectable several years before TSHR antibodies (92). Importantly, although in humans the maximum percentage of positive TgAb and TPOAb in humans did not approach 100% as in NOD.H2h4 mice, the time sequence of antibody reactivity to Tg, TPO, and the TSHR in transgenic NOD.H2h4 mice (Figure 3A) resembles that in humans (Figure 3B).
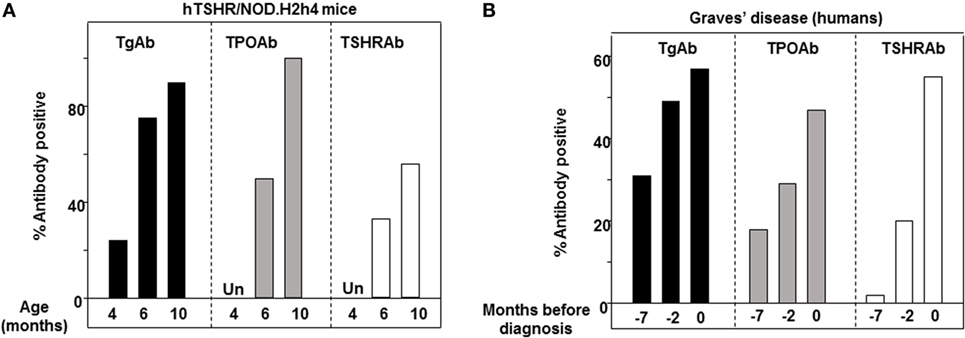
Figure 3. Intermolecular antigenic spreading from thyroglobulin (Tg) to thyrotropin receptor (TPO) and the thyrotropin receptor (TSHR). (A) Percentage of human thyrotropin receptor (hTSHR)/NOD.H2h4 female mice positive for TgAb, TPOAb, and TSHRAb (TSHR binding inhibition) aged 4, 6, and 10 months. (B) Prediagnostic TgAb, TPOAb, and TSHRAb levels (7, 2, and 0 years) in patients with Graves’ disease [data plotted from Ref. (92)].
Intramolecular Spreading
There is less extensive evidence for intramolecular thyroid autoantibody epitope spreading (Table 2): Latrofa and colleagues demonstrated that iodine prophylaxis revealed recognition of a previously cryptic TgAb epitope, but this phenomenon may involve the iodination of Tg, thereby generating a neo-antigen (97). In addition, as already mentioned, there are rare examples of TSHR antibody switching from TBAb to TSAb associated with thyroxine therapy, or the reverse, namely TBAb to switching to TSAb [reviewed in Ref. (59)]. As would be expected for antibodies with differing functional effects, the epitopes on the TSHR ectodomain recognized by TSAb and TBAb are different (103, 104), although they interact with closely overlapping portions of the amino terminus of the TSHR A-subunit (105).
The apparent antibody epitope spreading in maternally transferred TSHR antibodies from initial TBAb to TSAb is due to progressive dilution of the high titer TBAb overpowering the lower concentrations of TSAb as human IgG is metabolized [reviewed in Ref. (59)].
Implications of Epitope Spreading for Thyroid Autoimmunity
The phenomenon of epitope spreading provides important background information on thyroid autoantigen recognition. In particular, epitope spreading appears to be related to the amount and the size of each thyroid autoantigen, being greatest for Tg and less for both TPO and the TSHR A-subunit in the thyroid as well as in the thymus (Figure 4) [reviewed in Ref. (11)]. In part, epitope spreading involves the greater availability of peptides available to stimulate T cells and protein to stimulate B cells from the abundant, large Tg molecule compared with the more limited amount and smaller molecular size of TPO and even fewer peptides from the TSHR A-subunit [reviewed in Ref. (11)]. Central tolerance also plays a role, with responses regulated by intrathymic expression of antigens like the highly expressed transgenic human TSHR A-subunit (106).
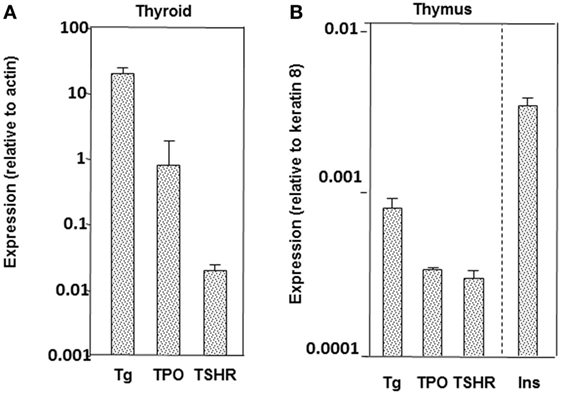
Figure 4. Relative expression of thyroglobulin (Tg), thyrotropin receptor (TPO), and the thyrotropin receptor (TSHR) in thyroid (A) and thymus (B) in mice (BALB/c strain). For the thymus, data are included for insulin, which is highly expressed in the thymus. Adapted with permission from the data in Ref. (107).
Knowledge of the autoantigen “cascade” suggests approaches for antigen-specific treatment. For example, in NOD mice, responses against islet antigens are prevented by inducing tolerance against proinsulin but not against IGRP (8), an autoantigen recognized later in the “cascade.” In the same way, it is possible that successful induction of tolerance against Tg in NOD.H2h4 mice could prevent the subsequent breakdown in tolerance to TPO (91) and perhaps even to the TSHR in hTSHR/NOD.H2h4 mice (65). In a mouse model of experimentally induced thyroiditis, increasing the circulating level of Tg strengthened self-tolerance and reduced the extent of experimental thyroiditis [reviewed in Ref. (108)]. These findings suggest that increasing Tg levels could possibly regulate autoimmunity to Tg in the hTSHR/NOD.H2h4 strain. However, as we previously reported, an antigen-specific approach used successfully in an induced model may not be directly applicable to a spontaneous model (45). Consequently, it may be necessary to test multiple modes of Tg presentation to downregulate the cascade of autoimmune responses to Tg, TPO, and the TSHR in hTSHR/NOD.H2h4 mice.
Conclusion
The evidence for intermolecular epitope spreading in thyroid autoimmunity is focused on autoantibodies, unlike the emphasis on T cell epitope spreading in multiple sclerosis and IDDM type 1 (or their animal models EAE and NOD mice). Unlike multiple sclerosis or EAE, original antigenic sin appears to be characteristic of thyroid autoimmunity as reflected by the recognition of an autoantibody IDR in humans and in the response of transgenic hTSHR/NOD.H2h4 mice to injection with inappropriate TSHR antigen. This difference likely reflects the importance of T cell immunity in EAE/MS versus autoantibodies in thyroid autoimmunity, particularly Graves’ disease for which stimulatory autoantibodies to the TSHR are the direct cause.
Finally, the pattern observed for intermolecular epitope spreading provides the rationale for antigen-specific manipulation to block thyroid autoantibody development by inducing tolerance to the first autoantigen in the antigen cascade, namely Tg. Because of its abundance, Tg may be the autoantigen of choice to explore antigen-specific treatment to block the development of pathogenic TSHR antibodies.
Author Contributions
SM and BR contributed to the concept, writing, and preparation of figures for this review.
Conflict of Interest Statement
The authors declare that the research was conducted in the absence of any commercial or financial relationships that could be construed as a potential conflict of interest.
The handling editor declared a past co-authorship with author SM.
Funding
National Institutes of Health Grants DK 54684 (SM) and previously funded National Institutes of Health Grants 19289 (BR) and 36182 (BR).
References
1. Virelizier JL, Allison AC, Schild GC. Antibody responses to antigenic determinants of influenza virus hemagglutinin. II. Original antigenic sin: a bone marrow-derived lymphocyte memory phenomenon modulated by thymus-derived lymphocytes. J Exp Med (1974) 140(6):1571–8. doi:10.1084/jem.140.6.1571
2. Webster RG, Kasel JA, Couch RB, Laver WG. Influenza virus subunit vaccines. II. Immunogenicity and original antigenic sin in humans. J Infect Dis (1976) 134(1):48–58. doi:10.1093/infdis/134.1.48
3. Berry JD, Peeling RW, Brunham RC. Analysis of the original antigenic sin antibody response to the major outer membrane protein of Chlamydia trachomatis. J Infect Dis (1999) 179(1):180–6. doi:10.1086/314538
4. Kim JH, Skountzou I, Compans R, Jacob J. Original antigenic sin responses to influenza viruses. J Immunol (2009) 183(5):3294–301. doi:10.4049/jimmunol.0900398
5. Steinman L. Absence of “original antigenic sin” in autoimmunity provides an unforeseen platform for immune therapy. J Exp Med (1999) 189(7):1021–4. doi:10.1084/jem.189.7.1021
6. Tuohy VK, Yu M, Yin L, Kawczak JA, Kinkel RP. Spontaneous regression of primary autoreactivity during chronic progression of experimental autoimmune encephalomyelitis and multiple sclerosis. J Exp Med (1999) 189(7):1033–42. doi:10.1084/jem.189.7.1033
7. Prasad S, Kohm AP, McMahon JS, Luo X, Miller SD. Pathogenesis of NOD diabetes is initiated by reactivity to the insulin B chain 9-23 epitope and involves functional epitope spreading. J Autoimmun (2012) 39(4):347–53. doi:10.1016/j.jaut.2012.04.005
8. Krishnamurthy B, Dudek NL, McKenzie MD, Purcell AW, Brooks AG, Gellert S, et al. Responses against islet antigens in NOD mice are prevented by tolerance to proinsulin but not IGRP. J Clin Invest (2006) 116(12):3258–65. doi:10.1172/JCI29602
9. Vanderpump MPJ, Tunbridge WMG, French JM, Appleton D, Bates D, Clark F, et al. The incidence of thyroid disorders in the community: a twenty-year follow-up of the Whickham survey. Clin Endocrinol (1995) 43:55–68. doi:10.1111/j.1365-2265.1995.tb01894.x
10. Hollowell JG, Staehling NW, Flanders WD, Hannon WH, Gunter EW, Spencer CA, et al. Serum TSH, T(4), and thyroid antibodies in the United States population (1988 to 1994): National Health and Nutrition Examination Survey (NHANES III). J Clin Endocrinol Metab (2002) 87(2):489–99. doi:10.1210/jcem.87.2.8182
11. McLachlan SM, Rapoport B. Breaking tolerance to thyroid antigens: changing concepts in thyroid autoimmunity. Endocr Rev (2014) 35(1):59–105. doi:10.1210/er.2013-1055
12. Rotondi M, de Martinis L, Coperchini F, Pignatti P, Pirali B, Ghilotti S, et al. Serum negative autoimmune thyroiditis displays a milder clinical picture compared with classic Hashimoto’s thyroiditis. Eur J Endocrinol (2014) 171(1):31–6. doi:10.1530/EJE-14-0147
13. Lanzavecchia A. Antigen-specific interaction between T and B cells. Nature (1985) 314:537–9. doi:10.1038/314537a0
14. Guo J, Quaratino S, Jaume JC, Costante G, Londei M, McLachlan SM, et al. Autoantibody-mediated capture and presentation of autoantigen to T cells via the Fc epsilon receptor by a recombinant human autoantibody Fab converted to IgE. J Immunol Methods (1996) 195:81–92. doi:10.1016/0022-1759(96)00091-9
15. Quaratino S, Osman M, Ruf J, McLachlan SM, Rapoport B, Londei M. Human autoantibodies modulate the T cell epitope repertoire but fail to unmask a pathogenic cryptic epitope. J Immunol (2005) 174:557–63. doi:10.4049/jimmunol.174.1.557
16. Dai Y, Carayanniotis KA, Eliades P, Lymberi P, Shepherd P, Kong Y, et al. Enhancing or suppressive effects of antibodies on processing of a pathogenic T cell epitope in thyroglobulin. J Immunol (1999) 162(12):6987–92.
17. Salvi M, Vannucchi G, Curro N, Campi I, Covelli D, Dazzi D, et al. Efficacy of B-cell targeted therapy with rituximab in patients with active moderate to severe Graves’ orbitopathy: a randomized controlled study. J Clin Endocrinol Metab (2015) 100(2):422–31. doi:10.1210/jc.2014-3014
18. Rapoport B, McLachlan SM. The thyrotropin receptor in Graves’ disease. Thyroid (2007) 17(10):911–22. doi:10.1089/thy.2007.0170
19. Endo K, Kasagi K, Konishi J, Ikekubo K, Tatsuyo O, Takeda Y, et al. Detection and properties of TSH-binding inhibitor immunoglobulins in patients with Graves’ disease and Hashimoto’s thyroiditis. J Clin Endocrinol Metab (1978) 46:734–9. doi:10.1210/jcem-46-5-734
20. Roitt IM, Campbell PN, Doniach D. The nature of the thyroid auto-antibodies present in patients with Hashimoto’s thyroiditis (lymhpadenoid goitre). Biochem J (1958) 69:248–57. doi:10.1042/bj0690248
21. Shulman S, Witebsky E. Studies on organ specificity. IX. Biophysical and immunochemical studies on human thyroid autoantibody. J Immunol (1960) 85:559–67.
22. Nye L, Pontes de Carvalho LC, Roitt IM. Restrictions in the response to autologous thyroglobulin in the human. Clin Exp Immunol (1980) 41:252–63.
23. Male DK, Champion BR, Pryce G, Matthews H, Shepherd P. Antigenic determinants of human thyroglobulin differentiated using antigen fragments. Immunology (1985) 54:419–26.
24. Ruf J, Toubert M, Czarnocka B, Durand-Gorde J, Ferrand M, Carayon P. Relationship between immunological structure and biochemical properties of human thyroid peroxidase. Endocrinology (1989) 125:1211–8. doi:10.1210/endo-125-3-1211
25. Czarnocka B, Pastuszko D, Carayon P, Ruf J, Gardas A. Majority of thyroid peroxidase in patients with autoimmune thyroid disease are directed to a single TPO domain. Autoimmunity (1996) 23:145–54. doi:10.3109/08916939608995338
26. Guo J, Wang Y, Jaume JC, Rapoport B, McLachlan SM. Rarity of autoantibodies to a major autoantigen, thyroid peroxidase, that interact with denatured antigen or with epitopes outside the immunodominant region. Clin Exp Immunol (1999) 117:19–29. doi:10.1046/j.1365-2249.1999.00934.x
27. Latrofa F, Phillips M, Rapoport B, McLachlan SM. Human monoclonal thyroglobulin autoantibodies: epitopes and immunoglobulin genes. J Clin Endocrinol Metab (2004) 89(10):5116–23. doi:10.1210/jc.2003-032173
28. Malthiery Y, Henry M, Zanelli E. Epitope mapping of human thyroglobulin reveals a central immunodominant region. FEBS Lett (1991) 279(2):190–2. doi:10.1016/0014-5793(91)80146-T
29. Gora M, Gardas A, Wiktorowicz W, Hobby P, Watson PF, Weetman AP, et al. Evaluation of conformational epitopes on thyroid peroxidase by antipeptide antibody binding and mutagenesis. Clin Exp Immunol (2004) 136(1):137–44. doi:10.1111/j.1365-2249.2004.02422.x
30. Jaume JC, Burek CL, Hoffman WH, Rose N, McLachlan SM, Rapoport B. Thyroid peroxidase autoantibody epitopic ‘fingerprints’ in juvenile Hashimoto’s thyroiditis: evidence for conservation over time and in families. Clin Exp Immunol (1996) 104:115–23. doi:10.1046/j.1365-2249.1996.d01-659.x
31. Chazenbalk GD, Portolano S, Russo D, Hutchison JS, Rapoport B, McLachlan SM. Human organ-specific autoimmune disease: molecular cloning and expression of an autoantibody gene repertoire for a major autoantigen reveals an antigenic dominant region and restricted immunoglobulin gene usage in the target organ. J Clin Invest (1993) 92:62–74. doi:10.1172/JCI116600
32. Czarnocka B, Janota-Bzowski M, McIntosh RS, Asghar MS, Watson PF, Kemp EH, et al. Immunoglobulin G kappa anti-thyroid peroxidase antibodies in Hashimoto’s thyroiditis: epitope mapping analysis. J Clin Endocrinol Metab (1997) 82:2639–44. doi:10.1210/jcem.82.8.4124
33. Bresson D, Cerutti M, Devauchelle G, Pugniere M, Roquet F, Bes C, et al. Localization of the discontinuous immunodominant region recognized by human anti-thyroperoxidase autoantibodies in autoimmune thyroid diseases. J Biol Chem (2003) 278(11):9560–9. doi:10.1074/jbc.M211930200
34. Jaume JC, Costante G, Nishikawa T, Phillips DIW, Rapoport B, McLachlan SM. Thyroid peroxidase autoantibody fingerprints in hypothyroid and euthyroid individuals. I. Cross-sectional study in elderly women. J Clin Endocrinol Metab (1995) 80:994–9. doi:10.1210/jc.80.3.994
35. Jaume JC, Parkes AB, Lazarus JH, Hall R, Costante G, McLachlan SM, et al. Thyroid peroxidase autoantibody fingerprints. II. A longitudinal study in postpartum thyroiditis. J Clin Endocrinol Metab (1995) 80:1000–5. doi:10.1210/jc.80.3.1000
36. Jaume JC, Guo J, Pauls DL, Zakarija M, McKenzie JM, Egeland JA, et al. Evidence for genetic transmission of thyroid peroxidase autoantibody epitopic “fingerprints”. J Clin Endocrinol Metab (1999) 84(4):1424–31. doi:10.1210/jc.84.4.1424
37. Brix TH, Hegedus L, Gardas A, Banga JP, Nielsen CH. Monozygotic twin pairs discordant for Hashimoto’s thyroiditis share a high proportion of thyroid peroxidase autoantibodies to the immunodominant region A. Further evidence for genetic transmission of epitopic “fingerprints”. Autoimmunity (2011) 44(3):188–94. doi:10.3109/08916934.2010.518575
38. Henry M, Zanelli E, Piechaczyk M, Pau B, Malthiery Y. A major human thyroglobulin epitope defined with monoclonal antibodies is mainly recognized by human autoantibodies. Eur J Immunol (1992) 22(2):315–9. doi:10.1002/eji.1830220205
39. Okosieme OE, Premawardhana LD, Jayasinghe A, Kaluarachi WN, Parkes AB, Smyth PP, et al. Thyroglobulin autoantibodies in iodized subjects: relationship between epitope specificities and longitudinal antibody activity. Thyroid (2005) 15(9):1067–72. doi:10.1089/thy.2005.15.1067
40. Estienne V, McIntosh RS, Ruf J, Asghar MS, Watson PF, Carayon P, et al. Comparative mapping of cloned human and murine antithyroglobulin antibodies: recognition by human antibodies of an immunodominant region. Thyroid (1998) 8(8):643–6. doi:10.1089/thy.1998.8.643
41. Okosieme OE, Premawardhana LD, Jayasinghe A, de Silva DG, Smyth PP, Parkes AB, et al. Thyroglobulin epitope recognition in a post iodine-supplemented Sri Lankan population. Clin Endocrinol (Oxf) (2003) 59(2):190–7. doi:10.1046/j.1365-2265.2003.01819.x
42. Okosieme OE, Evans C, Moss L, Parkes AB, Premawardhana LD, Lazarus JH. Thyroglobulin antibodies in serum of patients with differentiated thyroid cancer: relationship between epitope specificities and thyroglobulin recovery. Clin Chem (2005) 51(4):729–34. doi:10.1373/clinchem.2004.044511
43. Latrofa F, Ricci D, Montanelli L, Altea MA, Pucci A, Pinchera A, et al. Thyroglobulin autoantibodies of patients with subacute thyroiditis are restricted to a major B cell epitope. J Endocrinol Invest (2012) 35(8):712–4. doi:10.1007/BF03345804
44. Latrofa F, Ricci D, Montanelli L, Piaggi P, Mazzi B, Bianchi F, et al. Thyroglobulin autoantibodies switch to immunoglobulin (Ig)G1 and IgG3 subclasses and preserve their restricted epitope pattern after 131I treatment for Graves’ hyperthyroidism: the activity of autoimmune disease influences subclass distribution but not epitope pattern of autoantibodies. Clin Exp Immunol (2014) 178(3):438–46. doi:10.1111/cei.12438
45. Rapoport B, Banuelos B, Aliesky HA, Hartwig Trier N, McLachlan SM. Critical differences between induced and spontaneous mouse models of Graves’ disease with implications for antigen-specific immunotherapy in humans. J Immunol (2016) 197:4560–8. doi:10.4049/jimmunol.1601393
46. Jaume JC, Guo J, Wang Y, Rapoport B, McLachlan SM. Cellular thyroid peroxidase (TPO), unlike purified TPO and adjuvant, induces antibodies in mice that resemble autoantibodies in human autoimmune thyroid disease. J Clin Endocrinol Metab (1999) 84(5):1651–7. doi:10.1210/jcem.84.5.5666
47. Nakajima Y, Howells RD, Pegg C, Davies Jones E, Rees Smith B. Structure activity analysis of microsomal antigen/thyroid peroxidase. Mol Cell Endocrinol (1987) 53:15–23. doi:10.1016/0303-72078790187-0
48. Gardas A, Domek H. The effect of sulphydryl reagents on the human thyroid microsomal antigen. J Endocrinol Invest (1988) 11:385–8. doi:10.1007/BF03349061
49. Hamada N, Jaeduck N, Portmann L, Ito K, DeGroot LJ. Antibodies against denatured and reduced thyroid microsomal antigen in autoimmune thyroid disease. J Clin Endocrinol Metab (1987) 64:230–8. doi:10.1210/jcem-64-2-230
50. Bermann M, Magee M, Koenig RJ, Kaplan MM, Maastricht J, Johnson J, et al. Differential autoantibody responses to thyroid peroxidase in patients with Graves’ disease and Hashimoto’s thyroiditis. J Clin Endocrinol Metab (1993) 77:1098–101. doi:10.1210/jcem.77.4.8408460
51. Finke R, Seto P, Ruf J, Carayon P, Rapoport B. Determination at the molecular level of a B-cell epitope on thyroid peroxidase likely to be associated with autoimmune thyroid disease. J Clin Endocrinol Metab (1991) 73:919–21. doi:10.1210/jcem-73-4-919
52. Libert F, Ludgate M, Dinsart C, Vassart G. Thyroperoxidase, but not the thyrotropin receptor, contains sequential epitopes recognized by autoantibodies in recombinant peptides expressed in the pUEX vector. J Clin Endocrinol Metab (1991) 73:857–60. doi:10.1210/jcem-73-4-857
53. Banga JP, Barnett PS, Ewins DL, Page M, McGregor AM. Mapping of autoantigenic epitopes on recombinant thyroid peroxidase fragments using the polymerase chain reaction. Autoimmunity (1990) 6:257–68. doi:10.3109/08916939008998418
54. Maastricht J, Koenig RJ, Kaplan MM, Arscott P, Thompson N, Baker JR Jr. Identification of localized autoantibody epitopes in thyroid peroxidase. J Clin Endocrinol Metab (1992) 75:121–6. doi:10.1210/jc.75.1.121
55. Zanelli E, Henry M, Malthiery Y. Use of recombinant epitopes to study the heterogeneous nature of the autoantibodies against thyroid peroxidase in autoimmune thyroid disease. Clin Exp Immunol (1992) 87:80–6. doi:10.1111/j.1365-2249.1992.tb06417.x
56. Arscott PL, Koenig RJ, Kaplan MM, Glick GD, Baker JR Jr. Unique autoantibody epitopes in an immunodominant region of thyroid peroxidase. J Biol Chem (1996) 271:4966–73. doi:10.1074/jbc.271.9.4966
57. Shimojo N, Saito K, Kohno Y, Sasaki N, Tarutani O, Nakajima H. Antigenic determinants on thyroglobulin: comparison of the reactivities of different thyroglobulin preparations with serum antibodies and T cells of patients with chronic thyroiditis. J Clin Endocrinol Metab (1988) 66(4):689–95. doi:10.1210/jcem-66-4-689
58. Caturegli P, Mariotti S, Kuppers RC, Burek CL, Pinchera A, Rose NR. Epitopes on thyroglobulin: a study of patients with thyroid disease. Autoimmunity (1994) 18:41–9. doi:10.3109/08916939409014678
59. McLachlan SM, Rapoport B. Thyrotropin-blocking autoantibodies and thyroid-stimulating autoantibodies: potential mechanisms involved in the pendulum swinging from hypothyroidism to hyperthyroidism or vice versa. Thyroid (2013) 23(1):14–24. doi:10.1089/thy.2012.0374
60. Evans M, Sanders J, Tagami T, Sanders P, Young S, Roberts E, et al. Monoclonal autoantibodies to the TSH receptor, one with stimulating activity and one with blocking activity, obtained from the same blood sample. Clin Endocrinol (Oxf) (2010) 73(3):404–12. doi:10.1111/j.1365-2265.2010.03831.x
61. Ludwig RJ, Vanhoorelbeke K, Leypoldt F, Kaya Z, Bieber K, McLachlan SM, et al. Mechanisms of autoantibody-induced pathology. Front Immunol (2017) 8:603. doi:10.3389/fimmu.2017.00603
62. Luo J, Taylor P, Losen M, De Baets MH, Shelton GD, Lindstrom J. Main immunogenic region structure promotes binding of conformation-dependent myasthenia gravis autoantibodies, nicotinic acetylcholine receptor conformation maturation, and agonist sensitivity. J Neurosci (2009) 29(44):13898–908. doi:10.1523/JNEUROSCI.2833-09.2009
63. Sekiguchi M, Futei Y, Fujii Y, Iwasaki T, Nishikawa T, Amagai M. Dominant autoimmune epitopes recognized by pemphigus antibodies map to the N-terminal adhesive region of desmogleins. J Immunol (2001) 167(9):5439–48. doi:10.4049/jimmunol.167.9.5439
64. Misharin AV, Nagayama Y, Aliesky H, Mizutori Y, Rapoport B, McLachlan SM. Attenuation of induced hyperthyroidism in mice by pretreatment with thyrotropin receptor protein: deviation of thyroid-stimulating antibody to non-functional antibodies. Endocrinology (2009) 150(8):3944–52. doi:10.1210/en.2009-0181
65. Rapoport B, Aliesky HA, Banuelos B, Chen CR, McLachlan SM. A unique mouse strain that develops spontaneous, iodine-accelerated, pathogenic antibodies to the human thyrotrophin receptor. J Immunol (2015) 194(9):4154–61. doi:10.4049/jimmunol.1500126
66. Zikherman J, Parameswaran R, Weiss A. Endogenous antigen tunes the responsiveness of naive B cells but not T cells. Nature (2012) 489(7414):160–4. doi:10.1038/nature11311
67. Akkaraju S, Canaan K, Goodnow CC. Self-reactive B cells are not eliminated or inactivated by autoantigen expressed on thyroid epithelial cells. J Exp Med (1997) 186(12):2005–12. doi:10.1084/jem.186.12.2005
68. Anderson DE, Carlos MP, Nguyen L, Torres JV. Overcoming original (antigenic) sin. Clin Immunol (2001) 101(2):152–7. doi:10.1006/clim.2001.5114
69. Garcia-Quintanilla A. Overcoming viral escape with vaccines that generate and display antigen diversity in vivo. Virol J (2007) 4:125. doi:10.1186/1743-422X-4-125
70. Liu XS, Dyer J, Leggatt GR, Fernando GJ, Zhong J, Thomas R, et al. Overcoming original antigenic sin to generate new CD8 T cell IFN-gamma responses in an antigen-experienced host. J Immunol (2006) 177(5):2873–9. doi:10.4049/jimmunol.177.5.2873
71. Kim JH, Davis WG, Sambhara S, Jacob J. Strategies to alleviate original antigenic sin responses to influenza viruses. Proc Natl Acad Sci U S A (2012) 109(34):13751–6. doi:10.1073/pnas.0912458109
72. Ndifon W. A simple mechanistic explanation for original antigenic sin and its alleviation by adjuvants. J R Soc Interface (2015) 12(112):ii:20150627. doi:10.1098/rsif.2015.0627
73. Rapoport B, McLachlan SM. TSH receptor cleavage into subunits and shedding of the A-subunit; a molecular and clinical perspective. Endocr Rev (2016) 37:114–34. doi:10.1210/er.2015-1098
74. Saboori AM, Rose NR, Bresler HS, Vladut-Talor M, Burek CL. Iodination of human thyroglobulin (Tg) alters its immunoreactivity. I. Iodination alters multiple epitopes of human Tg. Clin Exp Immunol (1998) 113(2):297–302. doi:10.1046/j.1365-2249.1998.00643.x
75. Henry M, Malthiery Y, Zanelli E, Charvet B. Epitope mapping of human thyroglobulin. Heterogeneous recognition by thyroid pathologic sera. J Immunol (1990) 145(11):3692–8.
76. Saboori AM, Rose NR, Yuhasz SC, Amzel LM, Burek CL. Peptides of human thyroglobulin reactive with sera of patients with autoimmune thyroid disease. J Immunol (1999) 163(11):6244–50.
77. Duthoit C, Estienne V, Delom F, Durand-Gorde JM, Mallet B, Carayon P, et al. Production of immunoreactive thyroglobulin C-terminal fragments during thyroid hormone synthesis. Endocrinology (2000) 141(7):2518–25. doi:10.1210/endo.141.7.7573
78. Guo J, Yan X-M, McLachlan SM, Rapoport B. Search for the autoantibody immunodominant region on thyroid peroxidase: epitopic footprinting with a human monoclonal autoantibody locates a facet on the native antigen containing a highly conformational epitope. J Immunol (2001) 166:1327–33. doi:10.4049/jimmunol.166.2.1327
79. Guo J, McLachlan SM, Rapoport B. Localization of the thyroid peroxidase autoantibody immunodominant region to a junctional region containing portions of the domains homologous to complement control protein and myeloperoxidase. J Biol Chem (2002) 277(43):40189–95. doi:10.1074/jbc.M205524200
80. Bresson D, Pugniere M, Roquet F, Rebuffat SA, Guyen B, Cerutti M, et al. Directed mutagenesis in region 713-720 of human thyroperoxidase assigns 713KFPED717 residues as being involved in the B domain of the discontinuous immunodominant region recognized by human autoantibodies. J Biol Chem (2004) 279(37):39058–67. doi:10.1074/jbc.M403897200
81. Le SN, Porebski BT, McCoey J, Fodor J, Riley B, Godlewska M, et al. Modelling of thyroid peroxidase reveals insights into its enzyme function and autoantigenicity. PLoS One (2015) 10(12):e0142615. doi:10.1371/journal.pone.0142615
82. Nagayama Y, Wadsworth HL, Russo D, Chazenbalk GD, Rapoport B. Binding domains of stimulatory and inhibitory thyrotropin (TSH) receptor autoantibodies determined with chimeric TSH- lutropin/chorionic gonadotropin receptors. J Clin Invest (1991) 88:336–40. doi:10.1172/JCI115297
83. Sanders J, Chirgadze DY, Sanders P, Baker S, Sullivan A, Bhardwaja A, et al. Crystal structure of the TSH receptor in complex with a thyroid-stimulating autoantibody. Thyroid (2007) 17(5):395–410. doi:10.1089/thy.2007.0041
84. Sanders P, Young S, Sanders J, Kabelis K, Baker S, Sullivan A, et al. Crystal structure of the TSH receptor (TSHR) bound to a blocking-type TSHR autoantibody. J Mol Endocrinol (2011) 46(2):81–99. doi:10.1530/JME-10-0127
85. Thrasyvoulides A, Lymberi P. Evidence for intramolecular B-cell epitope spreading during experimental immunization with an immunogenic thyroglobulin peptide. Clin Exp Immunol (2003) 132(3):401–7. doi:10.1046/j.1365-2249.2003.02162.x
86. Thrasyvoulides A, Lymberi P. Antibodies cross-reacting with thyroglobulin and thyroid peroxidase are induced by immunization of rabbits with an immunogenic thyroglobulin 20mer peptide. Clin Exp Immunol (2004) 138(3):423–9. doi:10.1111/j.1365-2249.2004.02657.x
87. Estienne V, Duthoit C, Costanzo VD, Lejeune PJ, Rotondi M, Kornfeld S, et al. Multicenter study on TGPO autoantibody prevalence in various thyroid and non-thyroid diseases; relationships with thyroglobulin and thyroperoxidase autoantibody parameters. Eur J Endocrinol (1999) 141(6):563–9. doi:10.1530/eje.0.1410563
88. Latrofa F, Pichurin P, Guo J, Rapoport B, McLachlan SM. Thyroglobulin-thyroperoxidase autoantibodies are polyreactive, not bispecific: analysis using human monoclonal autoantibodies. J Clin Endocrinol Metab (2003) 88(1):371–8. doi:10.1210/jc.2002-021073
89. Flynn JC, Rao PV, Gora M, Alsharabi G, Wei W, Giraldo AA, et al. Graves’ hyperthyroidism and thyroiditis in HLA-DRB1*0301 (DR3) transgenic mice after immunization with thyrotropin receptor DNA. Clin Exp Immunol (2004) 135(1):35–40. doi:10.1111/j.1365-2249.2004.02333.x
90. McLachlan SM, Nagayama Y, Pichurin PN, Mizutori Y, Chen CR, Misharin A, et al. The link between Graves’ disease and Hashimoto’s thyroiditis: a role for regulatory T cells. Endocrinology (2007) 148(12):5724–33. doi:10.1210/en.2007-1024
91. Chen CR, Hamidi S, Braley-Mullen H, Nagayama Y, Bresee C, Aliesky HA, et al. Antibodies to thyroid peroxidase arise spontaneously with age in NOD.H-2h4 mice and appear after thyroglobulin antibodies. Endocrinology (2010) 151(9):4583–93. doi:10.1210/en.2010-0321
92. Hutfless S, Matos P, Talor MV, Caturegli P, Rose NR. Significance of prediagnostic thyroid antibodies in women with autoimmune thyroid disease. J Clin Endocrinol Metab (2011) 96(9):E1466–71. doi:10.1210/jc.2011-0228
93. Tamai H, Kasagi K, Takaichi Y, Takamatsu J, Komaki G, Matsubayashi S, et al. Development of spontaneous hypothyroidism in patients with Graves’ disease treated with antithyroidal drugs: clinical, immunological, and histological findings in 26 patients. J Clin Endocrinol Metab (1989) 69(1):49–53. doi:10.1210/jcem-69-1-49
94. Shigemasa C, Mitani Y, Taniguch T, Adachi T, Ueta Y, Urabe K, et al. Three patients who spontaneously developed persistent hypothyroidism during or following treatment with antithyroid drugs for Graves’ hyperthyroidism. Arch Int Med (1990) 150:1105–9. doi:10.1001/archinte.150.5.1105
95. Takasu N, Yamada T, Sato A, Nakagawa M, Komiya I, Nagasawa Y, et al. Graves’ disease following hypothyroidism due to Hashimoto’s disease: studies of eight cases. Clin Endocrinol (Oxf) (1990) 33(6):687–98. doi:10.1111/j.1365-2265.1990.tb03906.x
96. Kamath C, Young S, Kabelis K, Sanders J, Adlan MA, Furmaniak J, et al. Thyrotrophin receptor antibody characteristics in a woman with long-standing Hashimoto’s who developed Graves’ disease and pretibial myxoedema. Clin Endocrinol (Oxf) (2012) 77(3):465–70. doi:10.1111/j.1365-2265.2012.04397.x
97. Latrofa F, Fiore E, Rago T, Antonangeli L, Montanelli L, Ricci D, et al. Iodine contributes to thyroid autoimmunity in humans by unmasking a cryptic epitope on thyroglobulin. J Clin Endocrinol Metab (2013) 98(11):E1768–74. doi:10.1210/jc.2013-2912
98. Jacob JB, Kong YC, Nalbantoglu I, Snower DP, Wei WZ. Tumor regression following DNA vaccination and regulatory T cell depletion in neu transgenic mice leads to an increased risk for autoimmunity. J Immunol (2009) 182(9):5873–81. doi:10.4049/jimmunol.0804074
99. Rasooly L, Burek CL, Rose NR. Iodine-induced autoimmune thyroiditis in NOD-H2h4 mice. Clin Immunol Immunopathol (1996) 81:287–92. doi:10.1006/clin.1996.0191
100. Braley-Mullen H, Sharp GC, Medling B, Tang H. Spontaneous autoimmune thyroiditis in NOD.H-2h4 mice. J Autoimmun (1999) 12(3):157–65. doi:10.1006/jaut.1999.0272
101. Hutchings PR, Verma S, Phillips JM, Harach SZ, Howlett S, Cooke A. Both CD4(+) T cells and CD8(+) T cells are required for iodine accelerated thyroiditis in NOD mice. Cell Immunol (1999) 192(2):113–21. doi:10.1006/cimm.1998.1446
102. McLachlan SM, Aliesky H, Banuelos B, Que Hee SS, Rapoport B. Variable effects of dietary selenium in mice that spontaneously develop a spectrum of thyroid autoantibodies. Endocrinology (2017) 158(11):3754–64. doi:10.1210/en.2017-00275
103. Schwarz-Lauer L, Chazenbalk G, McLachlan SM, Ochi Y, Nagayama Y, Rapoport B. Evidence for a simplified view of autoantibody interactions with the TSH receptor. Thyroid (2002) 12(2):115–20. doi:10.1089/105072502753522347
104. Chazenbalk GD, Pichurin P, Chen CR, Latrofa F, Johnstone AP, McLachlan SM, et al. Thyroid-stimulating autoantibodies in Graves disease preferentially recognize the free A subunit, not the thyrotropin holoreceptor. J Clin Invest (2002) 110(2):209–17. doi:10.1172/JCI0215745
105. Furmaniak J, Sanders J, Rees SB. Blocking type TSH receptor antibodies. Auto Immun Highlights (2013) 4(1):11–26. doi:10.1007/s13317-012-0028-1
106. McLachlan SM, Aliesky HA, Banuelos B, Lesage S, Collin R, Rapoport B. High-level intrathymic thyrotrophin receptor expression in thyroiditis-prone mice protects against the spontaneous generation of pathogenic thyrotrophin receptor autoantibodies. Clin Exp Immunol (2017) 188(2):243–53. doi:10.1111/cei.12928
107. Misharin AV, Rapoport B, McLachlan SM. Thyroid antigens, not central tolerance, control responses to immunization in BALB/c versus C57BL/6 mice. Thyroid (2009) 19(5):503–9. doi:10.1089/thy.2008.0420
Keywords: thyroid autoantibodies, intermolecular and intramolecular epitope spreading, immunodominant region, original antigenic sin, thyroglobulin, thyroid peroxidase, thyrotropin receptor
Citation: McLachlan SM and Rapoport B (2017) Thyroid Autoantibodies Display both “Original Antigenic Sin” and Epitope Spreading. Front. Immunol. 8:1845. doi: 10.3389/fimmu.2017.01845
Received: 10 October 2017; Accepted: 06 December 2017;
Published: 20 December 2017
Edited by:
Ralf J. Ludwig, University of Lübeck, GermanyReviewed by:
Yaron Tomer, Albert Einstein College of Medicine, United StatesYi-chi Kong, Wayne State University School of Medicine, United States
Copyright: © 2017 McLachlan and Rapoport. This is an open-access article distributed under the terms of the Creative Commons Attribution License (CC BY). The use, distribution or reproduction in other forums is permitted, provided the original author(s) or licensor are credited and that the original publication in this journal is cited, in accordance with accepted academic practice. No use, distribution or reproduction is permitted which does not comply with these terms.
*Correspondence: Sandra M. McLachlan, bWNsYWNobGFuc0Bjc2hzLm9yZw==