- 1Division of Infectious Disease, Wadsworth Center, New York State Department of Health, Albany, NY, United States
- 2Latvian Biomedical Research and Study Centre, Riga, Latvia
- 3Department of Veterinary Medicine, Virginia–Maryland Regional College of Veterinary Medicine, University of Maryland, College Park, College Park, MD, United States
- 4Institute of Medical Microbiology and Infection Control, University Hospital of Frankfurt, Frankfurt am Main, Germany
- 5Department of Biomedical Science, State University of New York at Albany, Albany, NY, United States
- 6Faculty of Biology, University of Latvia, Riga, Latvia
The spirochete Borrelia burgdorferi is the causative agent of Lyme disease, the most common tick-borne disease in the US and Europe. No potent human vaccine is currently available. The innate immune complement system is vital to host defense against pathogens, as complement activation on the surface of spirochetes results in bacterial killing. Complement system is inhibited by the complement regulator factor H (FH). To escape killing, B. burgdorferi produces an outer surface protein CspZ that binds FH to inhibit complement activation on the cell surface. Immunization with CspZ alone does not protect mice from infection, which we speculate is because FH-binding cloaks potentially protective epitopes. We modified CspZ by conjugating to virus-like particles (VLP-CspZ) and eliminating FH binding (modified VLP-CspZ) to increase immunogenicity. We observed greater bactericidal antibody titers in mice vaccinated with modified VLP-CspZ: A serum dilution of 1:395 (modified VLP-CspZ) vs 1:143 (VLP-CspZ) yielded 50% borreliacidal activity. Immunizing mice with modified VLP-CspZ cleared spirochete infection, as did passive transfer of elicited antibodies. This work developed a novel Lyme disease vaccine candidate by conjugating CspZ to VLP and eliminating FH-binding ability. Such a strategy of conjugating an antigen to a VLP and eliminating binding to the target ligand can serve as a general model for developing vaccines against other bacterial infectious agents.
Introduction
Lyme disease is the most common vector-borne illness in North America and Europe (1). However, no vaccine is currently available for humans. In North America, Lyme disease is caused by the spirochete Borrelia burgdorferi sensu stricto, which is transmitted via Ixodes ticks (1). Upon tick feeding, spirochetes migrate from the ticks to the vertebrate hosts and infect the skin at the biting site, often resulting in an inflammatory skin lesion, called erythema migrans (2). If untreated, spirochetes disseminate via bloodstream to organs, causing disease manifestations including arthritis, carditis, and neuroborreliosis (1). To disseminate to distal tissues, B. burgdorferi needs to evade the complement system, an important host innate immune defense mechanism in the blood of vertebrate animals (3). Activation of the complement system results in the formation of C3 convertases, leading to the release of pro-inflammatory peptides, and pathogen opsonization and lysis (3). To avoid self-damage in the absence of pathogens, vertebrate animals produce complement inhibitors, such as Factor H (FH) and FH-like protein 1 (FHL-1, the spliced form of FH). FH and FHL-1 bind to C3b, a component of C3 convertases, which recruits complement protein factor I to degrade C3b and inhibit the formation of these convertases and inactivates the complement system (3).
Borrelia burgdorferi produces at least five distinct Complement Regulator Acquiring Surface Proteins including CspZ (CRASP-2). CspZ binds to human and mouse FH/FHL-1 to confer serum resistance in a gain-of-function B. burgdorferi by inhibiting complement activation on the spirochete surface (4–6). Whereas a cspZ deletion mutant of B. burgdorferi colonizes tissues at similar levels as its parental wild-type strain (7), mutant strains with transposon insertions in cspZ, when co-infected with a library of other transposon-inserted mutants, display reduced colonization of mouse tissues (8). These findings suggest that CspZ contributes a fitness advantage for spirochetes during infection. cspZ expression is detectable when spirochetes are in mammalian hosts and in vitro cultivation (7, 9), and inoculating mice with CspZ triggers antibody response against this protein (7, 10, 11). Although not all isolates from Lyme disease Borrelia species encode cspZ, the isolates from B. burgdorferi (North American species of Lyme disease spirochetes) all carry this gene (11). The cspZ alleles among these B. burgdorferi isolates were grouped into three types and share more than 90% of sequence identity (11). These observations suggest that CspZ may have vaccinogenic potential by inducing antibody-mediated bactericidal activity against B. burgdorferi. However, immunization with CspZ does not protect mice from infection (7, 11), raising a possibility that CspZ as a vaccine does not induce antibody titers robust enough to kill B. burgdorferi.
One strategy to enhance antibody titers and the ability of antibodies in eliminating pathogens is conjugating antigens to virus-like particles (VLPs) (12–14). Though no commercially available vaccines have yet been generated by VLP conjugation, this strategy has been tested in different animal models and shown to trigger greater levels of immune responses [e.g., Ref. (15–18)]. Another strategy is to mutate the immunogens to make them incapable of binding to their binding partners so the epitopes on the binding sites can be exposed (19). We thus modified CspZ by conjugating it to bacteriophage Qβ-derived VLP, combined with eliminating its FH-binding activity to test whether this modified CspZ could be an effective vaccine of Lyme disease. In this study, we demonstrated that vaccination with this modified CspZ induces antibodies that more efficiently eradicate spirochetes in vitro and prevents Lyme-associated arthritis and tissue colonization in vivo. This proof-of-concept study illustrates novel strategies to generate a potent CspZ-based Lyme disease vaccine. This technique of combining VLP conjugation and eliminating binding to the target ligand can be applied to generate effective vaccines against other infectious agents.
Materials and Methods
Ethics Statement
All mouse experiments were performed in strict accordance with all provisions of the Animal Welfare Act, the Guide for the Care and Use of Laboratory Animals, and the PHS Policy on Humane Care and Use of Laboratory Animals. The protocol (Docket Number 16-451) was approved by the Institutional Animal Care and Use Agency of Wadsworth Center, New York State Department of Health. All efforts were made to minimize animal suffering.
Mouse and Bacterial Strains
Three-week-old male C3H/HeN and Swiss Webster mice were purchased from Charles River (Wilmington, MA, USA) and Taconic (Hudson, NY, USA), respectively. The C3H/HeN mouse strain was utilized as this strain develops manifestations (e.g., arthritis) during B. burgdorferi infection and are thus commonly used to test the efficacy of Lyme disease vaccines (20, 21). The B. burgdorferi strain B31-A3 used in this study is a clonal isolate of B31 (22) and was grown at 33°C in BSK II complete medium (23). Cultures were tested with PCR to ensure a full plasmid profile prior to use, as previously described (24). Escherichia coli strains DH5α, BL21(DE3), and derivatives were grown at 37°C in Luria-Bertani (BD Bioscience, Franklin Lakes, NJ, USA) broth or agar, supplemented with kanamycin (25 µg/mL), ampicillin (100 µg/mL), or no antibiotics when appropriate.
Generation of VLP-CspZ Proteins
To produce recombinant glutathione-S-transferase (GST)-tagged CspZ proteins, the plasmid pGEX-6P1 encoding the open reading frames lacking the putative signal sequences of bbh06 (cspZ) from B. burgdorferi strains B31 (residue 21–236 of CspZ) or an altered open reading frame encoding CspZ-Y207A/Y211A (residue 21–236 of CspZ with tyrosine-207 and -211 replaced by alanine) generated previously (4, 6) was transformed into E. coli strain BL21(DE3). The GST-tagged CspZ or CspZ-Y207A/Y211A were produced and purified by GST affinity chromatography as described previously (4, 6) according to the manufacturer’s instructions (GE Healthcare, Pittsburgh, PA, USA). To produce recombinant CspZ proteins without affinity tags for VLP conjugation and vaccination, an cystein has been added to C-termini of both CspZ and CspZ-Y207A/Y211A for coupling these proteins to VLPs as described (25, 26). The genes encoding these proteins were cloned into the pETm_11 expression vector (EMBL) encoding an N-terminal 6xHis-tag followed by a TEV protease cleavage site, resulting in an amino acid sequence of MHHHHHHENLYFQS-CspZ-GSGC. E. coli XL1-Blue cells were transformed with the plasmids encoding cspZ or cspZ-Y207A/Y211A. The transformations were verified by sequencing the plasmid DNA extracted from isolated colonies. E. coli BL21(DE3) cells were transformed with these plasmids and grown in modified 2×TY medium at 37°C until mid-log phase. The cultures were then induced to produce CspZ with 0.2 mm isopropyl thio-β-d-galactoside, and grown overnight at 20°C. The cells were lysed by sonication. After removing the debris, the supernatant was loaded onto a HisTrap FF column (GE Healthcare, Chicago, IL, USA) and eluted with 300 mm imidazole at pH 7.5. The 6×His tag was removed by incubation with TEV protease at 4°C overnight. Imidazole was removed by dialyzing the proteins in PBS buffer. The protease, the digested 6×His tag, and un-cleaved proteins were removed using an additional round of HisTrap FF column purification. The purified protein fraction was concentrated using an Amicon centrifugal filter unit (Millipore, Billerica, MA, USA). The purity of the recombinant proteins was evaluated by SDS-PAGE. The bacteriophage Qβ-derived VLPs were generated as previously described (27). Purified CspZ proteins were chemically conjugated to VLPs with SMPH (Succinimidyl-6-[(β-maleimidopropionamido) hexanoate]) following the manufacturer’s protocol (ThermoFisher, Waltham, MA, USA). The unbounded protein was removed using a Superdex200 size exclusion column (GE Healthcare).
FH Binding Assays by ELISA
Quantitative ELISA for mouse FH binding by CspZ proteins was performed similarly to that previously described (28). Basically, 1 µg of BSA (negative control) or mouse FH (MyBiosource, San Diego, CA, USA) was coated onto microtiter plate wells. One hundred microliters of increasing concentrations (0.03125, 0.0625, 0.125, 0.25, 0.5, 1, 2 µM) of GST (negative control) or a GST tagged wild-type or mutant CspZ protein, including CspZ or CspZ-Y207A/Y211A were then added to the wells. To detect the binding of GST-tagged proteins, mouse anti-GST tag (Sigma-Aldrich, St. Louis, MO, USA; 1:200) and HRP-conjugated goat anti-mouse IgG (Promega, Madison, WI, USA; 1:1,000×) were used as primary and secondary antibodies. The plates were washed three times with PBST (0.05% Tween 20 in PBS), and 100 µL of tetramethyl benzidine (TMB) solution (ThermoFisher, Waltham, MA, USA) were added to each well and incubated for 5 min. The reaction was stopped by adding 100 µL of 0.5% hydro sulfuric acid to each well. Plates were read at 405 nm using a Tecan Sunrise Microplate reader (Tecan, Morrisville, NC, USA).
Mouse Immunization
Twenty-five micrograms of VLP, CspZ, VLP-CspZ, or VLP-CspZ-Y207A/Y211A were thoroughly mixed with 50 µL TiterMax Gold adjuvant (Norcross, GA, USA), which was utilized because it has been reported to induce higher and longer lasting titers with fewer injections than the other adjuvants (29). This vaccination was then inoculated into C3H/HeN mice intraperitoneally. Mice inoculated with 100 µL PBS were included as a negative control. Mice received boosters of the same composition at 14 and 28 days post immunization, for a total of three immunizations over 6 weeks (Figure S1A in Supplementary Material).
Quantification of Anti-CspZ Titers with ELISA
Forty-two days post immunization, 100 µL blood was collected from 10 mice via submandibular bleeding to isolate serum. The sera were used to determine the titers of immunoglobulin M or G against CspZ using kinetic ELISA as previously described (30). In brief, microtiter plate wells were coated with 1 µg of recombinant CspZ. After blocking with 5% BSA (Sigma-Aldrich) in phosphate-buffered saline, 50 µL of mouse serum diluted 1:100, 1:300, 1:900, 1:1,800, 1:3,600, 1:7,200, 1:144,000, or 1: 288,000 was added to each well. HRP-conjugated goat anti-mouse IgM or IgG (1:20,000; Bethyl, Montgomery, TX, USA) and 50 µL of tetramethyl benzidine (TMB) solution (ThermoFisher, Waltham, MA, USA) were subsequently added into the wells, and the binding was detected at 620 nm for 10 cycles of 60 s kinetic intervals with 10 s shaking duration in a Sunrise absorbance ELISA plate reader (Tecan, Männedorf, Switzerland). The greatest maximum slope of optical density/minute per sample was multiplied by the respective serum dilution factor to indicate the antibody titers (arbitrary Unit).
B. burgdorferi Bactericidal Activity of Serum from Immunized Mice
Forty-two days post immunization, 100 µL blood was collected from five mice via submandibular bleeding to isolate serum. The mouse sera were used to determine the bactericidal activity against B. burgdorferi with serum bactericidal assays modified from previous studies (31, 32). Prior to determining the bactericidal activity, these mouse sera were heat treated at 56°C for 30 min to inactivate the complement system in these sera. Then, 50 µL of diluted mouse serum (1:20, 1:40, 1:80, 1:160, 1:320, 1:640, 1:1,280, and 1:2,560) was mixed with 10 µL of complement preserved guinea pig serum (guinea pig complement, Sigma-Aldrich, # S1639) or heat-inactivated guinea pig serum (negative control) as well as B. burgdorferi strain B31-A3 (5 × 105 cells/mL) in 40 µL of BSK II complete medium and then incubated at 33°C for 24 h. Surviving spirochetes were quantified by directly counting only motile spirochetes using dark-field microscopy. The survival percentage was the proportion of serum-treated to untreated B. burgdorferi. The 50% borreliacidal titer representing the serum dilution rate that effectively killed 50% of spirochetes was calculated using dose–response stimulation fitting in GraphPad Prism 5.04 (GraphPad Software, La Jolla, CA, USA).
Passive Immunization of Mice
Six naive Swiss Webster mice were intraperitoneally inoculated with 100 µL of pooled serum from VLP-, CspZ-, VLP-CspZ-, or VLP-CspZ-Y207A/Y211A-immunized mice (Figure S1B in Supplementary Material). Six mice inoculated with pre-immune serum were included as negative control. They were then challenged subcutaneously with 104 infectious B. burgdorferi strain B31-A3 the next day. Mice were euthanized at 14 days post infection, and the inoculation site of skin, heart, tibiotarsus joints, bladder, and ears were collected and then placed at 33°C in BSK medium supplemented with antimicrobial agents (rifampin at 50 mg/mL, phosphomycin at 200 mg/mL, and amphotericin B at 8 mg/mL). Cultures were checked weekly for 4 weeks using dark-field microscopy to determine whether the live B. burgdorferi was present. A mouse was considered infected when at least one culture was positive.
Active Immunization of Mice and Tibiotarsus Joint Measurement
Forty-two days post immunization, the diameter of both tibiotarsus joints was measured with Digimax calipers (Bel-Art, Wayne. MJ, USA). Mice were then subcutaneously needle-infected with 104 B. burgdorferi strain B31-A3 suspended in 100 µL BSK II incomplete medium (Figure S1C in Supplementary Material). Negative control mice were injected with an equal volume of BSK II incomplete medium. The diameter of both tibiotarsus joints were measured prior to infection and then were re-measured 7 and 14 days post infection, and the diameters from each mouse averaged as Lyme-induced joint swelling is detectable as early as these time points (33).
Histopathology of B. burgdorferi Infected Mice
Three infected mice per vaccination type and three uninfected mice were sacrificed 14 days post infection to assess arthritis and carditis. Thus, tibiotarsus joints were collected for tissue histopathology. Tissues were fixed for 48 h in 10% neutral-buffered formalin, and subsequently decalcified for 1 week in 10% formic acid. Fixed tissues were prepared as slides stained with hematoxylin and eosin (Wadsworth Histopathology Core Facility, NYS Department of Health, Albany, NY, USA). Arthritis was evaluated in a blind fashion as described previously (30).
Quantification of B. burgdorferi Burden in Infected Mouse Tissue with Quantitative PCR (qPCR)
To quantify B. burgdorferi bacterial burden, 10 mice per vaccination type were sacrificed at 28 days post infection, and inoculation site of the skin, knees, and hearts were collected. DNA was purified from tissues using either DNeasy Blood and Tissue Kit (Qiagen, Valencia, CA, USA) or EZ-10 Spin Column Animal Genomic DNA Mini-Prep Kit (Bio Basic, Inc., Markham, ON, Canada). The quantity and quality of DNA were assessed by measuring the concentration of DNA and the ratio of the UV absorption at 280 nm to 260 nm using a Nanodrop 1000 UV/Vis spectrophotometer (Thermo Fisher Scientific, Waltham, MA, USA). qPCR was then performed to quantitate B. burgdorferi burden, as described previously (30). In brief, B. burgdorferi genomic equivalents were calculated using an Applied Biosystems 7500 Real-Time PCR system (Thermo Fisher Scientific, Waltham, MA, USA) in conjunction with PowerUp™ SYBR® Green Master Mix (Thermo Fisher Scientific, Waltham, MA, USA), based on amplification of the B. burgdorferi recA gene using primers BBRecAfp (5′-GTGGATCTATTGTATTAGATGAGGCTCTCG-3′) and BBRecArp (5′-GCCAAAGTTCTGCAACATTAACACCTAAAG-3′). Cycling parameters were 50°C for 2 min, 95°C for 10 min, and 45 cycles of 95°C for 15 s, and 60°C for 1 min. The number of recA copies was calculated by establishing a threshold cycle standard curve of a known number of recA gene extracted from B31-A3, and burdens were normalized to 10 ng of total DNA.
Statistical Analyses
Significant differences between groups were determined with one-tailed Fisher Exact Probability Test or one-way ANOVA and post hoc tests (GraphPad Software, La Jolla, CA, USA). A p-value < 0.05 was used to determine significance.
Results
The Generation and Verification of VLP-Conjugated CspZ Proteins
We re-evaluated the potential of CspZ as a vaccine by utilizing two different strategies: conjugating CspZ with VLP (“VLP-CspZ”) and further modifying CspZ to eliminate its FH-binding activity (“VLP-CspZ-Y207A/Y211A”). The point mutant CspZ-Y207A/Y211A has been shown with no human FH-binding activity (6). Because murine model of Lyme disease infection was used in this study to test the vaccine efficacy of these CspZ-derived proteins, we measured the mouse FH-binding activity of CspZ-Y207A/Y211A by quantitative ELISA. As shown in Figure S2 in Supplementary Material, CspZ binds to mouse FH in a dose dependent manner consistent with a previous finding (5) whereas CspZ-Y207A/Y211A does not bind to mouse FH. In addition, the VLP utilized in this study was derived from the RNA bacteriophage Qβ, which has been used for vaccine development studies in different animal models (15–18). We attached recombinant CspZ proteins to VLPs by adding an engineered C-terminal cysteine to CspZ and linking it to surface-exposed lysine amino groups of VLPs using SMPH (Succinimidyl 6-((beta-maleimidopropionamido)hexanoate)) cross-linker (Figure 1A). The efficiency of coupling was verified by SDS-PAGE. Similar to other VLP-conjugated proteins (25), oligomerized coat protein of VLP was observed on SDS-PAGE (Figure 1B). VLP integrity was maintained as observed under electro-microscopy (Figure 1C).
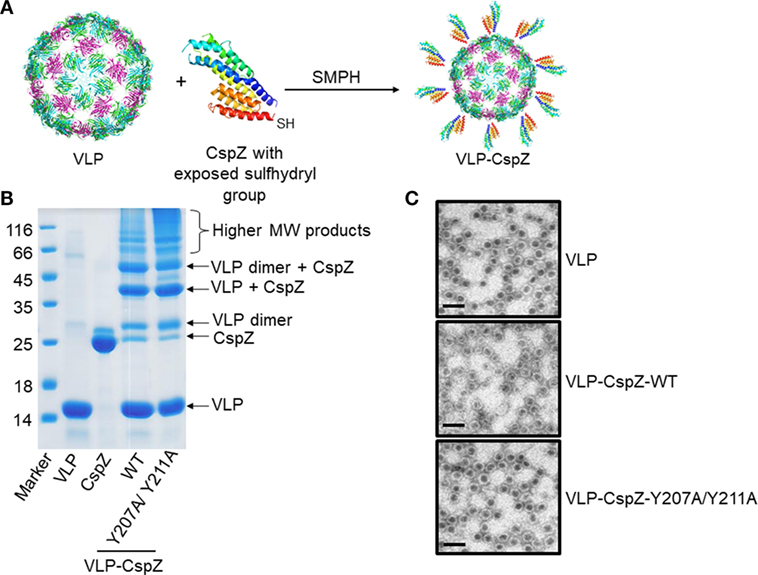
Figure 1. The generation and verification of virus-like particle (VLP)-conjugated CspZ proteins. (A) Purified wild-type CspZ or CspZ-Y207A/Y211A with an exposed a sulfhydryl group and virus-like particles (“VLP”) generated from Qβ phage were mixed with Succinimidyl 6-((beta-maleimidopropionamido) hexanoate) (“SMPH”) to crosslink VLP and each of these CspZ proteins (“VLP-CspZ”). (B) The sizes and purity of each conjugate were determined by a 15% SDS-PAGE. The molecular marker (kD) is in lane 1, followed by preparations of VLP (lane 2, “VLP”), CspZ (lane 3, “CspZ”), VLP-CspZ (lane 4, “VLP-CspZ-WT”), and VLP-CspZ-Y207A/Y211A (lane 5, “VLP-CspZ-Y207/Y211A”). The arrows identify known protein products as indicated. (C) Representative images of VLPs generated in this study. EM analysis of freshly purified VLP (“VLP”), VLP-CspZ (“VLP-CspZ-WT”), or VLP-CspZ-Y207A/Y211A (“VLP-CspZ-Y207A/Y211A”) following negative staining with 1% uranyl acetate. Scale bar = 100 nm.
Vaccinating mice with CspZ, VLP-CspZ, or VLP-CspZ-Y207A/Y211A induced similar levels of anti-CspZ antibodies. To examine whether the conjugation of CspZ with VLP and/or the elimination the ability of CspZ to bind FH enhances its immunogenicity, we immunized C3H/HeN mice with PBS, VLP, CspZ, VLP-CspZ, or VLP-CspZ-Y207A/Y211A (Figure S1 in Supplementary Material). This mouse strain, though deficient of TLR signaling (34), has been included in this study as C3H/HeN mice develop apparent manifestations (e.g., arthritis) during B. burgdorferi infection (35). Therefore, this mouse model has been commonly utilized for the Lyme disease vaccine study to recapitulate associated manifestations in humans (20, 21). We quantitatively measured the levels of antibodies against CspZ in the sera from these mice using ELISA. As expected, the titers of anti-CspZ IgG and IgM in VLP-treated mice were not different from PBS-treated mice (Figure 2). Consistent with previous findings (7, 11), vaccination with CspZ elicited antibody response against CspZ, which was 5- (for IgM) to 10-fold (for IgG) higher than PBS- or VLP-inoculated mice (Figure 2). VLP-CspZ and VLP-CspZ-Y207A/Y211A vaccinations also induced anti-CspZ antibodies [5- (for IgM) to 10-fold (for IgG) greater than PBS- and VLP-treated mice; Figure 2]. However, the anti-CspZ antibody responses induced by CspZ, VLP-CspZ, and VLP-CspZ-Y207A/Y211A vaccination were not different, suggesting that conjugating CspZ to VLP or eliminating FH-binding activity of this protein does not increase the total antibody response against CspZ.
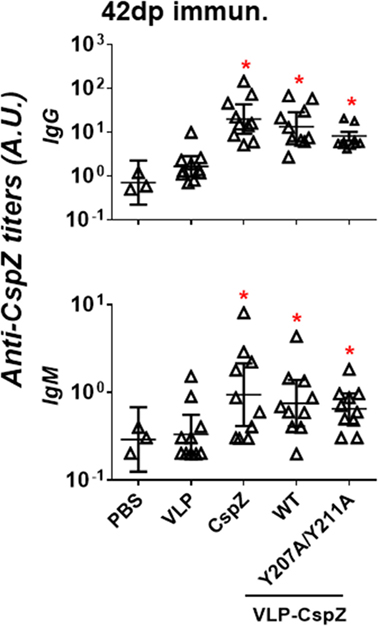
Figure 2. Immunization of CspZ, VLP-CspZ, and VLP-CspZ-Y207A/Y211A triggered undistinguishable antibody response against CspZ. Ten C3H/HeN mice were inoculated with PBS (“PBS”), virus-like particle (“VLP”), CspZ (“CspZ”), VLP-CspZ (“VLP-CspZ-WT”), or VLP-CspZ-Y207A/Y211A (“VLP-CspZ-Y207A/Y211A”), and the serum was obtained at 42 days post inoculation. The serum collected from three C3H/HeN mice at 42 days post inoculation of PBS was also included as negative control. The levels of IgG (top panel) and IgM (bottom panel) against CspZ were determined using quantitative ELISA as described in Section “Materials and Methods.” Data shown are the mean ± SD of three (PBS) or ten (all others) mice per group. Statistical significances (p < 0.05) of differences in antibody titers relative to PBS-inoculated mice were determined using a one-way ANOVA test and are indicated (“*”).
Sera from mice immunized with VLP-CspZ-Y207A/Y211A eradicated spirochetes more effectively than that from CspZ- or VLP-CspZ-inoculated mice. Although antibody titers obtained with unmodified and modified CspZ were similar, the ability of these antibodies in killing spirochetes may be different. We thus examined if eliminating FH binding or VLP conjugation to CspZ would elicit more robust borreliacidal antibody responses. Stepwise dilutions of serum from PBS-, VLP-, CspZ-, VLP-CspZ-, or VLP-CspZ-Y207A/Y211A-inoculated mice were mixed with guinea pig complement and B. burgdorferi, and the levels of spirochete survival were quantified after 24-h incubation. The 50% borreliacidal activity (the dilution rate in which 50% of spirochetes are eliminated) was calculated to quantitatively compare the borreliacidal differences of these sera. Whereas the serum from the PBS- or VLP-inoculated mice was incapable of eradicating spirochetes, the serum from CspZ-, VLP-CspZ-, or VLP-CspZ-Y207A/Y211A-immunized mice killed B. burgdorferi in a dose-dependent manner (Figure 3A). The serum from CspZ-vaccinated mice killed 50% of spirochetes at an average dilution rate of 1:43, whereas diluting the serum from VLP-CspZ-immunized mice at an average of 1:143 eliminated 50% of B. burgdorferi (threefold more effective than that from CspZ-vaccinated mice; Figure 3A; Table S1 in Supplementary Material). Interestingly, the serum from the VLP-CspZ-Y207A/Y211A-immunized mice eradicated 50% of spirochetes at the average dilution rate of 1:395, which was ninefold or threefold more effective than that from the mice immunized with CspZ or VLP-CspZ, respectively (Figure 3; Table S1 in Supplementary Material). Our findings suggest that vaccination of VLP-CspZ-Y207A/Y211A induces antibodies with the greatest borreliacidal activity.
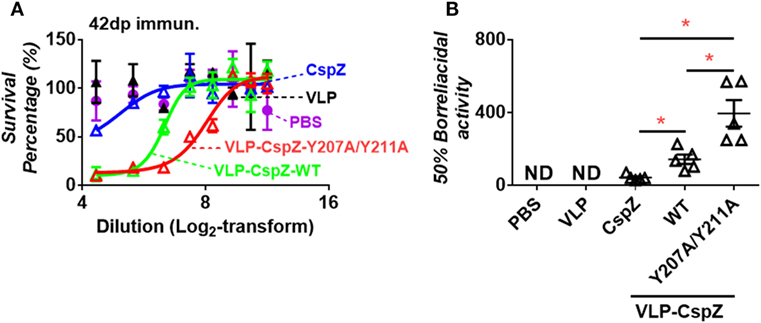
Figure 3. Serum from mice immunized with VLP-CspZ-Y207A/Y211A had more robust levels of bactericidal activity than VLP- or VLP-CspZ-vaccinated mice. Serum collected five C3H/HeN mice at 42 days post inoculation of virus-like particle (“VLP”), CspZ (“CspZ”), VLP-CspZ (“VLP-CspZ-WT”), or VLP-CspZ-Y207A/Y211A (“VLP-CspZ-Y207A/Y211A”) was mixed at indicated dilutions with guinea pig complement and 5 × 105 cells/mL Borrelia burgdorferi strain B31-A3. The serum collected from three C3H/HeN mice at 42 days post inoculation of PBS was also included as negative control. Surviving spirochetes were quantified using dark-field microscopy after 24-h of incubation. (A) The survival percentage was derived from the proportion of serum-treated to untreated B. burgdorferi. Data shown are the mean ± SEM of survival percentage derived from three fields under the microscope for each sample. (B) The 50% borreliacidal titer of each serum sample, representing the dilution rate of the serum that effectively killed 50% of spirochetes, was obtained from the cure-fitting in Panel (A) (see Materials and Methods). Data shown are the mean ± SEM of borreliacidal titers of each serum sample derived from five CspZ, VLP-CspZ, or VLP-CspZ-Y207A/Y211A mice per group. The 50% borreliacidal titers of the serum samples from PBS- or VLP-inoculated mice were not detectable (“ND”) as those serum samples displayed no bactericidal activity. Statistical significances (p < 0.05) of differences in bactericidal titers relative to CspZ-immunized mice were determined using a t-test and are indicated (“*”).
Passive Immunization of Naïve Mice with Serum from VLP-CspZ-Y207A/Y211A-Vaccinated Mice Prevented Lyme Disease
We next determined if passively immunizing mice with serum containing anti-CspZ antibodies with greater borreliacidal activity provides more effective protection against Lyme infection. Naïve mice were passively immunized with serum collected from VLP, CspZ, VLP-CspZ, or VLP-CspZ-Y207A/Y211A actively immunized mice or the pre-immune mouse serum, and then infected with B. burgdorferi (Figure S1B in Supplementary Material). As expected, the pre-immune mouse serum did not protect mice against B. burgdorferi infection (0/6; Table 1). The serum from VLP-immunized mice was unable to protect any passively immunized mice from being infected by spirochetes (0/6; Table 1). Similarly, no protection was observed in any mice passively immunized with serum from CspZ-vaccinated mice (0/6; Table 1). Passive immunization with serum from VLP-CspZ-vaccinated mice prevented Lyme infections in 33% of mice (2/6), but this protection efficiency is not statistically different from that in pre-immune serum inoculated mice (p = 0.22; Table 1). Interestingly, passively immunizing with the serum obtained from VLP-CspZ-Y207A/Y211A-vaccinated mice protected 100% of mice from Lyme infection (6/6; Table 1), and such efficiency is significantly greater than that in pre-immune mouse serum-inoculated mice (p = 0.002; Table 1). These results suggest that the serum from the mice vaccinated with VLP-CspZ-Y207A/Y211A completely protects naïve mice from Lyme infection via passive immunization.
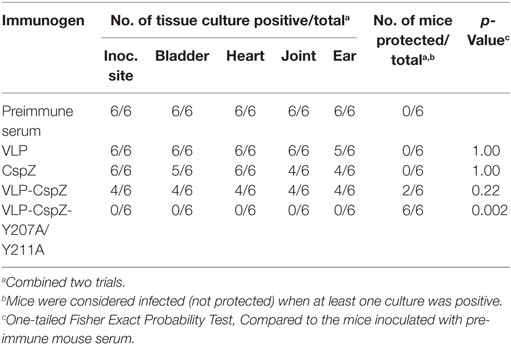
Table 1. Protection against Borrelia burgdorferi in mice passively immunized with serum raised from CspZ- or virus-like particle (VLP)-immunized mice.
Immunization with VLP-CspZ-Y207A/Y211A Provided Greater Protection from Lyme-Associated Arthritis than Vaccination with CspZ or VLP-CspZ
To test whether the CspZ antibodies with greater borreliacidal activity confer more efficient protection from Lyme arthritis via active immunization, C3H/HeN mice were actively immunized with VLP, CspZ, VLP-CspZ, or VLP-CspZ-Y207A/Y211A (Figure S1A in Supplementary Material). Joint diameters were measured at 7 and 14 days post infection (Figure S1C in Supplementary Material), as Lyme-induced joint swelling is detectable as early as these time points (33). C3H/HeN mice at the age group of 3- to 4-week old infected with 104 of B. burgdorferi strain B31 have been previously shown to develop apparent swelling at tibiotarsus joint after 2 weeks of infection (36). Similarly, we observed that the VLP-inoculated mice at the similar age group and the identical infection dose of same spirochete strain also displayed tibiotarsus joint swelling, with the levels most apparent at 7 and 14 days post infection (at least eightfold greater joint diameters than uninfected mice; Figure 4). CspZ and VLP-CspZ vaccinations reduced joint swelling at these time points (approximately two fold less than the mice inoculated with VLP). However, the joint diameters were still significantly greater than that of uninfected mice (p < 0.05), suggesting that CspZ or VLP-CspZ vaccination was incapable of completely alleviating the joint swelling to the levels of uninfected mice (Figure 4). Interestingly, the joint diameters in the mice immunized with VLP-CspZ-Y207A/Y211A were at least threefold less than VLP-immunized mice at 7 and 14 days post infection, but were no different than uninfected mice (Figure 4). Our results imply that vaccination of VLP-CspZ-Y207A/Y211A reduces the joint swelling to the levels of uninfected mice during Lyme disease infection.
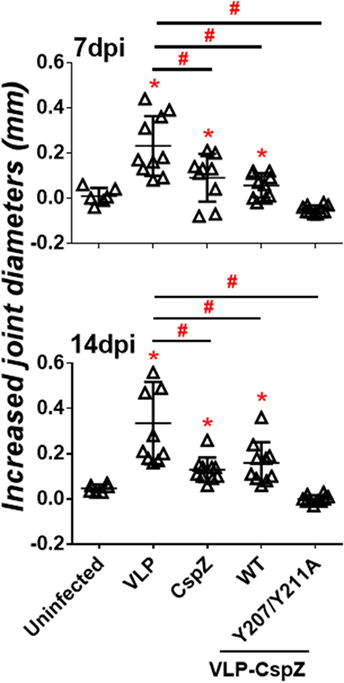
Figure 4. Immunizing mice with VLP-CspZ-Y207A/Y211A prevented joint swelling compared to virus-like particle (VLP) or VLP-CspZ vaccination. Ten C3H/HeN mice were vaccinated with VLP (“VLP”), CspZ (“CspZ”), VLP-CspZ (“VLP-CspZ-WT”), or VLP-CspZ-Y207A/Y211A (“VLP-CspZ-Y207A/Y211A”) prior to infection with 104 Borrelia burgdorferi strain B31-A3. The diameters of tibiotarsus joints were measured at (top panel) 7 and (bottom panel) 14 days post-infection, and from uninfected mice of the same age. The joint size of six uninfected mice was also included as negative control. The increased joint diameters were derived from subtracting the group average tibiotarsus joint diameter prior to infection (0 days post-infection). Data shown are the mean ± SD of 6 (uninfected) or 10 (all others) mice per group. Statistical significance (p < 0.05) of differences in tibiotarsus joint diameters of each group relative to uninfected mice were determined using a one-way ANOVA test and post hoc analysis and are indicated (“*”). Significant differences (p < 0.05) between infected groups are indicated (“#”).
Additionally, we histologically examined the severity of the arthritis in the mice vaccinated with VLP, CspZ, VLP-CspZ, or VLP-CspZ-Y207A/Y211A at 14 days post infection. VLP-inoculated mice developed apparent arthritis with inflammation at the joint, in which inflammatory cells infiltrated around the synovium (Figure 5). A similar arthritis phenotype was observed in CspZ- or VLP-CspZ-vaccinated mice (Figure 5). However, VLP-CspZ-Y207A/Y211A-vaccinated mice did not develop arthritis, with histopathology revealing inflammation similar to uninfected mice (Figure 5). C3H/HeN mice with the similar age group in this study infected with B. burgdorferi have been shown to display significant arthritis (36). Our finding thus suggests that vaccination of VLP-CspZ-Y207A/Y211 prevents mice from developing arthritis during Lyme infection.
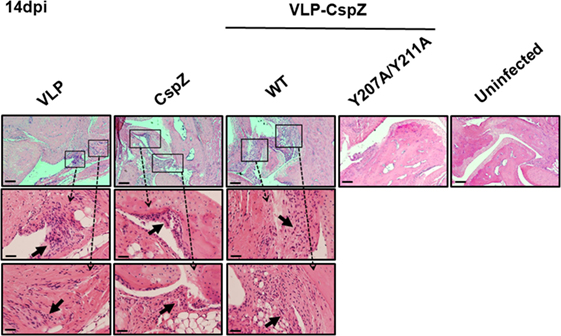
Figure 5. VLP-CspZ-Y207A/Y211A immunization prevents Lyme arthritis in Borrelia burgdorferi-infected C3H/HeN mice at levels similar to uninfected mice. Three C3H/HeN mice were vaccinated with virus-like particle (“VLP”), CspZ (“CspZ”), VLP-CspZ (“VLP-CspZ-WT”), or VLP-CspZ-Y207A/Y211A (“VLP-CspZ-Y207A”) and subsequently infected with 104 B. burgdorferi strain B31-A3. Tibiotarsus joints were collected 14 days post infection and also from three uninfected mice of the same age. To assess inflammation, tissues were fixed and stained with hematoxylin and eosin. The representative images from one mouse per group are shown here. Top panels are lower-resolution images [joint, 10× (bar, 160 µm)]; bottom panels are higher-resolution images [joint, 2 × 20 (bar, 80 µm)] of selected areas (insets in top panels). Arrows indicate infiltration of immune cells.
Immunization with VLP-CspZ-Y207A/Y211A Conferred Greater Protection against B. burgdorferi Tissue Colonization than CspZ or VLP-CspZ Vaccination
To evaluate if vaccination with modified CspZ conjugated to VLP clears spirochete tissue colonization at later stages of infection, mice were actively immunized with VLP, CspZ, VLP-CspZ, or VLP-CspZ-Y207A/Y211A, prior to infection with B. burgdorferi (Figure S1A in Supplementary Material). Bacterial burdens were quantitatively assessed in tissues from these mice at 28 days post infection using qPCR (Figure S1C in Supplementary Material). B. burgdorferi strain B31 has been shown to colonize the inoculation site of skin, joints, and heart of C3H/HeN mice (at the levels approximately 10 to 100 spirochetes per 10 ng DNA) after infection by needles with 104 of spirochetes for 28 days (30). When similar dose and the B. burgdorferi strain were introduced into the same age group of C3H/HeN mice, spirochetes also colonized these tissues of VLP-inoculated mice at similar levels (Figure 6; Table S2 in Supplementary Material; 12–27 spirochetes per 10 ng DNA). Consistent with previous findings (7, 11), we observed that B. burgdorferi colonized inoculation site of skin, joints, and heart of CspZ-immunized mice at a detectable level (Figure 6; Table S2 in Supplementary Material; 12–26 spirochetes per 10 ng DNA). This level was no different than that from VLP-inoculated mice (Figure 6). Further, the bacterial burdens in VLP-CspZ-immunized mice were below the detection limit in the heart and joints (detection limit = 10 bacteria copies per 10 ng DNA; Table S2 in Supplementary Material) and 2.7- to 4.4-fold lower than VLP-immunized mice (p < 0.05; Figure 6). However, there was no difference in the bacterial burden at the inoculation sites of VLP-CspZ and VLP-inoculated mice (Figure 6). Interestingly, vaccination of VLP-CspZ-Y207A/Y211A resulted in undetectable bacterial burdens at the inoculation site, joints, and heart during Lyme infection that were 2.8- to 5.4-fold lower than CspZ- and VLP-inoculated mice (p < 0.05; Figure 6). Our results indicate that immunization of VLP-CspZ-Y207A/Y211A reduces the spirochete colonization to an undetectable level during Lyme disease infection.
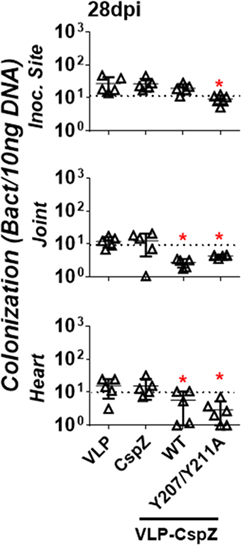
Figure 6. Vaccinating mice with VLP-CspZ-Y207A/Y211A eliminated Borrelia burgdorferi tissue colonization compared to virus-like particle (VLP) or VLP-CspZ immunization. C3H/HeN mice were immunized with VLP (“VLP”), CspZ (“CspZ”), or VLP-CspZ (“VLP-CspZ-WT”, five mice per group), or VLP-CspZ-Y207A/Y211A (“VLP-CspZ-Y207A/Y211A”, six mice per group) and subsequently infected with 104 B. burgdorferi strain B31-A3. Spirochete colonization at inoculation site of skin (“inoc. site”, top panel), knee joint (“joint”, middle panel), and heart (“heart”, bottom panel) was quantitatively measured 28 days post infection. Colonization was derived by normalizing the number of spirochetes detected by quantitative PCR to 10 ng total DNA. Data shown are the mean ± SD, of five (VLP, CspZ, VLP-CspZ) or six (VLP-CspZ-Y207A/Y211A) mice. Statistical significance (p < 0.05) of differences in bacterial burden relative to VLP-immunized mice was determined using a one-way ANOVA test and post hoc analysis and are indicated (“*”).
Discussion
A number of strategies have been used to develop a Lyme disease vaccine, including inoculation of dead or live spirochetes (20), or recombinant proteins from B. burgdorferi or Ixodes ticks (37). In this study, we chose B. burgdorferi CspZ as a potential vaccine candidate because of its antigenicity and its ability to facilitate evasion of complement system (4, 7, 11). While vaccination with CspZ elicits a robust antibody response, it does not protect mice from Lyme infection, possibly due to insufficient functional antibodies (i.e., bactericidal) (7, 11). We thus re-evaluated the efficacy of CspZ as a vaccine against Lyme disease by conjugating CspZ to VLP to generate VLP-CspZ, and combined this approach with eliminating the FH-binding activity of CspZ to generate VLP-CspZ-Y207A/Y211A (6). Conjugating antigens to the highly repetitive structures of VLPs may alter the topology of these antigens. This may eventually allow B cells to more efficiently recognize the epitopes and develop greater levels of antibodies with enhanced bactericidal activity (38, 39). In fact, vaccinating mice with other B. burgdorferi outer surface proteins OspA or OspC conjugated to VLP induces robust levels of protective antibody response (40, 41). Consistent with these findings, though neither VLP-CspZ nor VLP-CspZ-Y207A/Y211A triggered greater titers of anti-CspZ antibodies compared to mice immunized with CspZ, immunizing mice with either of these VLP-CspZ proteins induced antibodies with robust levels of bacterial killing activity. Eliminating the ability of CspZ to bind FH exposes the FH-binding site and, therefore, may increase the ability of the epitopes close to/within this site to induce bactericidal antibodies. In fact, immunization with point mutants of a Neisseria meningitidis FH-binding protein fHbp with reduced FH-binding activity induces greater levels of bactericidal antibodies in vaccinated human FH-transgenic mice and in non-human primates than immunization with wild-type fHbp (19, 42–45). This reduction in immunogenicity as a result of binding to host proteins thus is not restricted to FH-binding molecules (46).
We then tested the combination of VLP conjugation and eliminated FH-binding activity of CspZ as a vaccine in protecting mice from Lyme disease infection via active and passive immunization. TiterMax Gold adjuvant has been used as this adjuvant was reported to induce greater and longer lasting titers than other adjuvants (29). Our data showed complete in vivo protection against Lyme disease from passive immunization with VLP-CspZ-Y207A/Y211A, but not CspZ or VLP-CspZ. During active immunization, CspZ-immunized mice partially alleviated joint swelling compared to the mice inoculated VLP after infection with B. burgdorferi. This finding appears to contradict with a previous study in which no difference in joint swelling was observed between unvaccinated- and CspZ-vaccinated mice (7). However, the differences in methodologies and experimental design prevent direct comparison between these studies. For example, differences can be due to the infectious dose [104 in this study vs 105 in Ref. (7)] and the type of adjuvant [TiterMax Gold in this study vs Complete Freund’s adjuvant in Ref. (7)]. In spite of such differences, both studies found that vaccination with unmodified CspZ is ineffective at either preventing joint swelling (7) or reducing the joint swelling to the level of uninfected mice (Figure 4). In addition, vaccination with CspZ or VLP-CspZ did not prevent arthritis, which implies that the bactericidal ability of the antibodies induced by either of these proteins were insufficient in alleviating Lyme associated arthritis. Vaccination of VLP-CspZ-Y207A/Y211A prevented both joint swelling and arthritis, possibly due to the robust borreliacidal activity of the induced antibodies.
We also observed that B. burgdorferi colonizes colonization at both proximal (inoculation site) and distal mouse tissues (heart and joints) of unmodified CspZ-immunized mice, which is in agreement with previous observations (7, 11). Inoculating mice with either VLP-CspZ or VLP-CspZ-Y207A/Y211A decreased B. burgdorferi colonization to an undetectable level at distal tissues. However, VLP-CspZ-Y207A/Y211A vaccination cleared colonization at the inoculation site while VLP-CspZ immunization did not. One of the possibilities addressing this difference is that the clearance of B. burgdorferi in the inoculation site may require the antibodies with more robust bactericidal activity (e.g., the antibody induced by VLP-CspZ-Y207A/Y211A immunization) to penetrate the tight structure of the skin capillaries into this tissue (47). In this study, we have demonstrated that recombinant CspZ with both conjugating to VLP and eliminated its FH-binding activity is a protective antigen against Lyme disease infection in a murine model. Mice have been widely used as a model to test the efficacy of Lyme disease vaccine [e.g., Ref. (20, 37, 40, 41)]. Additionally, the observations from the previous generation of Lyme disease vaccine performed on mice reflect to the efficacy of this vaccine in humans (48–50). Thus, the findings in this study may provide useful information for the development of Lyme disease vaccine used in humans. Further, the specific strategy of VLP conjugation and eliminating binding to the target ligand may also be applied to antigens of other bacterial pathogens, potentially serving as a general model for vaccination development to ultimately improve human health.
Ethics Statement
All mouse experiments were performed in strict accordance with all provisions of the Animal Welfare Act, the Guide for the Care and Use of Laboratory Animals, and the PHS Policy on Humane Care and Use of Laboratory Animals. The protocol (Docket Number 16-451) was approved by the Institutional Animal Care and Use Agency (IACUC) of Wadsworth Center, New York State Department of Health. All efforts were made to minimize animal suffering.
Author Contributions
All authors contributed to the design and analysis of experiments. AM, IL, SK, XY, and Y-PL performed the experiments in this manuscript. AM, XY, PK, UP, Y-PL, and KT wrote the manuscript. All authors critically reviewed the manuscript.
Conflict of Interest Statement
The authors declare that the research was conducted in the absence of any commercial or financial relationships that could be construed as a potential conflict of interest.
Acknowledgments
The authors thank Stewart Sell and Jennifer Westfall for histopathological analysis, Inara Akopjana for qualified technical assistance for molecular cloning and protein expression, Sanjay Ram and Ching-Lin Hsieh for valuable advice and critical reading of the manuscript. The authors thank Deirdre Torrisi of Wadsworth Animal Core for assistance with mouse procedures, and Abigail Snyder-Keller and Helen Johnson of the Wadsworth Histopathology Core for generating the slides with H&E staining.
Funding
This work was supported by New York State Department of Health Wadsworth Center Start-Up Grant (to Y-PL and AM), NIH R01AI080615 and R01AI116620 (to UP and XY), NIH R01AI121401 (to PK), and ERDF grant 2014/0014/2DP/2.1.1.1.0/14/APIA/VIAA/013 (to KT, IL, and SK).
Supplementary Material
The Supplementary Material for this article can be found online at http://www.frontiersin.org/articles/10.3389/fimmu.2018.00181/full#supplementary-material.
Abbreviations
VLP, virus-like particle; VLP-CspZ, VLP-conjugated CspZ; VLP-CspZ-Y207A/Y211A, VLP-conjugated CspZ-Y207A/Y211A; B. burgdorferi, Borrelia burgdorferi sensu stricto; FH, Factor H; FHL-1, Factor H-like protein 1; CRASP-2, complement regulator-acquiring surface protein 2; A.U., arbitrary unit; qPCR, quantitative PCR; IACUC, Institutional Animal Care and Use Agency.
References
1. Steere AC, Strle F, Wormser GP, Hu LT, Branda JA, Hovius JW, et al. Lyme borreliosis. Nat Rev Dis Primers (2016) 2:16090. doi:10.1038/nrdp.2016.90
2. Radolf JD, Caimano MJ, Stevenson B, Hu LT. Of ticks, mice and men: understanding the dual-host lifestyle of Lyme disease spirochaetes. Nat Rev Microbiol (2012) 10:87–99. doi:10.1038/nrmicro2714
3. Zipfel PF, Skerka C. Complement regulators and inhibitory proteins. Nat Rev Immunol (2009) 9:729–40. doi:10.1038/nri2620
4. Hartmann K, Corvey C, Skerka C, Kirschfink M, Karas M, Brade V, et al. Functional characterization of BbCRASP-2, a distinct outer membrane protein of Borrelia burgdorferi that binds host complement regulators factor H and FHL-1. Mol Microbiol (2006) 61:1220–36. doi:10.1111/j.1365-2958.2006.05318.x
5. Rogers EA, Marconi RT. Delineation of species-specific binding properties of the CspZ protein (BBH06) of Lyme disease spirochetes: evidence for new contributions to the pathogenesis of Borrelia spp. Infect Immun (2007) 75:5272–81. doi:10.1128/IAI.00850-07
6. Siegel C, Schreiber J, Haupt K, Skerka C, Brade V, Simon MM, et al. Deciphering the ligand-binding sites in the Borrelia burgdorferi complement regulator-acquiring surface protein 2 required for interactions with the human immune regulators factor H and factor H-like protein 1. J Biol Chem (2008) 283:34855–63. doi:10.1074/jbc.M805844200
7. Coleman AS, Yang X, Kumar M, Zhang X, Promnares K, Shroder D, et al. Borrelia burgdorferi complement regulator-acquiring surface protein 2 does not contribute to complement resistance or host infectivity. PLoS One (2008) 3:3010e. doi:10.1371/journal.pone.0003010
8. Lin T, Gao L, Zhang C, Odeh E, Jacobs MB, Coutte L, et al. Analysis of an ordered, comprehensive STM mutant library in infectious Borrelia burgdorferi: insights into the genes required for mouse infectivity. PLoS One (2012) 7:e47532. doi:10.1371/journal.pone.0047532
9. Bykowski T, Woodman ME, Cooley AE, Brissette CA, Brade V, Wallich R, et al. Coordinated expression of Borrelia burgdorferi complement regulator-acquiring surface proteins during the Lyme disease spirochete’s mammal-tick infection cycle. Infect Immun (2007) 75:4227–36. doi:10.1128/IAI.00604-07
10. Kraiczy P, Seling A, Brissette CA, Rossmann E, Hunfeld KP, Bykowski T, et al. Borrelia burgdorferi complement regulator-acquiring surface protein 2 (CspZ) as a serological marker of human Lyme disease. Clin Vaccine Immunol (2008) 15:484–91. doi:10.1128/CVI.00415-07
11. Rogers EA, Abdunnur SV, Mcdowell JV, Marconi RT. Comparative analysis of the properties and ligand binding characteristics of CspZ, a factor H binding protein, derived from Borrelia burgdorferi isolates of human origin. Infect Immun (2009) 77:4396–405. doi:10.1128/IAI.00393-09
12. Kang SM, Compans RW. Enhancement of mucosal immunization with virus-like particles of simian immunodeficiency virus. J Virol (2003) 77:3615–23. doi:10.1128/JVI.77.18.9823-9830.2003
13. Jennings GT, Bachmann MF. The coming of age of virus-like particle vaccines. Biol Chem (2008) 389:521–36. doi:10.1515/BC.2008.064
14. Khan F, Porter M, Schwenk R, Debot M, Saudan P, Dutta S. Head-to-head comparison of soluble vs. Qbeta VLP circumsporozoite protein vaccines reveals selective enhancement of NANP repeat responses. PLoS One (2015) 10:e0142035. doi:10.1371/journal.pone.0142035
15. Ambuhl PM, Tissot AC, Fulurija A, Maurer P, Nussberger J, Sabat R, et al. A vaccine for hypertension based on virus-like particles: preclinical efficacy and phase I safety and immunogenicity. J Hypertens (2007) 25:63–72. doi:10.1097/HJH.0b013e32800ff5d6
16. Bachmann MF, Jennings GT. Therapeutic vaccines for chronic diseases: successes and technical challenges. Philos Trans R Soc Lond B Biol Sci (2011) 366:2815–22. doi:10.1098/rstb.2011.0103
17. Wiessner C, Wiederhold KH, Tissot AC, Frey P, Danner S, Jacobson LH, et al. The second-generation active Abeta immunotherapy CAD106 reduces amyloid accumulation in APP transgenic mice while minimizing potential side effects. J Neurosci (2011) 31:9323–31. doi:10.1523/JNEUROSCI.0293-11.2011
18. Beeh KM, Kanniess F, Wagner F, Schilder C, Naudts I, Hammann-Haenni A, et al. The novel TLR-9 agonist QbG10 shows clinical efficacy in persistent allergic asthma. J Allergy Clin Immunol (2013) 131:866–74. doi:10.1016/j.jaci.2012.12.1561
19. Beernink PT, Shaughnessy J, Braga EM, Liu Q, Rice PA, Ram S, et al. A meningococcal factor H binding protein mutant that eliminates factor H binding enhances protective antibody responses to vaccination. J Immunol (2011) 186:3606–14. doi:10.4049/jimmunol.1003470
20. Hahn BL, Padmore LJ, Ristow LC, Curtis MW, Coburn J. Live attenuated Borrelia burgdorferi targeted mutants in an infectious strain background protect mice from challenge infection. Clin Vaccine Immunol (2016) 23:725–31. doi:10.1128/CVI.00302-16
21. Kern A, Zhou CW, Jia F, Xu Q, Hu LT. Live-vaccinia virus encapsulation in pH-sensitive polymer increases safety of a reservoir-targeted Lyme disease vaccine by targeting gastrointestinal release. Vaccine (2016) 34:4507–13. doi:10.1016/j.vaccine.2016.07.059
22. Elias AF, Stewart PE, Grimm D, Caimano MJ, Eggers CH, Tilly K, et al. Clonal polymorphism of Borrelia burgdorferi strain B31 MI: implications for mutagenesis in an infectious strain background. Infect Immun (2002) 70:2139–50. doi:10.1128/IAI.70.4.2139-2150.2002
23. Barbour AG, Burgdorfer W, Grunwaldt E, Steere AC. Antibodies of patients with Lyme disease to components of the Ixodes dammini spirochete. J Clin Invest (1983) 72:504–15. doi:10.1172/JCI110998
24. Purser JE, Norris SJ. Correlation between plasmid content and infectivity in Borrelia burgdorferi. Proc Natl Acad Sci U S A (2000) 97:13865–70. doi:10.1073/pnas.97.25.13865
25. Spohn G, Jennings GT, Martina BE, Keller I, Beck M, Pumpens P, et al. A VLP-based vaccine targeting domain III of the West Nile virus E protein protects from lethal infection in mice. Virol J (2010) 7:146. doi:10.1186/1743-422X-7-146
26. Low JG, Lee LS, Ooi EE, Ethirajulu K, Yeo P, Matter A, et al. Safety and immunogenicity of a virus-like particle pandemic influenza A (H1N1) 2009 vaccine: results from a double-blinded, randomized phase I clinical trial in healthy Asian volunteers. Vaccine (2014) 32:5041–8. doi:10.1016/j.vaccine.2014.07.011
27. Kozlovska TM, Cielens I, Dreilinna D, Dislers A, Baumanis V, Ose V, et al. Recombinant RNA phage Q beta capsid particles synthesized and self-assembled in Escherichia coli. Gene (1993) 137:133–7. doi:10.1016/0378-1119(93)90261-Z
28. Lin YP, Lee DW, Mcdonough SP, Nicholson LK, Sharma Y, Chang YF. Repeated domains of leptospira immunoglobulin-like proteins interact with elastin and tropoelastin. J Biol Chem (2009) 284:19380–91. doi:10.1074/jbc.M109.004531
29. Bennett B, Check IJ, Olsen MR, Hunter RL. A comparison of commercially available adjuvants for use in research. J Immunol Methods (1992) 153:31–40. doi:10.1016/0022-1759(92)90302-A
30. Lin YP, Benoit V, Yang X, Martinez-Herranz R, Pal U, Leong JM. Strain-specific variation of the decorin-binding adhesin DbpA influences the tissue tropism of the lyme disease spirochete. PLoS Pathog (2014) 10:e1004238. doi:10.1371/journal.ppat.1004238
31. Aydintug MK, Gu Y, Philipp MT. Borrelia burgdorferi antigens that are targeted by antibody-dependent, complement-mediated killing in the rhesus monkey. Infect Immun (1994) 62:4929–37.
32. Floden AM, Gonzalez T, Gaultney RA, Brissette CA. Evaluation of RevA, a fibronectin-binding protein of Borrelia burgdorferi, as a potential vaccine candidate for Lyme disease. Clin Vaccine Immunol (2013) 20:892–9. doi:10.1128/CVI.00758-12
33. Petzke MM, Iyer R, Love AC, Spieler Z, Brooks A, Schwartz I. Borrelia burgdorferi induces a type I interferon response during early stages of disseminated infection in mice. BMC Microbiol (2016) 16:29. doi:10.1186/s12866-016-0644-4
34. Poltorak A, He X, Smirnova I, Liu MY, Van Huffel C, Du X, et al. Defective LPS signaling in C3H/HeJ and C57BL/10ScCr mice: mutations in Tlr4 gene. Science (1998) 282:2085–8. doi:10.1126/science.282.5396.2085
35. Ma Y, Seiler KP, Eichwald EJ, Weis JH, Teuscher C, Weis JJ. Distinct characteristics of resistance to Borrelia burgdorferi-induced arthritis in C57BL/6N mice. Infect Immun (1998) 66:161–8.
36. Chan K, Awan M, Barthold SW, Parveen N. Comparative molecular analyses of Borrelia burgdorferi sensu stricto strains B31 and N40D10/E9 and determination of their pathogenicity. BMC Microbiol (2012) 12:157. doi:10.1186/1471-2180-12-157
37. Embers ME, Narasimhan S. Vaccination against Lyme disease: past, present, and future. Front Cell Infect Microbiol (2013) 3:6. doi:10.3389/fcimb.2013.00006
38. Hinton HJ, Jegerlehner A, Bachmann MF. Pattern recognition by B cells: the role of antigen repetitiveness versus Toll-like receptors. Curr Top Microbiol Immunol (2008) 319:1–15. doi:10.1007/978-3-540-73900-5_1
39. Rynda-Apple A, Patterson DP, Douglas T. Virus-like particles as antigenic nanomaterials for inducing protective immune responses in the lung. Nanomedicine (Lond) (2014) 9:1857–68. doi:10.2217/nnm.14.107
40. Nassal M, Skamel C, Kratz PA, Wallich R, Stehle T, Simon MM. A fusion product of the complete Borrelia burgdorferi outer surface protein A (OspA) and the hepatitis B virus capsid protein is highly immunogenic and induces protective immunity similar to that seen with an effective lipidated OspA vaccine formula. Eur J Immunol (2005) 35:655–65. doi:10.1002/eji.200425449
41. Skamel C, Ploss M, Bottcher B, Stehle T, Wallich R, Simon MM, et al. Hepatitis B virus capsid-like particles can display the complete, dimeric outer surface protein C and stimulate production of protective antibody responses against Borrelia burgdorferi infection. J Biol Chem (2006) 281:17474–81. doi:10.1074/jbc.M513571200
42. Rossi R, Granoff DM, Beernink PT. Meningococcal factor H-binding protein vaccines with decreased binding to human complement factor H have enhanced immunogenicity in human factor H transgenic mice. Vaccine (2013) 31:5451–7. doi:10.1016/j.vaccine.2013.08.099
43. Giuntini S, Beernink PT, Granoff DM. Effect of complement Factor H on anti-FHbp serum bactericidal antibody responses of infant rhesus macaques boosted with a licensed meningococcal serogroup B vaccine. Vaccine (2015) 33:7168–75. doi:10.1016/j.vaccine.2015.10.135
44. Granoff DM, Costa I, Konar M, Giuntini S, Van Rompay KK, Beernink PT. Binding of complement factor H (FH) decreases protective anti-FH binding protein antibody responses of infant rhesus macaques immunized with a meningococcal serogroup B vaccine. J Infect Dis (2015) 212:784–92. doi:10.1093/infdis/jiv081
45. Granoff DM, Giuntini S, Gowans FA, Lujan E, Sharkey K, Beernink PT. Enhanced protective antibody to a mutant meningococcal factor H-binding protein with low-factor H binding. JCI Insight (2016) 1:e88907. doi:10.1172/jci.insight.88907
46. Zariri A, Van Dijken H, Hamstra HJ, Van Der Flier M, Vidarsson G, Van Putten JP, et al. Expression of human CEACAM1 in transgenic mice limits the Opa-specific immune response against meningococcal outer membrane vesicles. Vaccine (2013) 31:5585–93. doi:10.1016/j.vaccine.2013.07.069
47. Tabrizi M, Bornstein GG, Suria H. Biodistribution mechanisms of therapeutic monoclonal antibodies in health and disease. AAPS J (2010) 12:33–43. doi:10.1208/s12248-009-9157-5
48. Fikrig E, Barthold SW, Kantor FS, Flavell RA. Protection of mice against the Lyme disease agent by immunizing with recombinant OspA. Science (1990) 250:553–6. doi:10.1126/science.2237407
49. Keller D, Koster FT, Marks DH, Hosbach P, Erdile LF, Mays JP. Safety and immunogenicity of a recombinant outer surface protein A Lyme vaccine. JAMA (1994) 271:1764–8. doi:10.1001/jama.271.22.1764
Keywords: Lyme disease, CspZ, Borrelia, vaccine, virus-like particles, factor H
Citation: Marcinkiewicz AL, Lieknina I, Kotelovica S, Yang X, Kraiczy P, Pal U, Lin Y-P and Tars K (2018) Eliminating Factor H-Binding Activity of Borrelia burgdorferi CspZ Combined with Virus-Like Particle Conjugation Enhances Its Efficacy as a Lyme Disease Vaccine. Front. Immunol. 9:181. doi: 10.3389/fimmu.2018.00181
Received: 29 October 2017; Accepted: 22 January 2018;
Published: 08 February 2018
Edited by:
Fabio Bagnoli, GlaxoSmithKline, ItalyReviewed by:
Mario T. Philipp, Tulane University Primate Center, Tulane University, United StatesAxel T. Lehrer, University of Hawaii at Manoa, United States
Copyright: © 2018 Marcinkiewicz, Lieknina, Kotelovica, Yang, Kraiczy, Pal, Lin and Tars. This is an open-access article distributed under the terms of the Creative Commons Attribution License (CC BY). The use, distribution or reproduction in other forums is permitted, provided the original author(s) and the copyright owner are credited and that the original publication in this journal is cited, in accordance with accepted academic practice. No use, distribution or reproduction is permitted which does not comply with these terms.
*Correspondence: Yi-Pin Lin, eWktcGluLmxpbkBoZWFsdGgubnkuZ292;
Kaspars Tars, a2FzcGFyc0BiaW9tZWQubHUubHY=
†These authors have contributed equally to this work.