- 1Department of Pharmacology, School of Medicine, Shahid Beheshti University of Medical Sciences, Tehran, Iran
- 2Student Research Committee, Social Determinants of Health Center, Shahid Beheshti University of Medical Sciences, Tehran, Iran
- 3Ludwig Boltzmann Institute for Experimental and Clinical Traumatology in AUVA Research Center, Vienna, Austria
Multiple sclerosis (MS) is an inflammatory and degenerative disorder of the central nervous system with unknown etiology. It is accompanied by demyelination of the nerves during immunological processes in the presence of oxidative stress, hypoxia, cerebral hypo-perfusion, and dysregulation in matrix metalloproteinases (MMPs). Human amniotic mesenchymal stem cells (hAMSCs) as pluripotent stem cells possess some conspicuous features which could be of therapeutic value in MS therapy. hAMSCs could mimic the cascade of signals and secrete factors needed for promoting formation of stable neovasculature and angiogenesis. hAMSCs also have immunomodulatory and immunosuppressive effects on inflammatory processes and reduce the activity of inflammatory cells, migration of microglia and inhibit recruitment of certain immune cells to injury sites. hAMSCs attenuate the oxidative stress supported by the increased level of antioxidant enzymes and the decreased level of lipid peroxidation products. Furthermore, hAMSCs enhance neuroprotection and neurogenesis in brain injuries by inhibition of inflammation and promotion of neurogenesis. hAMSCs could significantly increase the expression of neurotrophic factors, which prevents neurons from initiating programmed cell death and improves survival, development, and function of neurons. In addition, they induce differentiation of neural progenitor cells to neurons. hAMSCs could also inhibit MMPs dysregulation and consequently promote the survival of endothelial cells, angiogenesis and the stabilization of vascular networks. Considering the mentioned evidences, we hypothesized here that hAMSCs and their conditioned medium could be of therapeutic value in MS therapy due to their unique properties, including immunomodulation and inflammation suppression; angiogenesis promotion; oxidative stress inhibition; neurogenesis induction and neuroprotection; matrix metalloproteinases regulation; and remyelination stimulation.
Introduction
Multiple sclerosis (MS) is an inflammatory disorder of the central nervous system which is accompanied by neural demyelination, axonal loss, and disability. Although the main etiology of MS is unknown, genetic, environmental and infectious agents may be among the factors that play a role in the development of MS (1). The main pathologic processes of MS include inflammatory and degenerative phases (2). The presence of inflammatory cells and their secreted molecules in the demyelinating lesions supports the notion that pathogenic T cells that react with myelin antigens play a key role in MS, which then results in the degeneration of neurons (3). In addition, the migration of autoreactive T cells to the central nervous system results in destroying the central neurons and their myelin sheaths, which is mediated by matrix metalloproteinases (MMPs) (4). Furthermore, oxidative stress, hypoxia and cerebral hypo-perfusion may lead to increased demyelinating lesions in MS (5, 6).
Although various pharmacological and non-pharmacological therapeutic approaches have been used in the treatment of MS, no definite cure of MS has been discovered to date. Moreover, all the approved therapeutic approaches are expected to be life-long, while their potential adverse effects may compromise their safety or patients' adherence to treatment. Therefore, it is imperative yet challenging to find new safe therapies with fewer delivery concerns and less adverse effects that are more effective in slowing or preventing MS progression, have the potential to reverse patients' disability, and consequently improve patients' adherence.
Cell-based therapy by mesenchymal stem cells (MSCs) is one of the therapeutic approaches that has drawn attention as a potential approach to address these challenges, considering initial promising research results (7). Nevertheless, there are uncertainties regarding the use of MSCs in MS treatment including tissue source, route of delivery, cell number, dosing, adverse effects, safety monitoring, and duration of action (8).
Human amniotic membrane is considered a potential source for MSCs which possesses unique biological properties, including anti-inflammatory, anti-fibrosis, anti-scarring and low immunogenicity characteristics (9, 10). Human amniotic mesenchymal stem cells (hAMSCs) are pluripotent stem cells that could be isolated from the fibroblastic layer of human amniotic membrane.
Altogether, it seems that hAMSCs have potential therapeutic features that could be promising in MS therapy. There is some evidence for some hAMSCs properties including immunomodulation and inflammation suppression (11); angiogenesis promotion (12, 13); oxidative stress inhibition (14); neurogenesis induction and neuroprotection (15); matrix metalloproteinase regulation (16); and remyelination stimulation.
Supporting Evidence for Hypothesis
Immunomodulation and Inflammation Suppression
Inflammation in the central nervous system is one of the major pathogenic processes in MS. Although the trigger of the inflammatory response in MS is still not clear, it is suggested that MS is developed when auto-reactive T cells target proteins which exist predominantly in myelin and on axons (17, 18). Besides T cells, B cells, macrophages, activated microglia, and dendritic cells act as key players in the pathogenesis of multiple sclerosis (19). When dendritic cells are exposed to myelin-derived antigens, they secrete cytokines which induce the differentiation of naive T cells into effector T cells in secondary lymphoid tissues (2). Inflammatory cells migrate across the blood-brain-barrier to the central nervous system and thus cause the inflammatory lesions which are characterized by an area of demyelination of nerves and axonal loss. In addition, poor T-regulatory functioning enhances the expansion of inflammatory responses (20).
Efficient treatment of MS depends on developing a therapeutic method that can specifically target and regulate immune responses. A number of immunomodulatory or immunosuppressive drugs including Interferon-β, Glatiramer acetate, Natalizumab, and Fingolimod have been designed to target the immune component of MS. Although these drugs have displayed beneficial effects for halting MS, they have shown little impact on its progression (21). On the other hand, mesenchymal stem cells could be applied as therapeutics in MS through imposing their immunomodulatory effect by inducing a shift in T cells from a pro-inflammatory to an anti-inflammatory state (22); inhibiting naive and memory T cell proliferation and maturation (23); inhibiting proliferation, secretion of proinflammatory cytokines, and cytotoxicity of natural killer cells and natural killer T cells; inhibiting B cell proliferation, and antibodies production; inhibiting the initial differentiation of monocytes to dendritic cells and impairing their activation, and antigen presentation (24); and inhibiting the chemotactic activity of neutrophils (25). In addition, they promote the generation of regulatory T cells (23), preserve neutrophil viability and function (26), and regulate macrophage recruitment (27) and function (28).
hAMSCs could lead to a decrease of peripheral blood mononuclear cells, interferon-gamma and interleukin-17 production (29). They might also reduce migration, recruitment, and activity of a broad range of immune cells, including T cells, natural killer cells, natural killer T cells, dendritic cells, B cells, neutrophils, monocytes, and macrophages at injury sites (30). The secretion of nitric oxide by hAMSCs could be the main cause of their immunosuppressive effect (11). Currently, several soluble factors, either produced constitutively by hAMSCs or as a result of cross-talk with target immune cells, have been shown to exert the immunomodulatory properties of MSCs, including indoleamine2,3- dioxygenase, prostaglandin E2, interleukin-10, interleukin-6, HLA-G, transforming growth factor-b1, and hepatocyte growth factor (23, 31–33). In addition, hAMSCs have shown a positive effect on immunosuppression in mice models of MS (2, 18).
Angiogenesis Promotion
Cerebral hypoperfusion as well as vascular factors are involved in neurovascular dysfunction, vascular oxidative stress, and relative tissue hypoxia, which could increase the risk of developing demyelinating lesions as observed in MS. Therefore, cerebral hypoperfusion may represent pathologic factors or neuroprotective processes involved in recovery or progression of MS (6).
Although various therapeutic approaches have been utilized to promote angiogenesis, most approaches still cannot fully mimic the process of natural vessel development. The use of hAMSCs has been explored to mimic the cascade of signals needed for enhancing viability and promoting formation of stable neovasculature (16). We also showed the inducing effects of hAMSCs conditioned media on the sprouting of endothelial cells (12, 13). MSCs' potential for angiogenesis relies on their ability to differentiate to smooth muscle cells and endothelial cells as well as their paracrine effects by angiogenetic factors. Angiogenic factors secreted by MSCs vary based on the source and include VEGF, bFGF, MCP-1, SDF-1, angiopoietin, monocyte chemoattractant protein, interleukin-6, placental growth factor, and cysteine-rich angiogenic inducer 61, which could regulate vascular network remodeling (34). Moreover, hAMSCs could promote angiogenesis by inducing the extracellular signaling-regulated kinase 1/2-MAPK signaling pathway (35). Therefore, conditioned medium from hAMSCs could be beneficial for promoting angiogenesis and would probably enhance tissue repair.
Oxidative Stress Inhibition
The inflammatory processes play a significant role in neural tissue injury (36) and in MS pathogenesis critically involve Reactive Oxygen Species (ROS). Infiltrated immune cells, macrophages and activated microglia could generate immense amounts of oxidizing radicals including superoxide, hydrogen peroxide and nitric oxide. In addition, the activation of immature myeloid cells induce synthesis of nitric oxide and reactive oxygen species (5). Free radicals can also activate nuclear transcription factor-kappa B (NF-κB). NF-κB upregulates the expression of genes involved in MS, including tumor necrosis factor-α (TNF-α), nitric oxide synthase (iNOS), intracellular adhesion molecule 1 (ICAM-1) and vascular-cell adhesion molecule 1 (VCAM-1) (37).
Treatment of mice model of MS with antioxidant enzymes markedly suppressed the severity of MS (38). In addition, mitochondrial stabilization and ROS-mediated phagocytosis of myelin may reduce axonal damage in mice models of MS (39–41).
The utilization of MSCs in mice models of MS can inhibit the production of inflammatory factors, including nitric oxide (NO), tumor necrosis factor, IL1-β and reactive oxygen species by activated microglia and preventing neuronal damage. The intravenous injection of MSCs showed a string antioxidant effect in a mice model of MS through the high expression of antioxidant enzymes including catalase, superoxide desmutase and poly (ADP-ribose) polymerase-1 during MSC treatment (42).
In addition, hAMSCs transplantation into transgenic mice increased the level of antioxidant enzymes and decreased the level of lipid peroxidation and oxidative stress (14).
Neurogenesis Induction, Neuroprotection, and Remyelination Stimulation
Neurodegeneration is considered as a major contributor to neurological disability in MS and might be the dominant underlying process of progressive MS. Whether the neurodegeneration is an independent process or due to inflammatory processes remains unknown (43). There are studies that report the mechanisms of neurodegeneration in MS, including the accumulation of amyloid precursor protein in neurons and a reduction in the N-acetyl-aspartate/Creatine ratio (2). In addition, damage to mitochondrial DNA and mitochondrial enzyme complexes may lead to neurodegeneration (44). Axonal density reduction in the white matter and spinal cords of MS patients is another probable mechanism of neurodegeneration (45).
Another process involved in pathogenesis of MS is demyelination. Demyelination causes myelin-producing oligodendrocytes to undergo apoptosis and thus results in myelin loss (46). In response to demyelination, activated resident oligodendrocyte progenitor cells proliferate, migrate to affected areas, and differentiate to replace lost oligodendrocytes, which might lead to myelin reconstitution and functional recovery (47, 48). However, remyelination is typically incomplete or defective and many lesions remain demyelinated (48, 49). This could be either due to the limited ability of mature oligodendrocytes to compensate for myelin loss (50) or to the failure of oligodendrocyte progenitor cells to successfully generate new myelinating cells (48). Therefore, oligodendrocyte progenitor cells cannot often compensate myelin loss on their own.
Treatment of MS with hAMSCs could significantly increase the expression of neurotrophic factors including NGF, CNTF, and BDNF (51). Neurotrophins prevent neurons from initiating programmed cell death and improve survival, development, and function of neurons (15, 52, 53). In a study, hAMSCs promoted neurological recovery in rats after intracranial hemorrhage. It was concluded that the mechanism of action was mediated by inhibition of inflammation and apoptosis, increasing neurotrophic factors expression, and promoting neurogenesis and angiogenesis (54). hAMSCs induce differentiation of progenitor cells to neurons (52). In addition, hAMSCs have the ability to differentiate neural and glial cells in response to induction medium (55). The level of neurotrophins significantly decreases in the CNS of MS patients and is correlated with neuron damage. Therefore, increasing the levels of neurotrophins—or at least maintaining their physiological levels—in MS patients might be of therapeutic value (56, 57). The therapeutic effects of hAMSCs were able to improve the motor functions of neurodegenerative diseases in mice models significantly (58), which indicates that hAMSCs have the potential to differentiate into neural cells. Therefore, hAMSCs could potentially promote neurogenesis, neuroprotection, and remyelination.
Matrix Metalloproteinase Regulation
MMPs are a family of a large number of proteolytic enzymes that have received much attention in neuro-inflammatory diseases. Leukocyte infiltration through the blood-brain-barrier is dependent on several factors including secretion of tumor necrotizing factor-α, gelatinase B/MMP-9 and gelatinase A/MMP-2 (59). It has been shown that the expression of MMPs leads to degradation of extracellular matrix proteins of the basal lamina which surrounds blood vessels (60). MMP-9 is a major matrix metalloproteinase in the pathogenesis of multiple sclerosis and experimental autoimmune encephalitis, which could enhance leukocyte migration, blood-brain-barrier disruption and myelin lysis (4). Different attempts have been made to develop inhibitors of MMPs for the potential treatment of diseases in which MMPs play a major role. In one study, treatment of MS patients with Natalizumab decreased the risk of progressive multifocal encephalopathy by inhibition of MMP-9 (61).
Mesenchymal stem cells application in inflammatory diseases resulted in decreased levels of MMPs or reduced MMPs activity. In another study, a conditioned medium of MSCs decreased disease severity by inhibition of the MMPs activity rate in inflammatory arthritis (62). In another study, MSCs were introduced as robust sources of MMPs inhibition that were mediated by Tissue Inhibitors of Metalloproteinase (TIMPs). This may have therapeutic effects in inflammatory and vascular diseases (63). It has been shown that treatment with mesenchymal stem cells inhibited dysregulation of both MMPs and TIMPs after focal ischemic stroke, which facilitated neurological and functional recovery after stroke (64).
Hypothesis
Considering the supporting evidences, we hypothesize that hAMSCs have potential therapeutic features in multiple sclerosis via angiogenesis promotion, inflammation suppression, oxidative stress inhibition, neurogenesis induction, neuroprotection, MMPs regulation, and remyelination stimulation (Figure 1).
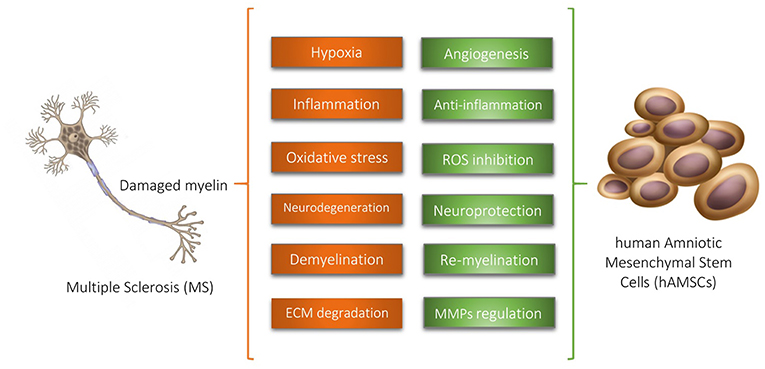
Figure 1. Human Amniotic Mesenchymal Stem Cells (hAMSCs) have potential therapeutic features in multiple sclerosis via supporting evidences illustrated above. ROS, Reactive Oxidative Species; ECM, Extracellular Matrix; MMPs, Matrix Metalloproteinases.
Evaluation of Hypothesis
The hypothesis will be evaluated by conducting in vitro and in vivo experimental studies to address the building block questions of the hypothesis.
In vitro Studies
To evaluate the in vitro effects of hAMSCs conditioned medium, oligodendrocyte cell lines such as the OLN-93 will be cultured. To determine neuroprotection and oxidation inhibition of hAMSCs conditioned medium, first oxidative stress and cell death will be induced by using H2O2 or cuprizone. Afterwards, viability, qualitative and quantitative levels of apoptotic markers as well as neurotrophin levels will be measured and mitochondrial assays will be conducted before and after treatment with hAMSCs conditioned medium. The concentration-dependence to exert the immunomodulatory actions of hAMSCs will be investigated by mixed lymphocyte reaction assay.
In vivo Studies
To evaluate the in vivo effects of hAMSCs conditioned medium, the toxic model of multiple sclerosis will be created in C57BL/6 mice by cuprizone. To measure the motor coordination and balance among mice, rotarod performance testing will be carried out before and after treatment with hAMSCs conditioned medium. To determine the effect of hAMSCs conditioned medium on angiogenesis, VEGF levels will be evaluated by ELISA kit. To determine inflammation suppression by hAMSCs conditioned medium, inflammatory and anti-inflammatory cytokines will be measured before and after treatment with hAMSCs conditioned medium by ELISA kits.
To evaluate direct injection of hAMSCs, the cells would be locally injected in the affected regions of the brain tissue. In addition, as MSCs express a variety of chemokine receptors including CXCR4 and CCR2, and cell adhesion molecules including CD44, integrins α4 and β1, and CD99 (65), they could be delivered via intravenous administration. Therefore, we aim to compare the effectiveness of the ways of administration of hAMSCs in MS models in future studies. In addition, to evaluate the effect of the number of cells administered in future studies, 1 × 106 hAMSCs (66) will be injected into the tail vein of rat and the number of cells will be adjusted if necessary. Results will be evaluated after 6 weeks for investigation of the acute phase of inflammation and 12 weeks to evaluate the chronic phase of the MS model.
To evaluate oxidative stress inhibition by hAMSCs, the reactive oxygen species and glutathione reduction is measured before and after treatment with hAMSCs conditioned medium by glutathione assay kit.
To evaluate the effects of hAMSCs conditioned media on OPC differentiation for neurogenesis and remyelination, oligodendrocyte precursor marker, Olig2, and adult myelin markers, PLP, MBP, MOG, will be measured by real-time PCR and Western blot analyses. To further investigate the effects of hAMSCs, cellular and molecular tests using immunohistochemistry, Western blot and real time-PCR analyses on the myelin genes and proteins as well as microscopic examinations will be carried out. Finally, to determine the effect of hAMSCs conditioned medium on MMPs regulation, MMPs activity will be determined by MMPs activity assay.
Discussion
hAMSCs have unique properties including inflammation suppression, angiogenesis promotion, oxidative stress inhibition, neurogenesis induction, neuroprotection, MMPs regulation, and remyelination stimulation, which are of potential therapeutic value for MS therapy. The anti-inflammatory feature of hAMSCs, along with their ability to promote neurogenesis and differentiation of progenitor cells to neurons, could make them valuable sources for prevention and therapy in both early and advanced stages of MS. hAMSCs possess other conspicuous characteristics to make them practical sources for cell-based therapies against MS, which are discussed further here in brief. The amniotic membrane, which will be obtained from elective Cesarean sections to eliminate contaminations, is an attractive source of MSCs since large quantities of hAMSCs could be cost-effectively collected without invasive procedures and ethical concerns (67). Although hAMSCs are capable of self-renewal and differentiation to all the three embryonic layers, they are not tumorigenic and thus could be considered safe and easy-to-access sources for cell-based MS therapy (68). As we have shown previously, cryopreservation could be used as a viable system for banking human amniotic cells with low cost in terms of expense, time and personnel involved and its ease of implementation. Therefore, they are suitable for banking and establishing ready-to-use sources for cell therapy (13). Since hAMSCs retain their reproducible biologic characteristics, they can be sufficiently expanded for use in regenerative medicine (69). In addition, the differentiation of hAMSCs to different cell lines relies on growth factors provided in the medium which can be altered in the laboratory. Amniotic stem cells can differentiate to neuron and glial cells (55); therefore, by regulating the growth factors, hAMSCs could potentially be applied in various neurological disorders. Neurological disorders occur among the elderly, and MSCs are affected by the aging process as indicated by the decrease in the bone marrow MSC pool and also reduced capacity to handle oxidative stress (70). Therefore, allograft transplantation of hAMSCs and the ability to provide large quantities of hAMSCs might make them the choice to substitute allogenic source of MSCs among the elderly. hAMSCs have low immunogenicity, which makes them suitable for transplantation (70). hAMSCs have the ability to pass the blood-brain-barrier and enter the central nervous system (71). Thus, they can be administered intravenously and there is no need for more invasive routes of administration. In addition, intravenous administration of hAMSCs enhances their global access to sites of inflammation and damage to secrete the required factors to alleviate MS considering that MS lesions are disseminated in space, meaning that patients have lesions in several areas of the CNS (72). A question that needs to be clarified is whether hAMSCs give the same effect regardless the degree of MS. The pathogenesis of MS is seemingly different in the early and late stages of the disease. There is less inflammation in the brain in primary progressive MS than in secondary progressive MS. In addition, increased blood T-cell reactivity to myelin sheaths was found in relapsing–remitting MS and secondary progressive MS (73). Therefore, anti-inflammatory and immunomodulatory qualities of hAMSCs could be effective in secondary progressive and relapsing–remitting MS. Acute axonal injury occurs focally in secondary progressive MS and diffusely in primary progressive MS (73). Therefore, neurogenesis, neuroprotection, and remyelination imposed by hAMSCs could be of value via systemic administration in primary progressive MS and via local injection in secondary progressive MS. Taken together, the anti-inflammatory feature of hAMSCs, along with their ability to promote neurogenesis and differentiation of progenitor cells to neurons, could make them valuable sources for prevention and therapy in both early and advanced stages of MS.
To the best of our knowledge, no studies have focused on the unique properties of hAMSCs for MS therapy. Therefore, it is essential to investigate the potential therapeutic values of hAMSCs for MS.
Conclusion
hAMSCs and their conditioned medium could be of therapeutic value in MS therapy due to their unique properties including immunomodulation and inflammation suppression; angiogenesis promotion; oxidative stress inhibition; neurogenesis induction and neuroprotection; MMPs regulation; and remyelination stimulation. Therefore, it is required to evaluate the hypothesis in future in vitro and in vivo studies.
Author Contributions
MA-K, S-HG, FS, and HN hypothesized the idea, wrote the manuscript and revised it. SB revised the manuscript.
Conflict of Interest Statement
The authors declare that the research was conducted in the absence of any commercial or financial relationships that could be construed as a potential conflict of interest.
Acknowledgments
Research reported in this publication was supported by Department of Pharmacology of School of Medicine, Shahid Beheshti University of Medical Sciences and Researcher Grant Committee under award number [963951] from the National Institutes for Medical Research Development (NIMAD), Tehran, Iran. Authors would also like to express their most sincere words of appreciation to Professor Heinz Redl, Dr. Susanne Wolbank, and Ms. Tahereh Tayebi for their valuable contribution.
References
1. Loma I, Heyman R. Multiple sclerosis: pathogenesis and treatment. Curr Neuropharmacol. (2011) 9:409–16. doi: 10.2174/157015911796557911
2. Al Jumah MA, Abumaree MH. The immunomodulatory and neuroprotective effects of mesenchymal stem cells (MSCs) in experimental autoimmune encephalomyelitis (EAE): a model of multiple sclerosis (MS). Int J Mol Sci. (2012) 13:9298–331. doi: 10.3390/ijms13079298
3. Compston A, Coles A. Multiple sclerosis. Lancet (2008) 372:1502–17. doi: 10.1016/S0140-6736(08)61620-7
4. Zhang Y, Dong H, Seeburg DP, Wojtkiewicz GR, Waterman P, Pulli B, et al. Multimodal molecular imaging demonstrates myeloperoxidase regulation of matrix metalloproteinase activity in neuroinflammation. Mol Neurobiol. (2018). doi: 10.1007/s12035-018-1137-2. [Epub ahead of print].
5. Ohl K, Tenbrock K, Kipp M. Oxidative stress in multiple sclerosis: central and peripheral mode of action. Exp Neurol. (2016) 277:58–67. doi: 10.1016/j.expneurol.2015.11.010
6. Monti L, Morbidelli L, Rossi A. Impaired cerebral perfusion in multiple sclerosis: relevance of endothelial factors. Biomark Insights (2018) 13:1177271918774800. doi: 10.1177/1177271918774800
7. Sarkar P, Rice CM, Scolding NJ. Cell therapy for multiple sclerosis. CNS Drugs (2017) 31:453–69. doi: 10.1007/s40263-017-0429-9
8. Scolding NJ, Pasquini M, Reingold SC, Cohen JA. Cell-based therapeutic strategies for multiple sclerosis. Brain (2017) 140:2776–96. doi: 10.1093/brain/awx154
9. Niknejad H, Peirovi H, Jorjani M, Ahmadiani A, Ghanavi J, Seifalian AM. Properties of the amniotic membrane for potential use in tissue engineering. Eur Cell Mater. (2008) 15:88–99. doi: 10.22203/eCM.v015a07
10. Tehrani FA, Modaresifar K, Azizian S, Niknejad H. Induction of antimicrobial peptides secretion by IL-1beta enhances human amniotic membrane for regenerative medicine. Sci Rep. (2017) 7:17022. doi: 10.1038/s41598-017-17210-7
11. Yan K, Zhang R, Chen L, Chen F, Liu Y, Peng L, et al. Nitric oxide-mediated immunosuppressive effect of human amniotic membrane-derived mesenchymal stem cells on the viability and migration of microglia. Brain Res. (2014) 1590:1–9. doi: 10.1016/j.brainres.2014.05.041
12. Niknejad H, Paeini-Vayghan G, Tehrani FA, Khayat-Khoei M, Peirovi H. Side dependent effects of the human amnion on angiogenesis. Placenta (2013) 34:340–5. doi: 10.1016/j.placenta.2013.02.001
13. Yazdanpanah G, Paeini-Vayghan G, Asadi S, Niknejad H. The effects of cryopreservation on angiogenesis modulation activity of human amniotic membrane. Cryobiology (2015) 71:413–8. doi: 10.1016/j.cryobiol.2015.09.008
14. Jiao H, Shi K, Zhang W, Yang L, Yang L, Guan F, et al. Therapeutic potential of human amniotic membrane-derived mesenchymal stem cells in APP transgenic mice. Oncol Lett. (2016) 12:1877–83. doi: 10.3892/ol.2016.4857
15. Kim EY, Lee KB, Kim MK. The potential of mesenchymal stem cells derived from amniotic membrane and amniotic fluid for neuronal regenerative therapy. BMB Rep. (2014) 47:135–40. doi: 10.5483/BMBRep.2014.47.3.289
16. Jiang F, Ma J, Liang Y, Niu Y, Chen N, Shen M. Amniotic mesenchymal stem cells can enhance angiogenic capacity via MMPs in vitro and in vivo. Biomed Res Int. (2015) 2015:324014. doi: 10.1155/2015/324014
17. Lopez-Diego RS, Weiner HL. Novel therapeutic strategies for multiple sclerosis–a multifaceted adversary. Nat Rev Drug Discov. (2008) 7:909–25. doi: 10.1038/nrd2358
18. McDonald CA, Payne NL, Sun G, Moussa L, Siatskas C, Lim R, et al. Immunosuppressive potential of human amnion epithelial cells in the treatment of experimental autoimmune encephalomyelitis. J Neuroinflammation (2015) 12:112. doi: 10.1186/s12974-015-0322-8
19. Hoglund RA, Maghazachi AA. Multiple sclerosis and the role of immune cells. World J Exp Med. (2014) 4:27–37. doi: 10.5493/wjem.v4.i3.27
20. Danikowski KM, Jayaraman S, Prabhakar BS. Regulatory T cells in multiple sclerosis and myasthenia gravis. J Neuroinflammation (2017) 14:117. doi: 10.1186/s12974-017-0892-8
21. Payne N, Siatskas C, Bernard CC. The promise of stem cell and regenerative therapies for multiple sclerosis. J Autoimmun. (2008) 31:288–94. doi: 10.1016/j.jaut.2008.04.002
22. Prasanna SJ, Gopalakrishnan D, Shankar SR, Vasandan AB. Pro-inflammatory cytokines, IFNgamma and TNFalpha, influence immune properties of human bone marrow and Wharton jelly mesenchymal stem cells differentially. PLoS ONE (2010) 5:e9016. doi: 10.1371/journal.pone.0009016
23. Magatti M, Vertua E, Cargnoni A, Silini A, Parolini O. The immunomodulatory properties of amniotic cells: the two sides of the coin. Cell Transplant. (2018) 27:31–44. doi: 10.1177/0963689717742819
24. Chiesa S, Morbelli S, Morando S, Massollo M, Marini C, Bertoni A, et al. Mesenchymal stem cells impair in vivo T-cell priming by dendritic cells. Proc Natl Acad Sci USA. (2011) 108:17384–9. doi: 10.1073/pnas.1103650108
25. Magana-Guerrero FS, Dominguez-Lopez A, Martinez-Aboytes P, Buentello-Volante B, Garfias Y. Human amniotic membrane mesenchymal stem cells inhibit neutrophil extracellular traps through TSG-6. Sci Rep. (2017) 7:12426. doi: 10.1038/s41598-017-10962-2
26. Maqbool M, Vidyadaran S, George E, Ramasamy R. Human mesenchymal stem cells protect neutrophils from serum-deprived cell death. Cell Biol Int. (2011) 35:1247–51. doi: 10.1042/CBI20110070
27. Magatti M, Vertua E, De Munari S, Caro M, Caruso M, Silini A, et al. Human amnion favours tissue repair by inducing the M1-to-M2 switch and enhancing M2 macrophage features. J Tissue Eng Regen Med. (2017) 11:2895–911. doi: 10.1002/term.2193
28. Kim KS, Kim HS, Park JM, Kim HW, Park MK, Lee HS, et al. Long-term immunomodulatory effect of amniotic stem cells in an Alzheimer's disease model. Neurobiol Aging (2013) 34:2408–20. doi: 10.1016/j.neurobiolaging.2013.03.029
29. Kang JW, Koo HC, Hwang SY, Kang SK, Ra JC, Lee MH, et al. Immunomodulatory effects of human amniotic membrane-derived mesenchymal stem cells. J Vet Sci. (2012) 13:23–31. doi: 10.4142/jvs.2012.13.1.23
30. Wang M, Yuan Q, Xie L. Mesenchymal stem cell-based immunomodulation: properties and clinical application. Stem Cells Int. (2018) 2018:3057624. doi: 10.1155/2018/3057624
31. Shi Y, Su J, Roberts AI, Shou P, Rabson AB, Ren G. How mesenchymal stem cells interact with tissue immune responses. Trends Immunol. (2012) 33:136–43. doi: 10.1016/j.it.2011.11.004
32. Meesuk L, Tantrawatpan C, Kheolamai P, Manochantr S. The immunosuppressive capacity of human mesenchymal stromal cells derived from amnion and bone marrow. Biochem Biophys Rep. (2016) 8:34–40. doi: 10.1016/j.bbrep.2016.07.019
33. Dabrowski FA, Burdzinska A, Kulesza A, Sladowska A, Zolocinska A, Gala K, et al. Comparison of the paracrine activity of mesenchymal stem cells derived from human umbilical cord, amniotic membrane and adipose tissue. J Obstet Gynaecol Res. (2017) 43:1758–68. doi: 10.1111/jog.13432
34. Gu W, Hong X, Potter C, Qu A, Xu Q. Mesenchymal stem cells and vascular regeneration. Microcirculation (2017) 24. doi: 10.1111/micc.12324
35. Wang Y, Chen X, Yin Y, Li S. Human amnion-derived mesenchymal stem cells induced osteogenesis and angiogenesis in human adipose-derived stem cells via ERK1/2 MAPK signaling pathway. BMB Rep. (2018) 51:194–9. doi: 10.5483/BMBRep.2018.51.4.005
36. Khalaj L, Peirovi H, Khodagholi F, Abdi A, Dargahi L, Ahmadiani A. Acute 17beta-estradiol pretreatment protects against abdominal aortic occlusion induced spinal cord ischemic-reperfusion injury. Neurochem Res. (2011) 36:268–80. doi: 10.1007/s11064-010-0314-0
37. Gilgun-Sherki Y, Melamed E, Offen D. The role of oxidative stress in the pathogenesis of multiple sclerosis: the need for effective antioxidant therapy. J Neurol. (2004) 251:261–8. doi: 10.1007/s00415-004-0348-9
38. van Horssen J, Witte ME, Schreibelt G, de Vries HE. Radical changes in multiple sclerosis pathogenesis. Biochim Biophys Acta (2011) 1812:141–50. doi: 10.1016/j.bbadis.2010.06.011
39. Liu Y, Hao W, Letiembre M, Walter S, Kulanga M, Neumann H, et al. Suppression of microglial inflammatory activity by myelin phagocytosis: role of p47-PHOX-mediated generation of reactive oxygen species. J Neurosci. (2006) 26:12904–13. doi: 10.1523/JNEUROSCI.2531-06.2006
40. Forte M, Gold BG, Marracci G, Chaudhary P, Basso E, Johnsen D, et al. Cyclophilin D inactivation protects axons in experimental autoimmune encephalomyelitis, an animal model of multiple sclerosis. Proc Natl Acad Sci USA. (2007) 104:7558–63. doi: 10.1073/pnas.0702228104
41. Ortiz GG, Pacheco-Moises FP, Bitzer-Quintero OK, Ramirez-Anguiano AC, Flores-Alvarado LJ, Ramirez-Ramirez V, et al. Immunology and oxidative stress in multiple sclerosis: clinical and basic approach. Clin Dev Immunol. (2013) 2013:708659. doi: 10.1155/2013/708659
42. Lanza C, Morando S, Voci A, Canesi L, Principato MC, Serpero LD, et al. Neuroprotective mesenchymal stem cells are endowed with a potent antioxidant effect in vivo. J Neurochem. (2009) 110:1674–84. doi: 10.1111/j.1471-4159.2009.06268.x
43. Bermel RA. Unravelling neurodegeneration in multiple sclerosis. Lancet Neurol. (2017) 16:764–6. doi: 10.1016/S1474-4422(17)30302-2
44. Su K, Bourdette D, Forte M. Mitochondrial dysfunction and neurodegeneration in multiple sclerosis. Front Physiol. (2013) 4:169. doi: 10.3389/fphys.2013.00169
45. Lee JY, Taghian K, Petratos S. Axonal degeneration in multiple sclerosis: can we predict and prevent permanent disability? Acta Neuropathol Commun. (2014) 2:97. doi: 10.1186/s40478-014-0097-7
46. Glenn JD, Smith MD, Kirby LA, Baxi EG, Whartenby KA. Disparate Effects of mesenchymal stem cells in experimental autoimmune encephalomyelitis and cuprizone-induced demyelination. PLoS ONE (2015) 10:e0139008. doi: 10.1371/journal.pone.0139008
47. El Waly B, Macchi M, Cayre M, Durbec P. Oligodendrogenesis in the normal and pathological central nervous system. Front Neurosci. (2014) 8:145. doi: 10.3389/fnins.2014.00145
48. Kremer D, Gottle P, Hartung HP, Kury P. Pushing Forward: Remyelination as the New Frontier in CNS Diseases. Trends Neurosci. (2016) 39:246–63. doi: 10.1016/j.tins.2016.02.004
49. Rivera FJ, Aigner L. Adult mesenchymal stem cell therapy for myelin repair in multiple sclerosis. Biol Res. (2012) 45:257–68. doi: 10.4067/S0716-97602012000300007
50. Pomeroy IM, Jordan EK, Frank JA, Matthews PM, Esiri MM. Focal and diffuse cortical degenerative changes in a marmoset model of multiple sclerosis. Mult Scler. (2010) 16:537–48. doi: 10.1177/1352458509360362
51. Shu J, He X, Li H, Liu X, Qiu X, Zhou T, et al. The beneficial effect of human amnion mesenchymal cells in inhibition of inflammation and induction of neuronal repair in EAE mice. J Immunol Res. (2018) 2018:5083797. doi: 10.1155/2018/5083797
52. Hempstead BL. Dissecting the diverse actions of pro- and mature neurotrophins. Curr Alzheimer Res. (2006) 3:19–24. doi: 10.2174/156720506775697061
53. Reichardt LF. Neurotrophin-regulated signalling pathways. Philos Trans R Soc Lond B Biol Sci. (2006) 361:1545–64. doi: 10.1098/rstb.2006.1894
54. Zhou H, Zhang H, Yan Z, Xu R. Transplantation of human amniotic mesenchymal stem cells promotes neurological recovery in an intracerebral hemorrhage rat model. Biochem Biophys Res Commun. (2016) 475:202–8. doi: 10.1016/j.bbrc.2016.05.075
55. Sanluis-Verdes A, Sanluis-Verdes N, Manso-Revilla MJ, Castro-Castro AM, Pombo-Otero J, Fraga-Marino M, et al. Tissue engineering for neurodegenerative diseases using human amniotic membrane and umbilical cord. Cell Tissue Bank (2017) 18:1–15. doi: 10.1007/s10561-016-9595-0
56. Chen X, Ma L, Jiang Y, Chen S, Zhu C, Liu M, et al. Minocycline up-regulates the expression of brain-derived neurotrophic factor and nerve growth factor in experimental autoimmune encephalomyelitis. Eur J Pharmacol. (2012) 686:124–9. doi: 10.1016/j.ejphar.2012.04.043
57. Modi KK, Sendtner M, Pahan K. Up-regulation of ciliary neurotrophic factor in astrocytes by aspirin: implications for remyelination in multiple sclerosis. J Biol Chem. (2013) 288:18533–45. doi: 10.1074/jbc.M112.447268
58. Rennie K, Gruslin A, Hengstschlager M, Pei D, Cai J, Nikaido T, et al. Applications of amniotic membrane and fluid in stem cell biology and regenerative medicine. Stem Cells Int. (2012) 2012:721538. doi: 10.1155/2012/721538
59. Gerwien H, Hermann S, Zhang X, Korpos E, Song J, Kopka K, et al. Imaging matrix metalloproteinase activity in multiple sclerosis as a specific marker of leukocyte penetration of the blood-brain barrier. Sci Transl Med. (2016) 8:364ra152. doi: 10.1126/scitranslmed.aaf8020
60. Sorokin L. The impact of the extracellular matrix on inflammation. Nat Rev Immunol. (2010) 10:712–23. doi: 10.1038/nri2852
61. Fissolo N, Pignolet B, Matute-Blanch C, Trivino JC, Miro B, Mota M, et al. Matrix metalloproteinase 9 is decreased in natalizumab-treated multiple sclerosis patients at risk for progressive multifocal leukoencephalopathy. Ann Neurol. (2017) 82:186–95. doi: 10.1002/ana.24987
62. Kay AG, Long G, Tyler G, Stefan A, Broadfoot SJ, Piccinini AM, et al. Mesenchymal stem cell-conditioned medium reduces disease severity and immune responses in inflammatory arthritis. Sci Rep. (2017) 7:18019. doi: 10.1038/s41598-017-18144-w
63. Lozito TP, Tuan RS. Mesenchymal stem cells inhibit both endogenous and exogenous MMPs via secreted TIMPs. J Cell Physiol. (2011) 226:385–96. doi: 10.1002/jcp.22344
64. Chelluboina B, Nalamolu KR, Mendez GG, Klopfenstein JD, Pinson DM, Wang DZ, et al. Mesenchymal stem cell treatment prevents post-stroke dysregulation of matrix metalloproteinases and tissue inhibitors of metalloproteinases. Cell Physiol Biochem. (2017) 44:1360–9. doi: 10.1159/000485533
65. Liu L, Eckert MA, Riazifar H, Kang DK, Agalliu D, Zhao W. From blood to the brain: can systemically transplanted mesenchymal stem cells cross the blood-brain barrier? Stem Cells Int. (2013) 2013:435093. doi: 10.1155/2013/435093
66. Nessler J, Benardais K, Gudi V, Hoffmann A, Salinas Tejedor L, Janssen S, et al. Effects of murine and human bone marrow-derived mesenchymal stem cells on cuprizone induced demyelination. PLoS ONE (2013) 8:e69795. doi: 10.1371/journal.pone.0069795
67. Miyamoto S, Ohnishi S, Onishi R, Tsuchiya I, Hosono H, Katsurada T, et al. Therapeutic effects of human amnion-derived mesenchymal stem cell transplantation and conditioned medium enema in rats with trinitrobenzene sulfonic acid-induced colitis. Am J Transl Res. (2017) 9:940–52.
68. Phermthai T, Thongbopit S, Pokathikorn P, Wichitwiengrat S, Julavijitphong S, Tirawanchai N. Carcinogenicity, efficiency and biosafety analysis in xeno-free human amniotic stem cells for regenerative medical therapies. Cytotherapy (2017) 19:990–1001. doi: 10.1016/j.jcyt.2017.04.004
69. Miranda-Sayago JM, Fernandez-Arcas N, Benito C, Reyes-Engel A, Carrera J, Alonso A. Lifespan of human amniotic fluid-derived multipotent mesenchymal stromal cells. Cytotherapy (2011) 13:572–81. doi: 10.3109/14653249.2010.547466
70. Wang Y, Yin Y, Jiang F, Chen N. Human amnion mesenchymal stem cells promote proliferation and osteogenic differentiation in human bone marrow mesenchymal stem cells. J Mol Histol. (2015) 46:13–20. doi: 10.1007/s10735-014-9600-5
71. Smith CL, Chaichana KL, Lee YM, Lin B, Stanko KM, O'Donnell T, et al. Pre-exposure of human adipose mesenchymal stem cells to soluble factors enhances their homing to brain cancer. Stem Cells Transl Med. (2015) 4:239–51. doi: 10.5966/sctm.2014-0149
72. Hackett C, Knight J, Mao-Draayer Y. Transplantation of Fas-deficient or wild-type neural stem/progenitor cells (NPCs) is equally efficient in treating experimental autoimmune encephalomyelitis (EAE). Am J Transl Res. (2014) 6:119–28.
Keywords: angiogenesis, anti-inflammation, antioxidant, amniotic membrane, mesenchymal stem cell, multiple sclerosis, neurogenesis
Citation: Abbasi-Kangevari M, Ghamari S-H, Safaeinejad F, Bahrami S and Niknejad H (2019) Potential Therapeutic Features of Human Amniotic Mesenchymal Stem Cells in Multiple Sclerosis: Immunomodulation, Inflammation Suppression, Angiogenesis Promotion, Oxidative Stress Inhibition, Neurogenesis Induction, MMPs Regulation, and Remyelination Stimulation. Front. Immunol. 10:238. doi: 10.3389/fimmu.2019.00238
Received: 15 October 2018; Accepted: 28 January 2019;
Published: 20 February 2019.
Edited by:
Jorge Matias-Guiu, Complutense University of Madrid, SpainReviewed by:
Marwa M. Mahfouz, Menofia University, EgyptLaura Leyva Fernández, University of Málaga, Spain
Copyright © 2019 Abbasi-Kangevari, Ghamari, Safaeinejad, Bahrami and Niknejad. This is an open-access article distributed under the terms of the Creative Commons Attribution License (CC BY). The use, distribution or reproduction in other forums is permitted, provided the original author(s) and the copyright owner(s) are credited and that the original publication in this journal is cited, in accordance with accepted academic practice. No use, distribution or reproduction is permitted which does not comply with these terms.
*Correspondence: Soheyl Bahrami, c29oZXlsLmJhaHJhbWlAdHJhdW1hLmxiZy5hYy5hdA==
Hassan Niknejad, bmlrbmVqYWRAc2JtdS5hYy5pcg==
†These authors share first authorship