- 1Department of Obstetrics and Gynecology, College of Medicine, Konyang University, Daejeon, South Korea
- 2Laboratory Animal Resource Centre, Daegu Gyeongbuk Institute of Science & Technology (DGIST), Daegu, South Korea
- 3Laboratory Animal Medicine, College of Veterinary Medicine, Chonnam National University, Gwangju, South Korea
- 4Myunggok Medical Research Institute, College of Medicine, Konyang University, Daejeon, South Korea
- 5Korean Collection for Type Cultures, Biological Resource Center, Korea Research Institute of Bioscience and Biotechnology, Daejeon, South Korea
Endometriosis is a chronic gynecological disorder, characterized by the presence of ectopic endometrial tissue outside the uterine cavity. Among several hypotheses, Sampson's theory of retrograde menstruation is still applicable. Recent studies have reported the importance of inflammation among endometrial tissue, the peritoneum, and immune cells. However, less is known regarding the role of bacterial infection in the pathophysiology of endometriosis. We hypothesized that Ureaplasma urealyticum infection might contribute to the development of endometriosis by inducing the production of inflammatory mediators by peritoneal mesothelial cells (PMCs), possibly through TLR2. Hence, our objective was to reveal whether PMC infection by U. urealyticum is associated with endometriosis. Moreover, we aimed to demonstrate the molecular mechanism involved in this relationship. We developed a new infection-induced mouse model of endometriosis with wild type and Tlr2-deficient mice. Based on the in vivo mouse model, U. urealyticum-infected mice showed significantly increased numbers and sizes of ectopic endometriotic lesions. U. urealyticum upregulated not only the production of IL-6, CXCL1, and CCL2, but also the expression of ICAM-1, VCAM-1, and MMP2 in murine PMCs. Similarly, endometrial stromal cells dose-dependently produced IL-6, CXCL1, and CCL2 in response to U. urealyticum infection. The series of inflammatory responses in PMCs was mediated mainly through TLR2. The phosphorylation of ERK and JNK was observed when U. urealyticum was added to PMCs and knock out of Tlr2 inhibited these MAPKs phosphorylation. Based on our co-culture study, U. urealyticum-infected PMCs exhibited significantly increased attachment to ESCs compared with uninfected PMCs. Collectively, U. urealyticum infection promotes the development of endometriosis by increasing inflammatory mediators, adhesion molecules, and MMP-2 expression in PMCs through TLR2 signaling. Through our results, we present a theory that infection-induced pelvic inflammation contributes to the initiation and progression of endometriosis. Appropriate treatment of reproductive tract infection may decrease the prevalence of endometriosis.
Introduction
Endometriosis is a chronic gynecological disorder, characterized by the presence of ectopic endometrial tissue outside the uterine cavity; it affects 6–10% of women of reproductive age (1). Further, its frequency in women with chronic pelvic pain or infertility ranges from 35 to 50% (1).
Among several hypotheses, Sampson's theory of retrograde menstruation is still applicable (2). Some other theories such as alteration of response to estrogen and progesterone, endometrial stem cell implantation, Müllerian remnant abnormalities, and coelomic metaplasia have postulated (3, 4). Recent studies have also reported the importance of interactions among endometrial tissue, the peritoneum, and immune cells during development into endometriosis (5–7). In the early development stage of endometriosis, acute inflammatory responses and tissue breakdown were induced by pro-inflammatory cytokines such as interleukin (IL)-6, IL-1β, interferon (IFN)-γ, and tumor necrosis factor (TNF). As inflammation progresses, tissue remodeling and repair, endometrial cell proliferation, angiogenesis, neurogenesis, and fibrosis are followed under the influence of regulatory T cells and T helper 2 cells (7–10).
According to recent studies, the menstrual blood of women with endometriosis is more frequently contaminated with Escherichia coli than that of controls, which corresponded to higher levels of endotoxin in the menstrual fluid (11, 12). In addition, lipopolysaccharide (LPS) promotes the proliferation and invasion of human endometrial stromal cells via the upregulation of cyclooxygenase-2 (COX-2) and prostaglandin E2 (PGE2), which can result in development into endometriosis (13). Moreover, based on an epidemiological study performed in Taiwan, endometriosis is more prevalent in women with low genital tract infection of the cervix, vagina, and vulva compared to that in women without disease (14).
Ureaplasma urealyticum is a gram-negative bacterium belonging to the family Mycoplasmataceae that has no cell wall. This species is an important opportunistic pathogen commonly found in the reproductive organs of sexually active females, and its prevalence ranges from 60 to 80% worldwide (15–17). U. urealyticum is involved in a variety of infectious diseases such as non-gonococcal urethritis, male infertility, bacterial vaginosis, chronic endometritis, pelvic inflammatory disease, spontaneous abortion, premature birth, and chorioamnionitis (18–21). However, the role of this bacterium in the progression of endometriosis has not been described.
The peritoneum, a common ectopic endometrial implantation site, is composed of a wide monolayer of mesothelial cells. Peritoneal mesothelial cells (PMCs) cover the body's serous cavity and internal organs (22). These PMCs participate in diverse cellular processes including tumor cell adhesion, tissue repair, inflammation, and host defense (22, 23). The sensing of bacterial pathogens in PMCs is mediated by some Toll-like receptors (TLRs) including TLR2 (23) which recognizes a molecular pattern of U. urealyticum (15, 24, 25). Further, in humans and mice, stimulated PMCs secrete several CC and CXC chemokines and cytokines including CXCL1/KC, CCL2/MCP-1, and IL-6 (26–29).
Based on this previous knowledge, we hypothesized that U. urealyticum infection might contribute to the development of endometriosis by inducing the production of inflammatory mediators by PMCs, possibly through TLR2. Hence, our objective was to reveal whether PMC infection by U. urealyticum is associated with endometriosis. Moreover, we aimed to demonstrate the molecular mechanism involved in the development of endometriosis.
Materials and Methods
Mice
Wild-type (WT) C57BL/6J female mice were purchased from DBL (Eumseong, South Korea). Tlr2-deficient female mice in a C57BL/6 background were purchased from Jackson Laboratories (Bar Harbor, ME, USA). The animals were housed in an animal room at a constant temperature (22–24°C) and light–dark cycle with 14 h of light and 10 h of dark. Food and water were available ad libitum. Mice were acclimatized to the laboratory room for 1 week before the experiment. Mice were sacrificed by cervical dislocation; female C57BL/6J (n = 92) and Tlr2-deficient mice (n = 31) of 4–8 weeks of age were used for this study. Animal studies were approved and conducted according to the regulations of the Institutional Animal Care and Use Committee (IACUC; approved protocol number: P-17-09-E-01) at Konyang University (Daejeon, Korea).
U. urealyticum Culture
Ureaplasma urealyticum (ATCC 27618) was reconstituted in American Type Culture Collection media 2616, in accordance with the instructions provided by the American Type Culture Collection. Bacteria were incubated under anaerobic conditions at 37°C until the medium changed from yellow to pink–red. The color change indicates U. urealyticum growth. After 12 h of color change, bacteria were gently pelleted and resuspended in growth medium. The bacterial concentrations of the suspensions were adjusted to 1 × 104 CFU/mL using the Mycoplasma IST-2 kit (BioMerieux, Marcy l'Etoile, France), according to the manufacturer's instructions (30). Next, we identified colonies using A7 Mycoplasma Agar (BioMerieux) to determine the exact number of bacteria. U. urealyticum was incubated on A7 Mycoplasma Agar for 24 h under anaerobic conditions using gaspak EZ large incubation (BD Biosciences, San Jose, CA, USA). The growth of U. urealyticum was confirmed microscopically. Colonies of U. urealyticum appeared granular and dark brown. At the end of culture, U. urealyticum-positive plates changed color from orange to red, and the presence of U. urealyticum colonies was morphologically confirmed under a microscope.
Mouse Model of Endometriosis
We developed an animal model of endometriosis by modifying the method of Hirata et al. (31). Briefly, both 6- to 8-week-old donor (WT mice, n = 48) and recipient female mice (WT, n = 24 and Tlr2-deficient mice, n = 24) were primed with pregnant mare serum gonadotropin (PMSG) (Prospec, East Brunswick, NJ, USA) at 5 IU/mouse for 48 h to stimulate endometrial growth and synchronize the estrus cycle. After 48 h, the donor mouse was sacrificed by cervical dislocation and the uterine horns were removed and put in a dish containing cold PBS. After peeling off the serosa and myometrium gently, endometrial tissue was minced with a razor blade. The endometrial fragments were suspended in 0.5 mL PBS and injected into the peritoneal cavities of recipient mice with an 18-gauge needle at a 1:1 ratio (donor to recipient). Three days before the induction of endometriosis, the recipients (16 uninfected mice: 8 WT and 8 Tlr2−/− mice, each and 32 U. urealyticum infected mice: 16 WT and 16 Tlr2−/− mice, each) were injected with bacteria (1 × 106/200 μL/mouse) into the peritoneal cavities. This injection was repeated every 3 days until the 27th day after endometrial cell injection, 11 times in total (Figure 1A). The recipient mice were euthanized 30 days after the induction of endometriosis. The number, location, and sizes of ectopic lesions were assessed under a dissecting microscope, which was followed by histological evaluation. Sizes of ectopic lesions were measured based on two perpendicular diameters using calipers. The lesion volume was calculated using the formula V = (4/3)πr12 r2 (r1 and r2 are radii, r1 < r2, as described previously) (32).
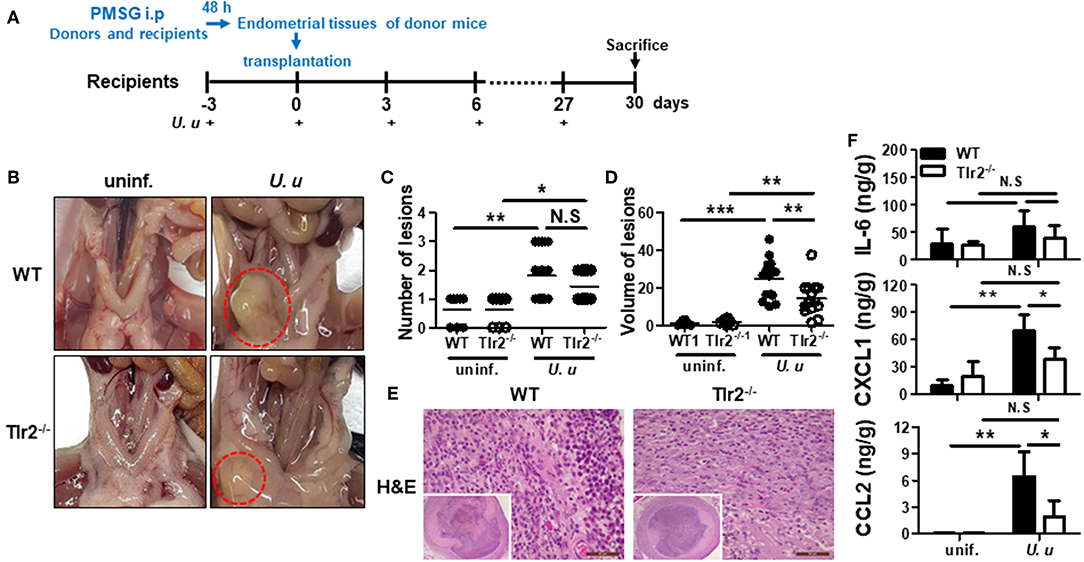
Figure 1. Experimental design and characterization of ectopic lesions in a mouse model of endometriosis induced by U. urealyticum infection. The experimental design of the endometriosis mouse model is shown. Sixteen uninfected mice were used in total; 8 WT and 8 Tlr2−/− mice, each. Also, 32 U. urealyticum infected mice were used; 16 WT and 16 Tlr2−/− mice, each (A). Images show the ectopic lesions harvested 30 days after the induction of endometriosis (B). Dotted lines indicate the ectopic lesions near the uterus (B). The average numbers and volume of the ectopic lesions are shown (C,D). H&E stain images (40x and 400x) are also presented (E). The concentrations of IL-6, CXCL1, and CCL2 in culture supernatants of harvested ectopic endometriotic lesions were measured by ELISA (F). All experiments were analyzed by a t-test and expressed as the mean ± SD. *P < 0.05; **P < 0.01; ***P < 0.001. −/−, knockout; N.S, not significant; uninf., uninfected; U. u, U. urealyticum.
Histological Staining
Formalin-fixed specimens (uterine tissues and ectopic lesions) obtained from the modeled mice were paraffin-embedded, cut into 4-μm sections, and stained with hematoxylin and eosin stain (H&E stain). Sections were examined for the presence of histologic hallmarks of endometriosis under a microscope.
Preparation and Stimulation of Mouse Peritoneal Mesothelial Cells
PMCs were isolated mainly from the peritoneum and some from external surfaces of the liver, spleen, and kidneys of 4-week-old WT (n = 12) and Tlr2-deficient (n = 7) female mice as described previously (27). This method can reduce the sacrificed number of PMC donor mice. Briefly, pieces of tissue were obtained from sacrificed mice and washed by injecting ~50 mL of phosphate-buffered saline (PBS; pH 7.4) and digested with 0.25% trypsin/EDTA solution (Gibco, Basel, Switzerland) for 50 min at 37°C. After discarding intact tissues and tissue debris, the cell suspension was centrifuged at 500 × g for 5 min. The pellet was resuspended in Dulbecco's modified Eagle's medium (DMEM; Invitrogen, Grand Island, NY, USA) supplemented with 15% FBS (Invitrogen), 2 mM L-glutamine (Gibco), and 1% penicillin–streptomycin (Gibco) and cultured overnight. The next day, floating cells were removed and adherent cells were cultured for 3 additional days. PMCs were seeded in 48-well plates at a concentration of 2 × 105 cells/well or in 6-well plates at a concentration of 1 × 106 cells/well and incubated in a 5% CO2 incubator at 37°C. The day after seeding, cells were treated with Pam3CSK4 (InvivoGen, San Diego, CA, USA), LPS (InvivoGen), or U. urealyticum at the indicated multiplicity of infection (MOI).
Enzyme-Linked Immunosorbent Assay
The concentrations of IL-6, CXCL1, and CCL2 in culture supernatants of PMCs, endometrial stromal cells (ESCs), and ectopic lesions were determined using a commercial enzyme-linked immunosorbent assay (ELISA) kit (R&D Systems, Minneapolis, MN, USA) and a 450 nm plate reader (Bio Tek, Winooski, VT, USA).
Western Blotting
PMCs were lysed in ice-cold RIPA buffer (Elpis Biotech, Daejeon, Korea) containing a protease inhibitor cocktail (Thermo Fisher Scientific, Waltham, Massachusetts, USA) and phosphatase inhibitor (Thermo Fisher Scientific). For cell lysates, standard western blotting was performed (33) as described using antibodies against MMP-2 and total and phospho-ERK, JNK, and p-38 (Cell Signaling Technology, Beverly, MA, USA). Immunofluorescent-labeled antibody to rabbit IgG (Cell Signaling Technology) was used to visualize western blots with the Fusion solo S chemidoc (Vilber, Collégien, France).
Flow Cytometry
PMCs were seeded in 6-well plates at a concentration of 1 × 106 cells/well and were infected with U. urealyticum (MOI 0.1) for 6, 12, and 24 h. Cells were stained with the following monoclonal antibodies (mAbs; BioLegend Inc., San Diego, CA, USA): fluorescein isothiocyanate (FITC)-conjugated anti- intercellular adhesion molecules-1 (ICAM-1; CD54) and anti- vascular cell adhesion molecule-1 (VCAM-1; CD106) mAbs (0.25 μg/106 cells in 100 μL, for each). Isotype-matched controls were run in parallel. A total of 10,000 cells were counted. Viable lymphocytes were gated based on their forward- and side-scatter profile. The samples were acquired using a BD FACSCalibur Flow Cytometer (BD Biosciences) and the data were analyzed using BD CellQuest Pro software (BD Biosciences).
Attachment Assay and Confocal Microscopy
PMCs labeled with CellTracker Orange (Molecular Probes, Eugene, OR, USA) at 10 μM for 30 min were seeded in 35-mm confocal dishes (SPL, hole size; 20 mm) at a concentration of 1.5 × 106 cells/well, and incubated in a 5% CO2 incubator at 37°C. The day after seeding, cells were stimulated with U. urealyticum at an MOI of 0.1 for 12 h. PMCs were washed twice with PBS to remove uninfected bacteria. For the co-culture system, ESCs were stained with CellTracker Green (Molecular Probes) at 10 μM for 30 min and seeded in dishes with prepared PMCs at a concentration of 1 × 105 cells/well. These cells were then incubated for three different amounts of time, specifically 15, 30, and 60 min. The cells were fixed with 4% paraformaldehyde (Sigma-Aldrich, St. Louis, MO, USA) for 10 min and then analyzed using a confocal microscope, LSM 700 (Zeiss, Jena, Germany) and ZEN 2012 black edition software.
In addition, we performed attachment assays with an immortalized human mesothelial cell line, MeT-5A (a kind gift from Dr. D.Y. Kim at Seoul National University, Seoul, Korea), and an immortalized human endometrial stromal cell line, T-HESCs (ATCC CRL-4003). These cells were stained with CellTracker Orange (MeT-5A) and CellTracker Green (T-HESC), respectively. We treated MeT-5A cells with Pam3CSK4 (1 μg/mL) instead of U. urealyticum. The cells were processed as described previously herein.
Isolation and Culture of Mouse Endometrial Stromal Cells
Endometrial cells were isolated from the uterus of WT female mice at 5–6 weeks of age. First, uteri were removed and cut lengthwise (34). The uteri from 8 mice were pooled and incubated with 0.25% trypsin (Gibco) and 2.5% pancreatin (Sigma-Aldrich) for 60 min at 4°C and 60 min at 22°C. Following transfer to 15 mL ice-cold (4°C) Hank's Balanced Salt Solution (HBSS; Gibco), digested uteri were vortexed to release epithelial cells. Uterine tissues were rinsed and vortexed additionally three times, and the resulting cell suspensions were pooled. After the removal of epithelial cells, the pooled uteri were incubated for 30 min at 37°C in HBSS with 0.02% EDTA, 0.05% trypsin, and 400 U/mL DNase (Gibco). After adding 2 mL FBS, the cell suspension was filtered through a 40-micron nylon mesh (Falcon) to remove debris and centrifuged at 500 × g for 10 min. The cells were resuspended in DMEM (without phenol red)/Hams F-12 nutrient mixed 1:1, supplemented with 20 mM Hepes, 100 mg/mL streptomycin, 100 U/mL penicillin, 2 mM L-glutamine, and 10% FBS (all from Gibco). After 4 days of culture, non-adherent cells were removed by changing the medium at 48 h intervals, and these adherent ESCs were used for ELISA and co-culture studies.
Statistical Analysis
The results of ELISA, western blotting, and flow cytometry were analyzed using repeated measures ANOVA, followed by multiple comparisons using Tukey's procedure. The average numbers and volume of the ectopic lesions were compared between two groups using t-test. Statistical analysis of the data was performed using GraphPad Prism version 5.00 (GraphPad Software, Inc., La Jolla, CA, USA). Data are presented as mean ± standard deviation (SD). Values of P < 0.05 or less were considered statistically significant.
Results
U. urealyticum Infection Enhances the Development of Ectopic Lesions in a Mouse Model
To verify the hypothesis that U. urealyticum infection in the peritoneal cavity is involved in the development of endometriosis, PMSG was injected into the peritoneal cavity of donor and recipient mice synchronized in their estrous cycles. U. urealyticum was injected into the peritoneal cavity of recipient mice at 3-day intervals from 3 days before endometriosis induction (Figure 1A). When inspecting the peritoneal cavity, there was no evidence of severe acute inflammation such as adhesions of intraperitoneal organs and abscess formation. Endometriosis-like lesions developed near the uterus (Figure 1B). Further, more ectopic lesions developed in the group infected with U. urealyticum than in the uninfected group (P < 0.01), but there was no difference between infected WT and Tlr2-deficient mice (Figure 1C). The volume of these ectopic lesions was significantly larger in the group infected with U. urealyticum than in the uninfected groups comprising WT and Tlr2-deficient mice (P < 0.001 and 0.01, respectively; Figure 1D). In particular, the volumes of ectopic lesions were larger in WT mice than in Tlr2-deficient mice (P < 0.01; Figure 1D).
Next, to further characterize the ectopic lesions obtained from our mouse model of endometriosis, lesions were subjected to histological examination by H&E stain. As shown in Figure 1E, these lesions were filled with inflammatory cells and luminal epithelial cell debris.
Thus, we investigated the inflammatory response in ectopic lesions caused by U. urealyticum infection. As a result, infected WT mice produced higher levels of CXCL1 and CCL2 than uninfected WT (P < 0.01, for each) and infected Tlr2-deficient (P < 0.05, for each) mice (Figure 1F). However, there was no significant difference in IL-6 production of infected WT mice compared to those of uninfected WT and infected Tlr2-deficient mice. From this endometriosis model, we also found that the intraperitoneal infection of U. urealyticum results in the development of ectopic lesions.
U. urealyticum Induces the Production of IL-6, CXCL1, and CCL2 in PMCs and Endometrial Stromal Cells
PMCs were stimulated with U. urealyticum at MOIs of 0.01, 0.05, and 0.1 for three different durations (6, 12, and 24 h) and a cytokine and chemokines were measured by ELISA. U. urealyticum infection led to the dose-dependent production of IL-6, CXCL1, and CCL2 in PMCs at every time point (P < 0.01 or < 0.001, at an MOI 0.1) (Supplementary Figure 1). Similarly, when ESCs were stimulated with different MOIs (0.01–0.1) of U. urealyticum for 24 h, the productions of IL-6, CXCL1, and CCL2 were significantly increased in a dose-dependent manner as compared with uninfected ESCs (Supplementary Figure 2). This indicates that U. urealyticum can induce an inflammatory response by PMCs and ESCs.
U. urealyticum Increases the Secretion of IL-6, CXCL1, and CCL2 in PMCs via the TLR2-Dependent Pathway
Previous reports showed that U. urealyticum possesses molecular patterns that can be recognized by TLR2. We thus investigated whether U. urealyticum could promote cytokine and chemokine production by PMCs via TLR2 activation.
First, we compared the characteristics of PMCs obtained from WT and Tlr2-deficient mice. WT and Tlr2-deficient PMCs were treated with two types of TLR ligands (TLR2 ligand, Pam3CSK4; TLR4 ligand, LPS). Regarding the IL-6, CXCL1, and CCL2 production, Pam3CSK4 increased the secretion of all three molecules from WT PMCs, but not from Tlr-2 deficient PMCs (P < 0.001, for each). In contrast, there was no significant difference in the production of IL-6, CXCL1, and CCL2 after LPS stimulation between WT and Tlr2-deficient PMCs (Figure 2A). These results suggested that Tlr2-deficient PMCs are deficient in TLR2 signaling and WT PMCs produce significant levels of inflammatory mediators upon stimulation by Pam3CSK4.
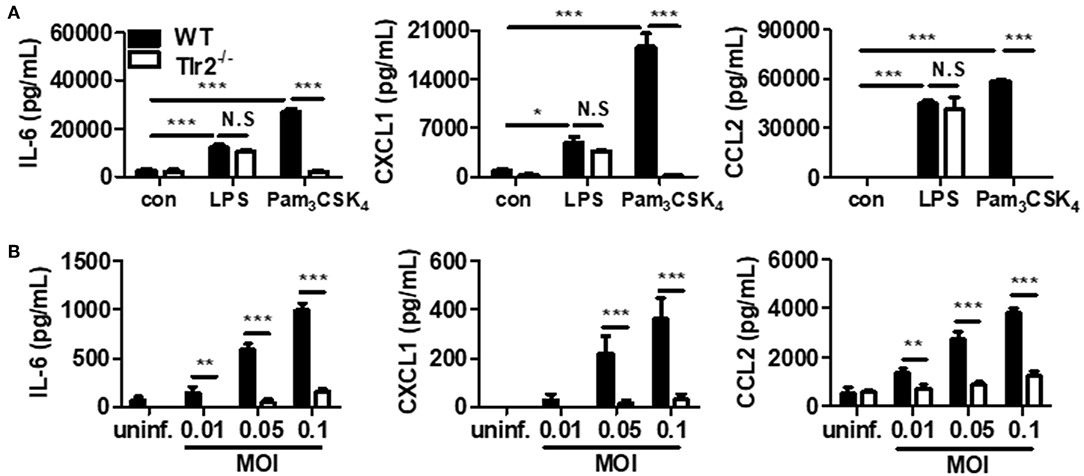
Figure 2. U. urealyticum increases the production of cytokines and chemokines in PMCs via TLR2. WT and Tlr2-deficient PMCs were incubated with Pam3CSK4 (100 ng/mL) and LPS (10 ng/mL) for 24 h (A). Different MOIs of U. urealyticum were applied and PMCs were incubated for 6 h (B). The concentrations of IL-6, CXCL1, and CCL2 in the culture supernatant were measured by ELISA. Data are shown as the mean ± SD of triplicate samples from one experiment representative of three independent experiments (*P < 0.05; **P < 0.01; ***P < 0.001). −/−, knockout; con, control; uninf., uninfected; N.S, not significant.
Next, we compared the production of a cytokine and chemokines between WT and Tlr2-deficient PMCs upon U. urealyticum infection. After 6 h of infection, the production of IL-6, CXCL1, and CCL2 increased in WT PMCs, but not in Tlr2-deficient PMCs (P < 0.001, for each at MOIs of 0.05 and 0.1; Figure 2B). Further, upon U. urealyticum infection at an MOI 0.01, the production of IL-6 and CCL2, but not CXCL1, was elevated (P < 0.01, each). These observations indicated that U. urealyticum infection induces a pro-inflammatory response in PMCs via the TLR2-dependent pathway.
U. urealyticum Activates ERK and JNK Pathways in PMCs
In response to bacterial infection, TLRs of the innate immune system release inflammatory mediators via the mitogen-activated protein kinase (MAPK) signaling pathways (35). To investigate whether MAPK signaling is involved, we infected WT and Tlr2-deficient PMCs with U. urealyticum at an MOI of 0.1. In WT PMCs, ERK was phosphorylated at 30, 60, and 120 min, whereas JNK was phosphorylated at 120 min post-infection. However, ERK and JNK phosphorylation was significantly abolished in Tlr2-deficient PMCs (Figures 3A,B). There were no significant differences in p38 phosphorylation between WT and Tlr2-deficient PMCs in response to U. urealyticum infection (Figure 3A). Next, we performed an inhibitor assay to determine whether ERK and JNK pathways are important to induce pro-inflammatory conditions in PMCs exposed to U. urealyticum. Inhibitors of ERK (PD98059) and JNK (SP600125) suppressed the production of IL-6, CXCL1, and CCL2 in U. urealyticum-infected WT PMCs (Figure 3C). These results indicated that U. urealyticum enhances the production of IL-6, CXCL1, and CCL2 via the ERK and JNK pathways in PMCs.
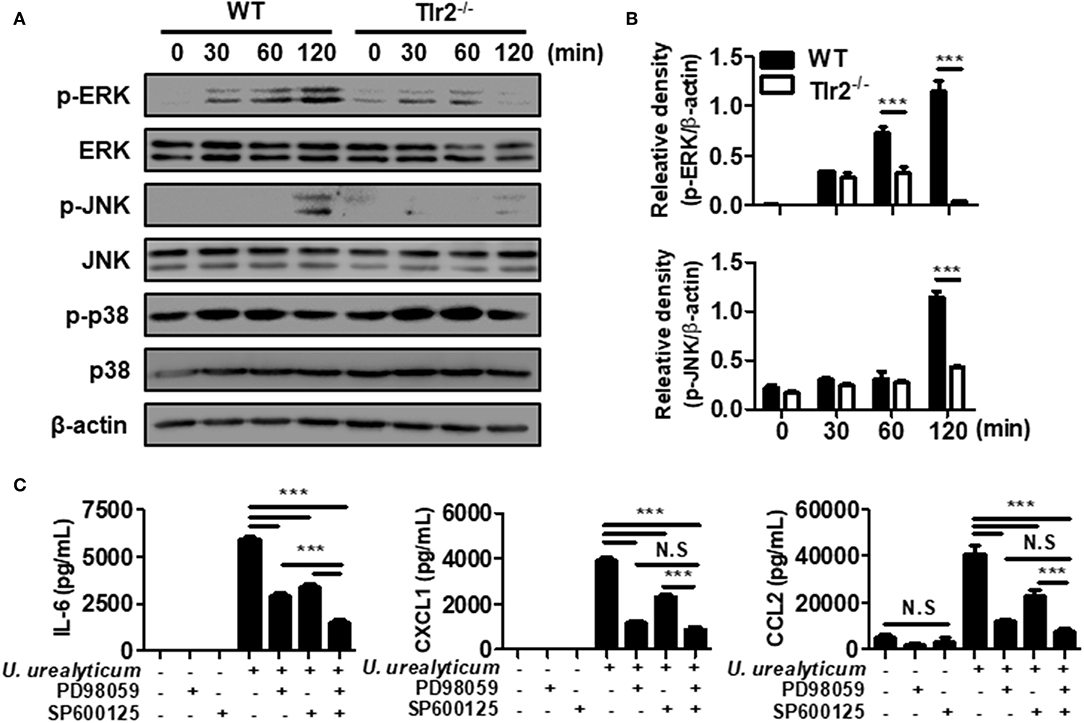
Figure 3. U. urealyticum enhances the activation of MAPKs in PMCs. WT and Tlr2-deficient PMCs were infected with U. urealyticum at an MOI of 0.1, and protein was extracted at the indicated time points. The phosphorylation of ERK, JNK, and p-38 was analyzed by western blotting (A). The relative density of ERK and JNK phosphorylation following U. urealyticum infection was compared to that of β-actin (B). After pre-treating WT PMCs with 40 μM of an ERK inhibitor (PD98059) and 20 μM of a JNK inhibitor (SP600125) for 2 h, the cells were infected with U. urealyticum at an MOI of 0.1 for 6 h (C). The concentrations of IL-6, CXCL1, and CCL2 in culture supernatants were measured by ELISA. Data are shown as the mean ± SD of triplicate samples from one experiment representative of three independent experiments (***P < 0.001). −/−, knockout; N.S, not significant.
U. urealyticum Enhances the Expression of ICAM-1 and VCAM-1
The expression of cell adhesion molecules such as ICAM-1 and VCAM-1 plays a vital role in the development of endometriosis, especially in the early stages of pathogenesis (36, 37). Accordingly, we investigated whether U. urealyticum considerably affects the expression of these markers in PMCs. Compared with the uninfected WT PMCs group, WT PMCs increased the expression of ICAM-1 and VCAM-1 by U. urealyticum infection at 12 and 24 h (P < 0.001, for each; Figures 4A,B). In infected Tlr2-deficient PMCs, those molecules were also upregulated at 24 h compared to levels in uninfected Tlr2-deficient PMCs group (P < 0.001, for each; Figures 4A,B), but these increases were diminished compared to those observed for infected WT PMCs group (P < 0.001, for each; Figures 4A,B). These results implied that U. urealyticum significantly upregulates ICAM-1 and VCAM-1 expression in PMCs, and that several mechanisms including the TLR2 pathway might control the expression of these adhesion molecules.
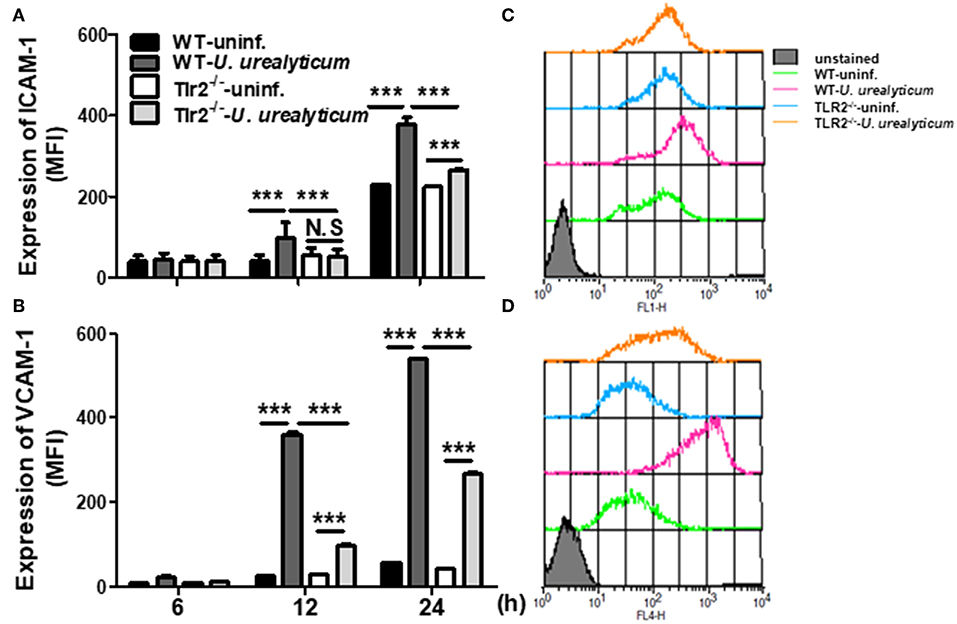
Figure 4. U. urealyticum infection increases the expression of adhesion molecules in PMCs. WT and Tlr2-deficient PMCs were infected with U. urealyticum (MOI of 0.1) for 6–24 h. The expression of ICAM-1 (A) and VCAM-1 (B) was analyzed by flow cytometry. Representative plots of ICAM-1 (C) and VCAM-1 (D) expression are shown. All experiments were performed in triplicate. Data are shown as the mean ± SD (n = 3). ***P < 0.001 compared to respective groups. N.S, not significant; −/−, knockout; uninf., uninfected; MFI, mean channel fluorescence intensity.
U. urealyticum Enhances the Expression of MMP-2 in PMCs
Matrix metalloproteinases (MMPs) play a crucial role in tissue remodeling associated with various pathological or physiological processes such as the infiltration and dissemination of malignant tumors or placental trophoblasts (38). We next determined whether U. urealyticum modulates MMP expression in PMCs. As shown in Figure 5, U. urealyticum infection significantly increased the expression levels of MMP-2 in WT PMCs at 12 (P < 0.01) and 24 h (P < 0.001) compared to those in Tlr2-deficient PMCs. However, expression of MMP-2 was not enhanced by U. urealyticum infection in Tlr2-deficient PMCs. Our results show that U. urealyticum enhances the expression of active MMP-2 in PMCs and that this is blocked in Tlr2-deficient PMCs.
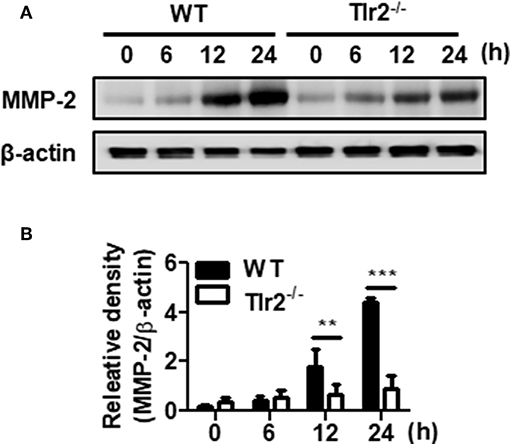
Figure 5. U. urealyticum enhances the expression of MMP-2 in PMCs. WT and Tlr2-deficient PMCs were infected with U. urealyticum (MOI of 0.1). Protein was extracted at the indicated time points. The expression of MMP-2 was examined by western blotting (A). The relative density of MMP-2 activation following U. urealyticum infection was compared to that of β-actin (B). Data are shown as the mean ± SD (n = 3). **P < 0.01; ***P < 0.001. −/−, knockout.
The Attachment of Endometrial Stromal Cells to Mesothelial Cells Is Facilitated by U. urealyticum Infection Based on a Co-culture System
We further investigated whether U. urealyticum modulates ESCs adhesion to PMCs using our co-culture system. For this, we infected mesothelial cells with U. urealyticum for 12 h to induce expression adhesion molecules and MMP and then co-incubated endometrial stromal cells with infected mesothelial cells.
In an experiment with human mesothelial cells (MeT-5A) and human endometrial stromal cells (T-HESC), TLR2 ligand, Pam3CSK4, increased the attachment of T-HESC to MeT-5A compared to that in the untreated group (P < 0.01; Figures 6A,B). We further performed an attachment experiment with ESCs and PMCs obtained from mice. ESC attachment was significantly increased upon co-culture with infected WT PMCs compared to that with uninfected WT PMCs (P < 0.05; Figure 6C). Moreover, this attachment was increased in infected WT cells compared to that with infected Tlr2-deficient PMCs (P < 0.01; Figures 6C,D). However, there was no significant difference for Tlr2-deficient PMCs with or without U. urealyticum infection (Figure 6C). Our results indicated that U. urealyticum facilitates the attachment of ESCs to PMCs via TLR2 signaling.
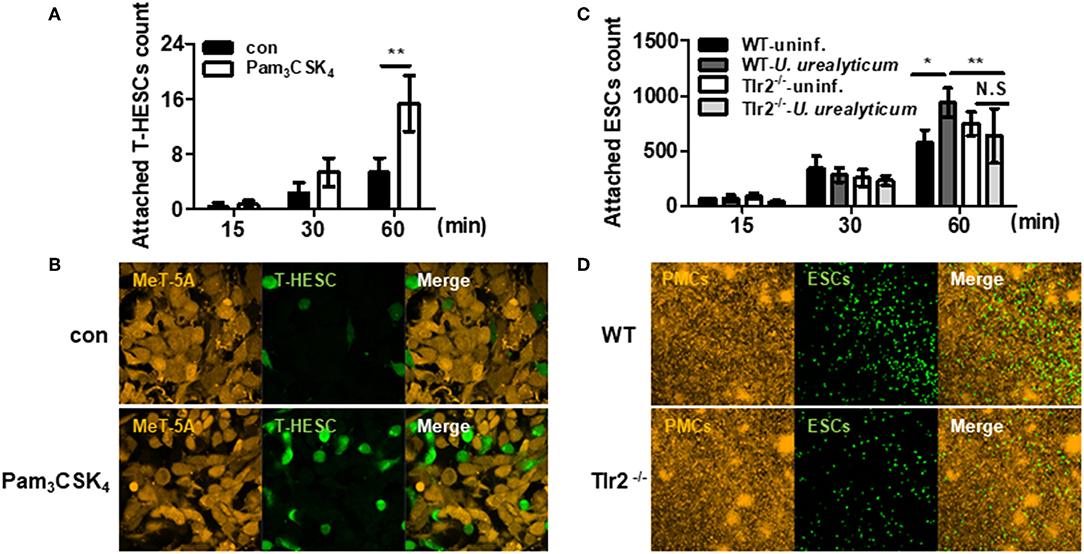
Figure 6. U. urealyticum promotes the attachment of endometrial stromal cells to mesothelial cells via TLR2. The MeT-5A and PMCs were stimulated with or without Pam3CSK4 and U. urealyticum. After a 12 h infection, T-HESC and ESCs were incubated for 15, 30, and 60 min (A–D). The attached T-HESC and ESCs were enumerated using ImageJ software (A,C). Images were obtained after 60 min with a 100x (B) and 10x (D) objective by confocal laser scanning microscopy. All experiments were performed in triplicate. Data are shown as the mean ± SD (n = 3). *P < 0.05; **P < 0.01. N.S, not significant; −/−, knockout. uninf., uninfected.
Discussion
In this study, we investigated whether pelvic infection can cause endometriosis and which mechanism is involved. Many previous reports regarding the pathogenesis of endometriosis have demonstrated abnormal features of the endometrium itself, hormone biosynthesis, immune cells, angiogenesis, neurogenesis, and cell death (4, 5, 39). However, the role of PMCs has been underestimated (40). Furthermore, recently, peritoneal inflammation triggered by bacteria arose as a new possible pathogenesis of endometriosis. Several studies have suggested a connection between bacterial infection in the female genital tract and endometriosis (11, 14, 41, 42). Therefore, new insights into the role of the peritoneal microenvironment with bacterial infections will provide a better understanding of endometriosis. U. urealyticum is one of the most common commensal microorganisms residing in the female reproductive tract and causes many obstetric and gynecological disorders (15–17). However, the importance of U. urealyticum infection in terms of endometriosis has been overlooked to date. Thus, we hypothesized that peritoneal inflammation and the breakdown of its integrity caused by U. urealyticum might contribute to the initiation and progression of pelvic endometriosis, probably through TLR2.
Based on our newly-developed in vivo mouse model, the number and size of ectopic lesions was significantly increased in U. urealyticum-infected mice compared to those in control mice (Figure 1). Our in vitro studies represented the PMCs response to U. urealyticum infection and the interaction between ESCs and PMCs at the initial stage of endometriosis development. Meanwhile, the in vivo animal study models a subsequent stage of endometriosis progression since ectopic lesions were observed 30 days post transplantation. Recently, Azuma et al. (12) reported that the repeated intraperitoneal injection of bacterial endotoxin (LPS) could induce chronic peritoneal inflammation and reproduce endometriosis in mice. However, that study induced endometriosis with endotoxin, but not with live bacteria. To our best knowledge, this is the first animal model of endometriosis triggered by intraperitoneal microbial infection.
Here, in assessing the interaction between U. urealyticum and PMCs, we showed that this bacterium could increase not only the production of IL-6, CXCL1, and CCL2, but also the expression of ICAM-1, VCAM-1, and MMP-2 from PMCs. There is a body of evidence that shows the relationship between PMC inflammation and development of endometriosis. In vitro experiments demonstrated endometrial fragment attached to only the site of damaged peritoneum with exposure of basement membrane or extracellular matrix (43). Moreover, inflammatory mediators (cytokines, chemokines, and growth factors) are aberrantly expressed in the peritoneal fluid of women with endometriosis (44–48). This local inflammatory microenvironment promotes the growth and maintenance of ectopic lesions through endometrial–peritoneal adhesion, invasion, angiogenesis, and proliferation via loss of tight junction, MMP, and epithelial-mesenchymal transition (40, 49).
The concentrations of IL-1β, IL-1 converting enzyme, IL-8, and regulated on activation, normal T-cell expressed and secreted (RANTES) were increased in the peritoneal fluid of patients with endometriosis (45, 47). Similarly, IL-1β, IL-6, TNF-α, and prostaglandin E2 (PGE2) were found to be rich in the peritoneal fluid of women with endometriosis (44, 46).
During the development of endometriosis, these cytokines and chemokines play a role. IL-6 is a pro-inflammatory cytokine that activates T and B cells and facilitates the recruitment of macrophages (5, 40). IL-8, a chemoattractant for macrophages and neutrophils, is vigorously secreted from the peritoneum of women with endometriosis upon stimulation by IL-1 and TNF-α (50). Since rodents do not have an IL-8 homolog, it is assumed that mice compensate for the absence of IL-8 with the ligands CXCL1 and CXCL2 (51). CXCL1 has neutrophil chemoattractant activity via CXCR2 binding (52), but has been scarcely studied with respect to endometriosis. CCL2 recruits macrophages and might be involved in the growth and maintenance of ectopic endometrium to secrete cytokines and growth factors and directly stimulate the proliferation of endometrial cells (53). Levels of CCL2 were previously found to be significantly correlated with the stage of endometriosis (54) and were higher in women with endometriosis than in women with tubal infertility (55). Similarly, in the peritoneal fluid of women with endometriosis, levels of CXCL1, CXCL2, CCL2, MCP-3, and HGF were found to be significantly elevated compared to those in control individuals (40, 53, 56).
However, most clinical studies have measured cytokines from peritoneal fluid, which could be influenced by various components such as PMCs, immune cells, and ectopic lesions (40). In this study, we observed the increased production and secretion of IL-6, CCL2, and CXCL1 from PMCs in response to U. urealyticum infection. These results are in line with suggestion from others that PMCs might actively participate in the generation of a pro-inflammatory environment in the peritoneum (40, 49). For the development of endometriosis, the adhesion and attachment of endometrial cells to the pelvic mesothelium is a critical step (57). Some adhesion molecules such as integrins α2β1 and α3β1, P-cadherin, ICAM-1, and VCAM-1 have been suggested to be key factors regulating the attachment of endometrial cells (40, 58, 59). Even though pro-inflammatory cytokines are known to upregulate these adhesion molecules (40), it is not clear whether those molecules increase when PMCs are infected by U. urealyticum. We observed the significant upregulation of ICAM-1 and VCAM-1 in PMCs following U. urealyticum infection. Their increased expression could provide suitable sites for the attachment of endometrial cells.
Next, we investigated MMP production from PMCs since the invasion of endometrial cells into the pelvic mesothelium is considered an essential factor for the development of endometriosis (40). Several studies have reported higher expression of MMP-2, MMP-9, MMP-14, and MMP-24 in the eutopic endometrium or ectopic lesions of women with endometriosis, as compared to levels in women without endometriosis (60–62). Peritoneal fluids and sera from endometriosis patients were also shown to contain higher levels of MMP-2 than those from controls (63). In our study, MMP-2 expression was also significantly increased in the PMCs by U. urealyticum infection. This peritoneal MMP expression might facilitate extracellular matrix remodeling during endometriosis development (40).
Through the co-culture experiments, we found that ESCs attached to PMCs and that U. urealyticum-infected PMCs showed significantly enhanced attachment to ESCs compared to uninfected PMCs. A similar co-culture examination without infection was reported by Nair et al. (64). They observed that ESCs attached to PMCs in 1 h and that ESC invasion occurred at 6 h. Through interactions between PMCs and ESCs in this co-culture system, several endometriosis-related genes including CD44, ERK, CSF-1, and c-Met were upregulated in both PMCs and ESCs. Another co-culture study with human ESCs and human PMCs showed the relaxation of PMC integrity at the beginning of ESC invasion and the loss of PMC adhesion to the substrate (65). These studies indicate that functional and morphological changes of PMCs potentiate the development of endometriosis, which might be triggered by U. urealyticum (15–17).
As the next step, we explored which mechanism is involved in the activation of PMCs following U. urealyticum infection. U. urealyticum-derived lipid-associated membrane proteins (LAMPs), which are lipoproteins, are considered a major factor for TLR2 activity (66). In this study, we found that U. urealyticum induces a series of inflammatory responses in PMCs mainly through TLR2. Moreover, Tlr2-deficient mice secreted lower levels of a pro-inflammatory cytokine (IL-6) and chemokines (CCL2 and CXCL1) and exhibited downregulated adhesion molecule (ICAM-1 and VCAM-1) and MMP-2 expression (Figures 2–5). Furthermore, ESC attachment to PMCs induced by U. urealyticum infection was significantly diminished in Tlr2-deficient PMCs (Figure 6). Recently, it was reported that U. urealyticum infection enhances TLR2-mediated IL-6 production from amniotic epithelial cells (15). Our results indicate that the host immune response against U. urealyticum infection is mainly mediated through TLR2.
We further assessed transcriptional factors activated in response to U. urealyticum. The phosphorylation of ERK and JNK was observed when U. urealyticum was applied to PMCs. However, these signaling events were significantly decreased in the PMCs of Tlr2-deficient mice. These results also suggest that TLR2 signaling is required for the activation of ERK and JNK MAP kinases in PMCs (Figures 1–3).
There are some limitations to our study. First, it was not performed with cells from patients with endometriosis. However, when we examined the attachment of T-HESC cells to MeT-5A cells using our co-culture system, a TLR2 ligand increased the attachment of these cells. This experiment with human cells strongly supports our results obtained from mice. Moreover, we did not investigate the presence of peritoneal U. urealyticum infection in women with pelvic endometriosis. If U. urealyticum could be isolated from the peritoneal washing or tissue, it could demonstrate a direct connection between these bacteria and disease. However, according to Kobayashi et al. (67), pelvic infection, initiating the adhesion and growth of ectopic endometrial tissue, is suppressed by the innate immunity of the host and following sterile inflammation, can continue and progress into endometriosis. Therefore, the identification of microorganisms in the pelvic cavity of women with developed endometriosis is less likely.
In summary, we observed that U. urealyticum infection increases inflammatory mediators, adhesion molecules, and MMP-2 expression in PMCs through TLR2 signaling and promotes endometriosis. We present a strong evidence that may support an infection theory in endometriosis development. To our best knowledge, this is the first study suggesting that U. urealyticum can provoke pelvic endometriosis in an animal model. Moreover, in this study, we developed a new animal model of endometriosis triggered by U. urealyticum infection. Through our results, we present a theory that infection-induced pelvic inflammation contributes to the initiation and progression of endometriosis. In conclusion, mesothelium inflammation induced by U. urealyticum might be one of the causes of pelvic endometriosis. Prevention of pelvic infection might decrease the prevalence of endometriosis. Further studies are warranted to elucidate this connection.
Data Availability Statement
All datasets generated for this study are included in the manuscript/Supplementary Files.
Ethics Statement
The animal study was reviewed and approved by the Institutional Animal Care and Use Committee (IACUC; approved protocol number: P-17-09-E-01) at Konyang University.
Author Contributions
EN, DK, and SL designed and conceived the study. EN performed data acquisition. EN, DK, JL, J-SK, JP, and SL performed data analysis and interpretation. EN, JH, and SL drafted the manuscript. All authors participated in the critical revision of the manuscript.
Funding
This work was supported by grants from the National Research Foundation of Korea (NRF) (2016RIDIA3B03935549), Priority Research Centers Program through the National Research Foundation of Korea (NRF) funded by the Ministry of Education, Science, and Technology (NRF-2017R1A6A1A03015713), and Konyang University Myunggok Research Fund (2014-04).
Conflict of Interest
The authors declare that the research was conducted in the absence of any commercial or financial relationships that could be construed as a potential conflict of interest.
Acknowledgments
We acknowledge K.M. Lee at Myunggok Research Institute for technical support.
Supplementary Material
The Supplementary Material for this article can be found online at: https://www.frontiersin.org/articles/10.3389/fimmu.2019.02373/full#supplementary-material
Abbreviations
ESC, endometrial stromal cell; MMP, matrix metalloproteinase; PMC, peritoneal mesothelial cell; WT, wild type.
References
1. Giudice LC, Kao LC. Endometriosis. Lancet. (2004) 364:1789–99. doi: 10.1016/S0140-6736(04)17403-5
2. Sampson JA. Metastatic or Embolic Endometriosis, due to the Menstrual Dissemination of Endometrial Tissue into the Venous Circulation. Am J Pathol. (1927) 3:93–110.43.
4. Vercellini P, Vigano P, Somigliana E, Fedele L. Endometriosis: pathogenesis and treatment. Nat Rev Endocrinol. (2014) 10:261–75. doi: 10.1038/nrendo.2013.255
5. May KE, Conduit-Hulbert SA, Villar J, Kirtley S, Kennedy SH, Becker CM. Peripheral biomarkers of endometriosis: a systematic review. Hum Reprod Update. (2010) 16:651–74. doi: 10.1093/humupd/dmq009
6. Khan KN, Kitajima M, Fujishita A, Nakashima M, Masuzaki H. Toll-like receptor system and endometriosis. J Obstet Gynaecol Res. (2013) 39:1281–92. doi: 10.1111/jog.12117
7. Panir K, Schjenken JE, Robertson SA, Hull ML. Non-coding RNAs in endometriosis: a narrative review. Hum Reprod Update. (2018) 24:497–515. doi: 10.1093/humupd/dmy014
8. Barcz E, Milewski L, Dziunycz P, Kaminski P, Ploski R, Malejczyk J. Peritoneal cytokines and adhesion formation in endometriosis: an inverse association with vascular endothelial growth factor concentration. Fertil Steril. (2012) 97:1380–6.e1. doi: 10.1016/j.fertnstert.2012.03.057
9. Li S, Fu X, Wu T, Yang L, Hu C, Wu R. Role of interleukin-6 and its receptor in endometriosis. Med Sci Monit. (2017) 23:3801–7. doi: 10.12659/MSM.905226
10. Osuga Y, Koga K, Hirota Y, Hirata T, Yoshino O, Taketani Y. Lymphocytes in endometriosis. Am J Reprod Immunol. (2011) 65:1–10. doi: 10.1111/j.1600-0897.2010.00887.x
11. Khan KN, Kitajima M, Hiraki K, Yamaguchi N, Katamine S, Matsuyama T, et al. Escherichia coli contamination of menstrual blood and effect of bacterial endotoxin on endometriosis. Fertil Steril. (2010) 94:2860–3.e1-3. doi: 10.1016/j.fertnstert.2010.04.053
12. Azuma Y, Taniguchi F, Nakamura K, Nagira K, Khine YM, Kiyama T, et al. Lipopolysaccharide promotes the development of murine endometriosis-like lesions via the nuclear factor-kappa B pathway. Am J Reprod Immunol. (2017) 77:e12631. doi: 10.1111/aji.12631
13. Iba Y, Harada T, Horie S, Deura I, Iwabe T, Terakawa N. Lipopolysaccharide-promoted proliferation of endometriotic stromal cells via induction of tumor necrosis factor alpha and interleukin-8 expression. Fertil Steril. (2004) 82(Suppl. 3):1036–42. doi: 10.1016/j.fertnstert.2004.04.038
14. Lin WC, Chang CY, Hsu YA, Chiang JH, Wan L. Increased risk of endometriosis in patients with lower genital tract infection: a nationwide cohort study. Medicine. (2016) 95:e2773. doi: 10.1097/MD.0000000000002773
15. Triantafilou M, De Glanville B, Aboklaish AF, Spiller OB, Kotecha S, Triantafilou K. Synergic activation of toll-like receptor (TLR) 2/6 and 9 in response to Ureaplasma parvum & urealyticum in human amniotic epithelial cells. PLoS ONE. (2013) 8:e61199. doi: 10.1371/journal.pone.0061199
16. Lee MY, Kim MH, Lee WI, Kang SY, Jeon YL. Prevalence and antibiotic susceptibility of Mycoplasma hominis and Ureaplasma urealyticum in pregnant women. Yonsei Med J. (2016) 57:1271–5. doi: 10.3349/ymj.2016.57.5.1271
17. Combaz-Sohnchen N, Kuhn A. A systematic review of mycoplasma and ureaplasma in urogynaecology. Geburtshilfe Frauenheilkd. (2017) 77:1299–303. doi: 10.1055/s-0043-119687
18. Deguchi T, Yoshida T, Miyazawa T, Yasuda M, Tamaki M, Ishiko H, et al. Association of Ureaplasma urealyticum (biovar 2) with nongonococcal urethritis. Sex Transm Dis. (2004) 31:192–5. doi: 10.1097/01.OLQ.0000114653.26951.71
19. Waites KB, Katz B, Schelonka RL. Mycoplasmas and ureaplasmas as neonatal pathogens. Clin Microbiol Rev. (2005) 18:757–89. doi: 10.1128/CMR.18.4.757-789.2005
20. Yoshida T, Deguchi T, Meda S, Kubota Y, Tamaki M, Yokoi S, et al. Quantitative detection of Ureaplasma parvum (biovar 1) and Ureaplasma urealyticum (biovar 2) in urine specimens from men with and without urethritis by real-time polymerase chain reaction. Sex Transm Dis. (2007) 34:416–9. doi: 10.1097/01.olq.0000243621.89212.40
21. Kasprzykowska U, Elias J, Elias M, Maczynska B, Sobieszczanska BM. Colonization of the lower urogenital tract with Ureaplasma parvum can cause asymptomatic infection of the upper reproductive system in women: a preliminary study. Arch Gynecol Obstet. (2014) 289:1129–34. doi: 10.1007/s00404-013-3140-1
22. Mutsaers SE, Wilkosz S. Structure and function of mesothelial cells. Cancer Treat Res. (2007) 134:1–19. doi: 10.1007/978-0-387-48993-3_1
23. Colmont CS, Raby AC, Dioszeghy V, Lebouder E, Foster TL, Jones SA, et al. Human peritoneal mesothelial cells respond to bacterial ligands through a specific subset of Toll-like receptors. Nephrol Dial Transplant. (2011) 26:4079–90. doi: 10.1093/ndt/gfr217
24. Romero R, Espinoza J, Goncalves LF, Kusanovic JP, Friel LA, Nien JK. Inflammation in preterm and term labour and delivery. Semin Fetal Neonatal Med. (2006) 11:317–26. doi: 10.1016/j.siny.2006.05.001
25. Moco NP, Martin LF, Pereira AC, Polettini J, Peracoli JC, Coelho KI, et al. Gene expression and protein localization of TLR-1,−2,−4 and−6 in amniochorion membranes of pregnancies complicated by histologic chorioamnionitis. Eur J Obstet Gynecol Reprod Biol. (2013) 171:12–7. doi: 10.1016/j.ejogrb.2013.07.036
26. Kato S, Yuzawa Y, Tsuboi N, Maruyama S, Morita Y, Matsuguchi T, et al. Endotoxin-induced chemokine expression in murine peritoneal mesothelial cells: the role of toll-like receptor 4. J Am Soc Nephrol. (2004) 15:1289–99.
27. Park JH, Kim YG, Shaw M, Kanneganti TD, Fujimoto Y, Fukase K, et al. Nod1/RICK and TLR signaling regulate chemokine and antimicrobial innate immune responses in mesothelial cells. J Immunol. (2007) 179:514–21. doi: 10.4049/jimmunol.179.1.514
28. Kim TH, Lee KB, Kang MJ, Park JH. Critical role of Toll-like receptor 2 in Bacteroides fragilis-mediated immune responses in murine peritoneal mesothelial cells. Microbiol Immunol. (2012) 56:782–8. doi: 10.1111/j.1348-0421.2012.00505.x
29. Xiao J, Gong Y, Chen Y, Yu D, Wang X, Zhang X, et al. IL-6 promotes epithelial-to-mesenchymal transition of human peritoneal mesothelial cells possibly through the JAK2/STAT3 signaling pathway. Am J Physiol Renal Physiol. (2017) 313:F310–F8. doi: 10.1152/ajprenal.00428.2016
30. D'Inzeo T, De Angelis G, Fiori B, Menchinelli G, Liotti FM, Morandotti GA, et al. Comparison of mycoplasma IES, mycofast revolution and mycoplasma IST2 to detect genital mycoplasmas in clinical samples. J Infect Dev Ctries. (2017) 11:98–101. doi: 10.3855/jidc.8039
31. Hirata T, Osuga Y, Yoshino O, Hirota Y, Harada M, Takemura Y, et al. Development of an experimental model of endometriosis using mice that ubiquitously express green fluorescent protein. Hum Reprod. (2005) 20:2092–6. doi: 10.1093/humrep/dei012
32. Bilotas M, Meresman G, Stella I, Sueldo C, Baranao RI. Effect of aromatase inhibitors on ectopic endometrial growth and peritoneal environment in a mouse model of endometriosis. Fertil Steril. (2010) 93:2513–8. doi: 10.1016/j.fertnstert.2009.08.058
33. Noh EJ, Kang MJ, Jeong YJ, Lee JY, Park JH, Choi HJ, et al. Withaferin A inhibits inflammatory responses induced by Fusobacterium nucleatum and Aggregatibacter actinomycetemcomitans in macrophages. Mol Med Rep. (2016) 14:983–8. doi: 10.3892/mmr.2016.5326
34. De Clercq K, Hennes A, Vriens J. Isolation of mouse endometrial epithelial and stromal cells for in vitro decidualization. J Vis Exp. (2017) 121:e55168. doi: 10.3791/55168
35. Kawasaki T, Kawai T. Toll-like receptor signaling pathways. Front Immunol. (2014) 5:461. doi: 10.3389/fimmu.2014.00461
36. Witz CA. Cell adhesion molecules and endometriosis. Semin Reprod Med. (2003) 21:173–82. doi: 10.1055/s-2003-41324
37. Oh YK, Ha YR, Yi KW, Park HT, Shin JH, Kim T, et al. Increased expression of resistin in ectopic endometrial tissue of women with endometriosis. Am J Reprod Immunol. (2017) 78:e12726. doi: 10.1111/aji.12726
38. Kessenbrock K, Plaks V, Werb Z. Matrix metalloproteinases: regulators of the tumor microenvironment. Cell. (2010) 141:52–67. doi: 10.1016/j.cell.2010.03.015
39. Yilmaz BD, Bulun SE. Endometriosis and nuclear receptors. Hum Reprod Update. (2019) 25:473–85. doi: 10.1093/humupd/dmz005
40. Young VJ, Brown JK, Saunders PT, Horne AW. The role of the peritoneum in the pathogenesis of endometriosis. Hum Reprod Update. (2013) 19:558–69. doi: 10.1093/humupd/dmt024
41. Kodati VL, Govindan S, Movva S, Ponnala S, Hasan Q. Role of shigella infection in endometriosis: a novel hypothesis. Med Hypotheses. (2008) 70:239–43. doi: 10.1016/j.mehy.2007.06.012
42. Khan KN, Fujishita A, Hiraki K, Kitajima M, Nakashima M, Fushiki S, et al. Bacterial contamination hypothesis: a new concept in endometriosis. Reprod Med Biol. (2018) 17:125–33. doi: 10.1002/rmb2.12083
43. Burney RO, Giudice LC. Pathogenesis and pathophysiology of endometriosis. Fertil Steril. (2012) 98:511–9. doi: 10.1016/j.fertnstert.2012.06.029
44. Harada T, Yoshioka H, Yoshida S, Iwabe T, Onohara Y, Tanikawa M, et al. Increased interleukin-6 levels in peritoneal fluid of infertile patients with active endometriosis. Am J Obstet Gynecol. (1997) 176:593–7. doi: 10.1016/S0002-9378(97)70553-2
45. Bersinger NA, von Roten S, Wunder DM, Raio L, Dreher E, Mueller MD. PAPP-A and osteoprotegerin, together with interleukin-8 and RANTES, are elevated in the peritoneal fluid of women with endometriosis. Am J Obstet Gynecol. (2006) 195:103–8. doi: 10.1016/j.ajog.2005.12.010
46. Giudice LC. Clinical practice. Endometriosis. N Engl J Med. (2010) 362:2389–98. doi: 10.1056/NEJMcp1000274
47. Sikora J, Mielczarek-Palacz A, Kondera-Anasz Z. Imbalance in cytokines from interleukin-1 family - role in pathogenesis of endometriosis. Am J Reprod Immunol. (2012) 68:138–45. doi: 10.1111/j.1600-0897.2012.01147.x
48. Young VJ, Ahmad SF, Duncan WC, Horne AW. The role of TGF-β in the pathophysiology of peritoneal endometriosis. Hum Reprod Update. (2017) 23:548–59. doi: 10.1093/humupd/dmx016
49. Wu M-H, Hsiao K-Y, Tsai S-J. Endometriosis and possible inflammation markers. Gynecol Minim Invasive Ther. (2015) 4:61–7. doi: 10.1016/j.gmit.2015.05.001
50. Vinatier D, Dufour P, Oosterlynck D. Immunological aspects of endometriosis. Hum Reprod Update. (1996) 2:371–84. doi: 10.1093/humupd/2.5.371
51. Lee J, Cacalano G, Camerato T, Toy K, Moore MW, Wood WI. Chemokine binding and activities mediated by the mouse IL-8 receptor. J Immunol. (1995) 155:2158–64.
52. Sawant KV, Poluri KM, Dutta AK, Sepuru KM, Troshkina A, Garofalo RP, et al. Chemokine CXCL1 mediated neutrophil recruitment: role of glycosaminoglycan interactions. Sci Rep. (2016) 6:33123. doi: 10.1038/srep33123
53. Arici A, Oral E, Attar E, Tazuke SI, Olive DL. Monocyte chemotactic protein-1 concentration in peritoneal fluid of women with endometriosis and its modulation of expression in mesothelial cells. Fertil Steril. (1997) 67:1065–72. doi: 10.1016/S0015-0282(97)81440-9
54. Zeyneloglu HB, Senturk LM, Seli E, Oral E, Olive DL, Arici A. The role of monocyte chemotactic protein-1 in intraperitoneal adhesion formation. Hum Reprod. (1998) 13:1194–9. doi: 10.1093/humrep/13.5.1194
55. Tao Y, Zhang Q, Huang W, Zhu H, Zhang D, Luo W. The peritoneal leptin, MCP-1 and TNF-α in the pathogenesis of endometriosis-associated infertility. Am J Reprod Immunol. (2011) 65:403–6. doi: 10.1111/j.1600-0897.2010.00920.x
56. Chen Y, Wang K, Xu Y, Guo P, Hong B, Cao Y, et al. Alteration of myeloid-derived suppressor cells, chronic inflammatory cytokines, and exosomal miRNA contribute to the peritoneal immune disorder of patients with endometriosis. Reprod Sci. (2019) 26:1130–8. doi: 10.1177/1933719118808923
57. Baranov V, Malysheva O, Yarmolinskaya M. Pathogenomics of endometriosis development. Int J Mol Sci. (2018) 19:E1852. doi: 10.3390/ijms19071852
58. Chen GT, Tai CT, Yeh LS, Yang TC, Tsai HD. Identification of the cadherin subtypes present in the human peritoneum and endometrioticlesions: potential role for P-cadherin in the development of endometriosis. Mol Reprod Dev. (2002) 62:289–94. doi: 10.1002/mrd.10121
59. Schutt AK, Atkins KA, Slack-Davis JK, Stovall DW. VCAM-1 on peritoneum and α4β1 integrin in endometrium and their implications in endometriosis. Int J Gynecol Pathol. (2015) 34:85–9. doi: 10.1097/PGP.0000000000000118
60. Chung HW, Lee JY, Moon HS, Hur SE, Park MH, Wen Y, et al. Matrix metalloproteinase-2, membranous type 1 matrix metalloproteinase, and tissue inhibitor of metalloproteinase-2 expression in ectopic and eutopic endometrium. Fertil Steril. (2002) 78:787–95. doi: 10.1016/S0015-0282(02)03322-8
61. Osteen KG, Yeaman GR, Bruner-Tran KL. Matrix metalloproteinases and endometriosis. Semin Reprod Med. (2003) 21:155–64. doi: 10.1055/s-2003-41322
62. Pitsos M, Kanakas N. The role of matrix metalloproteinases in the pathogenesis of endometriosis. Reprod Sci. (2009) 16:717–26. doi: 10.1177/1933719109333661
63. Huang HF, Hong LH, Tan Y, Sheng JZ. Matrix metalloproteinase 2 is associated with changes in steroid hormones in the sera and peritoneal fluid of patients with endometriosis. Fertil Steril. (2004) 81:1235–9. doi: 10.1016/j.fertnstert.2003.10.027
64. Nair AS, Nair HB, Lucidi RS, Kirchner AJ, Schenken RS, Tekmal RR, et al. Modeling the early endometriotic lesion: mesothelium-endometrial cell co-culture increases endometrial invasion and alters mesothelial and endometrial gene transcription. Fertil Steril. (2008) 90(4 Suppl.):1487–95. doi: 10.1016/j.fertnstert.2007.09.047
65. Chen Z, Dai Y, Dong Z, Li M, Mu X, Zhang R, et al. Co-cultured endometrial stromal cells and peritoneal mesothelial cells for an in vitro model of endometriosis. Integr Biol. (2012) 4:1090–5. doi: 10.1039/c2ib00172a
66. Ye GY, Wang KY, Gui QD, Wang M. Ureaplasma urealyticum-derived lipid-associated membrane proteins introduce IL-6, IL-8, and TNF-α cytokines into human amniotic epithelial cells via Toll-like receptor 2. J Zhejiang Univ Sci B. (2018) 19:654–61. doi: 10.1631/jzus.B1800005
Keywords: Ureaplasma urealyticum, endometriosis, mesothelial cells, infection, inflammation, Toll-like receptor 2
Citation: Noh EJ, Kim DJ, Lee JY, Park JH, Kim J-S, Han JW, Kim BC, Kim CJ and Lee SK (2019) Ureaplasma Urealyticum Infection Contributes to the Development of Pelvic Endometriosis Through Toll-Like Receptor 2. Front. Immunol. 10:2373. doi: 10.3389/fimmu.2019.02373
Received: 19 June 2019; Accepted: 23 September 2019;
Published: 04 October 2019.
Edited by:
Daniela Bosisio, University of Brescia, ItalyReviewed by:
Analía Gabriela Ricci, National Council for Scientific and Technical Research (CONICET), ArgentinaCapobianco Annalisa, San Raffaele Hospital (IRCCS), Italy
Copyright © 2019 Noh, Kim, Lee, Park, Kim, Han, Kim, Kim and Lee. This is an open-access article distributed under the terms of the Creative Commons Attribution License (CC BY). The use, distribution or reproduction in other forums is permitted, provided the original author(s) and the copyright owner(s) are credited and that the original publication in this journal is cited, in accordance with accepted academic practice. No use, distribution or reproduction is permitted which does not comply with these terms.
*Correspondence: Chul Jung Kim, a2NqMDMwN0BreXVoLmFjLmty; Sung Ki Lee, c2tsZWVAa3l1aC5hYy5rcg==